- 1Department of Pathology, Lake Erie College of Osteopathic Medicine (LECOM), Erie, PA, United States
- 2Department of Medical Education, School of Medicine, University of Texas Rio Grande Valley (UTRGV), Edinburg, TX, United States
Introduction
Vitamin D is a pleiotropic hormone that regulates calcium and phosphate homeostasis. The roles of vitamin D can be divided into skeletal and nonskeletal. Its skeletal roles include promoting calcium absorption in the intestines, maintaining adequate levels of calcium and phosphate in the bloodstream, and supporting bone mineralization, thus preventing conditions such as rickets and osteoporosis. Nonskeletal roles encompass a range of functions, including immune system modulation, cardiovascular health, and cell growth regulation. Sufficient concentrations of vitamin D in the bloodstream are 20–100 ng/ml in men, women, and children, but individuals with more pigmented skin, live in environments with less sun exposure, or who are older in age are at increased risk of vitamin D deficiency (1). In the past decade, vitamin D has been repeatedly shown to halt tumorigenesis, positively affect T-cell function, improve lung function, and play a role in the pathogenesis of diabetes mellitus. Arsenic has been shown to have harmful consequences on human health, and more individuals are exposed to increasing amounts of arsenic in their environment due to natural sources, such as mineral deposits in water, and unnatural sources, such as pollution. This brief article describes how arsenic might interact with vitamin D.
Arsenic toxicity
Chronic and acute exposure to arsenic causes various harmful consequences. According to the CDC, more than 60,000 ppb of arsenic in water can result in death. Arsenic is naturally found in the environment and enters water via the erosion of rocks that contain this element, or it can be incorporated into food and water via pollution (2). In China, rice contains high concentrations of arsenic, with an average consumption of 3.52 × 10−4 mg/kg of body weight per day, due to the soil (3). Different forms of arsenic include inorganic arsenic, monomethylarsonic acid, dimethylarsinic acid, arsenobetaine, and arsenic trioxide (As2O3). Short-term and long-term exposure to arsenic can have significant health implications, primarily through the ingestion of contaminated water, food, or air. In the short term, high levels of arsenic exposure can lead to acute symptoms such as abdominal pain, vomiting, diarrhea, and, in severe cases, cardiovascular and neurological issues (2). Specifically, acute ingestion of large quantities of arsenic can cause gastrointestinal symptoms within hours and can lead to bloody diarrhea, resulting in hypovolemic shock. Acute cardiovascular effects include QT interval prolongation, arrythmias, and tachycardia. Serious neurological issues include seizures and coma (2). On the other hand, long-term exposure to lower levels of arsenic, often through chronic ingestion of contaminated water, has been linked to more insidious health issues (2). Prolonged exposure is associated with an increased risk of various chronic conditions, including skin lesions (hyperpigmentation and hyperkeratosis), cardiovascular diseases (coronary heart disease, peripheral vascular disease, endothelial dysfunction, hypertension, and atherosclerosis), respiratory problems (chronic obstructive pulmonary disease), neurological conditions (peripheral neuropathy) and certain cancers, such as lung and bladder cancer (2, 4). Management of arsenic toxicity primarily involves removal of the element from further exposure, supportive care to address symptoms such as fluid and electrolyte imbalance, and specific therapies such as chelation therapy to enhance the elimination of arsenic from the body. Chelation therapy includes dimercaprol and dimercaptosuccinic acid. Long-term monitoring and follow-up are crucial for assessing and managing any lingering effects or complications. Notably, chronic arsenic toxicity poses a more subtle but pervasive threat to public health, emphasizing the importance of addressing arsenic contamination in environmental sources to prevent long-term adverse health consequences.
Vitamin D, arsenic, and tumorigenesis
Numerous studies have linked vitamin D inadequacy to increased incidences of colon, prostate, breast, and several hematological cancers. The most compelling evidence shows a strong relationship between vitamin D and colorectal cancer and hematological cancers. To a lesser extent, data show that prostate, breast, and skin cancers are also affected by vitamin D. In colon carcinoma cells, the vitamin D and vitamin D receptor (VDR) complex binds to β-catenin and is associated with complexes within the nucleus, stopping cell proliferation (5, 6). The vitamin D and VRD complex has also been shown to have antiproliferative effects on colorectal cancer by directly and indirectly repressing the WNT/β-catenin pathway, which is most active in colorectal cancer (7). Most importantly, a large portion of advanced colorectal cancer patients lack expression of the VRD gene SNAI1 (snail family transcriptional repressor 1) and SNAI2 (snail family transcriptional repressor 2) proteins, which directly bind and block transcription of the VDR promoter (8, 9), and supplementing these patients with vitamin D in the early stage of colorectal cancer is associated with positive outcomes but not in the advanced stages (5).
Vitamin D deficiency has also been shown to be significantly related to hematological and skin cancer. Vitamin D plays an important role in hematopoiesis and differentiation into myelocytes and granulocytes, hence causing uncontrolled proliferation in undifferentiated neoplastic cells, such as in acute myelocytic leukemia (AML). Vitamin D deficiency has been linked to poorer prognosis in patients with hematological malignancies by limiting differentiation, halting apoptosis, and sensitizing neoplastic cells to anticancer treatments (10, 11). Numerous studies have demonstrated that 1,25(OH)2D3 decreases the phosphorylation of the Janus kinase (JAK) and STAT signaling pathways, both of which are the most commonly overactive pathways in leukemia and lymphoma (12, 13). It is difficult to determine whether vitamin D has preventative effects on skin cancer since UV light is used to convert vitamin D to its active form but can also induce DNA damage. In squamous cell carcinoma, vitamin D and topical cholecalciferol supplementation has been shown to significantly delay the progression of this cancer (14, 15). Similarly, insufficient serum vitamin D levels have been shown to lead to a worse melanoma prognosis (15–18). Studies that were not able to reproduce these results have attributed the difference to confounding variables, such as UV radiation and polymorphisms in VDR (15, 19).
Arsenic has a unique effect on cancer; it can accelerate and decelerate the onset of cancer. The most common arsenic-induced skin cancer is squamous cell carcinoma in situ (20) (Figure 1). Arsenic is also associated with breast, lung, kidney, and liver cancers (21, 22). In fact, the International Agency for Research on Cancer classifies arsenic as a group 1 carcinogen due to its ability to cause lung cancer. In a nine-year longitudinal study, researchers determined the latency period between arsenic exposure by drinking water and the onset of cancer to be 40 years. These studies revealed that men and women have a significantly elevated risk of developing bladder cancer, with relative risks of 4.79 and 6.43, respectively, and of developing lung cancer, with relative risks of 3.38 and 2.41, respectively (22). On the other hand, numerous studies have demonstrated that arsenic trioxide can maintain remission in acute promyelocytic leukemia (APL) patients, has a low side effect profile in phase II and III clinical trials, and effectively treats APL in conjunction with chemotherapy in newly diagnosed APL patients (23). Recently, researchers have revealed a negative correlation between arsenic exposure by drinking water and the incidence of leukemia and lymphoma in men and women (24). These data demonstrate that arsenic has two different effects on hematological and solid cancers.
For the past decade and a half, researchers have shown that vitamin D and arsenic have synergistic effects on halting tumorigenesis and promoting apoptosis in cancer cells (Figure 2). In 2005, Kumagai and colleagues demonstrated that the combination of paricalcitol and As2O3 had antiproliferative effects on myeloid leukemia cells in vitro, especially in the HL-60 and NB-4 cell lines. Overall, paricalcitol and As2O3 increased CD14 expression, increased apoptosis, and significantly decreased the expression of the antiapoptotic proteins Bcl-2 and Bcl-xL in HL-60 cells. Kumagai and colleagues suggested that As2O3 inhibits CYP-24, which destabilizes the mitochondrial membrane and triggers apoptosis, and demonstrated how the mechanism of action of As2O3 and paricalcitol relies on phosphorylation of the ERK pathway. Their study also showed that the combination of As2O3 and paricalcitol decreased PML-RARA fusion protein levels in acute promyelocytic leukemia cells, but the underlying mechanism is unknown (25). The outcomes of this study are important because they could treat myeloid leukemias without adding to the side effect profile of traditional cancer therapies. In hematological malignancies, it has been repeatedly shown that arsenic trioxide and vitamin D synergistically induce apoptosis in tumor cells.
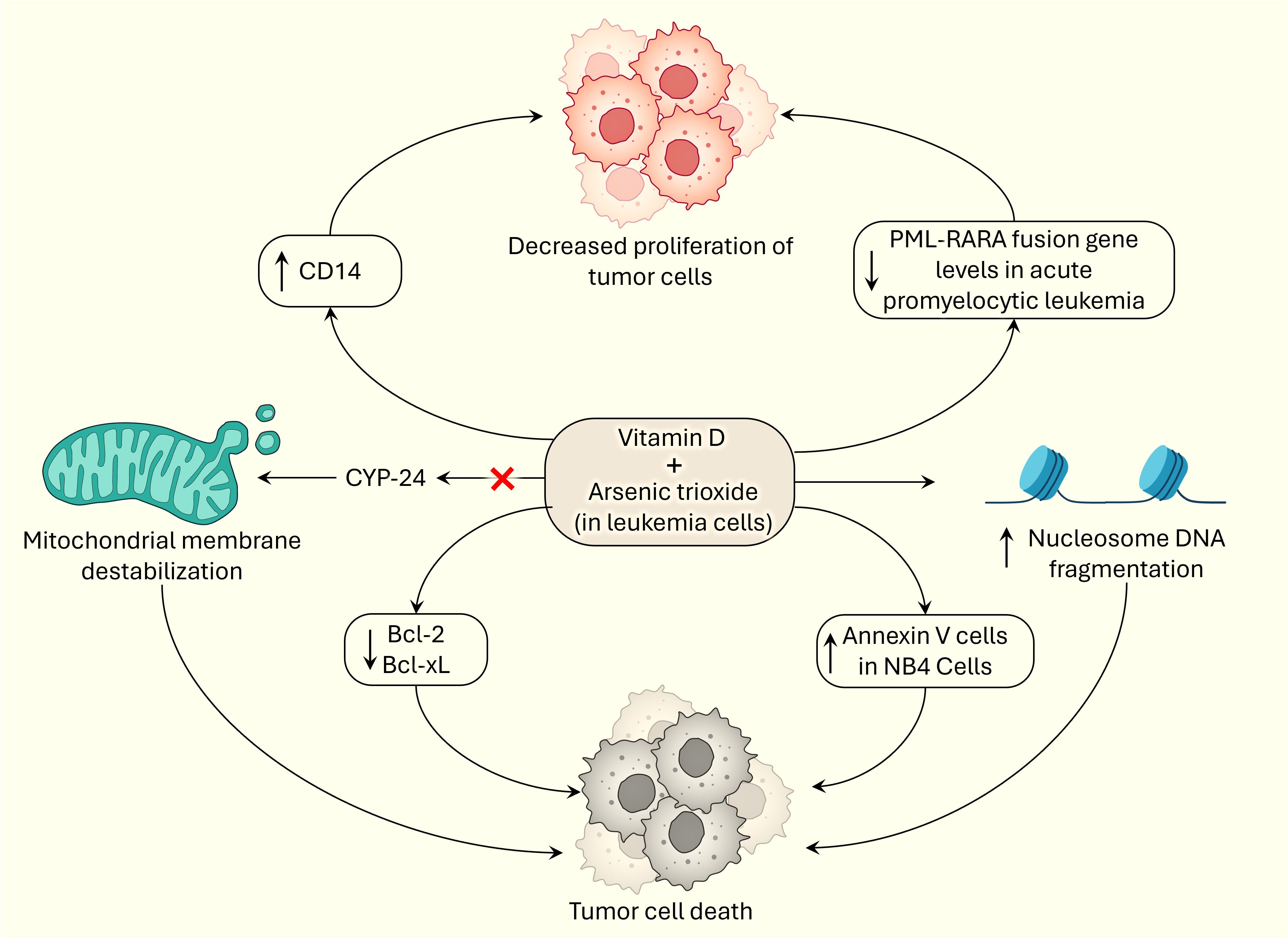
Figure 2 Synergistic effects of vitamin D and arsenic on tumorigenesis by inducing cell death and exerting anti-proliferative effects.
Rogers et al. also confirmed the cytotoxic, apoptotic, and synergistic effects of vitamin D3, rather than paricalcitol, and arsenic trioxide in in vitro HL-60 cells, a subtype of acute promyelocytic leukemia cells. They demonstrated apoptosis in HL-60 cells by displaying a significant increase in annexin V cells, apoptotic cells, and significant nucleosome DNA fragmentation, which is characteristic evidence of apoptosis in cells. It is hypothesized that vitamin D3 and As2O3 participate in the externalization of phosphatidylserine and nucleosomal DNA fragmentation during apoptosis. Lashkari et al. showed that vitamin D3 and As2O3 have synergistic, cytotoxic, and apoptotic effects in a time- and dose-dependent manner and significantly increase the BAX/BCL-2 ratio, specifically increasing BAX and reducing BCL-2 expression, further supporting the conclusions of Kumagai and colleagues (26); the investigators also showed a significant increase in Annexin V-positive NB4 cells, confirming the results of a study performed by Rogers et al. (27). These studies are encouraging because they further demonstrate the potential benefit of including arsenic trioxide and vitamin D in the treatment of hematological malignancies.
Unlike in hematological malignancies, arsenic may induce tumorigenesis in keratinocytes in individuals with deficient serum vitamin D, and sufficient levels of vitamin D were shown to reduce arsenic-induced tumorigenesis. In HaCaT skin keratinocytes, Yajima et al. showed that calcitriol inhibited arsenic-related anchorage-independent growth and decreased phosphorylation of the MEK, ERK1/2 and AKT pathways, which disagrees with the findings of Kumagai et al. Calcitriol also significantly downregulated Cyclin D1 and significantly upregulated p21CIP1 mRNAs; the opposite was true in arsenic-treated HaCaT cells. They also demonstrated that calcitriol significantly reduced arsenic uptake into HaCaT skin keratinocytes by halting the expression of the aquaporin genes AQP7, 9 and 10. Previous research has shown that arsenic can enter cells via Aquaporin 7 and 9 (28, 29). The calcitriol-treated cells had significantly reduced levels of intracellular arsenic, as measured by a plasma mass spectrophotometer. VDR expression significantly increased due to arsenic, but no significant change was detected with calcitriol. Yajima et al. proposed that calcitriol suppresses the expression of aquaporin genes, thereby preventing arsenic uptake and resulting in a reduction in arsenic-mediated tumorigenesis in skin keratinocytes (29). Within safe limits of sunlight exposure, UV can increase serum vitamin D levels and possibly reduce the occurrence of squamous cell carcinoma (14, 15). In conclusion, the relationship between arsenic and vitamin D is complex; in hematological malignancies, the two agents have been repeatedly shown to have synergistic effects on suppressing tumorigenesis. However, in keratinocytes, arsenic can accelerate the onset of squamous cell carcinoma. These studies illustrate that arsenic has different mechanisms of action in various cell types. More experimental studies need to be performed to further explain how arsenic, associated with vitamin D, affects other types of cancers, such as colorectal, prostate, and breast cancer.
Vitamin D, arsenic and T-cell functions
Vitamin D deficiency and arsenic have negative effects on T-cell function. Vitamin D deficiency is linked to Crohn’s disease, multiple sclerosis, rheumatoid arthritis, and systemic lupus erythematous, all of which involve T-cell pathology (30–32). Several studies have demonstrated that inadequate serum vitamin D results in overactivation of the Th1-mediated inflammatory response, and this mechanism is primarily how Th1 cells attack pancreatic beta cells in type 1 diabetes mellitus (33). Many studies have shown that arsenic depresses T-cell function. Several studies have confirmed that arsenic alters the expression of regulatory molecules and cytokine production and causes oxidative stress in T cells (34). Specifically, a cross-sectional study showed that individuals with arsenic-induced skin lesions had a significant reduction in the secretion of the cytokines IL-2, IL-4, and IL-5 by Th1/Th2 cells (35). Others have shown that arsenic inhibits T-cell proliferation (36). In vivo mouse models demonstrated that arsenic alters the mRNA expression of cytokines, causing an altered ratio of Th1/Th2/Th17 differentiation in the lungs and spleen (37). Chronic exposure to arsenic and vitamin D deficiency results in immunosuppression and immunotoxicity.
In addition to tumorigenesis, arsenic and vitamin D together affect immunity and helper T-cell (Th17) function. Burchiel et al. showed that elevated urinary arsenic and its metabolites, monomethylarsonic acid and dimethylarsinic acid, were associated with a decrease in anti-CD3/CD28-stimulated T-cell proliferation in smoking males. This association still existed, but was weaker, in nonsmoking males and not in females due to confounding variables such as lower mean serum vitamin D levels, less sun exposure due to occupation, and a small sample size of smoking females. They also state that males generally had higher serum vitamin D concentrations than females and attribute this difference to sun exposure, gender occupation differences and social norms, but both men and women met the criteria of having adequate vitamin D serum concentrations of >20ng/ml. In smoking men with high serum vitamin D, T-cell proliferation and urinary arsenic showed no correlation, illustrating the protective effect of vitamin D (38). The literature reports that vitamin D and CD28 expression are linked (39); it has been shown that the VDR binding regions are close to the promoter regions of CD28 and are likely direct targets of vitamin D gene transcription (38, 40). Researchers attribute the variation in T-cell activity and proliferation to VDR polymorphisms (26, 41). Parvez et al. showed that elevated urinary arsenic and its metabolites in combination with low vitamin D (less than 20 ng/ml) and sufficient levels of vitamin D (approximately 20 ng/ml) were associated with a decrease in the number of T17 cells, but only high levels had a protective effect against arsenic and Th17 cell count. We confirmed the correlation of elevated urinary arsenic and its metabolites with lower serum vitamin D levels. Parvez et al. suspected that arsenic-related oxidative stress inhibits Lck and Fyn protein tyrosine kinase (PTK) activity, causing a decrease in Th17 cell differentiation (42–45).
Vitamin D, arsenic, type 2 diabetes mellitus and lung functions
Vitamin D deficiency has been repeatedly shown to be linked to decreased lung capacity and function as well as type 2 diabetes mellitus. Among published studies, there are conflicting findings that show the relationship between vitamin D deficiency and lung function. One study showed a significant decrease in forced expiratory volume in one second (FEV1) and forced vital capacity (FVC) in individuals diagnosed with chronic obstructive pulmonary disease and a serum vitamin D concentration less than 20 ng/mL compared to those with chronic obstructive pulmonary disease alone, and confounding variables were controlled for (46–50). On the other hand, other studies have shown no significant change between serum vitamin D and FEV1 or FVC (51, 52), which is likely due to comorbidities. Possible mechanisms responsible for improved lung function with vitamin D sufficiency include airway remodeling (53) and immune dysregulation (54, 55). Nevertheless, there are conflicting data on the relationships among vitamin D deficiency, insulin resistance and type 2 diabetes mellitus. Both a case−control study and a meta-analysis revealed a significant correlation between low vitamin D and impaired fasting glucose levels in type 2 diabetes mellitus patients, and these findings can be attributed to how low vitamin D and elevated blood sugars together maximize total cholesterol and low-density lipoprotein cholesterol and high-density lipoprotein (46, 56). In contrast, another randomized controlled study and another meta-analysis showed that long-term daily vitamin D supplementation did not prevent the onset of type 2 diabetes mellitus (57, 58). In a prospective cohort study, McCarthy et al. showed that vitamin D deficiency increased the incidence of prediabetes, neither confirming nor denying the relationship between vitamin D and type 2 diabetes mellitus (59).
Arsenic exposure is known to have a positive correlation with diminished lung function and could be associated with type 2 diabetes mellitus. Several studies from different populations have repeatedly shown that arsenic is correlated with restrictive lung disease in humans (60). Specifically, a prospective cohort study demonstrated that arsenic at low and moderate doses significantly reduced the FEV1 and FEV in men, women, smokers, and nonsmokers (4). The same significant trend was observed in human fetuses who were exposed to arsenic in utero and whose FEV1 and FEV were measured at a median age of 7.4 years (61). It is unclear whether arsenic can cause type 2 diabetes mellitus in humans because much of the experimental data supporting this relationship is from mouse models in vitro. Arsenic exposure in laboratory rodents results in impaired glucose uptake, pancreatic β-cell defects, impaired adipocyte differentiation, and abnormal microRNA expression of several genes related to insulin secretion (62, 63). A cross-sectional human study showed a significant time-dependent and dose-dependent relationship between inorganic arsenic exposure in drinking water and the risk of developing type 2 diabetes mellitus (64).
Urinary arsenic was measured as micrograms of arsenic per gram of creatine (μg/g creatinine), and serum vitamin D was measured in nmol/L. Le et al. showed that subjects with vitamin D in the 1st quartile, having serum vitamin D levels less than 30.02 units, and urinary arsenic in the 4th quartile, having arsenic concentrations greater than 159.4 8 units, had a 302% increased risk of developing type 2 diabetes mellitus in comparison to subjects with a serum vitamin D level in the 4th quartile, greater than 55.99 units, and urinary arsenic in the 1st quartile, less than 69.67 units (65). This study demonstrated that the combination of vitamin D deficiency and elevated arsenic exposure puts individuals at increased risk for type 2 diabetes mellitus. This is important to investigate further since large portions of the world’s population are exposed to arsenic and deficient in vitamin D. Parvez et al. studied chronic exposure to arsenic in water in smoking men and showed a significant decrease in FEV1 and FVC. Among men who smoked and did not smoke, arsenic exposure significantly decreased the FVC but did not significantly change the FEV1. Subjects with adequate and high levels of serum vitamin D, with the same amount of arsenic exposure, had better FVC and FEV1 (4, 45). This study suggested that vitamin D has a protective effect on pulmonary function against chronic arsenic exposure by drinking water.
Conclusion
In conclusion, the intricate interplay between vitamin D and arsenic has significant implications for human health, particularly in the realms of tumorigenesis, T-cell function, lung health, and type 2 diabetes mellitus. The synergy observed between vitamin D and arsenic in inhibiting hematological malignancies suggests a potential avenue for therapeutic interventions. The role of arsenic in inducing tumorigenesis requires further investigation. Vitamin D seems to play a protective role that preserves T-cell function, proliferation, and differentiation against arsenic exposure and toxicity. Additionally, this article discusses the relationship between vitamin D deficiency, arsenic exposure, and the risk of developing type 2 diabetes mellitus, emphasizing the need for further investigation. Overall, the findings discussed in this manuscript suggest that arsenic can be therapeutic in some cells but harmful in others. However, these findings prompt more studies on how arsenic affects specific groups of human cells and the clinical implications of this effect on human health. It will also be important to study the potential benefits of safe sunlight exposure for individuals with arsenic poisoning (66).
Author contributions
MC: Writing – original draft. CU: Writing – review & editing. DB: Writing – review & editing. MR: Supervision, Conceptualization, Writing – review & editing.
Funding
The author(s) declare that no financial support was received for the research, authorship, and/or publication of this article.
Acknowledgments
We want to express our sincere gratitude to Dr. Nuraly Akimbekov, PhD (Al-Farabi Kazakh National University, Kazakhstan) for drawing the illustrations. MC has successfully obtained a Doctor of Osteopathic Medicine degree from the Lake Erie College of Osteopathic Medicine (LECOM), Erie, Pennsylvania, USA. CU and DB are medical students at the University of Texas Rio Grande Valley (UTRGV), Edinburg, Texas, USA.
Conflict of interest
The authors declare that the research was conducted in the absence of any commercial or financial relationships that could be construed as a potential conflict of interest.
The author(s) declared that they were an editorial board member of Frontiers, at the time of submission. This had no impact on the peer review process and the final decision.
Publisher’s note
All claims expressed in this article are solely those of the authors and do not necessarily represent those of their affiliated organizations, or those of the publisher, the editors and the reviewers. Any product that may be evaluated in this article, or claim that may be made by its manufacturer, is not guaranteed or endorsed by the publisher.
References
1. IOM, I. o. M., Food and Nutrition Board. Dietary Reference Intakes for Calcium and Vitamin D. Washington, DC: National Academy Press (2010).
2. Nurchi VM, Djordjevic AB, Crisponi G, Alexander J, Bjørklund G, Aaseth J. Arsenic toxicity: molecular targets and therapeutic agents. Biomolecules. (2020) 10. doi: 10.3390/biom10020235
3. Liao N, Seto E, Eskenazi B, Wang M, Li Y, Hua J. A comprehensive review of arsenic exposure and risk from rice and a risk assessment among a cohort of adolescents in Kunming, China. Int J Environ Res Public Health. (2018) 15. doi: 10.3390/ijerph15102191
4. Parvez F, Chen Y, Yunus M, Olopade C, Segers S, Slavkovich V, et al. Arsenic exposure and impaired lung function. Findings from a large population-based prospective cohort study. Am J Respir Crit Care Med. (2013) 188:813–9. doi: 10.1164/rccm.201212-2282OC
5. Klampfer L. Vitamin D and colon cancer. World J Gastrointest Oncol. (2014) 6:430–7. doi: 10.4251/wjgo.v6.i11.430
6. Larriba MJ, González-Sancho JM, Barbáchano A, Niell N, Ferrer-Mayorga G, Muñoz A. Vitamin D is a multilevel repressor of wnt/b-Catenin signaling in cancer cells. Cancers (Basel). (2013) 5:1242–60. doi: 10.3390/cancers5041242
7. Toropainen S, Väisänen S, Heikkinen S, Carlberg C. The down-regulation of the human MYC gene by the nuclear hormone 1alpha,25-dihydroxyvitamin D3 is associated with cycling of corepressors and histone deacetylases. J Mol Biol. (2010) 400:284–94. doi: 10.1016/j.jmb.2010.05.031
8. Barbáchano A, Ordóñez-Morán P, García JM, Sánchez A, Pereira F, Larriba MJ, et al. SPROUTY-2 and E-cadherin regulate reciprocally and dictate colon cancer cell tumourigenicity. Oncogene. (2010) 29:4800–13. doi: 10.1038/onc.2010.225
9. Larriba MJ, Martín-Villar E, García JM, Pereira F, Peña C, de Herreros AG, et al. Snail2 cooperates with Snail1 in the repression of vitamin D receptor in colon cancer. Carcinogenesis. (2009) 30:1459–68. doi: 10.1093/carcin/bgp140
10. Bittenbring JT, Neumann F, Altmann B, Achenbach M, Reichrath J, Ziepert M, et al. Vitamin D deficiency impairs rituximab-mediated cellular cytotoxicity and outcome of patients with diffuse large B-cell lymphoma treated with but not without rituximab. J Clin Oncol. (2014) 32:3242–8. doi: 10.1200/JCO.2013.53.4537
11. Kulling PM, Olson KC, Olson TL, Feith DJ, Loughran TP Jr. Vitamin D in hematological disorders and Malignancies. Eur J Haematol. (2017) 98:187–97. doi: 10.1111/ejh.12818
12. Olson KC, Kulling Larkin PM, Signorelli R, Hamele CE, Olson TL, Conaway MR, et al. Vitamin D pathway activation selectively deactivates signal transducer and activator of transcription (STAT) proteins and inflammatory cytokine production in natural killer leukemic large granular lymphocytes. Cytokine. (2018) 111:551–62. doi: 10.1016/j.cyto.2018.09.016
13. Carlberg C, Velleuer E. Vitamin D and the risk for cancer: A molecular analysis. Biochem Pharmacol. (2022) 196:114735. doi: 10.1016/j.bcp.2021.114735
14. Passarelli MN, Karagas MR, Mott LA, Rees JR, Barry EL, Baron JA. Risk of keratinocyte carcinomas with vitamin D and calcium supplementation: a secondary analysis of a randomized clinical trial. Am J Clin Nutr. (2020) 112:1532–9. doi: 10.1093/ajcn/nqaa267
15. Martin-Gorgojo A, Gilaberte Y, Nagore E. Vitamin D and skin cancer: an epidemiological, patient-centered update and review. Nutrients. (2021) 13. doi: 10.3390/nu13124292
16. Davies JR, Field S, Randerson-Moor J, Harland M, Kumar R, Anic GM, et al. An inherited variant in the gene coding for vitamin D-binding protein and survival from cutaneous melanoma: a BioGenoMEL study. Pigment Cell Melanoma Res. (2014) 27:234–43. doi: 10.1111/pcmr.12193
17. Moreno-Arrones OM, Zegeer J, Gerbo M, Manrique-Silva E, Requena C, Traves V, et al. Decreased vitamin D serum levels at melanoma diagnosis are associated with tumor ulceration and high tumor mitotic rate. Melanoma Res. (2019) 29:664–7. doi: 10.1097/CMR.0000000000000638
18. Moro R, Sánchez-Silva A, Aguerralde-Martin M, González-Cuevas R, Peruilh-Bagolini L, Traves V, et al. Prognostic value of vitamin D serum levels in cutaneous melanoma. Actas Dermosifiliogr. (2022) 113:347–53. doi: 10.1016/j.ad.2021.11.001
19. Silva RCD, Alves NMP, Pereira JJS, Coelho AVC, Arraes LC, Brandão LAC, et al. VDR polymorphisms influence immunological response in HIV-1+ individuals undergoing antiretroviral therapy. Genet Mol Biol. (2019) 42:351–6. doi: 10.1590/1678-4685-gmb-2017-0289
20. Panigrahi A, Chakraborty S, Sil A. Arsenical squamous cell carcinoma. Am J Med Sci. (2021) 361:e45. doi: 10.1016/j.amjms.2020.10.013
21. Smith AH, Marshall G, Roh T, Ferreccio C, Liaw J, Steinmaus C. Lung, bladder, and kidney cancer mortality 40 Years after arsenic exposure reduction. J Natl Cancer Inst. (2018) 110:241–9. doi: 10.1093/jnci/djx201
22. Marciniak W, Matoušek T, Domchek S, Paradiso A, Patruno M, Irmejs A, et al. Blood arsenic levels as a marker of breast cancer risk among BRCA1 carriers. Cancers (Basel). (2021) 13. doi: 10.3390/cancers13133345
23. Powell BL. Arsenic trioxide in acute promyelocytic leukemia: potion not poison. Expert Rev Anticancer Ther. (2011) 11:1317–9. doi: 10.1586/era.11.128
24. Lin MH, Li CY, Cheng YY, Guo HR. Arsenic in drinking water and incidences of leukemia and lymphoma: implication for its dual effects in carcinogenicity. Front Public Health. (2022) 10:863882. doi: 10.3389/fpubh.2022.863882
25. Kumagai T, Shih LY, Hughes SV, Desmond JC, O'Kelly J, Hewison M, et al. 19-Nor-1,25(OH)2D2 (a novel, noncalcemic vitamin D analogue), combined with arsenic trioxide, has potent antitumor activity against myeloid leukemia. Cancer Res. (2005) 65:2488–97. doi: 10.1158/0008-5472.CAN-04-2800
26. Lashkari M, Fatemi A, Farsinejad A, Valandani HM, Khalilabadi RM. Vitamin D3, arsenic trioxide, or combination therapy for acute promyelocytic leukemia. Gene Rep. (2022) 27:101576. doi: 10.1016/j.genrep.2022.101576
27. Rogers CS, Yedjou CG, Sutton DJ, Tchounwou PB. Vitamin D3 potentiates the antitumorigenic effects of arsenic trioxide in human leukemia (HL-60) cells. Exp Hematol Oncol. (2014) 3:9. doi: 10.1186/2162-3619-3-9
28. Bhattacharjee H, Rosen BP, Mukhopadhyay R. Aquaglyceroporins and metalloid transport: implications in human diseases. Handb Exp Pharmacol. (2009) 190:309–25. doi: 10.1007/978-3-540-79885-9_16
29. Yajima I, Tazaki A, Ohgami N, Kato M. Calcitriol inhibits arsenic-promoted tumorigenesis through regulation of arsenic-uptake in a human keratinocyte cell line. Am J Cancer Res. (2022) 12:5019–28.
30. Munger KL, Levin LI, Hollis BW, Howard NS, Ascherio A. Serum 25-hydroxyvitamin D levels and risk of multiple sclerosis. Jama. (2006) 296:2832–8. doi: 10.1001/jama.296.23.2832
31. Littorin B, Blom P, Schölin A, Arnqvist HJ, Blohmé G, Bolinder J, et al. Lower levels of plasma 25-hydroxyvitamin D among young adults at diagnosis of autoimmune type 1 diabetes compared with control subjects: results from the nationwide Diabetes Incidence Study in Sweden (DISS). Diabetologia. (2006) 49:2847–52. doi: 10.1007/s00125-006-0426-x
32. Merlino LA, Curtis J, Mikuls TR, Cerhan JR, Criswell LA, Saag KG. Vitamin D intake is inversely associated with rheumatoid arthritis: results from the Iowa Women's Health Study. Arthritis Rheum. (2004) 50:72–7. doi: 10.1002/art.11434
33. Gallo D, Baci D, Kustrimovic N, Lanzo N, Patera B, Tanda ML, et al. How does vitamin D affect immune cells crosstalk in autoimmune diseases? Int J Mol Sci. (2023) 24. doi: 10.3390/ijms24054689
34. Haque R, Chaudhary A, Sadaf N. Immunomodulatory role of arsenic in regulatory T cells. Endocr Metab Immune Disord Drug Targets. (2017) 17:176–81. doi: 10.2174/1871530317666170818114454
35. Biswas R, Ghosh P, Banerjee N, Das JK, Sau T, Banerjee A, et al. Analysis of T-cell proliferation and cytokine secretion in the individuals exposed to arsenic. Hum Exp Toxicol. (2008) 27:381–6. doi: 10.1177/0960327108094607
36. Burchiel SW, Lauer FT, Beswick EJ, Gandolfi AJ, Parvez F, Liu KJ, et al. Differential susceptibility of human peripheral blood T cells to suppression by environmental levels of sodium arsenite and monomethylarsonous acid. PloS One. (2014) 9:e109192. doi: 10.1371/journal.pone.0109192
37. Zhao L, Yang S, Guo Y, Sun G, Li B. Chronic arsenic exposure in drinking water interferes with the balances of T lymphocyte subpopulations as well as stimulates the functions of dendritic cells in vivo. Int Immunopharmacol. (2019) 71:115–31. doi: 10.1016/j.intimp.2019.03.022
38. Burchiel SW, Lauer FT, Factor-Litvak P, Liu X, Islam T, Eunus M, et al. Arsenic exposure associated T cell proliferation, smoking, and vitamin D in Bangladeshi men and women. PloS One. (2020) 15:e0234965. doi: 10.1371/journal.pone.0234965
39. Kongsbak M, Levring TB, Geisler C, von Essen MR. The vitamin d receptor and T cell function. Front Immunol. (2013) 4:148. doi: 10.3389/fimmu.2013.00148
40. Cantorna MT, Snyder L, Lin YD, Yang L. Vitamin D and 1,25(OH)2D regulation of T cells. Nutrients. (2015) 7:3011–21. doi: 10.3390/nu7043011
41. Yang L, Wu L, Fan Y, Ma J. Vitamin D receptor gene polymorphisms in association with diabetic nephropathy: a systematic review and meta-analysis. BMC Med Genet. (2017) 18:95. doi: 10.1186/s12881-017-0458-8
42. Castro-Sanchez P, Teagle AR, Prade S, Zamoyska R. Modulation of TCR signaling by tyrosine phosphatases: from autoimmunity to immunotherapy. Front Cell Dev Biol. (2020) 8:608747. doi: 10.3389/fcell.2020.608747
43. Dai P, Liu X, Li QW. [Function of the Lck and Fyn in T cell development]. Yi Chuan. (2012) 34:289–95. doi: 10.3724/SP.J.1005.2012.00289
44. Laird RM, Hayes SM. Roles of the Src tyrosine kinases Lck and Fyn in regulating gammadeltaTCR signal strength. PloS One. (2010) 5:e8899. doi: 10.1371/journal.pone.0008899
45. Parvez F, Lauer FT, Factor-Litvak P, Islam T, Eunus M, Horayara MA, et al. Exposure to arsenic and level of Vitamin D influence the number of Th17 cells and production of IL-17A in human peripheral blood mononuclear cells in adults. PloS One. (2022) 17:e0266168. doi: 10.1371/journal.pone.0266168
46. Persson LJ, Aanerud M, Hiemstra PS, Michelsen AE, Ueland T, Hardie JA, et al. Vitamin D, vitamin D binding protein, and longitudinal outcomes in COPD. PloS One. (2015) 10:e0121622. doi: 10.1371/journal.pone.0121622
47. Rafiq R, Prins HJ, Boersma WG, Daniels JM, den Heijer M, Lips P, et al. Effects of daily vitamin D supplementation on respiratory muscle strength and physical performance in vitamin D-deficient COPD patients: a pilot trial. Int J Chron Obstruct Pulmon Dis. (2017) 12:2583–92. doi: 10.2147/COPD
48. Heulens N, Korf H, Cielen N, De Smidt E, Maes K, Gysemans C, et al. Vitamin D deficiency exacerbates COPD-like characteristics in the lungs of cigarette smoke-exposed mice. Respir Res. (2015) 16:110. doi: 10.1186/s12931-015-0271-x
49. Ganji V, Al-Obahi A, Yusuf S, Dookhy Z, Shi Z. Serum vitamin D is associated with improved lung function markers but not with prevalence of asthma, emphysema, and chronic bronchitis. Sci Rep. (2020) 10:11542. doi: 10.1038/s41598-020-67967-7
50. Ahn KM, Kim SS, Lee SY, Lee SH, Park HW. Vitamin D deficiency and lung function decline in healthy individuals: a large longitudinal observation study. Respir Med. (2021) 182:106395. doi: 10.1016/j.rmed.2021.106395
51. Sanjari M, Soltani A, Habibi Khorasani A, Zareinejad M. The effect of vitamin D on COPD exacerbation: a double blind randomized placebo-controlled parallel clinical trial. J Diabetes Metab Disord. (2015) 15:33. doi: 10.1186/s40200-016-0257-3
52. Martineau AR, James WY, Hooper RL, Barnes NC, Jolliffe DA, Greiller CL, et al. Vitamin D3 supplementation in patients with chronic obstructive pulmonary disease (ViDiCO): a multicentre, double-blind, randomised controlled trial. Lancet Respir Med. (2015) 3:120–30. doi: 10.1016/S2213-2600(14)70255-3
53. Song Y, Qi H, Wu C. Effect of 1,25-(OH)2D3 (a vitamin D analogue) on passively sensitized human airway smooth muscle cells. Respirology. (2007) 12:486–94. doi: 10.1111/j.1440-1843.2007.01099.x
54. Afzal M, Kazmi I, Al-Abbasi FA, Alshehri S, Ghoneim MM, Imam SS, et al. Current overview on therapeutic potential of vitamin D in inflammatory lung diseases. Biomedicines. (2021) 9. doi: 10.3390/biomedicines9121843
55. Ilyas M, Agussalim A, Megawati M, Massi N, Djaharuddin I, Bakri S, et al. Relationship between vitamin D level and serum TNF-α Concentration on the severity of chronic obstructive pulmonary disease. Open Access Maced J Med Sci. (2019) 7:2298–304. doi: 10.3889/oamjms.2019.663
56. Li X, Liu Y, Zheng Y, Wang P, Zhang Y. The effect of vitamin D supplementation on glycemic control in type 2 diabetes patients: A systematic review and meta-analysis. Nutrients. (2018) 10. doi: 10.3390/nu10030375
57. Zheng JS, Luan J, Sofianopoulou E, Sharp SJ, Day FR, Imamura F, et al. The association between circulating 25-hydroxyvitamin D metabolites and type 2 diabetes in European populations: a meta-analysis and Mendelian randomisation analysis. PloS Med. (2020) 17:e1003394. doi: 10.1371/journal.pmed.1003394
58. Pittas AG, Dawson-Hughes B, Sheehan P, Ware JH, Knowler WC, Aroda VR, et al. Vitamin D supplementation and prevention of type 2 diabetes. N Engl J Med. (2019) 381:520–30. doi: 10.1056/NEJMoa1900906
59. McCarthy K, Laird E, O'Halloran AM, Walsh C, Healy M, Fitzpatrick AL, et al. Association between vitamin D deficiency and the risk of prevalent type 2 diabetes and incident prediabetes: a prospective cohort study using data from The Irish Longitudinal Study on Ageing (TILDA). EClinicalMedicine. (2022) 53:101654. doi: 10.1016/j.eclinm.2022.101654
60. Sanchez TR, Powers M, Perzanowski M, George CM, Graziano JH, Navas-Acien A. A meta-analysis of arsenic exposure and lung function: is there evidence of restrictive or obstructive lung disease? Curr Environ Health Rep. (2018) 5:244–54. doi: 10.1007/s40572-018-0192-1
61. Signes-Pastor AJ, Martinez-Camblor P, Baker E, Madan J, Guill MF, Karagas MR. Prenatal exposure to arsenic and lung function in children from the New Hampshire Birth Cohort Study. Environ Int. (2021) 155:106673. doi: 10.1016/j.envint.2021.106673
62. Navas-Acien A, Silbergeld EK, Streeter RA, Clark JM, Burke TA, Guallar E. Arsenic exposure and type 2 diabetes: a systematic review of the experimental and epidemiological evidence. Environ Health Perspect. (2006) 114:641–8. doi: 10.1289/ehp.8551
63. Beck R, Styblo M, Sethupathy P. Arsenic exposure and type 2 diabetes: microRNAs as mechanistic links? Curr Diabetes Rep. (2017) 17:18. doi: 10.1007/s11892-017-0845-8
64. Islam R, Khan I, Hassan SN, McEvoy M, D'Este C, Attia J, et al. Association between type 2 diabetes and chronic arsenic exposure in drinking water: a cross sectional study in Bangladesh. Environ Health. (2012) 11:38. doi: 10.1186/1476-069X-11-38
65. Lee BK, Kim Y. Association of diabetes mellitus with a combination of vitamin d deficiency and arsenic exposure in the korean general population: analysis of 2008-2009 korean national health and nutrition examination survey data. Ann Occup Environ Med. (2013) 25:7. doi: 10.1186/2052-4374-25-7
Keywords: arsenic, vitamin D, skin, tumor, T cell
Citation: Chittilla M, Uzoma C, Brewer D and Razzaque MS (2024) Potential association between arsenic and vitamin D. Front. Endocrinol. 15:1430980. doi: 10.3389/fendo.2024.1430980
Received: 10 May 2024; Accepted: 28 June 2024;
Published: 17 July 2024.
Edited by:
Andras Balla, Semmelweis University, HungaryReviewed by:
Rita Benkő, Semmelweis University, HungaryCopyright © 2024 Chittilla, Uzoma, Brewer and Razzaque. This is an open-access article distributed under the terms of the Creative Commons Attribution License (CC BY). The use, distribution or reproduction in other forums is permitted, provided the original author(s) and the copyright owner(s) are credited and that the original publication in this journal is cited, in accordance with accepted academic practice. No use, distribution or reproduction is permitted which does not comply with these terms.
*Correspondence: Mohammed S. Razzaque, mohammed.razzaque@utrgv.edu; msr.nagasaki@gmail.com