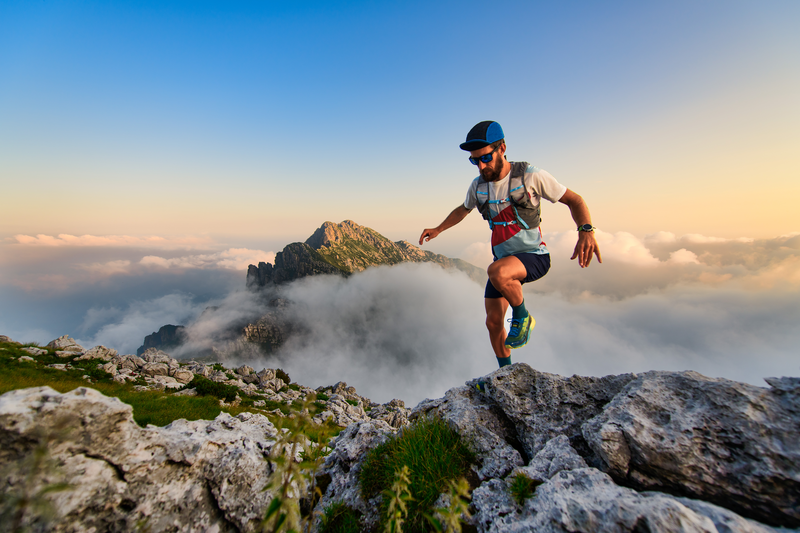
94% of researchers rate our articles as excellent or good
Learn more about the work of our research integrity team to safeguard the quality of each article we publish.
Find out more
ORIGINAL RESEARCH article
Front. Endocrinol. , 17 September 2024
Sec. Renal Endocrinology
Volume 15 - 2024 | https://doi.org/10.3389/fendo.2024.1424068
Objectives: The effects of nicotine metabolism on the kidneys of healthy individuals have not been determined. The nicotine metabolite ratio (NMR) indicates the rate of nicotine metabolism and is linked to smoking behaviors and responses to tobacco treatments. We conducted this study in order to investigated the relationship between nicotine metabolite ratio (NMR) and kidney function.
Methods: An analysis of cross-sectional data of adults was conducted using a population survey dataset (National Health and Nutrition Examination Survey Data 2013/2018 of the United States). A weighted multivariate regression analysis was conducted to estimate the correlation between NMR and kidney function. Furthermore, we apply fitting smooth curves to make the relationship between NMR and estimated glomerular filtration rate (eGFR) more visualized.
Results: The research included a total of 16153 participants. Weighted multivariate regression analyses adjusted for possible variables showed a negative relationship between NMR and estimated glomerular filtration rate (eGFR).The β (95%CI) of the regression equation between NMR and eGFR was -2.24 (-2.92, -1.55), the trend testing showed consistent results. NMR is positively correlated with urinary albumin creatinine ratio (uACR), but it is not statistically significant. A stratified analysis found a negative correlation between NMR and eGFR in all age, gender and diabetes subgroups, the results were not statistically significant among Mexican Americans and other races. Notably, each unit rise in NMR corresponded to a 4.54 ml/min·1.73m² lower eGFR in diabetic participants and a 6.04 ml/min·1.73m² lower eGFR in those aged 60 and above.
Conclusions: Our study shows that nicotine metabolite ratio is negatively associated with kidney function among most adults. It will be necessary to conduct more well-designed prospective clinical trials in order to determine the exact causal interactions between NMR and kidney function. Specific mechanisms also need to be further explored in basic experiments.
Recent changes in natural and social environments have altered people’s lifestyles. The rise in metabolic diseases highlights the ongoing concern about how human behavior affects physical health. Chronic kidney disease is linked to metabolism, and smoking-induced metabolic changes impacting physiology and pathology are also significant.
Chronic kidney disease (CKD) has become a major public health issue that seriously affects the health of the population. CKD is characterized by declining eGFR and albuminuria (1). There are approximately 10%-15% of people worldwide who suffer from chronic kidney disease, which poses serious public health burden (2). Study confirms that risk factors for CKD include hypertension (3), diabetes mellitus (4), overweight (5), COVID-19 infections (6), nephrotoxic drugs, ageing (7), and air pollution exposure (8).
In 2019, smoking tobacco accounted for 769 million deaths and 200 million disability-adjusted life years, and it was the leading cause of death among men (9). A 2021 survey indicates that approximately 18.7% of American adults currently use tobacco products. Within this demographic, 11.5% use cigarettes, 4.5% use electronic cigarettes, 3.5% use cigars, 2.1% use smokeless tobacco, and 0.9% use pipes (10). Smoking has been identified as a modifiable risk factor contributing to mortality in various diseases, including malignancies, cardiovascular diseases, respiratory diseases, and chronic kidney disease (CKD) (11–13). Research indicates that the link between tobacco use and kidney function is debated. Most studies show smoking harms kidney function, but a few do not find any connection.
Nicotine, the primary agent responsible for smoking addiction, is released and swiftly absorbed in the lungs, subsequently entering the brain where it binds to nicotinic acetylcholine receptors (nAChRs) in neural tissue (14). Nicotine exerts its effects through the activation of nicotinic acetylcholine receptors (nAChRs). Various non-neuronal cell types, including renal cells (such as podocytes, tubular epithelial cells, and mesangial cells), endothelial cells, and smooth muscle cells within the vascular system, are known to express nAChRs (15, 16). Research indicates that nicotine reduces kidney cell viability, induces the production of reactive oxygen species (ROS), and upregulates the expression of the α7 nicotinic acetylcholine receptor (7nAChR) (17). Nicotine exhibits a high affinity for brain tissues in individuals who smoke, thereby enhancing their receptor binding capacity (18). In autopsy samples obtained from human smokers, the kidneys exhibit a pronounced affinity for nicotine (19). Nicotine inhibits the proliferation of HK-2 cells through modulation of the AKT signaling pathway (20). By increasing ROS levels, forming stem cell-like spheres, and altering EMT, chronic nicotine exposure caused HK-2 cells to grow and become cancerous (21).
Nicotine is metabolized in the liver by cytochrome P4502A6, producing cotinine and hydroxycotinine (22). The accuracy of assessing smoking exposure through self-reported smoking habits is limited. Objective measurement of smoking levels can be achieved by quantifying cotinine, a metabolite of nicotine, in biological specimens such as saliva, plasma, and urine. Cotinine and hydroxycotinine are more frequently utilized as biomarkers for active smoking and exposure to tobacco smoke due to their higher potency and extended elimination half-life. The Nicotine Metabolite Ratio (NMR), defined as the ratio of hydroxycotinine (HC) to cotinine (COT), is a significant biomarker in the study of nicotine dependence. A higher NMR is indicative of a more rapid metabolism of nicotine, which is associated with increased levels of nicotine dependence (23–25). As a result, NMR can be used to estimate the risk of tobacco-related diseases. Prior research examining the association between tobacco exposure and renal impairment has predominantly relied on self-reported smoking behaviors. In this study, the nicotine metabolite ratio, utilized as an exposure factor, demonstrated greater accuracy, objectivity, and reliability compared to self-reported smoking data. The nicotine metabolite ratio, indicative of the rate of nicotine metabolism, reflects the degree of nicotine dependence in the human body. This metric may, to some extent, encourage researchers to investigate the intrinsic relationship between smoking and kidney disease at both metabolic and genetic levels.
In this study, we investigated whether there is an association between nicotine metabolite ratio (NMR) and renal function (eGFR and urinary albumin creatinine ratio) among individuals aged 18 and above residing in the United States.
The NHANES database collects health information and nutrition data of the United States population. The database, which began in the 1980s, updates its data every two years in a cycle and is free and open to the public, allowing researchers to download the data directly as needed. The biological samples contain serum, plasma, and urine from the participants and cover a wide range of measures. It also contains data from a large number of questionnaires covering a wide range of demographics, socio-economics, dietary and health concerns, and physical examination including physiological and body measurements, laboratory tests, and more (26). We used NHANES data from 2013-2018 with 29350 participants. 11398 people for being aged < 18y, 1717 with missing hydroxycotinine and cotinine values, 88 participants with serum creatinine unavailable were excluded. 3 excluded because extreme large NMR values. Ultimately, 16153 participants involved in the analyses (Figure 1).
Figure 1. Study flowchart. Flowchart showing study selection. Of 29350 participants in the 2013 to 2018 National Health and Nutrition Examination Survey(NHANES), 16153 remained after fulfilling inclusion and exclusion criteria.
The measurements of serum cotinine and hydroxycotinine are performed using an isotope-dilution high-performance liquid chromatography-atmospheric pressure chemical ionization tandem mass spectrometry (ID HPLC-APCI MS/MS). Additional details of the protocol were previously described (27). The determination of serum creatinine is by enzymatic methods. We calculated eGFR by the CKD-EPI creatinine formula which is based on serum creatinine levels as well as age and race. Research confirms that the CKD-EPI equation outperforms the MDRD equation in the presence of higher GFR, with less bias, more precision and accuracy (28). The following variable were included in our analysis as covariates: age, gender, race, education, BMI, systolic pressure, diastolic pressure, total cholesterol, serum albumin, uric acid, diabetes, CVD, stroke. The CVD was obtained from the medical conditions questionnaire. Those who answered “YES” to the following question “Have you ever been told by a physician or a health professional that you had stroke?” was considered having stroke. The study defined diabetes mellitus as self-diagnosed by a physician, taking oral hypoglycemics, or using insulin supplements, or fasting blood glucose greater than 7.0mmol/L. The detailed information can be found at http://www.cdc.gov/nchs/nhanes/.
EmpowerStats (http://www.empowerstats.com) was used for statistical analyses. We conducted a weighted analysis and variance estimation to address the significant variability present in our dataset. We express categorical variables as frequency and percentage distributions (n%). The continuous variables in this study deviate from a normal distribution; therefore, their central tendency and dispersion are represented using the median and interquartile range. For multiple comparisons, the weighted χ² test was used for categorical variables, and the weighted linear regression for continuous variables. A weighted multivariate regression analysis was conducted to estimate the correlation between NMR and kidney function (eGFR and urinary albumin creatinine ratio). The subgroup analysis was succeeded by stratified multivariate regression analyses. To account for the nonlinear relationship between NMR and kidney function, smooth curve fittings were employed. P value less than 0.05 was considered statistically significant.
A total of 16153 participants, aged 18 to 80 years, were included in our analysis. The weighted characteristics of the participants were subclassified according to NMR quartiles, as presented in Table 1. Significant differences in baseline characteristics were observed across the NMR quartiles. Participants in the highest NMR quartile, compared to those in other subgroups, were more likely to be female and exhibited higher levels of total cholesterol and educational attainment, as well as lower levels of uric acid and estimated glomerular filtration rate (eGFR). The high NMR group is older with a higher proportion of diabetes patients.
Table 1. Baseline characteristics of study participants from 2013 to 2018 National Health and Nutrition Examination Survey.
In this study, three models—unadjusted, simple, and fully adjusted—were developed to examine the interaction between nicotine metabolite ratio (NMR) and kidney function, as measured by estimated glomerular filtration rate (eGFR) and the urinary albumin creatinine ratio (uACR) (Table 2). Model 2 adjusts for age, gender, and race. Model 3 further includes education, blood pressure, BMI, cholesterol, uric acid, serum albumin, diabetes, CVD, and stroke. In the unadjusted model, a negative association was observed between NMR and eGFR (β = -14.17, 95% CI: -15.13, -13.22, P < 0.00001). This inverse relationship persisted in model 2 (β = -2.87, 95% CI: -3.59, -2.16, P < 0.00001) and model 3 (β = -2.24, 95% CI: -2.92, -1.55, P < 0.00001).We subsequently transformed the NMR data into categorical variables based on quartiles, and trend analysis yielded consistent results. NMR is positively correlated with uACR, but it is not statistically significant. A smooth curve is presented in Figure 2, illustrating the relationship between NMR and eGFR after adjusting for all relevant variables. Figure 3 depicts the regression coefficient β of eGFR across deciles of NMR levels, following comprehensive adjustment for confounding variables.
Figure 2. Figure 2 is based on Model 3. The association between NMR and kidney function. (A, C) Each black point represent a sample. The red line represents the curve fitted based on the scatter plot. (B, D) Red line represents the smooth curve fit between variables. Blue bands represent the 95% of confidence interval from the fit. Age, gender, race, education, body mass index, systolic pressure, diastolic pressure, total cholesterol, uric acid, serum albumin, diabetes, CVD, stroke were adjusted.
Figure 3. Regression coefficient β of eGFR by decile of serum NMR level plot. Showing regression coefficient β of eGFR by decile of serum NMR level after adjustment for age, gender, race, body mass index, education, systolic pressure, total cholesterol, uric acid, serum albumin, diabetes, CVD, stroke. The vertical lines indicate 95% CIs.
NMR-eGFR relationships stratified by age, gender, race, diabetes are shown in Figure 4. The negative correlation of NMR with eGFR remained significant among all age groups, aged < 40 (β = -1.97, 95%CI: -3.20, -0.74, P = 0.0017), 40-60 (β = -2.63, 95%CI: -3.89, -1.37, P < 0.0001), aged ≥ 60 years (β = -6.04, 95%CI: -7.27, -4.80, P < 0.0001), male (β = -2.13, 95%CI: -3.13, -1.23, P < 0.0001), female (β = -2.43, 95%CI: -3.36, -1.49, P < 0.0001), White people (β = -2.79, 95%CI: -3.74, -1.73, P < 0.0001), Black people (β = -3.00, 95%CI: -5.04, -0.96, P = 0.0040), non-diabetic(β = -1.79, 95%CI: -2.52, -1.07, P < 0.0001), diabetic (β = -4.54, 95%CI: -6.61, -2.47, P < 0.0001).It is particularly significant that among participants with diabetes and those aged ≥ 60 years, each unit increase in NMR was associated with a reduction in eGFR by 4.54 ml/min/1.73m² and 6.04 ml/min/1.73m², respectively.
Figure 4. Subgroup Analysis. Forest plot showing the association between NMR and eGFR for various subgroups of participants aged ≥ 18 years in the 2013 to 2018 National Health and Nutrition Examination Survey. Race/ethnicity was self - defined in a written questionnaire. Options were prespecified by the National Health and Nutrition Examination Survey Investigators.
As far as we know, this is the first large-scale research to examine the relationship between nicotine metabolite rate and kidney function. Over 480,000 Americans die each year from smoking. Heart attacks and strokes are two to four times more likely to occur for smokers (29). Smoking increases the risk of atherosclerosis, which leads to narrowing of blood vessels and hypercoagulability, which in turn increases thrombosis (30, 31). Cotinine, hydroxycotinine, and glucuronides account for 85-95% of nicotine excretion in urine (32, 33). According to our findings, NMR is a risk factor for renal impairment. Measurement of NMR levels may therefore be involved in screening models for chronic kidney disease as well as prognostic models to guide therapeutic interventions.
It is biologically plausible that tobacco exposure might interact with kidney function. Cigarette smoking can elevate carboxyhemoglobin levels, platelet activation, and prothrombotic factors (34). As a result, the kidneys experience inflammation, oxidative stress, and endothelial cell dysfunction (35, 36). In addition, smoking induces proteinuria and kidney function abnormalities through advanced glycation end - products, which increases vascular permeability (37). The principal mechanisms underlying the interaction between smoking and the onset and progression of chronic kidney disease in adults include glomerulosclerosis, oxidative stress, endothelial damage leading to mesangial proliferation, and tubulointerstitial fibrosis (36, 38, 39). Research showed nicotine promoted podocyte apoptosis by activating MAPK kinases and enhancing oxidative stress (40). According to recent studies, nicotine stimulation has been shown to induce apoptosis or epithelial-mesenchymal transition in renal tubular epithelial cells through the upregulation of reactive oxygen species (ROS) production (41). In mesangial cells, nicotine activates TGF- or Wnt/-catenin pathways, promoting cell growth and production of extracellular matrix (42, 43). A recent study has demonstrated that nicotine exerts deleterious effects on renal function by modulating the 7nAChR, NLRP6 inflammasome, endoplasmic reticulum (ER) stress, and autophagy pathways (17).
Slow nicotine metabolizers exhibit lower levels of inflammation (ICAM-1 and PGEM) compared to fast metabolizers, which is linked to kidney damage (44). Various cell types exhibit responses to ICAM-1, which serves as a marker for inflammation and chronic disease. (45). NMR serves as an indicator for both exposure to smoke and the biological impact of tobacco. A comprehensive review revealed that individuals who metabolize nicotine at a normal or rapid rate tend to smoke more cigarettes per day compared to those who metabolize it slowly (46). Having a higher NMR indicates greater exposure to kidney-damaging substances from smoking, such as volatile organic compounds and polycyclic aromatic hydrocarbons. Considering that the ratio of nicotine metabolites represent CYP2A6 activity, kidney injury may induce CYP2A6 enzyme activity, leading to an increase in nicotine metabolism rate. Limited knowledge exists regarding the impact of renal pathological changes on other pharmacokinetic parameters in relation to the NMR. Consequently, caution is advised when interpreting kidney-induced increases in the NMR as direct indicators of enhanced CYP2A6 activity.
In our study, a negative relationship was found between NMR and kidney function of 16153 survey participants. Our research results regarding glomerular filtration rate are consistent with previous studies. With increasing NMR, eGFR levels declined significantly. However, our study did not find a correlation between NMR and urinary albumin creatinine ratio. CKD incidence rate was significantly higher for smokers (47, 48). A prospective cohort study identified a vertical relationship between tobacco exposure and albuminuria, independent of diabetes (49). Further, prospective studies have shown that smoking contributes to impairment of kidney function in idiopathic membranous nephropathy (50) and IgA nephropathy (51, 52). In general healthy populations, the effect of smoking on kidney function is unclear. Smoking is associated with CKD, characterized by proteinuria or declining glomerular filtration rate, according to a study published in 2000 (53). A study showed the eGFR changes in smoking subjects were significantly smaller than those in non-smoking subjects (54). The risk of albuminuria was higher in smokers in a cross-sectional study (55). Among patients with stage III-V CKD, a 50% reduction in eGFR or the development of ESKD were not independently associated with current and former smokers (56). It has also been shown that smoking status does not correlate with the presence of proteinuria (57).
As recommended by the STROBE statement, we conducted further subgroup analyses to preferably represent the data set (58). Our findings indicated that a higher NMR was associated with a lower eGFR in both men and women. A study showed end-stage renal disease development was associated with smoking status in men, not in women (59). In addition, according to the subgroup analysis of racial stratification, NMR and eGFR are negatively correlated in White people and Black people, and the relationship is statistically significant, but not in Mexican American and other race. Race-specific differences may be explained by genetic risk factors, obesity status, and other factors. The association was more pronounced among older and diabetic participants. The results of this study were in accordance with previous studies suggesting smoking cigarettes was a significant risk factor for CKD in diabetic patients. A study from South Korea revealed that persistent smokers were 2.17 times far more likely to develop diabetic kidney disease than non-smokers (60). A similar conclusion was drawn in a study of people with type 1 diabetes, which estimated the 12-year cumulative risk of microalbuminuria, macroalbuminuria, and ESRD in current smokers, former smokers, and non-smokers (61).
Since we employed a national sample, our research can to some extent represent the population. The limitation of our study cannot be ignored. As a cross-sectional design, our research limits the inference of a causal relationship between NMR and kidney function. It is therefore necessary to conduct basic pharmacological studies and large-scale prospective studies in order to determine exactly how NMR influences kidney function. Furthermore, As a secondary analysis, we were not able to collect new data, so unmeasured covariates could potentially lead to residual confounding. Due to the fact that this study utilized a database that only consisted of patients from the United States, caution should be exercised when generalizing the findings to populations from other ethnicities. In view of the significant effect NMR has on renal function in elderly individuals and patients with diabetes, it may be possible to reduce the burden on these populations by regulating smoking within an appropriate range. However, the limitations of our research design preclude further elucidation of this matter.
According to our study, a negative correlation between NMR and kidney function was found. The correlation is particularly pronounced in individuals over the age of 60 and within the diabetic subgroup. Thus, Nicotine Metabolite Ratio (NMR), which indicates an individual’s rate of nicotine metabolism and is known to be detrimental to various physiological systems, may also impair renal function in adults. Smoking must be addressed in order to improve kidney health and reduce the risk of related health problems, particularly for elderly and diabetic patients. As part of chronic kidney disease management, healthcare providers should assess smoking status and provide support for quitting or reducing smoking. In addition, further research is needed on the pathological effects of nicotine or nicotine metabolism on the kidneys.
The survey data are publicly available on the Internet for data users and researchers throughout the world: https://www.cdc.gov/nchs/nhanes/index.htm.
The studies involving humans were approved by NCHS Ethics Review Board (ERB). The studies were conducted in accordance with the local legislation and institutional requirements. The participants provided their written informed consent to participate in this study. Written informed consent was obtained from the individual(s) for the publication of any potentially identifiable images or data included in this article.
XW: Writing – original draft. SS: Writing – review & editing.
The author(s) declare financial support was received for the research, authorship, and/or publication of this article. This study was supported by Qilu Traditional Chinese Medicine Advantage Specialist Cluster Project and Shandong Traditional Chinese Medicine Technology Project (Z-2023062T).
The authors declare that the research was conducted in the absence of any commercial or financial relationships that could be construed as a potential conflict of interest.
All claims expressed in this article are solely those of the authors and do not necessarily represent those of their affiliated organizations, or those of the publisher, the editors and the reviewers. Any product that may be evaluated in this article, or claim that may be made by its manufacturer, is not guaranteed or endorsed by the publisher.
1. Jha V, Garcia-Garcia G, Iseki K, Li Z, Naicker S, Plattner B, et al. Chronic kidney disease: global dimension and perspectives. Lancet. (2013) 382:260–72. doi: 10.1016/s0140-6736(13)60687-x
2. Altamura S, Pietropaoli D, Lombardi F, Del Pinto R, Ferri C. An overview of chronic kidney disease pathophysiology: the impact of gut dysbiosis and oral disease. Biomedicines. (2023) 11:3033. doi: 10.3390/biomedicines11113033
3. Drawz PE, Beddhu S, Kramer HJ, Rakotz M, Rocco MV, Whelton PK. Blood pressure measurement: A KDOQI perspective. Am J Kidney Dis. (2020) 75:426–34. doi: 10.1053/j.ajkd.2019.08.030
4. Dykes K, Desale S, Javaid B, Miatlovich K, Kessler C. A new reality for multiple myeloma renal failure: US data report on kidney transplant outcomes. Clin Lymphoma Myeloma Leuk. (2022) 22:e314–20. doi: 10.1016/j.clml.2021.11.002
5. Panwar B, Hanks LJ, Tanner RM, Muntner P, Kramer H, McClellan WM, et al. Obesity, metabolic health, and the risk of end-stage renal disease. Kidney Int. (2015) 87:1216–22. doi: 10.1038/ki.2014.384
6. Schmidt-Lauber C, Hänzelmann S, Schunk S, Petersen EL, Alabdo A, Lindenmeyer M, et al. Kidney outcome after mild to moderate COVID-19. Nephrol Dial Transplant. (2023) 38:2031–40. doi: 10.1093/ndt/gfad008
7. Prakash S, O'Hare AM. Interaction of aging and chronic kidney disease. Semin Nephrol. (2009) 29:497–503. doi: 10.1016/j.semnephrol.2009.06.006
8. Ye JJ, Wang SS, Fang Y, Zhang XJ, Hu CY. Ambient air pollution exposure and risk of chronic kidney disease: A systematic review of the literature and meta-analysis. Environ Res. (2021) 195:110867. doi: 10.1016/j.envres.2021.110867
9. Reitsma MB, Kendrick PJ, Ababneh E, Abbafati C, Abbasi-Kangevari M, Abdoli A, et al. Spatial, temporal, and demographic patterns in prevalence of smoking tobacco use and attributable disease burden in 204 countries and territories, 1990-2019: a systematic analysis from the Global Burden of Disease Study 2019. Lancet. (2021) 397:2337–60. doi: 10.1016/s0140-6736(21)01169-7
10. Cornelius ME, Loretan CG, Jamal A, Davis Lynn BC, Mayer M, Alcantara IC, et al. Tobacco product use among adults - United States, 2021. MMWR Morb Mortal Wkly Rep. (2023) 72:475–83. doi: 10.15585/mmwr.mm7218a1
11. Doll R, Peto R, Boreham J, Sutherland I. Mortality in relation to smoking: 50 years' observations on male British doctors. Bmj. (2004) 328:1519. doi: 10.1136/bmj.38142.554479.AE
12. Doll R, Peto R, Wheatley K, Gray R, Sutherland I. Mortality in relation to smoking: 40 years' observations on male British doctors. Bmj. (1994) 309:901–11. doi: 10.1136/bmj.309.6959.901
13. Pirie K, Peto R, Reeves GK, Green J, Beral V. The 21st century hazards of smoking and benefits of stopping: a prospective study of one million women in the UK. Lancet. (2013) 381:133–41. doi: 10.1016/s0140-6736(12)61720-6
14. Wittenberg RE, Wolfman SL, De Biasi M, Dani JA. Nicotinic acetylcholine receptors and nicotine addiction: A brief introduction. Neuropharmacology. (2020) 177:108256. doi: 10.1016/j.neuropharm.2020.108256
15. Rezonzew G, Chumley P, Feng W, Hua P, Siegal GP, Jaimes EA. Nicotine exposure and the progression of chronic kidney disease: role of the α7-nicotinic acetylcholine receptor. Am J Physiol Renal Physiol. (2012) 303:F304–12. doi: 10.1152/ajprenal.00661.2011
16. Chen J, Xiao H, Xue R, Kumar V, Aslam R, Mehdi SF, et al. Nicotine exacerbates diabetic nephropathy through upregulation of Grem1 expression. Mol Med. (2023) 29:92. doi: 10.1186/s10020-023-00692-9
17. Zheng CM, Lee YH, Chiu IJ, Chiu YJ, Sung LC, Hsu YH, et al. Nicotine causes nephrotoxicity through the induction of NLRP6 inflammasome and alpha7 nicotinic acetylcholine receptor. Toxics. (2020) 8:92. doi: 10.3390/toxics8040092
18. Breese CR, Marks MJ, Logel J, Adams CE, Sullivan B, Collins AC, et al. Effect of smoking history on [3H]nicotine binding in human postmortem brain. J Pharmacol Exp Ther. (1997) 282:7–13.
19. Benowitz NL, Hukkanen J, Jacob P 3rd. Nicotine chemistry, metabolism, kinetics and biomarkers. Handb Exp Pharmacol. (2009) 192:29–60. doi: 10.1007/978-3-540-69248-5_2
20. Chang YW, Singh KP. Duration-dependent effects of nicotine exposure on growth and AKT activation in human kidney epithelial cells. Mol Cell Biochem. (2018) 448:51–60. doi: 10.1007/s11010-018-3312-1
21. Chang YW, Singh KP. Nicotine-induced oxidative stress contributes to EMT and stemness during neoplastic transformation through epigenetic modifications in human kidney epithelial cells. Toxicol Appl Pharmacol. (2019) 374:65–76. doi: 10.1016/j.taap.2019.04.023
22. Nakajima M, Yamamoto T, Nunoya K, Yokoi T, Nagashima K, Inoue K, et al. Characterization of CYP2A6 involved in 3'-hydroxylation of cotinine in human liver microsomes. J Pharmacol Exp Ther. (1996) 277:1010–5.
23. Dempsey D, Tutka P, Jacob P 3rd, Allen F, Schoedel K, Tyndale RF, et al. Nicotine metabolite ratio as an index of cytochrome P450 2A6 metabolic activity. Clin Pharmacol Ther. (2004) 76:64–72. doi: 10.1016/j.clpt.2004.02.011
24. Johnstone E, Benowitz N, Cargill A, Jacob R, Hinks L, Day I, et al. Determinants of the rate of nicotine metabolism and effects on smoking behavior. Clin Pharmacol Ther. (2006) 80:319–30. doi: 10.1016/j.clpt.2006.06.011
26. Levy RV, Brathwaite KE, Sarathy H, Reidy K, Kaskel FJ, Melamed ML. Analysis of active and passive tobacco exposures and blood pressure in US children and adolescents. JAMA Netw Open. (2021) 4:e2037936. doi: 10.1001/jamanetworkopen.2020.37936
27. Jacob P 3rd, Yu L, Wilson M, Benowitz NL. Selected ion monitoring method for determination of nicotine, cotinine and deuterium-labeled analogs: absence of an isotope effect in the clearance of (S)-nicotine-3',3'-d2 in humans. Biol Mass Spectrom. (1991) 20:247–52. doi: 10.1002/bms.1200200503
28. Levey AS, Stevens LA, Schmid CH, Zhang YL, Castro AF 3rd, Feldman HI, et al. A new equation to estimate glomerular filtration rate. Ann Intern Med. (2009) 150:604–12. doi: 10.7326/0003-4819-150-9-200905050-00006
29. Son YB, Kim TB, Min HJ, Yang J, Kim MG, Jo SK, et al. Smoking amplifies the risk of albuminuria in individuals with high sodium intake: the Korea National Health and Nutrition Examination Survey (KNHANES) 2008-2011 and 2014-2018. Kidney Res Clin Pract. (2023). doi: 10.23876/j.krcp.22.133
30. Ambrose JA, Barua RS. The pathophysiology of cigarette smoking and cardiovascular disease: an update. J Am Coll Cardiol. (2004) 43:1731–7. doi: 10.1016/j.jacc.2003.12.047
31. Centers for Disease C, Prevention, National Center for Chronic Disease P, Health P, Office on S, Health. Publications and Reports of the Surgeon General. How Tobacco Smoke Causes Disease: The Biology and Behavioral Basis for Smoking-Attributable Disease: A Report of the Surgeon General. Atlanta (GA): Centers for Disease Control and Prevention (US); (2010). eng.
32. Yamazaki H, Horiuchi K, Takano R, Nagano T, Shimizu M, Kitajima M, et al. Human blood concentrations of cotinine, a biomonitoring marker for tobacco smoke, extrapolated from nicotine metabolism in rats and humans and physiologically based pharmacokinetic modeling. Int J Environ Res Public Health. (2010) 7:3406–21. doi: 10.3390/ijerph7093406
33. Nakajima M, Yokoi T. Interindividual variability in nicotine metabolism: C-oxidation and glucuronidation. Drug Metab Pharmacokinet. (2005) 20:227–35. doi: 10.2133/dmpk.20.227
34. Barua RS, Ambrose JA. Mechanisms of coronary thrombosis in cigarette smoke exposure. Arterioscler Thromb Vasc Biol. (2013) 33:1460–7. doi: 10.1161/atvbaha.112.300154
35. Caimi G, Hopps E, Montana M, Carollo C, Calandrino V, Incalcaterra E, et al. Nitric oxide metabolites (nitrite and nitrate) in several clinical condition. Clin Hemorheol Microcirc. (2014) 56:359–69. doi: 10.3233/ch-131758
36. Salvatore SP, Troxell ML, Hecox D, Sperling KR, Seshan SV. Smoking-related glomerulopathy: expanding the morphologic spectrum. Am J Nephrol. (2015) 41:66–72. doi: 10.1159/000371727
37. Lan L, Han Y, Ren W, Jiang J, Wang P, Hu Z. Advanced glycation end−products affect the cytoskeletal structure of rat glomerular endothelial cells via the Ras−related C3 botulinum toxin substrate 1 signaling pathway. Mol Med Rep. (2015) 11:4321–6. doi: 10.3892/mmr.2015.3317
38. Nagasawa Y, Yamamoto R, Rakugi H, Isaka Y. Cigarette smoking and chronic kidney diseases. Hypertens Res. (2012) 35:261–5. doi: 10.1038/hr.2011.205
39. Boor P, Casper S, Celec P, Hurbánková M, Beno M, Heidland A, et al. Renal, vascular and cardiac fibrosis in rats exposed to passive smoking and industrial dust fibre amosite. J Cell Mol Med. (2009) 13:4484–91. doi: 10.1111/j.1582-4934.2008.00518.x
40. Lan X, Lederman R, Eng JM, Shoshtari SS, Saleem MA, Malhotra A, et al. Nicotine induces podocyte apoptosis through increasing oxidative stress. PLoS One. (2016) 11:e0167071. doi: 10.1371/journal.pone.0167071
41. Arany I, Reed DK, Grifoni SC, Chandrashekar K, Booz GW, Juncos LA. A novel U-STAT3-dependent mechanism mediates the deleterious effects of chronic nicotine exposure on renal injury. Am J Physiol Renal Physiol. (2012) 302:F722–9. doi: 10.1152/ajprenal.00338.2011
42. Jaimes EA, Tian RX, Joshi MS, Raij L. Nicotine augments glomerular injury in a rat model of acute nephritis. Am J Nephrol. (2009) 29:319–26. doi: 10.1159/000163593
43. Lan X, Wen H, Aslam R, Shoshtari SSM, Mishra A, Kumar V, et al. Nicotine enhances mesangial cell proliferation and fibronectin production in high glucose milieu via activation of Wnt/β-catenin pathway. Biosci Rep. (2018) 38:BSR20180100. doi: 10.1042/bsr20180100
44. Carroll DM, Murphy SE, Benowitz NL, Strasser AA, Kotlyar M, Hecht SS, et al. Relationships between the nicotine metabolite ratio and a panel of exposure and effect biomarkers: findings from two studies of U.S. Commercial cigarette smokers. Cancer Epidemiol Biomarkers Prev. (2020) 29:871–9. doi: 10.1158/1055-9965.Epi-19-0644
45. Frank PG, Lisanti MP. ICAM-1: role in inflammation and in the regulation of vascular permeability. Am J Physiol Heart Circ Physiol. (2008) 295:H926–h927. doi: 10.1152/ajpheart.00779.2008
46. West O, Hajek P, McRobbie H. Systematic review of the relationship between the 3-hydroxycotinine/cotinine ratio and cigarette dependence. Psychopharmacol (Berl). (2011) 218:313–22. doi: 10.1007/s00213-011-2341-1
47. Hallan SI, Orth SR. Smoking is a risk factor in the progression to kidney failure. Kidney Int. (2011) 80:516–23. doi: 10.1038/ki.2011.157
48. Xia J, Wang L, Ma Z, Zhong L, Wang Y, Gao Y, et al. Cigarette smoking and chronic kidney disease in the general population: a systematic review and meta-analysis of prospective cohort studies. Nephrol Dial Transplant. (2017) 32:475–87. doi: 10.1093/ndt/gfw452
49. Meigs JB, D'Agostino RB Sr., Nathan DM, Rifai N, Wilson PW. Longitudinal association of glycemia and microalbuminuria: the Framingham Offspring Study. Diabetes Care. (2002) 25:977–83. doi: 10.2337/diacare.25.6.977
50. Yamaguchi M, Ando M, Yamamoto R, Akiyama S, Kato S, Katsuno T, et al. Smoking is a risk factor for the progression of idiopathic membranous nephropathy. PLoS One. (2014) 9:e100835. doi: 10.1371/journal.pone.0100835
51. Wang S, Qin A, Pei G, Jiang Z, Dong L, Tan J, et al. Cigarette smoking may accelerate the progression of IgA nephropathy. BMC Nephrol. (2021) 22:239. doi: 10.1186/s12882-021-02453-4
52. Yamamoto R, Nagasawa Y, Shoji T, Iwatani H, Hamano T, Kawada N, et al. Cigarette smoking and progression of IgA nephropathy. Am J Kidney Dis. (2010) 56:313–24. doi: 10.1053/j.ajkd.2010.02.351
53. Janssen WM, Hillege H, Pinto-Sietsma SJ, Bak AA, De Zeeuw D, de Jong PE. Low levels of urinary albumin excretion are associated with cardiovascular risk factors in the general population. Clin Chem Lab Med. (2000) 38:1107–10. doi: 10.1515/cclm.2000.165
54. Miyatake N, Moriyasu H, Sakano N, Tada S, Suzue T, Hirao T. Influence of cigarette smoking on estimated glomerular filtration rate (eGFR) in Japanese male workers. Acta Med Okayama. (2010) 64:385–90. doi: 10.18926/amo/41324
55. Pinto-Sietsma SJ, Mulder J, Janssen WM, Hillege HL, de Zeeuw D, de Jong PE. Smoking is related to albuminuria and abnormal renal function in nondiabetic persons. Ann Intern Med. (2000) 133:585–91. doi: 10.7326/0003-4819-133-8-200010170-00008
56. Provenzano M, Serra R, Michael A, Bolignano D, Coppolino G, Ielapi N, et al. Smoking habit as a risk amplifier in chronic kidney disease patients. Sci Rep. (2021) 11:14778. doi: 10.1038/s41598-021-94270-w
57. Jee SH, Boulware LE, Guallar E, Suh I, Appel LJ, Miller ER 3rd. Direct, progressive association of cardiovascular risk factors with incident proteinuria: results from the Korea Medical Insurance Corporation (KMIC) study. Arch Intern Med. (2005) 165:2299–304. doi: 10.1001/archinte.165.19.2299
58. von Elm E, Altman DG, Egger M, Pocock SJ, Gøtzsche PC. The strengthening the reporting of observational studies in epidemiology(STROBE) statement: guidelines for reporting observational studies. Ann Internal Med. (2007) 147:573–7. doi: 10.7326/0003-4819-147-8-200710160-00010
59. Pscheidt C, Nagel G, Zitt E, Kramar R, Concin H, Lhotta K. Sex- and time-dependent patterns in risk factors of end-stage renal disease: A large Austrian cohort with up to 20 years of follow-up. PLoS One. (2015) 10:e0135052. doi: 10.1371/journal.pone.0135052
60. Yeom H, Lee JH, Kim HC, Suh I. The association between smoking tobacco after a diagnosis of diabetes and the prevalence of diabetic nephropathy in the korean male population. J Prev Med Public Health. (2016) 49:108–17. doi: 10.3961/jpmph.15.062
Keywords: non-linear, nicotine metabolite ratio, kidney function, NHANES, cross-sectional research
Citation: Wang X and Su S (2024) The hidden impact: the rate of nicotine metabolism and kidney health. Front. Endocrinol. 15:1424068. doi: 10.3389/fendo.2024.1424068
Received: 10 July 2024; Accepted: 27 August 2024;
Published: 17 September 2024.
Edited by:
Oleg Palygin, Medical University of South Carolina, United StatesReviewed by:
Marharyta Semenikhina, Medical University of South Carolina, United StatesCopyright © 2024 Wang and Su. This is an open-access article distributed under the terms of the Creative Commons Attribution License (CC BY). The use, distribution or reproduction in other forums is permitted, provided the original author(s) and the copyright owner(s) are credited and that the original publication in this journal is cited, in accordance with accepted academic practice. No use, distribution or reproduction is permitted which does not comply with these terms.
*Correspondence: Shanshan Su, c3VzaGFuODIyM0AxNjMuY29t
Disclaimer: All claims expressed in this article are solely those of the authors and do not necessarily represent those of their affiliated organizations, or those of the publisher, the editors and the reviewers. Any product that may be evaluated in this article or claim that may be made by its manufacturer is not guaranteed or endorsed by the publisher.
Research integrity at Frontiers
Learn more about the work of our research integrity team to safeguard the quality of each article we publish.