- 1First Department of Critical Care Medicine & Pulmonary Services, School of Medicine, National and Kapodistrian University of Athens, Evangelismos Hospital, Athens, Greece
- 2Biochemical Department, Evangelismos Hospital, Athens, Greece
- 3Department of Endocrinology, Diabetes and Metabolism, National Expertise Center for Rare Endocrine Diseases, Evangelismos Hospital, Athens, Greece
Objective: Critically ill patients, including those with brain injuries (BI), are frequently hospitalized in an intensive care unit (ICU). As with other critical states, an adequate stress response is essential for survival. Research on the hypothalamic-pituitary-adrenal gland (HPA) axis function in BI has primarily focused on assessing ACTH and cortisol levels. However, the immunological, metabolic, and hemodynamic effects of glucocorticoids (GCs) are mediated through the glucocorticoid receptor (GCR), a ubiquitously distributed intracellular receptor protein. Data on GCR-α expression and its signaling in acute BI injury are lacking.
Methods: We designed a prospective observational study, carried out in one academic multi-disciplinary ICU. Forty-two critically ill patients with acute (BI)were included. These patients suffered from traumatic BI (N= 20), subarachnoid hemorrhage (N= 12), intracranial hemorrhage (N= 7), or ischemic stroke (N= 3). All patients were steroid-free. Twenty-four age and sex-matched healthy controls were used for comparison.
Results: Expression of GCR-α and the glucocorticoid-inducible leucine zipper (GILZ), serum cortisol, interleukins (IL) 6, 8, 10 and TNF- α, and the BI biomarkers glial fibrillary acidic protein (GFAP) and total Tau were measured on ICU admission (within 48 hours) and 5-7 days from admission. Compared to healthy controls, in the critically ill patients with BI, GCR-α mRNA expression was significantly downregulated on admission, and after 5-7 days in the ICU (2.3-fold, p<0.05 and 2.6-fold, p<0.01, respectively). Even though GCR-α was downregulated, its downstream gene, GILZ, was expressed at the same levels as in normal controls on admission and was significantly upregulated 5-7 days following admission (2-fold, p<0.001). TNF-α levels were undetectable at both time-points. GCR-α expression levels inversely correlated with IL-6. The levels of cortisol and the BI biomarkers did not differ between the 2 time-points.
Conclusions: We provide novel evidence on the downregulated expression and upregulated signaling of the ligand-binding and functionally active GCR-α isoform in the polymorphonuclear neutrophils (PMNs) of critically ill patients with BI. The increased GILZ expression indicates an increased GC sensitivity in the PMNs of BI critically ill patients.
1 Introduction
Severe acute brain injury (BI), representing a significant percentage of intensive care unit (ICU) admissions (1), is primarily caused by traumatic brain injury (TBI), intracerebral hemorrhage (ICH), aneurysmal subarachnoid hemorrhage (aSAH), and acute ischemic stroke (AIS). In acute BI, as in other critical states, an adequate stress response is essential for survival (2). The stress response aims at restoring homeostasis and involves the activation of the hypothalamic–pituitary–adrenal (HPA) axis. This cascade involves the release of corticotropin-releasing hormone (CRH), adrenocorticotropic hormone (ACTH), and cortisol, a vital hormone in regulating stress response, inflammation, and metabolism. Cortisol then inhibits CRH and ACTH secretion via a negative feedback loop (3).
The management of acute brain injuries in the ICU remains a challenge. For many years, corticosteroids have been used in the treatment of patients with brain injuries, as they are believed to lower the intracranial pressure. However, the plethora of randomized controlled clinical and experimental studies exploring corticosteroids in BI have yielded conflicting results (4, 5).
Dysfunction of the HPA axis, mostly in the form of a hyporeactive HPA axis, has been documented in both acute traumatic and non-traumatic brain injuries, with evidence suggesting that these disruptions may persist even after recovery (6–11). BI may affect the HPA axis either by primary injury to the hypothalamic-hypophyseal region or by secondary events, including vascular damage, oedema, vasospasm, and inflammation, which in turn may affect adrenal function (12).
Research on HPA function in BI has primarily focused on assessing ACTH and cortisol levels, whether basal or stimulated. However, it is crucial to acknowledge that the immunological, metabolic, and hemodynamic effects of glucocorticoids (GCs) usually depend on its interaction with the ubiquitously expressed glucocorticoid receptor (GCR) in the cytoplasm. Two human major isoforms of GCR have been identified, with GCR-α being the predominant isoform of the receptor that binds to steroids (13). GCR-β does not bind GCs, it does not transactivate or transrepress target genes, however it acts as a dominant negative inhibitor of GCR-α activity (14). The cortisol-GCR complex directly binds to glucocorticoid responsive elements (GREs) found in GC-target genes, such as the glucocorticoid-inducible leucine zipper (GILZ), which is used as an indicator of glucocorticoid signaling due to its responsiveness to glucocorticoid stimulation (15). Apart from these classical genomic effects via the intracellular GCR, GCs have been found to also exert rapid non-genomic effects by several mechanisms including the activation of a membrane-bound glucocorticoid receptor (mGCR) (16–19).
Dysfunction at the GCR level is equally significant and warrants attention, however no information on GCR signaling is available in BI patients. In a prior study involving unselected critically ill patients, our findings revealed elevated levels of GCR-α, yet without concurrent induction of GILZ expression on ICU admission, suggesting a state of GC resistance (20). On the other hand, in a subsequent study from our group involving ICU COVID-19 patients, both GCR-α and GILZ expression levels were increased, indicating a robust activation of the HPA axis (21). These findings suggest that variations in GCR-α expression and function, as evaluated through GILZ, may exist across different causes of critical illness.
The objective of the present study was to examine the expression of GCR-α and GILZ in critically ill patients with traumatic and non-traumatic BI. This population differs from the general ICU cohorts in that the primary pathology involves direct damage to the brain tissue, which could also result in reduced adrenal stimulation. In this setting, the response of target cells to circulating steroids may be critical. Therefore, we designed a prospective, observational study in which we measured the expression of GCR-α and GILZ in the polymorphonuclear neutrophils (PMNs) of ICU patients with BI and compared their expression to normal controls. Furthermore, we explored possible correlations of GCR-α and GILZ expression with BI biomarkers and with cytokines implicated in the GC response during critical illness.
2 Materials and methods
2.1 Ethical approval
The study was approved by the ‘Evangelismos’ Hospital Research Ethics Committee (467/11-1-2023, Study of ICU patients with brain injury: the role of glucocorticoid receptors and newer brain injury biomarkers on their course, prognosis, and outcomes) and all procedures carried out on patients were in compliance with the Helsinki Declaration. Informed written consent was obtained from all patients’ next-of-kin.
2.2 Study design
A prospective, single-center, observational study of 42 ICU patients with BI hospitalized in “Evangelismos” General Hospital (Athens, Greece), over a twelve-month period (January 2023 – December 2023). A control group of 24 healthy subjects, matched for age and sex was also included.
2.3 Study population
Prior to enrolment, we screened all consecutive patients for eligibility. Inclusion criteria were new (<48 h) ICU admissions with a diagnosis of BI (including TBI, aSAH, ICH, and AIS). Exclusion criteria were time from initial admission to eligibility assessment >48h, age less than 18 years, pregnancy, diagnosis of brain death, imminent death, estimated length of ICU stay <72 hours, re-admission or transfer from another ICU, contagious diseases (human immunodeficiency virus, hepatitis), oral or inhaled intake of corticosteroids for a period of more than one month prior to ICU admission, and administration of steroids during ICU stay (e.g. hydrocortisone for septic shock). Upon admission in the ICU, the following variables were recorded: age, sex, comorbidities, BI diagnosis, acute physiology and chronic health evaluation (APACHE II) score, sequential organ failure (SOFA) score, vital signs, and laboratory data. The following scales were used for grading the patients’ severity: The Glasgow coma scale (GCS), the Marshal CT classification system (CT score for TBI), the Fisher scale (CT score for aSAH), the World federation of neurological surgeons scale (WFNS) (clinical score for aSAH), the Alberta stroke program early CT score (ASPECTS) (CT score for AIS), and intracerebral blood volume estimation was assessed with the ABC/2 score. Duration of mechanical ventilation and ICU stay along with mortality (28-day and ICU mortality) were also noted. Twenty-four age- and sex-matched healthy blood donors comprised the control group and were used for comparisons.
2.4 Blood collection – polymorphonuclear neutrophil isolation
Venous blood samples were drawn from the critically ill BI patients between 08.00 and 08.30 h at two time points: within 48 hours from admission (baseline; 42 patients) and thereafter, at days 5-7 (second time-point, 23 patients). A second blood sample was not obtained when steroid administration due to mainly septic shock, allergic reactions, airway oedema, bronchospasm, transfer to another ICU, or if death occurred. Figure 1 illustrates the study flow diagram of the enrolment and sampling process.
A single blood sample was also drawn from 24 healthy blood donors between 08.00 and 08.30 h. Blood samples were collected in red top Vacutainers tubes (BD Biosciences, Franklin Lakes, NJ, USA) for serum preparation and blue top Vacutainers citrate tubes (BD Biosciences) for polymorhonuclear neutrophil (PMN) isolation. PMNs were isolated from the peripheral blood, as previously described (14). Briefly, blood was collected in 17% citric acid and allowed to sediment in 3% Dextran/0.9% NaCl for 1 hr. Leukocyte-rich supernatant was collected and any remaining erythrocytes were lysed by hypotonic shock. PMNs were separated by gradient centrifugation on Histopaque (Sigma, Sigma-Aldrich Corp., St Louis, MO, USA) and the isolated PMNs were resuspended in Trizol and stored at -80°C until RNA extraction.
2.5 Total RNA extraction and quantitative reverse-transcription PCR
Total RNA was isolated from PMNs, the RNA was reverse transcribed, and a highly sensitive validated quantitative real-time PCR method was used for the quantification of GAPDH, GCR-α, and GILZ mRNAs using specific primers (20, 22). Using the comparative CT method 2−DDCT (23) and the healthy donor samples as a calibrator, the relative quantification of the expression analysis of all blood samples from the critically ill patients was carried out. GAPDH expression was used for the normalization of the target gene expression levels between the different samples.
2.6 Total cortisol measurement
Total cortisol was determined in the morning blood samples with the Elecsys Cortisol II assay (Roche Diagnostics GmbH, Mannheim, Germany; detection limit 0.054 μg/dl and coefficient of variation 3.1%) with the electrochemiluminescence immunoassay “ECLIA” on a cobas e 411 immunoassay analyzer (Hitachi for Roche Diagnostics International AG, Rotkreuz, Switzerland). The samples were run in duplicates and cortisol levels were expressed in μg/dl. The expected morning normal values of the assay are 4.82‐19.5 μg/dl.
2.7 Measurement of cytokines and brain injury biomarkers
The human interleukin (IL) - 6, 8, 10, and tumor necrosis factor (TNF)-α immunoassays from Invitrogen (Thermo Fisher Scientific Inc., Waltham, MA, USA; detection limits IL-6, 0.92 pg/ml; IL-8, 2 pg/ml; IL-10, 2 pg/ml; TNF-α, 5 pg/ml), glial fibrillary acidic protein (GFAP) (OriGene Technologies, Inc., Rockville, MD, USA; detection limit 85 pg/ml), and microtubule-associated tau protein (total Tau) (Wuhan Fine Biotech Co., Ltd.; detection limit 4.688 pg/ml) were used for the measurements, according to the manufacturers’ instructions.
2.8 Statistical analysis
Data are presented as individual values, mean ± standard deviation (SD), or median with interquartile range (IQR). Two-group comparisons were performed using the Student’s t-test, the non-parametric Mann-Whitney test, or the Wilcoxon matched-pairs signed rank test, as appropriate. One-way ANOVA for repeated measures and Kruskal-Wallis ANOVA followed by Dunn’s post hoc test was used for more than two groups comparisons. Correlations were performed by Spearman’s correlation coefficient. All analyses were performed using the GraphPad Prism 6 statistical program (GraphPad Software, Inc). All p values are two-sided; p< 0.05 were considered significant. Prior to the study a power analysis was performed. Based on a previous study on GCR-α expression in PMN cells (12), to detect a 4.7-fold increase of GCR-α expression with a Cohen-d effect size of 0.876, an α-error probability <0.05, and a power >80%, it was estimated that a cohort of 42 critically ill patients and a group of 15 matched healthy controls would suffice.
3 Results
3.1 Characteristics of the study population
Of the 305 patients diagnosed with acute BI, 42 patients were included in the study. The most common cause of exclusion was steroid use prior to ICU admission. Of the 42 patients, 23 patients underwent a second blood sampling. Reasons for not completing the two-step sampling process were administration of steroids (N= 16), death (N= 2), or transfer to another ICU (N= 1). Patients’ demographics, clinical characteristics, and laboratory data on ICU admission, and outcomes are presented in Table 1. The BI-related clinical and radiological characteristics are listed in Table 2.
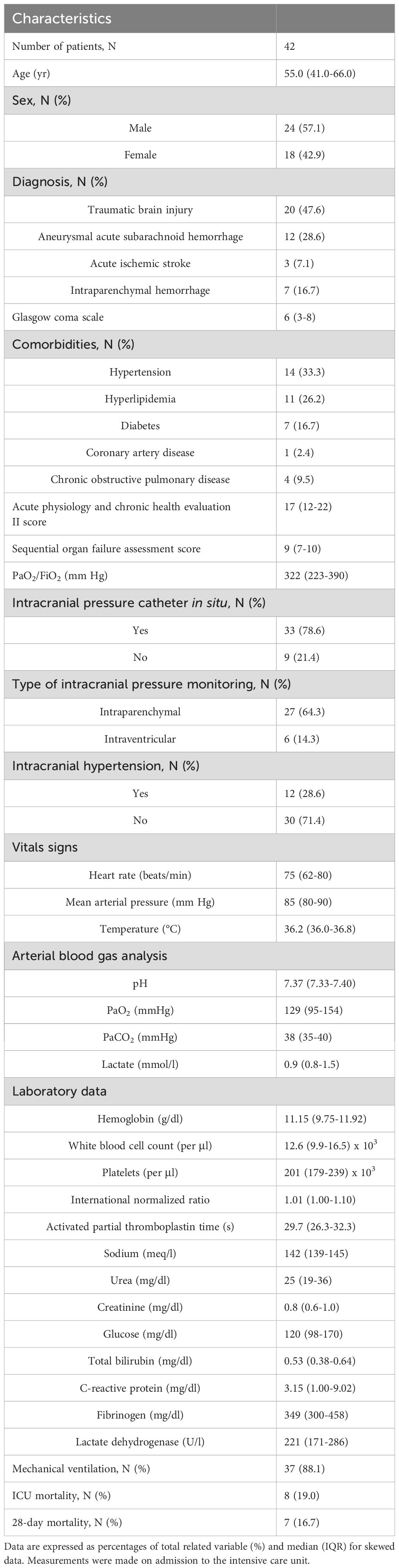
Table 1. Patient demographics, clinical characteristics, laboratory data, and outcomes of the study population.
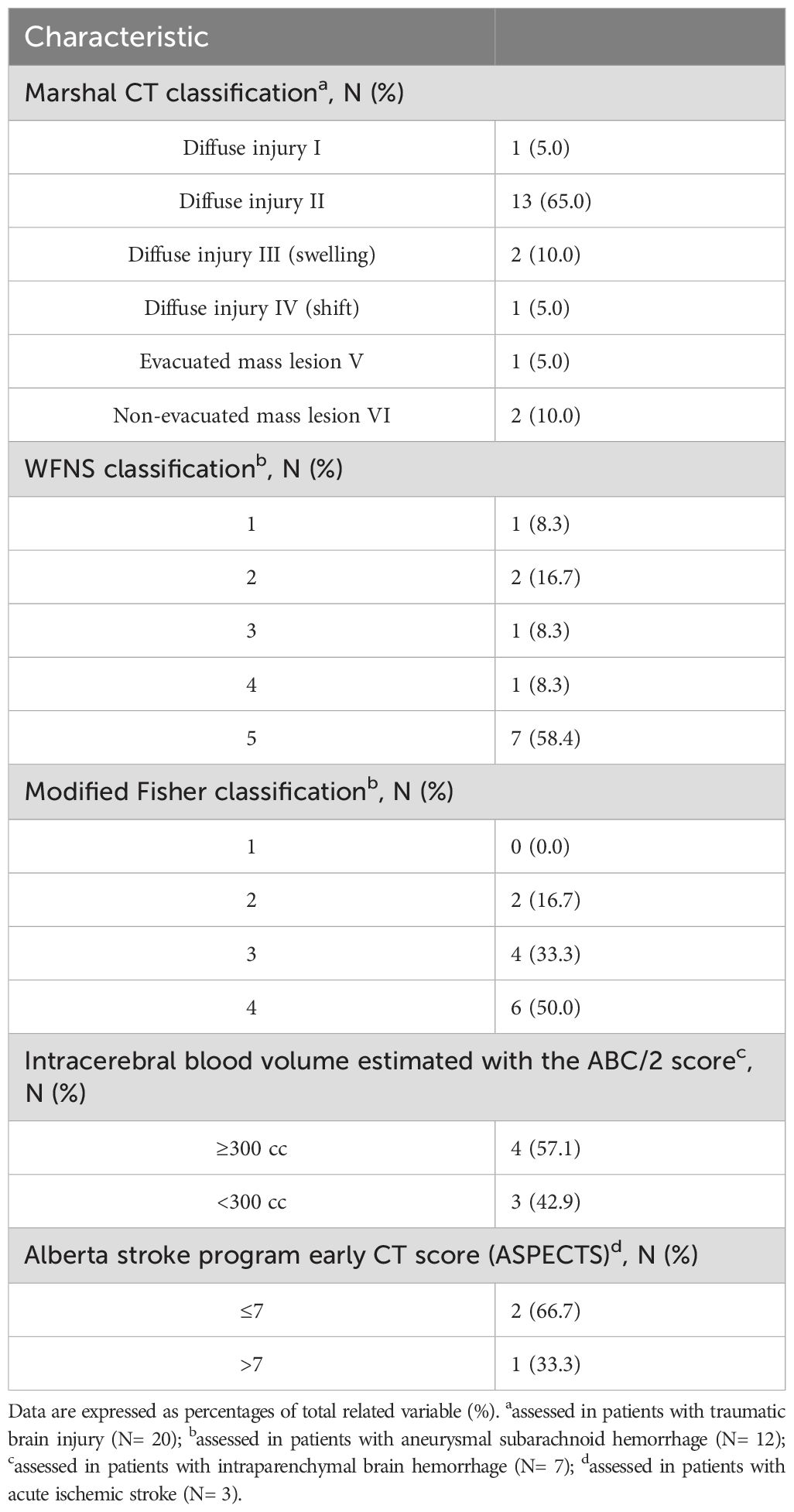
Table 2. Clinical and radiological characteristics of the study population on admission to the intensive care unit.
3.2 GCR-α expression in critically ill patients with brain injury
A control group comprised of 24 healthy blood donors, 54.2% male, with a median age of 53 (47–55) years was used for the relative quantification of the expression analysis of the critically ill patients. Compared to healthy controls, critically ill patients with BI had decreased GCR-α expression on ICU admission [0.60 (0.36-1.14); Figure 2A; p= 0.014]. Likewise, during ICU stay, on day 5-7, the decreased expression was maintained [0.53 (0.32-0.71); Figure 2A; p= 0.0018)]. For the 23 patients who had two blood draws, we also used repeated measures analysis to determine whether expression changed between time points. We found no differences between the two time-points [0.49 (0.36-0.79) vs 0.53 (0.32-0.71); Figure 2B; p> 0.05].
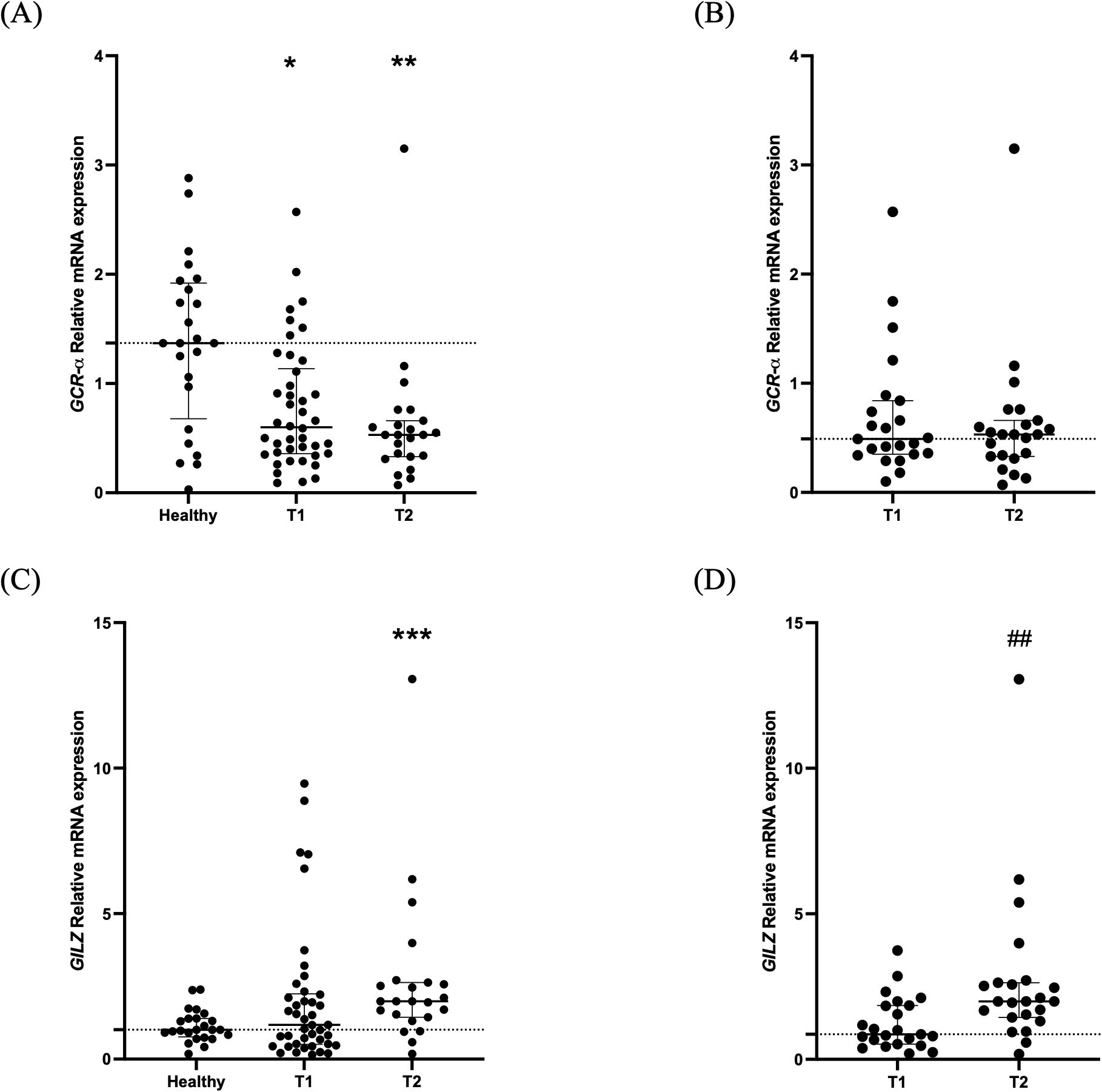
Figure 2. GCR-α expression and signaling in critically ill patients with brain injury. Distribution of GCR-α expression (A), and GILZ expression (C) in 42 critically ill patients with brain injury on ICU admission (within 48 hours-T1, N= 42) and 5-7 days after (T2, N= 23). Distribution of GCR-α expression (B) and GILZ expression (D) in the 23 critically ill brain injury patients with samples at both time-points. Data are presented as scatter plots, indicating the median value and 25th to 75th centiles. Dashed horizontal line depicts the median value of the healthy subjects (A, C) or of the brain injury patients at T1 (N= 23; B, D). (A, C), *p< 0.05, ** p< 0.01, and ***p< 0.001 by Mann-Whitney test compared to the healthy controls; (B, D), ##p< 0.01 by the Wilcoxon matched-pairs signed rank test compared to T1.
The patients were then assigned to 2 subgroups based on diagnosis; patients with TBI (N= 20) and patients with non-traumatic BI (NTBI-ICH, aSAH, and AIS, N= 22). GCR-a expression was decreased at both time-points compared to the healthy controls in the TBI subgroup [T1, 0.53 (0.35-0.90) and T2 (N=12), 0.54 (0.17-0.62), p< 0.01]. In the NTBI subgroup, there was a tendency for lower expression at both time-points [T1, 0.62 (0.37-1.32), p= 0.051 and T2 (N= 11), 0.45 (0.34-0.89), p= 0.069]. When the two subgroups were compared against each other, no differences in GCR-α expression were found in either time-point (all p> 0.05).
Lastly, when the patients were divided in survivors and non-survivors according to 28-day mortality, GCR-α expression on admission was 0.60 (0.36-0.93) in the patients who survived vs 0.86 (0.29-1.95) in the non-survivors (p= 0.41).
3.3 GCR-α signaling/GILZ expression in critically ill patients with brain injury
Compared to healthy controls, critically ill patients had a comparable mRNA expression of the GC-inducible gene GILZ on ICU admission [1.18 (0.51-2.25); Figure 2B; p= 0.55). On day 5-7, GILZ levels increased [1.99 (1.38-2.67; Figure 2C; p= 0.0007). As in GCR-α, we also compared GILZ expression between the two time-points for the 23 patients who had two blood samples. We found an increase in the second time-point [0.86 (0.50-1.92) vs 1.99 (1.38-2.67); Figure 2D; p< 0.01].
GILZ expression was similar to healthy controls on ICU admission and increased at the 2nd time-point in both TBI and NTBI subgroups. Specifically, for TBI [0.95 (0.56-2.08), p> 0.05 and 2.05 (1.37-2.69), p< 0.05, respectively]. For NTBI [1.44 (0.44-2.39), p> 0.05 and 1.95 (1.19-3.26, p< 0.05, respectively]. GILZ expression did not differ between the two BI subgroups at either time-point (all p> 0.05).
Finally, on admission GILZ expression was comparable between 28-day survivors and non-survivors [1.27 (0.51-2.25) vs. 1.11 (0.53-5.69), respectively, p= 0.96].
3.4 Cortisol, cytokines, and BI biomarker levels in critically ill patients with brain injury
Cortisol levels did not differ between the two time-points [7.9 (4.1-25.4) μg/dl vs 16.9 (8.5-23.3) μg/dl; Figure 3A; p= 0.26]. The change in cytokine levels during the 7-day period is demonstrated in Figures 3B-D. IL-6 levels were higher on ICU admission compared to day 5-7 [75.3 (21.6-128.0) pg/ml vs 25.1 (10.5-48.4) pg/ml; Figure 3B; p= 0.018]. IL-8 and IL-10 median levels were within normal limits on admission and remained unchanged [38.1 (25.1-49.3) pg/ml vs 36.6 (24.3-47.4) pg/ml; Figure 3C; p= 0.74] and [7.4 (5.0-20.6) pg/ml vs 6.5 (3.4-12.7) pg/ml; Figure 3D; p= 0.26]. TNF-α levels were undetectable on ICU admission and during ICU stay.
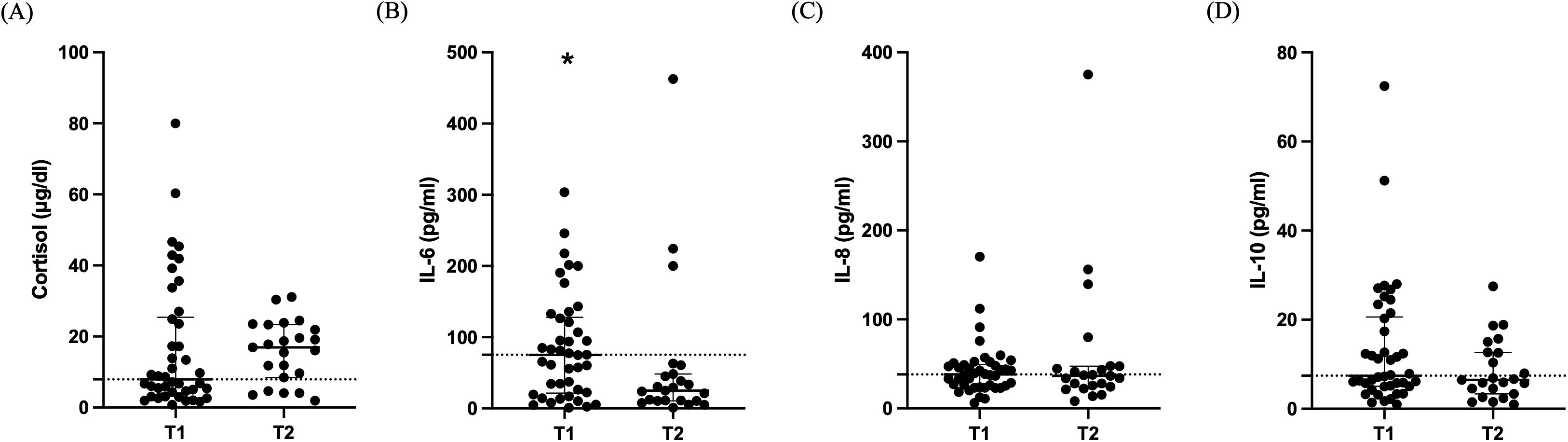
Figure 3. Cortisol and cytokines in critically ill patients with brain injury. Distribution of cortisol (A), IL-6 (B), IL-8 (C), and IL-10 (D) in 42 critically ill patients with brain injury on ICU admission (within 48 hours-T1, N= 42) and 5-7 days after (T2, N= 23). Data are presented as scatter plots, indicating the median value and 25th to 75th centiles. Dashed horizontal line depicts the median value of the brain injury patients at T1. *p< 0.05 by Mann-Whitney test.
Regarding the BI biomarkers, GFAP levels were high at both time-points [1.022 (0.085-10.51) ng/ml vs 0.951 (0.085-10.66) ng/ml; Figure 4A; p= 0.92]. Similarly, total Tau levels were elevated [46.2 (9.4-131.6) pg/ml vs 84.8 (22.1-185.2) pg/ml; Figure 4B; p= 0.22].
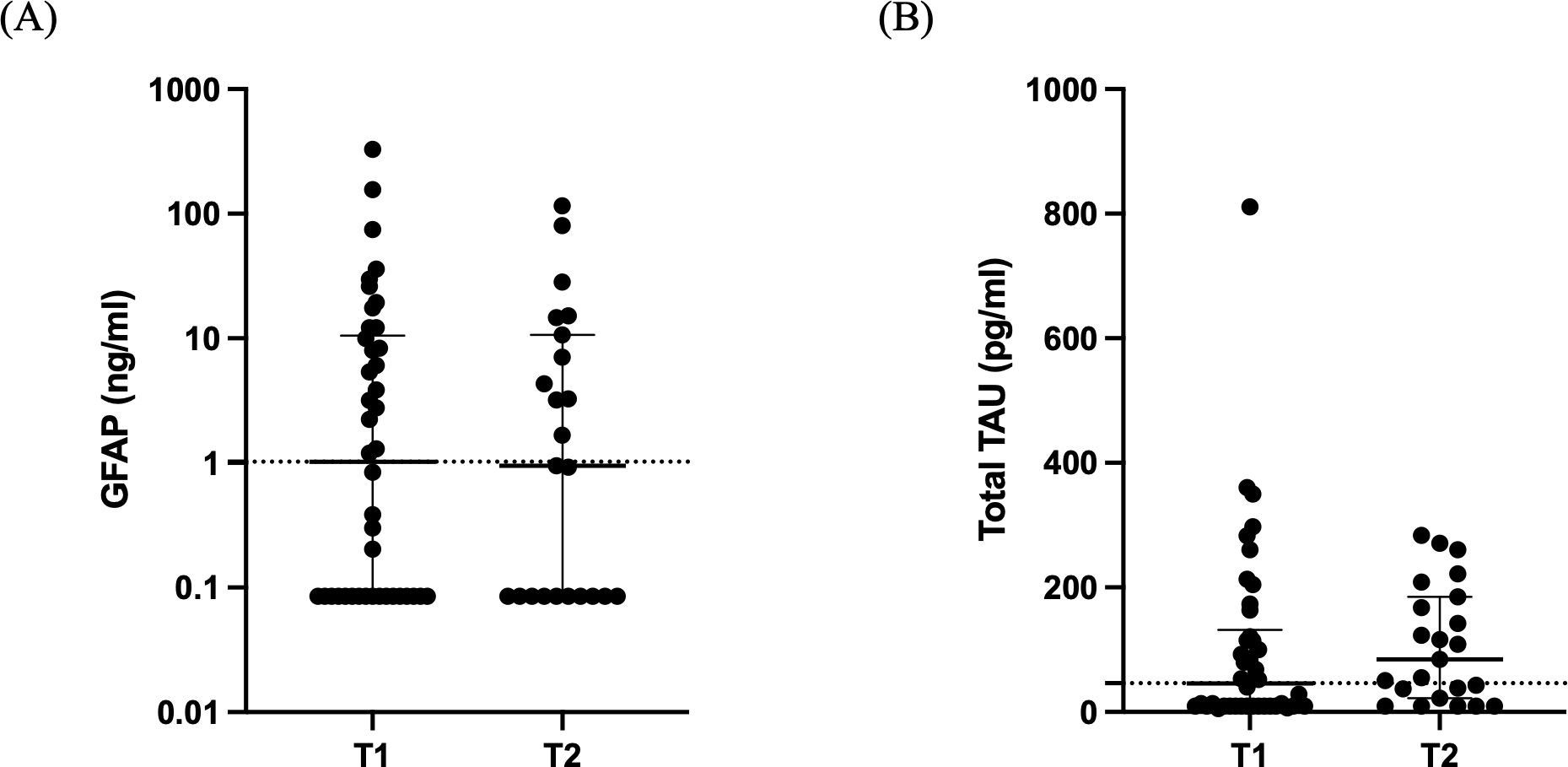
Figure 4. Brain injury biomarkers. Distribution of GFAP (A) and total Tau (B) in 42 critically ill patients with brain injury on ICU admission (within 48 hours-T1, N= 42) and 5-7 days after (T2, N= 23). Data are presented as scatter plots, indicating the median value and 25th to 75th centiles. Dashed horizontal line depicts the median value of the brain injury patients at T1. GFAP, glial fibrillary acidic protein.
3.5 Correlations of genes’ expression, cortisol, cytokines, and brain injury biomarkers
On admission, GCR-α expression inversely correlated with IL-6 and IL-10 levels (r= -0.36, p= 0.018 and r= -0.33, p= 0.034, respectively), but not with IL-8 (Figures 5A, B). Only the inverse correlation with IL-6 remained at the second time-point (r= -0.62, p= 0.003; Figures 5D, E). GILZ expression inversely correlated with IL-6 levels only (r= -0.38, p= 0.012) on ICU admission (Figures 5C, F). Of note, in the healthy patients expression of GCR-α and GILZ strongly correlated (r= 0.59, p= 0.003; Figure 5G), whereas this correlation was not found in the BI patients in either time-point (p= 0.74 and p= 0.26, respectively; Figures 5H, I). GCR-α and GILZ expression did not correlate with cortisol levels, nor with the BI biomarkers GFAP and total Tau. Moreover, no correlations were seen between GILZ expression levels and the clinical and radiological characteristics. The statistically significant correlations of gene expression and cytokine levels are depicted in Figure 5, while in Table 3 all correlations between gene expression, cortisol, cytokines, BI biomarkers, and clinical and radiological characteristics are shown.
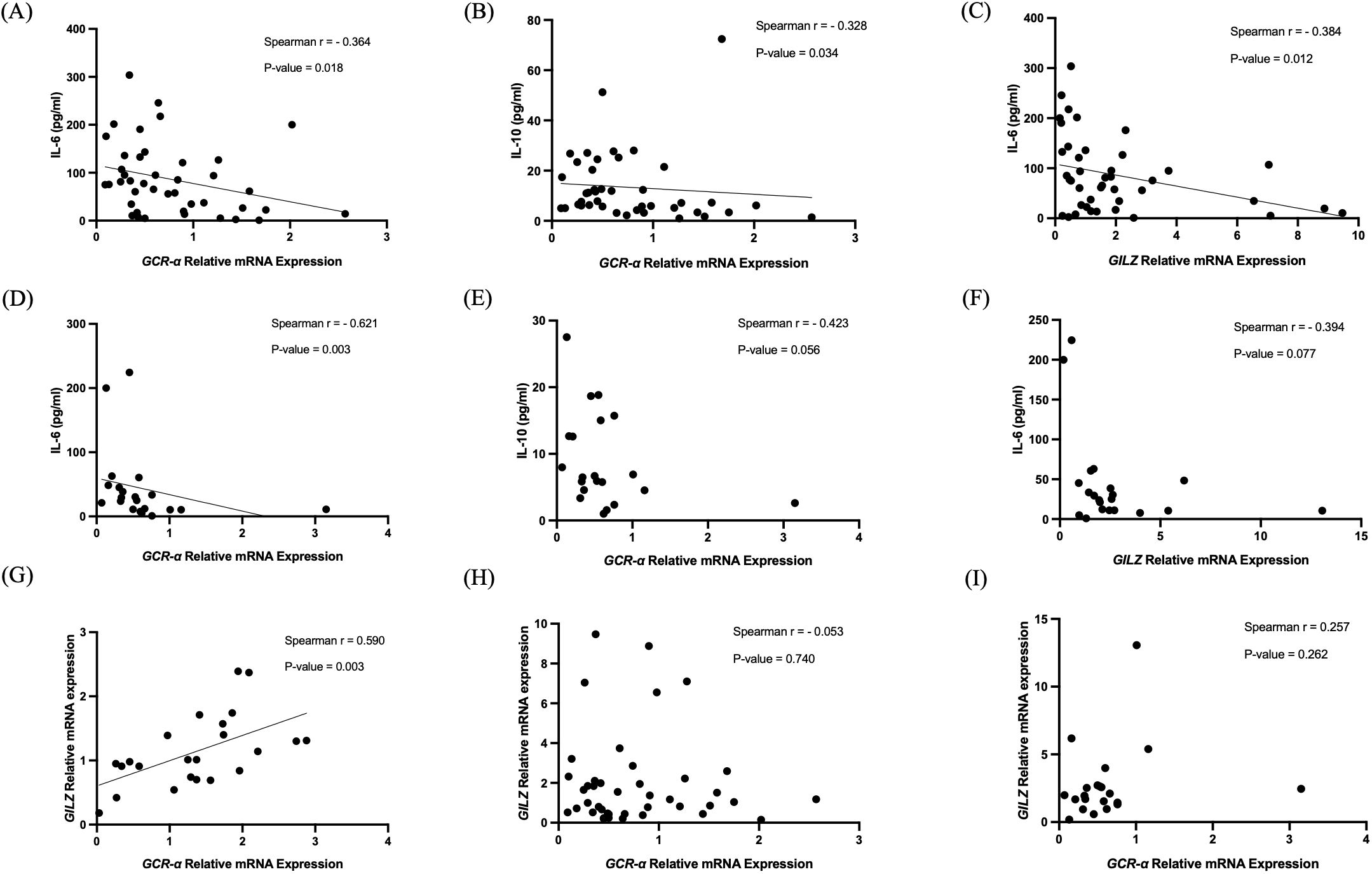
Figure 5. Correlations of GCR-α and GILZ mRNA expression. Correlation of GCR-α mRNA expression levels with IL-6 (A, D) and IL-10 (B, E) on ICU admission (within 48 hours-T1) and 5-7 days after (T2), correlation of GILZ mRNA expression levels with IL-6 (C, F) on ICU admission (within 48 hours-T1) and 5-7 days after (T2), and correlations between GCR-α and GILZ mRNA expression levels in healthy controls (G) and in BI patients at both time-points (H, I). Statistical analysis was performed using the Spearman’s correlation coefficient.
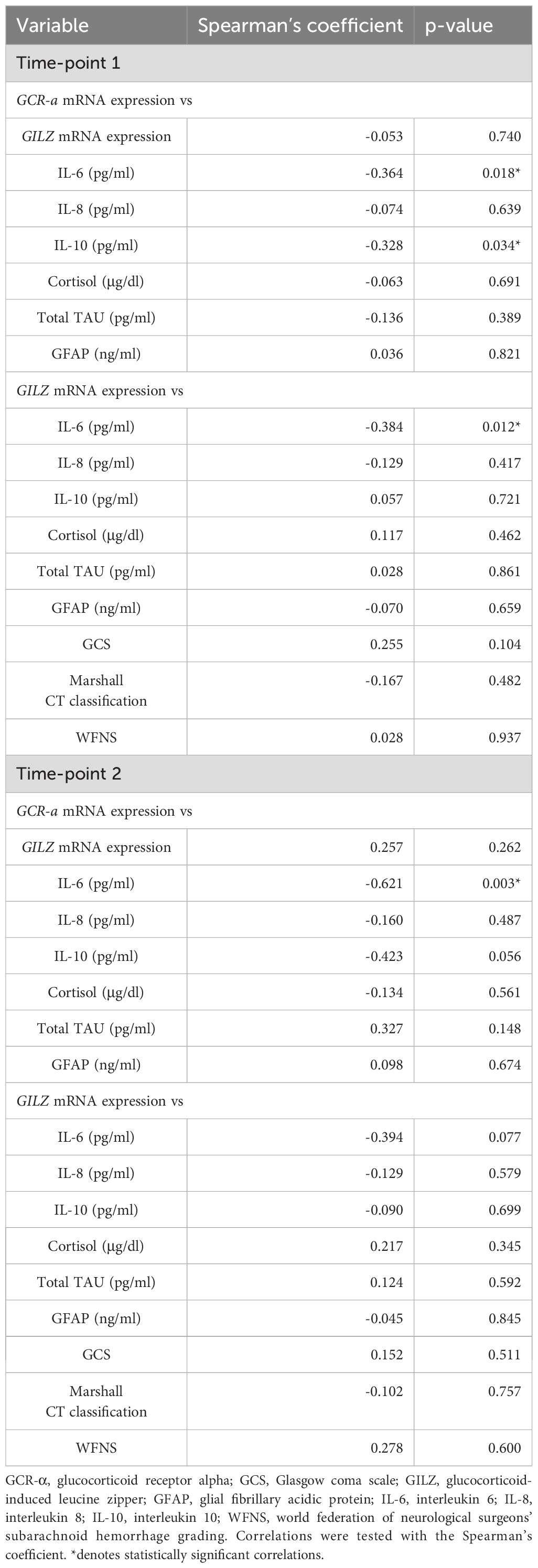
Table 3. Correlations of GCR-α and GILZ mRNA expression in BI patients with cortisol, cytokines, BI biomarkers, and clinical and radiological characteristics.
4 Discussion
In this prospective study we assessed GCR-α and GILZ expression, as well as serum cortisol, cytokines, and BI biomarker levels in critically ill BI patients. The novel finding was that compared to healthy controls, GCR-α expression was low in the PMNs of critically ill BI patients, and cortisol levels were not increased. Nevertheless, GCR signaling occurred, as confirmed by increased expression of GILZ, suggesting that our critically ill BI patient cohort does not exhibit GC resistance. Of note, IL-6 levels exhibited an inverse correlation with both GCR-α and GILZ expression levels, which aligns with the established suppression of expression of the IL-6 gene by GCs through the GCR-α. Finally, although GCR-a and GILZ expression levels significantly correlated in the healthy controls, this correlation was not seen in BI patients. Overall, our findings suggest that BI patients comprise a distinct subpopulation within the spectrum of critical illness.
While traumatic and non-traumatic BI differ in etiologies, they encompass acquired BI with shared pathophysiology (24). Few studies have assessed GCR expression, number, and affinity, or GILZ expression in critically ill patients. These studies have mostly focused on heterogeneous ICU populations, mostly comprising septic patients, and have yielded varied and occasionally conflicting outcomes. While some studies have reported normal GCR count and affinity (25), others have reported evidence of GC resistance, characterized by reduced affinity in peripheral blood mononuclear leukocytes (26), diminished GCR-α expression in T-cells (27), and transient increases in GCR-β expression in mononuclear cells during sepsis (28). Conversely, increased GCR expression and cortisol levels in whole blood during acute-phase sepsis has also been reported (29), implying heightened GC sensitivity. However, elevated GCR-α levels do not necessarily indicate increased GC sensitivity, requiring an assessment of GILZ levels to demonstrate effective GC signaling. In a pilot study it was shown that neutrophils from patients with acute respiratory distress syndrome (ARDS) can express GILZ, whose levels were related to ARDS severity (30).
The concomitant assessment of GCR-α and GILZ expression has been explored only recently. We have previously found elevated GCR-α expression in the neutrophils of critically ill patients of various causes upon ICU admission, with no induction of GILZ, indicating ineffective GC signaling (20, 22). In a different ICU cohort with viral sepsis due to COVID-19 we observed increased GCR-α and GILZ expression in whole blood, suggesting either appropriate HPA axis activation or differential GILZ expression among blood cells. The latter is supported by a study of Téblick et al. on a cohort primarily consisting of septic critically ill patients, which demonstrated substantial suppression of GCR-α and GILZ in neutrophils throughout ICU stay, while monocytes exhibited low/normal GCR-α levels alongside increased GILZ levels (31). Taken together, it becomes evident that the observed changes in GCR-α and GILZ expression represent adaptations to the underlying causes of critical illness and may be tissue and cell-specific, and time-dependent.
In BI patients, we initially observed normal GILZ expression levels despite low GCR-α expression, which increased at a later stage (5-7 days) without a corresponding rise in total cortisol levels. Although increased free cortisol levels might explain these findings, there is limited data on corticosteroid-binding globulins (CBGs) and free cortisol levels in brain injury patients, with one study reporting low free cortisol levels (32). Moreover, CBGs are not expected to decrease as significantly as in sepsis-associated critical illness. Therefore, we believe that the most plausible explanation is the lack of significant GC resistance initially in this cohort, contrasting with critically ill patients of other etiologies, where we observed increased GCR-α expression and low GILZ expression levels (20, 22), indicative of GC resistance, and further improvement of GC sensitivity as the inflammatory response subsides.
An explanation for the observed absence of notable GC resistance is that our BI patients had a low-grade inflammatory response, as evident by the undetectable levels of TNF-α, which contrasts with previously studied critically ill populations. This distinction is important since the inflammatory response may have an impact on GCR signaling. Pro-inflammatory cytokines can regulate human GCR gene expression. They can either inhibit expression by reshaping the GCR nuclear cofactor profile (33) or increase the steady-state levels of the GCR-β protein isoform over GCR-α, making GCR-β the predominant endogenous receptor isoform, thereby disrupting GC action (34). The increased ratio of the beta isoform to the alpha may in turn increase neutrophil insensitivity towards GCs (35). Hence, data suggest that inflammation itself may contribute to GC resistance (34, 36–38), and thus the absence of significant inflammation may explain the lack of GC resistance in this BI cohort.
The observed inverse relationship between GILZ expression levels and IL-6 levels aligns with cortisol’s established role as a suppressor of IL-6, further supporting the notion of an enhanced GC effect in this cohort. Certainly, additional factors such as other hormones or cytokines might also stimulate GILZ expression, potentially contributing to the observed upregulation of GILZ in our population. Furthermore, we cannot exclude the potential of aldosterone inducing GILZ expression through the mineralocorticoid receptor (MR) (39, 40), a receptor also present in the PMNs (41).
In 2005, a large randomized controlled trial on 10,008 adults tested the effect of corticosteroids on death and disability following all head injuries with some Glasgow coma scale (GCS) abnormality. High doses of GCs were related to increased mortality, attributed to immunosuppressive effects, leading to the suggestion that corticosteroids should not be used routinely in the treatment of head injury (42). The results of the CRASH trial may not be applicable uniformly to all brain injury patients and there may be a certain subset of patients who may benefit from corticosteroids. It may be hypothesized that our group of BI patients is not expected to benefit from exogenous administration of GCs, as those administered in the CRASH trial.
Finally, despite the strong correlation between GCR-α and GILZ expression in healthy subjects, this was notably absent in BI patients at both time-points. Interestingly, in a prior study on COVID-19 sepsis we found a robust correlation between the expression levels of GILZ and GCR-α (21). The lack of correlation suggests a dissociation between the adrenal response, GCR expression, and signaling in BI. This finding warrants further exploration, since it cannot be attributed to BI severity, as indicated by the lack of any significant associations of GCR-α and GILZ expression levels with serum levels of BI biomarkers, clinical and radiological characteristics, or with the observed patient outcomes.
Amongst the strengths of our study is that, to the best of our knowledge, this is the first study that specifically investigated both GCR-α and GILZ expression in BI cases, whereas in the previous studies HPA assessment was explored solely through ACTH and cortisol measurements (9, 43). Furthermore, the inclusion of a relatively homogenous BI cohort, with a relatively uniform profile with elevated levels of BI biomarkers, who did not receive exogenous steroid administration and were not confounded by sepsis, constitutes another strength of the present study.
The limitations of our study should also be stated. First, it involved a relatively small number of patients, yet similar to the size of other cohorts examined in prior studies investigating the role of GCR expression levels in critical illness. Second, GCR-a and GILZ expression measurements were performed in PMNs, rather than monocytes/macrophages, which are considered more relevant circulating target cells for corticosteroid-mediated immune host response in critical illness. Cortisol and cytokine levels were not measured in the healthy blood donors. We only measured GCR-α mRNA expression levels, and not its protein levels. We also only measured total cortisol levels. Free cortisol measurement mirrors better cortisol availability (44), however it is not readily available, and hence total cortisol concentration remains the most suitable marker of adrenal function. Moreover, we have previously shown that in critical illness, tissue cortisol levels correlate to a moderate degree with both total and free cortisol (45). Finally, we did not explore alternative pathways regulating GILZ expression, including aldosterone and vasopressin.
5 Conclusions
Our data suggest a unique hypothalamic–pituitary–adrenal (HPA) activation pattern in critically ill patients without significant inflammatory responses, such as those with BI. We provide novel evidence on the downregulated expression and upregulated signaling of the ligand-binding and functionally active GCR-α isoform in the PMNs of critically ill patients with BI. The increased GILZ expression indicates an increased GC sensitivity in the PMNs of BI critically ill patients, suggesting an effective and likely appropriate response to the stress induced by BI, potentially negating the necessity for exogenous steroid administration.
Data availability statement
The raw data supporting the conclusions of this article will be made available by the authors, without undue reservation.
Ethics statement
The studies involving humans were approved by‘Evangelismos’ Hospital Research Ethics Committee. The studies were conducted in accordance with the local legislation and institutional requirements. The participants provided their written informed consent to participate in this study.
Author contributions
NSL: Formal analysis, Investigation, Methodology, Data curation, Validation, Writing – original draft. CSV: Formal analysis, Validation, Conceptualization, Supervision, Visualization, Writing – review & editing. GP: Formal analysis, Data curation, Investigation, Methodology, Writing – original draft. AC: Data curation, Investigation, Methodology, Writing – original draft. CK: Data curation, Investigation, Methodology, Writing – original draft. MP: Data curation, Investigation, Methodology, Writing – original draft. VG: Data curation, Investigation, Methodology, Writing – original draft. AK: Project administration, Resources, Supervision, Writing – review & editing. DAV: Writing – review & editing, Conceptualization, Formal analysis, Validation, Visualization. ID: Conceptualization, Validation, Visualization, Writing – review & editing, Project administration, Resources, Supervision. AGV: Supervision, Visualization, Writing – review & editing, Conceptualization, Formal analysis, Investigation, Methodology.
Funding
The author(s) declare that no financial support was received for the research, authorship, and/or publication of this article.
Conflict of interest
The authors declare that the research was conducted in the absence of any commercial or financial relationships that could be construed as a potential conflict of interest.
Publisher’s note
All claims expressed in this article are solely those of the authors and do not necessarily represent those of their affiliated organizations, or those of the publisher, the editors and the reviewers. Any product that may be evaluated in this article, or claim that may be made by its manufacturer, is not guaranteed or endorsed by the publisher.
References
1. Pelosi P, Ferguson ND, Frutos-Vivar F, Anzueto A, Putensen C, Raymondos K, et al. Management and outcome of mechanically ventilated neurologic patients. Crit Care Med. (2011) 39:1482–92. doi: 10.1097/CCM.0b013e31821209a8
2. Weil ZM, White B, Whitehead B, Karelina K. The role of the stress system in recovery after traumatic brain injury: A tribute to bruce S. Mcewen. Neurobiol Stress. (2022) 19:864858. doi: 10.3389/fimmu.2022.864858
3. Vassiliadi DA, Vassiliou AG, Ilias I, Tsagarakis S, Kotanidou A, Dimopoulou I. Pituitary-adrenal responses and glucocorticoid receptor expression in critically ill patients with covid-19. Int J Mol Sci. (2021) 22. doi: 10.3390/ijms222111473
4. Alderson P, Roberts I. Corticosteroids in acute traumatic brain injury: systematic review of randomised controlled trials. Bmj. (1997) 314:1855–9. doi: 10.1136/bmj.314.7098.1855
5. Taylor MA, Kokiko-Cochran ON. Context is key: glucocorticoid receptor and corticosteroid therapeutics in outcomes after traumatic brain injury. Front Cell Neurosci. (2024) 18:1351685. doi: 10.3389/fncel.2024.1351685
6. Dimopoulou I, Kouyialis AT, Orfanos S, Armaganidis A, Tzanela M, Thalassinos N, et al. Endocrine alterations in critically ill patients with stroke during the early recovery period. Neurocrit Care. (2005) 3:224–9. doi: 10.1385/ncc:3:3:224
7. Dimopoulou I, Kouyialis AT, Tzanella M, Armaganidis A, Thalassinos N, Sakas DE, et al. High incidence of neuroendocrine dysfunction in long-term survivors of aneurysmal subarachnoid hemorrhage. Stroke. (2004) 35:2884–9. doi: 10.1161/01.Str.0000147716.45571.45
8. Dimopoulou I, Tsagarakis S. Hypothalamic-pituitary dysfunction in critically ill patients with traumatic and nontraumatic brain injury. Intensive Care Med. (2005) 31:1020–8. doi: 10.1007/s00134-005-2689-y
9. Dimopoulou I, Tsagarakis S, Kouyialis AT, Roussou P, Assithianakis G, Christoforaki M, et al. Hypothalamic-pituitary-adrenal axis dysfunction in critically ill patients with traumatic brain injury: incidence, pathophysiology, and relationship to vasopressor dependence and peripheral interleukin-6 levels. Crit Care Med. (2004) 32:404–8. doi: 10.1097/01.CCM.0000108885.37811.CA
10. Garrahy A, Sherlock M, Thompson CJ. Management of endocrine disease: neuroendocrine surveillance and management of neurosurgical patients. Eur J Endocrinol. (2017) 176:R217–r33. doi: 10.1530/eje-16-0962
11. Llompart-Pou JA, Raurich JM, Pérez-Bárcena J, Barceló A, Ibáñez J, Ayestarán JI. Acute hypothalamic-pituitary-adrenal response in traumatic brain injury with and without extracerebral trauma. Neurocrit Care. (2008) 9:230–6. doi: 10.1007/s12028-008-9115-6
12. Taheri S, Karaca Z, Mehmetbeyoglu E, Hamurcu Z, Yilmaz Z, Dal F, et al. The role of apoptosis and autophagy in the hypothalamic-pituitary-adrenal (Hpa) axis after traumatic brain injury (Tbi). Int J Mol Sci. (2022) 23. doi: 10.21203/rs.3.rs-1159219/v1
13. Hollenberg SM, Weinberger C, Ong ES, Cerelli G, Oro A, Lebo R, et al. Primary structure and expression of a functional human glucocorticoid receptor cdna. Nature. (1985) 318:635–41. doi: 10.1038/318635a0
14. Bamberger CM, Bamberger AM, de Castro M, Chrousos GP. Glucocorticoid receptor beta, a potential endogenous inhibitor of glucocorticoid action in humans. J Clin Invest. (1995) 95:2435–41. doi: 10.1172/jci117943
15. Smit P, Russcher H, de Jong FH, Brinkmann AO, Lamberts SW, Koper JW. Differential regulation of synthetic glucocorticoids on gene expression levels of glucocorticoid-induced leucine zipper and interleukin-2. J Clin Endocrinol Metab. (2005) 90:2994–3000. doi: 10.1210/jc.2004-2298
16. Ayrout M, Simon V, Bernard V, Binart N, Cohen-Tannoudji J, Lombès M, et al. A novel non genomic glucocorticoid signaling mediated by a membrane palmitoylated glucocorticoid receptor cross talks with gnrh in gonadotrope cells. Sci Rep. (2017) 7:1537. doi: 10.1038/s41598-017-01777-2
17. Scheschowitsch K, Leite JA, Assreuy J. New insights in glucocorticoid receptor signaling-more than just a ligand-binding receptor. Front Endocrinol (Lausanne). (2017) 8:16. doi: 10.3389/fendo.2017.00016
18. Tasker JG, Di S, Malcher-Lopes R. Minireview: rapid glucocorticoid signaling via membrane-associated receptors. Endocrinology. (2006) 147:5549–56. doi: 10.1210/en.2006-0981
19. Vernocchi S, Battello N, Schmitz S, Revets D, Billing AM, Turner JD, et al. Membrane glucocorticoid receptor activation induces proteomic changes aligning with classical glucocorticoid effects. Mol Cell Proteomics. (2013) 12:1764–79. doi: 10.1074/mcp.M112.022947
20. Vassiliou AG, Stamogiannos G, Jahaj E, Botoula E, Floros G, Vassiliadi DA, et al. Longitudinal evaluation of glucocorticoid receptor alpha/beta expression and signalling, adrenocortical function and cytokines in critically ill steroid-free patients. Mol Cell Endocrinol. (2020) 501:110656. doi: 10.1016/j.mce.2019.110656
21. Vassiliou AG, Athanasiou N, Keskinidou C, Jahaj E, Tsipilis S, Zacharis A, et al. Increased glucocorticoid receptor alpha expression and signaling in critically ill coronavirus disease 2019 patients. Crit Care Med. (2021) 49:2131–6. doi: 10.1097/ccm.0000000000005097
22. Vassiliou AG, Floros G, Jahaj E, Stamogiannos G, Gennimata S, Vassiliadi DA, et al. Decreased glucocorticoid receptor expression during critical illness. Eur J Clin Invest. (2019) 49:e13073. doi: 10.1111/eci.13073
23. Livak KJ, Schmittgen TD. Analysis of relative gene expression data using real-time quantitative pcr and the 2(-delta delta C(T)) method. Methods. (2001) 25:402–8. doi: 10.1006/meth.2001.1262
24. Goldman L, Siddiqui EM, Khan A, Jahan S, Rehman MU, Mehan S, et al. Understanding acquired brain injury: A review. Biomedicines. (2022) 10. doi: 10.2307/j.ctv23hcfdp
25. Sigal GA, Maria DA, Katayama ML, Wajchenberg BL, Brentani MM. Glucocorticoid receptors in mononuclear cells of patients with sepsis. Scandinavian J Infect Dis. (1993) 25:245–8. doi: 10.3109/00365549309008491
26. Molijn GJ, Koper JW, van Uffelen CJ, de Jong FH, Brinkmann AO, Bruining HA, et al. Temperature-induced down-regulation of the glucocorticoid receptor in peripheral blood mononuclear leucocyte in patients with sepsis or septic shock. Clin Endocrinol (Oxf). (1995) 43:197–203. doi: 10.1111/j.1365-2265.1995.tb01915.x
27. Ledderose C, Mohnle P, Limbeck E, Schutz S, Weis F, Rink J, et al. Corticosteroid resistance in sepsis is influenced by microrna-124–induced downregulation of glucocorticoid receptor-alpha. Crit Care Med. (2012) 40:2745–53. doi: 10.1097/CCM.0b013e31825b8ebc
28. Guerrero J, Gatica HA, Rodriguez M, Estay R, Goecke IA. Septic serum induces glucocorticoid resistance and modifies the expression of glucocorticoid isoforms receptors: A prospective cohort study and in vitro experimental assay. Crit Care. (2013) 17:R107. doi: 10.1186/cc12774
29. Vardas K, Ilia S, Sertedaki A, Charmandari E, Briassouli E, Goukos D, et al. Increased glucocorticoid receptor expression in sepsis is related to heat shock proteins, cytokines, and cortisol and is associated with increased mortality. Intensive Care Med Exp. (2017) 5:10. doi: 10.1186/s40635-017-0123-8
30. Espinasse MA, Hajage D, Montravers P, Piednoir P, Dufour G, Tubach F, et al. Neutrophil expression of glucocorticoid-induced leucine zipper (Gilz) anti-inflammatory protein is associated with acute respiratory distress syndrome severity. Ann Intensive Care. (2016) 6:105. doi: 10.1186/s13613-016-0210-0
31. Téblick A, Van Dyck L, Van Aerde N, van der Perre S, Pauwels L, Derese I, et al. Impact of duration of critical illness and level of systemic glucocorticoid availability on tissue-specific glucocorticoid receptor expression and actions: A prospective, observational, cross-sectional human and two translational mouse studies. EBioMedicine. (2022) 80:104057. doi: 10.1016/j.ebiom.2022.104057
32. Kusmenkov T, Braunstein M, Schneider HJ, Bidlingmaier M, Prall WC, Flatz W, et al. Initial free cortisol dynamics following blunt multiple trauma and traumatic brain injury: A clinical study. J Int Med Res. (2019) 47:1185–94. doi: 10.1177/0300060518819603
33. Dendoncker K, Timmermans S, Vandewalle J, Eggermont M, Lempiäinen J, Paakinaho V, et al. Tnf-A Inhibits glucocorticoid receptor-induced gene expression by reshaping the gr nuclear cofactor profile. Proc Natl Acad Sci U.S.A. (2019) 116:12942–51. doi: 10.1073/pnas.1821565116
34. Webster JC, Oakley RH, Jewell CM, Cidlowski JA. Proinflammatory cytokines regulate human glucocorticoid receptor gene expression and lead to the accumulation of the dominant negative beta isoform: A mechanism for the generation of glucocorticoid resistance. Proc Natl Acad Sci U.S.A. (2001) 98:6865–70. doi: 10.1073/pnas.121455098
35. Strickland I, Kisich K, Hauk PJ, Vottero A, Chrousos GP, Klemm DJ, et al. High constitutive glucocorticoid receptor beta in human neutrophils enables them to reduce their spontaneous rate of cell death in response to corticosteroids. J Exp Med. (2001) 193:585–93. doi: 10.1084/jem.193.5.585
36. Miller AH, Pariante CM, Pearce BD. Effects of cytokines on glucocorticoid receptor expression and function. Glucocorticoid resistance and relevance to depression. Adv Exp Med Biol. (1999) 461:107–16. doi: 10.1007/978-0-585-37970-8_7
37. Pace TW, Hu F, Miller AH. Cytokine-effects on glucocorticoid receptor function: relevance to glucocorticoid resistance and the pathophysiology and treatment of major depression. Brain Behav Immun. (2007) 21:9–19. doi: 10.1016/j.bbi.2006.08.009
38. Pace TW, Miller AH. Cytokines and glucocorticoid receptor signaling. Relevance to Major Depression. Ann N Y Acad Sci. (2009) 1179:86–105. doi: 10.1111/j.1749-6632.2009.04984.x
39. Rashmi P, Colussi G, Ng M, Wu X, Kidwai A, Pearce D. Glucocorticoid-induced leucine zipper protein regulates sodium and potassium balance in the distal nephron. Kidney Int. (2017) 91:1159–77. doi: 10.1016/j.kint.2016.10.038
40. Ueda K, Fujiki K, Shirahige K, Gomez-Sanchez CE, Fujita T, Nangaku M, et al. Genome-wide analysis of murine renal distal convoluted tubular cells for the target genes of mineralocorticoid receptor. Biochem Biophys Res Commun. (2014) 445:132–7. doi: 10.1016/j.bbrc.2014.01.125
41. Vassiliou AG, Vassiliadi DA, Keskinidou C, Jahaj E, Botoula E, Tsagarakis S, et al. Down-regulation of the mineralocorticoid receptor (Mr) and up-regulation of hydroxysteroid 11-beta dehydrogenase type 2 (Hsd11b2) isoenzyme in critically ill patients. Clin Med Res. (2023) 21:6–13. doi: 10.3121/cmr.2023.1743
42. Edwards P, Arango M, Balica L, Cottingham R, El-Sayed H, Farrell B, et al. Final results of mrc crash, a randomised placebo-controlled trial of intravenous corticosteroid in adults with head injury-outcomes at 6 months. Lancet. (2005) 365:1957–9. doi: 10.1016/s0140-6736(05)66552-x
43. Dimopoulou I, Tsagarakis S, Theodorakopoulou M, Douka E, Zervou M, Kouyialis AT, et al. Endocrine abnormalities in critical care patients with moderate-to-severe head trauma: incidence, pattern and predisposing factors. Intensive Care Med. (2004) 30:1051–7. doi: 10.1007/s00134-004-2257-x
44. le Roux CW, Chapman GA, Kong WM, Dhillo WS, Jones J, Alaghband-Zadeh J. Free cortisol index is better than serum total cortisol in determining hypothalamic-pituitary-adrenal status in patients undergoing surgery. J Clin Endocrinol Metab. (2003) 88:2045–8. doi: 10.1210/jc.2002-021532
Keywords: acute brain injury, corticosteroid response, critical illness, glucocorticoid receptor alpha, glucocorticoid-inducible leucine zipper
Citation: Lotsios NS, Vrettou CS, Poupouzas G, Chalioti A, Keskinidou C, Pratikaki M, Giannopoulou V, Kotanidou A, Vassiliadi DA, Dimopoulou I and Vassiliou AG (2024) Glucocorticoid receptor response and glucocorticoid-induced leucine zipper expression in neutrophils of critically ill patients with traumatic and non-traumatic brain injury. Front. Endocrinol. 15:1414785. doi: 10.3389/fendo.2024.1414785
Received: 09 April 2024; Accepted: 23 August 2024;
Published: 09 September 2024.
Edited by:
V Sujith Sajja, Walter Reed Army Institute of Research, United StatesReviewed by:
Jinn-Rung Kuo, Chi Mei Medical Center, TaiwanRichard S. Lee, Johns Hopkins University, United States
Joanna Spencer-Segal, University of Michigan, United States
Copyright © 2024 Lotsios, Vrettou, Poupouzas, Chalioti, Keskinidou, Pratikaki, Giannopoulou, Kotanidou, Vassiliadi, Dimopoulou and Vassiliou. This is an open-access article distributed under the terms of the Creative Commons Attribution License (CC BY). The use, distribution or reproduction in other forums is permitted, provided the original author(s) and the copyright owner(s) are credited and that the original publication in this journal is cited, in accordance with accepted academic practice. No use, distribution or reproduction is permitted which does not comply with these terms.
*Correspondence: A.G. Vassiliou, YWx2YXNzNzVAZ21haWwuY29t
†These authors have contributed equally to this work and share first authorship