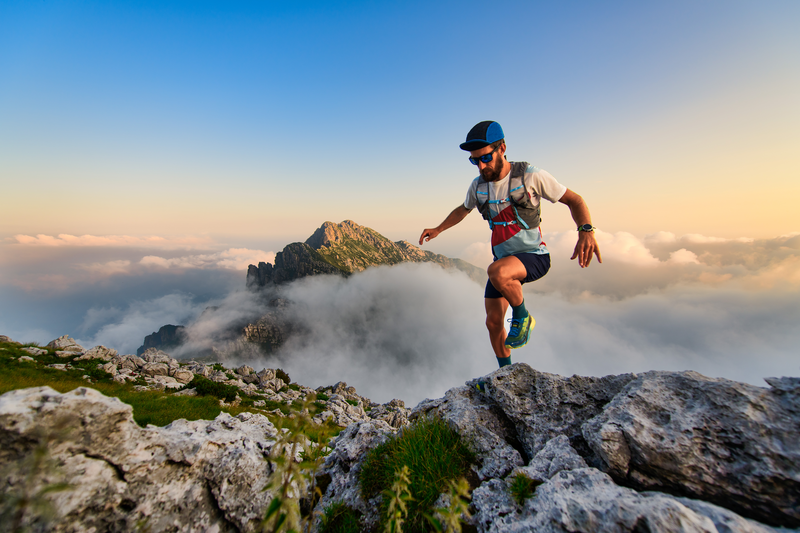
95% of researchers rate our articles as excellent or good
Learn more about the work of our research integrity team to safeguard the quality of each article we publish.
Find out more
ORIGINAL RESEARCH article
Front. Endocrinol. , 04 October 2024
Sec. Reproduction
Volume 15 - 2024 | https://doi.org/10.3389/fendo.2024.1425639
Objectives: Thyroid dysfunction is commonly associated with the risk of infertility in both females and males. However, recent randomized controlled trials have demonstrated that thyroid function levels in females are not significantly related to infertility, and evidence on the association between male thyroid function and infertility is limited. We aim to investigate the association between thyroid function levels and infertility in both females and males.
Method: A two-sample Mendelian randomization study was conducted using four methods, with the inverse variance weighted method (IVW) as the primary approach. Data on thyroid function as the exposure were obtained from the ThyroidOmics Consortium and UK Biobank, including over 700,000 individuals from a large meta-analysis of genome-wide association studies for thyroid function and dysfunction. The outcome data for infertility in both sex encompassed more than 70,000 individuals from the FinnGen Consortium. All participants were adults of European ancestry. The MR Egger regression intercept and Cochran’s Q test were employed to evaluate directional pleiotropy and heterogeneity.
Results: The results indicated no causal effect of thyroid-stimulating hormone (TSH) and free tetraiodothyronine (fT4) on female and male infertility. Furthermore, no causal association between hypo- and hyperthyroidism and infertility were identified. Notably, we observed a causal relationship between high TSH and endometriosis-related infertility (OR=0.82, 95% CI: 0.74–0.91, P = 1.49E-04).
Conclusions: This study did not find evidence for casual relationship between thyroid function levels and risk of infertility. The findings suggest that overall thyroid function levels may not be a significant predictor of infertility risk.
Numerous observational studies have established a link between thyroid dysfunction and infertility (1). However, the extent of thyroid dysfunction’s impact on the female reproductive system varies, and its effects on fertility are inconsistent (2–4). The influence of thyroid dysfunction on male fertility remains understudied, despite evidence suggesting a potential connection. Infertility, characterized by the inability to conceive after 12 months of regular, unprotected sexual intercourse, is a significant public health issue with a global prevalence of 8-12% (5–8). Optimal thyroid function is crucial for maintaining reproductive fertility, as thyroid hormones (THs) interact with nuclear receptors in reproductive organs, modulating their development and function (9–11). Changes in sex steroid levels and sex hormone-binding globulin among individuals with hyper- and hypothyroidism regardless of sex has been reported previously (12–16).
In women, infertility is prevalent in those with Hashimoto’s and Graves’ diseases, affecting approximately 50% of patients (17). Elevated thyroid-stimulating hormone (TSH) levels have been observed in women with unexplained infertility compared to controls (18). However, the clinical significance of TSH levels in the high-normal range (2.5–4.9 mIU/L) for pregnancy outcomes has been debated, with some studies showing no adverse effects on clinical pregnancy, live birth, and miscarriage rates (19). A comparison between patients with TSH levels <2.5 mIU/L and those with TSH levels >2.5 mIU/L showed no significant effect on conception, clinical pregnancy, first-trimester pregnancy loss, or live birth rates (20). Furthermore, The role of levothyroxine (LT4) treatment in infertility has also been inconclusive in randomized controlled trials (RCTs) (3, 4).
While the effects of thyroid dysfunction on female reproductive function have been extensively studied, its impact on male reproductive function is less clear. Some studies suggest that thyroid hormone disorders can lead to sexual dysfunction, potentially reversible with normalization of TH levels (21–23). THs are involved in ejaculation physiology and can affect testicular function through nuclear receptors (10, 24–27). However, the evidence linking thyroid dysfunction directly to male infertility is limited, and there is no consensus on the impact of thyroid function on fertility in both sexes.
Autoimmune thyroid diseases significantly impair fertility by creating a cytotoxic environment that negatively affects oocyte maturation and embryonic development. These conditions are particularly prevalent in women with infertility related to endometriosis or polycystic ovary syndrome (PCOS) (28, 29). Despite debates on TSH level benchmarks for thyroid dysfunction, the ATA and ETA propose that women with infertility starting ART take a low-dose levothyroxine regimen to keep TSH under 2.5 mIU/L, four weeks before ovarian stimulation, to enhance fertility treatment outcomes (30, 31).
Given the potential biases in previous observational studies due to limitations and confounding factors, there is a clear need for more robust experimental designs. This study aims to provide clarity by investigating the genetic causal associations between thyroid function, hypothyroidism, hyperthyroidism, and infertility using Mendelian randomization (MR) methodology.
The Genome Wide Association Studies (GWAS) meta-analysis summary statistics pertaining to thyroid function were obtained from the ThyroidOmics Consortium (www.thyroidomics.com), which investigates the determinants and effects of thyroid diseases and thyroid function, using diverse omics technologies in multiple large and well-phenotyped cohort studies. Thyroid function was assessed using sex-stratified TSH and free tetraiodothyronine (fT4) levels as conducted by Teumer et al. (32), whereas sex-stratified statistics for hypo- and hyperthyroidism as binary exposures were obtained from the UK Biobank. To address the potential limitations of clinical diagnosis of hypothyroidism and hyperthyroidism in the outcome data, we included non-sex-stratified high and low TSH traits (compared to cohort-specific reference ranges) as the exposure groups. The numerical values of reference ranges, determined by the 2.5th and 97.5th percentiles of TSH while considering assay variability, demographic traits, and environmental factors such as iodine intake, are similar among the studies included. This case-control GWAS encompassed a total of 27 studies for high TSH and 20 studies for low TSH for subsequent analysis (Supplementary Table S1) (33).
The factors that contribute to infertility exhibit substantial differences between men and women, influenced by distinct anatomical structures and physiological processes. This group comprises individuals diagnosed with infertility according to the International Classification of Diseases, Tenth Revision (ICD-10, 2016 version), as reported by the FinnGen Consortium. The FinnGen data was collected through an extensive recruitment process that included hospital biobanks and disease-specific ‘legacy’ cohorts. Our approach to case identification was methodically stratified by sex, ensuring that the outcomes of our MR study accurately capture the distinct impacts of sex on infertility. The case group was identified using FinnGen’s clinical endpoints, which are based on a thorough set of criteria including diagnoses from healthcare registers, hospital discharge summaries, and other relevant medical records (Supplementary Table S2). To ensure a valid comparison, the control group was composed of individuals from the general population without the condition of interest. These controls were precisely matched to cases based on key demographic variables—age, sex, and ethnicity—to minimize potential confounding effects. This rigorous matching process is central to the robustness of our study design.
To minimize ancestry-related potential confounding effects, we restricted the analyses to unrelated participants with European ancestry. Significant crossover or overlap was not observed between participants in the GWAS for thyroid function and infertility. Ethical approval was not required since publicly available summary-level statistics were utilized in this study.
MR necessitates that the genetic instruments exhibit a strong association with thyroid function-related traits (p < 5 × 10−8). To account for linkage disequilibrium (r2 >0.001), a pruning step was performed on the candidate IVs, ensuring that any association between instruments and the outcome was exposure-driven. Additionally, variants within a 1-Mb distance from other IVs displaying a stronger association were excluded to mitigate bias. These single nucleotide polymorphisms (SNPs) were chosen as IVs. We evaluated the potential weak instrumental bias using the F-statistic, calculated as F= β 2 exposure/SE 2 exposure. SNPs with F-statistic <10 was considered weak IVs and excluded from further analysis.
Statistical analyses were mainly performed using R software version 4.3.1 (https://www.rproject.org/, accessed on 20 February 2024) with the “Two-Sample MR” package serving as the primary analysis tool. The effect allele was defined as the allele associated with increased TSH and fT4 levels and increased risk of hypothyroidism, hyperthyroidism, and high and low TSH (exposure). The traits of low and high TSH versus reference range TSH may overlap with other exposure factors selected in this study, particularly hypothyroidism and hyperthyroidism. However, addressing the subclinical forms of hyperthyroidism and hypothyroidism cannot be overlooked. The primary analysis involved the inverse variance weighting (IVW) method, which required validating all SNPs as IVs. The weighted median method provided reliable estimates when at least 50% of the weight was derived from valid genetic variants (34). MR Egger yields estimate robust to pleiotropy, even when fewer than 50% of the genetic variants are considered valid (35). The weighted mode method was also applied (36). A p-value below 0.05 was considered statistically significant.
Sensitivity analysis was conducted using three methods: heterogeneity testing, horizontal pleiotropy testing, and leave-one-out analysis. Cochran’s Q statistic was used to examine the heterogeneity of individual SNPs in IVW tests, and in cases where heterogeneity was detected (Qpval < 0.05), the random effects IVW approach was employed. The MR-Egger intercept was used to assess horizontal pleiotropy, and obtaining a significant result in this test is considered unacceptable. The leave-one-out approach systematically eliminates each SNP to assess the individual influence of each SNP.
For women, we identified 20 SNPs associated with TSH, 11 SNPs associated with fT4, and 13 and 4 SNPs associated with hypothyroidism and hyperthyroidism, respectively. For men, we identified 25 SNPs associated with TSH, 9 SNPs associated with fT4, and 8 and 3 SNPs associated with hypo- and hyperthyroidism, respectively. Moreover, 9 and 14 SNPs associated with high and low TSH (overall), respectively, were selected (Supplementary Table S3). The F-statistic values of each SNP at every filtering step were all > 10 (range: 30.05-474.85), indicating that all selected IVs were valid and not susceptible to weak instrument bias. The MR analytical results of association of TSH, fT4, hyperthyroidism, hypothyroidism, and high and low TSH with male and female infertility are presented in Supplementary Table S4.
No association between one standard deviation (SD) increase in genetically predicted TSH and the risk of infertility in both sexes was observed in the MR analyses (women, IVW: odds ratio [OR]=0.98, 95% confidence interval [CI]: 0.90–1.07, P = 0.66; men, IVW: OR=1.06, 95% CI: 0.86–1.30, P = 0.59). No evidence of heterogeneity or horizontal pleiotropy was found in the sensitivity analyses (Supplementary Table S4; Supplementary Figures S1, S2).
One SD increase in genetically predicted fT4 was not associated with the risk of female and male infertility after MR analyses (women, IVW: OR=0.99, 95% CI: 0.86–1.14, P = 0.89; men, IVW: OR=0.86, 95% CI: 0.51–1.43, P = 0.56). Sensitivity analyses revealed heterogeneity (women Qpval=0.03, men Qpval=0.01), indicating variability within the study population. However, no evidence of horizontal pleiotropy was observed. Hence, the IVW analysis was conducted using a random effects model (Supplementary Table S4; Supplementary Figures S1, S2).
Hypothyroidism was not significantly associated with the risk of female and male infertility (Supplementary Table S3). Similarly, the MR analysis did not reveal a causal relationship between hyperthyroidism and infertility in both sexes. No evidence of heterogeneity or horizontal pleiotropy was found after sensitivity analyses (Supplementary Table S3). When conducting the analysis on hyperthyroidism, we observed heterogeneity between hyperthyroidism in women and infertility (Qpval=0.04). Therefore, a random-effects model, IVW, was used to analyze the causal relationship in women. No evidence of horizontal pleiotropy was observed. The results revealed no causal relationship between hyperthyroidism and infertility in both sexes (Supplementary Table S4; Supplementary Figures S1, S2).
High TSH was not significantly associated with the risk of female and male infertility (women, IVW: OR=0.98, 95% CI: 0.92–1.03, P = 0.40; men, IVW: OR=1.06, 95% CI: 0.88–1.27, P = 0.54). Similarly, the MR analysis did not reveal a causal relationship between low TSH and infertility in both sexes. No evidence of heterogeneity or horizontal pleiotropy was found after the sensitivity analyses (Supplementary Table S4; Supplementary Figures S1, S2).
The six categories of thyroid function variables (TSH, fT4, hypothyroidism, hyperthyroidism, high TSH, and low TSH) were subjected to MR analysis with five different infertility factors (endometriosis related infertility, cervical and vaginal origin, uterine origin, tubal origin, and anovulation-associated infertility). Notably, a causal association was observed between high TSH and endometriosis-related infertility in women (OR=0.82, 95% CI=0.74–0.91, P = 1.49E-04) (Figure 1). However, no significant causal associations were observed for other conditions (Supplementary Table S4; Supplementary Figures S3–S7).
Figure 1. Mendelian Randomization (MR) Analyses Regarding the Effect of High TSH and Endometriosis-related Infertility in Women. (A) Scatter plot for instrument high TSH associations against instrument endometriosis-related infertility associations (x-axis). (B) Forest plot for the assessment of individual and overall effects of each instrumental variable in the analysis. (C) Leave-one-out analysis. (D) Funnel plot for visually assess the presence of publication bias and asymmetry in the distribution of estimated causal effects.
This study represents the first comprehensive investigation of the causal relationship between thyroid function and infertility, leveraging genetic variation and a diverse array of MR methods. The implementation of sex-stratified cohorts was pivotal, effectively controlling for confounders and bolstering the internal and external validity of our findings. Our MR analysis, while not establishing a causal nexus between genetically elevated TSH and fT4 levels and infertility, provides novel insights into the complex dynamics between thyroid function and reproductive health. This finding prompts a critical reassessment of the existing body of evidence, highlighting the nuanced relationship between thyroid hormones and fertility.
Our results revealed that genetically increased TSH and fT4 levels in both men and women were not causally associated with infertility. Furthermore, no evidence was found supporting a causal relationship between genetically predicted hypothyroidism, hyperthyroidism, high TSH, low TSH, and infertility in both sexes. Additionally, while we observed a causal relationship between hypothyroidism and endometriosis-related infertility in women, no causal associations were observed between other thyroid function-related exposures and infertility factors. This observation merits further investigation and discussion as it may provide insights into specific subpopulations within the context of infertility.
Previous studies have reported that thyroid function levels may affect fertility in both sexes. Physiological research indicated that hypo- and hyperthyroidism were linked to sex hormone-binding globulin, sex hormone levels, ovarian reserve, and even sexual function (37–44). Multiple studies support the association of male hypothyroidism with abnormal sperm morphology and hyperthyroidism with abnormal sperm motility and DNA damage (37, 45, 46). These research findings suggest that thyroid dysfunction may lead to infertility. However, clinical research on the relationship between male thyroid disorders and infertility is limited. Conversely, for women, numerous observational studies suggest a potential association between thyroid dysfunction and female infertility, with most focusing on hypothyroidism. Observational studies have found that, after excluding absolute causes of infertility, TSH levels exceeding 4.0 mIU/L are closely associated with adverse fertility outcomes, whereas elevated thyroid antibodies are not related to these outcomes (47, 48). Two observational studies targeting subclinical hypothyroidism and infertility populations suggest a potential association between the two (49, 50). Additionally, an association has been observed between elevated TSH levels and reduced clinical pregnancy rates (51). Two RCTs demonstrated that LT4 supplementation in infertile women with subclinical hypothyroidism significantly improved embryo quality, increased clinical pregnancy rates following assisted reproductive technology, and reduced the risk of miscarriage (52, 53).
However, numerous studies have found no evidence of such an association, suggesting that this correlation might be influenced by other endocrine disruptions related to thyroid dysfunction. Despite the heightened risk of miscarriage, cases of spontaneous conception have been observed in individuals with overt hypothyroidism (54, 55). A study found that women with clinical hypothyroidism and subclinical hypothyroidism did not show significant changes in ovarian reserves (56). Moreover, a recent RCT found no association between TSH levels ≥2.5 or the presence of anti-thyroid antibodies and fecundity, pregnancy loss, or live birth in healthy reproductive-aged women with a history of miscarriage (2). Variations in TSH levels, whether within the normal range or reaching subclinical hypothyroidism status, are not associated with clinical pregnancy and delivery rates (57, 58). Additional high-quality RCTs have demonstrated the lack of LT4 supplementation effect on the pregnancy rate among women with subclinical hypothyroidism undergoing in vitro fertilization or among women with normal thyroid function but positive for anti-thyroperoxidase antibodies (3, 4, 59, 60). The effect of hyperthyroidism on female fertility remains unclear owing to a lack of reliable evidence (47, 61). Untreated hyperthyroidism in women is associated with a higher risk of spontaneous miscarriage, although most women with hyperthyroidism still experience ovulation (61, 62).
Overall, a cornerstone of our research is the support and expansion of previous observational studies linking thyroid dysfunction with female infertility, particularly hypothyroidism. It is imperative to consider issues such as low quality of evidence and significant heterogeneity in these observational studies, which may arise from small sample sizes, suboptimal study designs, varied selection criteria for infertility, and differences in methods used to measure thyroid autoimmunity, THs, and reference values for TSH. We serendipitously discovered a correlation between high TSH levels and a decreased risk of endometriosis-related infertility, which aligns with a previous study (63). In the balance of TSH and THs, T3 and T4 specifically stimulate the proliferation of ectopic endometrial cells and the production of reactive oxygen species (63, 64). Furthermore, using a mouse model of toxic hypothyroidism, a significant reduction in induced endometriosis through uterine horn surgical implantation was observed (63).
MR analysis offers significant advantages in controlling unmeasured confounding and reverse causation. This is particularly pertinent in studying the relationship between thyroid function and infertility, as thyroid dysfunction often triggers systemic metabolic instability. Our study focused on genetically increased TSH and fT4 levels as primary factors, along with genetic predisposition to hypothyroidism and hyperthyroidism as important supplementary factors, providing a comprehensive evaluation of thyroid function. Notably, we stratified the analysis by sex in the MR study, recognizing significant disparities in thyroid dysfunction prevalence and underlying physiological mechanisms (65). Failure to account for sex differences in the study design could introduce potential limitations in rigor. Our findings not only support numerous observational studies on the association between thyroid dysfunction and female infertility but also contribute significantly to the limited research evidence on the relationship between male thyroid dysfunction and infertility.
However, we acknowledge the limitations inherent in our study design. The utilization of public GWAS databases, while advantageous for sample size, restricted access to granular clinical details. The absence of age stratification and detailed classification of infertility causes, particularly for anovulation-related infertility, limits the depth of our analysis. Additionally, the study’s focus on individuals of European ancestry may impact the broader generalizability of our findings.
Although a causal relationship between high TSH levels and a decreased risk of endometriosis-related infertility was found, no association was observed with other thyroid functions as potential exposures due to the negative feedback regulation between TSH and THs. Additionally, the study did not explore reverse causality because of insufficient instrumental variables for sex-stratified infertility. Additionally, the distribution of female infertility causes should be interpreted within the context of the Finnish population, as the stratified data on female infertility included may not accurately reflect the actual epidemiology (6). In light of these considerations, future research should endeavor to employ larger-scale GWAS summary data and a more diverse array of genetic instruments. This approach will be instrumental in validating and expanding upon our findings.
Our study did not find evidence for a direct causal relationship between thyroid function levels and infertility in both sexes. The findings suggest that overall thyroid function levels may not be a significant predictor of infertility risk. Future research, including additional RCTs with more detailed categorization of thyroid function status, may provide further insights into the complex relationship between thyroid function and fertility.
The original contributions presented in the study are included in the article/Supplementary Material. Further inquiries can be directed to the corresponding authors. The two-sample MR approach is based on data freely available from the public domain.
According to local legislation and institutional requirements, the study involving human participants did not require ethical approval. In line with national legislation and institutional requirements, written informed consent was not obtained from the participants or their legal guardians/next of kin for their involvement in this study.
QL: Writing – original draft, Writing – review & editing, Conceptualization, Data curation, Formal analysis, Project administration, Software, Validation. YQ: Writing – original draft, Writing – review & editing, Conceptualization, Data curation, Investigation, Methodology, Project administration, Validation, Visualization. JJ: Methodology, Software, Validation, Writing – review & editing. SL: Formal analysis, Investigation, Writing – review & editing. CZ: Resources, Visualization, Writing – review & editing. GC: Project administration, Supervision, Writing – review & editing. JW: Funding acquisition, Project administration, Supervision, Writing – review & editing.
The author(s) declare financial support was received for the research, authorship, and/or publication of this article. This research was funded by the National Natural Science Foundation of China, grant number 82070878 and 82370786, as well as the Natural Science Foundation of Fujian Province, grant number 2022J01521.
The authors would like to thank the ThyroidOmics consortium, UK Biobank, and FinnGen consortium for sharing the data and making this project possible.
The authors declare that the research was conducted in the absence of any commercial or financial relationships that could be construed as a potential conflict of interest.
All claims expressed in this article are solely those of the authors and do not necessarily represent those of their affiliated organizations, or those of the publisher, the editors and the reviewers. Any product that may be evaluated in this article, or claim that may be made by its manufacturer, is not guaranteed or endorsed by the publisher.
The Supplementary Material for this article can be found online at: https://www.frontiersin.org/articles/10.3389/fendo.2024.1425639/full#supplementary-material
Supplementary Figure S1 | Association of genetically predicted thyroid function with risk of female infertility, analyzed with four different Mendelian randomization (MR) methods. SNPs, single-nucleotide polymorphisms; OR, odds ratio; 95% CI, 95% confidence interval.
Supplementary Figure S2 | Association of genetically predicted thyroid function with risk of male infertility, analyzed with four different Mendelian randomization (MR) methods. SNPs, single-nucleotide polymorphisms; OR, odds ratio; 95% CI, 95% confidence interval.
Supplementary Figure S3 | Association of genetically predicted thyroid function with risk of endometriosis related infertility, analyzed with four different Mendelian randomization (MR) methods. SNPs, single-nucleotide polymorphisms; OR, odds ratio; 95% CI, 95% confidence interval.
Supplementary Figure S4 | Association of genetically predicted thyroid function with risk of cervigal, vaginal, other or unspecified origin infertility, analyzed with four different Mendelian randomization (MR) methods. SNPs, single-nucleotide polymorphisms; OR, odds ratio; 95% CI, 95% confidence interval.
Supplementary Figure S5 | Association of genetically predicted thyroid function with risk of uterine origin infertility, analyzed with four different Mendelian randomization (MR) methods. SNPs, single-nucleotide polymorphisms; OR, odds ratio; 95% CI, 95% confidence interval.
Supplementary Figure S6 | Association of genetically predicted thyroid function with risk of tubal origin infertility, analyzed with four different Mendelian randomization (MR) methods. SNPs, single-nucleotide polymorphisms; OR, odds ratio; 95% CI, 95% confidence interval.
Supplementary Figure S7 | Association of genetically predicted thyroid function with risk of anovulation related infertility, analyzed with four different Mendelian randomization (MR) methods. SNPs, single-nucleotide polymorphisms; OR, odds ratio; 95% CI, 95% confidence interval.
1. Bartáková J, Potluková E, Rogalewicz V, Fait T, Schöndorfová D, Telička Z, et al. Screening for autoimmune thyroid disorders after spontaneous abortion is cost-saving and it improves the subsequent pregnancy rate. BMC Pregnancy Childbirth. (2013) 13:217. doi: 10.1186/1471-2393-13-217
2. Plowden TC, Schisterman EF, Sjaarda LA, Zarek SM, Perkins NJ, Silver R, et al. Subclinical hypothyroidism and thyroid autoimmunity are noat associated with fecundity, pregnancy loss, or live birth. J Clin Endocrinol Metab. (2016) 101:2358–65. doi: 10.1210/jc.2016-1049
3. Wang H, Gao H, Chi H, Zeng L, Xiao W, Wang Y, et al. Effect of levothyroxine on miscarriage among women with normal thyroid function and thyroid autoimmunity undergoing in vitro fertilization and embryo transfer: A randomized clinical trial. Jama. (2017) 318:2190–8. doi: 10.1001/jama.2017.18249
4. Dhillon-Smith RK, Middleton LJ, Sunner KK, Cheed V, Baker K, Farrell-Carver S, et al. Levothyroxine in women with thyroid peroxidase antibodies before conception. New Engl J Med. (2019) 380:1316–25. doi: 10.1056/NEJMoa1812537
5. Carson SA, Kallen AN. Diagnosis and management of infertility: A review. Jama. (2021) 326:65–76. doi: 10.1001/jama.2021.4788
6. Vander Borght M, Wyns C. Fertility and infertility: Definition and epidemiology. Clin Biochem. (2018) 62:2–10. doi: 10.1016/j.clinbiochem.2018.03.012
7. Inhorn MC, Patrizio P. Infertility around the globe: new thinking on gender, reproductive technologies and global movements in the 21st century. Hum Reprod Update. (2015) 21:411–26. doi: 10.1093/humupd/dmv016
8. Phillips K, Olanrewaju RA, Omole F. Infertility: evaluation and management. Am Fam Physician. (2023) 107:623–30.
9. Carosa E, Lenzi A, Jannini EA. Thyroid hormone receptors and ligands, tissue distribution and sexual behavior. Mol Cell Endocrinol. (2018) 467:49–59. doi: 10.1016/j.mce.2017.11.006
10. La Vignera S, Vita R, Condorelli RA, Mongioì LM, Presti S, Benvenga S, et al. Impact of thyroid disease on testicular function. Endocrine. (2017) 58:397–407. doi: 10.1007/s12020-017-1303-8
11. Silva JF, Ocarino NM, Serakides R. Thyroid hormones and female reproduction. Biol Reprod. (2018) 99:907–21. doi: 10.1093/biolre/ioy115
12. Gabrielson AT, Sartor RA, Hellstrom WJG. The impact of thyroid disease on sexual dysfunction in men and women. Sex Med Rev. (2019) 7:57–70. doi: 10.1016/j.sxmr.2018.05.002
13. Krassas GE, Markou KB. The impact of thyroid diseases starting from birth on reproductive function. Hormones (Athens). (2019) 18:365–81. doi: 10.1007/s42000-019-00156-y
14. Kotopouli M, Stratigou T, Antonakos G, Christodoulatos GS, Karampela I, Dalamaga M. Early menarche is independently associated with subclinical hypothyroidism: a cross-sectional study. Horm Mol Biol Clin Investig. (2019) 38. doi: 10.1515/hmbci-2018-0079
15. Dittrich R, Beckmann MW, Oppelt PG, Hoffmann I, Lotz L, Kuwert T, et al. Thyroid hormone receptors and reproduction. J Reprod Immunol. (2011) 90:58–66. doi: 10.1016/j.jri.2011.02.009
16. Kjaergaard AD, Marouli E, Papadopoulou A, Deloukas P, Kus A, Sterenborg R, et al. Thyroid function, sex hormones and sexual function: a Mendelian randomization study. Eur J Epidemiol. (2021) 36:335–44. doi: 10.1007/s10654-021-00721-z
17. Quintino-Moro A, Zantut-Wittmann DE, Tambascia M, MaChado Hda C, Fernandes A. High prevalence of infertility among women with graves' Disease and hashimoto's thyroiditis. Int J Endocrinol. (2014) 2014:982705. doi: 10.1155/2014/982705
18. Orouji Jokar T, Fourman LT, Lee H, Mentzinger K, Fazeli PK. Higher TSH levels within the normal range are associated with unexplained infertility. J Clin Endocrinol Metab. (2018) 103:632–9. doi: 10.1210/jc.2017-02120
19. Tuncay G, Karaer A, İnci Coşkun E, Baloğlu D, Tecellioğlu AN. The impact of thyroid-stimulating hormone levels in euthyroid women on intrauterine insemination outcome. BMC Womens Health. (2018) 18:51. doi: 10.1186/s12905-018-0541-0
20. Seungdamrong A, Steiner AZ, Gracia CR, Legro RS, Diamond MP, Coutifaris C, et al. Preconceptional antithyroid peroxidase antibodies, but not thyroid-stimulating hormone, are associated with decreased live birth rates in infertile women. Fertil Steril. (2017). doi: 10.1016/j.fertnstert.2017.08.026
21. Krassas GE, Tziomalos K, Papadopoulou F, Pontikides N, Perros P. Erectile dysfunction in patients with hyper- and hypothyroidism: how common and should we treat? J Clin Endocrinol Metab. (2008) 93:1815–9. doi: 10.1210/jc.2007-2259
22. Meikle AW. The interrelationships between thyroid dysfunction and hypogonadism in men and boys. Thyroid. (2004) 14 Suppl 1:S17–25. doi: 10.1089/105072504323024552
23. Carani C, Isidori AM, Granata A, Carosa E, Maggi M, Lenzi A, et al. Multicenter study on the prevalence of sexual symptoms in male hypo- and hyperthyroid patients. J Clin Endocrinol Metab. (2005) 90:6472–9. doi: 10.1210/jc.2005-1135
24. Wagner MS, Wajner SM, Maia AL. The role of thyroid hormone in testicular development and function. J Endocrinol. (2008) 199:351–65. doi: 10.1677/joe-08-0218
25. Wagner MS, Wajner SM, Maia AL. Is there a role for thyroid hormone on spermatogenesis? Microsc Res Tech. (2009) 72:796–808. doi: 10.1002/jemt.20759
26. Condorelli RA, La Vignera S, Mongioì LM, Alamo A, Giacone F, Cannarella R, et al. Thyroid hormones and spermatozoa: in vitroEffects on sperm mitochondria, viability and DNA integrity. J Clin Med. (2019) 8. doi: 10.3390/jcm8050756
27. Fumel B, Froment P, Holzenberger M, Livera G, Monget P, Fouchécourt S. Expression of dominant-negative thyroid hormone receptor alpha1 in Leydig and Sertoli cells demonstrates no additional defect compared with expression in Sertoli cells only. PloS One. (2015) 10:e0119392. doi: 10.1371/journal.pone.0119392
28. Artini PG, Uccelli A, Papini F, Simi G, Di Berardino OM, Ruggiero M, et al. Infertility and pregnancy loss in euthyroid women with thyroid autoimmunity. Gynecological endocrinology: Off J Int Soc Gynecol Endocrinol. (2013) 29:36–41. doi: 10.3109/09513590.2012.705391
29. Bucci I, Giuliani C, Di Dalmazi G, Formoso G, Napolitano G. Thyroid autoimmunity in female infertility and assisted reproductive technology outcome. Front Endocrinol. (2022) 13:768363. doi: 10.3389/fendo.2022.768363
30. Alexander EK, Pearce EN, Brent GA, Brown RS, Chen H, Dosiou C, et al. 2017 Guidelines of the american thyroid association for the diagnosis and management of thyroid disease during pregnancy and the postpartum. Thyroid: Off J Am Thyroid Assoc. (2017) 27:315–89. doi: 10.1089/thy.2016.0457
31. Poppe K, Bisschop P, Fugazzola L, Minziori G, Unuane D, Weghofer A. 2021 European Thyroid Association Guideline on Thyroid Disorders prior to and during Assisted Reproduction. Eur Thyroid J. (2021) 9:281–95. doi: 10.1159/000512790
32. Teumer A, Chaker L, Groeneweg S, Li Y, Di Munno C, Barbieri C, et al. Genome-wide analyses identify a role for SLC17A4 and AADAT in thyroid hormone regulation. Nat Commun. (2018) 9:4455. doi: 10.1038/s41467-018-06356-1
33. Sterenborg R, Steinbrenner I, Li Y, Bujnis MN, Naito T, Marouli E, et al. Multi-trait analysis characterizes the genetics of thyroid function and identifies causal associations with clinical implications. Nat Commun. (2024) 15:888. doi: 10.1038/s41467-024-44701-9
34. Bowden J, Davey Smith G, Haycock PC, Burgess S. Consistent estimation in mendelian randomization with some invalid instruments using a weighted median estimator. Genet Epidemiol. (2016) 40:304–14. doi: 10.1002/gepi.21965
35. Bowden J, Del Greco MF, Minelli C, Davey Smith G, Sheehan NA, Thompson JR. Assessing the suitability of summary data for two-sample Mendelian randomization analyses using MR-Egger regression: the role of the I2 statistic. Int J Epidemiol. (2016) 45:1961–74. doi: 10.1093/ije/dyw220
36. Hemani G, Zheng J, Elsworth B, Wade KH, Haberland V, Baird D, et al. The MR-Base platform supports systematic causal inference across the human phenome. Elife. (2018) 7. doi: 10.7554/eLife.34408
37. Krassas GE, Poppe K, Glinoer D. Thyroid function and human reproductive health. Endocrine Rev. (2010) 31:702–55. doi: 10.1210/er.2009-0041
38. Zhang C, Xia G, Tsang BK. Interactions of thyroid hormone and FSH in the regulation of rat granulosa cell apoptosis. Front Biosci (Elite Ed). (2011) 3:1401–13. doi: 10.2741/e342
39. Aghajanova L, Lindeberg M, Carlsson IB, Stavreus-Evers A, Zhang P, Scott JE, et al. Receptors for thyroid-stimulating hormone and thyroid hormones in human ovarian tissue. Reprod Biomed Online. (2009) 18:337–47. doi: 10.1016/s1472-6483(10)60091-0
40. Kabodmehri R, Sharami SH, Sorouri ZZ, Gashti NG, Milani F, Chaypaz Z, et al. The relationship between thyroid function and ovarian reserve: a prospective cross-sectional study. Thyroid Res. (2021) 14:22. doi: 10.1186/s13044-021-00112-2
41. Dumoulin SC, Perret BP, Bennet AP, Caron PJ. Opposite effects of thyroid hormones on binding proteins for steroid hormones (sex hormone-binding globulin and corticosteroid-binding globulin) in humans. Eur J Endocrinol. (1995) 132:594–8. doi: 10.1530/eje.0.1320594
42. Southren AL, Olivo J, Gordon GG, Vittek J, Brener J, Rafii F. The conversion of androgens to estrogens in hyperthyroidism. J Clin Endocrinol Metab. (1974) 38:207–14. doi: 10.1210/jcem-38-2-207
43. Ridgway EC, Longcope C, Maloof F. Metabolic clearance and blood production rates of estradiol in hyperthyroidism. J Clin Endocrinol Metab. (1975) 41:491–7. doi: 10.1210/jcem-41-3-491
44. Krysiak R, Marek B, Okopień B. Sexual function and depressive symptoms in men with overt hyperthyroidism. Endokrynologia Polska. (2019) 70:64–71. doi: 10.5603/EP.a2018.0069
45. Krassas GE, Pontikides N. Male reproductive function in relation with thyroid alterations. Best Pract Res Clin Endocrinol Metab. (2004) 18:183–95. doi: 10.1016/j.beem.2004.03.003
46. Ourique GM, Finamor IA, Saccol EM, Riffel AP, Pês TS, Gutierrez K, et al. Resveratrol improves sperm motility, prevents lipid peroxidation and enhances antioxidant defences in the testes of hyperthyroid rats. Reprod Toxicol (Elmsford NY). (2013) 37:31–9. doi: 10.1016/j.reprotox.2013.01.006
47. Unuane D, Velkeniers B. Impact of thyroid disease on fertility and assisted conception. Best Pract Res Clin Endocrinol Metab. (2020) 34:101378. doi: 10.1016/j.beem.2020.101378
48. Raber W, Nowotny P, Vytiska-Binstorfer E, Vierhapper H. Thyroxine treatment modified in infertile women according to thyroxine-releasing hormone testing: 5 year follow-up of 283 women referred after exclusion of absolute causes of infertility. Hum Reprod (Oxford England). (2003) 18:707–14. doi: 10.1093/humrep/deg142
49. Feldthusen AD, Pedersen PL, Larsen J, Toft Kristensen T, Ellervik C, Kvetny J. Impaired fertility associated with subclinical hypothyroidism and thyroid autoimmunity: the danish general suburban population study. J pregnancy. (2015) 2015:132718. doi: 10.1155/2015/132718
50. Abalovich M, Mitelberg L, Allami C, Gutierrez S, Alcaraz G, Otero P, et al. Subclinical hypothyroidism and thyroid autoimmunity in women with infertility. Gynecological endocrinology: Off J Int Soc Gynecol Endocrinol. (2007) 23:279–83. doi: 10.1080/09513590701259542
51. Hiraoka T, Wada-Hiraike O, Hirota Y, Hirata T, Koga K, Osuga Y, et al. The impact of elevated thyroid stimulating hormone on female subfertility. Reprod Med Biol. (2016) 15:121–6. doi: 10.1007/s12522-015-0221-9
52. Abdel Rahman AH, Aly Abbassy H, Abbassy AA. Improved in vitro fertilization outcomes after treatment of subclinical hypothyroidism in infertile women. Endocrine practice: Off J Am Coll Endocrinol Am Assoc Clin Endocrinologists. (2010) 16:792–7. doi: 10.4158/ep09365.Or
53. Kim CH, Ahn JW, Kang SP, Kim SH, Chae HD, Kang BM. Effect of levothyroxine treatment on in vitro fertilization and pregnancy outcome in infertile women with subclinical hypothyroidism undergoing in vitro fertilization/intracytoplasmic sperm injection. Fertil Steril. (2011) 95:1650–4. doi: 10.1016/j.fertnstert.2010.12.004
54. Abalovich M, Gutierrez S, Alcaraz G, Maccallini G, Garcia A, Levalle O. Overt and subclinical hypothyroidism complicating pregnancy. Thyroid: Off J Am Thyroid Assoc. (2002) 12:63–8. doi: 10.1089/105072502753451986
55. Sarkar D. Recurrent pregnancy loss in patients with thyroid dysfunction. Indian J Endocrinol Metab. (2012) 16:S350–1. doi: 10.4103/2230-8210.104088
56. Kucukler FK, Gorkem U, Simsek Y, Kocabas R, Guler S. Evaluation of ovarian reserve in women with overt or subclinical hypothyroidism. Arch Med Sci: AMS. (2018) 14:521–6. doi: 10.5114/aoms.2016.58621
57. d'Assunção VRN, Montagna E, d'Assunção LEN, Caldas MMP, Christofolini DM, Barbosa CP, et al. Effect of thyroid function on assisted reproduction outcomes in euthyroid infertile women: A single center retrospective data analysis and a systematic review and meta-analysis. Front Endocrinol. (2022) 13:1023635. doi: 10.3389/fendo.2022.1023635
58. Birjandi B, Ramezani Tehrani F, Amouzegar A, Tohidi M, Bidhendi Yarandi R, Azizi F. The association between subclinical hypothyroidism and TPOAb positivity with infertility in a population-based study: Tehran thyroid study (TTS). BMC Endocrine Disord. (2021) 21:108. doi: 10.1186/s12902-021-00773-y
59. Cai Y, Zhong L, Guan J, Guo R, Niu B, Ma Y, et al. Outcome of in vitro fertilization in women with subclinical hypothyroidism. Reprod Biol Endocrinol: RB&E. (2017) 15:39. doi: 10.1186/s12958-017-0257-2
60. Negro R, Mangieri T, Coppola L, Presicce G, Casavola EC, Gismondi R, et al. Levothyroxine treatment in thyroid peroxidase antibody-positive women undergoing assisted reproduction technologies: a prospective study. Hum Reprod (Oxford England). (2005) 20:1529–33. doi: 10.1093/humrep/deh843
61. Andersen SL, Olsen J, Wu CS, Laurberg P. Spontaneous abortion, stillbirth and hyperthyroidism: a danish population-based study. Eur Thyroid J. (2014) 3:164–72. doi: 10.1159/000365101
62. Joshi JV, Bhandarkar SD, Chadha M, Balaiah D, Shah R. Menstrual irregularities and lactation failure may precede thyroid dysfunction or goitre. J postgraduate Med. (1993) 39:137–41.
63. Peyneau M, Kavian N, Chouzenoux S, Nicco C, Jeljeli M, Toullec L, et al. Role of thyroid dysimmunity and thyroid hormones in endometriosis. Proc Natl Acad Sci USA. (2019) 116:11894–9. doi: 10.1073/pnas.1820469116
64. Saccardi C, Gizzo S, Ludwig K, Guido M, Scarton M, Gangemi M, et al. Endometrial polyps in women affected by levothyroxine-treated hypothyroidism–histological features, immunohistochemical findings, and possible explanation of etiopathogenic mechanism: a pilot study. BioMed Res Int. (2013) 2013:503419. doi: 10.1155/2013/503419
Keywords: thyroid dysfunction, thyroid-stimulating hormone, free tetraiodothyronine, infertility, Mendelian randomization
Citation: Liu Q, Qiu Y, Jiang J, Long S, Zhu C, Chen G and Wen J (2024) Causal association between thyroid function and the risk of infertility: a Mendelian randomization study. Front. Endocrinol. 15:1425639. doi: 10.3389/fendo.2024.1425639
Received: 30 April 2024; Accepted: 16 September 2024;
Published: 04 October 2024.
Edited by:
Jung Ryeol Lee, Seoul National University, Republic of KoreaReviewed by:
Sanja Medenica, Clinical Center of Montenegro, MontenegroCopyright © 2024 Liu, Qiu, Jiang, Long, Zhu, Chen and Wen. This is an open-access article distributed under the terms of the Creative Commons Attribution License (CC BY). The use, distribution or reproduction in other forums is permitted, provided the original author(s) and the copyright owner(s) are credited and that the original publication in this journal is cited, in accordance with accepted academic practice. No use, distribution or reproduction is permitted which does not comply with these terms.
*Correspondence: Junping Wen, anVucGluZ3dlbkAxNjMuY29t; Gang Chen, Y2hlbmdhbmdmakAxNjMuY29t
†These authors have contributed equally to this work
Disclaimer: All claims expressed in this article are solely those of the authors and do not necessarily represent those of their affiliated organizations, or those of the publisher, the editors and the reviewers. Any product that may be evaluated in this article or claim that may be made by its manufacturer is not guaranteed or endorsed by the publisher.
Research integrity at Frontiers
Learn more about the work of our research integrity team to safeguard the quality of each article we publish.