- 1Department of Biostatistics, School of Public Health, Nanjing Medical University, Nanjing, China
- 2Department of Histology and Embryology, School of Basic Medical Sciences, Nanjing Medical University, Nanjing, China
- 3Innovative Chinese Medicine Research Institute, Engineering Research Center of Modern Preparation Technology of Traditional Chinese Medicine, Shanghai University of Traditional Chinese Medicine, Shanghai, China
- 4State Key Laboratory of Reproductive Medicine and Offspring Health, Nanjing Medical University, Nanjing, China
Background: Polycystic ovary syndrome (PCOS), a prevalent endocrine disorder in women of reproductive age, is mainly ameliorated through drugs or lifestyle changes, with limited treatment options. To date, numerous researchers have found that fertility nutrient supplements may benefit female reproductive health, but their direct impact on polycystic ovary syndrome risk remains unclear.
Methods: Our research employs Mendelian Randomization to assess how fertility nutrients affect PCOS risk. Initially, we reviewed 49 nutrients and focused on 10: omega-3 fatty acids, calcium, dehydroepiandrosterone, vitamin D, betaine, D-Inositol, berberine, curcumin, epigallocatechin gallate, and metformin. Using methodologies of Inverse Variance Weighting and Mendelian Randomization-Egger regression, we examined their potential causal relationships with PCOS risk.
Results: Our findings indicate omega-3 fatty acids reduced PCOS risk (OR=0.73, 95% CI: 0.57-0.94, P=0.016), whereas betaine increased it (OR=2.60, 95% CI: 1.09-6.17, P=0.031). No definitive causal relations were observed for calcium, dehydroepiandrosterone, vitamin D, D-Inositol, and metformin (P>0.05). Drug target Mendelian Randomization analysis suggested that increased expression of the berberine target gene BIRC5 in various tissues may raise PCOS risk (OR: 3.00-4.88; P: 0.014-0.018), while elevated expressions of curcumin target gene CBR1 in Stomach and epigallocatechin gallate target gene AHR in Adrenal Gland were associated with reduced PCOS risk (OR=0.48, P=0.048; OR=0.02, P=0.018, respectively).
Conclusions: Our research reveals that specific fertility nutrients supplementation, such as omega-3 fatty acids, berberine, and curcumin, may reduce the risk of PCOS by improving metabolic and reproductive abnormalities associated with it.
1 Introduction
Polycystic Ovary Syndrome (PCOS) is a common metabolic disturbance and heterogeneous endocrine disorder among women of reproductive age (1), with an estimated prevalence of 6%-10% during this period (2, 3). It is mainly characterized by hyperandrogenism, anovulation, and/or oligo-ovulation. Studies have shown that women with PCOS exhibit persistent menstrual irregularities, infertility, and obesity. Moreover, even after conception, PCOS can lead to adverse pregnancy outcomes such as gestational hypertension, spontaneous miscarriages, and preterm births (4–6).
Despite its high prevalence, the exact etiology of PCOS remains unclear. Research indicates that PCOS is a multifactorial disease, often involving genetic, metabolic, and environmental abnormalities (7). Hyperandrogenism during different developmental stages is considered as a primary driver of the metabolic and reproductive dysregulations associated with PCOS (8). Insulin resistance is also commonly viewed as a significant etiological factor in PCOS, with approximately 50% to 80% of women with the syndrome exhibiting signs of insulin resistance (9, 10). Current treatment strategies for PCOS primarily involve lifestyle interventions or pharmacological treatments targeting pathological symptoms. Studies suggest that a weight reduction of 5% to 10% in overweight or obese women may restore regular menstruation and ovulation (11, 12); the use of metformin or D-chiro-D-Inositol can increase insulin sensitivity and improve the condition in patients with PCOS (13); and clomiphene citrate can block hypothalamic estrogen receptors, stimulating follicle development through a negative feedback mechanism (14). However, overall, the clinical drugs available are limited, and curing PCOS remains challenging.
Appropriate nutritional supplementation during preconception and pregnancy is beneficial for the development of the mother, embryos, fetuses, and pregnancy outcomes. These supplements are generally recognized as “Fertility Nutrients” or “Fertility Supplements” (15, 16). For example, supplementation with coenzyme Q10, melatonin, and vitamins A, C, and E can effectively promote follicular development and maturation in women with premature ovarian aging and diminished ovarian reserve (17–22). Supplementation with Docosahexaenoic acid (DHA), and minerals such as calcium, magnesium, and selenium, can effectively regulate hormone endocrine levels and insulin metabolism abnormalities in patients with PCOS (23–26). Supplementation with curcumin and vitamin D can effectively regulate overall blood glucose concentrations in pregnant women (21, 27). Additionally, supplementation with folic acid and betaine can significantly lower serum homocysteine levels and prevent fetal neural tube defects during preconception and pregnancy (18, 28). All of the above shows associations between nutrient supplementation and reproductive health, thus revealing whether reproductive nutrient supplementation can reduce the risk of PCOS is of great significance.
At present, common observational epidemiological research methods, such as meta-analyses and clinical randomized controlled trials, can associate medication or reproductive nutrient supplementation with PCOS. However, due to the difficulty in confirming whether all potential confounding factors and other possible influencing factors have been excluded from the correlation between exposure and outcome, the causal inference conclusions drawn from traditional analyses often lack rigor.
With the emergence of large-scale Genome-Wide Association Studies (GWAS) at the end of the 20th century, researchers have been able to use genetic variants to infer causal relationships between exposure factors (such as nutritional supplements, drugs, drug targets, or risk factors for disease) and outcome factors (such as diseases or biological events). Mendelian Randomization (MR) is a novel genetic analysis method that uses genetic variants strongly associated with exposure as instrumental variables to assess the causal relationship between exposure and outcome (29). This method relies on three essential assumptions: (1) Genetic variants are strongly associated with exposure. (2) Genetic variants are not associated with any confounders of the exposure-outcome relationship. (3) Genetic factors only affect the outcome through the exposure. MR avoids the confounding factors and reverse causality issues present in observational studies, providing a more reliable causal relationship than observational research.
Based on two-sample Mendelian Randomization studies, this research uses fertility supplements as the exposure factors and Polycystic Ovary Syndrome as the outcome to explore the causal relationship. This approach may provide more potential feasible solutions for the clinical treatment and prevention of PCOS.
2 Materials and methods
2.1 Acquisition and selection of exposure data
Using the IEU OpenGWAS database (https://gwas.mrcieu.ac.uk/) and the Drug Bank database (https://go.drugbank.com/), a search was conducted for 49 common fertility supplements (see Supplementary Table 1). A total of 10 supplements suitable for Mendelian Randomization (MR) analysis were selected for study, including DHA, calcium, Dehydroisoandrosterone (DHEA), vitamin D, betaine, and metformin, with their respective GWAS_IDs being met-d-DHA, ukb-b-7043, ebi-a-GCST004941, ebi-a-GCST90000618, met-a-362, and ukb-a-159 (Table 1). Data for D-Inositol (30) were downloaded from the GWAS Catalog (2023) website (https://www.ebi.ac.uk/gwas/).
Additionally, berberine, curcumin, and epigallocatechin gallate (EGCG) were searched, and 10 target genes were identified respectively as BIRC5, qacR, PPARG, VDR, ABCC5, CBR1, GSTP1, AHR, DNMT1, and DHFRL1 (Table 2). Public data from GTEx (https://www.gtexportal.org/) were utilized to obtain the cis-eQTLs associated with the expression of these target genes in 49 human tissues. These cis-eQTLs were used as instrumental variables for drug target MR analysis.
2.2 Acquisition of outcome data
The GWAS_ID for PCOS was retrieved from the IEU OpenGWAS project database (https://gwas.mrcieu.ac.uk/) as finn-b-E4_POCS. This data originates from publicly published GWAS summary statistics in 2021, encompassing a total of 16,379,676 SNPs.
2.3 Two-sample MR analysis
We conducted a two-sample Mendelian Randomization (MR) analysis (31) using R software (version 4.2.3) and the TwoSampleMR package (version 0.5.7). For the seven fertility nutritional components with GWAS_ID, causal relationships with PCOS were assessed using mendelian randomization analysis methods (instrumental variable logistic regression with SNPs as instrumental variables) such as inverse variance weighted (IVW), MR-Egger regression, simple mode, weighted median, and weighted mode. Odds ratios (OR) and their 95% confidence intervals (CI) were estimated, and a p-value < 0.05 was considered statistically significant.
Heterogeneity was tested using Cochran’s Q test, with a p-value < 0.05 indicating the presence of heterogeneity. Pleiotropy was assessed using the MR-Egger intercept test, with a p-value < 0.05 indicating the presence of pleiotropy. Sensitivity analysis was conducted using the leave-one-out method to evaluate the robustness of the findings, checking if the removal of a single variant affected the relationship between exposure and outcome. Forest plots were used to visualize causal associations, and scatter plots were used to visualize the effect sizes of each genetic instrument on exposures and outcomes.
2.4 Drug target MR analysis
For the three fertility nutritional components without GWAS data, we analyzed the instrumental variables containing multiple SNP loci using a fixed-effect inverse variance weighted MR method. For instrumental variables containing only a single SNP locus, the Wald ratio method was used.
3 Results
Firstly, this study took seven reproductive nutritional supplements with GWAS data—DHA, calcium, DHEA, vitamin D, betaine, D-Inositol, and metformin—as exposure factors (Table 1). After genome-wide screening (p < 5 × 10^-5) and clumping (r^2 < 0.001, kb = 10,000), 216, 126, 104, 376, 61, 99, and 196 SNPs were included respectively. These SNPs were strongly associated with the exposure factors and were independent of each other (Figure 1).
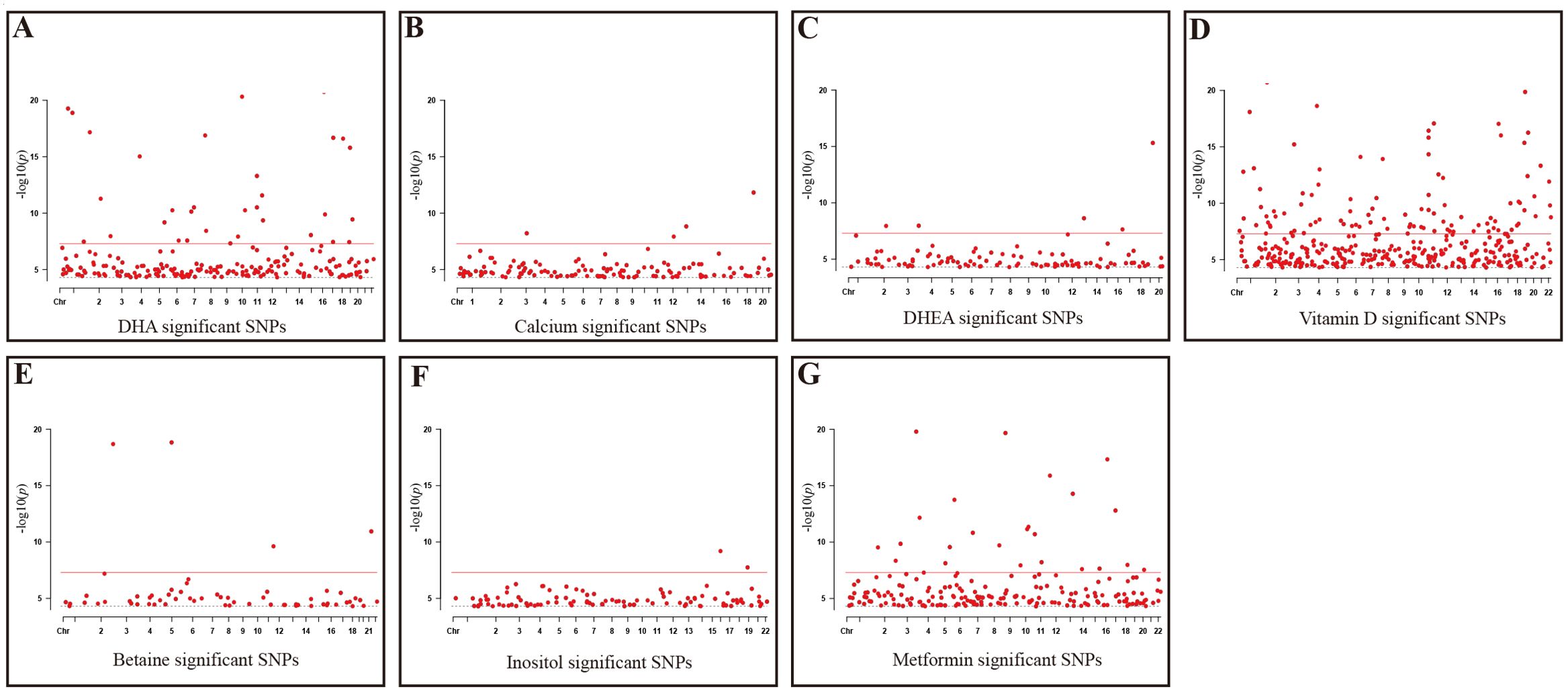
Figure 1. Significant SNPs in GWAS Data for reproductive nutritional components. (A) DHA; (B) Calcium; (C) DHEA; (D) Vitamin D; (E) Betaine; (F) Inositol; (G) Metformin. X-axis: Chromosomal positions of SNPs; Y-axis: SNPs correspond to negative log10 values of p-values; Gray dashed line: P=5e-5 threshold; Red solid line: P=5e-8 threshold.
At the same time, this study used the eQTL data corresponding to the target action points of three fertility nutritional components without GWAS data—berberine, curcumin, and EGCG—as instrumental variables. After screening (p < 5 × 10^-5), the target genes for berberine: BIRC5 and qacR, included 389 and 0 SNPs respectively; the target genes for curcumin: PPARG, VDR, ABCC5, CBR1, and GSTP1, included 1058, 530, 4150, 4809, and 5693 SNPs respectively; the target genes for EGCG: AHR, DNMT1, and DHFRL1, included 88, 76, and 0 SNPs respectively (Figure 2).
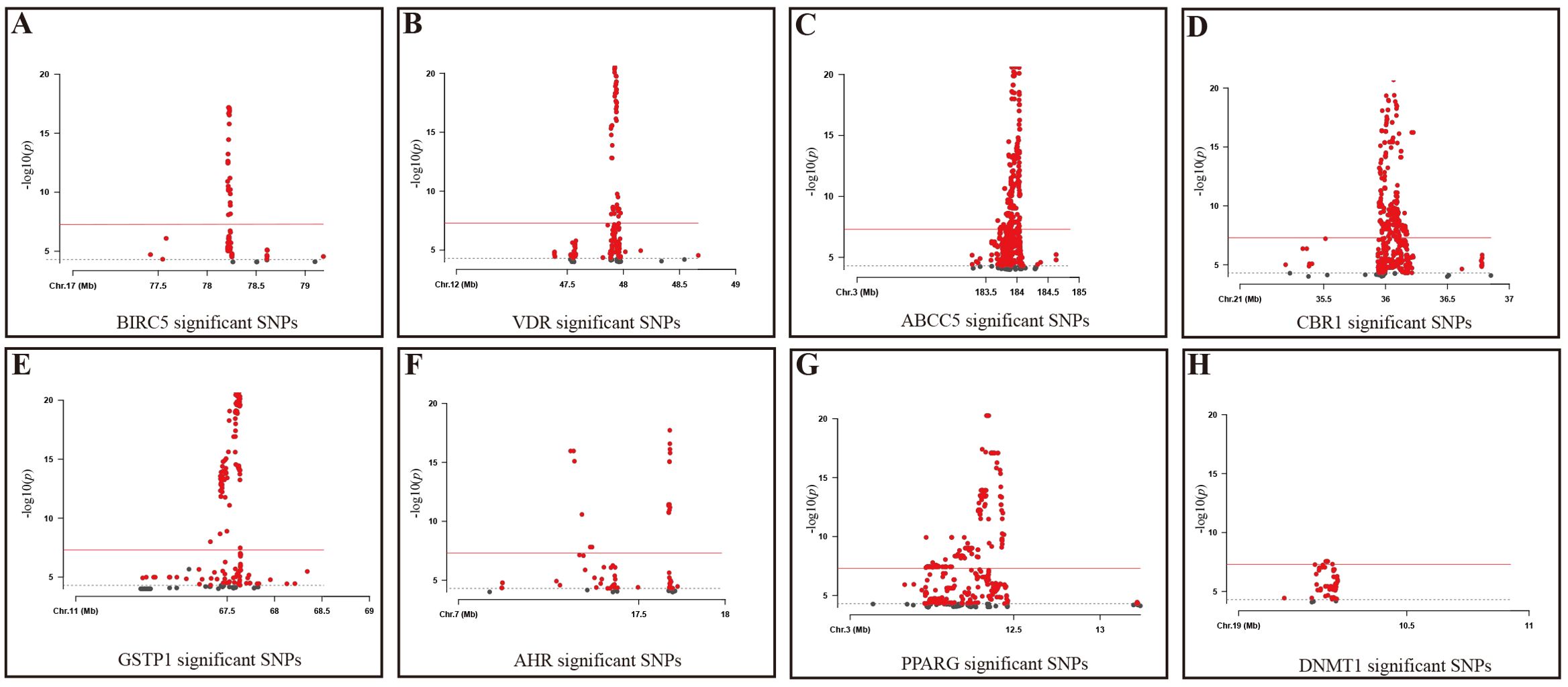
Figure 2. Significant SNPs in Target Genes for fertility nutritional components. (A) BIRC5; (B) VDR; (C) ABCC5; (D) CBR1; (E) GSTP1; (F) AHR; (G) PPARG; (H) DNMT1; Gray dashed line: P=5e-5 threshold; Red solid line: P=5e-8 threshold. X-axis: Chromosomal position of the SNP; Y-axis: SNPs correspond to negative log10 values of p-values.
Subsequently, we conducted a two-sample MR analysis on the seven fertility nutritional supplements with GWAS data. The IVW results showed that DHA could decrease the risk of PCOS (OR=0.73, 95% CI: 0.57-0.94, P=0.016), while betaine could increase the risk of PCOS (OR=2.60, 95% CI: 1.09-6.17, P=0.031). For calcium, DHEA, vitamin D, D-Inositol, and metformin, the MR analyses across all five regression models showed p-values greater than 0.05, indicating no statistical significance (Figure 3). In MR analysis, the IVW regression analysis is of particular importance; if the IVW result is statistically significant, and the effect value (b) of other non-statistically significant models is in the same direction as the IVW b value, the exposure factor can still be considered to have a causal relationship with the outcome. The scatter plot for DHA and PCOS is shown in Figure 4A. The forest plot for the effect estimates of individual SNPs on PCOS is provided in Supplementary Figure 1.
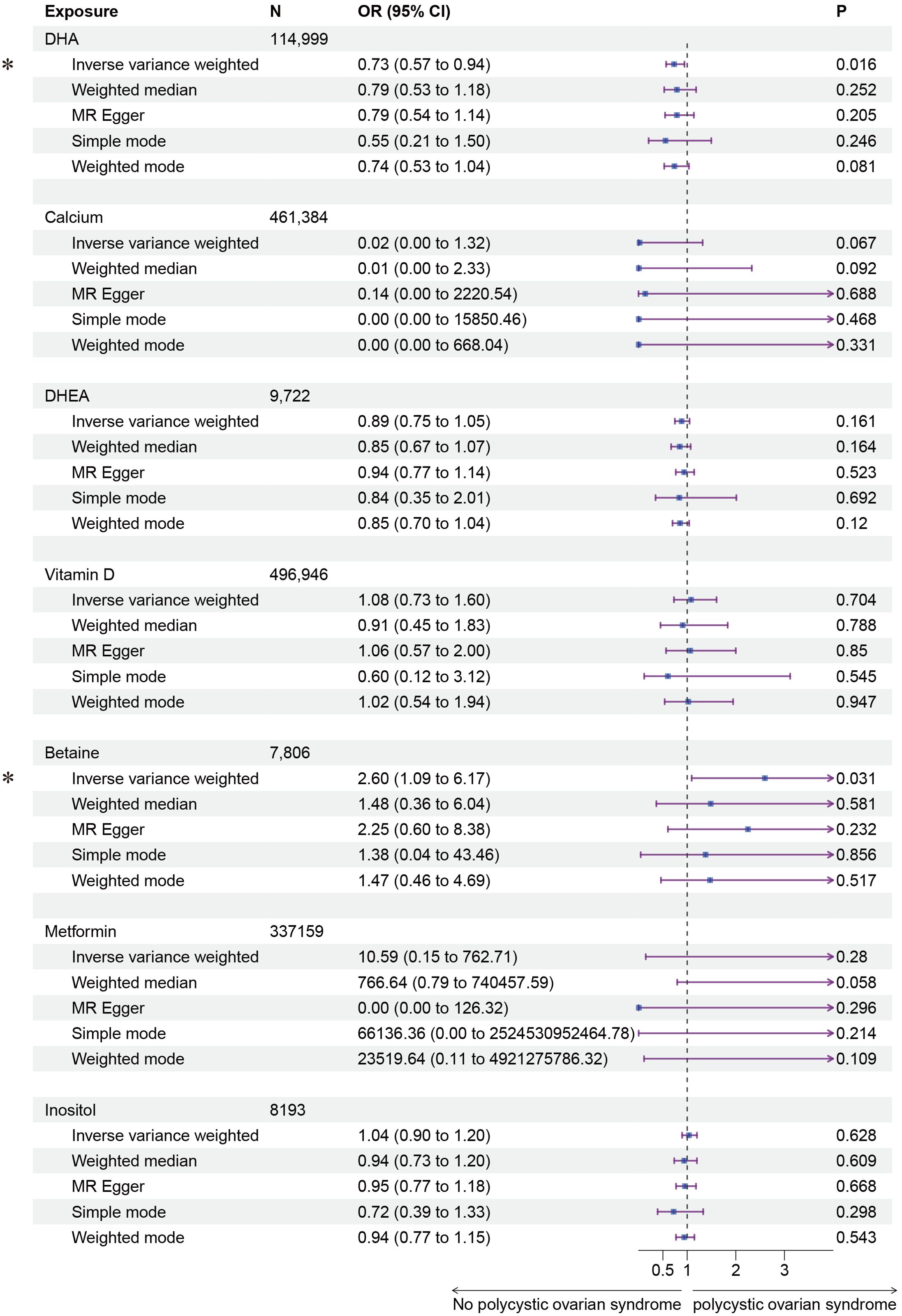
Figure 3. Forest plot of the results of MR Analysis of fertility nutrients and PCOS. Exposure, Exposure Factor; N, Sample Size; OR, Odds Ratio; 95% CI, 95% Confidence Interval; P, p-value; an asterisk “*” denotes P<0.05 as a statistically significant result in the Mendelian Randomization analysis.
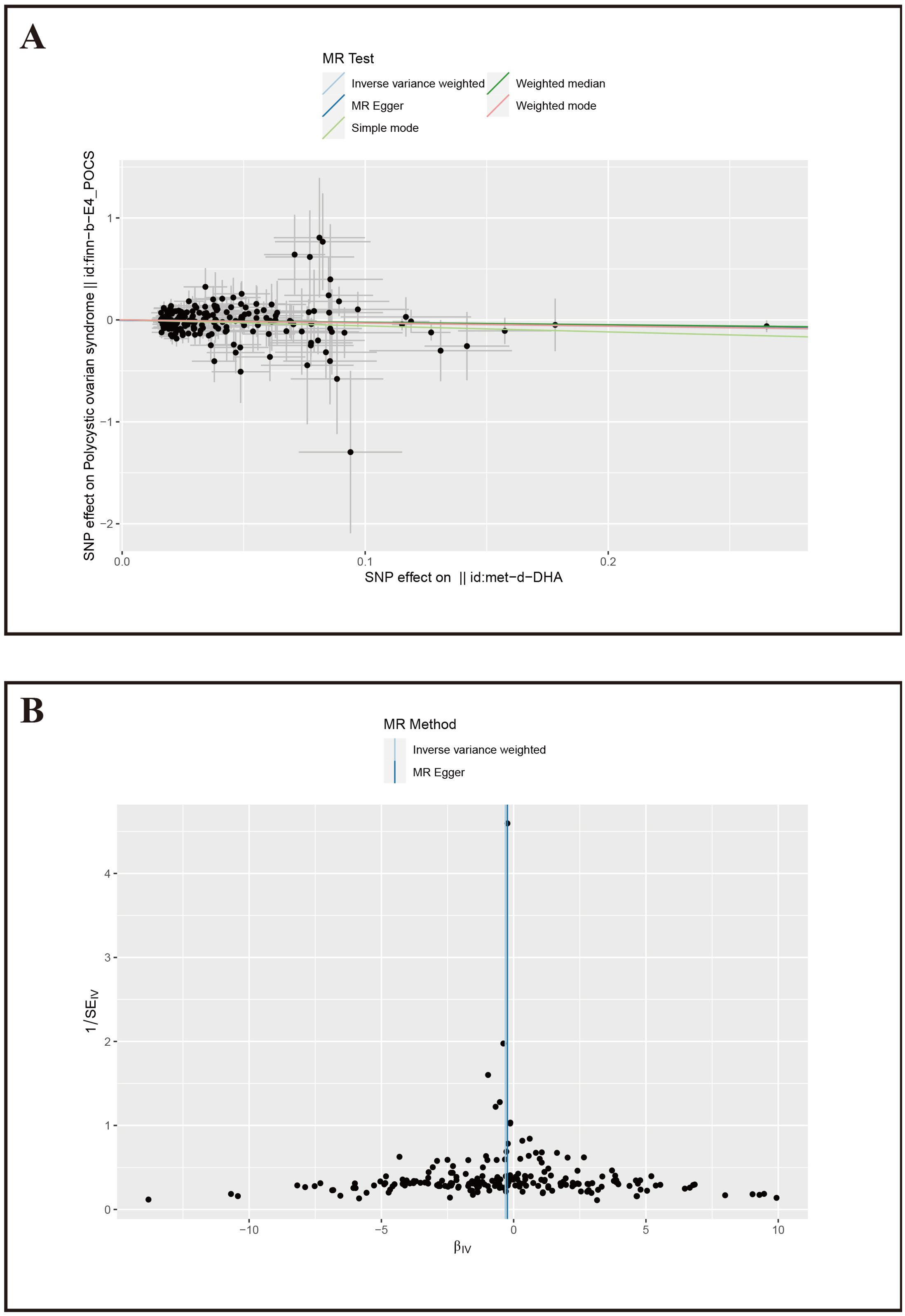
Figure 4. Mendelian Randomization results for DHA and PCOS. (A) Scatter plot of SNP effects on PCOS, X-axis: Effect values of SNPs on DHA; Y-axis: Effect values of SNPs on PCOS. (B) Volcano plot, βIV: Instrumental variable effect value; 1/SEIV: Inverse of the standard error.
Furthermore, we conducted sensitivity tests for the MR analysis results of DHA and PCOS. The IVW results showed Cochran’s Q=203.16, P=0.50; MR-Egger results showed Cochran’s Q=203.46, P=0.52, with both methods having p-values greater than 0.05, indicating no heterogeneity among the instrumental variables. The MR-Egger intercept was 0.0045, P=0.58, suggesting no pleiotropy among the included SNPs. The funnel plot also indicated that the causal effect estimates of IVW were symmetrically distributed overall, showing no heterogeneity (Figure 4B). The study also used the Leave-one-out method to test the impact of the remaining SNPs after removing a single SNP, and it was observed that there were no outlier SNPs or SNPs affecting the results (Supplementary Figure 2), thereby confirming the reliability of our results.
Finally, we conducted Mendelian randomization (MR) analyses on the drug targets of three fertility nutritional components: berberine, curcumin, and EGCG. The results indicated that the upregulation of the berberine target gene BIRC5 in tissues such as “Cells _ Cultured _ fibroblasts”, “Esophagus _ Mucosa”, “Skin _ Not _ Sun _ Exposed _ Suprapubic”, and “Skin _ Sun _ Exposed _ Lower _ leg” could increase the risk of PCOS (OR=4.88, 95% CI: 1.31-18.24, P=0.018; OR=3.00, 95% CI: 1.25-7.17, P=0.014; OR=3.63, 95% CI: 1.26-10.42, P=0.017; OR=4.23, 95% CI: 1.32-13.53, P=0.015 respectively). In Adrenal _ Gland tissue, the upregulation of the EGCG target gene AHR could decrease the risk of PCOS (OR=0.02, 95% CI: 0.00-0.52, P=0.018), and in Stomach tissue, the upregulation of the curcumin target gene CBR1 could decrease the risk of PCOS (OR=0.48, 95% CI: 0.23-0.99, P=0.048) (Figures 5–7).
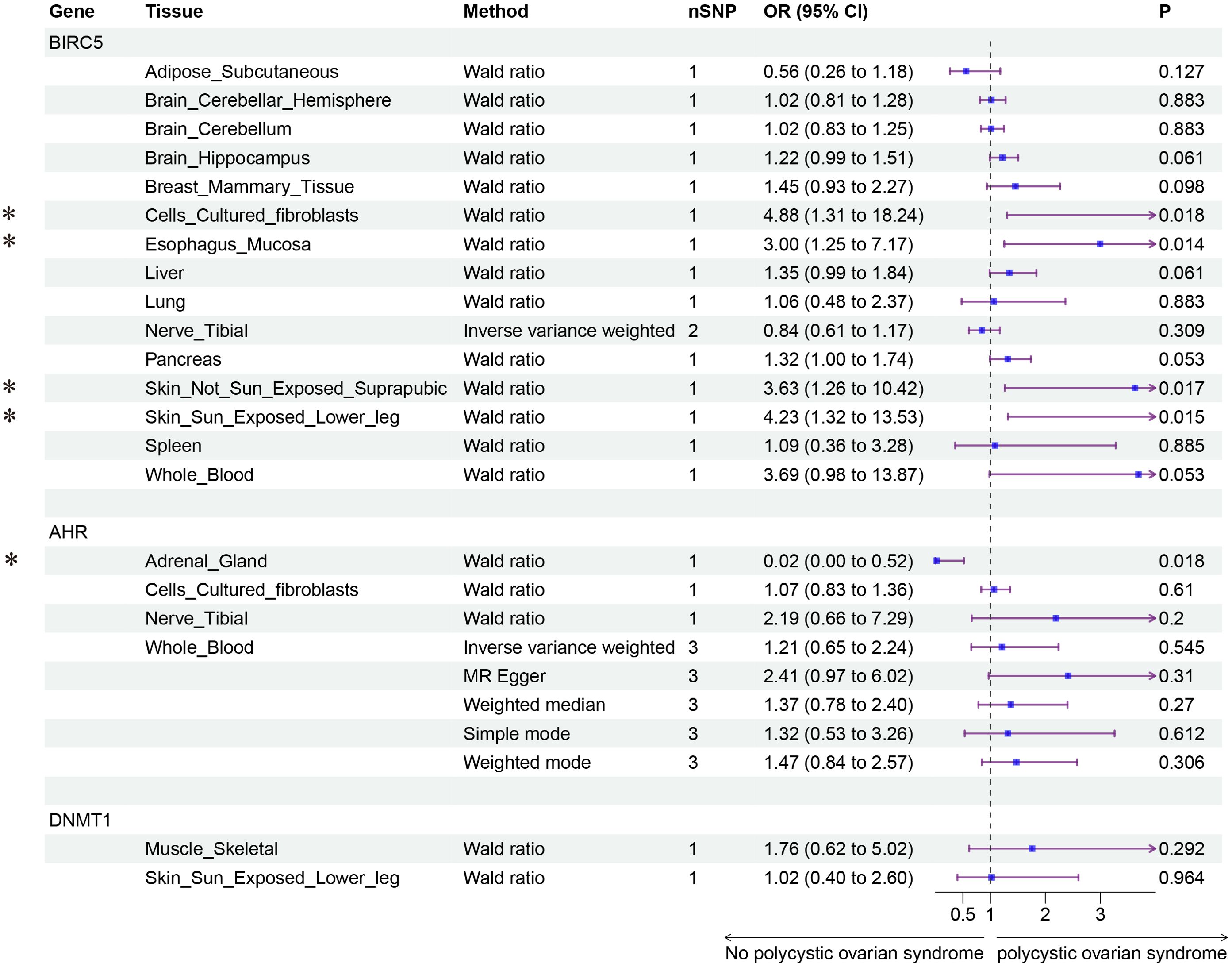
Figure 5. Forest plot of the results of MR Analysis of berberine (BIRC5) and EGCG (AHR and DNMT1) target gene SNPs and PCOS. Gene, Target gene; Tissue, Tissue where SNPs are located; nSNPs, Number of effective SNPs (P<5e-5); OR, Odds Ratio; 95% CI, 95% Confidence Interval; P, p-value corresponding to SNPs in MR results; an asterisk “*” denotes P<0.05 as a statistically significant result in the Mendelian Randomization analysis.
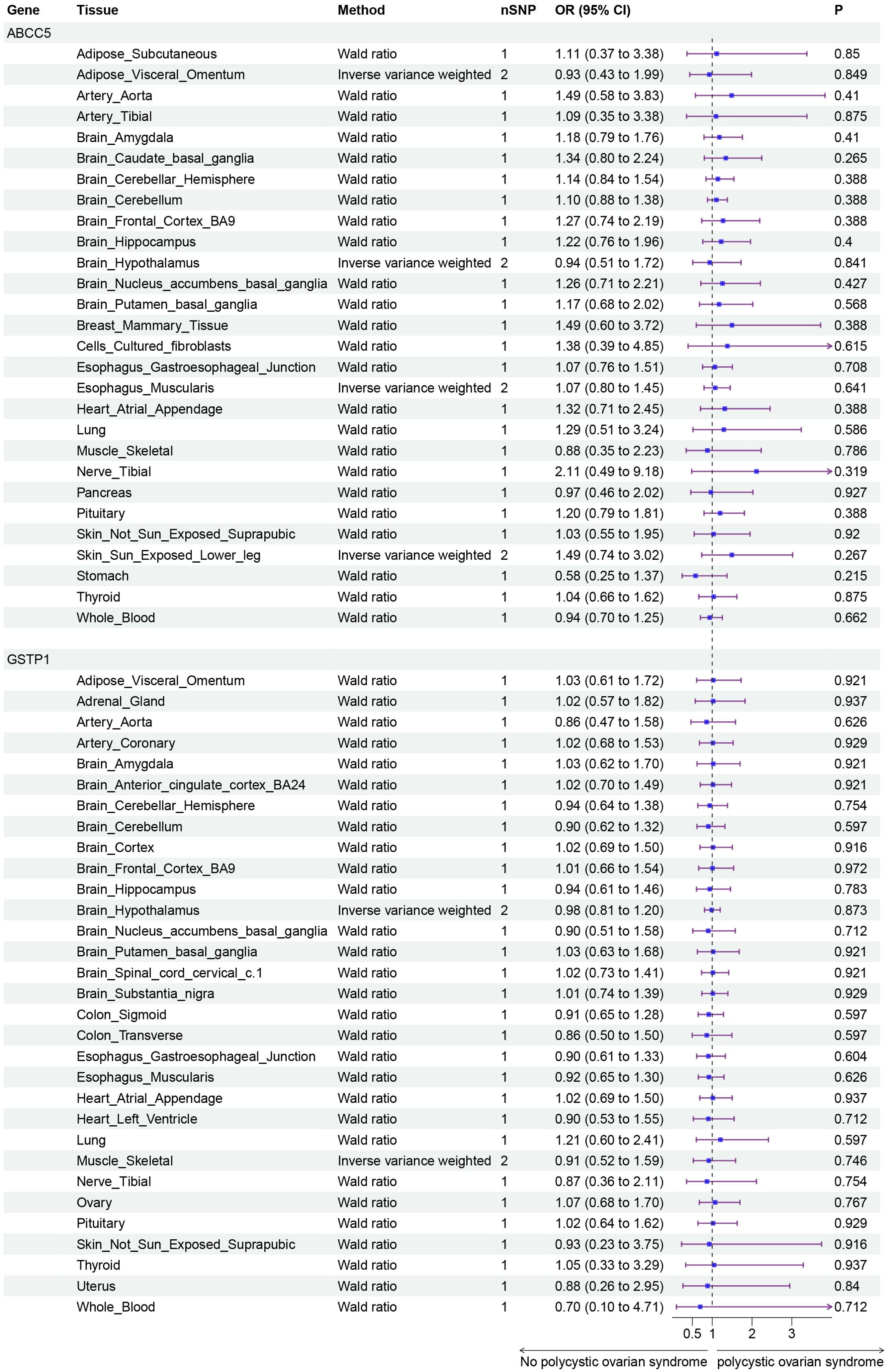
Figure 6. Forest plot of the results of MR Analysis of curcumin target gene (ABCC5 and GSTP1) SNPs and PCOS. Gene, Target gene; Tissue, Tissue where SNPs are located; nSNPs, Number of effective SNPs (P<5e-5); OR, Odds Ratio; 95% CI, 95% Confidence Interval; P, p-value corresponding to SNPs in MR results.
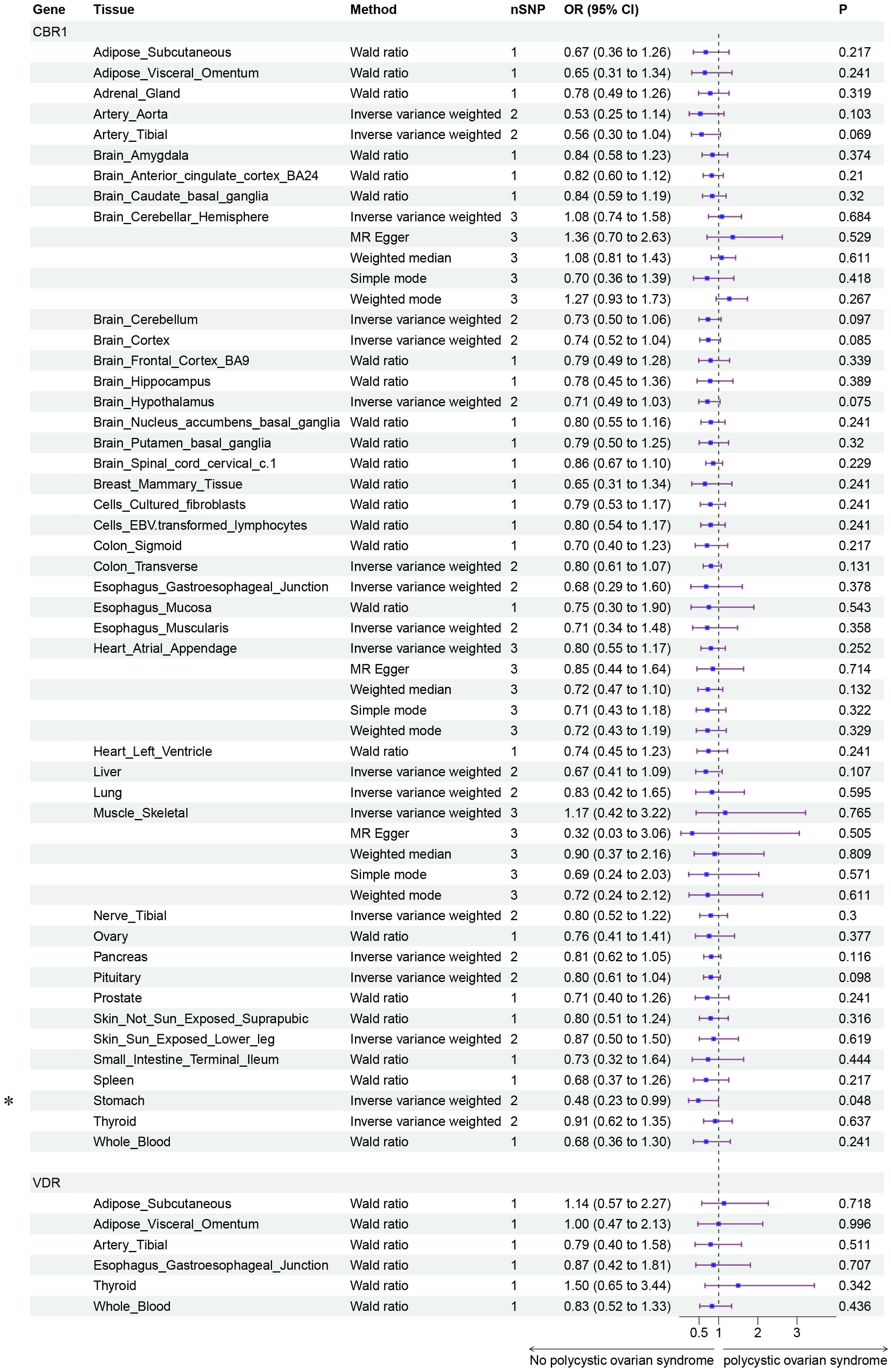
Figure 7. Forest plot of the results of MR Analysis of curcumin (CBR1 and VDR) target gene SNPs and PCOS. Gene, Target gene; Tissue, Tissue where SNPs are located; nSNPs, Number of effective SNPs (P<5e-5); OR, Odds Ratio; 95% CI, 95% Confidence Interval; P, p-value corresponding to SNPs in MR results; an asterisk “*” denotes P<0.05 as a statistically significant result in the Mendelian Randomization analysis.
4 Discussion
Polycystic Ovary Syndrome (PCOS) is a common reproductive endocrine metabolic disease among women of reproductive age, characterized by hyperandrogenism, ovulatory dysfunction, and the presence of polycystic ovaries (32). Due to the heterogeneity of these features, diagnosing and treating PCOS has always been challenging. This study utilized a two-sample Mendelian randomization approach to assess the causal relationship between reproductive nutritional supplementation and the risk of developing PCOS.
In this study, we evaluated the potential causal relationship between fertility nutritional components, using GWAS data, and the risk of PCOS. The components assessed included DHA, calcium, DHEA, vitamin D, betaine, D-Inositol, metformin. The results suggest that DHA may reduce the risk of PCOS (OR=0.73, 95% CI: 0.57-0.94, P=0.016), while betaine may increase the risk of PCOS (OR=2.60, 95% CI: 1.09-6.17, P=0.031). No significant associations were found between the other nutritional components and PCOS.
Women with PCOS often exhibit insulin resistance, characterized by excessive insulin secretion and reduced glucose utilization by insulin (33). Numerous basic and clinical studies have shown that DHA (omega-3 polyunsaturated fatty acids) can beneficially impact PCOS through various pathways and mechanisms, including reducing insulin resistance, modulating adipokine production, exerting anti-inflammatory effects, and enhancing endothelial function (34–36). Consistent with these findings, our study also indicates that DHA intake decreases the risk of PCOS.
Metformin, as an insulin sensitizer, is widely used to ameliorate insulin resistance in patients with PCOS. However, the reduction in gastrointestinal glucose absorption caused by metformin can lead to symptoms such as nausea, vomiting, and diarrhea, which may interrupt PCOS treatment (33). Hence, alternatives with fewer side effects would be beneficial for the recovery of PCOS patients. D-Inositol has long been considered a potential substitute for metformin in the treatment of PCOS. A meta-analysis has indicated that D-Inositol supplementation for PCOS can help normalize the ovarian cycle, regulate carbohydrate metabolism, and significantly improve hyperandrogenemia symptoms by reducing free and total testosterone levels and increasing sex hormone-binding globulin levels (37). However, our study’s findings show that increased levels of metformin and D-Inositol do not have a significant causal relationship with the risk of PCOS, indicating that the currently reported GWAS data related to those compounds are limited. Therefore, MR serves as a predictive tool, and its results have suggestive value, and further observational studies and clinical trials are warranted.
During the treatment of patients with PCOS, in addition to improving insulin resistance, reducing homocysteine levels may also enhance reproductive outcomes (38, 39). As a methyl donor, betaine works in conjunction with betaine-homocysteine methyltransferase to convert homocysteine into methionine, thereby lowering homocysteine levels (40). This suggests that betaine supplementation could improve symptoms of hyperhomocysteinemia in PCOS patients, thereby improving PCOS outcomes. However, our MR study results indicated that increased levels of betaine might exacerbate the risk of PCOS, which contradicts previous research. The causal relationship between betaine levels and PCOS lacks direct clinical evidence and requires further investigation to explore this relationship.
For the fertility nutritional components without GWAS data—berberine, curcumin, and EGCG—we conducted the analysis using drug target genes as instrumental variables. Our findings suggest that the elevated expression of the target gene BIRC5 for berberine is linked to a heightened risk of PCOS, while increased expression of target genes for curcumin and EGCG is associated with a reduced risk of PCOS.
Berberine is an alkaloid with a variety of pharmacological effects, including anti-inflammatory, antioxidant, and insulin sensitivity-improving actions (41, 42). This study identified that the elevated expression of the berberine target gene BIRC5 across various tissues is associated with an increased risk of PCOS. The protein encoded by BIRC5, SURVIVIN, is a member of the cell cycle regulatory proteins involved in inhibiting apoptosis and promoting cell proliferation (43). Elevated expression of BIRC5 is associated with the development of various cancers, and abnormal expression of BIRC5 has also been found in the ovarian granulosa cells of PCOS patients (44, 45). Hao et al. have indicated that alkaloids like berberine and cantharidin may reduce the levels of proteins such as BIRC5, CDK1, and CCNB1 to combat chronic hepatitis B virus (46). Thus, berberine might exert its therapeutic effects on PCOS by suppressing the expression of the BIRC5 gene, thereby decreasing the expression of SURVIVIN, ultimately improving PCOS outcomes. However, this hypothesis requires further validation through more cellular and animal studies.
Curcumin, the principal active component of turmeric, is known for its extensive anti-inflammatory and antioxidant properties and has been shown to play a role in the management of diabetes and cardiovascular diseases (47, 48). In our study, the expression of the curcumin target gene CBR1 in stomach tissue was associated with a reduced risk of PCOS. CBR1 is a reductase involved in drug metabolism and cellular antioxidative defense mechanisms (49). The regulatory effect of curcumin on CBR1 may be linked to its potential in ameliorating metabolic abnormalities in PCOS patients, but the specific mechanisms and efficacy of this action remain to be further explored.
Epigallocatechin gallate (EGCG), one of the most active components in green tea, exhibits significant antioxidant, anti-inflammatory, and potential anticancer effects (50, 51). In this study, the expression of the target gene AHR for EGCG in adrenal tissue was significantly associated with a reduced risk of PCOS. However, studies have shown that Bisphenol A induces insulin resistance, a potential factor in the development of PCOS, by activating the AHR receptor to inhibit GLUT4 expression, leading to aberrant glucose metabolism (52). This finding is inconsistent with our MR results, and the specific mechanism by which AHR affects PCOS requires further research.
The aforementioned findings provide potential directions for both clinical and basic research into the treatment and prevention of PCOS. These nutritional components may improve clinical outcomes in PCOS by affecting the expression of specific genes and related biological pathways.
Mendelian randomization analysis is a powerful tool for studying causal relationships, addressing confounding and reverse causality issues in traditional observational studies by using genetic variants as instrumental variables. In our study, we employed multiple MR methods to test causal relationships and conducted heterogeneity and pleiotropy tests, as well as sensitivity analyses, to ensure the robustness of our results. Despite the enhanced credibility of our analyses through these methods, our study still has some limitations.
Firstly, our analysis relies on existing GWAS datasets, which may lack diversity in terms of ethnicity, geography, and environmental background, thereby potentially limiting the generalizability of our findings. For example, the PCOS GWAS data utilized in our study are derived solely from European populations, rather than global populations. Additionally, due to the limitations of GWAS summary data, Mendelian randomization analysis can only estimate odds ratios (OR), even though relative risk (RR) is more appropriate for estimating risk.
Secondly, the association between the nutrients we studied and PCOS might be influenced by other genetic and environmental factors that were not fully controlled for in our analysis. For example, calcium’s effect approached borderline significance (P = 0.067) and was favorable in reducing PCOS. The larger standard error and wider confidence interval could be due to the sample size, suggesting calcium as a potential beneficial factor warranting further investigation. Our results may be limited by the strength of the association between the genetic variants used as instrumental variables and the exposure to nutritional components, as well as the number of these genetic variants.
Nevertheless, our study provides new insights, identifies potential genetic variants, and offfers targeted research recommendations for further clinical research and the development of therapeutic strategies.
5 Conclusions
In conclusion, our research suggests that specific fertility nutritional components, such as DHA, berberine, and curcumin, may reduce the risk of PCOS by improving metabolic and reproductive abnormalities. Future research should focus on verifying the therapeutic efficacy and mechanisms of action of these nutrients on PCOS, while also assessing their potential values in the prevention and treatment of PCOS. Attention should also be given to the relationship between nutrient supplementation and other phenotypes of PCOS. Furthermore, direct intervention studies, such as randomized controlled trials, can better elucidate the impact of nutritional supplementation strategies on PCOS and provide more specific guidance for patients. It is important to note that our current data are derived from European cohorts and may not be broadly representative. The applicability of our conclusions to populations outside Europe requires further validation and analysis.
Data availability statement
The original contributions presented in the study are included in the article/Supplementary Material. Further inquiries can be directed to the corresponding authors.
Author contributions
FS: Data curation, Formal analysis, Methodology, Writing – original draft. SX: Data curation, Methodology, Writing – original draft. HZ: Data curation, Methodology, Writing – original draft. FZ: Data curation, Methodology, Writing – original draft. XW: Data curation, Methodology, Writing – original draft. HW: Conceptualization, Funding acquisition, Writing – review & editing.
Funding
The author(s) declare financial support was received for the research, authorship, and/or publication of this article. This work was supported by National Natural Science Foundation of China to HW (NO. 82374225) and National Natural Science Foundation of China to FS (NO. 82473732).
Conflict of interest
The authors declare that the research was conducted in the absence of any commercial or financial relationships that could be construed as a potential conflict of interest.
Publisher’s note
All claims expressed in this article are solely those of the authors and do not necessarily represent those of their affiliated organizations, or those of the publisher, the editors and the reviewers. Any product that may be evaluated in this article, or claim that may be made by its manufacturer, is not guaranteed or endorsed by the publisher.
Supplementary material
The Supplementary Material for this article can be found online at: https://www.frontiersin.org/articles/10.3389/fendo.2024.1420004/full#supplementary-material
References
1. Wood JR, Dumesic DA, Abbott DH, Strauss JF. Molecular abnormalities in oocytes from women with polycystic ovary syndrome revealed by microarray analysis. J Clin Endocrinol Metab. (2007) 92:705–13. doi: 10.1210/jc.2006-2123
2. Livadas S, Diamanti-Kandarakis E. Polycystic ovary syndrome: definitions, phenotypes and diagnostic approach. Front Horm Res. (2013) 40:1–21. doi: 10.1159/000341673
3. Pasquali R, Gambineri A. Glucose intolerance states in women with the polycystic ovary syndrome. J Endocrinol Invest. (2013) 36:648–53. doi: 10.1007/BF03346757
4. Bjercke S, Dale PO, Tanbo T, Storeng R, Ertzeid G, Abyholm T. Impact of insulin resistance on pregnancy complications and outcome in women with polycystic ovary syndrome. Gynecol Obstet Invest. (2002) 54:94–8. doi: 10.1159/000067719
5. Joham AE, Boyle JA, Ranasinha S, Zoungas S, Teede HJ. Contraception use and pregnancy outcomes in women with polycystic ovary syndrome: data from the Australian Longitudinal Study on Women’s Health. Hum Reprod. (2014) 29:802–8. doi: 10.1093/humrep/deu020
6. Mirza FG, Tahlak MA, Rjeili RB, Hazari K, Ennab F, Hodgman C, et al. Polycystic ovarian syndrome (PCOS): does the challenge end at conception? Int J Environ Res Public Health. (2022) 19:14914. doi: 10.3390/ijerph192214914
7. Franks S, McCarthy MI, Hardy K. Development of polycystic ovary syndrome: involvement of genetic and environmental factors. Int J Androl. (2006) 29:278–85. doi: 10.1111/j.1365-2605.2005.00623.x
8. Sanchez-Garrido MA, Tena-Sempere M. Metabolic dysfunction in polycystic ovary syndrome: Pathogenic role of androgen excess and potential therapeutic strategies. Mol Metab. (2020) 35:100937. doi: 10.1016/j.molmet.2020.01.001
9. Sirmans SM, Pate KA. Epidemiology, diagnosis, and management of polycystic ovary syndrome. Clin Epidemiol. (2013) 6:1–13. doi: 10.2147/CLEP.S37559
10. Ozegowska KE, Pawelczyk LA. The role of insulin and selected adipocytokines in patients with polycystic ovary syndrome (PCOS) - a literature review. Ginekol Pol. (2015) 86:300–4. doi: 10.17772/gp/2077
11. Pasquali R, Antenucci D, Casimirri F, Venturoli S, Paradisi R, Fabbri R, et al. Clinical and hormonal characteristics of obese amenorrheic hyperandrogenic women before and after weight loss. J Clin Endocrinol Metab. (1989) 68:173–9. doi: 10.1210/jcem-68-1-173
12. Kiddy DS, Hamilton-Fairley D, Bush A, Short F, Anyaoku V, Reed MJ, et al. Improvement in endocrine and ovarian function during dietary treatment of obese women with polycystic ovary syndrome. Clin Endocrinol (Oxf). (1992) 36:105–11. doi: 10.1111/j.1365-2265.1992.tb02909.x
13. Kiani AK, Donato K, Dhuli K, Stuppia L, Bertelli M. Dietary supplements for polycystic ovary syndrome. J Prev Med Hyg. (2022) 63:E206–13. doi: 10.15167/2421-4248/jpmh2022.63.2S3.2762
14. Kettel LM, Roseff SJ, Berga SL, Mortola JF, Yen SS. Hypothalamic-pituitary-ovarian response to clomiphene citrate in women with polycystic ovary syndrome. Fertil Steril. (1993) 59:532–8. doi: 10.1016/S0015-0282(16)55795-1
15. Ramakrishnan U, Grant F, Goldenberg T, Zongrone A, Martorell R. Effect of women’s nutrition before and during early pregnancy on maternal and infant outcomes: a systematic review. Paediatr Perinat Epidemiol. (2012) 26 Suppl 1:285–301. doi: 10.1111/j.1365-3016.2012.01281.x
16. Skoracka K, Ratajczak AE, Rychter AM, Dobrowolska A, Krela-Kaźmierczak I. Female fertility and the nutritional approach: the most essential aspects. Adv Nutr. (2021) 12:2372–86. doi: 10.1093/advances/nmab068
17. Tarín JJ, Pérez-Albalá S, Cano A. Oral antioxidants counteract the negative effects of female aging on oocyte quantity and quality in the mouse. Mol Reprod Dev. (2002) 61:385–97. doi: 10.1002/mrd.10041
18. Ebisch IMW, Thomas CMG, Peters WHM, Braat DDM, Steegers-Theunissen RPM. The importance of folate, zinc and antioxidants in the pathogenesis and prevention of subfertility. Hum Reprod Update. (2007) 13:163–74. doi: 10.1093/humupd/dml054
19. Clagett-Dame M, Knutson D. Vitamin A in reproduction and development. Nutrients. (2011) 3:385–428. doi: 10.3390/nu3040385
20. Luddi A, Capaldo A, Focarelli R, Gori M, Morgante G, Piomboni P, et al. Antioxidants reduce oxidative stress in follicular fluid of aged women undergoing IVF. Reprod Biol Endocrinol. (2016) 14:57. doi: 10.1186/s12958-016-0184-7
21. Zhou K, Zhang J, Xu L, Wu T, Lim CED. Chinese herbal medicine for subfertile women with polycystic ovarian syndrome. Cochrane Database Syst Rev. (2016) 10:CD007535. doi: 10.1002/14651858.CD007535.pub3
22. Yang L, Xu H, Chen Y, Miao C, Zhao Y, Xing Y, et al. Melatonin: multi-target mechanism against diminished ovarian reserve based on network pharmacology. Front Endocrinol (Lausanne). (2021) 12:630504. doi: 10.3389/fendo.2021.630504
23. Tinggi U. Selenium: its role as antioxidant in human health. Environ Health Prev Med. (2008) 13:102–8. doi: 10.1007/s12199-007-0019-4
24. Cormick G, Belizán JM. Calcium intake and health. Nutrients. (2019) 11:1606. doi: 10.3390/nu11071606
25. Alesi S, Ee C, Moran LJ, Rao V, Mousa A. Nutritional supplements and complementary therapies in polycystic ovary syndrome. Adv Nutr. (2022) 13:1243–66. doi: 10.1093/advances/nmab141
26. Lu L, Li X, Lv L, Xu Y, Wu B, Huang C. Associations between omega-3 fatty acids and insulin resistance and body composition in women with polycystic ovary syndrome. Front Nutr. (2022) 9:1016943. doi: 10.3389/fnut.2022.1016943
27. Gao H, Li Y, Yan W, Gao F. The effect of vitamin D supplementation on blood lipids in patients with polycystic ovary syndrome: A meta-analysis of randomized controlled trials. Int J Endocrinol. (2021) 2021:8849688. doi: 10.1155/2021/8849688
28. Li T, Dong G, Kang Y, Zhang M, Sheng X, Wang Z, et al. Increased homocysteine regulated by androgen activates autophagy by suppressing the mammalian target of rapamycin pathway in the granulosa cells of polycystic ovary syndrome mice. Bioengineered. (2022) 13:10875–88. doi: 10.1080/21655979.2022.2066608
29. Smith GD, Ebrahim S. Mendelian randomization”: can genetic epidemiology contribute to understanding environmental determinants of disease? Int J Epidemiol. (2003) 32:1–22. doi: 10.1093/ije/dyg070
30. Chen Y, Lu T, Pettersson-Kymmer U, Stewart ID, Butler-Laporte G, Nakanishi T, et al. Genomic atlas of the plasma metabolome prioritizes metabolites implicated in human diseases. Nat Genet. (2023) 55:44–53. doi: 10.1038/s41588-022-01270-1
31. Hemani G, Zheng J, Elsworth B, Wade KH, Haberland V, Baird D, et al. The MR-Base platform supports systematic causal inference across the human phenome. Elife. (2018) 7:e34408. doi: 10.7554/eLife.34408
32. Goodarzi MO, Dumesic DA, Chazenbalk G, Azziz R. Polycystic ovary syndrome: etiology, pathogenesis and diagnosis. Nat Rev Endocrinol. (2011) 7:219–31. doi: 10.1038/nrendo.2010.217
33. Notaro ALG, Neto FTL. The use of metformin in women with polycystic ovary syndrome: an updated review. J Assist Reprod Genet. (2022) 39:573–9. doi: 10.1007/s10815-022-02429-9
34. Faghfoori Z, Fazelian S, Shadnoush M, Goodarzi R. Nutritional management in women with polycystic ovary syndrome: A review study. Diabetes Metab Syndr. (2017) 11 Suppl 1:S429–32. doi: 10.1016/j.dsx.2017.03.030
35. Yang K, Zeng L, Bao T, Ge J. Effectiveness of Omega-3 fatty acid for polycystic ovary syndrome: a systematic review and meta-analysis. Reprod Biol Endocrinol. (2018) 16:27. doi: 10.1186/s12958-018-0346-x
36. Salek M, Clark CCT, Taghizadeh M, Jafarnejad S. N-3 fatty acids as preventive and therapeutic agents in attenuating PCOS complications. EXCLI J. (2019) 18:558–75. doi: 10.17179/excli2019-1534
37. Greff D, Juhász AE, Váncsa S, Váradi A, Sipos Z, Szinte J, et al. D-D-Inositol is an effective and safe treatment in polycystic ovary syndrome: a systematic review and meta-analysis of randomized controlled trials. Reprod Biol Endocrinol. (2023) 21:10. doi: 10.1186/s12958-023-01055-z
38. Zhang B, Qi X, Zhao Y, Li R, Zhang C, Chang H-M, et al. Elevated CD14++CD16+ Monocytes in hyperhomocysteinemia-associated insulin resistance in polycystic ovary syndrome. Reprod Sci. (2018) 25:1629–36. doi: 10.1177/1933719118756772
39. Urjumelashvili M, Kristesashvili J, Asanidze E. Homocysteine level in patients with polycystic ovary syndrome with and without insulin resistance. Georgian Med News. (2022) 331:72–7.
40. Truitt C, Hoff WD, Deole R. Health functionalities of betaine in patients with homocystinuria. Front Nutr. (2021) 8:690359. doi: 10.3389/fnut.2021.690359
41. Yin J, Xing H, Ye J. Efficacy of berberine in patients with type 2 diabetes mellitus. Metabolism. (2008) 57:712–7. doi: 10.1016/j.metabol.2008.01.013
42. Zhang Y, Li X, Zou D, Liu W, Yang J, Zhu N, et al. Treatment of type 2 diabetes and dyslipidemia with the natural plant alkaloid berberine. J Clin Endocrinol Metab. (2008) 93:2559–65. doi: 10.1210/jc.2007-2404
43. Altieri DC. Survivin, versatile modulation of cell division and apoptosis in cancer. Oncogene. (2003) 22:8581–9. doi: 10.1038/sj.onc.1207113
44. Zhen H-N, Zhang X, Hu P-Z, Yang T-T, Fei Z, Zhang J-N, et al. Survivin expression and its relation with proliferation, apoptosis, and angiogenesis in brain gliomas. Cancer. (2005) 104:2775–83. doi: 10.1002/cncr.21490
45. Pan M-H, Ju J-Q, Li X-H, Xu Y, Wang J-D, Ren Y-P, et al. Inhibition of survivin induces spindle disorganization, chromosome misalignment, and DNA damage during mouse embryo development. Cell Cycle. (2020) 19:2148–57. doi: 10.1080/15384101.2020.1794545
46. Li S, Hao L, Hu X, Li L. A systematic study on the treatment of hepatitis B-related hepatocellular carcinoma with drugs based on bioinformatics and key target reverse network pharmacology and experimental verification. Infect Agent Cancer. (2023) 18:41. doi: 10.1186/s13027-023-00520-z
47. Sahebkar A. Are curcuminoids effective C-reactive protein-lowering agents in clinical practice? Evidence from a meta-analysis. Phytother Res. (2014) 28:633–42. doi: 10.1002/ptr.5045
48. Kotha RR, Luthria DL. Curcumin: biological, pharmaceutical, nutraceutical, and analytical aspects. Molecules. (2019) 24:2930. doi: 10.3390/molecules24162930
49. Shi SM, Di L. The role of carbonyl reductase 1 in drug discovery and development. Expert Opin Drug Metab Toxicol. (2017) 13:859–70. doi: 10.1080/17425255.2017.1356820
50. Fujiki H, Suganuma M, Okabe S, Sueoka E, Suga K, Imai K, et al. Mechanistic findings of green tea as cancer preventive for humans. Proc Soc Exp Biol Med. (1999) 220:225–8. doi: 10.1046/j.1525-1373.1999.d01-38.x
51. Zhang Z, Zhang X, Bi K, He Y, Yan W, Yang CS, et al. Potential protective mechanisms of green tea polyphenol EGCG against COVID-19. Trends Food Sci Technol. (2021) 114:11–24. doi: 10.1016/j.tifs.2021.05.023
Keywords: polycystic ovary syndrome, fertility nutrients, Mendelian Randomization, drug target Mendelian Randomization, reproductive health
Citation: Shao F, Xu S, Zhao H, Zhang F, Wang X and Wang H (2024) Causal relationship between fertility nutrients supplementation and PCOS risk: a Mendelian randomization study. Front. Endocrinol. 15:1420004. doi: 10.3389/fendo.2024.1420004
Received: 19 April 2024; Accepted: 03 September 2024;
Published: 24 September 2024.
Edited by:
Bassel H. Al Wattar, Anglia Ruskin University, United KingdomReviewed by:
Jiaqi Zhang, The First Affiliated Hospital of Dalian Medical University, ChinaYujia Zhang, Centers for Disease Control and Prevention (CDC), United States
Copyright © 2024 Shao, Xu, Zhao, Zhang, Wang and Wang. This is an open-access article distributed under the terms of the Creative Commons Attribution License (CC BY). The use, distribution or reproduction in other forums is permitted, provided the original author(s) and the copyright owner(s) are credited and that the original publication in this journal is cited, in accordance with accepted academic practice. No use, distribution or reproduction is permitted which does not comply with these terms.
*Correspondence: Hui Wang, anniewang@njmu.edu.cn