- 1Department of Education, Taichung Veterans General Hospital, Taichung, Taiwan
- 2School of Medicine, Chung Shan Medical University, Taichung, Taiwan
- 3Division of Endocrinology and Metabolism, Department of Internal Medicine, Taichung Veterans General Hospital, Taichung, Taiwan
- 4School of Medicine, National Yang Ming Chiao Tung University, Taipei, Taiwan
- 5Department of Computer Science & Information Engineering, National Taiwan University, Taipei, Taiwan
- 6Department of Food and Nutrition, Providence University, Taichung, Taiwan
- 7Institute of Biomedical Sciences, National Chung Hsing University, Taichung, Taiwan
- 8Graduate Institute of Food Safety, National Chung Hsing University, Taichung, Taiwan
- 9Department of Chest Medicine, Taichung Veterans General Hospital, Taichung, Taiwan
- 10Mycobacterial Center, Taichung Veterans General Hospital, Taichung, Taiwan
- 11Department of Post-Baccalaureate Medicine, College of Medicine, National Chung Hsing University, Taichung, Taiwan
Background: Patients with type 2 diabetes mellitus (DM) have a high prevalence of chronic kidney disease (CKD). Energy imbalance and inflammation may be involved in the pathogenesis of CKD. We examined the effects of brain-derived neurotrophic factor (BDNF) and vascular cell adhesion molecule-1 (VCAM-1) on CKD in patients with type 2 DM.
Methods: Patients with type 2 DM were enrolled for this cross-sectional study. Fasting serum was prepared to measure the BDNF and VCAM-1 levels. An estimated glomerular filtration rate (eGFR) <60 mL/min/1.73 m2 was used as the criterion for identifying patients with CKD.
Results: Of the 548 enrolled participants, 156 had CKD. Patients with CKD exhibited significantly lower BDNF (median of 21.4 ng/mL, interquartile range [IQR]: 17.0–27.0 ng/mL vs. median of 25.9 ng/mL, IQR: 21.0–30.4 ng/mL, P <0.001) and higher VCAM-1 (median of 917 ng/mL, IQR: 761–1172 ng/mL vs. median of 669 ng/mL, IQR: 552–857 ng/mL, P <0.001) levels than those without CKD. Serum BDNF levels were inversely correlated with VCAM-1 levels (Spearman’s rank correlation coefficient = -0.210, P <0.001). The patients were divided into four subgroups based on median BDNF and VCAM-1 levels (24.88 ng/mL and 750 ng/mL, respectively). Notably, patients in the high VCAM-1 and low BDNF group had the highest prevalence (50%) of CKD. Multivariate logistic regression revealed a significantly higher odds ratio (OR) of CKD in the high VCAM-1 and low BDNF group (OR = 3.885, 95% CI: 1.766–8.547, P <0.001), followed by that in the high VCAM-1 and high BDNF group (OR = 3.099, 95% CI: 1.373–6.992, P =0.006) compared with that in the low VCAM-1 and high BDNF group. However, the risk of CKD in the low VCAM-1 and low BDNF group was not significantly different from that in the low VCAM-1 and high BDNF group (P =0.266).
Conclusion: CKD in patients with type 2 DM is associated with low serum BDNF and high VCAM-1 levels. BDNF and VCAM-1 have a synergistic effect on CKD. Thus, BDNF and VCAM-1 can be potential biomarkers for CKD risk stratification in patients with type 2 DM.
1 Introduction
Chronic kidney disease (CKD) poses an increasing public health concern. The global prevalence of patients with an estimated glomerular filtration rate (eGFR) <60 mL/min/1.73 m2 was approximately 4% in 2017 (1). The high mortality rate and costs associated with CKD, coupled with increasing dependency on caregivers, become considerable challenge (2–4). According to the United States Renal Data System annual data report for 2021, 59.9% of the patients with incident end-stage kidney disease had diabetes mellitus (DM) (5). According to the data from the National Health and Nutrition Examination Survey 2007–2014 (6), approximately 18% of patients with type 2 DM had an eGFR <60 mL/min/1.73 m2. Therefore, there is a notable correlation between CKD and type 2 DM.
Brain-derived neurotrophic factor (BDNF) plays a crucial role in energy homeostasis in humans. Circulating BDNF levels are significantly lower in patients with type 2 DM than in healthy controls (7, 8). Moreover, low serum BDNF levels have been linked to various chronic complications of DM, including diabetic retinopathy and neuropathy (9, 10). Interestingly, a notable correlation has been found between eGFR and serum BDNF levels in individuals without DM (11). However, the association between BDNF and CKD in patients with type 2 DM remains unexplored.
Vascular cell adhesion molecule-1 (VCAM-1) is a glycoprotein expressed in endothelial cells (12) and promotes the attachment of white blood cells to the inner lining of blood vessels (13). It has been reported that serum VCAM-1 levels are notably increased in patients with type 2 DM (14). Moreover, this increase was associated with the development of diabetic microvascular complications (14–16).
In patients without known DM, low serum BDNF levels have been reported to be associated with high VCAM-1 levels (17). However, patients with type 2 DM have significantly lower BDNF and higher VCAM-1 levels than healthy controls (8, 14). We hypothesized that circulating BDNF levels are inversely associated with VCAM-1 in patients with type 2 DM, and that this association plays a role in CKD. Therefore, we conducted a cross-sectional study on patients with type 2 DM and examined the risk of CKD categorized according to serum BDNF and VCAM-1 levels.
2 Materials and methods
2.1 Patients
This cross-sectional study was conducted at Taichung Veterans General Hospital. Patients were enrolled from the outpatient department between March 2018 and October 2018. The inclusion criteria were as follows: age ≥45 years, clinical diagnosis of DM by physicians, and poor glucose control (defined as at least one incidence of glycated hemoglobin [HbA1c] levels >9.0% within previous one year based on the medical record). Patients with the following status at enrollment were excluded: diabetic classification other than type 2 DM, C-reactive protein (CRP) levels >60 mg/L, chronic active infection such as tuberculosis, history of immunocompromise or use of immunosuppressors, and incomplete study assessments. After reviewing the medical history and anthropometric measurements, blood and spot urine samples were collected following overnight fasting. This study was approved by the Institutional Review Board of Taichung Veterans General Hospital, and written informed consent was obtained from all participants.
2.2 Biochemical assessment
Plasma glucose levels were determined using the glucose oxidase-peroxidase method (Wako Diagnostics, Tokyo, Japan). HbA1c levels were determined using boronate-affinity high-performance liquid chromatography (NGSP-certified, Primus Corp., Kansas City, MO, USA). Serum creatinine, CRP, and lipid levels were determined using commercial kits (Beckman Coulter, Fullerton, CA, USA). Serum free BDNF levels were determined using an immunoassay kit (R&D Systems, Minneapolis, MN, USA), with an intra-assay coefficient of variation (CV) of 6.2% and an inter-assay CV of 8.1%. Serum VCAM-1 levels were determined using an immunoassay kit (R&D Systems, Minneapolis, MN, USA), with an intra-assay CV of 2.3% and an inter-assay CV of 7.8%. Urinary albumin levels were evaluated using the polyethylene glycol-enhanced immunoturbidimetric method (Advia 1800 system, Siemens, NY, USA).
The eGFR was calculated using the Chronic Kidney Disease Epidemiology Collaboration equation (18). CKD was defined as a condition with an eGFR <60 mL/min/1.73 m2. The urine albumin-to-creatinine ratio (UACR) was calculated by dividing the levels of urine albumin (mg) with those of urine creatinine (g). Increased albuminuria was defined as a UACR ≥30 mg/g (19). Obesity was defined as a body mass index (BMI) ≥27 kg/m2 (20). Hypertension was characterized by systolic blood pressure ≥130 mmHg, diastolic blood pressure ≥80 mmHg, or a documented history of antihypertensive medication use. Low high-density lipoprotein (HDL) cholesterol was defined as an HDL level <1.0 mmol/L (40 mg/dL) in men and <1.3 mmol/L (50 mg/dL) in women. Hypercholesterolemia was defined as a total cholesterol level ≥4.14 mmol/L (160 mg/dL) or a low-density lipoprotein (LDL) cholesterol level ≥2.59 mmol/L (100 mg/dL). Hypertriglyceridemia was defined as a triglyceride level ≥1.7 mmol/L (150 mg/dL) (21).
2.3 Statistical analyses
Continuous variables are presented as means along with the corresponding standard deviations, whereas data of UACR, BDNF, and VCAM-1 in patients with or without CKD are presented as medians along with interquartile range (IQR). Categorical variables are presented as numbers along with their respective percentages. Because the assessed continuous variables did not exhibit normal distribution based on the Kolmogorov-Smirnov test, we employed the Mann-Whitney U test to assess the differences in continuous variables between individuals with and without CKD. Simultaneously, a Mann-Whitney U test was applied to examine the differences in serum BDNF and VCAM-1 levels between the paired groups categorized according to the risk factors associated with CKD. Spearman’s rank correlation coefficient (rho) was used to determine the correlation between continuous variables. A trend test was used to examine the changes in the ratio of serum BDNF to VCAM-1 across the groups categorized by eGFR. We compared the CKD risks in different models by examining the C-index. The performance of the index in BDNF + VCAM-1 model was compared with that of the BDNF or VCAM-1 alone model. Furthermore, the patients were divided into four subgroups based on median BDNF and VCAM-1 levels. A trend test was performed to examine the linearly increasing trend in CKD prevalence among the four subgroups. In addition, univariate logistic regression analyses were used to explore the odds ratios (OR) and 95% confidence interval (CI) of CKD for the four subgroups and the quartiles of BDNF or VCAM-1. In the multivariate regression analysis, the assessed variables which were significantly associated with both CKD and the BDNF or VCAM-1 levels were included. Statistical analyses were performed using the SPSS version 22.0 software (IBM Corp., Armonk, NY, USA), and C index was assessed using R software v3.4.
3 Results
Among the 548 participants enrolled in this study, 156 had CKD (CKD group), and 392 did not have CKD (non-CKD group). Table 1 presents the baseline characteristics of the patients in these two groups. Patients with CKD had significantly lower serum BDNF (median of 21.4 ng/mL, IQR: 17.0–27.0 ng/mL vs. median of 25.9 ng/mL, IQR: 21.0–30.4 ng/mL, P < 0.001) and higher serum VCAM-1 (median of 917 ng/mL, IQR: 761–1172 ng/mL vs. median of 669 ng/mL, IQR: 552–857 ng/mL, P < 0.001) levels than those without CKD. Patients with CKD were significantly older (69.7 ± 9.0 vs. 60.4 ± 8.5 years, P < 0.001) and mostly males (66.0% vs. 53.3%, P = 0.009). Patients with CKD had significantly lower diastolic blood pressure (71 ± 13 vs. 75 ± 11 mmHg, P < 0.001), lower total cholesterol (3.9 ± 0.9 vs. 4.2 ± 1.0 mmol/L, P < 0.001), lower LDL cholesterol (2.1 ± 0.7 vs. 2.4 ± 0.8 mmol/L, P < 0.001), and lower HDL cholesterol (1.2 ± 0.3 vs. 1.3 ± 0.4 mmol/L, P < 0.001) levels, but higher triglyceride levels (1.9 ± 1.5 vs. 1.6 ± 1.2 mmol/L, P < 0.001). In addition to a significantly lower eGFR (48.0 ± 11.2 vs. 78.7 ± 11.6 mL/min/1.73 m2, P < 0.001), the CKD group had a significantly higher UACR (median of 132 mg/g, IQR: 24–448 mg/g) in comparison with that (median of 19 mg/g, IQR: 8–74 mg/g; P < 0.001) in the non-CKD group. Patients with CKD had significantly lower levels of alanine aminotransferase (24 ± 16 vs. 28 ± 23 U/L, P = 0.006) than those without CKD. Regarding the current use of medications, the CKD group had significantly higher proportions of patients using antiplatelet drugs (20.5% vs. 11.7%, P = 0.012), antihypertensive drugs (53.8% vs. 34.2%, P < 0.001), and insulin injection (55.8% vs. 45.4%, P = 0.036). The proportion of patients using oral antihyperglycemic drugs was not significantly different (P = 0.626) between groups; however, the CKD group had significantly lower proportions of patients using metformin (P < 0.001) and sodium-glucose cotransporter 2 inhibitors (P = 0.002) but a significantly higher proportion of patients using dipeptidyl peptidase-4 inhibitors (P = 0.036) than the non-CKD group. BMI, systolic blood pressure, glucose, HbA1c, and CRP levels, proportion of current smokers, and proportions of patients with previous coronary artery disease (CAD) history, hypertension, use of statins, and use of glucagon-like peptide-1 receptor agonists were not significantly different (P > 0.05) between the CKD and non-CKD groups.
To highlight the potential factors that might be associated with BDNF or VCAM-1, we have listed the serum BDNF or VCAM-1 levels in patients categorized by the assessed factors in Table 2. Young age, female sex, hyperglycemia (including fasting glucose and HbA1c), high total cholesterol, hypertriglyceridemia, normal UACR, use of statins and metformin, and no use of α-glucosidase inhibitor were significantly associated with high serum BDNF levels. Moreover, age, CAD history, hypertension, low cholesterol (including total, LDL, and HDL cholesterol) levels, increased UACR, use of insulin therapy and antihypertensive drugs, and no use of statins or metformin were significantly associated with high serum VCAM-1 levels. We divided the patients into two groups based on median serum BDNF level (<24.88 ng/mL and ≥24.88 ng/mL), and the group with lower BDNF levels exhibited higher serum VCAM-1 levels (906 ± 463 vs. 750 ± 272 ng/mL, P <0.001) compared with the group with higher BDNF levels. Similarly, we divided the patients into two groups based on median serum VCAM-1 levels (<750 ng/mL and ≥750 ng/mL), and the group with lower VCAM-1 levels displayed higher serum BDNF levels (26.2 ± 6.5 vs. 23.8 ± 9.1 ng/mL, P < 0.001) than that in the group with higher VCAM-1 levels. Serum VCAM-1 levels showed a significant inverse correlation with serum BDNF levels (rho = -0.210, P < 0.001; Figure 1).
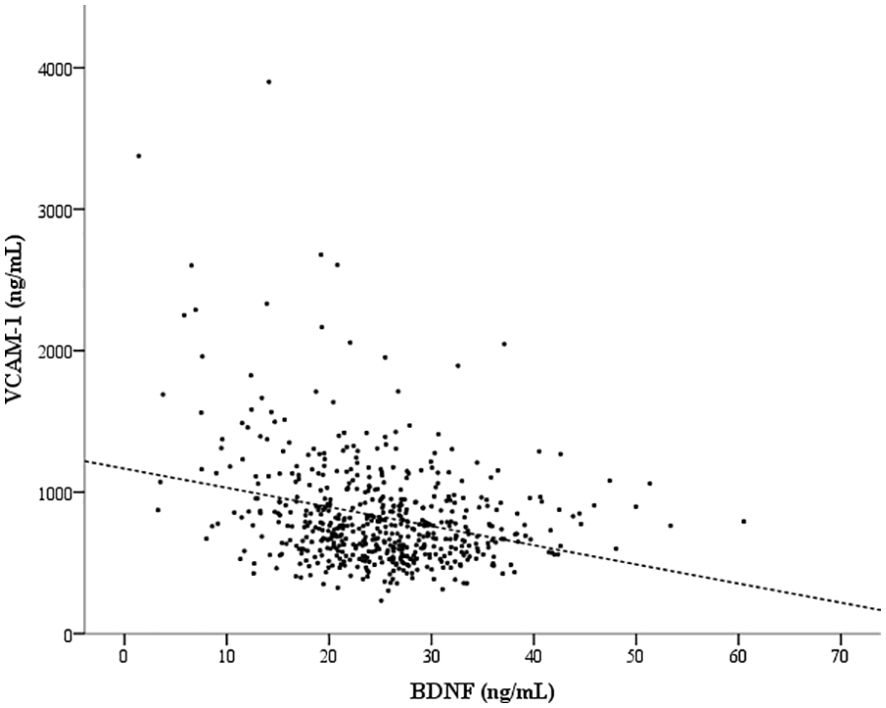
Figure 1. An inverse correlation between the serum brain-derived neurotrophic factor (BDNF) and vascular cell adhesion molecule-1 (VCAM-1) levels. Spearman’s rank correlation coefficient (rho) was -0.210 (P < 0.001) for the correlation between the serum BDNF and VCAM-1 levels.
To further assess the relationship of CKD-associated characteristics with BDNF or VCAM-1, Table 3 shows the rho values for the correlation of the continuous variables that were significantly different between the CKD and non-CKD groups (as shown in Table 1) with serum BDNF or VCAM-1 levels. In the CKD group, BDNF showed a significant positive correlation with eGFR (rho = 0.180, P = 0.024) and an inverse correlation with age (rho = -0.183, P = 0.022). In the non-CKD group, BDNF showed a significant positive correlation with triglycerides (rho = 0.175, P < 0.001), total cholesterol (rho = 0.164, P = 0.001), and LDL cholesterol (rho = 0.104, P = 0.040) and an inverse correlation with age (rho = -0.186, P < 0.001) and VCAM-1 (rho = -0.123, P = 0.014). In the CKD group, VCAM-1 had significantly positive correlation with UACR (rho = 0.248, P = 0.002) and age (rho = 0.233, P = 0.003) and an inverse correlation with eGFR (rho = -0.389, P < 0.001) and diastolic blood pressure (rho = -0.184, P = 0.021). In the non-CKD group, VCAM-1 showed a significant positive correlation with age (rho = 0.205, P < 0.001), UACR (rho = 0.141, P = 0.005), and alanine aminotransferase (rho = 0.116, P = 0.021) and an inverse correlation with eGFR (rho = -0.192, P < 0.001), total cholesterol (rho = -0.139, P = 0.006), and LDL cholesterol (rho = -0.137, P = 0.007).
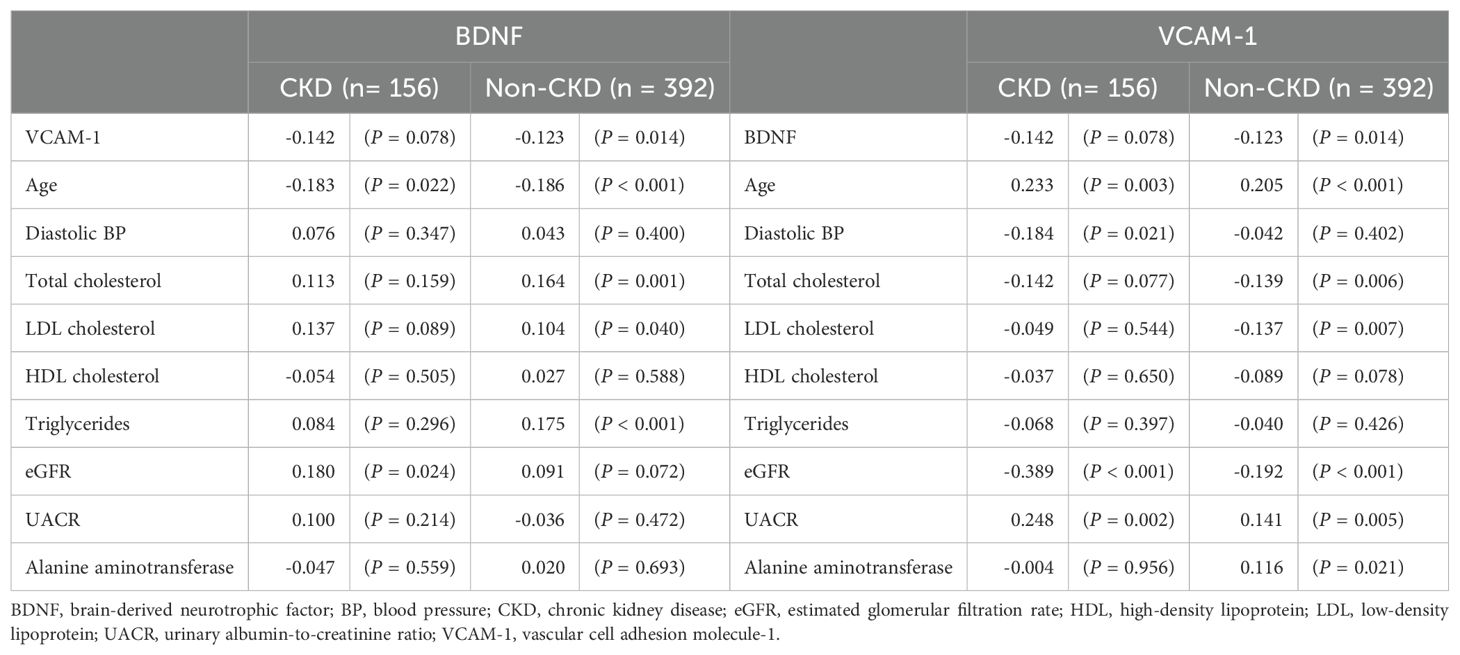
Table 3. Spearman’s rank correlation coefficient (rho) between the CKD-associated characteristics and serum BDNF or VCAM-1 levels in the CKD and non-CKD groups.
Figure 2 shows the ratios of serum BDNF (ng) to VCAM-1 (μg) in patients according to their eGFR values: 43.1 ± 17.3 ng/μg in 65 patients with eGFR ≥ 90 mL/min/1.73 m2, 39.3 ± 17.5 ng/μg in 327 patients with eGFR between 60 and 89 mL/min/1.73m2, 28.1 ± 13.9 ng/μg in 111 patients with eGFR between 45 and 59 mL/min/1.73 m2, 20.2 ± 7.9 ng/μg in 36 patients with eGFR between 30 and 44 mL/min/1.73 m2, and 14.2 ± 7.0 ng/μg in 9 patients with eGFR < 30 mL/min/1.73 m2. The ratio of serum BDNF to VCAM-1 levels decreased as the CKD stages progressed (P value for the trend < 0.001). Figure 3 shows the receiver operating characteristic curves to differentiate CKD using the BDNF alone model, the VCAM-1 alone model, and the BDNF + VCAM-1 model. The C index was 0.645 (95% CI: 0.531–0.762), 0.746 (95% CI: 0.643–0.844), and 0.756 (95% CI: 0.657–0.853); respectively. The C index is significantly higher in the BDNF + VCAM-1 model than in the BDNF alone model (P < 0.001), but not significantly different from that in the VCAM-1 model (P = 0.263).
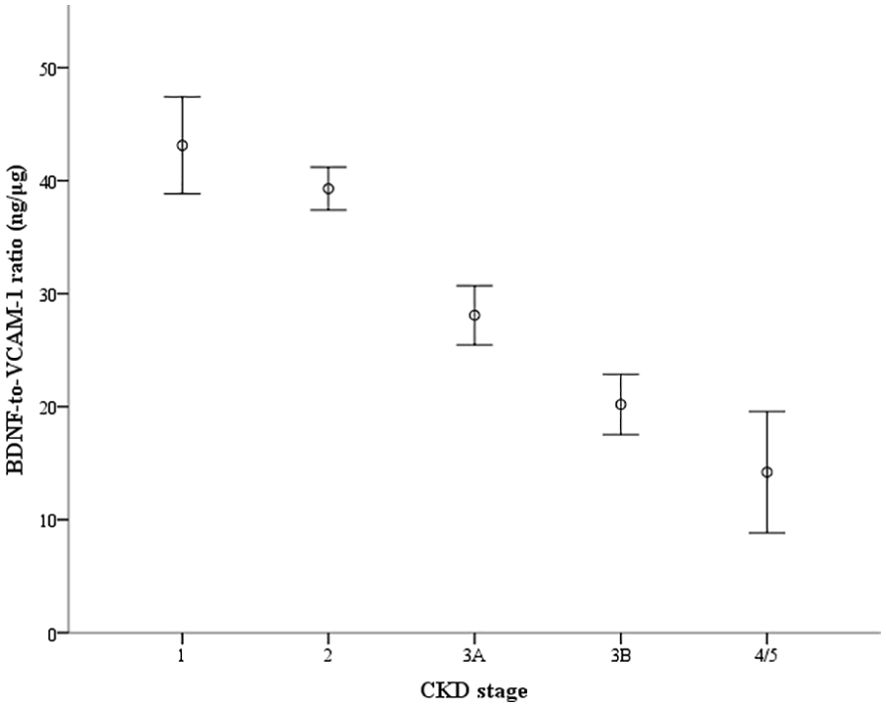
Figure 2. The ratio of serum brain-derived neurotrophic factor (BDNF) to vascular cell adhesion molecule-1 (VCAM-1) levels according to the groups categorized by estimated glomerular filtration rate (eGFR). The ratio (ng/μg) is depicted with the error bar showing the 95% confidence interval. The groups are staged according to eGFR as follows: stage 1 (n=65): eGFR ≥ 90 mL/min/1.73 m2, stage 2 (n = 327): eGFR between 60 and 89 mL/min/1.73 m2, stage 3A (n = 111): eGFR between 45 and 59 mL/min/1.73 m2, stage 3B (n = 36): eGFR between 30 and 44 mL/min/1.73 m2, and stage 4/5 (n=9): eGFR < 30 mL/min/1.73 m2). P value for the trend <0.001.
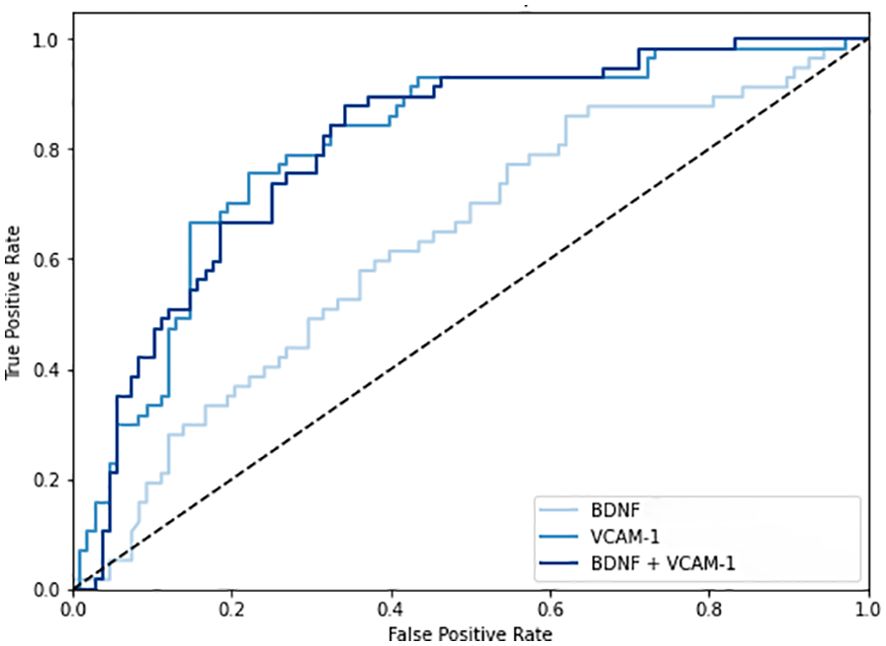
Figure 3. The receiver operating characteristic curves to differentiate chronic kidney disease (CKD). The curves are shown in three different models: the brain-derived neurotrophic factor (BDNF) alone model, the vascular cell adhesion molecule-1 (VCAM-1) alone model, and the BDNF + VCAM-1 model.
We further divided the patients into four subgroups based on median BDNF and VCAM-1 levels. The highest prevalence of CKD (50%; 77 of 154 patients) was observed in the high VCAM-1 and low BDNF group; the prevalence of CKD was 35% (42 of 120 patients) in the high VCAM-1 and high BDNF group and 20% (24 of the 120 patients) in the low VCAM-1 and low BDNF group; the lowest prevalence of CKD (8.4%; 13 of 154 patients) was observed in the low VCAM-1 and high BDNF group (the P value for the trend was <0.001, Figure 4). In the univariate regression analyses, the highest OR of 10.846 (95% CI: 5.662–20.777, P < 0.001) for CKD was observed in the high VCAM-1 and low BDNF group, and it was followed by 5.840 (95% CI: 2.956–11.537, P < 0.001) in the high VCAM-1 and high BDNF group, and 2.712 (95% CI: 1.316–5.588, P = 0.007) in the low VCAM-1 and low BDNF group, compared with that in the low VCAM-1 and high BDNF group (Table 4). The risks of CKD were significantly associated with a decrease in serum BDNF levels and an increase in serum VCAM-1 levels compared to the first quartile groups.
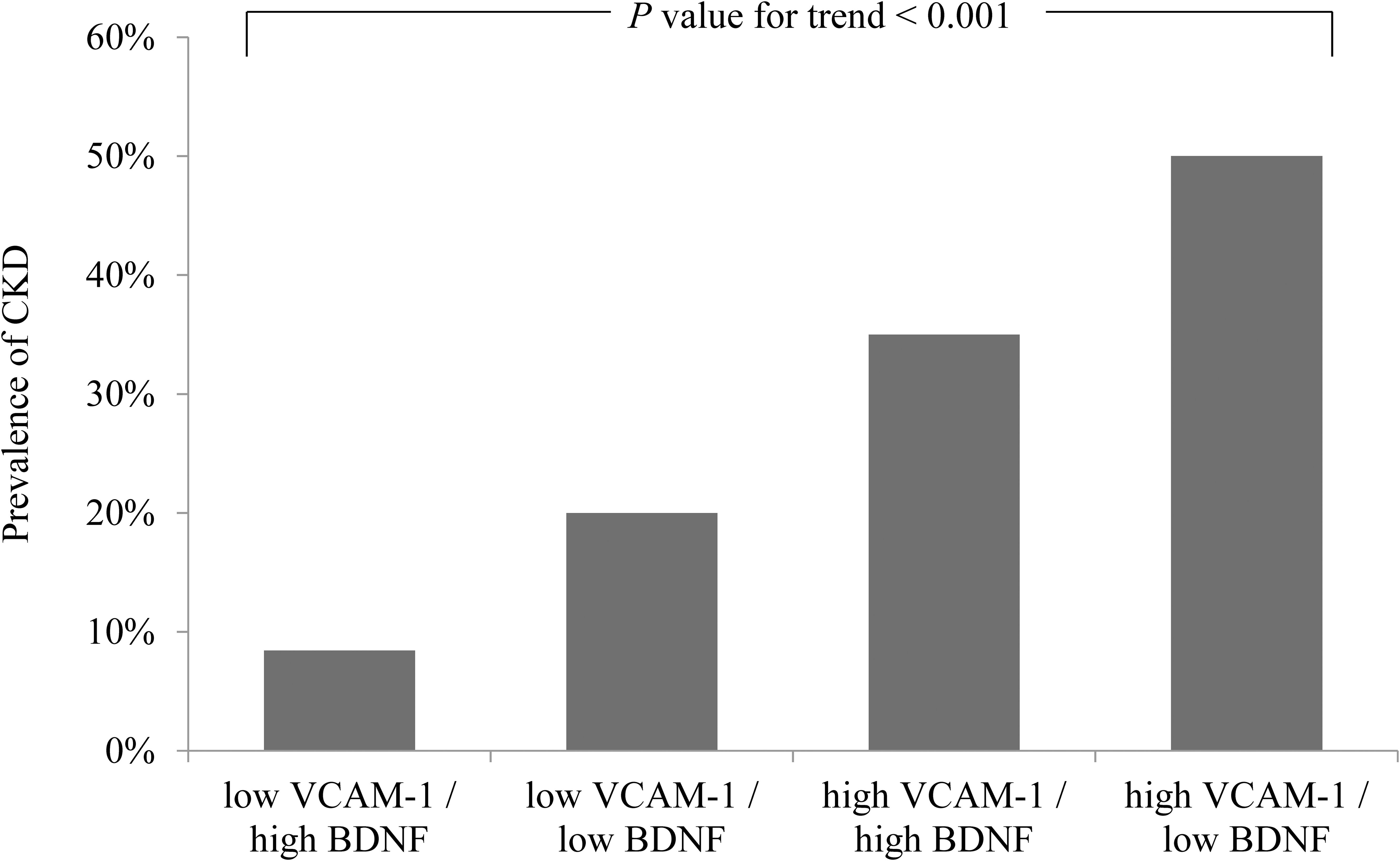
Figure 4. Prevalence of chronic kidney disease (CKD) according to serum brain-derived neurotrophic factor (BDNF) and vascular cell adhesion molecule-1 (VCAM-1) levels. The prevalence of CKD is shown in the four subgroups categorized by the median values of BDNF (24.88 ng/mL) and VCAM-1 (750 ng/mL).
To determine the independent association between CKD and these four subgroups, we selected confounding factors that were significantly associated with both CKD in Table 1 and the BDNF or VCAM-1 levels in Table 2. Therefore, we included the variables, namely age, sex, total cholesterol, LDL cholesterol, HDL cholesterol, triglycerides, UACR, use of antihypertensive drugs, use of angiotensin-converting enzyme inhibitors or angiotensin II receptor blockers, use of calcium channel blockers, use of diuretics, use of insulin therapy, and use of metformin in the multivariate logistic regression analysis (Table 5). The highest OR of 3.885 (95% CI: 1.766–8.547, P < 0.001) for CKD was observed in the high VCAM-1 and low BDNF group, and it was followed by 3.099 (95% CI: 1.373–6.992, P = 0.006) in the high VCAM-1 and high BDNF group compared with that in the low VCAM-1 and high BDNF group. Although the OR for CKD was 1.634 (95% CI: 0.688–3.884) in the low VCAM-1 and low BDNF group, the difference was not statistically significant compared with the low VCAM-1 and high BDNF group (P = 0.266).
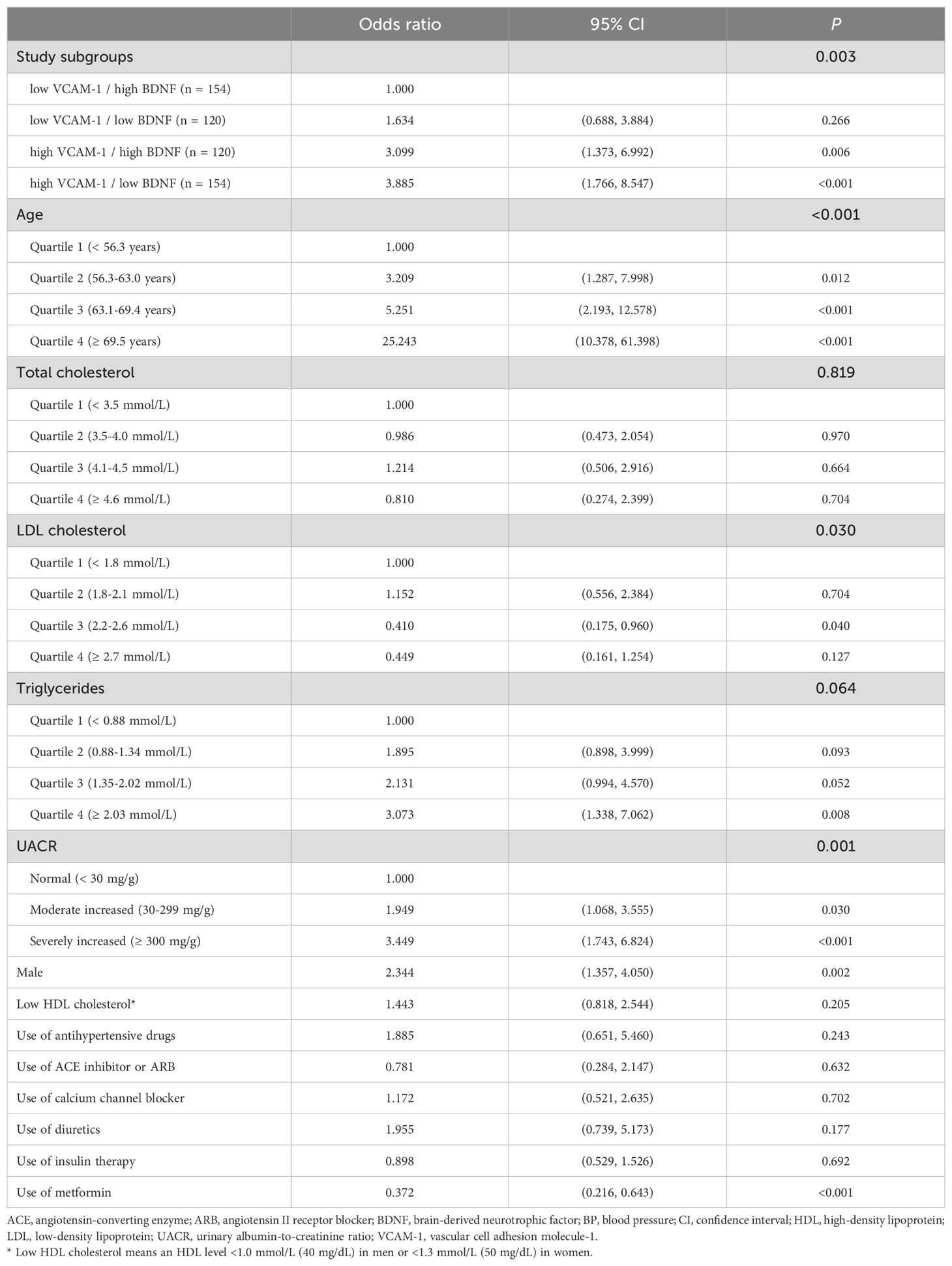
Table 5. Odds ratios (95% CI) for chronic kidney disease based on the associated risk factors in the multivariate regression analysis.
4 Discussion
In the present study, we found that CKD in patients with type 2 DM was associated with low BDNF and high VCAM-1 levels in serum. Moreover, serum VCAM-1 levels were inversely correlated with BDNF levels, and high VCAM-1 levels had a synergistic effect with low BDNF levels on CKD in patients with type 2 DM. Hyperglycemia may downregulate BDNF expression in proximal tubular cells and enhance fibrosis in renal tissue (22, 23). Similar to the dendritic spines of neuronal cells, podocyte processes express tropomyosin-related kinase B receptor (TrkB), a specific BDNF receptor, and exhibit TrkB-dependent trophic activity by enhancing the phosphorylation of cofilin, a family of actin-binding proteins essential for maintaining normal podocyte architecture and facilitating structural changes in actin during the initiation and recovery phases of podocyte injury (24, 25). Podocyte injury following chemical damage can be repaired by administrating BDNF in the in vitro and animal models (26).
Circulating VCAM-1 levels are inversely correlated with eGFR and positively correlated with UACR in patients with type 2 DM (27). VCAM-1 functions as an adhesion molecule for leukocytes, facilitating the interaction of tubular proteins with mononuclear cells to promote interstitial fibrosis and subsequent CKD under diabetic conditions (28). Li et al. (29) also reported that high circulating VCAM-1 levels were associated with poor recovery of renal function after acute renal failure in patients with sepsis.
In addition to BDNF protecting against CKD and VCAM-1 promoting CKD development, Su et al. (30) reported that cultured human umbilical vein endothelial cells (HUVECs) exposed to uremic toxins in serum of patients with CKD inhibited the BDNF expression, and subsequently induced VCAM-1 expression and endothelial dysfunction in the in vitro study. This mechanism also explains the increased risk of CAD in patients with low serum BDNF and high serum VCAM-1 levels (17). In the present study, although the correlation between BDNF and VCAM-1 was only significant in the non-CKD group, the correlation coefficient in the CKD group, which had a relatively small sample size, was similar to that in the non-CKD group. The strength of the present study is that we showed a synergistic effect of high VCAM-1 and low BDNF levels on CKD in patients with type 2 DM.
Decreased circulating BDNF levels are associated with an increased risk of CAD, which is also associated with CKD (31). Furthermore, low BDNF levels can also be predictive of cardiovascular events and mortality (32–34). Similarly, excessive VCAM-1 expression in the arterial tissue has been observed in patients with advanced CKD, and serum VCAM-1 levels are predictive of cardiovascular events (35). Changes in BDNF levels are linked to the endothelial dysfunction, a crucial early step in atherosclerotic development (36). Atherosclerotic cardiovascular disease can induce inflammation and kidney injury (37, 38). CAD has been thought as an independent predictor of end-stage renal disease in patients with type 2 DM (39). Therefore, BDNF probably plays a protective role in inflammatory-vascular interactions involved not only in cognitive dysfunction but also in chronic cardiovascular and kidney disorders (17, 40, 41).
In the present study, old age and male sex were independent risk factors for CKD. In line with our findings, an increased prevalence of CKD was observed in old age and male population based on the National Health Insurance Research Database (NHIRD) (42, 43). In addition to hyperglycemia, hypertriglyceridemia was also reported as an important risk factor for CKD in patients with type 2 DM (44, 45). We found different patterns of medication use between patients with and without CKD in the present study. According to data from the Joint Asia Diabetes Evaluation Program, a higher proportion of patients used antihypertensive drugs and a lower proportion of patients used metformin were observed in the CKD group than that in the non-CKD group (46). Nonetheless, a synergistic effect of low BDNF and high VCAM-1 levels was observed after adjusting for these potential confounders.
Our study had some limitations. First, the cross-sectional design of the present study restricts the ability to establish causal correlations. Second, we recruited participants with poor glucose control, defined as having at least one HbA1c measurement exceeding 9.0% within the previous year. However, more than half (51.5%) of the enrolled patients had HbA1c levels <8.5% when blood samples were collected. Third, we did not directly explore the molecular pathways connecting BDNF and VCAM-1 to the CKD process. Finally, all participants were enrolled from the same teaching hospital. Therefore, further large-scale studies with longitudinal follow-up are needed to investigate the effects of BDNF and VCAM-1 on CKD in patients with type 2 DM.
5 Conclusion
Low serum BDNF and high VCAM-1 levels were significantly associated with CKD in patients with type 2 DM. Furthermore, serum VCAM-1 levels inversely correlated with BDNF levels and had a synergistic effect with low BDNF levels on CKD. The molecular pathway connecting BDNF and VCAM-1 may be involved in the CKD in patients with type 2 DM.
Data availability statement
The raw data supporting the conclusions of this article will be made available by the authors, without undue reservation.
Ethics statement
The studies involving humans were approved by Institutional Review Board of Taichung Veterans General Hospital. The studies were conducted in accordance with the local legislation and institutional requirements. The participants provided their written informed consent to participate in this study.
Author contributions
YC: Conceptualization, Writing – original draft. YL: Conceptualization, Methodology, Writing – review & editing. YuC: Conceptualization, Funding acquisition, Methodology, Writing – review & editing. YiC: Conceptualization, Data curation, Methodology, Writing – review & editing. JW: Conceptualization, Writing – original draft. JL: Conceptualization, Funding acquisition, Writing – review & editing. WH: Conceptualization, Investigation, Methodology, Writing – review & editing. IL: Conceptualization, Data curation, Formal analysis, Funding acquisition, Investigation, Methodology, Project administration, Resources, Supervision, Validation, Writing – review & editing.
Funding
The author(s) declare financial support was received for the research, authorship, and/or publication of this article. This research was funded by Taichung Veterans General Hospital, Taiwan (grant numbers TCVGH-1123501C, TCVGH-1133501C, TCVGH-NCHU1110119, TCVGH-PU1088102, and TCVGH-PU1098105), National Health Research Institute, Taiwan (grant number NHRI-EX110-10927HT), and the Ministry of Science and Technology, Taiwan (grant numbers MOST 109-2314-B-075A-004 and MOST 110-2314-B-075A-004 -MY3).
Acknowledgments
The authors thank the Health Bureau of Taichung City Government for supporting this study and the Biostatistics Task Force of Taichung Veterans General Hospital for the statistical analyses.
Conflict of interest
The authors declare that the research was conducted in the absence of any commercial or financial relationships that could be construed as a potential conflict of interest.
Publisher’s note
All claims expressed in this article are solely those of the authors and do not necessarily represent those of their affiliated organizations, or those of the publisher, the editors and the reviewers. Any product that may be evaluated in this article, or claim that may be made by its manufacturer, is not guaranteed or endorsed by the publisher.
References
1. Global Burden of Disease Chronic Kidney Disease Collaboration. Global, regional, and national burden of chronic kidney disease, 1990-2017: A systematic analysis for the global burden of disease study 2017. Lancet. (2020) 395:709–33. doi: 10.1016/s0140-6736(20)30045-3
2. Wang V, Vilme H, Maciejewski ML, Boulware LE. The economic burden of chronic kidney disease and end-stage renal disease. Semin Nephrol. (2016) 36:319–30. doi: 10.1016/j.semnephrol.2016.05.008
3. Ríos Burrows N, Koyama A, Pavkov ME. Reported cases of end-stage kidney disease-United States, 2000-2019. Am J Transplant. (2022) 22:1483–6. doi: 10.1111/ajt.16657
4. Deng Y, Li N, Wu Y, Wang M, Yang S, Zheng Y, et al. Global, regional, and national burden of diabetes-related chronic kidney disease from 1990 to 2019. Front Endocrinol (Lausanne). (2021) 12:672350. doi: 10.3389/fendo.2021.672350
5. United States Renal Data System. 2023 USRDS Annual Data Report: Epidemiology of kidney disease in the United States (2023). Bethesda, MD: National Institutes of Health, National Institute of Diabetes and Digestive and Kidney Diseases. Available online at: https://usrds-adr.niddk.nih.gov/2023/chronic-kidney-disease (Accessed March 15, 2024).
6. Wang T, Xi Y, Lubwama R, Hannanchi H, Iglay K, Koro C. Chronic kidney disease among us adults with type 2 diabetes and cardiovascular diseases: A national estimate of prevalence by kdigo 2012 classification. Diabetes Metab Syndr. (2019) 13:612–5. doi: 10.1016/j.dsx.2018.11.026
7. Rozanska O, Uruska A, Zozulinska-Ziolkiewicz D. Brain-derived neurotrophic factor and diabetes. Int J Mol Sci. (2020) 21:841. doi: 10.3390/ijms21030841
8. Davarpanah M, Shokri-Mashhadi N, Ziaei R, Saneei P. A systematic review and meta-analysis of association between brain-derived neurotrophic factor and type 2 diabetes and glycemic profile. Sci Rep. (2021) 11:13773. doi: 10.1038/s41598-021-93271-z
9. Ola MS, Nawaz MI, El-Asrar AA, Abouammoh M, Alhomida AS. Reduced levels of brain derived neurotrophic factor (Bdnf) in the serum of diabetic retinopathy patients and in the retina of diabetic rats. Cell Mol Neurobiol. (2013) 33:359–67. doi: 10.1007/s10571-012-9901-8
10. Azoulay D, Abed S, Sfadi A, Sheleg O, Shaoul E, Shehadeh M, et al. Low brain-derived neurotrophic factor protein levels and single-nucleotide polymorphism val66met are associated with peripheral neuropathy in type II diabetic patients. Acta Diabetol. (2020) 57:891–8. doi: 10.1007/s00592-020-01508-6
11. Hsu CY, Sheu WH, Lee IT. Brain-derived neurotrophic factor associated with kidney function. Diabetol Metab Syndr. (2023) 15:16. doi: 10.1186/s13098-023-00991-5
12. Kong DH, Kim YK, Kim MR, Jang JH, Lee S. Emerging roles of vascular cell adhesion molecule-1 (Vcam-1) in immunological disorders and cancer. Int J Mol Sci. (2018) 19:1057. doi: 10.3390/ijms19041057
13. Pankow JS, Decker PA, Berardi C, Hanson NQ, Sale M, Tang W, et al. Circulating cellular adhesion molecules and risk of diabetes: the multi-ethnic study of atherosclerosis (Mesa). Diabetes Med. (2016) 33:985–91. doi: 10.1111/dme.13108
14. Fadel MM, Abdel Ghaffar FR, Zwain SK, Ibrahim HM, Badr EA. Serum netrin and vcam-1 as biomarker for Egyptian patients with type II diabetes mellitus. Biochem Biophys Rep. (2021) 27:101045. doi: 10.1016/j.bbrep.2021.101045
15. Xu Y, Hou H, Zhao L. The role of vcam-1 in diabetic retinopathy: A systematic review and meta-analysis. J Diabetes Complications. (2023) 37:108380. doi: 10.1016/j.jdiacomp.2022.108380
16. Siddiqui K, George TP, Mujammami M, Isnani A, Alfadda AA. The association of cell adhesion molecules and selectins (Vcam-1, icam-1, E-selectin, L-selectin, and P-selectin) with microvascular complications in patients with type 2 diabetes: A follow-up study. Front Endocrinol (Lausanne). (2023) 14:1072288. doi: 10.3389/fendo.2023.1072288
17. Lee IT, Wang JS, Lee WJ, Lin SY, Fu CP, Liang KW, et al. The synergistic effect of vascular cell adhesion molecule-1 and coronary artery disease on brain-derived neurotrophic factor. Clin Chim Acta. (2017) 466:194–200. doi: 10.1016/j.cca.2017.01.026
18. Levey AS, Stevens LA, Schmid CH, Zhang YL, Castro AF 3rd, Feldman HI, et al. A new equation to estimate glomerular filtration rate. Ann Intern Med. (2009) 150:604–12. doi: 10.7326/0003-4819-150-9-200905050-00006
19. ElSayed NA, Aleppo G, Aroda VR, Bannuru RR, Brown FM, Bruemmer D, et al. 11. Chronic kidney disease and risk management: standards of care in diabetes-2023. Diabetes Care. (2023) 46:S191–202. doi: 10.2337/dc23-S011
20. Pan WH, Lee MS, Chuang SY, Lin YC, Fu ML. Obesity pandemic, correlated factors and guidelines to define, screen and manage obesity in Taiwan. Obes Rev. (2008) 9 Suppl 1:22–31. doi: 10.1111/j.1467-789X.2007.00434.x
21. Diabetes Association Of The Republic Of China T. Executive summary of the daroc clinical practice guidelines for diabetes care- 2018. J Formos Med Assoc. (2020) 119:577–86. doi: 10.1016/j.jfma.2019.02.016
22. Hang PZ, Ge FQ, Li PF, Liu J, Zhu H, Zhao J. The regulatory role of the bdnf/trkb pathway in organ and tissue fibrosis. Histol Histopathol. (2021) 36:1133–43. doi: 10.14670/hh-18-368
23. Zhao P, Li X, Li Y, Zhu J, Sun Y, Hong J. Mechanism of mir-365 in regulating bdnf-trkb signal axis of hfd/stz induced diabetic nephropathy fibrosis and renal function. Int Urol Nephrol. (2021) 53:2177–87. doi: 10.1007/s11255-021-02853-3
24. Hotulainen P, Hoogenraad CC. Actin in dendritic spines: connecting dynamics to function. J Cell Biol. (2010) 189:619–29. doi: 10.1083/jcb.201003008
25. Faul C, Asanuma K, Yanagida-Asanuma E, Kim K, Mundel P. Actin up: regulation of podocyte structure and function by components of the actin cytoskeleton. Trends Cell Biol. (2007) 17:428–37. doi: 10.1016/j.tcb.2007.06.006
26. Li M, Armelloni S, Zennaro C, Wei C, Corbelli A, Ikehata M, et al. Bdnf repairs podocyte damage by microrna-mediated increase of actin polymerization. J Pathol. (2015) 235:731–44. doi: 10.1002/path.4484
27. Liu JJ, Yeoh LY, Sum CF, Tavintharan S, Ng XW, Liu S, et al. Vascular cell adhesion molecule-1, but not intercellular adhesion molecule-1, is associated with diabetic kidney disease in asians with type 2 diabetes. J Diabetes Complications. (2015) 29:707–12. doi: 10.1016/j.jdiacomp.2015.02.011
28. Jia Y, Xu H, Yu Q, Tan L, Xiong Z. Identification and verification of vascular cell adhesion protein 1 as an immune-related hub gene associated with the tubulointerstitial injury in diabetic kidney disease. Bioengineered. (2021) 12:6655–73. doi: 10.1080/21655979.2021.1976540
29. Li Y, Huang Q, Fang M, Liu M, Guo J, Wang Z. Prognostic value of serum levels of multiple adhesion factors in patients with sepsis-induced acute kidney injury. Int Urol Nephrol. (2023) 55:1229–37. doi: 10.1007/s11255-022-03394-z
30. Su H, Liu B, Chen H, Zhang T, Huang T, Liu Y, et al. Lncrna anril mediates endothelial dysfunction through bdnf downregulation in chronic kidney disease. Cell Death Dis. (2022) 13:661. doi: 10.1038/s41419-022-05068-1
31. Golden E, Emiliano A, Maudsley S, Windham BG, Carlson OD, Egan JM, et al. Circulating brain-derived neurotrophic factor and indices of metabolic and cardiovascular health: data from the baltimore longitudinal study of aging. PloS One. (2010) 5:e10099. doi: 10.1371/journal.pone.0010099
32. Kaess BM, Preis SR, Lieb W, Beiser AS, Yang Q, Chen TC, et al. Circulating brain-derived neurotrophic factor concentrations and the risk of cardiovascular disease in the community. J Am Heart Assoc. (2015) 4:e001544. doi: 10.1161/jaha.114.001544
33. Hsu CY, Sheu WH, Lee IT. Brain-derived neurotrophic factor reduces long-term mortality in patients with coronary artery disease and chronic kidney disease. Front Cardiovasc Med. (2022) 9:881441. doi: 10.3389/fcvm.2022.881441
34. Lee IT, Li YH, Sheu WH. Brain-derived neurotrophic factor during oral glucose tolerance test predicts cardiovascular outcomes. Int J Mol Sci. (2020) 21:5008. doi: 10.3390/ijms21145008
35. Lee PT, Liao IC, Lee CH, Hsu LW, Liu PY. Expression of vascular cell adhesion molecule-1 in peripheral artery disease is enriched in patients with advanced kidney disease. Acta Cardiol Sin. (2021) 37:591–9. doi: 10.6515/acs.202111_37(6).20210701b
36. Ishii T, Warabi E, Mann GE. Mechanisms underlying unidirectional laminar shear stress-mediated nrf2 activation in endothelial cells: amplification of low shear stress signaling by primary cilia. Redox Biol. (2021) 46:102103. doi: 10.1016/j.redox.2021.102103
37. Fujii H, Kono K, Nishi S. Characteristics of coronary artery disease in chronic kidney disease. Clin Exp Nephrol. (2019) 23:725–32. doi: 10.1007/s10157-019-01718-5
38. Cho E, Kim M, Ko YS, Lee HY, Song M, Kim MG, et al. Role of inflammation in the pathogenesis of cardiorenal syndrome in a rat myocardial infarction model. Nephrol Dial Transplant. (2013) 28:2766–78. doi: 10.1093/ndt/gft376
39. Sabe MA, Claggett B, Burdmann EA, Desai AS, Ivanovich P, Kewalramani R, et al. Coronary artery disease is a predictor of progression to dialysis in patients with chronic kidney disease, type 2 diabetes mellitus, and anemia: an analysis of the trial to reduce cardiovascular events with aranesp therapy (TREAT). J Am Heart Assoc. (2016) 5:e002850. doi: 10.1161/JAHA.115.002850
40. Jiang Y, Wei N, Lu T, Zhu J, Xu G, Liu X. Intranasal brain-derived neurotrophic factor protects brain from ischemic insult via modulating local inflammation in rats. Neuroscience. (2011) 172:398–405. doi: 10.1016/j.neuroscience.2010.10.054
41. Anita NZ, Zebarth J, Chan B, Wu CY, Syed T, Shahrul D, et al. Inflammatory markers in type 2 diabetes with vs. Without cognitive impairment; a systematic review and meta-analysis. Brain Behav Immun. (2022) 100:55–69. doi: 10.1016/j.bbi.2021.11.005
42. Lin KD, Hsu CC, Ou HY, Wang CY, Chin MC, Shin SJ. Diabetes-related kidney, eye, and foot disease in Taiwan: an analysis of nationwide data from 2005 to 2014. J Formos Med Assoc. (2019) 118 Suppl 2:S103–10. doi: 10.1016/j.jfma.2019.07.027
43. Huang YY, Lin KD, Jiang YD, Chang CH, Chung CH, Chuang LM, et al. Diabetes-related kidney, eye, and foot disease in Taiwan: an analysis of the nationwide data for 2000-2009. J Formos Med Assoc. (2012) 111:637–44. doi: 10.1016/j.jfma.2012.09.006
44. Gong L, Wang C, Ning G, Wang W, Chen G, Wan Q, et al. High concentrations of triglycerides are associated with diabetic kidney disease in new-onset type 2 diabetes in China: findings from the China cardiometabolic disease and cancer cohort (4c) study. Diabetes Obes Metab. (2021) 23:2551–60. doi: 10.1111/dom.14502
45. Zaman SB, Karim MA, Hossain N, Al Kibria GM, Islam SMS. Plasma triglycerides as a risk factor for chronic kidney disease in type 2 diabetes mellitus: evidence from Northeastern Thailand. Diabetes Res Clin Pract. (2018) 138:238–45. doi: 10.1016/j.diabres.2018.02.011
Keywords: brain-derived neurotrophic factor, chronic kidney disease, synergistic effect, type 2 diabetes mellitus, vascular cell adhesion molecule-1
Citation: Chiang YH, Li YH, Chan YC, Cheng YC, Wu J, Lin JA, Huang WC and Lee IT (2024) Low brain-derived neurotrophic factor and high vascular cell adhesion molecule-1 levels are associated with chronic kidney disease in patients with type 2 diabetes mellitus. Front. Endocrinol. 15:1403717. doi: 10.3389/fendo.2024.1403717
Received: 19 March 2024; Accepted: 29 August 2024;
Published: 17 September 2024.
Edited by:
Antonino Sidoti, Azienda USL Toscana Sud Est, ItalyReviewed by:
Prema Velusamy, University of Texas at Arlington, United StatesArchana Gaur, All India Institute of Medical Sciences, India
Copyright © 2024 Chiang, Li, Chan, Cheng, Wu, Lin, Huang and Lee. This is an open-access article distributed under the terms of the Creative Commons Attribution License (CC BY). The use, distribution or reproduction in other forums is permitted, provided the original author(s) and the copyright owner(s) are credited and that the original publication in this journal is cited, in accordance with accepted academic practice. No use, distribution or reproduction is permitted which does not comply with these terms.
*Correspondence: I-Te Lee, aXRsZWVAdmdodGMuZ292LnR3
†These authors have contributed equally to this work