- 1Department of Medicine and Surgery, University of Parma and Unit of Pediatrics, University Hospital of Parma, Parma, Italy
- 2Unit of Paediatrics, University Hospital of Parma, P. Barilla Children’s Hospital, Parma, Italy
- 3Microbiome Research Hub, University of Parma, Parma, Italy
- 4Unit of Pediatrics, University Hospital of Modena, Department of Medical and Surgical Sciences for Mothers, Children and Adults, University of Modena and Reggio Emilia, Reggio Emilia, Italy
The thyroid gland regulates most of the physiological processes. Environmental factors, including climate change, pollution, nutritional changes, and exposure to chemicals, have been recognized to impact thyroid function and health. Thyroid disorders and cancer have increased in the last decade, the latter increasing by 1.1% annually, suggesting that environmental contaminants must play a role. This narrative review explores current knowledge on the relationships among environmental factors and thyroid gland anatomy and function, reporting recent data, mechanisms, and gaps through which environmental factors act. Global warming changes thyroid function, and living in both iodine-poor areas and volcanic regions can represent a threat to thyroid function and can favor cancers because of low iodine intake and exposure to heavy metals and radon. Areas with high nitrate and nitrite concentrations in water and soil also negatively affect thyroid function. Air pollution, particularly particulate matter in outdoor air, can worsen thyroid function and can be carcinogenic. Environmental exposure to endocrine-disrupting chemicals can alter thyroid function in many ways, as some chemicals can mimic and/or disrupt thyroid hormone synthesis, release, and action on target tissues, such as bisphenols, phthalates, perchlorate, and per- and poly-fluoroalkyl substances. When discussing diet and nutrition, there is recent evidence of microbiome-associated changes, and an elevated consumption of animal fat would be associated with an increased production of thyroid autoantibodies. There is some evidence of negative effects of microplastics. Finally, infectious diseases can significantly affect thyroid function; recently, lessons have been learned from the SARS-CoV-2 pandemic. Understanding how environmental factors and contaminants influence thyroid function is crucial for developing preventive strategies and policies to guarantee appropriate development and healthy metabolism in the new generations and for preventing thyroid disease and cancer in adults and the elderly. However, there are many gaps in understanding that warrant further research.
1 Introduction
The main function of the thyroid gland is to produce triiodothyronine (T3) and thyroxine (T4). Thyroid hormones are key regulators of basal energy expenditure and metabolism, regular development and differentiation of cells, neurodevelopmental processes in the first years of life, linear growth, body composition and weight.
Normal thyroid function is regulated by the hypothalamic thyrotropin-releasing hormone (TRH) that stimulates the release of the thyroid-stimulating hormone (TSH) from the pituitary gland, which in turn drives synthesis and secretion of the thyroid hormones from the thyroid gland (1). The development of the thyroid gland begins around the third week of gestation, originating from an evagination of the pharyngeal floor in correspondence with the second branchial arch. At the end of the 7th week of gestation, the embryonic thyroid is in its final position in front of the trachea. The production and secretion of thyroid hormones into the blood starts as early as the 10th- 12th week of gestation (2). Since prenatal life, normal thyroid hormone production plays a key role in several physiological functions, such as the growth and development of the organism, including neurological and cognitive functions (3). In newborns, changes and defects in thyroid anatomy and function are related with pathological conditions, the most common being congenital hypothyroidism (CH), affecting 1 in 3,000 newborns (4); thyroid dysgenesis (agenesis, hypoplasia, and ectopy), thyroid dyshormonogenesis, hypothalamic-pituitary axis alterations and thyroid hormone resistance are all observed in this condition. Neonatal hyperthyroidism instead is associated mainly with Graves’ disease in mothers because of the transplacental passage of Thyrotropin-Receptor antibodies (TRAb) (5).
In children, acquired hypothyroidism is caused primarily by autoimmune thyroiditis with a current prevalence of 1–2% among children and adolescents (6), whereas the most frequent cause of hyperthyroidism is represented by Graves’ disease, more common in girls than in boys, with an incidence of 0.1 in 100,000 children and 3 in 100,000 adolescents (7). Low iodine availability can cause acquired hypothyroidism (8).
Thyroid cancers are less common in children than in adults, but it has been estimated that 1.8% of thyroid malignancies diagnosed in the US are in people aged less than 20 years. In recent years, a significant increase in pediatric thyroid cancers has been reported, in particular papillary thyroid carcinoma (PTC) and follicular thyroid carcinoma (FTC), whereas anaplastic (ATC), medullary (MTC), and poorly differentiated (PDTC) thyroid carcinomas are rarer (9).
In adults, thyroid diseases represent the most frequent endocrine disorders. The global prevalence of autoimmune diseases in pediatric age is about 5%. Among these, the most frequent autoimmune diseases are represented by autoimmune thyroid diseases, which include both Graves’ disease (GD) and Hashimoto’s thyroiditis (HT) (10). The epidemiological data of hypothyroidism ranges from 0.2% to 5.3% in Europe and from 0.3% to 3.7% in the USA, and is frequently associated with iodine deficiency and autoimmune thyroiditis. The prevalence of hyperthyroidism instead is similar in Europe (0.7%) and in the USA (0.5%). Graves’ disease is the major cause of hyperthyroidism in adults as in children, followed by toxic multinodular goiter and thyroid adenoma (11). Several studies have identified the importance genetic predisposition. Despite this, 20–25% of the phenotypic variation in autoimmune thyroiditis is due to environmental and/or epigenetic factors that also may be influenced by the environment (12).
All the different thyroid conditions mentioned so far have shown changes in their prevalence over time; this has suggested an important effect on the environment, including pollution and contaminants. Climate change, air and water pollution, nutrition and infectious agents can play an important role both in prenatal and postnatal life, influencing endocrine functions. Several studies have been conducted with the aim to investigate the role of environmental factors on thyroid function.
The synthesis, transportation and metabolism of thyroid hormones can be modified by toxic agents, that can interfere with hormone receptors and allow the beginning of autoimmune processes.
Also, air pollution exposure can cause thyroid diseases, and studies have considered the effects of carbon monoxide (CO), nitrogen dioxide (NO2), sulfur dioxide (SO2), ozone (O3), and particulate matter <2,5 μm (PM2.5), <10 μm (PM10) (13).
There is, in addition, a growing interest in endocrine-disrupting chemicals (EDCs) due to their wide use and pervasive presence in nature. All EDCs can change endocrine functions, as they can mimic or interfere with the normal functioning of the endocrine system and play a crucial role in regulating various physiological processes in the human body, including thyroid function (14, 15). EDCs include industrial agents (dioxins, polychlorinated biphenyls (PCBs) and alkylphenols), agricultural agents (herbicides, pesticides, fungicides, and insecticides), bisphenols (BPA, BPS, BPF), phthalates, drugs (ketoconazole, mitotane, cardiac glycosides, carbamazepine and nitrofurans) and heavy metals (cadmium, arsenic, nickel, beryllium and chromium) (16), and they are related to potential interference with normal thyroid function, and exposure is associated with a higher prevalence of thyroid diseases (17, 18).
The aim of this narrative review is to explore and consider comprehensively how environmental factors, such as the above-mentioned environmental pollution, climate change, EDCs, diet and micro-nutrients, and infectious agents influence thyroid function and disease. At variance with previous studies, we have focused also on the available evidence in relationship with the different ages of life, in particular, the prenatal, perinatal, infancy, adolescence, and adult periods of life were considered.
2 Materials and methods
2.1 Literature search strategy and selection criteria
The research of the literature was done using specific research strings in Pubmed, Scopus, and Mendeley, via MeSH using specific keywords. The Cochrane Library database and all bibliographic sources available on scientific databases, such as the WHO and the European international official sites, were considered in addition. Original articles, including animal and in vitro studio, reviews, and epidemiological studies were selected, focusing initially on those published in the last 10 years. Initially, the identified records were imported into Mendeley. After removing duplicates, reviewers separately screened the included papers found through the literature search by title and abstract, using the eligibility criteria. For specific topics when literature was scarce, we included even older publications. The following keywords were used for the search: thyroid hormones, TSH, fT3, fT4, T3, and T4, thyroid, thyroid gland, thyroid dysfunction, thyroid function, endocrine disrupting chemicals, EDCs, BPA, BPS, BPF, phthalates, pesticides, pollutants, air pollution, water pollution, Particulate matter (PM)2,5, PM10, heavy metals, climate changes, nutrients, diet, covid19, infections, pregnancy, prenatal, perinatal period, infancy, adolescence, adult life, that were differently matched for all required strings (e.g., Figure 1).
2.2 Data extraction
Following title and abstract screening, relevant papers were further evaluated. To collect data from the selected papers, a predefined data extraction strategy was used. The extracted data included information such as authors, year of publication, title, abstract, contaminants analyzed, research domain, and study type. Finally, the remaining articles were evaluated after reading the complete content. Furthermore, one author conducted additional screening and data extraction for the most recent publications.
2.3 Narrative synthesis
A narrative synthesis was conducted to organize the search findings. To identify the most recent problematic points, the synthesis focused on specific environmental factors and contaminants that influence thyroid development and function.
3 Effects of environmental pollutants on thyroid function and disease
Climate change, driven by human activities such as deforestation and burning fossil fuels, has far-reaching consequences for health. Rising temperatures, air pollution, extreme weather events, and changes in disease patterns represent current direct and indirect threats to child health, particularly to the thyroid gland.
The US Environmental Protection Agency (EPA) defines pollution as “any substances in water, soil, or air that degrades the natural quality of the environment; offends [the senses]; causes a health hazard; or [impairs] the usefulness of natural resources” (19). The WHO considers air pollution as a major environmental health issue that affects everyone in low-, middle-, and high-income countries (13). Also, European paediatric scientific societies are highly concerned about the influence of environmental pollution on child health (20). Both the European Society for Paediatric Endocrinology (ESPE) and the European Society for Endocrinology (ESE) are collaborating on a common project, the EndoCompass project (https://www.ese-hormones.org/what-we-do/research/endocompass-european-research-roadmap/), that among many other aspects considers the effect of environmental contaminants, pollution, and climate change.
Air pollutants mainly include particulate matter (PM), ozone pollution (O3), carbon monoxide (CO), nitrogen oxides (NO2 and NOx), and sulfur dioxide (SO2). PM is a mixture of suspended particles with different chemical compositions, classified by size (PM10 and PM2.5) (12); in particular, PM2.5 particles differ from other PM particles having a higher penetration capacity and reactivity, so that PM2.5 has become the most relevant air pollutant causing the greatest threat to global public health (13).
Nitrate is a common contaminant of drinking water, particularly in agricultural areas, due to the usage of nitrogen-containing fertilizers. High amounts of nitrate might also be present in some fruits and vegetables (21).
Heavy metals are naturally present in the environment, although their concentrations vary a lot in different geographic areas. They include both essential metals, required micro-nutrients for biological processes (Fe: Iron, Cu: Copper, Zn: Zinc, Se: Selenium, etc.), and toxic chemicals that may damage cell biology and promote malignant transformation (As: Arsenic, Cd: Cadmium, Hg: Mercury, Ni: nickel, etc.). In recent decades, metal pollution of the environment has increased worldwide (22). Water, soil, and atmosphere are vehicles that may be enriched with heavy metals due to their proximity to sources, including natural emissions (non-anthropogenic) and pollution secondary to human activities (anthropogenic). Industry, agriculture, and technology are activities related to higher heavy metal exposure (23).
There are strong links between environmental pollution and adverse health effects in humans; children, in particular, are vulnerable at every level of development (24). Studies have focused mainly on allergic diseases related to increased allergen production, infectious diseases, respiratory diseases, diabetes, and cardiovascular diseases (25).
The following paragraphs focus on the possible associations of environmental pollution on thyroid function in the different ages of life.
3.1 Climate and thyroid function
Climate change is a global issue that affects environment, from increased temperatures, floods, droughts, and wildfires to sea level changes and loss of biodiversity. Heatwaves can lead to dehydration, heat exhaustion, endocrine imbalance, and even heatstroke. Rising temperatures can disrupt the thyroid’s ability to maintain hormonal balance. Researchers have observed an association between outdoor temperature and thyroid hormone levels, with increasing TSH and fT3 during autumn-winter periods and decreasing of these during spring-summer periods (26).
Climate change contributes to the release of pollutants and chemicals into the environment, as nitrates. Several studies have shown that the climate significantly impacts the process of nitrate accumulation in plant tissue, with nitrate concentration being lower in years with high rainfall. Seasonal heavy rains wash away soil minerals, while temperature spikes contribute to elevated nitrate and nitrite concentrations; moreover, high temperatures induce moisture loss in fruits and vegetables, all of these amplifying nitrate and nitrite levels in agricultural products, especially vegetables (27), which have a negative impact on the thyroid gland. Also, increased volcanic activity in recent years is associated with a higher risk of thyroid cancer, which will be further described below (23, 28).
Global warming also causes the evaporation of iodide from seawater, and in regions distant from the coast, it results in a depletion of iodide in plant foods and drinking water and iodine deficiency (29). Furthermore, using data-mining techniques, it has been shown that nowadays, climate variables in the aridity index and precipitation, soil leaching, and pH decrease, all predict soil selenium losses that could increase the prevalence of selenium deficiency (30), increasing in turn serum TSH levels.
3.2 Effects of particulate matter exposure on thyroid function
Only a few studies have investigated, to date, associations between PM exposure and thyroid function during childhood.
In the newborn, data is not only scarce but also conflicting. The ENVIRONAGE birth cohort study (N:499 newborns) focused on PM2.5 exposure during the third trimester of pregnancy, reporting an inverse association between exposure and cord blood TSH levels and FT4/FT3 ratio (31). At variance, other studies reported that prenatal exposure to PM2.5 and PM10 air pollution was associated with higher newborn total T4 concentrations (32, 33). Howe et al. identified months 3 to 7 for PM2.5 exposure and 1 to 8 for PM10 exposure of pregnancy as critical windows of exposure, which have been associated with higher thyroxine levels (32). Irizar et al. also suggested that exposure to PM2.5 during pregnancy was positively associated with TT4 levels in newborns: TT4 levels collected 3 days after birth increased by 0.206 μg/dl for each 1 μg/m3 of increase in exposure to PM2.5 during pregnancy. This study analyzed but found no association between NO2 exposure levels and TT4 levels at birth (33). The apparent difference between the results of these studies is unclear, and probably due to differences in assay methods as most of T4 is bound to serum transport proteins, such as thyroxin-binding globulin (TBG), and free T4 makes up only for a small fraction (0.03%) of TT4 (34).
The findings in newborns/infants are, however, in agreement with the data collected in adults, as shown in the Di@bet.es study (35), where a significant negative association between exposures to PM2.5 and thyroid hormone levels was described, moreover, with relatively low PM2.5 concentration ranges, well below the existing European Ambient Air Quality Directive target values (PM2.5<25 µg/m3) (20). To date, the WHO guidelines recommend a maximum annual PM2.5 exposure < 5 µg/m3 (16).
There are no published data on the effects of other pollutants on thyroid function in the pediatric age (36).
3.3 Effects of exposure to nitrates and nitrites on thyroid function
There are limited studies that have investigated NO2 pollution and thyroid hormone levels at birth, without observing any significant association (32, 33). However, one study from China found that higher maternal NO2 exposure was linked to the risk of congenital hypothyroidism in newborns (37), but further research is needed.
According to WHO, drinking water is the most likely primary source of nitrate exposure. Another source can be vegetables and fruits when nitrate concentrations are > 10 mg/L (38). Studies from Bulgaria, Slovakia, Germany and the USA have reported associations between nitrate exposure and thyroid function in different age periods: some of these have reported that school children and pregnant women exposed to high nitrate levels in drinking water (75 mg/L) had a higher risk of developing goiter and thyroid disorders than school children and pregnant women exposed to low nitrate levels (8 mg/L) (39–42). Another study conducted in Slovakia school children described a significantly greater thyroid volume in children from high-exposure nitrate areas (> 200 mg/L) compared with children of comparable age from low-exposure nitrate areas (< 2 mg/L). No differences in the levels of T3 or T4 were found, but a higher prevalence of TSH levels in the range > 4.0 mIU/L was observed, in addition to increased anti-thyroid peroxidase antibodies in the children living in high-exposure nitrate areas. These studies confirmed excessive nitrate levels in drinking water might be a risk factor for thyroid dysfunction in vulnerable population groups (40, 41).
3.4 Relationships between environmental pollution and thyroid cancer
The global incidence of thyroid cancer has increased rapidly, with a rate of +1.1% per year in the last decades (43–45). In fact, according to the Surveillance, Epidemiology, and End Results (SEER) program, new cases of thyroid cancer in people under the age of 20 years account for 1.8% of all thyroid malignancies diagnosed. Among the pediatric population, adolescents seem to have a 10-fold greater incidence than younger children, with a female-to-male proportion (5:1). In particular, in 15- to 19-year-old adolescents, thyroid cancer represents the eighth most frequently diagnosed cancer, and the second most common cancer among girls (45). However, a large geographical heterogeneity in incidence rates has been evidenced. Epidemiology and country-specific trends in children and adolescents seem similar to those highlighted in adults. Although some authors suggest that this might account for overdiagnosis in the pediatric population (43), the hypothesis of environmental factors being of importance for thyroid cancer must be taken into serious consideration. Currently, it is well known that exposure to some environmental pollutants, such as radioiodine, plays a role in carcinogenesis. Following the Chornobyl disaster, in fact, areas next to the nuclear power plant registered an increase in thyroid cancers; the Belarus pediatric population, in particular, was screened and presented a rapid increase in thyroid cancer incidence in children after 1986, especially in the most radionuclide contaminated areas (44, 45).
The International Agency for Research on Cancer (IARC) has classified outdoor air pollution and particulate matter (PM) in outdoor air pollution as carcinogenic to humans, and in accordance with the WHO, in 2013, particulate matter air pollution was declared as carcinogenic (30). Air pollution contains several chemicals capable of producing genomic instability, which is an underlying feature of cancer development (46, 47). The mechanism by which PM2.5 might cause thyroid cancer is unclear; probably, it is related to volatile organic compounds that are bound to particulate matter that might penetrate the bloodstream through the alveolar barrier.
Both a study conducted in the US (48) and one conducted in Brazil (49) showed an association between particulate matter and the development of thyroid cancer; a 10 μg/m3 increase in PM2.5 concentration over 12, 24, and 36 months was associated with a greater likelihood of being diagnosed with Papillary thyroid carcinoma (PTC), with this likelihood increasing with increasing duration of exposure to PM2.5. Additionally, a study of 550,000 patients in China found that emissions from industrial waste gas (consisting mainly of particulate matter, sulfur dioxide, and nitrogen dioxide) were associated with increased thyroid cancer (50). At variance with these findings, Park et al. found that exposure to particulate matter was negatively associated with the incidence of thyroid cancer (51). These conflicting findings could be due to the fact that this study did not discuss the methods used to measure the levels of particulate matter, and it was not clear how these levels were linked to each patient, limiting the generalizability of these findings.
A further study demonstrated that the probability of developing papillary thyroid cancer increased with a longer duration of PM2.5 exposure: a 5 μg/m3 increase of PM2.5 concentrations over 12 months of exposure was not associated with the incidence of PTC, but the odds of developing PTC after a 5-μg/m3 increase in PM2.5 exposure was increased by 18% in 24-month and by 23% after 36-months of exposure (52).
Heavy metals are considered among known carcinogenic environmental pollutants. The carcinogenic effect of metals on target cells can occur through several mechanisms, such as causing changes in bioavailability and intracellular distribution of peptides by interacting with intracellular proteins, enzymes, and other cell components. The possible carcinogenic mechanisms involve an increase in oxidative stress, that may cause oxidative DNA damage, interfere with DNA repair systems, deregulate growth control mechanisms, and modify DNA methylation patterns (30).
Living in volcanic areas represents a further risk factor as some of the highest incidences of thyroid cancer are registered in volcanic regions (53, 54), and these areas have higher concentrations of heavy metals. However, the causative role of heavy metals is far from being properly understood. To date, in fact, the dose and duration of exposure to heavy metals that might be harmful are not well defined. For some heavy metals (Cd, Hg), for which carcinogenic role is known, there are no specific exposure levels that indicate toxicity, as toxicity is not related only to environmental concentrations, but depends on other mechanisms also that have not yet been taken into consideration (30, 55). Furthermore, exposure to a combination of heavy metals rather than a single could account for the toxicity (56, 57). This concept is generally valid for environmental exposure to pollutants as each individual is exposed to multiple pollutants that react with the human body chemical environment, and the final effect is currently largely unknown.
Many heavy metals (As, Cd, Cr, Hg, and Pb) have never been tested yet as potential human thyroid carcinogens. Studies have highlighted negative associations between thyroid volume and Cr, Se, and Zn contents in the hair samples of children. In the meantime, a link between thyroid volume and Pb and Mn concentrations has been proposed, presuming that these metals may interfere with the thyroid gland either directly or indirectly by influencing iodine intake or thyroid hormone and TSH levels (58, 59).
Volcanic activity is also related to an increase in environmental Radon concentrations, although a specific association of thyroid cancer with Radon levels has never been put forward (60). Belonging to the same ethnic group was also demystified as a cause of the increased incidence. In fact, people from volcanic islands residing in non-volcanic areas were unaffected (61).
Thus, studies to date regarding the role of environmental pollution with respect to thyroid function are still scarce, especially in childhood. Therefore, further studies are needed, investigating both the correlation in different age groups and studies to identify the pathogenetic mechanisms by which pollutants affect the thyroid gland.
4 The effects of endocrine-disrupting chemicals on thyroid function
EDCs can enter the environment through manufacturing processes, agricultural practices, waste disposal, etc. Contamination can occur through skin contact, food digestion, inhalation of contaminated products, or by vertical transmission via the placenta. EDCs exert their effects through multiple mechanisms. Some chemicals can bind to hormone receptors in the body, either activating or inhibiting their function. Others can interfere with hormone production, transport, metabolism, or clearance. In addition, they may have other modes of action, as causing oxidative stress, genetic susceptibility, and epigenetic modifications (e.g., DNA methylation, miRNA) (17, 62, 63), ultimately resulting in gene expression changes (62, 64, 65) in particular when exposure occurs during prenatal and early postnatal life.
Specifically, some studies have demonstrated that EDCs such as bisphenol A (BPA), di-2-ethylhexyl phthalate (DEHP), dibutyl phthalate (DBP), and pesticides exert some of their endocrine-disrupting activity by influencing methylation patterns. Furthermore, polychlorinated biphenyls (PCBs), BPA, and phthalates have been found to induce or inhibit the expression of other epigenetic regulating systems, as the miRNA network. Tributyltin and polycyclic aromatic hydrocarbons (PAHs) bind to Peroxisome proliferator-activated receptors (PPARs), modifying both its methylation status and the expression of its target genes, ultimately affecting insulin sensitivity. Furthermore, exposure to all of these EDCs can impact the expression and enzymatic activity of DNA methyltransferases, potentially interfering with enzymes like aromatase (65), ultimately modifying estrogen and androgen content. Importantly, changes to the epigenome induced by exposure to EDCs may have transgenerational effects, influencing health and disease across multiple generations even after the initial exposure has occurred (63–65).
The potential impact of EDCs on thyroid hormone regulation in children is of particular concern as thyroid hormones play a crucial role in the growth and central nervous system development, and control the overall physiological homeostasis (64). The fetal thyroid gland is underdeveloped during the first half of pregnancy, relying exclusively on maternal thyroid hormones, particularly T4, which crosses the placenta, thus, normal brain development requires a perfect thyroid hormone balance. Moreover, congenital hypothyroidism, or changes in thyroid hormone levels during pregnancy and at birth, can cause permanent neurodevelopmental disorders in infants (64, 66, 67).
In recent years, there has been increasing evidence of the effects of EDCs exposure on thyroid hormone levels from animal experiments and epidemiological studies. Structural similarities have been shown between some EDCs and thyroid hormones (THs) (64, 68, 69), and it has been proven that they can disrupt the THs synthesis, release, action on target tissues, and transportation by displacing the hormones from their binding proteins or by changing the content/activity of transporters. These EDCs include bisphenol A (BPA), phthalates, perchlorate, dioxin, polychlorinated biphenyls (PCBs), polybrominated diphenyl ethers (PBDEs), organophosphate pesticides (OPs), per- and polyfluoroalkyl substances (PFAS), and triclosan (20, 68). They can dysregulate the HPT axis, potentially leading to the development of thyroid gland hypertrophy and hyperplasia, hypothyroidism or hyperthyroidism, and even thyroid cancer (20, 64, 69, 70). The known effects of single EDCs on thyroid function are reported in Figure 2.
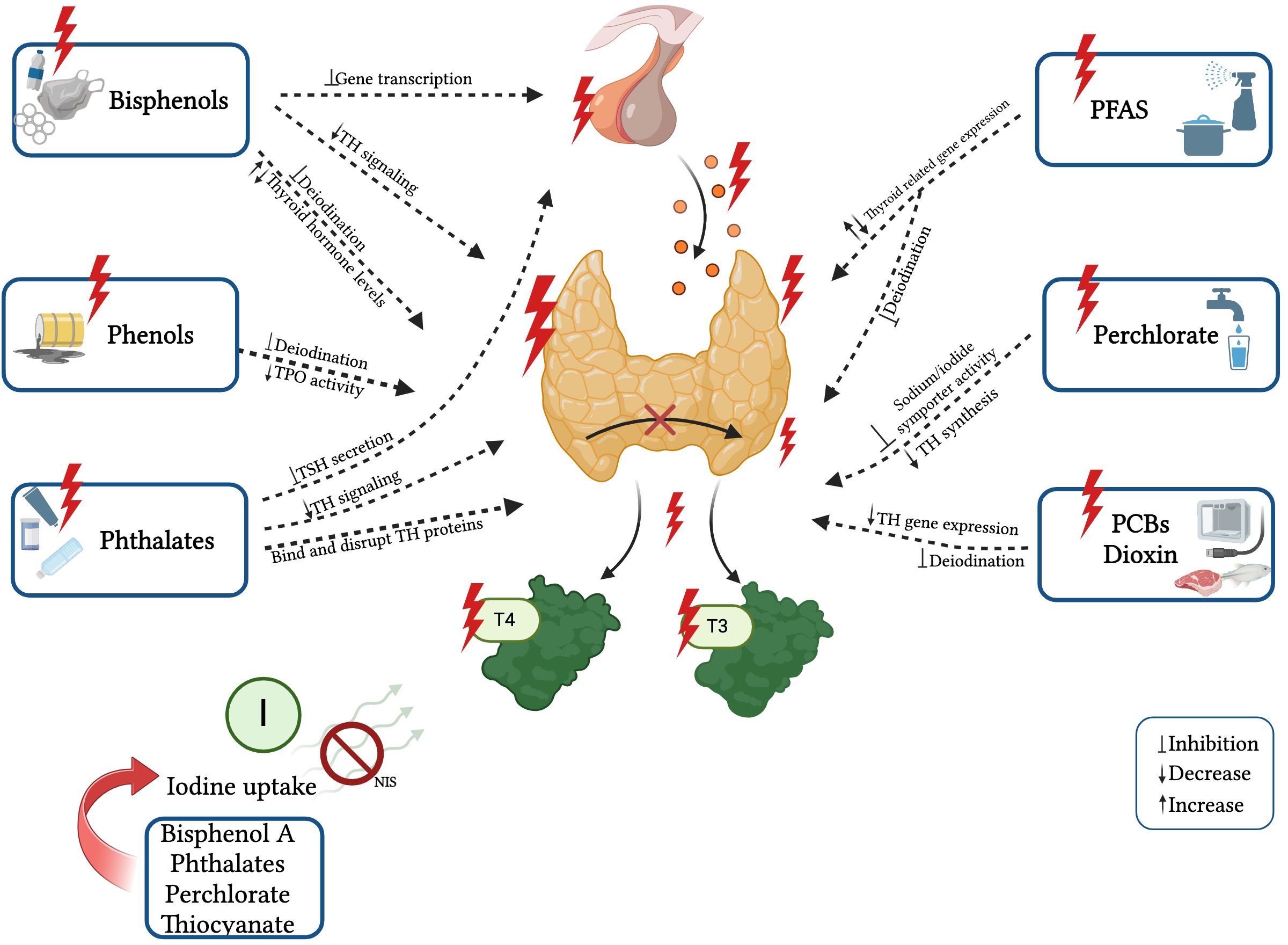
Figure 2 Known effects and mechanisms of action of single EDCs on thyroid function. Created with BioRender.com.
4.1 The effects of bisphenols and phthalate metabolites on thyroid function
Bisphenol A and phthalates that are prevalent in plastics and personal care products have been correlated with fluctuations in thyroid hormone levels, disturbances in thyroid-stimulating hormone (TSH) regulation, and with hindered development of the thyroid gland (20, 64, 66, 71). BPA, phthalates, perchlorate, and thiocyanate decrease thyroidal iodine uptake by competitively inhibiting the sodium-iodide symporter (NIS) (64, 72, 73). Moreover, bisphenols can modify thyroid hormone action by antagonizing thyroid receptor action, altering gene expression, and mimicking thyroid transport proteins (17, 74).
Chao Xiong et al. reported that maternal BPA exposure during the first trimester of pregnancy was associated with higher neonatal TSH levels and that BPS exposure throughout pregnancy was also associated with TSH levels in newborns. In addition, co-exposure to both bisphenols during the first trimester of pregnancy was significantly associated with neonatal TSH levels, and females might be more susceptible (75). A further prospective cohort study defined the impact of maternal exposure to bisphenols (BPs) on offspring’s thyroid hormone levels in cord blood; BPA exposure was associated with lower total thyroxine (TT4) and free triiodothyronine (fT3) concentrations, BPS exposure with lower TSH in boys, and BPAF exposure with higher TT3 and fT3, particularly in girls. The exposure to a mixture of BPs was associated with higher TT3 levels and fT3 in both sexes. The mechanisms involved and affected by these bisphenols are represented by transcriptional changes in thyroid hormone synthesis genes and changes in related gene transcription, and impaired iodine uptake, affecting overall thyroid function (76). The most affected genes were thyroid hormone synthesis genes such as SLC5A5 and Thyroid Peroxidase (TPO) genes, and transcription genes PAX8, FOXE1, and NKX2–1 (77).
Thyroid volume was described to be increased with age after BPA exposure in 718 Chinese, 9–11 year-old children, and the incidence of multiple thyroid nodules also increased with age (78). The longitudinal SMBCS study found that higher maternal urinary BPA levels during pregnancy were associated with increased fT4 levels in cord serum and predicted a high risk of behavioral difficulties in children at 10 years of age, particularly in boys (79).
When considering phthalates, exposure to di-(2-ethylhexyl) phthalate (DEHP) metabolites in rats was mainly associated with altered pituitary TSH secretion and disrupted thyroid hormone homeostasis with changes in receptor expression levels (80). Furthermore, in vivo studies have shown that the complex effects of DEHP and BPA mixtures may lead to reduced thyroid weight, which ultimately would affect the development of the thyroid gland (81). In humans, instead, some studies suggest that individual maternal urinary phthalate metabolite concentrations would have no effect on cord serum TSH levels (72, 82), but the data are controversial. Huang et al. reported that maternal phthalate levels in the second trimester of pregnancy, were positively associated with T3 and fT4 levels in cord serum (83). Moreover, phthalates antagonize the binding of T3 to thyroid receptor-β, limiting T3 cell uptake, and disrupting sodium-iodine transporter transcription, lowering thyroxine (T4) and T3 levels in pregnant women and children (84, 85).
The Columbia Center study showed that maternal urinary mono-(2-ethylhexyl) phthalate (MEHP) levels were positively linked with fT4 in children at age 3 years (24). Moreover, there is a link between early childhood phthalate exposure and thyroid function from 6 to 12 years of age. Two further recent studies have shown, that phthalate exposure was associated with decreased TSH and increased T3 in children (85, 86). Researchers have also described an overall positive association of phthalate mixtures with TT3 serum levels in adolescents (85, 87, 88).
A large US study in 356 adolescents aged 12–19 years showed a positive correlation between TT3 and TSH with mono‐(carboxyisoctyl) phthalate (MCOP) and a negative correlation of TSH with MCNP. Moreover, elevated levels of phthalates in pubertal boys and girls were associated with higher DNA methylation levels at the thyroid hormone receptor interactor 6 (TRIP6) promoter region, a gene associated with pubertal onset (87, 88). A further recent longitudinal study of 166 children described a positive relationship between mono (2-ethyl-5-hydroxyhexyl) phthalate (MEHHP) and fT4, and a negative relationship between mono-methyl phthalate (MMP) and T3, T4, and fT4 serum levels (89). Besides, DEHP metabolites have been reported to be positively associated with TT3, and MCPP to be negatively associated with fT4 and TT4 in teenagers aged 12 to 19 years of age (88).
4.2 Effects of perchlorate, dioxin and persistent organic pollutants on thyroid function
Perchlorate, commonly present in both drinking water and specific food items, competitively hinders the absorption of iodine by the thyroid gland, subsequently reducing the synthesis of thyroid hormones, and has been related with decreased cognitive function in offspring (89).
Prenatal exposure to dioxin (TCDD exposure) has been reported to be associated with changes in thyroid function in 2-year-old children born to women living in Seveso where an explosion that occurred 40 years ago has yet health implications, and lower fT3 and fT4 levels are observed in the children born to these mothers compared with the general Italian population (90). In 2 to 5-year-old children, prenatal dioxin exposure can increase TSH and fT4 levels, which were found especially in males (91).
Persistent organic pollutants such as PBDEs and PCBs showed associations with reduced serum thyroid hormone levels, altered expression of TH-responsive genes, modifications in thyroid hormone-binding proteins, and an elevated likelihood of hypothyroidism (20, 64, 66, 71).
In the neonatal period, prenatal exposure to dioxin-like contaminants has been described to be associated with higher neonatal free thyroxine (fT4), especially in boys. Researchers didn’t find any significant relationship between total PCBs or non-dioxin-like PCBs exposure and any maternal or neonatal thyroid hormone, implying that non-accidental PCB exposure caused thyroid damage primarily through dioxin-like action (89).
4.3 Effects of exposure to organophosphate pesticides, per- and poly-fluoroalkyl substances, on thyroid function
Organophosphate pesticides decrease thyroid hormone levels and modify thyroid hormone metabolism. Experimental in vivo studies have shown that the changes in TH levels cause decreased circulating transthyretin, affecting the hepatic metabolism of T4, inducing histological changes in the thyroid gland, and reducing brain weight in the offspring (20, 66, 71, 92).
Per- and poly-fluoroalkyl substances (PFAS) have been shown to reduce circulating thyroid hormone levels by interfering with intracellular signaling cascades, like mitogen-activated protein kinase (MAPK) pathway, which can alter thyroid hormone production, secretion, and cellular responses (93). Thyroid hormone levels can be modified by PFAS exposure both prenatally and postnatally, and higher PFAS mixture concentrations in babies have been found to be associated with lower total thyroxine levels (74, 94), but not with TSH levels in neonates (95). The flame retardant DE-71 (a mixture of polybrominated diphenyl ethers) has been reported to inhibit human differentiated thyroid cell function in vitro, to inhibit Thyroid globulin (Tg)-release from TSH-stimulated thyrocytes, and to inhibit the expression of mRNA encoding for Tg, TPO, and TSHr (96). A comprehensive meta-analysis from thirty-two cohort studies has investigated the relations between prenatal exposure to organochlorine, PFAS, and other EDCs, levels of maternal thyroid hormones during pregnancy, and neonatal thyroid hormone levels describing negative associations of organochlorine and PFAS exposure and neonatal TT4 levels, suggesting potential adverse effects (66, 97); the authors hypothesized a change in the balance between thyroid hormone biosynthesis and elimination (97) an induction of microsomal enzymes as uridine diphosphate glucuronosyltransferase, and an increased T4 to T3 conversion by inducing deiodinase (98, 99).
4.4 Effect of exposure to other EDCs on thyroid function
Thyroid peroxidase (TPO) activity can be inhibited by thiocyanates and phenols as they affect the conversion of iodide to iodine and subsequently coupling processes inhibiting the production of thyroid hormones and causing altered thyroid homeostasis (70, 73). Phenols, especially in baby girls, are associated with TSH levels, but not with total TT3 and fT4 during the pregnancy and at birth. This leads to the hypothesis that genetic variants in the Iodothyronine Deiodinase 1 (DIO1) and Iodothyronine Deiodinase 2 (DIO2) genes can modulate these associations (100). Prenatal triclosan exposure was found to affect Thyroid Peroxidase Antibodies (TPOAb) levels in three-year-old children, resulting in 328% TPOAb higher levels (101). Notably, populations with high thyroid antibody status appear to exhibit increased susceptibility to the effects of in-utero exposure to thyroid-disrupting chemicals, emphasizing the potential long-term impact of such exposures on thyroid health (90).
Interestingly, phytoestrogens, which also act as EDCs, have an influence on thyroid hormones in adolescents as well. Yun Fan et al. reported that elevated levels of the urinary phytoestrogen enterolactone (ENT) were positively associated with TSH levels in 12–19-year-old girls and with TSH and TT3 in this age category in boys. Moreover, equol (EQU) levels were negatively associated with TT4 in both sexes. So, we need to monitor phytoestrogen intake via meals as well (102).
Finally, some effects are sex-specific, as previously described for bisphenols, phthalates, and PCBs in boys (76, 79, 89), and as for triclosan, parabens, and OH-MPHP, negatively associated with T4, in girls (103).
It should also be mentioned that in the near future machine learning will be of great help to identify chemicals that interfere with the endocrine system. A recent study has highlighted using these techniques a few new chemicals predicted to interfere with the TSH receptor (104).
The currently known effects of single EDCs on thyroid function are reported in Table 1.
5 Effects of diet, nutrition, food pollution and microplastics on thyroid function
The relationship between diet and thyroid health plays a crucial role in maintaining hormonal balance and preventing thyroid dysfunctions. A nutrient-rich dietary style, including essential elements such as iodine, selenium, iron and vitamin D, can promote the proper synthesis of thyroid hormones and regulate their activity. Moreover, there are currently no dedicated nutritional recommendations for patients with thyroid diseases. Existing guidance relies solely on the opinions and advice of individual specialists, often providing incomplete and sometimes contradictory information. Recent studies have demonstrated that high consumption of animal fats can induce an increase in the production of thyroid autoantibodies (105). The saturated fatty acids found in animal fats can indeed trigger a pro-inflammatory response through the activation of cytokine transcription. Studies in rats have confirmed that excessive consumption of animal fats determines thyroid dysfunction and may contribute to the pathogenesis of hypothyroidism (106). A study conducted by Ruggeri et al. on euthyroid patients with Hashimoto’s disease showed that markers of oxidative stress (evaluated through advanced glycation end-products - AGEs) were significantly higher in patients compared to controls, and the activity of the enzymes glutathione peroxidase and thioredoxin reductase, as well as plasma antioxidant activity, was reduced in the patients compared to controls. When evaluating the dietary habits of the enrolled cohort, it emerged that patients consumed animal-derived foods (meat, fish, and dairy) more frequently compared to the control group, which preferred a diet richer in plant-based foods (legumes, fruits, vegetables) (107). This suggests the positive influence of a low intake of animal foods on the oxidative-antioxidant balance. An anti-inflammatory diet, rich in vitamins, minerals, polyphenols, and phytosterols, seemed to be able to reduce the circulating levels of thyroid autoantibodies (108). Natural antioxidants as vitamins A, C and E are found mostly in plant-based products, including a wide variety of fruits and vegetables. These studies highlighted the importance of recommending a Mediterranean diet as an adjuvant in the treatment and prevention of thyroid autoimmunity, thanks to its antioxidant properties (107, 108).
Diet can also influence the composition of the microbiota. Several studies have illustrated the change in microbiota composition following dietary modifications. The intestinal bacterial flora plays a crucial role in maintaining metabolic, nutritional, and even immunological homeostasis (109). The hypothesis of a thyroid-gut axis is becoming increasingly concrete. In addition to influencing the absorption of minerals important for thyroid function (iodine, selenium, zinc, and iron), the microbiome is involved in endogenous and exogenous thyroid hormone metabolism. The presence of iodothyronine deiodinase, an enzyme that converts T4 into its active T3 form or into reverse T3 (rT3), has been found in the intestinal wall, thus influencing total body T3 levels (110). A review by our group reported how the microbiota plays a role in metabolizing endocrine disruptors introduced through diet, by means of a bidirectional interaction that leads to dysbiosis and changes in pathways involved in the development of various metabolic diseases (63). Dysbiosis and the subsequent negative influence on the immune system and intestinal permeability can promote the development of inflammatory and autoimmune diseases, including thyroid diseases (110–112).
Food pollution may modify these factors, which will, in turn, affect thyroid function.
As we described, food may be exposed to endocrine-disrupting chemicals through pesticides, fertilizers, canned products, and plastic particles that exhaust and alter thyroid function (17). Ultra-processed food is an industrially manufactured product taken from natural foods or synthesized from other organic components (113). Its consumption could also change thyroid function. A unique prospective cohort study by Zhang et al. found that ultra-processed food consumption was associated with higher risk of subclinical thyroid dysfunction in adults (114).
Another concern is related to the increasing global prevalence of nitrate and nitrite accumulation in vegetables and fruits owe to climate changes (29). As nitrates and nitrites in the human body undergo metabolic conversion into nitric oxide, higher levels can disrupt thyroid function. Nitrate can competitively interfere with iodine uptake by the thyroid gland and influence hormonal synthesis. Moreover, meat consumption with higher levels of nitrates/nitrites can stimulate the synthesis of endogenous N-nitroso compounds. Both nitrosamines and N-nitroso compounds have carcinogenic effects (42, 115). A meta-analysis concluded that a dietary high nitrate intake was connected with an increased risk of thyroid cancer (odds ratio [OR] = 1.40, 95% confidence intervals [CI]: 1.02, 1.77) (116).
Finally, the accumulation of nano- and microplastics in meat and fish can lead to chronic exposure and accumulation of these in tissues. It is hypothesized that microplastics may disrupt the epithelial barrier and alter immunological responses (63). Animal studies have described that chronic exposure to polystyrene nanoplastics inhibits serum T3 and circulating levels of thyroid hormones, which significantly elevates TSH (117).
Additionally, contaminated seafood and seafood products can contain heavy metals like mercury, which are bioaccumulated in the thyroid cells and can contribute to the development of autoimmune thyroiditis, hypothyroidism, and thyroid cancer (118).
6 The effect of infectious agents on thyroid function: lessons from the COVID-19 pandemic
The risk of infections and their effects represents one of the biggest environmental threats and planetary emergencies to date, as shown by the COVID-19 pandemic.
Since December 2019, the Coronavirus Disease 2019 (COVID-19), caused by the etiologic agent Severe Acute Respiratory Syndrome Coronavirus-2 (SARS-CoV-2), has been the cause of high mortality and morbidity all over the world. At the end of May 2023, over 767 million human infections and over 6.9 million deaths have been reported worldwide (119).
The pediatric population, instead, since the beginning of the pandemic, has been less affected than adults, with a global milder clinical presentation and low rates of hospitalization and death. However, rare and severe complications may also develop in children, with a systemic involvement due to a multisystem inflammatory syndrome (MIS-C) (120).
SARS-CoV-2 is an enveloped RNA virus that belongs to the Coronaviridae family (Nidovirales order). Its genome is a single-stranded positive-sense RNA that contains four structural proteins: spike protein (S), membrane protein (M), nucleocapsid protein (N) and envelope protein (E) (121). The spike protein is a viral surface protein and consists of two subunits (S1 and S2). It is involved in the viral attachment and in the host cell entry: the S1 subunit is able to bind the angiotensin-converting enzyme 2 (ACE2) receptor while, in a second step, the S2 subunit allows the virus to enter the cell by membrane fusion (122).
ACE2 is widespread in different organs, including the respiratory and gastrointestinal tract, the brain, the kidneys, the heart, and vessels, and this is the reason why, although the main viral target still remains the respiratory epithelium, SARS-CoV-2 can potentially infect multiple tissues (123). Moreover, ACE2 is also expressed in several endocrine tissues, such as the ovaries, the testis but also the hypothalamus, the pituitary, and the thyroid gland, with a consequent possible impact of the infection on endocrine function (124). SARS-CoV-2 can attack, in fact, thyroid follicular cells via ACE2 with possible consequences on thyroid function (125). In addition, besides the direct invasion of the thyroid tissue, indirect mechanisms can also alter thyroid function. First, both the hypothalamus and the pituitary gland, which regulate the thyroid axis, express ACE2 receptors, being additional targets of the viral infection. Second, it is worth noticing that thyroid hormones are strongly linked to the regulation of the immune system (126). Patients with severe forms of COVID-19 infection characterized by a hyperinflammatory state due to the cytokine storm may develop an acute hypothalamus/pituitary/thyroid axis dysfunction that may cause both acute hypothyroidism and immune-mediated damage (127).
In critical patients, euthyroid sick syndrome or non-thyroidal illness syndrome, characterized by decreased fT3 but normal TSH levels, has been described as probably due to the abnormal hyperinflammatory state: in fact, in most cases, this condition was transient and resolved over time (128, 129).
Moreover, a certain increase in incidence of hypo- and hyperthyroidism as de-novo diagnosis or exacerbations of pre-existing disorders were described, thus, the indication of routine evaluation of thyroid function in infected patients, in particular in those presenting severe forms of COVID-19 disease was recommended (130, 131).
Considering specifically the paediatric population, it is important to remark that there is still a considerable lack of information on the impact of COVID-19 disease on thyroid function, and all the evidence described above derives from studies conducted on adult patients. In fact, since the beginning of the pandemic, only about 17.9% of the total registered cases concerned children, partially explaining this current lack of data; moreover, children rarely develop severe disease with the hyperinflammatory response, thus protecting them from thyroid acute dysfunctions (132).
Later in the course of the pandemic, after the beginning of the vaccination program, an increasing number of cases of possible alterations in thyroid function after the administration of COVID-19 vaccines were reported, in parallel with reassuring data on global safety and efficacy.
The most common suspected adverse event reported was subacute thyroiditis, followed by Graves disease with other incidental reports of painful thyroiditis, silent thyroiditis, concurrent Graves disease and subacute thyroiditis, thyroid eye disease, overt hypothyroidism, atypical subacute thyroiditis, and painless thyroiditis with thyrotoxic periodic paralysis (133).
Recently, new data on the possible correlation between COVID-19 vaccines and thyroid inflammatory disease have been reported with the confirmation that subacute thyroiditis may be considered an uncommon complication that may follow COVID-19 vaccination. Interestingly, as described by Şendur et al., this adverse effect tends to affect young and middle-aged adult women with a certain genetic background. Conversely, to date, no cases involving children or adolescents have yet been reported (134).
It is worth noticing that the types of COVID-19 vaccinations that most frequently cause adverse effects on thyroid function are mRNA-based vaccines, followed by adenovirus-vectored SARS-CoV-2 vaccines and inactivated vaccines (134). Considering that most of the reported post-vaccine thyroid complications had a favorable outcome with resolution after treatment, the benefits of COVID-19 vaccinations clearly still prevail over the possible development of any thyroid disease.
7 Conclusions and future directions
Many environmental factors can influence the development of the thyroid gland and its function throughout life, and there is a need for monitoring and further understanding.
Air and water pollutants, climate change, endocrine-disrupting chemicals, nutrients and food pollutants, and infectious agents can all have a significant effect on thyroid cancer, but current data are still scarce. In particular, we lack information on determinants during childhood and have limited knowledge and understanding of many pollutant mechanisms of action.
The effects of Endocrine Disruptors and thyroid function are currently the most studied among the factors described above, and both epidemiological data and studies of mechanisms of action are available but are yet insufficient and have not covered all ages and critical windows of exposure.
There is a lack of studies, in particular, in the prenatal and perinatal periods, notwithstanding the global attention on the first 1,000 days of life, as in-utero exposure to contaminants is known to determine epigenetic changes with transgenerational effects, and to be associated with an increase in non-communicable diseases in later life.
The collective evidence from various studies underscores the intricate relationship between endocrine-disrupting chemicals and thyroid function in children. The complex interplay between EDCs and thyroid function involves many mechanisms. Even minor changes in thyroid hormone functions, especially during critical developmental periods, may have lasting health effects, particularly on neurocognitive development. Therefore, understanding the association between EDCs and thyroid disease in childhood is crucial for developing preventive strategies, promoting regulatory measures, and minimizing exposure to these chemicals, ultimately safeguarding thyroid health.
Infectious diseases currently represent a planetary emergency among others, and many can affect thyroid function; in particular, it has been demonstrated that the SARS-CoV-2 infection is a possible cause of thyroid inflammation and can trigger autoimmune processes in adults. COVID-19 vaccination has been supposed to be able to induce autoimmune thyroiditis in a few young and middle-aged adult women, although the overall advantages of the vaccination still override these possible risks. Data in children and newborns are still lacking.
Future studies should focus on the role of all environmental factors on thyroid function starting from pregnancy, as these will have effects throughout life.
Author contributions
MS: Conceptualization, Writing – review & editing, Data curation, Formal Analysis, Methodology, Supervision, Writing – original draft. AS: Conceptualization, Investigation, Visualization, Writing – original draft, Writing – review & editing. MP: Data curation, Writing – review & editing. VP: Formal Analysis, Writing – original draft, Writing – review & editing. VD: Investigation, Writing – original draft. AG: Writing – original draft. MG: Investigation, Writing – original draft. MM: Writing – original draft, Investigation. AM: Investigation, Writing – original draft. RR: Investigation, Writing – original draft, Writing – review & editing. SB: Writing – review & editing. LI: Formal Analysis, Writing – review & editing. SE: Writing – review & editing. BP: Supervision, Writing – review & editing.
Funding
The author(s) declare that no financial support was received for the research, authorship, and/or publication of this article.
Conflict of interest
The authors declare that the research was conducted in the absence of any commercial or financial relationships that could be construed as a potential conflict of interest.
The author(s) declared that they were an editorial board member of Frontiers, at the time of submission. This had no impact on the peer review process and the final decision.
Publisher’s note
All claims expressed in this article are solely those of the authors and do not necessarily represent those of their affiliated organizations, or those of the publisher, the editors and the reviewers. Any product that may be evaluated in this article, or claim that may be made by its manufacturer, is not guaranteed or endorsed by the publisher.
References
1. Pirahanchi Y, Toro F, Jialal I. Physiology, Thyroid Stimulating Hormone. (2023) May 1. Treasure Island (FL: StatPearls Publishing (2024).
2. Mohebati A, Shaha AR. Anatomy of thyroid and parathyroid glands and neurovascular relations. Clin Anat. (2012) 25:19–31. doi: 10.1002/ca.21220
3. Bílek R, Dvořáková M, Grimmichová T, Jiskra J. Iodine, thyroglobulin and thyroid gland. Physiol Res. (2020) 69:S225–36. doi: 10.33549/physiolres.934514
4. van Trotsenburg P, Stoupa A, Léger J, Rohrer T, Peters C, Fugazzola L, et al. Congenital hypothyroidism: A 2020–2021 consensus guidelines update-an ENDO-European reference network initiative endorsed by the European society for pediatric endocrinology and the european society for endocrinology. Thyroid. (2021) 31:387–419. doi: 10.1089/thy.2020.0333
5. Chapman AK, Farmer ZJ, Mastrandrea LD, Matlock KA. Neonatal thyroid function and disorders. Clin Obstet Gynecol. (2019) 62:373–87. doi: 10.1097/GRF.0000000000000434
6. Penta L, Cofini M, Lanciotti L, Leonardi A, Principi N, Esposito S. Hashimoto’s disease and thyroid cancer in children: Are they associated? Front Endocrinol (Lausanne). (2018) 9:565. doi: 10.3389/fendo.2018.00565
7. Dimachkieh AL, Kazahaya K, Chelius DC Jr. Assessment and management of thyroid disease in children. Otolaryngol Clin North Am. (2019) 52:957–67. doi: 10.1016/j.otc.2019.06.009
8. Wassner AJ. Pediatric hypothyroidism: diagnosis and treatment. Paediatr Drugs. (2017) 19:291–301. doi: 10.1007/s40272–017-0238–0
9. Paulson VA, Rudzinski ER, Hawkins DS. Thyroid cancer in the pediatric population. Genes (Basel). (2019) 10:723. doi: 10.3390/genes10090723
10. Wang L, Wang FS, Gershwin ME. Human autoimmune diseases: a comprehensive update. J Intern Med. (2015) 278:369–95. doi: 10.1111/joim.12395
11. Taylor PN, Albrecht D, Scholz A, Gutierrez-Buey G, Lazarus JH, Dayan CM, et al. Global epidemiology of hyperthyroidism and hypothyroidism. Nat Rev Endocrinol. (2018) 14:301–16. doi: 10.1038/nrendo.2018.18
12. Gómez-Roig MD, Pascal R, Cahuana MJ, García-Algar O, Sebastiani G, Andreu-Fernández V, et al. Environmental exposure during pregnancy: Influence on prenatal development and early life: A comprehensive review. Fetal Diagn Ther. (2021) 48:245–57. doi: 10.1159/000514884
13. WHO global air quality guidelines: particulate matter (PM2.5 and PM10), ozone, nitrogen dioxide, sulfur dioxide and carbon monoxide. WHO. World Health Organization. Available online at: https://www.who.int/publications/i/item/9789240034228 (Accessed September 15, 2023).
14. Bergman A. United Nations Environment Programme., & World Health Organization. State of the science of endocrine disrupting chemicals - 2012 an assessment of the state of the science of endocrine disruptors (2013) (Accessed September 15, 2023).
15. ECHA/EFSA. Guidance for the identification of endocrine disruptors in the context of Regulations (EU) No 528/2012 and (EC) No 1107/2009. EFSA J. (2018) 16:5311.
16. Lauretta R, Sansone A, Sansone M, Romanelli F, Appetecchia M. Endocrine disrupting chemicals: Effects on endocrine glands. Front Endocrinol (Lausanne). (2019) 10:178. doi: 10.3389/fendo.2019.00178
17. Benvenga S, Elia G, Ragusa F, Paparo SR, Sturniolo MM, Ferrari SM, et al. Endocrine disruptors and thyroid autoimmunity. Best Pract Res Clin Endocrinol Metab. (2020) 34:101377. doi: 10.1016/j.beem.2020.101377
18. Calsolaro V, Pasqualetti G, Niccolai F, Caraccio N, Monzani F. Thyroid disrupting chemicals. Int J Mol Sci. (2017) 18:2583. doi: 10.3390/ijms18122583
19. Guide to Environmental Issues: Glossary of Terms & Acronyms. US Environmental Protection Agency (EPA), USA. Available online at: https://sor.epa.gov/sor_internet/registry/termreg/searchandretrieve/glossariesandkeywordlists/search.do;jsessionid=zd-ZzuSl3XF96MLglmD0ZwM_ofe4UVef4GQEb2dCia7n1u2tV7AH!-1582707454?details=&vocabName=Environmental%20Issues%20Glossary&filterTerm=pollution&checkedAcronym=false&checkedTerm=false&hasDefinitions=false&filterTerm=pollution&filterMatchCriteria=Contains (Accessed September 15, 3023).
20. Directive 2008/50/EC of the European Parliament and of the Council of 21 May 2008 on ambient air quality and cleaner air for Europe. Available online at: https://eur-lex.europa.eu/eli/dir/2008/50/2015–09-18 (Accessed September 29, 2023).
21. Zendehbad M, Mostaghelchi M, Mojganfar M, Cepuder P, Loiskandl W. Nitrate in groundwater and agricultural products: intake and risk assessment in northeastern Iran. Environ Sci pollut Res Int. (2022) 29:78603–19. doi: 10.1007/s11356–022-20831–9
22. Vareda JP, Valente AJM, Durães L. Assessment of heavy metal pollution from anthropogenic activities and remediation strategies: A review. J Environ Manage. (2019) 246:101–18. doi: 10.1016/j.jenvman.2019.05.126
23. Vigneri R, Malandrino P, Gianì F, Russo M, Vigneri P. Heavy metals in the volcanic environment and thyroid cancer. Mol Cell Endocrinol. (2017) 457:73–80. doi: 10.1016/j.mce.2016.10.027
24. Miller MD, Marty MA, Landrigan PJ. Children’s environmental health: Beyond national boundaries. Pediatr Clin North Am. (2016) 63:149–65. doi: 10.1016/j.pcl.2015.08.008
25. Bernasconi S, Levy C, Cohen R, Giardino I, Pettoello-Mantovani M, Somekh E. Climate change and environmental pollution induced risks on children's health: Are pediatricians prepared to meet the challenge? J Pediatr. (2021) 238:346–347.e2. doi: 10.1016/j.jpeds.2021.08.001
26. Zeng Y, He H, Wang X, Zhang M, An Z. Climate and air pollution exposure are associated with thyroid function parameters: a retrospective cross-sectional study. J Endocrinol Invest. (2021) 44:1515–23. doi: 10.1007/s40618–020-01461–9
27. Hosseini MJ, Dezhangah S, Esmi F, S Gharavi-Nakhjavani M, Hashempour-Baltork F, Mirza Alizadeh A. A worldwide systematic review, meta-analysis and meta-regression of nitrate and nitrite in vegetables and fruits. Ecotoxicol Environ Saf. (2023) 257:114934. doi: 10.1016/j.ecoenv.2023.114934
28. IARC. Outdoor air pollution a leading environmental cause of cancer deaths (2023). Available online at: https://www.iarc.who.int/wp-content/uploads/2018/07/pr221E.pdf (Accessed October 16, 2023).
29. Corella JP, Maffezzoli N, Spolaor A, Vallelonga P, Cuevas CA, Scoto F, et al. Climate changes modulated the history of Arctic iodine during the Last Glacial Cycle. Nat Commun. (2022) 13:88. doi: 10.1038/s41467–021-27642–5
30. Jones GD, Droz B, Greve P, Gottschalk P, Poffet D, McGrath SP, et al. Selenium deficiency risk predicted to increase under future climate change. Proc Natl Acad Sci USA. (2017) 114:2848–53. doi: 10.1073/pnas.1611576114
31. Janssen BG, Saenen ND, Roels HA, Madhloum N, Gyselaers W, Lefebvre W, et al. Fetal thyroid function, birth weight, and in utero exposure to fine particle air pollution: A birth cohort study. Environ Health Perspect. (2017) 125:699–705. doi: 10.1289/EHP508
32. Howe CG, Eckel SP, Habre R, Girguis MS, Gao L, Lurmann FW, et al. Association of prenatal exposure to ambient and traffic-related air pollution with newborn thyroid function: Findings from the children's health study. JAMA Netw Open. (2018) 1:e182172. doi: 10.1001/jamanetworkopen.2018.2172
33. Irizar A, Txintxurreta A, Molinuevo A, Jimeno-Romero A, Anabitarte A, Álvarez JI, et al. Association between prenatal exposure to air pollutants and newborn thyroxine (T4) levels. Environ Res. (2021) 197:111132. doi: 10.1016/j.envres.2021.111132
34. Ekins R. Analytic measurements of free thyroxine. Clin Lab Med. (1993) 13:599–630. doi: 10.1016/S0272–2712(18)30428–1
35. Valdés S, Doulatram-Gamgaram V, Maldonado-Araque C, Lago-Sampedro A, García-Escobar E, García-Serrano S, et al. Ambient air pollution and thyroid function in Spanish adults. A nationwide population-based study (Di@bet.es study). Environ Health. (2022) 21:76. doi: 10.1186/s12940–022-00889–1
36. Heo YJ, Kim HS. Ambient air pollution and endocrinologic disorders in childhood. Ann Pediatr Endocrinol Metab. (2021) 26:158–70. doi: 10.6065/apem.2142132.066
37. Qi C, Shang L, Yang W, Huang L, Yang L, Xin J, et al. Maternal exposure to O3 and NO2 may increase the risk of newborn congenital hypothyroidism: a national data-based analysis in China. Environ Sci pollut Res Int. (2021) 28:34621–9. doi: 10.1007/s11356–021-13083–6
38. WHO. Nitrate and nitrite in drinking-water: Background document for development of WHO Guidelines for Drinking-water Quality. (WHO/SDE/WSH/07.01/16/Rev/1). World Health Organization. (2011) (Geneva, Switzerland: World Health Organization). p. 41.
39. OEHHA. OEHHA (California Office of Environmental Health Hazard Assessment). Nitrate and Nitrite in Drinking Water (2018). Available online at: https://oehha.ca.gov/media/downloads/water/chemicals/nitrateresponse081118.pdf (Accessed September 15, 3023).
40. Tajtakova M, Semanova Z, Tomkova Z, Szokeova E, Majoros J, Radikova Z, et al. Increased thyroid volume and frequency of thyroid disorders signs in schoolchildren from nitrate polluted area. Chemosphere. (2006) 62:559–64.
41. Drozd VM, Saenko VA, Brenner AV, Drozdovitch V, Pashkevich VI, Kudelsky AV, et al. Major factors affecting incidence of childhood thyroid cancer in Belarus after the chernobyl accident: Do nitrates in drinking water play a role? PLoS One. (2015) 10:e0137226. doi: 10.1371/journal.pone.0137226
42. Ward MH, Kilfoy BA, Weyer PJ, Anderson KE, Folsom AR, Cerhan JR. Nitrate intake and the risk of thyroid cancer and thyroid disease. Epidemiology. (2010) 21:389–95. doi: 10.1097/EDE.0b013e3181d6201d
43. Vaccarella S, Lortet-Tieulent J, Colombet M, Davies L, Stiller CA, Schüz J, et al. IICC-3 contributors. Global patterns and trends in incidence and mortality of thyroid cancer in children and adolescents: a population-based study. Lancet Diabetes Endocrinol. (2021) 9:144–52. doi: 10.1016/S2213–8587(20)30401–0
44. Stefan AI, Piciu A, Mester A, Apostu D, Badan M, Badulescu CI. Pediatric thyroid cancer in europe: An overdiagnosed condition? A literature review. Diagnostics (Basel). (2020) 10:112. doi: 10.3390/diagnostics10020112
45. Francis GL, Waguespack SG, Bauer AJ, Angelos P, Benvenga S, Cerutti JM, et al. American thyroid association guidelines task force. Management guidelines for children with thyroid nodules and differentiated thyroid cancer. Thyroid. (2015) 25:716–59. doi: 10.1089/thy.2014.0460
46. Mathews JD, Forsythe AV, Brady Z, Butler MW, Goergen SK, Byrnes GB, et al. Cancer risk in 680,000 people exposed to computed tomography scans in childhood or adolescence: data linkage study of 11 million Australians. BMJ. (2013) 346:f2360. doi: 10.1136/bmj.f2360
47. Drozd V, Saenko V, Branovan DI, Brown K, Yamashita S, Reiners C. A search for causes of rising incidence of differentiated thyroid cancer in children and adolescents after chernobyl and fukushima: comparison of the clinical features and their relevance for treatment and prognosis. Int J Environ Res Public Health. (2021) 18:3444. doi: 10.3390/ijerph18073444
48. UNITED NATIONS. Sources and Effects of Ionizing Radiation. Volume I: Report to the General Assembly, Scientific Annexes A and B. United Nations sales publications. E.10.XI.3 UNSCEAR 2008 Report (2011) ((UNSCEAR), United Nations, (UNSCEAR), New York). p. 179.
49. Marcello MA, Malandrino P, Almeida JF, Martins MB, Cunha LL, Bufalo NE, et al. The influence of the environment on the development of thyroid tumors: a new appraisal. Endocr Relat Cancer. (2014) 21:T235–54. doi: 10.1530/ERC-14–0131
50. Sadeghi H, Rafei M, Bahrami M, Haghdoost A, Shabani Y. Attributable risk fraction of four lifestyle risk factors of thyroid cancer: a meta-analysis. J Public Health (Oxf). (2018) 40:e91–8. doi: 10.1093/pubmed/fdx088
51. Pellegriti G, De Vathaire F, Scollo C, Attard M, Giordano C, Arena S, et al. Papillary thyroid cancer incidence in the volcanic area of Sicily. J Natl Cancer Inst. (2009) 101:1575–83. doi: 10.1093/jnci/djp354
52. Arnbjörnsson E, Arnbjörnsson A, Olafsson A. Thyroid cancer incidence in relation to volcanic activity. Arch Environ Health. (1986) 41:36–40. doi: 10.1080/00039896.1986.9935763
53. Lubin JH, Adams MJ, Shore R, Holmberg E, Schneider AB, Hawkins MM, et al. Thyroid cancer following childhood low-dose radiation exposure: A pooled analysis of nine cohorts. J Clin Endocrinol Metab. (2017) 102:2575–83. doi: 10.1210/jc.2016–3529
54. Journy N, Ancelet S, Rehel JL, Mezzarobba M, Aubert B, Laurier D, et al. Predicted cancer risks induced by computed tomography examinations during childhood, by a quantitative risk assessment approach. Radiat Environ Biophys. (2014) 53:39–54. doi: 10.1007/s00411–013-0491–8
55. Loomis D, Grosse Y, Lauby-Secretan B, El Ghissassi F, Bouvard V, Benbrahim-Tallaa L, et al. The carcinogenicity of outdoor air pollution. Lancet Oncol. (2013) 14:1262–3.
56. Santibáñez-Andrade M, Quezada-Maldonado EM, Osornio-Vargas Á, Sánchez-Pérez Y, García-Cuellar CM. Air pollution and genomic instability: the role of particulate matter in lung carcinogenesis. Environ pollut. (2017) 229:412–22.
57. Crepeau P, Zhang Z, Udyavar R, Morris-Wiseman L, Biswal S, Ramanathan M Jr, et al. Socioeconomic disparity in the association between fine particulate matter exposure and papillary thyroid cancer. Environ Health. (2023) 22:20. doi: 10.1186/s12940–023-00972–1
58. Yanagi Y, Assunção JV, Barrozo LV. The impact of atmospheric particulate matter on cancer incidence and mortality in the city of São Paulo, Brazil. Cad Saude Publica. (2012) 28:1737–48. doi: 10.1590/s0102–311x2012000900012
59. Cong X. Air pollution from industrial waste gas emissions is associated with cancer incidences in Shanghai, China. Environ Sci pollut Res Int. (2018) 25:13067–78. doi: 10.1007/s11356–018-1538–9
60. Park SJ, Min C, Yoo DM, Choi HG. National cohort and meteorological data based nested case-control study on the association between air pollution exposure and thyroid cancer. Sci Rep. (2021) 11:21562. doi: 10.1038/s41598–021-00882–7
61. Karzai S, Zhang Z, Sutton W, Prescott J, Segev DL, McAdams-DeMarco M, et al. Ambient particulate matter air pollution is associated with increased risk of papillary thyroid cancer. Surgery. (2022) 171:212–9. doi: 10.1016/j.surg.2021.05.002
62. Tang ZR, Xu XL, Deng SL, Lian ZX, Yu K. Oestrogenic endocrine disruptors in the placenta and the fetus. In Int J Mol Sci. (2020) 21:1519. doi: 10.3390/ijms21041519
63. Street ME, Shulhai A-M, Rotondo R, Giannì G and Caffarelli C. Current knowledge on the effects of environmental contaminants in early life nutrition. Front Nutr. (2023) 10:1120293. doi: 10.3389/fnut.2023.1120293
64. Street ME, Angelini S, Bernasconi S, Burgio E, Cassio A, Catellani C, et al. Current knowledge on endocrine disrupting chemicals (EDCs) from animal biology to humans, from pregnancy to adulthood: highlights from a national italian meeting. Int J Mol Sci. (2018) 19:1647. doi: 10.3390/ijms19061647
65. Nettore IC, Franchini F, Palatucci G, Macchia PE, Ungaro P. Epigenetic mechanisms of endocrine- disrupting chemicals in obesity. Biomedicines. (2021) 9:1716. doi: 10.3390/biomedicines9111716
66. Sun M, Cao X, Wu Y, Shen L, Wei G. Prenatal exposure to endocrine-disrupting chemicals and thyroid function in neonates: A systematic review and meta-analysis. Ecotoxicol Environ Saf. (2022) 231:113215. doi: 10.1016/j.ecoenv.2022.113215
67. Pearce EN. Endocrine disruptors and thyroid health. Endocr Pract. (2024) 30:172–6. doi: 10.1016/j.eprac.2023.11.002
68. Oliveira KJ, Chiamolera MI, Giannocco G, Pazos-Moura CC, Ortiga-Carvalho TM. Thyroid Function Disruptors: from nature to chemicals. J Mol Endocrinol. (2018) 13:JME–18–0081. doi: 10.1530/JME-18–0081
69. Di Pietro G, Forcucci F, Chiarelli F. Endocrine disruptor chemicals and children’s health. Int J.Mol.Sci. (2023) 24:2671. doi: 10.3390/ijms24032671
70. Leemans M, Couderq S, Demeneix B and Fini J-B. Pesticides with potential thyroid hormone-disrupting effects: A review of recent data. Front Endocrinol. (2019) 10:743. doi: 10.3389/fendo.2019.00743
71. Guarnotta V, Amodei R, Frasca F, Aversa A, Giordano C. Impact of chemical endocrine disruptors and hormone modulators on the endocrine system. Int J Mol Sci. (2022) 23:5710. doi: 10.3390/ijms23105710
72. Romano ME, Eliot MN, Zoeller RT, Hoofnagle AN, Calafat AM, Karagas MR, et al. Maternal urinary phthalate metabolites during pregnancy and thyroid hormone concentrations in maternal and cord sera: The HOME Study. Int J Hyg Environ Health. (2018) 221:623–31. doi: 10.1016/j.ijheh.2018.03.010
73. Serrano-Nascimento C, Nunes MT. Perchlorate, nitrate, and thiocyanate: Environmental relevant NIS-inhibitors pollutants and their impact on thyroid function and human health. Front Endocrinol. (2022) 13:995503. doi: 10.3389/fendo.2022.995503
74. Predieri B, Iughetti L, Bernasconi S, Street ME. Endocrine disrupting chemicals’ Effects in children: what we know and what we need to learn? Int J Mol Sci. (2022) 23:11899. doi: 10.3390/ijms231911899
75. Xiong C, Xu L, Dong X, Cao Z, Wang Y, Chen K, et al. Trimester-specific associations of maternal exposure to bisphenols with neonatal thyroid stimulating hormone levels: A birth cohort study. Sci Total Environ. (2023) 880:163354. doi: 10.1016/j.scitotenv.2023.163354
76. Xi J, Su X, Wang Z, Ji H, Chen Y, Liu X, et al. The associations between concentrations of gestational bisphenol analogues and thyroid related hormones in cord blood: A prospective cohort study. Ecotoxicol Environ Saf. (2023) 256:114838. doi: 10.1016/j.ecoenv.2023.114838
77. Wu Y, Beland FA, Fang JL. Effect of triclosan, triclocarban, 2,2',4,4'-tetrabromodiphenyl ether, and bisphenol A on the iodide uptake, thyroid peroxidase activity, and expression of genes involved in thyroid hormone synthesis. Toxicol In Vitro. (2016) 32:310–9. doi: 10.1016/j.tiv.2016.01.014
78. Wang N, Zhou Y, Fu C, Wang H, Huang P, Wang B, et al. Influence of bisphenol A on thyroid volume and structure independent of iodine in school children. PLoS One. (2015) 10:e0141248. doi: 10.1371/journal.pone.0141248
79. Guo J, Wu C, Zhang J, Li W, Lv S, Lu D, et al. Maternal and childhood urinary phenol concentrations, neonatal thyroid function, and behavioral problems at 10 years of age: The SMBCS study. Sci Total Environ. (2020) 743:140678. doi: 10.1016/j.scitotenv.2020.140678
80. Sun D, Zhou L, Wang S, Liu T, Zhu J, Jia Y, et al. Effect of Di-(2-ethylhexyl) phthalate on the hypothalamus-pituitary-thyroid axis in adolescent rat. Endocr J. (2022) 69:217–24. doi: 10.1507/endocrj.EJ17–0272r
81. Zhang X, Zhao Y, Cheng C, Li L, Xiao M, Zhang G, et al. Combined effects of di (2-ethylhexyl) phthalate and bisphenol A on thyroid hormone homeostasis in adolescent female rats. Environ Sci pollut Res Int. (2020) 27:40882–92. doi: 10.1007/s11356–020-09949-w
82. Morgenstern R, Whyatt RM, Insel BJ, Calafat AM, Liu X, Rauh VA, et al. Phthalates and thyroid function in preschool age children: Sex specific associations. Environ Int. (2017) 106:11–8. doi: 10.1016/j.envint.2017.05.007
83. Huang HB, Kuo PL, Chang JW, Jaakkola JJK, Liao KW, Huang PC. Longitudinal assessment of prenatal phthalate exposure on serum and cord thyroid hormones homeostasis during pregnancy - Tainan birth cohort study (TBCS). Sci Total Environ. (2018) 619–620:1058–65. doi: 10.1016/j.scitotenv.2017.11.048
84. Ghisari M, Bonefeld-Jorgensen EC. Effects of plasticizers and their mixtures on estrogen receptor and thyroid hormone functions. Toxicol Lett. (2009) 189:67–77.
85. Xiang St, Cao Y, Dong J, Li C, Qiu J, Li X. The association between urinary phthalate metabolites and serum thyroid function in US adolescents. Sci Rep. (2023) 13:11601. doi: 10.1038/s41598–023-38644–2
86. Kim KN, Kim HY, Lim YH, Shin CH, Kim JI, Kim BN, et al. Prenatal and early childhood phthalate exposures and thyroid function among school-age children. Environ Int. (2020) 141:105782. doi: 10.1016/j.envint.2020.105782
87. Lopez-Rodriguez D, Franssen D, Heger S, Parent AS. Endocrine-disrupting chemicals and their effects on puberty. Best Pract Res Clin Endocrinol Metab. (2021) 35:101579. doi: 10.1016/j.beem.2021.101579
88. Meeker JD, Ferguson KK. Relationship between urinary phthalate and bisphenol A concentrations and serum thyroid measures in U.S. adults and adolescents from the National Health and Nutrition Examination Survey (NHANES) 2007–2008. Environ Health Perspect. (2011) 119:1396e1402. doi: 10.1289/ehp.1103582
89. Baba T, Ito S, Yuasa M, Yoshioka E, Miyashita C, Araki A, et al. Association of prenatal exposure to PCDD/Fs and PCBs with maternal and infant thyroid hormones: The Hokkaido Study on Environment and Children's Health. Sci Total Environ. (2018) 615:1239–46. doi: 10.1016/j.scitotenv.2017.09.038
90. Warner M, Rauch S, Ames J, Mocarelli P, Brambilla P, Signorini S, et al. Prenatal dioxin exposure and thyroid hormone levels in the Seveso second generation study. Environ Res. (2020) 183:109280. doi: 10.1016/j.envres.2020.109280
91. Su PH, Chen JY, Chen JW, Wang SL. Growth and thyroid function in children with in utero exposure to dioxin: a 5-year follow-up study. Pediatr Res. (2010) 67:205–10. doi: 10.1203/PDR.0b013e3181c8f04b
92. Cediel-Ulloa A, Lupu DL, Johansson Y, Hinojosa M, Özel F, Rüegg J. Impact of endocrine disrupting chemicals on neurodevelopment: the need for better testing strategies for endocrine disruption-induced developmental neurotoxicity. Expert Rev Endocrinol Metab. (2022) 17:2. doi: 10.1080/17446651.2022.2044788
93. Dong H, Curran I, Williams A, Bondy G, Yauk CL, Wade MG. Hepatic miRNA profiles and thyroid hormone homeostasis in rats exposed to dietary potassium perfluorooctanesulfonate (PFOS). Environ Toxicol Pharmacol. (2016) 41:201–10. doi: 10.1016/j.etap.2015.12.009
94. Preston EV, Webster TF, Claus Henn B, McClean MD, McClean MD, Gennings C, Oken E, et al. Prenatal exposure to per- and polyfluoroalkyl substances and maternal and neonatal thyroid function in the project viva cohort: A mixtures approach. Environ Int. (2020) 139:105728. doi: 10.1016/j.envint.2020.105728
95. Jensen RC, Glintborg D, Timmermann CAG, Nielsen F, Boye H, Madsen JB, et al. Higher free thyroxine associated with PFAS exposure in first trimester. Odense Child Cohort. Environ Res. (2022) 212:113492. doi: 10.1016/j.envres.2022.113492
96. Kronborg TM, Hansen JF, Rasmussen ÅK, Vorkamp K, Nielsen CH, Frederiksen M, et al. The flame retardant DE-71 (a mixture of polybrominated diphenyl ethers) inhibits human differentiated thyroid cell function in vitro. PLoS One. (2017) 12:e0179858.
97. Zoeller RT. Environmental chemicals impacting the thyroid: targets and consequences. Thyroid. (2007) 17:811–7. doi: 10.1089/thy.2007.0107
98. Chevrier J, Eskenazi B, Bradman A, Fenster L, Barr DB. Associations between prenatal exposure to polychlorinated biphenyls and neonatal thyroid-stimulating hormone levels in a Mexican-American population, Salinas Valley, California. Environ Health Perspect. (2007) 115:1490–6. doi: 10.1289/ehp.9843
99. Ramhøj L, Hass U, Gilbert ME, Wood C, Svingen T, Usai D, et al. Evaluating thyroid hormone disruption: investigations of long-term neurodevelopmental effects in rats after perinatal exposure to perfluorohexane sulfonate (PFHxS). Sci Rep. (2020) 10:2672. doi: 10.1038/s41598–020-59354-z
100. Sarzo B, Abumallouh R, Marín N, Llop S, Beneito A, Lopez-Flores I, et al. Association between phenols and thyroid hormones: The role of iodothyronine deiodinase genes. Environ pollut. (2022) 311:119926. doi: 10.1016/j.envpol.2022.119926
101. Braun JM, Chen A, Hoofnagle A, Papandonatos GD, Jackson-Browne M, Hauser R, et al. Associations of early life urinary triclosan concentrations with maternal, neonatal, and child thyroid hormone levels. Horm Behav. (2018) 101:77–84. doi: 10.1016/j.yhbeh.2017.11.009
102. Fan Y, Qian H, Wu Z, Li Z, Li X, Zhang Y, et al. Exploratory analysis of the associations between urinary phytoestrogens and thyroid hormones among adolescents and adults in the United States: National Health and Nutrition Examination Survey 2007–2010. Environ Sci pollut Res Int. (2022) 29:2974–84. doi: 10.1007/s11356–021-14553–7
103. Coiffier O, Nakiwala D, Rolland M, Malatesta A, Lyon-Caen S, Chovelon B, et al. Exposure to a mixture of non-persistent environmental chemicals and neonatal thyroid function in a cohort with improved exposure assessment. Environ Int. (2023) 173:107840. doi: 10.1016/j.envint.2023.107840
104. Chen S, Yu M, Yao Y, Li Y, He A, Zhou Z, et al. Thyroid hormone biomonitoring: A review on their metabolism and machine-learning based analysis on effects of endocrine disrupting chemicals. Environ Health. (2024). doi: 10.1021/envhealth.3c00184
105. Kaličanin D, Brčić L, Ljubetić K, Barić A, Gračan S, Brekalo M, et al. Differences in food consumption between patients with Hashimoto's thyroiditis and healthy individuals. Sci Rep. (2020) 10:10670.
106. Zhang X, Chen W, Shao S, Xu G, Song Y, Xu C, et al. A high-fat diet rich in saturated and mono-unsaturated fatty acids induces disturbance of thyroid lipid profile and hypothyroxinemia in male rats. Mol Nutr Food Res. (2018) 62:e1700599.
107. Ruggeri RM, Giovinazzo S, Barbalace MC, Cristani M, Alibrandi A, Vicchio TM, et al. Influence of Dietary Habits on Oxidative Stress Markers in Hashimoto’s Thyroiditis [published correction appears in Thyroid. 2021 Apr;31(4):709]. Thyroid. (2021) 31:96–105. doi: 10.1089/thy.2020.0299
108. Szczuko M, Syrenicz A, Szymkowiak K, Przybylska A, Szczuko U, Pobłocki J, et al. Doubtful justification of the gluten-free diet in the course of hashimoto’s disease. Nutrients. (2022) 14:1727.
109. Mikulska AA, Karaźniewicz-Łada M, Filipowicz D, Ruchała M, Główka FK. Metabolic characteristics of hashimoto’s thyroiditis patients and the role of microelements and diet in the disease management-an overview. Int J Mol Sci. (2022) 23:6580.
110. Knezevic J, Starchl C, Tmava Berisha A, Amrein K. Thyroid-gut-axis: How does the microbiota influence thyroid function? Nutrients. (2020) 12:1769.
111. Calcaterra V, Mameli C, Rossi V, Magenes VC, Massini G, Perazzi C, et al. What we know about the relationship between autoimmune thyroid diseases and gut microbiota: a perspective on the role of probiotics on pediatric endocrinology. Minerva Pediatr (Torino). (2022) 74:650–71.
112. Shu Q, Kang C, Li J, Hou Z, Xiong M, Wang X, et al. Effect of probiotics or prebiotics on thyroid function: A meta-analysis of eight randomized controlled trials. PLoS One. (2024) 19:e0296733. doi: 10.1371/journal.pone.0296733
113. Gibney MJ. Ultra-processed foods: definitions and policy issues. Curr Dev Nutr. (2018) 3:nzy077. doi: 10.1093/cdn/nzy077
114. Zhang J, Zhu F, Cao Z, Rayamajhi S, Zhang Q, Liu L, et al. Ultra-processed food consumption and the risk of subclinical thyroid dysfunction: a prospective cohort study. Food Funct. (2022) 13:3431–40. doi: 10.1039/d1fo03279h
115. Karwowska M, Kononiuk A. Nitrates/nitrites in food-risk for nitrosative stress and benefits. Antioxidants (Basel). (2020) 9:241. doi: 10.3390/antiox9030241
116. Said Abasse K, Essien EE, Abbas M, Yu X, Xie W, Sun J, et al. Association between dietary nitrate, nitrite intake, and site-specific cancer risk: A systematic review and meta-analysis. Nutrients. (2022) 14:666. doi: 10.3390/nu14030666
117. Amereh F, Eslami A, Fazelipour S, Rafiee M, Zibaii MI, Babaei M. Thyroid endocrine status and biochemical stress responses in adult male Wistar rats chronically exposed to pristine polystyrene nanoplastics. Toxicol Res. (2019) 8:953–63. doi: 10.1039/c9tx00147
118. Pamphlett R, Doble PA, Bishop DP. Mercury in the human thyroid gland: Potential implications for thyroid cancer, autoimmune thyroiditis, and hypothyroidism. PLoS One. (2021) 16:e0246748. doi: 10.1371/journal.pone.0246748
119. World Health Organization. (2023). Available online at: https://www.who.int/publications/m/item/weekly-epidemiological-update-on-covid-19—1-june-2023 (Accessed September 29, 2023).
120. Li B, Zhang S, Zhang R, Chen X, Wang Y, Zhu C. Epidemiological and clinical characteristics of COVID-19 in children: A systematic review and meta-analysis. Front Pediatr. (2020) 8:591132. doi: 10.3389/fped.2020.591132
121. Wang MY, Zhao R, Gao LJ, Gao XF, Wang DP, Cao JM. SARS-CoV-2: Structure, biology, and structure-based therapeutics development. Front Cell Infect Microbiol. (2020) 10:587269. doi: 10.3389/fcimb.2020.587269
122. Jackson CB, Farzan M, Chen B, Choe H. Mechanisms of SARS-CoV-2 entry into cells. Nat Rev Mol Cell Biol. (2022) 23:3–20. doi: 10.1038/s41580–021-00418-x
123. Ashraf UM, Abokor AA, Edwards JM, Waigi EW, Royfman RS, Hasan SA, et al. SARS-CoV-2, ACE2 expression, and systemic organ invasion. Physiol Genomics. (2021) 53:51–60. doi: 10.1152/physiolgenomics.00087.2020
124. Gnocchi M, D'Alvano T, Lattanzi C, Messina G, Petraroli M, Patianna VD, et al. Current evidence on the impact of the COVID-19 pandemic on paediatric endocrine conditions. Front Endocrinol (Lausanne). (2022) 13:913334. doi: 10.3389/fendo.2022.913334
125. Li MY, Li L, Zhang Y, Wang XS. Expression of the SARS-CoV-2 cell receptor gene ACE2 in a wide variety of human tissues. Infect Dis Poverty. (2020) 9:45. doi: 10.1186/s40249–020-00662-x
126. De Vito P, Incerpi S, Pedersen JZ, Luly P, Davis FB, Davis PJ. Thyroid hormones as modulators of immune activities at the cellular level. Thyroid. (2011) 21:879–90.
127. Chen W, Tian Y, Li Z, Zhu J, Wei T, Lei J. Potential interaction between SARS-CoV-2 and thyroid: A review. Endocrinology. (2021) 162:bqab004. doi: 10.1210/endocr/bqab004
128. Inaba H, Aizawa T. Coronavirus disease 2019 and the thyroid - progress and perspectives. Front Endocrinol (Lausanne). (2021) 12:70833. doi: 10.3389/fendo.2021.70833
129. Khoo B, Tan T, Clarke SA, Mills EG, Patel B, Modi M, et al. Thyroid function before, during and after COVID-19. J Clin Endocrinol Metab. (2021) 106:e803–11.
130. Zhang Y, Lin F, Tu W, Zhang J, Choudhry AA, Ahmed O, et al. Medical team from xiangya hospital to support hubei, China. thyroid dysfunction may be associated with poor outcomes in patients with COVID-19. Mol Cell Endocrinol. (2021) 521:111097. doi: 10.1016/j.mce.2020.111097
131. Lui DTW, Lee CH, Chow WS, Lee ACH, Tam AR, Fong CHY, et al. Thyroid dysfunction in relation to immune profile, disease status, and outcome in 191 patients with COVID-19. J Clin Endocrinol Metab. (2021) 106:e926–35. doi: 10.1210/clinem/dgaa813
132. American Academy of Pediatrics. Children and COVID-19: state-Level Data Report. Available online at: www.aap.org/en/pages/2019-novel-coronavirus-covid-19-infections/children-and-covid-19-state-level-data-report/ (Accessed August 27, 2023).
133. Jafarzadeh A, Nemati M, Jafarzadeh S, Nozari P, Mortazavi SMJ. Thyroid dysfunction following vaccination with COVID-19 vaccines: a basic review of the preliminary evidence. J Endocrinol Invest. (2022) 45:1835–63. doi: 10.1007/s40618–022-01786–7
Keywords: thyroid, thyroid hormones, environmental pollution, food pollution, endocrine disruptors, COVID-19
Citation: Street ME, Shulhai A-M, Petraroli M, Patianna V, Donini V, Giudice A, Gnocchi M, Masetti M, Montani AG, Rotondo R, Bernasconi S, Iughetti L, Esposito SM and Predieri B (2024) The impact of environmental factors and contaminants on thyroid function and disease from fetal to adult life: current evidence and future directions. Front. Endocrinol. 15:1429884. doi: 10.3389/fendo.2024.1429884
Received: 08 May 2024; Accepted: 28 May 2024;
Published: 19 June 2024.
Edited by:
Mariacarolina Salerno, University of Naples Federico II, ItalyReviewed by:
Malgorzata Gabriela Wasniewska, University of Messina, ItalyMarek Niedziela, Poznan University of Medical Sciences, Poland
Copyright © 2024 Street, Shulhai, Petraroli, Patianna, Donini, Giudice, Gnocchi, Masetti, Montani, Rotondo, Bernasconi, Iughetti, Esposito and Predieri. This is an open-access article distributed under the terms of the Creative Commons Attribution License (CC BY). The use, distribution or reproduction in other forums is permitted, provided the original author(s) and the copyright owner(s) are credited and that the original publication in this journal is cited, in accordance with accepted academic practice. No use, distribution or reproduction is permitted which does not comply with these terms.
*Correspondence: Maria E. Street, bWFyaWFlbGlzYWJldGguc3RyZWV0QHVuaXByLml0