- 1Research Department, Weill Cornell Medicine-Qatar, Doha, Qatar
- 2Endocrinology Department, Sidra Medicine, Doha, Qatar
- 3College of Health & Life Sciences, Hamad Bin Khalifa University, Doha, Qatar
- 4Institute of Cardiovascular Medicine, University of Manchester, Manchester, United Kingdom
Melanocortin 4 receptor (MC4R) mutations are the commonest cause of monogenic obesity through dysregulation of neuronal pathways in the hypothalamus and prefrontal cortex that regulate hunger and satiety. MC4R also regulates neuropathic pain pathways via JNK signaling after nerve injury. We show evidence of corneal small fiber degeneration in 2 siblings carrying a heterozygous missense variant c.508A>G, p.Ille170Val in the MC4R gene. Both children were treated with once weekly semaglutide for 6 months with no change in weight, and only a minor improvement in HbA1c and lipid profile. However, there was evidence of nerve regeneration with an increase in corneal nerve fiber density (CNFD) [child A (13.9%), child B (14.7%)], corneal nerve branch density (CNBD) [child A (110.2%), child B (58.7%)] and corneal nerve fiber length (CNFL) [child A (21.5%), child B (44.0%)].
Introduction
Obesity is a multifactorial disease due to genetic predisposition and environmental factors. Whilst polygenic variants are frequent, they confer small effect sizes, whilst rare pathogenic variants in single genes with large effect sizes account for ~5% of pediatric obesity (1). Clinically, patients with monogenic obesity present with impaired satiety and hyperphagia in early childhood with severe early-onset obesity due to dysregulation of the central leptin-melanocortin neuronal pathways (2, 3). We have recently characterized two novel homozygous variants that yielded antagonistic proteins to leptin receptor activation resulting in intense hyperphagia and severe obesity in one child in Qatar (4). We have also identified pathogenic variants in around 14.8% of 243 individuals with early-onset obesity, in whom variants in the MC4R gene accounted for 19% (2), far higher than other studies (0.5–8.5%) (5). MC4R is expressed on neurons in the hypothalamus and prefrontal cortex, which regulate hunger and satiety (6, 7).
Lifestyle interventions in patients with MC4R mutations have shown minimal benefit (8–10) and even bariatric surgery has shown limited long-term benefit (11–13). Targeted agonism of the MC4R with setmelanotide showed ~10% body weight loss in patients with POMC deficiency and LEPR deficiency (14), and there are case reports showing weight loss after GLP-1 therapy in individuals with pathogenic variants of MC4R (15–17). A recent case report of a 13-year-old female with a heterozygous c.206T>G(p.I69R) variant in the MC4R gene showed that treatment with liraglutide achieved a 19.2% reduction in body weight at 32 weeks, but significant gastrointestinal side-effects led to cessation of treatment and a return to her former weight within 13 weeks (18).
GLP-1 receptor agonism leads to improvements in weight, blood pressure, lipids with a decrease in reactive oxygen species (ROS) and inflammation which could impact on neurons (19). Indeed, GLP-1R-mediated ERK-signaling in diabetic rodents has been shown to protect large motor fiber function and small fiber structure by a mechanism independent of glycemic control (20). We have previously shown corneal nerve regeneration after bariatric surgery (21) and after treatment with the once weekly GLP-1 agonist exenatide (22). More recently, in 14 patients with type 2 diabetes, treatment with semaglutide or dulaglutide improved nerve area and sural nerve amplitude (23). We have now undertaken corneal confocal microscopy to assess for evidence of nerve regeneration following treatment with the once weekly GLP-1 RA semaglutide, in two siblings with MC4R gene mutation.
Methods
Two siblings, a 10-year-old boy (patient A) and an 8-year-old girl (patient B) with intense hyperphagia, impaired satiety, and severe, early onset obesity and a 10-year-old healthy boy were studied.
Anthropometry
Weight (kg) was measured using the body composition analyzer (TANITA DC-430MAIII) and height (cm) using the stadiometer (SECA model), both were recorded to the nearest 0.1 g or cm, respectively (24). The cut-off points to classify weight status were established using the International Obesity Task Force (IOFT) (25) and World health Organization (WHO) growth chart (26). BMI is a poor predictor of adiposity due to the indirect relationship to fat content (27), and we have therefore additionally assessed body composition using the TANITA scale to derive body fat percent (BF%) and fat mass (kg).
Cardiometabolic panel assessments
Glycated hemoglobin (HbA1c), total cholesterol (TC), LDL cholesterol (LDL-C), HDL cholesterol (HDL-C), and triglycerides (TG) were assessed.
Neuropathy and neuropathic pain assessments
Vibration perception threshold
The stimulator was applied on the pulp of both big toes, and the stimulus strength increased slowly from zero until the vibration was first perceived by indicating “yes”. Vibration sensation was recorded as an average for both feet in volts (28). A VPT of ≥ 15V was considered to be impaired vibration perception (29).
Monofilament
A 10 g monofilament (Semmes-Weinstein monofilament) was applied with a sufficient force to cause the filament to bend at a total of 9 sites per foot, on both feet. Loss of protective sensation was recorded as “no feeling in ≥ 8 sites” (30).
Corneal confocal microscopy
Corneal confocal microscopy was undertaken in all three children using the Heidelberg Retina Tomograph III Rostock Cornea Module (Heidelberg Engineering, Heidelberg, Germany). Both eyes were anaesthetized with 2 drops of Bausch & Lomb Minims ® (Oxybuprocaine hydrochloride 0.4% w/v). A drop of hypotears gel (Carbomer 0.2% eye gel) was placed on the tip of the objective lens and a sterile disposable TomoCap was placed over the lens, allowing optical coupling of the objective lens to the cornea. Six images were selected from the sub basal nerve plexus (SBNP) in the central cornea and corneal nerve fiber density (CNFD) (fibers/mm2) corneal nerve branch density (CNBD) (branches/mm2), and corneal nerve fiber length (CNFL) (mm/mm2) were quantified manually using CCMetrics. The investigator was blind to the study group when analyzing the CCM images.
Douleur neuropathique en 4 questionnaire
Neuropathic pain was assessed using the Douleur Neuropathique en 4 (DN4) questionnaire which can distinguish neuropathic from non-neuropathic pain. The diagnosis of painful neuropathy was based on a DN4 questionnaire score of ≥4, which has a high sensitivity (80%) and specificity (92%) for diagnosing painful diabetic neuropathy in adults (31).
Results
Clinical demographics of the cases are described in Table 1. Child A weighed 100.3kg and child B weighed 58.6kg. Both siblings carry a heterozygous missense variant c.508A>G, p.Ille170Val in the MC4R gene, a rare variant with Minor allele frequency (MAF) in gnomAD of 0.00009. The variant is predicted to have PM1/PM2/PP3/PP5/BS2 classes, which is classified as a pathogenic variant according to the ACMG variant classification. It is located in the transmembrane helix of the MC4R gene and is known to impair cyclic-AMP, leading to severe obesity (32, 33).
GLP-1 treatment
Both children with obesity were treated with once weekly semaglutide 0.5mg for 1 month and then 1.0 mg once weekly for 5 months.
Clinical/anthropometric and metabolic variables
Body weight increased in child A (0.6%) and decreased in child B (-2.0%). BMI decreased in child A (-6.5%) and child B (-11.5%) due to an increase in height (child A +3.8% and child B +5.2%). Whereas percentage body fat (6.8%) and fat mass (7.3%) increased in child A and percentage body fat (-10.7%) and fat mass (-12.5%) decreased in child B (Table 1). There were reduction in HbA1c in child A (-5.2%) and child B (-3.6%), total cholesterol decreased in child A (-13.6%) and increased in child B (5.9%), LDL did not change in child A (0%) and increased in child B (5.9%), HDL decreased in child A (-28.6%) and increased in child B (30.0%), whilst triglycerides decreased in both child A (-20.0%) and child B (-14.3%).
Neuropathy measures
Vibration perception threshold was normal in child A (3.0V) and child B (1.5V) and decreased in child A (1.8V, -40%) but increased in child B (5.5V, +266%). Sensation to the monofilament at all 8 sites on the foot was normal at baseline and did not change at follow up. Both children scored zero for the DN4 questionnaire at baseline and after treatment.
Both siblings had a lower CNFD (child A-30.2 fiber/mm2, child B-35.4 fiber/mm2 vs. HC 39.6 fiber/mm2), CNBD (child A-31.2 branch/mm2, child B-35.4 branch/mm2 vs. HC-73.9 branch/mm2), and CNFL (child A-18.6 mm/mm2, child B-19.3 mm/mm2 vs. HC 26.53 mm/mm2) compared to the healthy control (Figures 1A, B, D). After 6 months of treatment with semaglutide, there was evidence of nerve regeneration (Figures 1A–E) with an increase in CNFD [child A (13.9%), child B (14.7%)] (Figure 2A), CNBD [child A (110.2%), child B (58.7%)] (Figure 2B) and CNFL [child A (21.5%), child B (44.0%)] (Figure 2C).
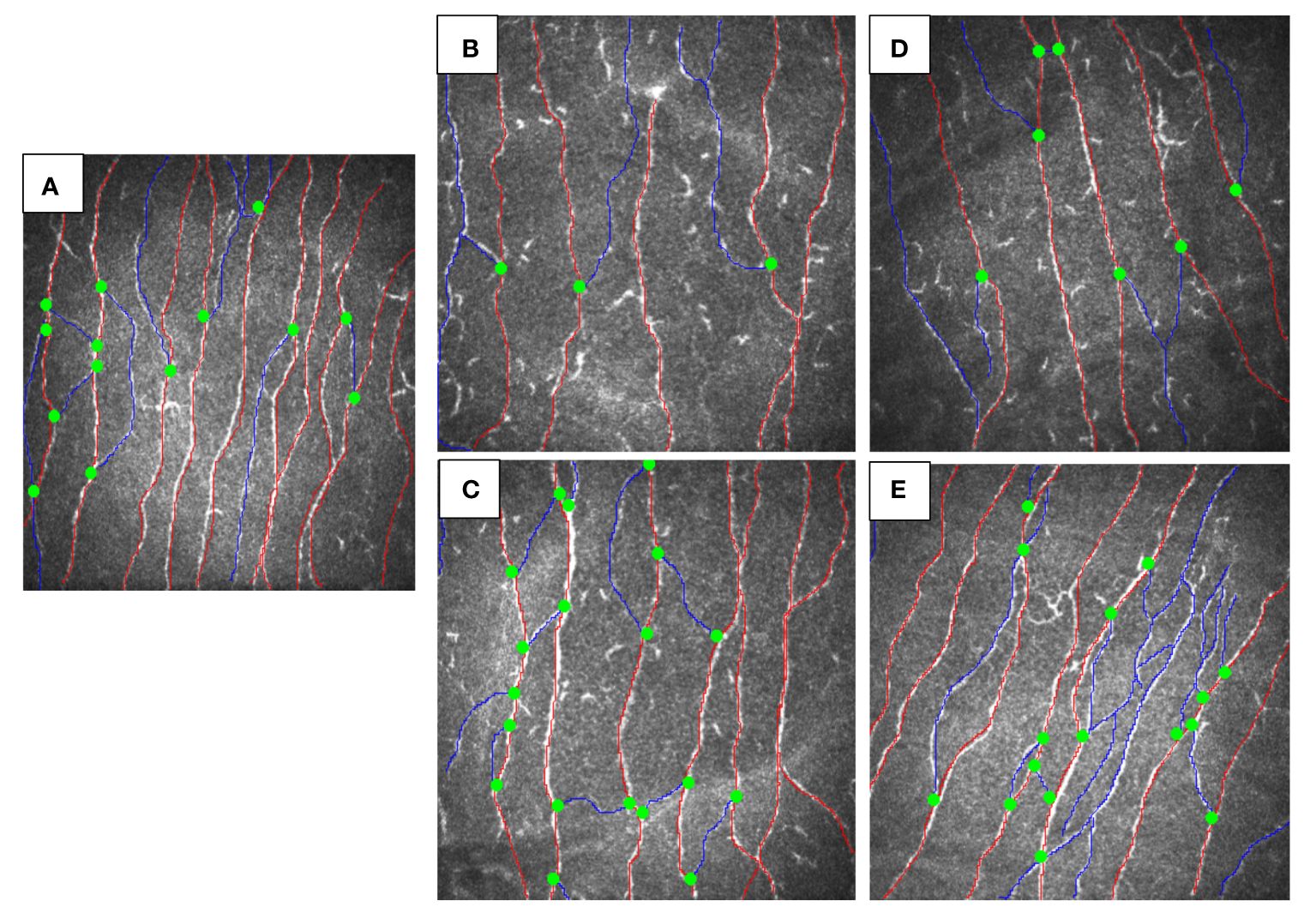
Figure 1 CCM images of siblings with MC4R mutation before and after treatment vs. healthy control. (A) healthy control, (B) child A before treatment, (C) child A after 6-months treatment with GLP-1, (D) child B before treatment, (E) child B after 6-months treatment with semaglutide.
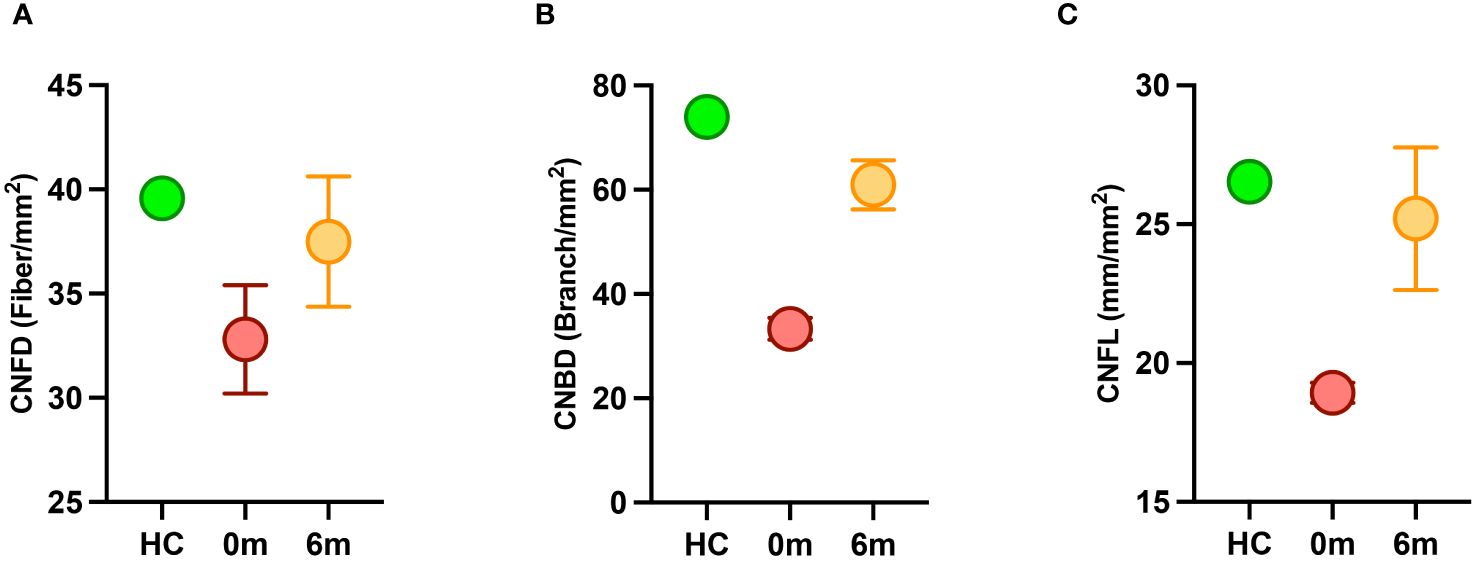
Figure 2 Corneal nerve parameters in children with MC4R mutation before (red) and after (orange) treatment with semaglutide compared to a healthy control (HC) (green). (A) CNFD, corneal nerve fiber density; (B) CNBD, corneal nerve branch density; (C) CNFL, corneal nerve fiber length.
Discussion
This study shows evidence of sub-clinical nerve degeneration with regeneration following treatment with the GLP-1 agonist semaglutide in two children with a MC4R gene mutation and severe obesity. First, we show evidence of corneal small nerve fiber degeneration, with preserved vibration perception and sensation to the 10g monofilament, and normal DN4 indicative of subclinical neurodegeneration, detected with corneal confocal microscopy. Clinical neuropathy has been reported in a 27-year old male with a MC4R gene mutation and morbid obesity, but this was attributed to the presence of T2DM (34).
We also show that 6 months of treatment with semaglutide was associated with small nerve fiber regeneration, but with a no major effect on weight, HbA1c and lipids, arguing for alternate mechanisms beyond an improvement in weight and glycemia as a basis for nerve regeneration in these two children with MC4R gene mutation. Obesity perse is a risk factor for small fiber neuropathy (35, 36) and we have previously shown nerve regeneration with an improvement in weight and metabolic parameters after bariatric surgery (37, 38). Furthermore, GLP-1 receptor agonists reduce weight and improve many of the risk factors for neuropathy including hyperglycemia, blood pressure and hyperlipidemia (39). Indeed, previous case reports with the daily GLP-1 agonist liraglutide have shown a reduction in weight and improvement in glycemia (15–17). However, in our recent cohort study we showed no major impact of liraglutide on weight or HbA1c in children with obesity (40).
There is a body of evidence that MC4R mediates neuropathic (41) and inflammatory pain (42) and the MC4R antagonist HS014 has been shown to increase paw withdrawal threshold and heat withdrawal latency in rat models of neuropathic pain (43). In the present study there was no evidence of neuropathic pain based on the DN4 score in children with MC4R mutation and it did not change with semaglutide. DN4 has not been validated in children with obesity, but it has been used to assess neuropathic pain in children with leprosy-related neuropathic pain (44) and sickle cell disease (45).
With regard to alternate mechanisms for nerve regeneration, sirtuin-1 (SIRT-1) inactivation has been implicated in obesity and neurodegeneration and SIRT-1 activation is associated with nerve regeneration following peripheral nerve injury (46, 47). Of note, GLP-1 therapies are SIRT-1 activators (48, 49) which may be associated with the nerve regeneration observed in our patients, independent of change in weight and glycemia. Additionally, GLP-1 receptors are expressed in the dorsal root ganglion and peripheral nerves (50–53) and in a T1DM animal model, GLP-1 treatment led to intraepidermal nerve fiber regeneration without a change in weight or glucose (20). Furthermore, previously, in adults with T2DM treated with once weekly exenatide and pioglitazone, we showed evidence of small nerve fiber regeneration, despite an increase in weight (22).
We acknowledge this is a study of only two children with the MC4R mutation with a limited duration of follow up after treatment with semaglutide. However, we believe that our study provides novel insights into the complications associated with MC4R gene mutation as evidenced by subclinical neurodegeneration. Furthermore, we show nerve regeneration after treatment with semaglutide, without an improvement in weight or glycemia, indicating an independent effect of GLP-1 therapy, which merits further study.
Data availability statement
The original contributions presented in the study are included in the article/supplementary materials, further inquiries can be directed to the corresponding author/s.
Ethics statement
The studies involving humans were approved by Sidra Medicine and WCM-Q IRB committees. The studies were conducted in accordance with the local legislation and institutional requirements. Written informed consent for participation in this study was provided by the participants’ legal guardians/next of kin. Written informed consent was obtained from the individual(s), and minor(s)’ legal guardian/next of kin, for the publication of any potentially identifiable images or data included in this article.
Author contributions
HG: Writing – original draft, Project administration, Methodology, Investigation, Formal analysis, Data curation, Conceptualization. IM: Writing – review & editing, Data curation. HD: Writing – review & editing, Data curation. MP: Writing – review & editing, Data curation. TA: Writing – review & editing, Data curation. KH: Writing – review & editing, Supervision. RM: Writing – review & editing, Supervision.
Funding
The author(s) declare that no financial support was received for the research, authorship, and/or publication of this article.
Conflict of interest
The authors declare that the research was conducted in the absence of any commercial or financial relationships that could be construed as a potential conflict of interest.
Publisher’s note
All claims expressed in this article are solely those of the authors and do not necessarily represent those of their affiliated organizations, or those of the publisher, the editors and the reviewers. Any product that may be evaluated in this article, or claim that may be made by its manufacturer, is not guaranteed or endorsed by the publisher.
References
1. El-Sayed Moustafa JS, Froguel P. From obesity genetics to the future of personalized obesity therapy. Nat Rev Endocrinol. (2013) 9:402–13. doi: 10.1038/nrendo.2013.57
2. Mohammed I, Haris B, Al-Barazenji T, Vasudeva D, Tomei S, Al Azwani I, et al. Understanding the genetics of early-onset obesity in a cohort of children from Qatar. J Clin Endocrinol Metab. (2023) 108:3201–13. doi: 10.1210/clinem/dgad366
3. Huvenne H, Dubern B, Clement K, Poitou C. Rare genetic forms of obesity: Clinical approach and current treatments in 2016. Obes Facts. (2016) 9:158–73. doi: 10.1159/000445061
4. Funcke JB, Moepps B, Roos J, von Schnurbein J, Verstraete K, Fröhlich-Reiterer E, et al. Rare antagonistic leptin variants and severe, early-onset obesity. N Engl J Med. (2023) 388:2253–61. doi: 10.1056/NEJMoa2204041
5. Aykut A, Ozen S, Goksen D, Ata A, Onay H, Atik T, et al. Melanocortin 4 receptor (MC4R) gene variants in children and adolescents having familial early-onset obesity: genetic and clinical characteristics. Eur J Pediatr. (2020) 179:1445–52. doi: 10.1007/s00431-020-03630-7
6. Farooqi IS. Monogenic human obesity syndromes. Handb Clin Neurol. (2021) 181:301–10. doi: 10.1016/B978-0-12-820683-6.00022-1
7. Ross RA, Kim A, Das P, Li Y, Choi YK, Thompson AT, et al. Prefrontal cortex melanocortin 4 receptors (MC4R) mediate food intake behavior in male mice. Physiol Behav. (2023) 269:114280. doi: 10.1016/j.physbeh.2023.114280
8. Reinehr T, Hebebrand J, Friedel S, Toschke AM, Brumm H, Biebermann H, et al. Lifestyle intervention in obese children with variations in the melanocortin 4 receptor gene. Obes (Silver Spring). (2009) 17:382–9. doi: 10.1038/oby.2008.422
9. Hainerova I, Larsen LH, Holst B, Finkova M, Hainer V, Lebl J, et al. Melanocortin 4 receptor mutations in obese Czech children: studies of prevalence, phenotype development, weight reduction response, and functional analysis. J Clin Endocrinol Metab. (2007) 92:3689–96. doi: 10.1210/jc.2007-0352
10. Trier C, Hollensted M, Schnurr TM, Lund MAV, Nielsen TRH, Rui G, et al. Obesity treatment effect in Danish children and adolescents carrying Melanocortin-4 Receptor mutations. Int J Obes (Lond). (2021) 45:66–76. doi: 10.1038/s41366-020-00673-6
11. Vos N, Oussaada SM, Cooiman MI, Kleinendorst L, Ter Horst KW, Hazebroek EJ, et al. Bariatric surgery for monogenic non-syndromic and syndromic obesity disorders. Curr Diabetes Rep. (2020) 20:44. doi: 10.1007/s11892-020-01327-7
12. Poitou C, Puder L, Dubern B, Krabusch P, Genser L, Wiegand S, et al. Long-term outcomes of bariatric surgery in patients with bi-allelic mutations in the POMC, LEPR, and MC4R genes. Surg Obes Relat Dis. (2021) 17:1449–56. doi: 10.1016/j.soard.2021.04.020
13. Cooiman MI, Alsters SIM, Duquesnoy M, Hazebroek EJ, Meijers-Heijboer HJ, Chahal H, et al. Long-term weight outcome after bariatric surgery in patients with melanocortin-4 receptor gene variants: a case-control study of 105 patients. Obes Surg. (2022) 32:837–44. doi: 10.1007/s11695-021-05869-x
14. Clement K, van den Akker E, Argente J, Bahm A, Chung WK, Connors H, et al. Efficacy and safety of setmelanotide, an MC4R agonist, in individuals with severe obesity due to LEPR or POMC deficiency: single-arm, open-label, multicenter, phase 3 trials. Lancet Diabetes Endocrinol. (2020) 8:960–70. doi: 10.1016/S2213-8587(20)30364-8
15. Iepsen EW, Zhang J, Thomsen HS, Hansen EL, Hollensted M, Madsbad S, et al. Patients with obesity caused by melanocortin-4 receptor mutations can be treated with a glucagon-like peptide-1 receptor agonist. Cell Metab. (2018) 28:23–32.e3. doi: 10.1016/j.cmet.2018.05.008
16. Iepsen EW, Have CT, Veedfald S, Madsbad S, Holst JJ, Grarup N, et al. GLP-1 receptor agonist treatment in morbid obesity and type 2 diabetes due to pathogenic homozygous melanocortin-4 receptor mutation: A case report. Cell Rep Med. (2020) 1:100006. doi: 10.1016/j.xcrm.2020.100006
17. Welling MS, de Groot CJ, Kleinendorst L, van der Voorn B, Burgerhart JS, van der Valk ES, et al. Effects of glucagon-like peptide-1 analogue treatment in genetic obesity: A case series. Clin Obes. (2021) 11:e12481. doi: 10.1111/cob.12481
18. Camtosun E, Akinci A, Kayas L, Ciftci N, Tekedereli I. Liraglutide treatment in a morbidly obese adolescent with a MC4R gene variant: Side effects reduce success. J Clin Res Pediatr Endocrinol. (2023) 15:225–9. doi: 10.4274/jcrpe
19. Goldney J, Sargeant JA, Davies MJ. Incretins and microvascular complications of diabetes: neuropathy, nephropathy, retinopathy and microangiopathy. Diabetologia. (2023) 66:1832–45. doi: 10.1007/s00125-023-05988-3
20. Jolivalt CG, Fineman M, Deacon CF, Carr RD, Calcutt NA. GLP-1 signals via ERK in peripheral nerve and prevents nerve dysfunction in diabetic mice. Diabetes Obes Metab. (2011) 13:990–1000. doi: 10.1111/j.1463-1326.2011.01431.x
21. Adam S, Azmi S, Ho JH, Liu Y, Ferdousi M, Siahmansur T, et al. Improvements in diabetic neuropathy and nephropathy after bariatric surgery: a prospective cohort study. Obes Surg. (2021) 31:554–63. doi: 10.1007/s11695-020-05052-8
22. Ponirakis G, Abdul-Ghani MA, Jayyousi A, Almuhannadi H, Petropoulos IN, Khan A, et al. Effect of treatment with exenatide and pioglitazone or basal-bolus insulin on diabetic neuropathy: a substudy of the Qatar Study. BMJ Open Diabetes Res Care. (2020) 8():e001420. doi: 10.1136/bmjdrc-2020-001420
23. Dhanapalaratnam R, Issar T, Lee ATK, Poynten AM, Milner KL, Kwai NCG, et al. Glucagon-like peptide-1 receptor agonists reverse nerve morphological abnormalities in diabetic peripheral neuropathy. Diabetologia. (2024) 67:561–6. doi: 10.1007/s00125-023-06072-6
24. Cadenas-Sanchez C, Nystrom C, Sanchez-Delgado G, Martinez-Tellez B, Mora-Gonzalez J, Risinger AS, et al. Prevalence of overweight/obesity and fitness level in preschool children from the north compared with the south of Europe: an exploration with two countries. Pediatr Obes. (2016) 11:403–10. doi: 10.1111/ijpo.12079
25. Cole TJ, Lobstein T. Extended international (IOTF) body mass index cut-offs for thinness, overweight and obesity. Pediatr Obes. (2012) 7:284–94. doi: 10.1111/j.2047-6310.2012.00064.x
26. Group WHOMGRS. WHO Child Growth Standards based on length/height, weight and age. Acta Pediatr Suppl. (2006) 450:76–85. doi: 10.1111/j.1651-2227.2006.tb02378.x
27. Vanderwall C, Randall Clark R, Eickhoff J, Carrel AL. BMI is a poor predictor of adiposity in young overweight and obese children. BMC Pediatr. (2017) 17:135. doi: 10.1186/s12887-017-0891-z
28. Louraki M, Tsentidis C, Kallinikou D, Katsalouli M, Kanaka-Gantenbein C, Kafassi N, et al. Reproducibility of vibration perception threshold values in children and adolescents with type 1 diabetes mellitus and associated factors. Prim Care Diabetes. (2014) 8:147–57. doi: 10.1016/j.pcd.2013.11.002
29. Ponirakis G, Elhadd T, Chinnaiyan S, Dabbous Z, Siddiqui M, Al-Muhannadi H, et al. Prevalence and management of diabetic neuropathy in secondary care in Qatar. Diabetes Metab Res Rev. (2020) 36:e3286. doi: 10.1002/dmrr.3286
30. Booth J, Young MJ. Differences in the performance of commercially available 10-g monofilaments. Diabetes Care. (2000) 23:984–8. doi: 10.2337/diacare.23.7.984
31. Spallone V, Morganti R, D'Amato C, Greco C, Cacciotti L, Marfia GA. Validation of DN4 as a screening tool for neuropathic pain in painful diabetic polyneuropathy. Diabetes Med. (2012) 29:578–85. doi: 10.1111/j.1464-5491.2011.03500.x
32. Farooqi IS, Keogh JM, Yeo GS, Lank EJ, Cheetham T, O'Rahilly S. Clinical spectrum of obesity and mutations in the melanocortin 4 receptor gene. N Engl J Med. (2003) 348:1085–95. doi: 10.1056/NEJMoa022050
33. Hinney A, Hohmann S, Geller F, Vogel C, Hess C, Wermter AK, et al. Melanocortin-4 receptor gene: case-control study and transmission disequilibrium test confirm that functionally relevant mutations are compatible with a major gene effect for extreme obesity. J Clin Endocrinol Metab. (2003) 88:4258–67. doi: 10.1210/jc.2003-030233
34. Buchbinder S, Bartsch U, Muller M, Zorn M, Nawroth PP, Schilling T. A novel missense mutation T101N in the melanocortin-4 receptor gene associated with obesity. Genet Mol Res. (2011) 10:1042–9. doi: 10.4238/vol10-2gmr948
35. Herman RM, Brower JB, Stoddard DG, Casano AR, Targovnik JH, Herman JH, et al. Prevalence of somatic small fiber neuropathy in obesity. Int J Obes (Lond). (2007) 31:226–35. doi: 10.1038/sj.ijo.0803418
36. Johnson SA, Shouman K, Shelly S, Sandroni P, Berini SE, Dyck PJB, et al. Small fiber neuropathy incidence, prevalence, longitudinal impairments, and disability. Neurology. (2021) 97:e2236–e47. doi: 10.1212/WNL.0000000000012894
37. Azmi S, Ferdousi M, Liu Y, Adam S, Iqbal Z, Dhage S, et al. Bariatric surgery leads to an improvement in small nerve fiber damage in subjects with obesity. Int J Obes (Lond). (2021) 45:631–8. doi: 10.1038/s41366-020-00727-9
38. Iqbal Z, Kalteniece A, Ferdousi M, Adam S, D'Onofrio L, Ho JH, et al. Corneal keratocyte density and corneal nerves are reduced in patients with severe obesity and improve after bariatric surgery. Invest Ophthalmol Vis Sci. (2021) 62:20. doi: 10.1167/iovs.62.1.20
39. Wilding JPH, Batterham RL, Calanna S, Davies M, Van Gaal LF, Lingvay I, et al. Once-weekly semaglutide in adults with overweight or obesity. N Engl J Med. (2021) 384:989–1002. doi: 10.1056/NEJMoa2032183
40. Dauleh H, Pasha M, Gad H, Haris B, Petrovski G, Afyouni H, et al. Single-center experience of using liraglutide in adolescents with obesity +/- type 2 diabetes. Cureus. (2024) 16:e58720. doi: 10.7759/cureus.58720
41. Sharfman NM, Kelley LK, Secci ME, Gilpin NW. Melanocortin-4 receptor signaling in the central amygdala mediates chronic inflammatory pain effects on nociception. Neuropharmacology. (2022) 210:109032. doi: 10.1016/j.neuropharm.2022.109032
42. Li ZX, Liu BW, He ZG, Xiang HB. Melanocortin-4 receptor regulation of pain. Biochim Biophys Acta Mol Basis Dis. (2017) 1863:2515–22. doi: 10.1016/j.bbadis.2017.05.021
43. Shikdar N, Alghamdi F. Influence of selective melanocortin-4 receptor antagonist HS014 on hypersensitivity after nervous system injuries in a model of rat neuropathic pain: A narrative review of the literature. Cureus. (2021) 13:e17681. doi: 10.7759/cureus.17681
44. Morgan KJ, Anghelescu DL. A review of adult and pediatric neuropathic pain assessment tools. Clin J Pain. (2017) 33:844–52. doi: 10.1097/AJP.0000000000000476
45. Sigalla J, Duparc Alegria N, Le Roux E, Toumazi A, Thiollier AF, Holvoet L, et al. Neuropathic pain in children with sickle cell disease: The hidden side of the vaso-occlusive crisis. Children (Basel). (2021) 67:561–6. doi: 10.3390/children8020084
46. Romeo-Guitart D, Leiva-Rodriguez T, Fores J, Casas C. Improved motor nerve regeneration by SIRT1/hif1a-mediated autophagy. Cells. (2019) 16:e58720. doi: 10.3390/cells8111354
47. Chandrasekaran K, Salimian M, Konduru SR, Choi J, Kumar P, Long A, et al. Overexpression of Sirtuin 1 protein in neurons prevents and reverses experimental diabetic neuropathy. Brain. (2019) 142:3737–52. doi: 10.1093/brain/awz324
48. Bastien-Dionne PO, Valenti L, Kon N, Gu W, Buteau J. Glucagon-like peptide 1 inhibits the sirtuin deacetylase SirT1 to stimulate pancreatic beta-cell mass expansion. Diabetes. (2011) 60:3217–22. doi: 10.2337/db11-0101
49. Xiang J, Qin L, Zhong J, Xia N, Liang Y. GLP-1RA liraglutide and semaglutide improves obesity-induced muscle atrophy via SIRT1 pathway. Diabetes Metab Syndr Obes. (2023) 16:2433–46. doi: 10.2147/DMSO.S425642
50. Merchenthaler I, Lane M, Shughrue P. Distribution of pre-pro-glucagon and glucagon-like peptide-1 receptor messenger RNAs in the rat central nervous system. J Comp Neurol. (1999) 403:261–80. doi: 10.1002/(ISSN)1096-9861
51. Ban K, Noyan-Ashraf MH, Hoefer J, Bolz SS, Drucker DJ, Husain M. Cardioprotective and vasodilatory actions of glucagon-like peptide 1 receptor are mediated through both glucagon-like peptide 1 receptor-dependent and -independent pathways. Circulation. (2008) 117:2340–50. doi: 10.1161/CIRCULATIONAHA.107.739938
52. Vrang N, Larsen PJ. Preproglucagon derived peptides GLP-1, GLP-2 and oxyntomodulin in the CNS: role of peripherally secreted and centrally produced peptides. Prog Neurobiol. (2010) 92:442–62. doi: 10.1016/j.pneurobio.2010.07.003
Keywords: monogenic obesity, neurodegeneration, nerve regeneration, GLP-1 - glucagon-like peptide-1, semaglutide
Citation: Gad H, Mohammed I, Dauleh H, Pasha M, Al-Barazenji T, Hussain K and Malik RA (2024) Case report: Nerve fiber regeneration in children with melanocortin 4 receptor gene mutation related obesity treated with semaglutide. Front. Endocrinol. 15:1385463. doi: 10.3389/fendo.2024.1385463
Received: 12 February 2024; Accepted: 07 June 2024;
Published: 21 June 2024.
Edited by:
Shan Gao, Capital Medical University, ChinaReviewed by:
Ian James Martins, University of Western Australia, AustraliaSerhiy Nyankovskyy, Danylo Halytsky Lviv National Medical University, Ukraine
Copyright © 2024 Gad, Mohammed, Dauleh, Pasha, Al-Barazenji, Hussain and Malik. This is an open-access article distributed under the terms of the Creative Commons Attribution License (CC BY). The use, distribution or reproduction in other forums is permitted, provided the original author(s) and the copyright owner(s) are credited and that the original publication in this journal is cited, in accordance with accepted academic practice. No use, distribution or reproduction is permitted which does not comply with these terms.
*Correspondence: Rayaz A. Malik, ram2045@qatar-med.cornell.edu