- 1Department of Molecular Biology and Genetics, Faculty of Science, Sivas Cumhuriyet University, Sivas, Türkiye
- 2Advanced Technology Research and Application Center (CUTAM), Sivas Cumhuriyet University, Sivas, Türkiye
- 3Department of Obstetrics and Gynecology, Haseki Training and Research Hospital Affiliated with the University of Health Sciences, Istanbul, Türkiye
- 4Department of Obstetrics and Gynecology, Faculty of Medicine, Sivas Cumhuriyet University, Sivas, Türkiye
- 5Department of Biology, Faculty of Science, Sivas Cumhuriyet University, Sivas, Türkiye
Introduction: Sedentary lifestyles and diets with high glycemic indexes are considered to be contributing factors to the development of obesity, type 2 diabetes in humans. Metformin, a biguanide medication commonly used to treat type 2 diabetes, has been observed to be associated with longevity; however, the molecular mechanisms underlying this observation are still unknown.
Methods: The effects of metformin and high glucose, which have important roles in aging-related disease such as diabetes and cancer, were studied in lin-35 worms because they are associated with cancer-associated pRb function in mammals and have a tumour suppressor property.
Results and Discussion: According to our results, the negative effect of high glucose on egg production of lin-35 worms was greater than that of N2 worms. High glucose shortened lifespan and increased body length and width in individuals of both strains. Metformin treatment alone extended the lifespan of N2 and lin-35 worms by reducing fertilization efficiency. However, when metformin was administered in the presence of high glucose, the lifespan of lin-35 worms was clearly longer compared to N2 worms. Additionally, we conclude that glucose and metformin in lin35 worms can extend life expectancy through a DAF-16/FOXO-independent mechanism. Furthermore, the results of this study will provide a new perspective on extending mammalian lifespan through the model organism C. elegans.
1 Introduction
The impact of longevity-promoting agents, or geroprotectors, on the development of spontaneous tumours may offer crucial insights into the interplay between aging and carcinogenesis (1). Based on current understanding of aging factors, mechanisms, and theories, these pharmacological interventions have occasionally been associated with unfavourable side effects (2, 3). By contrasting the information about the mechanisms of geroprotector action with its effect on tumours, we can better comprehend the interplay between aging and carcinogenesis, two essential biological processes.
Metformin, a biguanide derived from the French lilac plant, Galega officinalis, is the most commonly prescribed anti-diabetic medication. It is effective in lowering blood sugar levels with minimal side effects and has received significant attention as a compound with the potential to promote healthy aging (4, 5). Metformin has been shown to have positive effects in the treatment of cardiovascular disease, certain types of cancer, and to enhance cognition in older adults in addition to its anti-diabetic properties (4, 6, 7). The substantial interest in advantages of metformin is heightened by strong evidence that it promotes healthy aging and lengthens life in a range of model organisms (4, 6, 8). Furthermore, compared to matched healthy controls, metformin has been linked to longer survival rates in patients with type 2 diabetes (6, 7).
Although the anti-hyperglycaemic properties of metformin have been well-documented, the complex mechanisms underlying its action remain incompletely understood. Metformin exerts a notable influence on the metabolic machinery, specifically targeting the cellular energy sensor AMPK, mTOR, and the mitochondrial complex I (9). Different molecular processes may be activated by different doses of metformin. The intricate relationship between metformin and physiology is further complicated by its interactions with the microbiome, which can have significant negative effects on health in humans, Drosophila melanogaster, and the nematode C. elegans (6, 9). The picture that shows up is that metformin interacts with physiology on multiple levels, which could cause a population to respond differently on an individual basis (9).
From C. elegans to mammals, the insulin signalling pathway has been highly conserved throughout evolution (10, 11). Mammalian tyrosine kinase receptors bind to insulin and its close homolog IGF-1, blocking the transcription factor FOXO, which is an essential transcriptional regulator of various cellular processes, including metabolism, longevity, stress response, and apoptosis (10–12). Reducing the activity of this pathway through mutation of the C. elegans DAF-2 insulin/IGF-1 receptor gene doubles lifespan and slows the aging process (13). Lifespan of numerous organisms have been demonstrated to be regulated by the insulin/IGF-1 signalling (IIS) pathway (10, 11, 14).
Our current understanding of the impact of high glucose and metformin on the C. elegans insulin/IGF-1 signalling pathway and lifespan is extremely limited, despite the well-established link between the insulin/IGF-1 signalling pathway and aging in this organism.
By inducing and preserving cellular quiescence, the DREAM (Dp/Retinoblastoma (Rb)-like/E2F/MuvB) transcriptional repressor complex, which is conserved from invertebrates to mammals, acts as the gatekeeper of the mammalian cell cycle (15). The highly conserved DREAM transcriptional repressor complex, which includes Dp, Retinoblastoma (Rb)-like, E2F, and MuvB, mediates this cell cycle quiescence pathway (16). Lin-35, an orthologue of human retinoblastoma protein (pRB), a component of the worm DREAM complex, is a pocket protein found in C. elegans, and one of the important mechanisms it plays a role in is apoptosis (17). The finding that lin-35 suppresses the expression of lin-3, a member of the EGF family, raises the possibility that paracrine growth factor signalling suppression is a mechanism by which members of the Rb family slow down cell growth and thereby prevent cancer (18). Thus, it has been proposed that lin-35 function analysis may offer important insights into new Rb family tumours suppression mechanisms (19). Finally, research in non-mammalian systems like C. elegans has revealed several additional cell cycle and developmental roles for the E2F-pRb network, some of which have been demonstrated to be connected to pRb functions that have been proposed to be related to cancer in mammals (15). Lin-35 is linked to cancer-related pRb function and has a tumours suppressor effect. Therefore, lin-35 worms were included in this study to investigate the effects of metformin and high glucose, which have important roles in aging-related diseases such as diabetes and cancer.
In current study, we investigated the effects of high glucose, metformin and the combination of the both on in vivo fertilisation, lifespan and body size in N2 and lin-35 worms. We also evaluated differential mRNA expression of insulin/IGF signalling (IIS) components associated with key nutrient sensing pathways of the best-validated antiaging interventions. Additionally, the results of this study investigating the combined effects of glucose and metformin on the mutant strain lin-35, which is associated with the tumours suppressor pRb function in mammals, will provide a new perspective on extending mammalian lifespan through the model organism C. elegans.
2 Materials and methods
2.1 C. elegans and Escherichia coli strains
Wild-type (N2 Bristol) and mutant AWR58-lin-35 (kea7[lin-35p::degron::GFP::lin-35]) I; keaSi10 II C. elegans strains and OP50 strain of E. coli as food source of C. elegans were purchased from the Caenorhabditis Genetics Centre (CGC), University of Minnesota (Minneapolis–St. Paul, MN 55455, U.S.A).
2.2 E. coli OP50 growth conditions
E. coli OP50 strain is used as a food source for C. elegans and is grown monoxenically. E. coli OP50 is a uracil auxotroph whose growth is limited to NGM. A limited bacterial culture is preferred because it allows easier observation and better mating of worms (20). Starting culture is used to isolate single colonies on a streaked plate of a rich medium such as Luria-Bertani (LB) agar [10 g Bacto-tryptone, 5 g Bacto-yeast, 5 g NaCL, 15 g agar, prepared with H2O for 1 liter, pH 7.5]. Aseptically inoculate a single colony from the plate into a rich solution of LB Broth [10 g Bacto-tryptone, 5 g Bacto-yeast, 5 g NaCl, 1 liter with H2O, pH adjusted to 7 using 0.1 M NaOH]. It can be stored for several months at room temperature. Incubate the cultures overnight at 37°C in a shaking incubator. After incubation, check for growth, which is characterised by a hazy haze in the medium. The E. coli OP50 solution is then ready to be used for seeding NGM petri plates. The E. coli OP50 petri plate and the liquid culture should be stored at 4°C and can remain usable for several months (21).
2.3 C. elegans culture
Worms were maintained according to Brenner’s instructions previously described (20). Obtaining axenised C. elegans eggs and synchronous cultures of C. elegans were performed according to the method previously described (22). In our study, the lin-35 strain purchased from CGC was treated with auxin before drug application, as it was stated that it needed to be auxinised, especially for the degradation of DREAM proteins. After synchronisation of lin-35 worms, worms were placed in 1×M9 buffer with 1mM auxin and E. coli was added. Worms were placed in the moist and hot box prepared beforehand and left for 30 minutes (23). After incubation, drug applications of auxinised lin-35 worms were carried out.
2.4 Drugs and reagents
The metformin was purchased from Biosynth, Turkey (1115-70-4) dissolved in ddH2O as concentrated 1.2 M stock solution (stored at −20 °C). Auxin was purchased from Bio Basic, Turkey (Q7202130) dissolved in 1X M9 buffer as concentrated 1 M stock solution stored at +4 °C.
2.5 Fertilisation assay
For the assay, the age-matched adult worm (three worm per well for each strains) were transferred to 24-well plates containing 1 mL/well S Medium [1L S Basal, 10 mL 1 M potassium citrate pH: 6, 10 mL trace metals solution (1.86 g disodium EDTA, 0.69 g FeSO4 •7 H2O, 0.2 g MnCl2•4 H2O, 0.29 g ZnSO4•7H2O, 0.025 g CuSO4•5 H2O, dissolved in 1L H2O), 3 mL 1 M CaCl2, 3 mL 1 M MgSO4] with 3µL of E. coli OP50 and increasing concentrations of metformin (1mM, 10mM, 25mM, 50mM and 100mM) in presence of 25mM glucose and cultured at 20°C. The number of eggs laid in each well for 10 days was counted under microscope. The experiments were repeated twice, and each experiment was performed in quadruplicate (24).
2.6 Lifespan assays
The L4 stage worms (~12 worms per well for each strains) were transferred to 24-well plates containing 1 mL/well S Medium with 3µL of E. coli OP50, 25mM glucose and increasing concentrations of metformin (1mM, 10mM, 25mM, 50mM and 100mM), and worms were cultured at 20°C for lifespan assay. FUDR (5-Fluoro-2’-deoxyuridine) was used to prevent reproduction. The medium was changed every 7 days and worms counted under the microscope every other day. Animals that did not move after being gently nudged to move the plate were deemed dead. For body size analyses of worms, 0-, 7-, and 14-day photomicrographs were obtained using the Zeiss Axiovert A1 with Zeiss Digital Microscope Camera (AxioCam ICc 5). Body length was determined using ImageJ software. Data were analysed using GraphPad Prism. The experiments were repeated twice, and each experiment was performed in duplicate (22).
2.7 RNA isolation and cDNA synthesis
Following synchronisation, about 3000 L4 stage worms from two distinct generations of N2 and lin-35 were placed in 24-well plates with 1 mL/well S Medium and 3µL of E. coli OP50. The worms were then cultivated for seven days at 20°C. Following a 7-day incubation period with either glucose or metformin, worm samples were prepared for RNA isolation using the previously described procedure (25). Total RNA was obtained using a commercially available kit, following the manufacturer’s instructions (HibriGen Biotechnology, Istanbul, Turkey, Total RNA Isolation kit; Cat. No: MG-RNA-01-100). The iScript cDNA Synthesis Kit (Bio-Rad, Hercules, CA, USA; Cat. No: 1708891) was used to synthesise cDNA with 500 ng/µL of RNA, following the manufacturer’s guidelines. The generated cDNA was either analysed immediately by qPCR or stored at +4°C for future use.
2.8 Quantitative real-time PCR
Expression in N2 and lin-35 at L4 stage worm of components of the IIS system (daf-16a, daf-16b, daf-16d/f, total daf-16 and daf2) mRNA was evaluated by RT-qPCR. Following the manufacturer’s instructions for real-time amplifications, quantitative PCR was carried out using the StepOnePlus™ Real Time PCR equipment (Applied Biosystems, USA) and the SYBR green assay. In a total reaction volume of 20 µL, the experiment was carried out using 10 µL of BlasTaqTM 2X qPCR Master Mix (Abm, Canada, USA), 0.5 µL of each primer, and 2 µL of cDNA template. Primer details are given in Table 1. Every reaction was conducted using 96-well plates. The real-time instrument was used to conduct PCR in accordance with the previously outlined procedure (22). In the study our research group conducted, the most stable genes for N2 and lin-35 strains were found to be act-1 and cdc-42 reference genes (29). Therefore, the previously reported approach was employed to normalise mRNA levels using act-1 and cdc-42 (30). The comparative threshold approach, 2-ΔCt, was utilised to compute the relative expression of genes. Primers were purchased from BMLabosis (Ankara, Turkey). Ct values greater than 35 produced inconsistent findings and were considered below the assay’s detection limit.
2.9 Statistical analysis
All experiments were repeated at least twice. The outcomes of the repeated experiments were comparable. The statistical software GraphPad Prisim 6.0 (GraphPad software, San Diego, CA) was utilised for the analysis. For survival, log-rank tests were used. A one-way ANOVA test was used to compare statistical results between groups. When significant differences were discovered, the Newman-Keuls test was used to compare groups. p<0.05 was considered statistically significant in all analyses. The data is shown as mean SEM.
3 Results
3.1 Effects of glucose and metformin on C. elegans fertilisation
According to the results of the effect of glucose on the egg-laying capacity of worms, it was observed that 25mM glucose significantly reduced the number of eggs laid by both N2 and lin-35 worms compared to the control (Figures 1A, B). According to the results of the effects of metformin on egg laying of worms, a significant inhibitory effect of metformin was observed at the four highest concentrations (10, 25, 50 and 100 mM) in N2 worms treated only with metformin (Figure 1C), while it had a significant inhibitory effect at the all concentrations (1, 10, 25, 50 and 100 mM) in lin-35 worms (Figure 1D). In both strains, the strongest inhibitory effect was observed in the presence of 100mM metformin. On the other hand, the egg-laying capacity of N2 worms treated with both 25mM glucose and different concentrations of metformin decreased significantly in the last four concentrations (10, 25, 50 and 100 mM) (Figure 1E). The inhibitory effect at these four concentrations was statistically stronger compared to treatment with metformin alone in N2 worms. On the other hand, when metformin was applied at increasing concentrations in the presence of 25mM glucose in lin-35 worms, the inhibitory effect remained weaker than the condition in which only metformin was applied. The inhibitory effect of metformin with high glucose on egg laying of lin-35 worms was significant at concentrations of 25, 50, and 100 mM (Figure 1F).
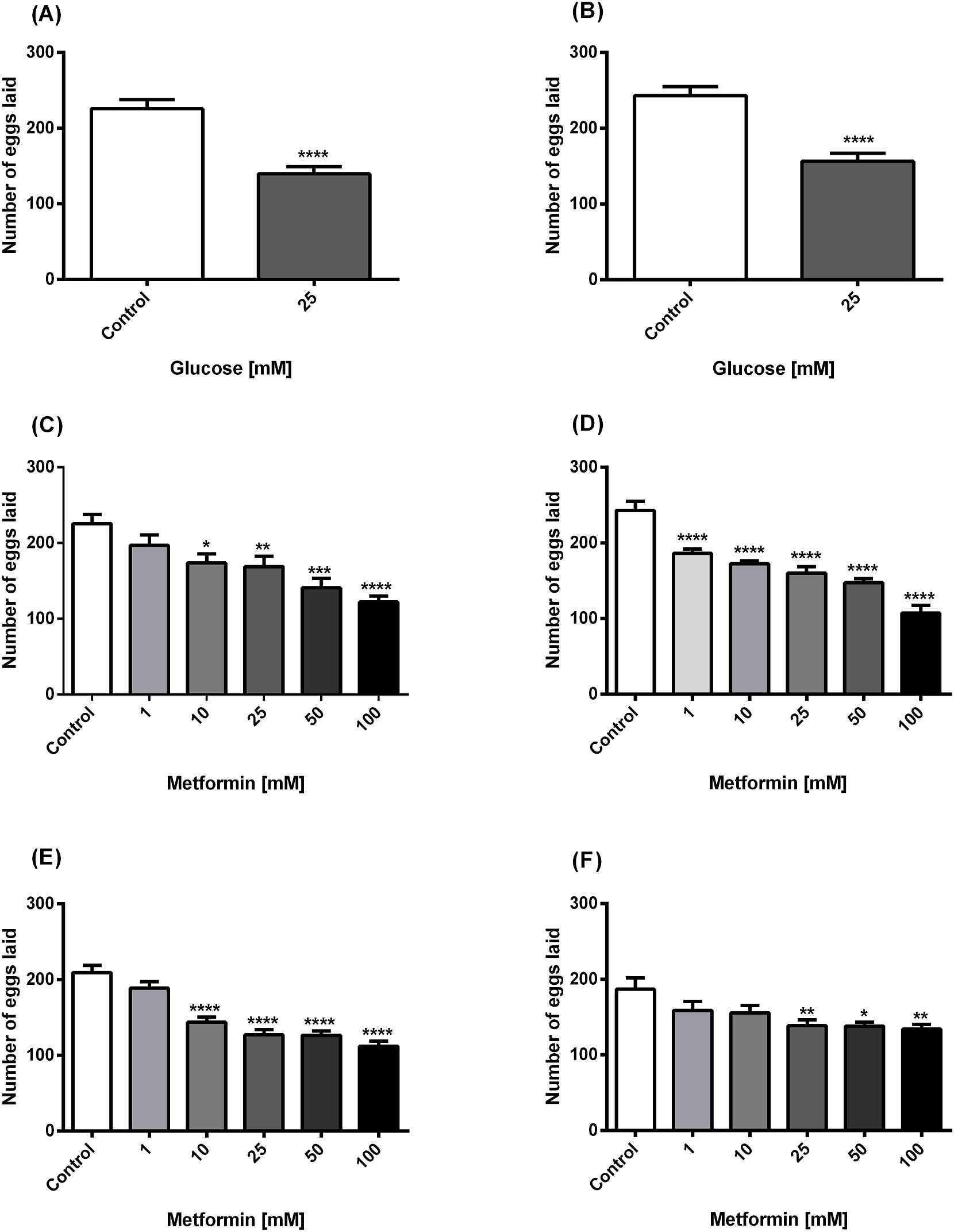
Figure 1. Panels (A, B) The effect of high glucose on egg-laying in N2 (A) and lin-35 (B) worms. Panels (C, D) Effect of metformin on egg-laying in N2 (C) and lin-35 (D) worms in the absence of glucose. Panels (E, F) Effect of metformin on egg-laying in N2 (E) and lin-35 (F) worms in the presence of 25mM glucose. The experiments were carried out on synchronised populations. Worms were assessed to be exposed to only glucose or to metformin in the presence/absence of glucose. During the egg stage, synchronised populations of wild-type and mutant C. elegans were transplanted to 24-well plates containing liquid medium with glucose (25mM) or increasing concentrations of metformin (1-100 mM) with or without glucose. Significance relates to a comparison of egg-laying behaviour in C. elegans exposed to glucose or metformin with or without glucose versus control. Control groups in panels (A, B) contain no glucose, panels (C, D) contain no metformin, while controls in panels (E, F) contain only 25 mM glucose. The number of eggs laid by each worm was counted every day. Values represent the mean ± SEM of two independent experiments in quadruplicate. *p < 0.05, **p<0.001, ***p<0.001, ****p<0.0001; n=3.
3.2 Screening of glucose and metformin for lifespan effects of C. elegans
We confirmed the effects of high glucose and metformin in the presence or absence of high glucose on worm lifespan. Our findings showed that the lifespan of N2 and Lin-35 worms decreased under high glucose condition, but it was extended when treated with metformin alone or metformin combined with high glucose (Figures 2, 3). 25mM glucose reduced the average lifespan of N2 and lin-35 worms by 13.04% and 9.41%, respectively (Figuress 2A, B, 3A). Metformin at concentrations of 1, 10, 25, 50, and 100 mM increased the average lifespan of N2 worms by 38.04%, 46.75%, 34.78%, 21.74%, and 21.74%, respectively (Figures 2C, 3B, white bars), while lin-35 worms increased by 48.24%, 57.65%, 51.76%, 51.76%, and 41.18%, respectively (Figures 2D, 3B, black bars). In the presence of 25mM glucose, 1, 10, 25, 50, and 10 mM metformin increased the lifespan of N2 worms by 4.65%, 6.98%, 9.30%, 3.88%, and 0.78%, respectively (Figures 2E, 3C, white bars), the lifespan of lin-35 worms was increased by 38.61%, 44.55%, 32.67%, 26.73%, and 26.73%, respectively (Figures 2F, 3C, black bars). When glucose alone was applied, the lifespan of N2 worms was reduced at a higher rate than that of lin-35 worms. On the other hand, when metformin alone was administered at all concentrations, the lifespan of lin-35 worms increased more than that of lin-35 worms. When both 25 mM glucose and different concentrations of metformin were administered together, the lifespan of lin-35 worms increased more than that of N2 worms at all concentrations. As expected, the extension of the lifespan of the worms was observed to be independent of concentration. That is, as the inhibitor concentration increased, a parallel increase in life span was not observed (Figures 2, 3).
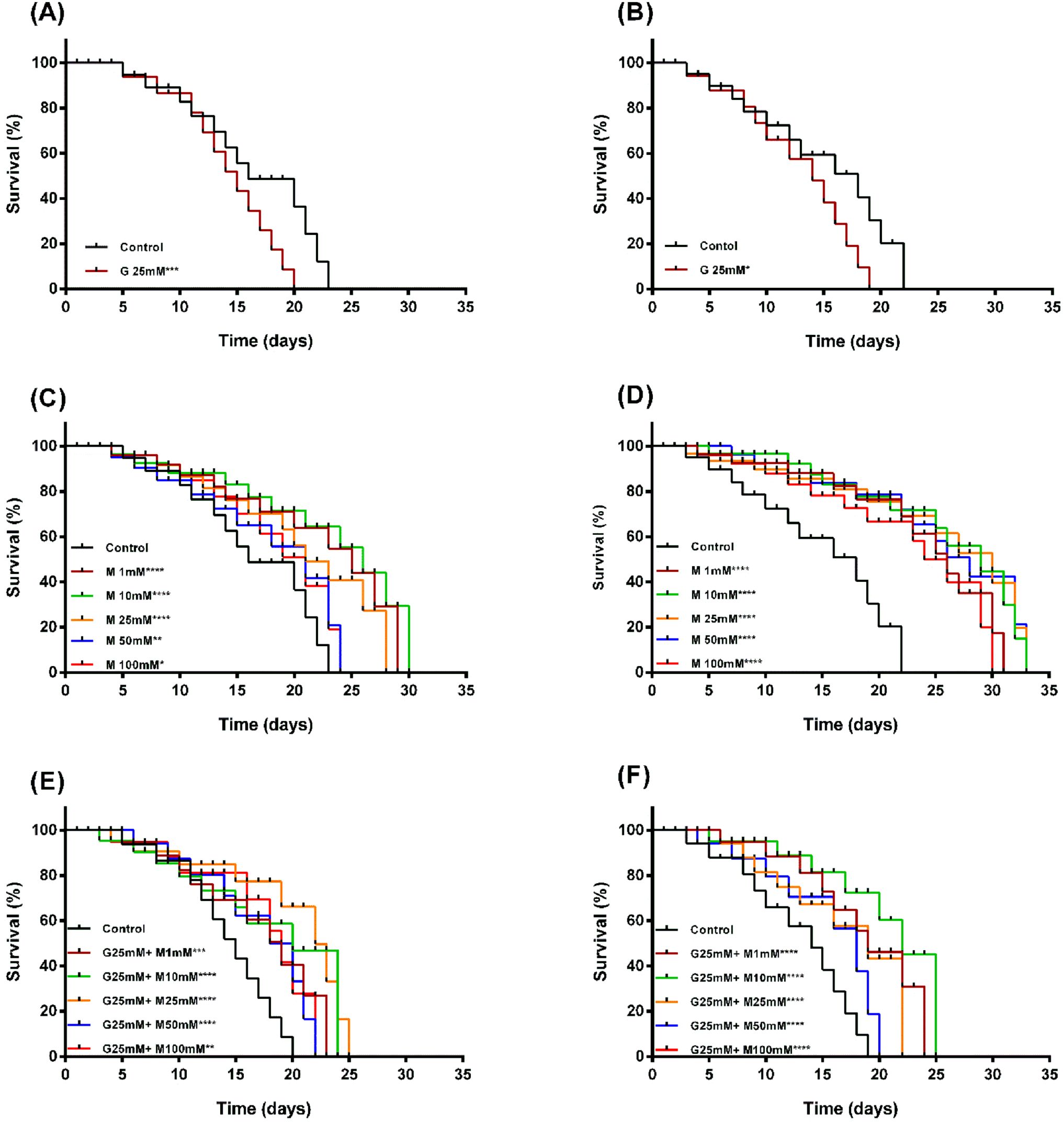
Figure 2. Panels (A, B) Lifespan survival curves of N2 (A) and lin-35 (B) worms in the presence of glucose. Panels (C, D) Lifespan survival curves of N2 (C) and lin-35 (D) worms in the presence of metformin without glucose. Panels (E, F) Lifespan survival curves of N2 (E) and lin-35 (F) worms in the presence of metformin and 25mM glucose. The experiments were carried out on synchronised populations. The synchronised populations of wild-type and mutant C. elegans were transplanted to 24-well plates containing liquid medium with glucose (25mM) or increasing concentrations of metformin (1-100mM) with or without glucose. Significance relates to a comparison of lifespan in C. elegans exposed to glucose or to metformin with or without glucose versus control. Control groups in panels (A, B) contain no glucose, panels (C, D) contain no metformin, while controls in panels (E, F) contain only 25 mM glucose. Labels state the name and concentration of the drug (G, Glucose; M, Metformin). Values represent the mean ± SEM of two independent experiments in quadruplicate. *p < 0.05, **p<0.001, ***p<0.001, ****p<0.0001.
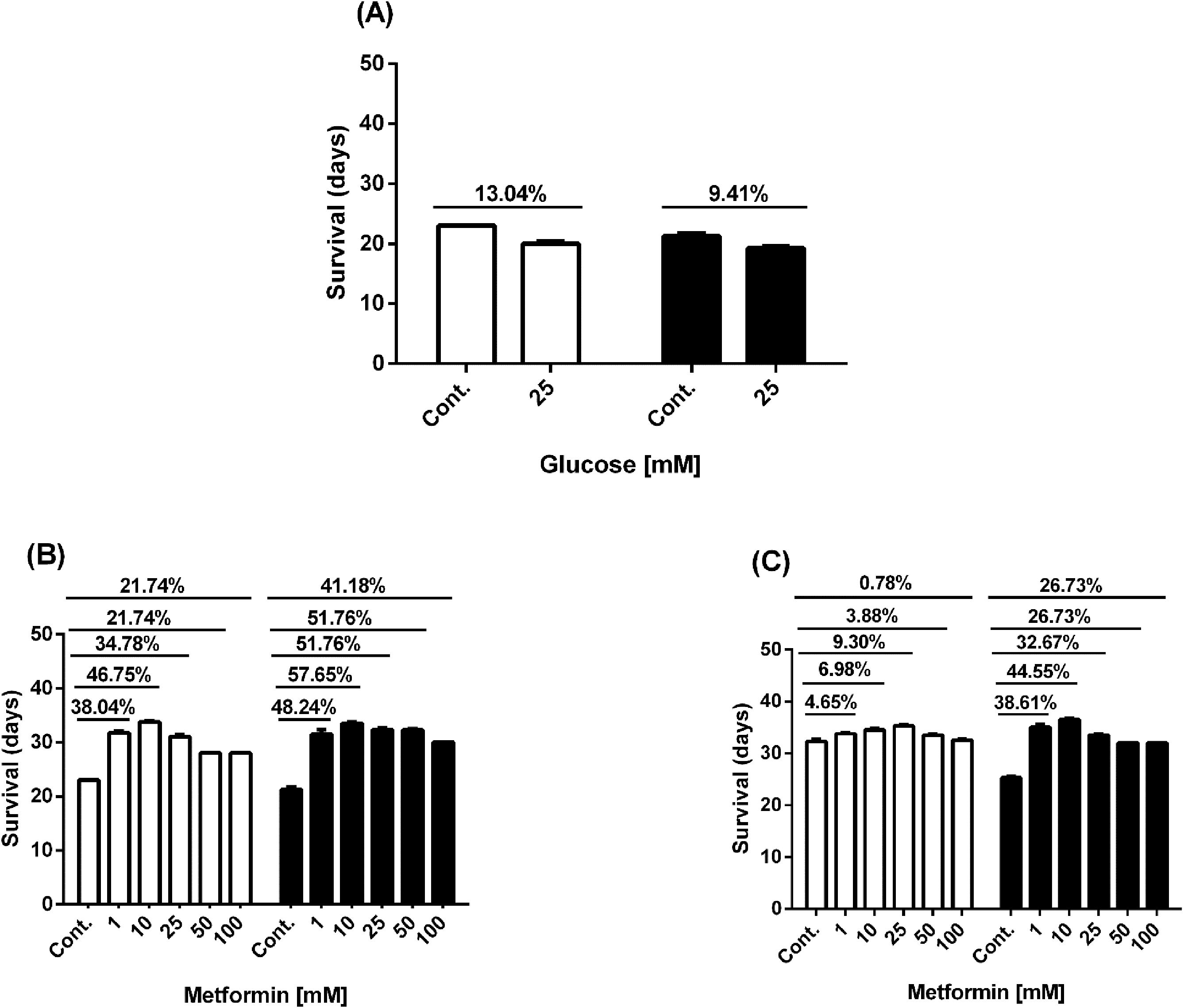
Figure 3. Graph showing the % increase in lifespan of N2 (white bars) and lin-35 (black bars) worms in the presence of glucose (A), metformin without glucose (B) and metformin with 25mM glucose (C) compared to control.
3.3 Body size measurement
To investigate the effect of metformin and high glucose on the body size of N2 and lin-35 worms, we measured their body length and width after 14 days (Figure 4). A significant increase in both body length and body width was observed in N2 and lin-35 worms exposed to 25 mM glucose (Figures 4A, B). The body length of N2 worms exposed to metformin alone significantly decreased at 10, 25, 50 and 100 mM metformin concentrations (Figures 4C, D, black bars), whereas the length of lin-35 worms significantly decreased in the presence of 25, 50, and 100 mM metformin (Figure 4C, grey bars). The body width of N2 worms treated with only metformin decreased significantly in the presence of 1, 10, 25, 50 and 100 mM metformin (Figure 4D, grey bars), while in lin-35 worms at 10, 25, 50 and 100 mM metformin. On the other hand, when metformin was administered in the presence of 25 mM glucose, the body size (length and width) of both N2 and lin-35 worms decreased significantly at all metformin concentrations (except body width of lin35 at 1mM metformin) (Figures 4E, F).
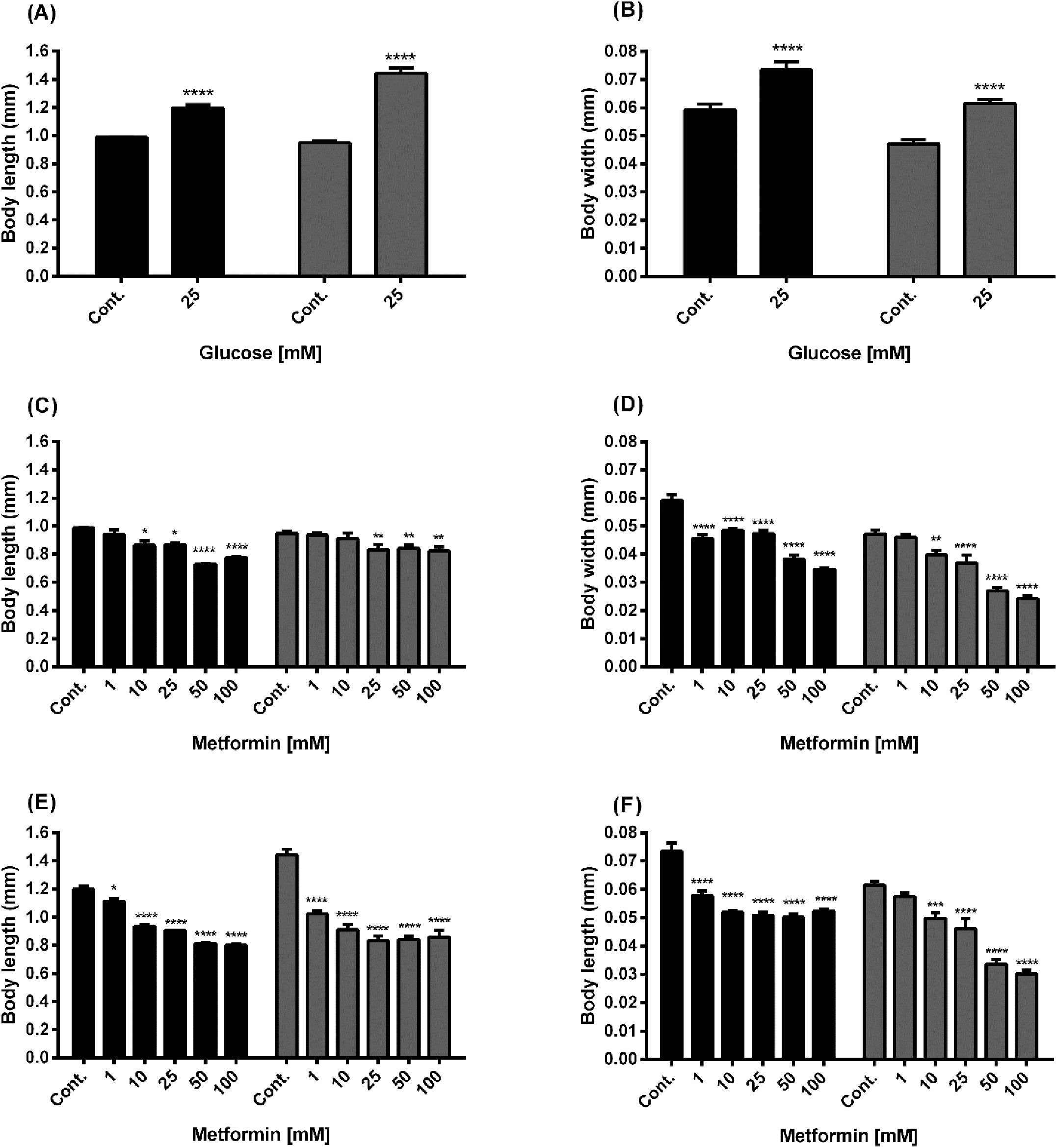
Figure 4. Body size of wild-type N2 (black bars) and mutant lin-35 strains (grey bars) grown in S-medium including only glucose or different concentrations of metformin in presence or absence of glucose. Panels (A, B) Body length (A) and width (B) of N2 and lin-35 worms grown in S-medium including high glucose compared with control. Panels (C, D) Body length (C) and width (D) of N2 and lin-35 worms grown in S-medium including metformin without glucose. Panels (E, F) Body length (E) and width (F) of N2 and lin-35 worms grown in S-medium including metformin and 25mM glucose. Body length and width size derived N2 and lin-35 worms after 14 days is in mm using ImageJ software. Values represent the mean ± SEM of two independent experiments in duplicate. *p < 0.05, **p<0.001, ***p<0.001, ****p<0.0001.
3.4 mRNA expression of components of the IIS system in C. elegans
In N2 (Figure 5, black bars) and lin-35 (Figure 5, grey bars) cultured in S-medium containing 25mM glucose (Figures 5A–E) or 100 mM of metformin, mRNA expression of the daf-16a, daf-16b, daf-16d/f, total daf-16 and daf-2 were measured by quantitative RT-PCR.
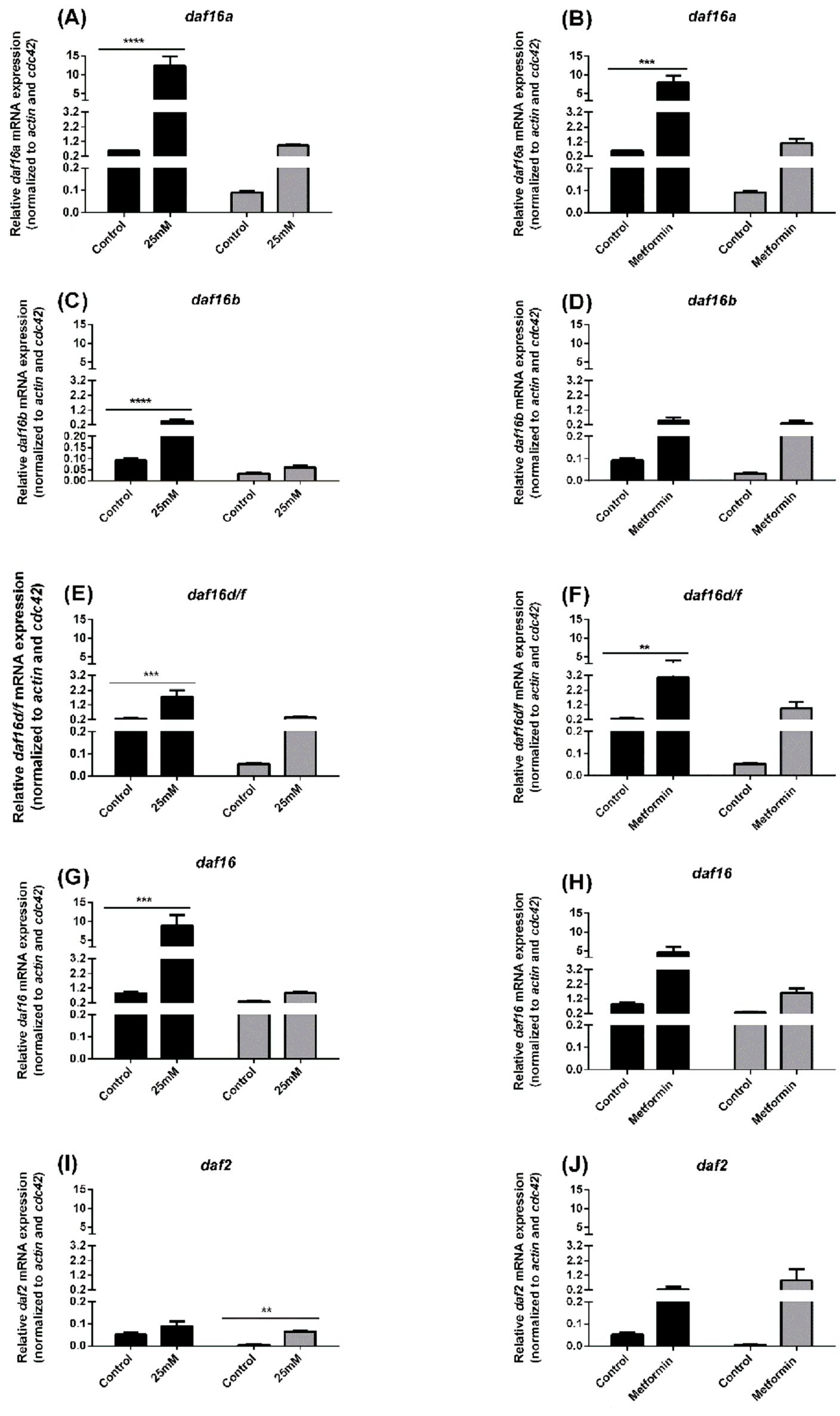
Figure 5. daf-16a, daf-16b, daf-16d/f, total daf-16 and daf2 mRNA expression in N2 (black bars) and lin-35 (grey bars) worms in presence of 25mM glucose (A, C, E, G, I) or presence of 100 mM metformin (B, D, F, H, J). daf-16a, daf-16b, daf-16d/f, total daf-16 and daf2 mRNA expression was measured relative to the levels of the house-keeping genes cdc-42 and act-1. Values represent the mean ± SEM of two measurements from two independent experiments. **p<0.01, ***p<0.001, ****p<0.0001.
Compared with control, daf-16a, daf-16b, daf-16d/f, total daf-16 mRNA expression was significantly increased in N2 worms cultured with 25 mM glucose, whereas there was no significant increase in lin-35 worms (Figures 5A–D). On the other hand, while there was no significant difference in daf-2 mRNA expression in N2 worms compared to the control, an increase was observed in lin-35 worms (Figure 5E). While daf-16a, daf-16d/f and daf-2 mRNA expression was significantly increased in N2 worms cultured with 100 mM metformin compared to control, a significant increase in mRNA expression of all IIS components (daf-16a, daf-16b, daf-16d/f, total daf-16 and daf2) was observed in lin-35 worms (Figures 5F–J).
4 Discussion
Metformin is a lipophilic biguanide that promotes peripheral glucose utilisation while suppressing hepatic gluconeogenesis. Due to its safety, effectiveness and tolerability, it is a first-line medication for Type 2 diabetic patients who need to control their blood sugar levels (31). A potential anti-tumorigenic effect of metformin is exerted by activating AMP-kinase, which mediates inhibition of the mammalian target of rapamycin (mTOR) (32). Results from several clinical studies have shown that type 2 diabetes patients treated with metformin may have a lower risk of cancer (31). Therefore, in this study, we tried to elucidate the relationship between metformin, diabetes, and cancer on the model organism C. elegans. The best validated antiaging studies have been demonstrated in the insulin/IGF signalling (IIS) and rapamycin target (TOR) pathways, two major nutrient sensing pathways (33). The insulin/insulin-like growth factor (IGF) signalling pathway to mTOR is essential for the survival and growth of normal cells and also contributes to the formation and progression of cancer (34). The lin-35 strain present in the current study is the orthologue of pRb in mammals and has a tumours suppressor effect by inhibiting mTOR signalling (18). In this study, the effect of high glucose and metformin in the presence and absence of high glucose on the lifespan of lin-35 worms with Rb mutation was investigated and whether this effect was through the insulin signalling pathway. This effect was also investigated in wild-type N2 worms to reveal the difference in effect with lin-35 worms. Thus, this model created in the C. elegans model organism will provide a new perspective to elucidate the relationship between tumours, diabetes, and aging. To our knowledge, there have been no previous studies on how the combination of metformin and high glucose affects fertilisation and survival for C. elegans. Therefore, the results of this study investigating the combined effects of glucose and metformin on the mutant strain lin-35, which is associated with the tumours suppressor pRb function in mammals, will provide a new perspective on mammalian life extension through the model organism C elegans. For this purpose, we examined the effects of high glucose, metformin, and combination of both on fertilisation. Self-reproducing hermaphrodites of C. elegans store sperm in spematheca and produce oocytes. Hermaphrodites store fertilised eggs in their uteruses during the first day of the L4/adult molt, so a young adult hermaphrodite will have 10-15 eggs stored in their uterus at any given time (Schafer, 2005). We found that the number of days to fertility of N2 and lin-35 worms treated with high glucose was shortened (1 day) compared to the control group. On the other hand, we found that when only metformin was administered, it prolonged the fertilisation period of N2 and lin-35 worms, resulting in egg-laying for approximately 3 and 2 more days, respectively. In N2 and lin-35 worms treated with metformin and high glucose, this period was extended by 2 and 1 days, respectively. In both conditions (metformin alone or metformin in the presence of high glucose), the number of days to egg-laying was longer in N2 worms than in lin-35 worms. In addition, when only metformin was applied, the decrease in the number of eggs of lin-35 worms was stronger, whereas when high glucose and metformin were applied, a higher inhibitory effect was observed in N2 worms. Furthermore, under conditions where high glucose, metformin, or both are administered, the relative number of progeny produced per day in N2 and lin-35 worms is reduced. Therefore, we hypothesised that high glucose, metformin, and both affect the reproduction of worms and that this is probably due to defects in egg-laying capacity. Our results on the effect of only high glucose treatment on fertility in N2 worms are consistent with those reported by Teshiba et al., Lu and Qiu, Alcántar-Fernández et al. (35–37) and our results regarding metformin treatment were consistent with the results of Onken and Driscoll, Cabreiro et al. (38, 39). On the other hand, in this study, the combined effect of high glucose and metformin on the fertilisation of N2 worms was revealed for the first time. Additionally, our results regarding the effects of high glucose, metformin, and both on fertility of lin-35 worms are reported for the first time with this study. In these studies investigating the effect of glucose on C. elegans fertilisation (35–37), it was generally studied on NGM agar at very high concentrations (100 mM), which create toxic effects for the organisms. This is due to the high bioavailability of administered glucose by the worms. Since the high effectiveness of bioavailability in drug applications on worms cultured in NGM agar has always been controversial, it has been recommended to apply drugs at high concentrations for high efficiency of bioavailability (40). However, high drug applications at concentrations well above these normal values, cell culture and patient studies fail to show parallelism. Therefore, in our current study, worms were exposed to glucose and metformin in liquid media in order not to create any doubt about bioavailability, since glucose and metformin spread more homogeneously in liquid media and the bioavailability of worms is high in liquid media. It has also been reported that metformin is unstable at high temperatures (41). The drug must be added before the NGM agar cools and gels, so this appears to be a disadvantage in applications of temperature-sensitive drugs such as metformin. In such cases, drug application in C. elegans liquid culture prevents such disadvantages.
In previously reported studies, C. elegans lifespan was also shortened in the presence of high glucose (42, 43). In these two studies, the effects of adding 2% glucose (equivalent to approximately 11M glucose) to NGM agar on the lifespan of worms were investigated and they found that the lifespan of worms treated with 2% glucose was significantly shortened. In the study conducted by Schlotterer and colleagues, 40mM glucose was applied to create an environment similar to hyperglycaemic conditions in diabetic patients, since a glucose treatment of 40 mmol/L in agar resulted in a glucose concentration of 14 mmol/L in the whole body extract of C. elegans and they observed that the lifespan of worms decreased (44). According to our longevity analysis results, both wild-type N2 and mutant lin-35 worms shortened their lifespan in the presence of 25 mM glucose. We also found that the lifespan of N2 worms was further extended after 25 mM glucose treatment compared to lin-35 worms (Figure 1). As a result, consistent with the results of previous studies investigating the effect of glucose on longevity (even though very high glucose concentrations were studied), in our current study, high glucose reduced both the egg-laying efficiency and lifespan of N2 and lin-35 worms.
Experiments are still needed to clarify the molecular processes via which metformin confers health advantages, despite the considerable potential of metformin to support healthy aging (45–47). In order to close this knowledge gap, we first investigated the possibility that metformin could have physiological effects in C. elegans that are comparable to those seen in mammals. A review of additional studies carried out with the same objective was conducted. In one of these studies, conducted by Onken and Driscoll, the mean survival of wild type animals grown with 50 mM metformin has been reported to increase by nearly 40%, while wild type cultures grown with 1 mM and 10 mM metformin showed a survival rate comparable to controls grown without metformin (38). In addition, it was demonstrated by Cabreiro and associates that metformin at 25, 50, and 100 mM prolonged the average lifespan of N2 worms by 18%, 36%, and 3%, respectively. Additionally, they discovered that the ability metformin to prolong lifespan was dependent on living Escherichia coli, and that without these microorganisms, metformin reduced lifespan of C. elegans (39). Another study conducted by Onken and colleagues showed that exposure to 50 and 70 mM metformin extended the lifespan of N2 worms by 35% and 41%, respectively (9). In current study, we monitored survival rates of C. elegans occurring at increasing concentrations of metformin. Based on the results of the effectiveness of metformin alone on longevity, metformin in the presence of all concentrations extended the lifespan of both wild-type N2 and mutant lin-35 worms. We also found that the lifespan of lin-35 worms was further extended after metformin treatment compared to N2 worms (Figures 2, 3). As a result, only metformin treatment extended the lifespan of N2 and lin-35 worms by reducing fertilisation efficiency. Additionally, lifespan of N2 worms increased more at lower concentrations (1 and 10mM), whereas lifespan of lin-35 worms were observed at higher metformin concentrations (10, 25 and 50mM) than N2 worms. We hypothesised that metformin administration at higher concentrations may have had a toxic effect on N2 worms. However, when metformin was administered in the presence of high glucose, the lifespan of lin-35 worms was clearly longer compared to N2 worms. Under conditions where high glucose and metformin were administered together, the decrease in the rate of increase in lifespan in N2 worms administered only metformin was sharper than in lin-35 worms. This seems to be consistent with the data that metformin use in also patients with diabetes or aging-related diseases slows down aging and extends lifespan in C. elegans (48), we aimed to determine whether growth on high-glucose diets causes a change in body length and width. Alcántar-Fernández and colleagues observed that worms reared at 20, 40, 80, or 100 mM glucose were longer and thicker than control worms (37). Consistent with these results, in our current study, there was a significant increase in the length and width of both N2 and lin-35 worms when worms were treated with high glucose.
In accordance with studies that analysed the body size of worms exposed to metformin and showed that metformin caused a decrease in body size (49), in our study, metformin caused significant decreases in the body size of N2 and lin-35 worms. In present study, only after metformin treatment, significant decreases were observed in both body length and width of wild-type N2 and lin-35 worms, especially those exposed to 50 and 100 mM metformin concentrations, while when metformin was applied in the presence of high glucose, it was possible to observe a decrease in the body size of the worms at all metformin concentrations. In addition, when 50 and 100 mM metformin was administered alone and in combination with glucose, the greatest decrease in body width was observed, especially in lin-35 worms.
Furthermore, a study with mice showed that long-term treatment with metformin (0.1% w/w in diet) extends health span and lifespan in mice, while a higher dose (1% w/w) was toxic. Additionally, metformin has been reported to cause weight reduction in mice (50). In another study conducted by Anisimov et al., after metformin was administered to male and female mice, a slight increase in mean and maximum lifespan was observed in females, while a decrease in mean and maximum lifespan was observed in male (51). Another study reported that body weight decreased significantly in the group of mice with metformin in their diet compared to the control group (52). Similar to the results of previous studies in C. elegans and mice, in our current study, high glucose increased the body size of worms and shortened their lifespan; metformin treatment extended the lifespan of the worms and reduced their body size and fertility.
In C. elegans, daf-16 activation inhibits both aging and tumour growth. These two processes are essentially linked since tumour rates grow as the animal ages (1). It has been discovered that Daf-16/FOXO-regulated genes that affect the insulin/IGF-1 pathway as influencing the formation of C. elegans cancer formation (53). Although some of these genes are required for the full effect of daf-2 mutations on cell death, none appears to be required for the full effect on cell division. Since both increased apoptosis and decreased cell proliferation contribute to the tumour-protective effects of daf-2 mutations, downstream genes therein act cumulatively, as do the downstream targets of daf-16 is likely to act in a cumulative manner to influence tumour growth (54). Almost half of the genes that affect tumour growth have also been shown to affect the survival of animals without tumours. This suggests that the ability of the insulin/IGF-1 pathway to couple longevity and tumour resistance lies downstream of DAF-16 (55).
Downregulation of the insulin signalling system enhances C. elegans longevity and locomotor healthspan in a FOXO/DAF-16-dependent manner (13, 56–58). Insulin/IGF-1 signalling suppresses the transcriptional activity of DAF-16/FOXO (12). This inhibition happens as follows: Increased daf-2 signalling leads to the phosphorylation of age-1, pdk-1, and akt-1/2, which keeps the daf-16 protein in the cytosol and deactivates DAF-16/FOXOs. In the absence of daf-2, age-1, and akt-1/2, as in food shortage, daf-16 migrates to the nucleus and stimulates the expression of genes relevant to lifespan (59). When insulin/IGF-1 signalling is decreased, lifespan doubles, and this needs daf-16 (57). Deleting daf-16 in otherwise healthy animals accelerates tissue aging and reduces lifespan by approximately 20% (57, 60). We hypothesised that glucose could reduce the lifespan of C. elegans by affecting elements of the insulin/IGF-1 signalling system, since glucose enhances insulin secretion in mammals. According to our mRNA expression analysis results, in the presence of 25 mM glucose, daf-16a, daf-16b, daf-16d/f, and total daf-16 mRNA expression increased significantly in N2 worms compared to the control group, while no statistically significant change in daf-2 mRNA expression was observed. In lin-35 worms, while there was no significant change in daf-16a, daf-16b, daf-16d/f and total daf-16 mRNA expression, a statistically significant increase was observed in daf-2 mRNA expression. These results support the concept that glucose treatment for N2 worms upregulates the insulin/IGF-1 signalling pathway (42), which counteracts the lower activity of the insulin/IGF-1 receptor in these animals, but do not appear to be consistent for lin-35 worms.
Furthermore, to determine if metformin increases life expectancy via the insulin-signalling pathway, we measured the mRNA expression of Insulin/IGF-1 signalling pathway components that are required for the insulin-signalling pathway’s longevity effects. Onken and Driscol examined the effect of metformin on lifespan in daf-16 mutants. They found that daf-16 mutants grown on 50 mM metformin exhibited lifespan extension approximately equal to wild-type data and suggested that metformin may extend lifespan by a DAF-16/FOXO-independent mechanism (38). In our current study, in the presence of 100 mM metformin, daf-16a, daf-16d/f mRNA expression was significantly increased in N2 worms compared to the control group, while no significant change in daf-2 mRNA expression was observed. On the other hand, a statistically significant increase in mRNA expression of all Insulin/IGF-1 signalling components was observed in lin-35 worms compared to the control. Longevity in C. elegans is associated with increased expression of daf16 and decreased expression of daf-2. We thus conclude that metformin may enhance lifespan via a DAF-16/FOXO-independent mechanism, which is consistent with the notion that metformin confers health-span advantages via a mechanism other than the insulin-like signalling pathway.
5 Conclusion
The present observations show that the negative effect of high glucose on the egg production of lin-35 worms was greater than that of N2 worms. At the same time, high glucose shortened the egg-laying time of individuals of both strains. In addition, high glucose shortened the lifespan and increased body length and width of individuals of both strains. Metformin treatment alone extended the lifespan of N2 and lin-35 worms by reducing fertilisation efficiency. The lifespan of N2 worms was further increased at lower concentrations (1 and 10 mM), while the lifespan of lin-35 worms was observed at higher metformin concentrations (10, 25 and 50 mM) compared to N2 worms. Moreover, when metformin was administered alone, the lifespan of lin-35 worms was extended to a greater extent than that of N2 worms. However, when metformin was administered in the presence of high glucose, the lifespan of lin-35 worms was clearly longer compared to N2 worms. In conditions where high glucose and metformin were administered together, the rate of increase in lifespan was greater in lin-35 worms compared to N2 worms in conditions where metformin alone was administered. Therefore, in the presence of high glucose and metformin, the lifespan of cancer mutant lin-35 worms was longer than N2 worms. This seems consistent with the data that metformin use slows down aging and extends life in patients with diabetes and/or aging-related diseases.
We conclude that in lin35 worms, glucose and metformin can extend life expectancy through a DAF-16/FOXO-independent mechanism. However, expression of IIS signalling pathway components reflects only mRNA levels and not actual protein levels. More research is needed on this topic, as mRNA levels do not necessarily reflect protein levels. It would also be useful to examine the protein expression levels of IIS components that play an active role in longevity and fertility processes. In addition, although it is known that the IIS signalling pathways are closely related to lifespan, the current study has shown for the first time how the combination of high glucose and metformin affects the lifespan of worms related with cancer. Moreover, the current study will be key in the literature to investigate the mechanistic pathway of high glucose and/or metformin treatment. Therefore, it will encourage more mechanism-oriented studies to confirm especially the qPCR data in our current study. Therefore, in the future, elucidating these mechanisms through molecular and biochemical experiments, especially in lin-35 worms with the cancer-related gene, will be useful in elucidating the C. elegans diabetes-cancer model.
Data availability statement
The raw data supporting the conclusions of this article will be made available by the authors, without undue reservation.
Author contributions
ŞB: Conceptualization, Data curation, Formal Analysis, Funding acquisition, Investigation, Methodology, Project administration, Resources, Software, Supervision, Validation, Visualization, Writing – original draft, Writing – review & editing. AC: Conceptualization, Writing – review & editing. ÖÖ: Conceptualization, Data curation, Investigation, Methodology, Visualization, Writing – original draft, Writing – review & editing. AP: Conceptualization, Investigation, Methodology, Writing – review & editing. NY: Conceptualization, Writing – review & editing. SD: Conceptualization, Writing – review & editing.
Funding
The author(s) declare that financial support was received for the research, authorship, and/or publication of this article. Project support was provided by Sivas Cumhuriyet University Scientific Research Projects (CUBAP) (Grant No. F-2021-636).
Acknowledgments
The authors are thankful for the ongoing support of the Advanced Technology and Research Center (CUTAM), Sivas Cumhuriyet University. Further, we would like to thank Nevra Nur Emlik and Gizem Demirkoparan, senior students of the Department of Molecular Biology and Genetics at Sivas Cumhuriyet University, for providing the necessary convenience and encouragement for the maintain of the C. elegans culture.
Conflict of interest
The authors declare that the research was conducted in the absence of any commercial or financial relationships that could be construed as a potential conflict of interest.
Publisher’s note
All claims expressed in this article are solely those of the authors and do not necessarily represent those of their affiliated organizations, or those of the publisher, the editors and the reviewers. Any product that may be evaluated in this article, or claim that may be made by its manufacturer, is not guaranteed or endorsed by the publisher.
References
1. Anisimov VN. Metformin for aging and cancer prevention. Aging (Albany NY). (2010) 2:760–74. doi: 10.18632/aging.100230
2. Anisimov VN. Premature ageing prevention: limitations and perspectives of pharmacological interventions. Curr Drug Targets. (2006) 7:1485–503. doi: 10.2174/1389450110607011485
3. Anisimov VN. Carcinogenesis and aging 20 years after: escaping horizon. Mech Ageing Dev. (2009) 130:105–21. doi: 10.1016/j.mad.2008.02.004
4. Novelle MG, Ali A, Diéguez C, Bernier M, De Cabo R. Metformin: a hopeful promise in aging research. Cold Spring Harbor Perspect Med. (2016) 6:a025932. doi: 10.1101/cshperspect.a025932
5. Glossmann HH, Lutz O. Metformin and aging: a review. Gerontology. (2019) 65:581–90. doi: 10.1159/000502257
6. Pryor R, Cabreiro F. Repurposing metformin: an old drug with new tricks in its binding pockets. Biochem J. (2015) 471:307–22. doi: 10.1042/BJ20150497
7. Valencia WM, Palacio A, Tamariz L, Florez H. Metformin and ageing: improving ageing outcomes beyond glycaemic control. Diabetologia. (2017) 60:1630–8. doi: 10.1007/s00125-017-4349-5
8. Barzilai N, Crandall JP, Kritchevsky SB, Espeland MA. Metformin as a tool to target aging. Cell Metab. (2016) 23:1060–5. doi: 10.1016/j.cmet.2016.05.011
9. Onken B, Sedore CA, Coleman-Hulbert AL, Hall D, Johnson E, Jones EG, et al. Metformin treatment of diverse Caenorhabditis species reveals the importance of genetic background in longevity and healthspan extension outcomes. Aging Cell. (2022) 21:e13488. doi: 10.1111/acel.13488
10. Barbieri M, Bonafe M, Franceschi C, Paolisso G. Insulin/IGF-I-signaling pathway: an evolutionarily conserved mechanism of longevity from yeast to humans. Am J Physiology-Endocrinology Metab. (2003) 285:E1064–71. doi: 10.1152/ajpendo.00296.2003
11. Katic M, Kahn C. The role of insulin and IGF-1 signaling in longevity. Cell Mol Life Sci CMLS. (2005) 62:320–43. doi: 10.1007/s00018-004-4297-y
12. Salih DA, Brunet A. FoxO transcription factors in the maintenance of cellular homeostasis during aging. Curr Opin Cell Biol. (2008) 20:126–36. doi: 10.1016/j.ceb.2008.02.005
13. Kimura KD, Tissenbaum HA, Liu Y, Ruvkun G. daf-2, an insulin receptor-like gene that regulates longevity and diapause in Caenorhabditis elegans. Science. (1997) 277:942–6. doi: 10.1126/science.277.5328.942
14. Kenyon C. The plasticity of aging: insights from long-lived mutants. Cell. (2005) 120:449–60. doi: 10.1016/j.cell.2005.02.002
15. Goetsch PD, Garrigues JM, Strome S. Loss of the Caenorhabditis elegans pocket protein LIN-35 reveals MuvB’s innate function as the repressor of DREAM target genes. PloS Genet. (2017) 13:e1007088. doi: 10.1371/journal.pgen.1007088
16. Sadasivam S, Decaprio JA. The DREAM complex: master coordinator of cell cycle-dependent gene expression. Nat Rev Cancer. (2013) 13:585–95. doi: 10.1038/nrc3556
17. Schertel C, Conradt B. C. elegans orthologs of components of the RB tumor suppressor complex have distinct pro-apoptotic functions. Development. (2007) 134:3691–701. doi: 10.1242/dev.004606
18. Polley SR, Fay DS. A network of genes antagonistic to the LIN-35 retinoblastoma protein of Caenorhabditis elegans. Genetics. (2012) 191:1367–80. doi: 10.1534/genetics.112.140152
19. Bender AM, Kirienko NV, Olson SK, Esko JD, Fay DS. lin-35/Rb and the CoREST ortholog spr-1 coordinately regulate vulval morphogenesis and gonad development in C. elegans. Dev Biol. (2007) 302:448–62. doi: 10.1016/j.ydbio.2006.09.051
20. Brenner S. The genetics of Caenorhabditis elegans. Genetics. (1974) 77:71–94. doi: 10.1093/genetics/77.1.71
21. Byerly L, Cassada RC, Russell RL. The life cycle of the nematode Caenorhabditis elegans. I. Wild-type growth and reproduction. Dev Biol. (1976) 51:23–33. doi: 10.1016/0012-1606(76)90119-6
22. Berk Ş. Insulin and IGF-1 extend the lifespan of Caenorhabditis elegans by inhibiting insulin/insulin-like signaling and mTOR signaling pathways: C. elegans - Focused cancer research. Biochem Biophys Res Commun. (2024) 729:150347. doi: 10.1016/j.bbrc.2024.150347
23. Martinez M, Matus DQ. Auxin-mediated protein degradation in caenorhabditis elegans. Bio Protoc. (2020) 10:e3589. doi: 10.21769/BioProtoc.3589
24. Berk Ş. Aloe vera Gel Extract Prolongs Lifespan in Caenorhabditis elegans. Cumhuriyet Sci J. (2024) 45:40–6. doi: 10.17776/csj.1400264
25. Demirel T, Özdemir ÖÜ, Berk Ş. Caenorhabditis elegans (Maupas 1899). Russian J Nematol. (2024) 32:15–30. doi: 10.24412/0869-6918-2024-1-15-30
26. Bansal A, Kwon ES, Conte D Jr., Liu H, Gilchrist MJ, Macneil LT, et al. Transcriptional regulation of Caenorhabditis elegans FOXO/DAF-16 modulates lifespan. Longev Healthspan. (2014) 3:5. doi: 10.1186/2046-2395-3-5
27. Xiao X, Zhang X, Bai J, Li J, Zhang C, Zhao Y, et al. Bisphenol S increases the obesogenic effects of a high-glucose diet through regulating lipid metabolism in Caenorhabditis elegans. Food Chem. (2021) 339:127813. doi: 10.1016/j.foodchem.2020.127813
28. Hoogewijs D, Houthoofd K, Matthijssens F, Vandesompele J, Vanfleteren JR. Selection and validation of a set of reliable reference genes for quantitative sod gene expression analysis in C. elegans. BMC Mol Biol. (2008) 9:9. doi: 10.1186/1471-2199-9-9
29. Özdemir ÖÜ, Yurt K, Pektaş AN, Berk Ş. Evaluation and normalization of a set of reliable reference genes for quantitative sgk-1 gene expression analysis in Caenorhabditis elegans-focused cancer research. Nucleosides Nucleotides Nucleic Acids. (2024), 1–20. doi: 10.1080/15257770.2024.2317413
30. Vandesompele J, De Preter K, Pattyn F, Poppe B, Van Roy N, De Paepe A, et al. Accurate normalization of real-time quantitative RT-PCR data by geometric averaging of multiple internal control genes. Genome Biol. (2002) 3:research0034.0031. doi: 10.1186/gb-2002-3-7-research0034
31. Mallik R, Chowdhury TA. Metformin in cancer. Diabetes Res Clin Pract. (2018) 143:409–19. doi: 10.1016/j.diabres.2018.05.023
32. Melnik BC, Schmitz G. Metformin: an inhibitor of mTORC1 signaling. J Endocrinol Diabetes Obes. (2014) 2:1029. doi: 10.1016/j.diabres.2018.05.023
33. Henrique Mazucanti C, Victor Cabral-Costa J, Rodrigues Vasconcelos A, Zukas Andreotti D, Scavone C, Mitiko Kawamoto E. Longevity pathways (mTOR, SIRT, Insulin/IGF-1) as key modulatory targets on aging and neurodegeneration. Curr topics medicinal Chem. (2015) 15:2116–38. doi: 10.2174/1568026615666150610125715
34. Floyd S, Favre C, Lasorsa FM, Leahy M, Trigiante G, Stroebel P, et al. The insulin-like growth factor-I–mTOR signaling pathway induces the mitochondrial pyrimidine nucleotide carrier to promote cell growth. Mol Biol Cell. (2007) 18:3545–55. doi: 10.1091/mbc.e06-12-1109
35. Teshiba E, Miyahara K, Takeya H. Glucose-induced abnormal egg-laying rate in Caenorhabditis elegans. Bioscience Biotechnology Biochem. (2016) 80:1436–9. doi: 10.1080/09168451.2016.1158634
36. Lu Z, Qiu Z. High glucose concentration restricts fat consumption in Caenorhabditis elegans. Int J Clin Exp Med. (2017) 10:10554–9.
37. Alcántar-Fernández J, Navarro RE, Salazar-Martínez AM, Pérez-Andrade ME, Miranda-Ríos J. Caenorhabditis elegans respond to high-glucose diets through a network of stress-responsive transcription factors. PloS One. (2018) 13:e0199888. doi: 10.1371/journal.pone.0199888
38. Onken B, Driscoll M. Metformin induces a dietary restriction-like state and the oxidative stress response to extend C. elegans Healthspan via AMPK, LKB1, and SKN-1. PloS One. (2010) 5:e8758. doi: 10.1371/journal.pone.0008758
39. Cabreiro F, Au C, Leung KY, Vergara-Irigaray N, Cochemé HM, Noori T, et al. Metformin retards aging in C. elegans by altering microbial folate and methionine metabolism. Cell. (2013) 153:228–39. doi: 10.1016/j.cell.2013.02.035
40. Kokel D, Li Y, Qin J, Xue D. The nongenotoxic carcinogens naphthalene and para-dichlorobenzene suppress apoptosis in Caenorhabditis elegans. Nat Chem Biol. (2006) 2:338–45. doi: 10.1038/nchembio791
41. Sharma VK, Nautiyal V, Goel KK, Sharma A. Assessment of thermal stability of metformin hydrochloride. Asian J Chem. (2010) 22:3561.
42. Lee SJ, Murphy CT, Kenyon C. Glucose shortens the life span of C. elegans by downregulating DAF-16/FOXO activity and aquaporin gene expression. Cell Metab. (2009) 10:379–91. doi: 10.1016/j.cmet.2009.10.003
43. Beaudoin-Chabot C, Wang L, Celik C, Abdul Khalid AT-F, Thalappilly S, Xu S, et al. The unfolded protein response reverses the effects of glucose on lifespan in chemically-sterilized C. elegans. Nat Commun. (2022) 13:5889. doi: 10.1038/s41467-022-33630-0
44. Schlotterer A, Kukudov G, Bozorgmehr F, Hutter H, Du X, Oikonomou D, et al. C. elegans as model for the study of high glucose–mediated life span reduction. Diabetes. (2009) 58:2450–6. doi: 10.2337/db09-0567
45. Anisimov V, Egormin P, Bershtein L, Zabezhinskii M, Piskunova T, Popovich I, et al. Metformin decelerates aging and development of mammary tumors in HER-2/neu transgenic mice. Bull Exp Biol Med. (2005) 139:721–3. doi: 10.1007/s10517-005-0389-9
46. Anisimov VN, Berstein LM, Egormin PA, Piskunova TS, Popovich IG, Zabezhinski MA, et al. Effect of metformin on life span and on the development of spontaneous mammary tumors in HER-2/neu transgenic mice. Exp gerontology. (2005) 40:685–93. doi: 10.1016/j.exger.2005.07.007
47. Anisimov VN, Berstein LM, Egormin PA, Piskunova TS, Popovich IG, Zabezhinski MA, et al. Metformin slows down aging and extends life span of female SHR mice. Cell Cycle. (2008) 7:2769–73. doi: 10.4161/cc.7.17.6625
48. Tain LS, Lozano E, Sáez AG, Leroi AM. Dietary regulation of hypodermal polyploidization in C. elegans. BMC Dev Biol. (2008) 8:28. doi: 10.1186/1471-213X-8-28
49. Wu L, Zhou B, Oshiro-Rapley N, Li M, Paulo JA, Webster CM, et al. An ancient, unified mechanism for metformin growth inhibition in C. elegans and cancer. Cell. (2016) 167:1705–1718.e1713. doi: 10.1016/j.cell.2016.11.055
50. Martin-Montalvo A, Mercken EM, Mitchell SJ, Palacios HH, Mote PL, Scheibye-Knudsen M, et al. Metformin improves healthspan and lifespan in mice. Nat Commun. (2013) 4:2192. doi: 10.1038/ncomms3192
51. Anisimov VN, Popovich IG, Zabezhinski MA, Egormin PA, Yurova MN, Semenchenko AV, et al. Sex differences in aging, life span and spontaneous tumorigenesis in 129/Sv mice neonatally exposed to metformin. Cell Cycle. (2015) 14:46–55. doi: 10.4161/15384101.2014.973308
52. Smith DLJR, Elam CFJR, Mattison JA, Lane MA, Roth GS, Ingram DK, et al. Metformin supplementation and life span in fischer-344 rats. Journals Gerontology: Ser A. (2010) 65A:468–74. doi: 10.1093/gerona/glq033
53. Pinkston-Gosse J, Kenyon C. DAF-16/FOXO targets genes that regulate tumor growth in Caenorhabditis elegans. Nat Genet. (2007) 39:1403–9. doi: 10.1038/ng.2007.1
54. Pinkston JM, Garigan D, Hansen M, Kenyon C. Mutations that increase the life span of C. elegans inhibit tumor growth. Science. (2006) 313:971–5. doi: 10.1126/science.1121908
55. Lund J, Tedesco P, Duke K, Wang J, Kim SK, Johnson TE. Transcriptional profile of aging in C. elegans. Curr Biol. (2002) 12:1566–73. doi: 10.1016/s0960-9822(02)01146-6
56. Bolanowski MA, Russell RL, Jacobson LA. Quantitative measures of aging in the nematode Caenorhabditis elegans. I. Population and longitudinal studies of two behavioral parameters. Mech Ageing Dev. (1981) 15:279–95. doi: 10.1016/0047-6374(81)90136-6
57. Kenyon C, Chang J, Gensch E, Rudner A, Tabtiang R. A C. elegans mutant that lives twice as long as wild type. Nature. (1993) 366:461–4. doi: 10.1038/366461a0
58. Huang C, Xiong C, Kornfeld K. Measurements of age-related changes of physiological processes that predict lifespan of Caenorhabditis elegans. Proc Natl Acad Sci United States America. (2004) 101:8084–9. doi: 10.1073/pnas.0400848101
59. Uno M, Nishida E. Lifespan-regulating genes in C. elegans. NPJ Aging Mech Dis. (2016) 2:1–8. doi: 10.1038/npjamd.2016.10
Keywords: aging, C. elegans, glucose, lifespan, lin-35, metformin
Citation: Berk Ş, Cetin A, Özdemir ÖÜ, Pektaş AN, Yurtcu N and Dastan SD (2024) The combination of metformin and high glucose increased longevity of Caenorhabditis elegans a DAF-16/FOXO-independent manner: cancer/diabetic model via C. elegans. Front. Endocrinol. 15:1435098. doi: 10.3389/fendo.2024.1435098
Received: 19 May 2024; Accepted: 16 October 2024;
Published: 04 November 2024.
Edited by:
Alessandro Cellerino, Normal School of Pisa, ItalyReviewed by:
Durai Sellegounder, Buck Institute for Research on Aging, United StatesGamze Göger, Afyonkarahisar Health Sciences University, Türkiye
Copyright © 2024 Berk, Cetin, Özdemir, Pektaş, Yurtcu and Dastan. This is an open-access article distributed under the terms of the Creative Commons Attribution License (CC BY). The use, distribution or reproduction in other forums is permitted, provided the original author(s) and the copyright owner(s) are credited and that the original publication in this journal is cited, in accordance with accepted academic practice. No use, distribution or reproduction is permitted which does not comply with these terms.
*Correspondence: Şeyda Berk, c2JlcmtAY3VtaHVyaXlldC5lZHUudHI=
†These authors have contributed equally to this work and share first authorship