- 1Division of Endocrinology and Metabolism, Department of Internal Medicine, Korea University College of Medicine, Seoul, Republic of Korea
- 2Department of Biostatistics, Korea University College of Medicine, Seoul, Republic of Korea
Background: Mounting evidence indicates the importance of the interplay between skeletal muscles and lipid metabolism. Remnant cholesterol (remnant-C) is considered one of the principal residual risk factors for cardiovascular disease and metabolic disorders; however, there are limited studies on the impact of remnant-C on sarcopenia.
Methods: Data from the Korea National Health and Nutrition Examination Surveys (KNHANES) between 2008 and 2011 were used in this nationwide population-based study. In total, 17,408 participants were enrolled in this study. The subjects were categorized into four groups according to the quartile of remnant-C values. We conducted multivariable logistic regression analysis to evaluate the association between remnant-C and muscle mass measured using dual-energy X-ray absorptiometry.
Results: A total of 1,791 participants (10.3%) presented low muscle mass, and there was a sequential increase in the percentage of low muscle mass across remnant-C quartiles (Q1, 5.2%; Q2, 8.7%; Q3, 11.5%; Q4, 15.7%). In the full adjusted model, those in the highest remnant-C quartile group showed significantly increased odds ratio (OR) for low muscle mass compared with those in the lowest remnant-C group after adjusting for various confounding factors (OR = 1.33, 95% confidence interval (CI) = 1.06–1.68, P <0.05). A wide range of subgroups and sensitivity analyses showed consistent results, supporting the robustness of our findings.
Conclusions: Increased remnant-C value was associated with a high risk of low muscle mass in the Korean population. Remnant-C may be a novel marker for the prediction and management of sarcopenia in aging societies.
Introduction
Sarcopenia is characterized by the loss of skeletal muscle mass, lower muscle strength, and/or physical performance, which imposes a global health burden, leading to an increased risk of falls, functional decline, and mortality (1). The prevalence of sarcopenia is estimated to be 5%–13% in population aged 60–70 years, and 11%–50% in those aged 80 or above (2). It is projected to increase at an accelerated pace in aging societies. Despite its adverse health outcomes, it is difficult to manage sarcopenia in clinical practice, because there are currently no FDA-approved drugs targeting sarcopenia. Therefore, evaluating and managing traditional and novel risk factors is important to minimize the adverse health outcomes caused by sarcopenia.
Recently, remnant cholesterol (remnant-C) has drawn wide attention as a lipid profile based on the cholesterol content of remnant lipoprotein particles, which are derived by lipolysis from triglyceride-rich lipoproteins (TRLs), including chylomicron remnants, very-low-density lipoproteins, and intermediate-density lipoproteins (3). In spite of definitive role of low-density lipoprotein cholesterol (LDL-C) lowering, there is a substantial residual risk of atherosclerotic cardiovascular disease (ASCVD). Owing to its atherosclerotic characteristics, remnant-C has been proposed as a key parameter for assessing the residual risk of ASCVD beyond traditional lipid profiles (4). Furthermore, remnant-C may be more atherogenic than LDL-C because of its pro-inflammatory effects and persistent retention time in the vasculature (5). Several longitudinal studies have demonstrated a remarkable association between remnant-C and ASCVD, even after LDL-C reduction to the recommended target (4, 5). Moreover, other metabolic diseases such as type 2 diabetes mellitus, metabolic syndrome, and non-alcoholic fatty liver disease (NAFLD) have been reported to be associated with remnant-C in previous studies (6, 7). Therefore, new medications targeting remnant-C, such as novel inhibitors of Apo-CIII and angiopoietin-like 3 (ANGPLT3), have been actively explored (8).
Dyslipidemia can induce ectopic fat deposition, and lipid accumulation between and within muscle cells disturbs normal physiology, leading to decreased muscle mass and quality (9). Mitochondrial dysfunction, oxidative stress, and increased inflammatory responses through various mediators such as proinflammatory cytokines can explain this vicious relationship between lipids and muscles, although the exact molecular mechanism remains to be elucidated (9). Considering that skeletal muscle is involved in regulating lipid metabolism and remnant-C is linked to various metabolic disorders (10, 11), the association between remnant-C and sarcopenia might be an interesting research topic. Few studies have reported an association between sarcopenia/sarcopenic obesity and dyslipidemia as a component of metabolic syndrome (12, 13). However, limited studies have investigated the association between remnant-C and sarcopenia.
Therefore, this study aimed to identify the relationship between remnant-C and low muscle mass, a major factor defining sarcopenia, using nationwide population-based data.
Methods
Data source and study design
We used data from the Korean National Health and Nutrition Examination Surveys (KNHANES) between 2008 and 2011. The KNHANES is a nationwide cross-sectional survey regularly conducted by the Korean Center for Disease Control and Prevention to monitor the health and nutritional status of the general population in South Korea (14). Each KNHANES is comprised of independent datasets as previously demonstrated (15). The 4th KNHANES was conducted from 2007 to 2009, and the 5th survey was conducted from 2010 to 2012. We used data from 2nd and 3rd year of the 4th KNANES (IV-2, IV-3) as well as 1st and 2nd year of the 5th survey (V-1, V2) because they included assessments of muscle mass.
Among the 21,303 subjects from the KNHANES 2008–2011, participants aged <20 years (n = 2,173) were excluded. Subjects with TG level ≥400 mg/dL (n = 424) and missing data (n = 1,330) were also excluded. We considered remnant-C minus values (n = 6) as outlier data and excluded from the analysis. Ultimately, 17,408 participants were included in the analysis (7,390 men, 10,018 women; Figure 1).
This study was approved by the Institutional Review Board of Korea University and was conducted in accordance with the Declaration of Helsinki of the World Medical Association.
Assessment of remnant cholesterol and definition of low muscle mass
Blood samples were collected after an overnight fast. Remnant-C was calculated as total cholesterol minus LDL-C and HDL-C. Appendicular skeletal muscle mass (ASM) was measured using dual-energy X-ray absorptiometry. Low muscle mass was defined as ASM (kg) divided by body mass index (BMI, kg/m2) <0.789 for men and <0.512 for women, according to The Foundation for the National Institutes of Health Sarcopenia Project (16).
Covariates
Hypertension was defined as blood pressure ≥140/90 mmHg or the use of anti-hypertensive medication. Diabetes was defined as fasting blood glucose (FBG) ≥126 mg/dL or use of oral hypoglycemic agents or insulin. Dyslipidemia was defined as fasting total cholesterol ≥240 mg/dL or use of lipid lowering agents. Metabolic syndrome was defined according to the modified National Cholesterol Education Program criteria (17). Estimated glomerular filtration rate (eGFR) was calculated from Chronic Kidney Disease Epidemiology Collaboration equation (CKD-EPI) (18), and CKD was defined as eGFR ≤60 mL/min/1.73 m2. Homeostasis model assessment of insulin resistance (HOMA-IR) was calculated as fasting serum insulin (μIU/mL) × fasting glucose (mg/dL)/405. Smoking status was categorized as never smoked, ex-smoker, or current smoker. High risk drinking was defined as ≥7 cups for men, ≥5 cups for women at a time, and ≥2 times per week. Regular exercise was defined as moderate or high-intensity physical activity on a regular basis (≥3 times per week and ≥20 min at a time) (19). Household incomes were divided into quartiles.
Statistical analysis
The subjects were divided into four groups according to the quartile of remnant-C values (6). The characteristics of the participants are expressed as mean ± standard deviation (SD) for continuous variables and as number (%) for categorical variables. Differences between groups were compared using Student’s t-test for continuous variables and the chi-square test for categorical variables. Complex sample analysis was conducted using unadjusted and adjusted multivariable logistic regression models to evaluate the association between remnant-C and low muscle mass. Subgroup analysis was performed and stratified by age, sex, BMI, LDL-C level, eGFR, FBG level, and systolic blood pressure (SBP). Additionally, a sensitivity analysis was performed to control for the effects of lipid-lowering agents and underlying diseases. P value <0.05 was considered statistically significant. All the analyses were performed using SAS version 9.4 (SAS Institute Inc., Cary, NC, USA).
Results
Baseline characteristics of the study population
The baseline characteristics of the study participants according to the remnant-C quartiles are summarized in Table 1. Participants in the higher remnant-C quartiles were more likely to be male and older than those in the lower remnant-C quartiles. They had higher BMI, waist circumference, blood pressure, FBG, HOMA-IR, total cholesterol, LDL-C, triglycerides (TG), and liver enzymes, as well as lower eGFR. The proportions of current smokers and heavy drinkers were higher in participants in high remnant-C quartile group, and they tended to exercise less regularly. The prevalence of coronary heart disease, cerebrovascular disease, hypertension, metabolic syndrome, diabetes, and hypercholesterolemia was also higher in participants in the high remnant-C quartiles.
Of the 17,408 participants, 1,791 (10.3%) had low muscle mass. There was a sequential increase in the percentages of low muscle mass across the remnant-C quartiles from Q1 to Q4 [5.2%, 8.7%, 11.5%, and 15.7%, respectively (P for trend <0.01)] (Figure 2).
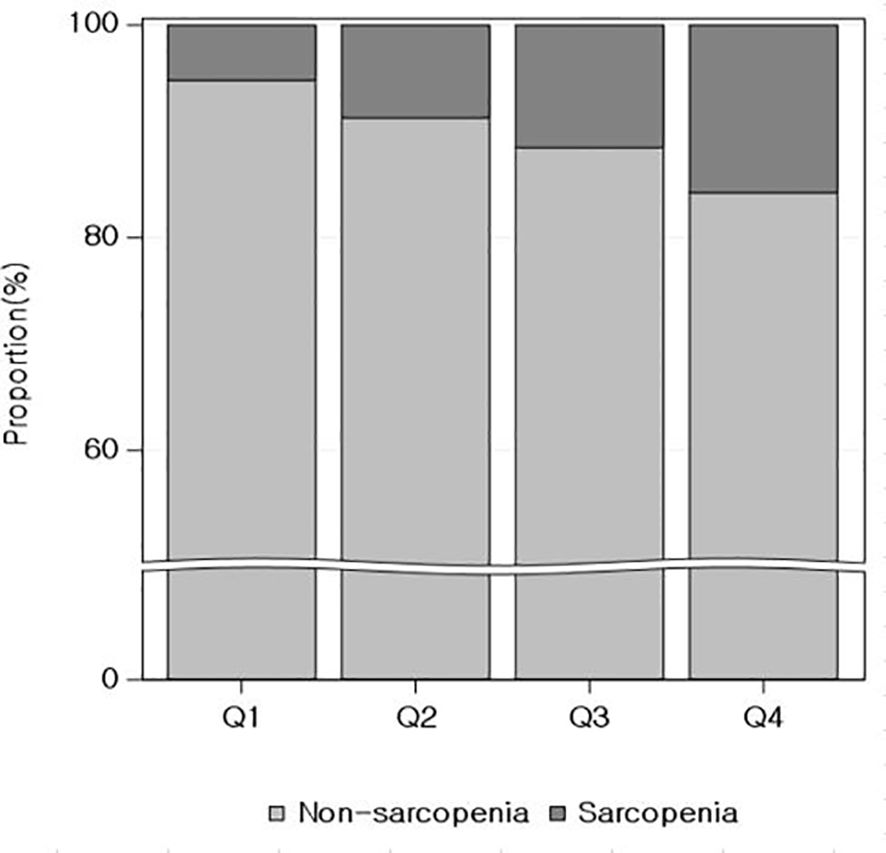
Figure 2. Relationship between quartiles of remnant cholesterol and the proportion of low muscle mass.
Association between remnant-C and risk of low muscle mass
The odds ratios (ORs) and 95% confidence intervals (CIs) for low muscle mass according to remnant-C quartiles are presented in Table 2. The crude model exhibited sequential increases in ORs across remnant-C quartiles (OR [95% CI]: first quartile [Q1], reference; second quartile [Q2], 1.7 [1.38-2.09]; third quartile [Q3], 2.18 [1.8-2.65]; fourth quartile [Q4], 3.44 [2.84-4.17]). This sequential trend persisted after adjusting for age and sex (Q2, 1.31 [1.06-1.61]; Q3, 1.43 [1.17-1.74]; Q4, 2.05 [1.68-2.52]) (Model 1). After adjusting for age, sex, and BMI, the second quartile group showed a higher risk of low muscle mass than the third quartile group; however, the fourth quartile group still showed the highest risk among all groups. In the fully adjusted model, the trend persisted and statistical significance was maintained. The highest remnant-C quartile group presented a 33% increased risk of low muscle mass compared with the lowest quartile group (Model 6, Q4, 1.33 [1.06-1.68], P <0.05).
Subgroup analysis
Stratification by age, sex, BMI, LDL-C, eGFR, FBG, and SBP was performed and the relationship between remnant-C and low muscle mass was investigated (Figure 3). In all subgroups, the highest quartile group (Q4) of remnant-C had an increased risk of low muscle mass compared with the rest three quartile groups (Q1-Q3). In hypertensive patients, the association between remnant-C and increased risk of low muscle mass was more remarkable than those without hypertension, implicating the synergistic effect of hypertension and dyslipidemia on muscle (P for interaction <0.05). However, age, sex, BMI, LDL-C, eGFR, and FBS did not significantly modify the effect.
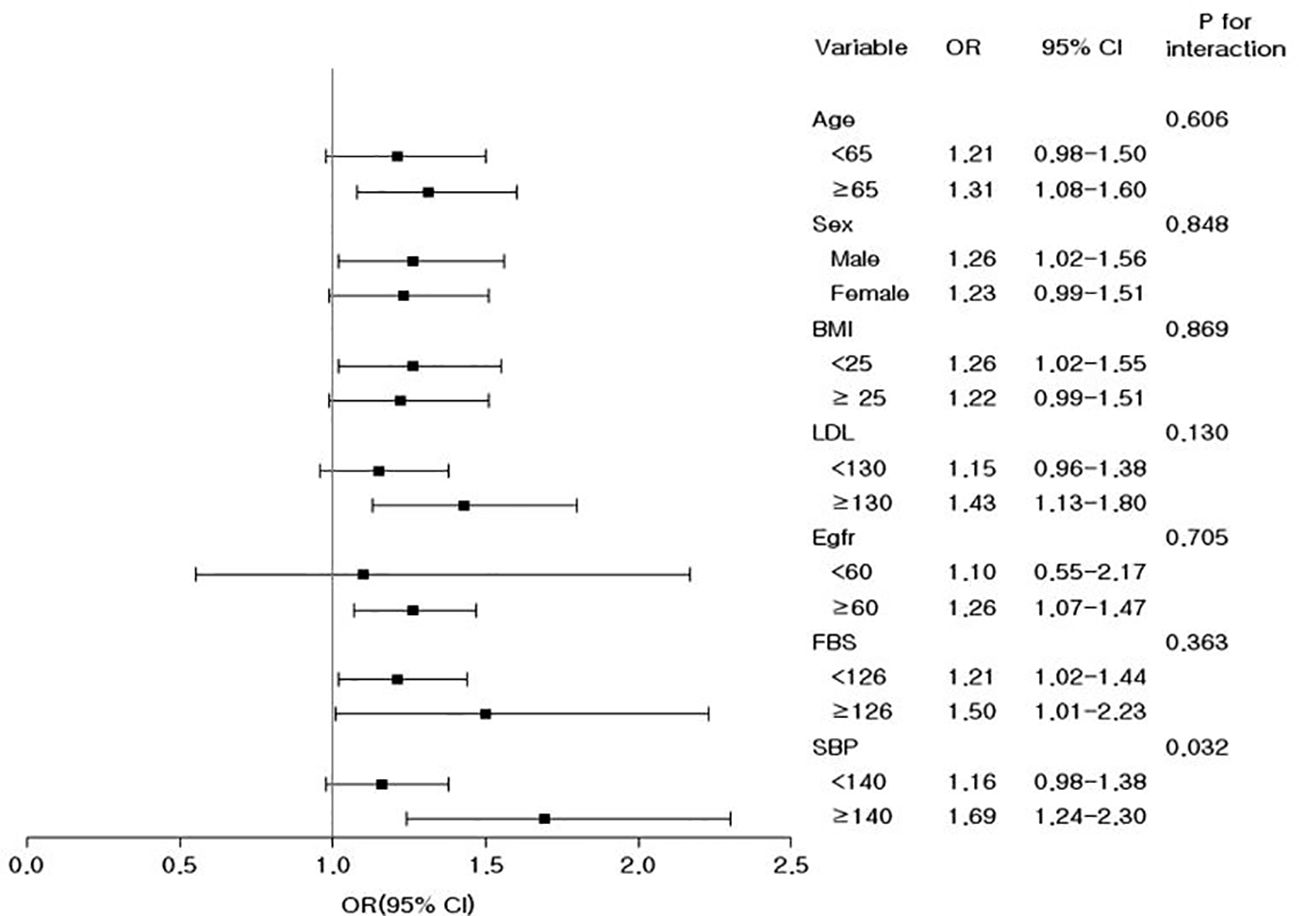
Figure 3. Odds ratios and 95% confidence intervals for the prevalence of low muscle mass in the highest quartile versus the remaining three quartiles of remnant cholesterol in the subgroups. Adjusted for age, sex, body mass index, fasting glucose, estimated glomerular filtration rate, systolic blood pressure, dyslipidemia medication, history of cerebrovascular and coronary heart disease, drinking, smoking, exercise, and income.
Sensitivity analysis
To reinforce our results, we conducted a sensitivity analysis (Table 3). As lipid-lowering agents can significantly influence blood lipid profiles, we analyzed the participants after excluding those using dyslipidemia medication and confirmed results consistent with the main analysis (Model 6: Q4, 1.31 [1.03-1.67], P <0.05). Furthermore, to clarify the independent effect of remnant-C on low muscle mass, regardless of underlying diseases, we excluded subjects with a history of coronary syndrome, stroke, diabetes, and CKD, respectively. The results corresponded with those of the main analysis, and statistical significance was maintained in all models.
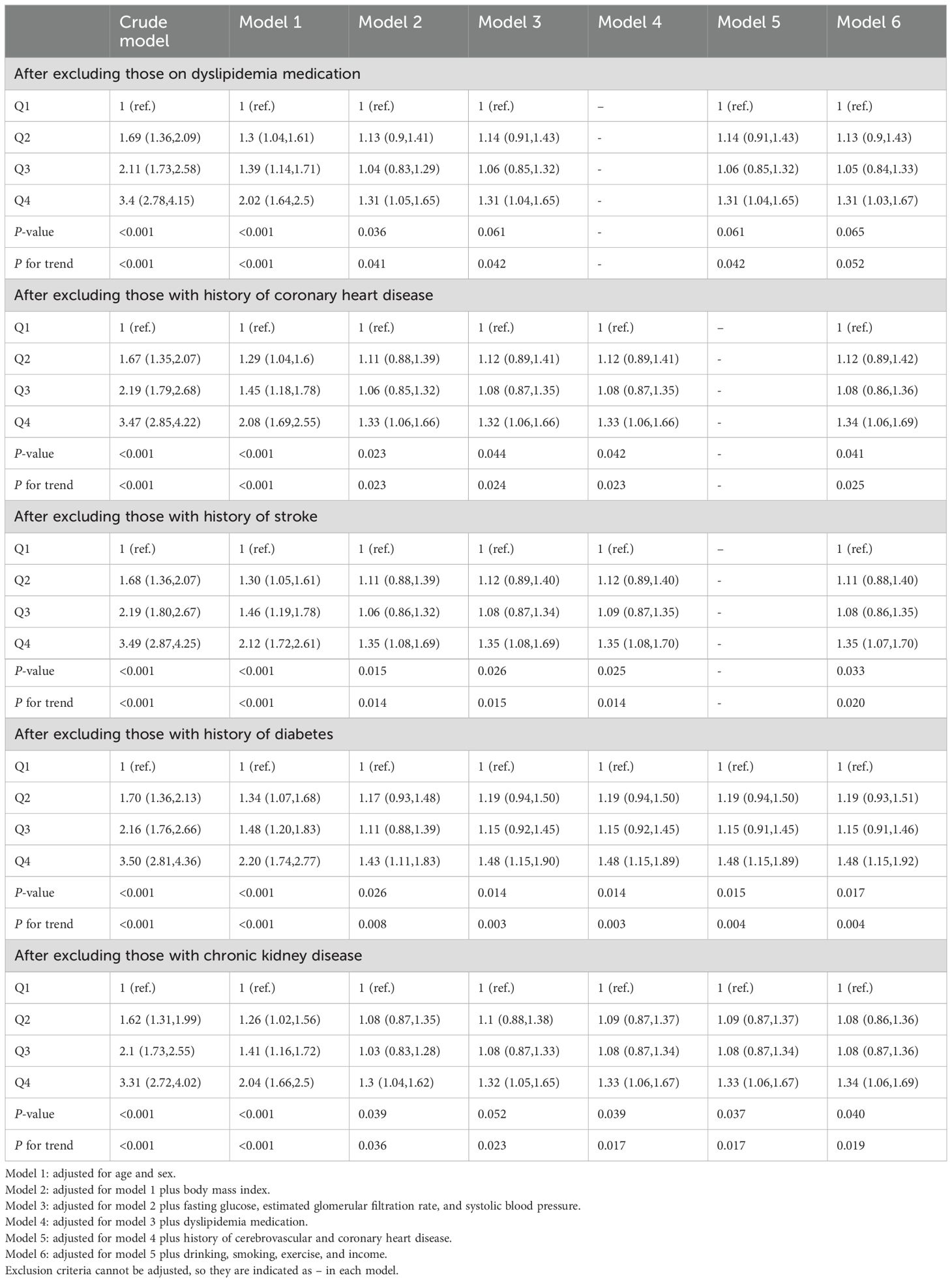
Table 3. Odds ratios and 95% confidence intervals for low muscle mass according to medication and underlying diseases.
Discussion
The present study showed that Korean adults with elevated levels of remnant-C demonstrated a high prevalence of low muscle mass. These findings remained consistent even after adjusting for potential confounding factors in our large national population-based database. The present results suggest that patients with increased remnant-C may be needed to evaluate sarcopenia and its associated comorbidities.
Changes in body composition, such as redistribution of muscle and fat, are characteristic during human aging. Decreased muscle mass can result in loss of independence in everyday life and exacerbate diseases of vital organs, leading to high mortality via signaling mediators called myokines (9). Therefore, the global burden and importance of sarcopenia are inevitably increasing in a progressive aging society. Currently, the interventions for preventing and treating sarcopenia focus on physical exercise and protein supplementations (20). However, the enforcement of resistance exercises with proper nutritional support for the elderly is challenging because of anabolic resistance and poor long-term compliance (20, 21). Therefore, investigating novel prophylactic and therapeutic targets for sarcopenia is required.
Several epidemiological studies and clinical trials have shown that remnant-C predicts the risk of ASCVD, which may surpass LDL-C and ApoB levels (22). On a per particle basis, remnant particles have up to four-fold greater cholesterol than LDL-C particles owing to their larger size (23). By including remnant-C levels in a conventional risk model, the reclassification of individuals to predict future cardiovascular events is improved in primary and secondary prevention settings (24). Remnant-C has attracted attention as a residual risk factor for ASCVD because it is permeable to the arterial intima and is retained in the arterial wall, making it atherogenic (25). The accumulated remnant-C is absorbed by macrophages and smooth muscle cells, resulting in the formation of foam cells and finally develop atherosclerotic plaques (4, 26). Skeletal muscle is highly vascularized, and vascular system from feeding arteries branching into arterioles and capillaries play a significant role in muscle metabolism, mainly through transporting nutrition and oxygen (27). Remnants of TRLs may easily cross the endothelium in the vasculature of skeletal muscle. Accumulation of remnant-C would facilitate foam cell formation, leading to atherosclerotic plaques. Thickened blood vessel walls and narrowed vessels might hamper sufficient nutrition and oxygen supply to skeletal muscle, which is critical in muscle growth and function.
Few studies have evaluated the relationship between dyslipidemia and sarcopenia/sarcopenic obesity. Lu et al. reported a 12-fold increase in the risk of metabolic syndrome in patients with sarcopenic obesity compared to the normal group (13). As components of the metabolic syndrome, there is a significant association between sarcopenic obesity and serum triglyceride and HDL-C levels (13). Baek et al. examined dyslipidemia as a separate factor associated with sarcopenia/sarcopenic obesity in the elderly Korean population (12). Bi and Dong et al. showed that dyslipidemia is positively related with sarcopenia in the elderly population, and this association was significantly affected by age, sex, and location of the participants (28). Vella et al. reported that abdominal muscle area was inversely correlated with total cholesterol, triglycerides, and very low density cholesterol (29). In addition, plasma HDL-C level has been reported as a meaningful predictor of sarcopenia in healthy old people (30). Gong et al. reported that the levels of TRLs and their remnants were higher in the sarcopenia group than in the control group in 84 elderly Chinese subjects, using bioelectrical impedance analysis (BIA) to assess skeletal muscle mass (10). With established cardiovascular benefits of lipid lowering drugs, such as statins, there is still a controversy on the association between lipid lowering drugs and sarcopenia (31). Further research needs to be done to elucidate effects of dyslipidemia medications on muscle. Our results underscore the relationship between dyslipidemia and sarcopenia, which imposes a critical burden on future aging societies. In subgroup analysis, the relationship between remnant-C and low muscle mass was more prominent in hypertensive patients. Although there are some previous studies demonstrating that hypertension is associated with increased risk of sarcopenia (32), there is still a controversy about the impact of hypertension on sarcopenia (33). Whether comorbidity of dyslipidemia and hypertension confers synergistic effect on muscle health is yet to be elucidated. Nevertheless, possible link between ischemic heart disease and frailty, whose characteristics share common factors with sarcopenia, has been raised (34). More comprehensive future research in clinical and biological fields is required to clarify the interconnection among dyslipidemia, cardiovascular disease, and muscle disease.
The underlying mechanism linking remnant-C to low muscle mass is not yet fully understood. Saturated fatty acids such as palmitate induce muscle atrophy by modulating insulin signaling. Palmitate reduces Akt activity and increases nuclear FoxO3 protein levels, which leads to increased expression of atrophy-inducing genes (atrogenes), such as the E3 ubiquitin ligase atrogin-1/MAFbx and the autophagy mediator Bnip3 (35). Palmitate treatment increases intramyocellular lipids and induces myotube atrophy (36). The accumulation of lipids and their derivatives, such as diacylglycerol and ceramides, has been reported to be toxic to muscles. One of their harmful effects is insulin resistance, which is a pivotal pathophysiological factor in sarcopenia. Previous research has indicated that the deposition of free fatty acids in muscles disrupts insulin signaling, leading to insulin resistance (37). TG involved in TRLs are hydrolyzed by lipoprotein lipase on the surface of the capillary endothelium in skeletal muscles (23), producing fatty acids as metabolites that reduce insulin sensitivity. Grice et al. reported that excessive cholesterol accumulation in skeletal muscle membranes is an early reversible aspect of insulin resistance in high fat diet-fed mice (38). Cholesterol accumulation within cells can activate RhoA, which is significantly involved in cellular functions, and damage them in the liver and heart (39, 40). Given that RhoA is important in the differentiation and proliferation of skeletal muscle cells, the accumulation of cholesterol itself might disturb the normal physiology of skeletal muscle, such as by balancing protein anabolism/catabolism or maintaining glucose homeostasis. Excessive remnants could contribute to ectopic fat deposition in skeletal muscle and lead to sarcopenic state, although whether and how specific composition of lipids have different effects on muscle is not fully identified.
As remnant-C has been reported to be associated with low-grade inflammation (41), inflammatory responses may explain the association between remnant-C and sarcopenia. Unlike LDL-C, remnant-C which passed through the vessel wall easily forms foam cell because remnant-C needs no modification to be trapped by macrophage (42). Foam cell is a well-known attribute of inflammation (43). Remnant-C could induce pro-inflammatory cytokines such as tumor necrosis factor-α and interleukine-1, 6, 8 (44), and skeletal muscle react to pro-inflammatory cytokines with muscle atrophy (9). Pro-inflammatory cytokines may antagonize the anabolism of insulin-like growth factor-1 (IGF-1) in muscle and promote cell apoptosis, which result in decreased muscle mass (45). Accumulating evidence suggests that sarcopenia is closely associated with chronic low-grade inflammation (46).
Increased oxidative stress and reactive oxygen species production have been reported to be associated with increased TRLs and their lipolysis (47). Remnant-C levels are inversely correlated with HDL-C levels, which could prevent oxidative stress (22). Furthermore, TRLs lipolysis elicits endothelial cell inflammation and dysfunction by stimulating the production of reactive oxygen species (48). Excessive oxidative stress and the production of reactive oxygen species induce protein misfolding and reduce protein synthesis (37), which may contribute to low muscle mass.
This study had several limitations. First, this is a cross-sectional study; therefore, the causal relationship between remnant-C and sarcopenia cannot be elucidated. Secondly, although low muscle mass is an important component of sarcopenia, the lack of information on muscle strength and physical performance in the present database does not fully explain the relationship between remnant-C and sarcopenia. Future studies including assessments of muscle strength and physical performance would be needed to clarify the relationship between remnant-C and sarcopenia. Third, there was no detailed information on the dyslipidemia medications. However, we analyzed the participants after excluding those taking lipid-lowering agents and confirmed consistent results. Fourth, although we have excluded confounding effects of lipid-associated diseases including CVD, diabetes, or CKD, we could not exclude muscle diseases such as Duchenne muscular dystrophy due to lack of detailed information. The effect of muscle-associated diseases should be addressed for more validated results in the future. Finally, directly measured remnant-C levels are not available in the KNHANES database. However, a relatively high concordance between measured and calculated remnant-C values was reported in a previous study (49). Furthermore, individuals with increased calculated remnant-C levels are at an increased risk of incident CVD independent of LDL-C levels in Korean population-based studies (50).
The strength of this study is that it is the first to show an association between remnant-C and low muscle mass using a validated nationwide survey with a large sample size, standard data collection methods, and the availability of a large number of confounding factors. Moreover, the results were confirmed by a wide range of subgroup and sensitivity analyses, excluding the confounding effects of underlying diseases, such as CVD, diabetes, and CKD.
Conclusion
In conclusion, remnant-C is associated with low muscle mass in the Korean population and may be a promising marker for predicting and managing sarcopenia. Our results provide new insights into the impact of remnant-C on skeletal muscle in an aging society. Further research is needed to better understand the molecular mechanism linking remnant cholesterol and skeletal muscle and to support its clinical usefulness.
Data availability statement
The datasets presented in this study can be found in online repositories. The names of the repository/repositories and accession number(s) can be found in the article/supplementary material.
Author contributions
SJ: Writing – review & editing, Writing – original draft, Conceptualization. S-YH: Writing – review & editing, Formal Analysis, Conceptualization. AJ: Writing – review & editing. KK: Writing – review & editing. JY: Writing – review & editing. NamK: Writing – review & editing. HY: Writing – review & editing. SK: Writing – review & editing. NanK: Writing – review & editing. SB: Writing – review & editing. KC: Writing – review & editing, Writing – original draft.
Funding
The author(s) declare financial support was received for the research, authorship, and/or publication of this article. This work was supported by the National Research Foundation of Korea (NRF) grant funded by the Korea government (MSIT) (RS-2023-00220894). This work was supported by Korea University Guro Hospital (Z2300011) and grant funded by Korea University (K2325931, K2409361).
Acknowledgments
Thanks to the public database and the efforts of the authors.
Conflict of interest
The authors declare that the research was conducted in the absence of any commercial or financial relationships that could be construed as a potential conflict of interest.
Publisher’s note
All claims expressed in this article are solely those of the authors and do not necessarily represent those of their affiliated organizations, or those of the publisher, the editors and the reviewers. Any product that may be evaluated in this article, or claim that may be made by its manufacturer, is not guaranteed or endorsed by the publisher.
References
1. Cruz-Jentoft AJ, Sayer AA. Sarcopenia. Lancet. (2019) 393:2636–46. doi: 10.1016/S0140-6736(19)31138-9
2. von Haehling S, Morley JE, Anker SD. An overview of sarcopenia: facts and numbers on prevalence and clinical impact. J cachexia sarcopenia Muscle. (2010) 1:129–33. doi: 10.1007/s13539-010-0014-2
3. Nordestgaard BG, Varbo A. Triglycerides and cardiovascular disease. Lancet. (2014) 384:626–35. doi: 10.1016/S0140-6736(14)61177-6
4. Quispe R, Martin SS, Michos ED, Lamba I, Blumenthal RS, Saeed A, et al. Remnant cholesterol predicts cardiovascular disease beyond LDL and ApoB: a primary prevention study. Eur Heart J. (2021) 42:4324–32. doi: 10.1093/eurheartj/ehab432
5. Ganda OP. Triglyceride-rich lipoproteins, remnant-cholesterol, and atherosclerotic cardiovascular disease. Curr Opin Lipidol. (2023) 34:105–13. doi: 10.1097/MOL.0000000000000875
6. Huh JH, Roh E, Lee SJ, Ihm SH, Han KD, Kang JG. Remnant cholesterol is an independent predictor of type 2 diabetes: A nationwide population-based cohort study. Diabetes Care. (2023) 46:305–12. doi: 10.2337/dc22-1550
7. Chen JF, Su YJ, Su X, Luo F. Remnant cholesterol has a non-linear association with non-alcoholic fatty liver disease. Diabetes Res Clin Pr. (2023) 201. doi: 10.1016/j.diabres.2023.110733
8. Rosenson RS, Shaik A, Song W. New therapies for lowering triglyceride-rich lipoproteins: JACC focus seminar 3/4. J Am Coll Cardiol. (2021) 78:1817–30. doi: 10.1016/j.jacc.2021.08.051
9. Li CW, Yu K, Shyh-Chang N, Jiang Z, Liu T, Ma S, et al. Pathogenesis of sarcopenia and the relationship with fat mass: descriptive review. J Cachexia Sarcopenia Muscle. (2022) 13:781–94. doi: 10.1002/jcsm.12901
10. Gong H, Liu Y, Lyu X, Dong L, Zhang X. Lipoprotein subfractions in patients with sarcopenia and their relevance to skeletal muscle mass and function. Exp Gerontol. (2022) 159:111668. doi: 10.1016/j.exger.2021.111668
11. Zou Y, Kuang MB, Zhong YJ, Jiang CY. Remnant cholesterol can identify individuals at higher risk of metabolic syndrome in the general population. Sci Rep-Uk. (2023) 13. doi: 10.1038/s41598-023-33276-y
12. Baek S, Nam G, Han K, Choi S, Jung S, Bok A, et al. Sarcopenia and sarcopenic obesity and their association with dyslipidemia in Korean elderly men: the 2008–2010 Korea National Health and Nutrition Examination Survey. J endocrinological Invest. (2014) 37:247–60. doi: 10.1007/s40618-013-0011-3
13. Lu C-W, Yang K-C, Chang H-H, Lee L-T, Chen C-Y, Huang K-C. Sarcopenic obesity is closely associated with metabolic syndrome. Obes Res Clin Pract. (2013) 7:e301–e7. doi: 10.1016/j.orcp.2012.02.003
14. Kweon S, Kim Y, Jang MJ, Kim Y, Kim K, Choi S, et al. Data resource profile: the Korea National Health and Nutrition Examination Survey (KNHANES). Int J Epidemiol. (2014) 43:69–77. doi: 10.1093/ije/dyt228
15. Han E, Lee Y-H, Kim G, Kim SR, Lee B-W, Kang ES, et al. Sarcopenia is associated with albuminuria independently of hypertension and diabetes: KNHANES 2008–2011. Metabolism. (2016) 65:1531–40. doi: 10.1016/j.metabol.2016.07.003
16. Studenski SA, Peters KW, Alley DE, Cawthon PM, McLean RR, Harris TB, et al. The FNIH sarcopenia project: rationale, study description, conference recommendations, and final estimates. J Gerontol A Biol Sci Med Sci. (2014) 69:547–58. doi: 10.1093/gerona/glu010
17. Grundy SM, Cleeman JI, Daniels SR, Donato KA, Eckel RH, Franklin BA, et al. Diagnosis and management of the metabolic syndrome: an American Heart Association/National Heart, Lung, and Blood Institute Scientific Statement. Circulation. (2005) 112:2735–52. doi: 10.1161/CIRCULATIONAHA.105.169404
18. Levey AS, Stevens LA, Schmid CH, Zhang YL, Castro AF 3rd, Feldman HI, et al. A new equation to estimate glomerular filtration rate. Ann Intern Med. (2009) 150:604–12. doi: 10.7326/0003-4819-150-9-200905050-00006
19. Lee YH, Kim SU, Song K, Park JY, Ahn SH, Lee BW, et al. Sarcopenia is associated with significant liver fibrosis independently of obesity and insulin resistance in nonalcoholic fatty liver disease: nationwide surveys (KNHANES 2008-2011). Hepatology. (2016) 63:776–86. doi: 10.1002/hep.28376
20. Al Saedi A, Debruin DA, Hayes A, Hamrick M. Lipid metabolism in sarcopenia. Bone. (2022) 164:116539. doi: 10.1016/j.bone.2022.116539
21. Kumar V, Selby A, Rankin D, Patel R, Atherton P, Hildebrandt W, et al. Age-related differences in the dose-response relationship of muscle protein synthesis to resistance exercise in young and old men. J Physiol. (2009) 587:211–7. doi: 10.1113/jphysiol.2008.164483
22. Wang K, Wang R, Yang J, Liu X, Shen H, Sun Y, et al. Remnant cholesterol and atherosclerotic cardiovascular disease: Metabolism, mechanism, evidence, and treatment. Front Cardiovasc Med. (2022) 9:913869. doi: 10.3389/fcvm.2022.913869
23. Ginsberg HN, Packard CJ, Chapman MJ, Borén J, Aguilar-Salinas CA, Averna M, et al. Triglyceride-rich lipoproteins and their remnants: metabolic insights, role in atherosclerotic cardiovascular disease, and emerging therapeutic strategies—a consensus statement from the European Atherosclerosis Society. Eur Heart J. (2021) 42:4791–806. doi: 10.1093/eurheartj/ehab551
24. Doi T, Nordestgaard BG, Langsted A. Can remnant cholesterol (triglyceride-rich lipoproteins) reclassify estimated risk of atherosclerotic cardiovascular disease? Curr Opin Endocrinology Diabetes Obes. (2023) 30:128–35. doi: 10.1097/MED.0000000000000799
25. Boren J, Taskinen M-R, Björnson E, Packard CJ. Metabolism of triglyceride-rich lipoproteins in health and dyslipidaemia. Nat Rev Cardiol. (2022) 19:577–92. doi: 10.1038/s41569-022-00676-y
26. Burnett JR, Hooper AJ, Hegele RA. Remnant cholesterol and atherosclerotic cardiovascular disease risk. J Am Coll Cardiol. (2020) 76:2736–9. doi: 10.1016/j.jacc.2020.10.029
27. Latroche C, Gitiaux C, Chretien F, Desguerre I, Mounier R, Chazaud B. Skeletal muscle microvasculature: A highly dynamic lifeline. Physiol (Bethesda). (2015) 30:417–27. doi: 10.1152/physiol.00026.2015
28. Bi BQ, Dong XY, Yan ML, Zhao Z, Liu RT, Li SG, et al. Dyslipidemia is associated with sarcopenia of the elderly: a meta-analysis. BMC Geriatr. (2024) 24. doi: 10.1186/s12877-024-04761-4
29. Vella CA, Nelson MC, Unkart JT, Miljkovic I, Allison MA. Skeletal muscle area and density are associated with lipid and lipoprotein cholesterol levels: The Multi-Ethnic Study of Atherosclerosis. J Clin lipidology. (2020) 14:143–53. doi: 10.1016/j.jacl.2020.01.002
30. Abu−Libdeh W, Khrais J, Suwan L, Hamdan F, Qandil A, Kaml AA, et al. Relation of plasma high−Density lipoproteins−Cholesterol with sarcopenia in patients with chronic obstructive pulmonary. Indian J Respir Care¦ Volume. (2022) 11:328.
31. Mellen RH, Girotto OS, Marques EB, Laurindo LF, Grippa PC, Mendes CG, et al. Insights into pathogenesis, nutritional and drug approach in sarcopenia: A systematic review. Biomedicines. (2023) 11. doi: 10.3390/biomedicines11010136
32. Kara M, Kara O, Ceran Y, Kaymak B, Kaya TC, Citir BN, et al. SARcopenia assessment in hypertension: the SARAH study. Am J Phys Med Rehabil. (2023) 102:130–6. doi: 10.1097/PHM.0000000000002045
33. Gao QQ, Hu KY, Yan CJ, Zhao B, Mei F, Chen F, et al. Associated factors of sarcopenia in community-dwelling older adults: A systematic review and meta-analysis. Nutrients. (2021) 13. doi: 10.3390/nu13124291
34. Liperoti R, Vetrano DL, Palmer K, Targowski T, Cipriani MC, Lo Monaco MR, et al. Association between frailty and ischemic heart disease: a systematic review and meta-analysis. BMC Geriatr. (2021) 21. doi: 10.1186/s12877-021-02304-9
35. Woodworth-Hobbs ME, Hudson MB, Rahnert JA, Zheng B, Franch HA, Price SR. Docosahexaenoic acid prevents palmitate-induced activation of proteolytic systems in C2C12 myotubes. J Nutr Biochem. (2014) 25:868–74. doi: 10.1016/j.jnutbio.2014.03.017
36. Bryner RW, Woodworth-Hobbs ME, Williamson DL, Alway SE. Docosahexaenoic Acid protects muscle cells from palmitate-induced atrophy. ISRN Obes. (2012) 2012:647348. doi: 10.5402/2012/647348
37. Park SS, Seo YK. Excess accumulation of lipid impairs insulin sensitivity in skeletal muscle. Int J Mol Sci. (2020) 21. doi: 10.3390/ijms21061949
38. Grice BA, Barton KJ, Covert JD, Kreilach AM, Tackett L, Brozinick JT, et al. Excess membrane cholesterol is an early contributing reversible aspect of skeletal muscle insulin resistance in C57BL/6NJ mice fed a Western-style high-fat diet. Am J Physiology-Endocrinology Metab. (2019) 317:E362–E73. doi: 10.1152/ajpendo.00396.2018
39. Xue T, Li C, Zhang H, Han Y, Wu J. Effects of Aster B-mediated intracellular accumulation of cholesterol on inflammatory process and myocardial cells in acute myocardial infarction. Hellenic J Cardiol. (2022) 63:32–9. doi: 10.1016/j.hjc.2021.06.003
40. Wang X, Cai B, Yang X, Sonubi OO, Zheng Z, Ramakrishnan R, et al. Cholesterol stabilizes TAZ in hepatocytes to promote experimental non-alcoholic steatohepatitis. Cell Metab. (2020) 31:969–86.e7. doi: 10.1016/j.cmet.2020.03.010
41. Varbo A, Benn M, Tybjærg-Hansen A, Nordestgaard BG. Elevated remnant cholesterol causes both low-grade inflammation and ischemic heart disease, whereas elevated low-density lipoprotein cholesterol causes ischemic heart disease without inflammation. Circulation. (2013) 128:1298–309. doi: 10.1161/CIRCULATIONAHA.113.003008
42. Elías-López D, Doi T, Nordestgaard BG, Kobylecki CJ. Remnant cholesterol and low-grade inflammation jointly in atherosclerotic cardiovascular disease: implications for clinical trials. Curr Opin Clin Nutr Metab Care. (2024) 27:125–35. doi: 10.1097/MCO.0000000000000999
43. Dushkin MI. Macrophage/foam cell is an attribute of inflammation: mechanisms of formation and functional role. Biochem (Mosc). (2012) 77:327–38. doi: 10.1134/S0006297912040025
44. Yu D, Wang Z, Zhang X, Qu B, Cai Y, Ma S, et al. Remnant cholesterol and cardiovascular mortality in patients with type 2 diabetes and incident diabetic nephropathy. J Clin Endocrinol Metab. (2021) 106:3546–54. doi: 10.1210/clinem/dgab533
45. Zhao JL, Zeng LF, Liang GH, Dou YX, Zhou GH, Pan JK, et al. Higher systemic immune-inflammation index is associated with sarcopenia in individuals aged 18-59 years: a population-based study. Sci Rep-Uk. (2023) 13. doi: 10.1038/s41598-023-49658-1
46. Bautmans I, Van Puyvelde K, Mets T. Sarcopenia and functional decline: pathophysiology, prevention and therapy. Acta Clin Belg. (2009) 64:303–16. doi: 10.1179/acb.2009.048
47. Vekic J, Stromsnes K, Mazzalai S, Zeljkovic A, Rizzo M, Gambini J. Oxidative stress, atherogenic dyslipidemia, and cardiovascular risk. Biomedicines. (2023) 11. doi: 10.3390/biomedicines11112897
48. Wang L, Gill R, Pedersen TL, Higgins LJ, Newman JW, Rutledge JC. Triglyceride-rich lipoprotein lipolysis releases neutral and oxidized FFAs that induce endothelial cell inflammation. J Lipid Res. (2009) 50:204–13. doi: 10.1194/jlr.M700505-JLR200
49. Varbo A, Nordestgaard BG. Directly measured vs. calculated remnant cholesterol identifies additional overlooked individuals in the general population at higher risk of myocardial infarction. Eur Heart J. (2021) 42:4833–43. doi: 10.1093/eurheartj/ehab293
Keywords: remnant cholesterol, dyslipidemia, sarcopenia, muscle mass, triglyceride-rich lipoproteins
Citation: Jang SY, Hwang S-Y, Jang A, Kim KJ, Yu JH, Kim NH, Yoo HJ, Kim NH, Baik SH and Choi KM (2024) Association of remnant cholesterol with sarcopenia in Korean adults: a nationwide population-based study using data from the KNHANES. Front. Endocrinol. 15:1391733. doi: 10.3389/fendo.2024.1391733
Received: 26 February 2024; Accepted: 05 August 2024;
Published: 23 August 2024.
Edited by:
Mei Dong, Qilu Hospital of Shandong University, ChinaReviewed by:
Yunlong Yang, Fudan University, ChinaClaudia Loreti, Agostino Gemelli University Polyclinic (IRCCS), Italy
Copyright © 2024 Jang, Hwang, Jang, Kim, Yu, Kim, Yoo, Kim, Baik and Choi. This is an open-access article distributed under the terms of the Creative Commons Attribution License (CC BY). The use, distribution or reproduction in other forums is permitted, provided the original author(s) and the copyright owner(s) are credited and that the original publication in this journal is cited, in accordance with accepted academic practice. No use, distribution or reproduction is permitted which does not comply with these terms.
*Correspondence: Kyung Mook Choi, bWVkaWNhN0BnbWFpbC5jb20=