- 1Department of Pharmacology, School of Basic Medical Sciences, Heilongjiang University of Chinese Medicine, Harbin, China
- 2Department of Typhoid, School of Basic Medical Sciences, Heilongjiang University of Chinese Medicine, Harbin, China
- 3Department of Synopsis of the Golden Chamber, School of Basic Medical Sciences, Heilongjiang University of Chinese Medicine, Harbin, China
As an important gas signaling molecule, hydrogen sulfide (H2S) affects multiple organ systems, including the nervous, cardiovascular, digestive, and genitourinary, reproductive systems. In particular, H2S not only regulates female reproductive function but also holds great promise in the treatment of male reproductive diseases and disorders, such as erectile dysfunction, prostate cancer, varicocele, and infertility. In this review, we summarize the relationship between H2S and male reproductive organs, including the penis, testis, prostate, vas deferens, and epididymis. As lower urinary tract symptoms have a significant impact on penile erection disorders, we also address the potential ameliorative effects of H2S in erectile dysfunction resulting from bladder disease. Additionally, we discuss the regulatory role of H2S in cavernous smooth muscle relaxation, which involves the NO/cGMP pathway, the RhoA/Rho-kinase pathway, and K+ channel activation. Recently, various compounds that can alleviate erectile dysfunction have been reported to be at least partly dependent on H2S. Therefore, understanding the role of H2S in the male reproductive system may help develop novel strategies for the clinical treatment of male reproductive system diseases.
1 Introduction
In recent years, the incidence of male reproductive system diseases has increased, attracting substantial attention from researchers worldwide. Male reproductive system diseases primarily affect the penis, testis, prostate, vas deferens, and epididymis (1). Various microorganisms, environmental factors, and long-term smoking or alcohol abuse may affect male reproductive function (2–5), leading to diseases such as erectile dysfunction (ED) (6), prostate cancer (PCa) (7), varicocele (8), and infertility (9). Studies have shown that oxidative stress is an important factor contributing to the occurrence and development of these diseases. However, owing to the complex pathogenesis of male reproductive system diseases, the corresponding treatment strategies are not well-established. Therefore, identifying precise targets is necessary to optimize the diagnosis and treatment of male reproductive system diseases.
H2S is the third major gas signaling molecule after nitric oxide (NO) and carbon monoxide (CO) and possesses strong antioxidant activity (10). It is involved in the regulation of important pathophysiological processes, including inflammation, oxidative stress, autophagy, and apoptosis, in the cardiovascular, nervous, and digestive systems (11, 12). In addition, recent studies have shown that H2S affects the reproductive system in both men and women. H2S regulates female reproductive function through K+ channels and various signaling pathways, including the ERK1/2/NF-κB and Nrf2 pathways (13), and exerts protective effects on the male reproductive system. It plays an important role in early spermatogenesis and late maturation of spermatogenic cells and may prevent damage to the reproductive system by promoting the proliferation of spermatogonia, regulating the corpus cavernosum (CC) of the penis, and mediating erection as well as other related functions (14–16). In this review, we summarize the relationship between H2S and the male reproductive system, discuss the pathological mechanisms of male reproductive system diseases, and propose novel strategies for the early diagnosis (17) and prompt treatment of these diseases.
2 Distribution of H2S synthases in the male reproductive system
Endogenous H2S is produced from L-cysteine (L-Cys) via desulfurization catalyzed by cystathionine γ-lyase (CSE) and cystathionine β-synthase (CBS) or from 3-mercaptopyruvate via 3-mercaptopyruvate sulfurtransferase (3-MPST) (18, 19). 3-mercaptopyruvate is derived from two sources as follows: L-Cys via cysteine aminotransferase (CAT) (CAT/3-MPST pathway) and D-cysteine via amino acid oxidase (DAO) (DAO/3-MPST pathway) (20). A study reported that H2S exhibited specificity to vascular smooth muscle (21), which may be related to differences in the mechanisms of H2S production. Given that endogenous H2S is synthesized through various pathways, the distribution of H2S synthases in various parts of male reproductive organs (Figure 1) may indicate the source and production mode of H2S.
Srilatha et al. (22) detected the presence of endogenous H2S in smooth muscle tissue homogenates from the CC of rabbits. Although they used rabbit CC and did not identify the exact source of H2S, their study was the first to show that H2S can be produced in the penis. Subsequently, d’Emmanuele et al. (23) evaluated the mRNA and protein expression of CBS and CSE in human corpus cavernosum (HCC) and validated that endogenous H2S was produced in HCC by the two enzymes. Immunohistochemical analysis showed that CSE was located in vascular smooth muscle cells (SMCs) in the penile artery, bundles of muscular tissue in HCC trabeculae, and peripheral nerves, whereas CBS was primarily located in bundles of muscular tissue in trabeculae. Furthermore, CBS and CSE have been found in human prostate tissue sections. CBS is mainly expressed in the glandular lumen and epithelial cells of the prostate, whereas CSE is distributed in the SMCs of the periacinar stroma (24, 25). These experimental results suggest that H2S is predominantly synthesized by CSE instead of CBS in male reproductive organs.
The tissue samples used in most existing studies have been derived from animals, such as rats and mice. However, the species and location of H2S synthases in animals are different from those in humans. In rats, all five H2S synthases, namely, CSE, CBS, CAT, DAO, and 3-MPST, are expressed in penile tissues at both mRNA and protein levels; however, CBS is not expressed in corpus cavernosum smooth muscle cells (CCSMCs) (26, 27). The mRNA expression of the five enzymes has been detected at two specific sites in rat prostate tissues (dorsolateral [PR-D] and ventral [PR-V]); however, these sites lack the protein expression of CSE and DAO. In particular, the expression of CBS is substantially higher in PR-V than in PR-D, the expression of 3-MPST is higher in PR-D than in PR-V, and the expression of CAT is similar between the two sites. Additionally, immunoreactivity of CBS, 3-MPST, and CAT has been observed primarily in rat glandular epithelial cells (19). In mice, CBS, CSE, and 3-MPST are primarily expressed in the CC (28). CSE is located in the endothelial cells of the cavern and vessels in the CC, with its expression being higher than that of CBS and 3-MPST. CBS is located in the cavernous subendothelial smooth muscle, vascular smooth muscle, and peripheral nerves, whereas CSE and 3-MPST are not found at these sites (29). A study showed that the expression of CSE in mouse prostate tissues substantially decreased with age, whereas that of CBS remained unaffected. Moreover, knockout of CSE reduced the production of H2S by approximately 80%, indicating that H2S is predominantly synthesized by CSE in the mouse prostate (30).
To the best of our knowledge, studies investigating the effects of H2S on the testis have used only experimental animal models; therefore, related data on human tissues are not yet available. In rats, CBS is abundant in Leydig and Sertoli cells, which can be observed in the interstitial space and basement membrane of seminiferous tubules, respectively. In addition, its expression is moderate in the immature reproductive cells of the peripheral region of the tubules but very low in the mature reproductive cells of the central region of the tubules. CSE is found in vascular walls in the interstitial space of the testis, Sertoli cells, and immature reproductive cells (31). CBS, CSE, and 3-MPST are expressed in the testis and germ cells in mice (32). Data on the vas deferens (VD) are scarce. A study on humans, rats, and mice showed that the expression of CSE and CBS was high in the luminal epithelium and smooth muscle of VD (33). Furthermore, the mRNA expression of CBS and CSE is high in the body and tail regions of rat epididymis (34). In particular, CBS is found in epididymal epithelial cells, whereas CSE is found in the thin layer of SMCs underneath the epididymal epithelium. These findings suggest that species-specific differences should be considered when using animals to investigate the effects of H2S on the reproductive system.
3 Effects of H2S on the male reproductive system
3.1 Effects of H2S on the penis
3.1.1 Regulation of mechanisms underlying penile erection
Erection is a neurovascular event whose form and function mostly depend on the relaxation of the cavernous smooth muscle and arteries in the penis. When parasympathetic nerves are stimulated to release neurotransmitters, the smooth muscle of HCC (a highly vascularized structure) and human penile resistance arteries relax. Subsequently, blood flows into the cavernous sinuses and inflates them, which in turn pressurizes the veins to reduce blood outflow and increases intracavernosal pressure (ICP), eventually leading to penile erection (35). Altogether, arterial blood flow exceeding venous blood return causes and maintains normal penile erection, with smooth muscle relaxation playing a key role in the process.
The neurotransmitter-like regulatory effects of H2S on vascular smooth muscle have been reported in previous studies (36). Srilatha et al. (37) were the first to demonstrate that H2S has a neuroregulatory effect on penile erection. They examined the effects of exogenous H2S on the penis by injecting sodium hydrosulfide (NaHS) into the CC of non-human primates. The results showed that both penile length and ICP were remarkably increased, and the changes before erection were similar to those observed in the positive control group (injected with prostaglandin E1). Furthermore, injection of DL-propargyl glycine (PAG, a CSE inhibitor) into the external jugular vein of rats notably weakened the ICP induced by electrical stimulation of the cavernous nerve with platinum wire electrodes. These findings suggest that neuronal excitation induces the release of H2S to mediate penile erection. The detection of CSE in the human peripheral penile nerve supports these findings (23). In addition, Jupiter et al. (38) demonstrated that exogenous H2S promoted penile erection in rats.
At present, the exact mechanism through which H2S relaxes the cavernous smooth muscle remains unclear. The previously reported mechanisms include synergistic action of H2S with NO, activation of K+ channels, and regulation of the RhoA/ROCK pathway (Table 1); among which, the first mechanism is the most controversial. NO is considered the primary mediator of erectile function (56). Endogenous NO is generated by NO synthase (NOS) from L-arginine and consists of three isoforms as follows: neuronal NOS (nNOS), endothelial NOS (eNOS), and inducible NOS (iNOS) (57). NO increases cGMP levels by activating soluble guanylate cyclase (sGC); subsequently, cGMP acts as a second intracellular messenger to regulate calcium channels and contractile proteins involved in the relaxation of cavernous smooth muscle (58). As early as 1997, Hosoki et al. (59) proposed the possibility of synergism between H2S and NO. They found that 100-μM NaHS had a weak effect on relaxing thoracic aortic smooth muscle in rats; however, when administered in the presence of 10-nM sodium nitroprusside (SNP, an NO donor), NaHS strongly relaxed the smooth muscle. Similarly, in the presence of 30-μM NaHS, SNP increased the relaxation of thoracic aortic smooth muscle by 13 times.
On the contrary, the results of some studies on CC do not support the synergism between NO and H2S. Srilatha et al. (22) incubated noradrenaline-precontracted rabbit cavernosum tissue strips with nω-nitro-L-arginine (L-NAME, a non-selective NO synthase inhibitor) and subsequently treated them with NaHS (100 μM–3.2 mM). The results showed that muscle relaxation induced by NaHS was not affected by L-NAME. Furthermore, the strips were precontracted in the presence of guanethidine and atropine and incubated with aminooxyacetic acid (AOAA, a CBS inhibitor) and β-cyanoalanine (β-CA, a CSE inhibitor) or PAG, respectively. After electrical stimulation, H2S inhibitors did not affect non-adrenergic, non-cholinergic nitrergic (NANC) relaxation. Similarly, L-NAME had minimal inhibitory effects on muscle relaxation induced by NaHS (1 μM–10 mM) (23). A study on live rats showed that intracavernosal injection of sodium sulfide (Na2S, 0.03–1 mg/kg) increased ICP, whereas intravenous injection of L-NAME had no considerable effect on ICP (38). Moreover, intracavernosal injection of Na2S did not alter the SNP-induced erectile response. In mouse CC, NO deficiency may increase the expression of CSE and 3-MPST, leading to an increase in the production of H2S and H2S-induced muscle relaxation (28). This effect may be compensatory and disproves that the relaxation effects of H2S are dependent on NO. Altogether, the abovementioned findings indicate that H2S does not promote penile erection through synergism with NO.
Some studies investigating the relationship between H2S and NO in the CC tissue support the synergy between them. H2S has been shown to increase the expression of NOS. Meng et al. (39) showed that the mRNA and protein levels of eNOS were remarkably higher in rat CC tissues treated with NaHS than in untreated tissues, whereas those of nNOS were not considerably different between treated and untreated tissues. Yilmaz et al. (42) showed that L-NAME decreased the protein expression of eNOS and nNOS in the penile tissues of hypertensive rats, whereas addition of NaHS (0.037 mg/kg) prevented this change. Consistent with these two studies, another study showed that NaHS (30 mg/kg) increased NO levels in rat CC tissues (43). Furthermore, H2S has been shown to increase the activity of eNOS. Meng et al. (39) showed that eNOS notably increased the production of NO from L-arginine by 5 times in more than rat CC tissues treated with NaHS (1 mM), suggesting that H2S enhanced the activity of eNOS. Knockout of CSE may not alter total eNOS levels but may remarkably reduce the levels of its active form p-eNOS (16).
These contradictory findings suggest that tissue- and species-specific crosstalk exists between H2S and NO and the mechanism through which H2S relaxes the cavernous smooth muscle is independent of the NO/cGMP signaling pathway. Endogenous H2S can inhibit the targeted degradation of cGMP by inhibiting phosphodiesterase (PDE) (60). Several studies have demonstrated that H2S in the penis regulates cGMP levels by acting on sGC. Stimulation of HCC with both endogenous and exogenous H2S (L-Cys [1 μM–1 mM] and NaHS [1 μM–1 mM], respectively) increases cGMP levels, which may be restored upon treatment with 1H-[1,2,4]oxadiazolo[4,3-a] quinoxalin-1-one (ODQ, an sGC inhibitor) (61). The reduction of H2S levels in CSE-knockout mice results in an impaired redox state of sGC, decreasing cGMP levels in the penis (16). These findings suggest that H2S bypasses NO to upregulate cGMP and hence affects its downstream signaling.
The four primary types of potassium (K+) channels expressed in arterial smooth muscle cells include Ca2+-activated (KCa), adenosine triphosphate (ATP)-sensitive (KATP), inwardly rectifying (Kir), and voltage-gated (Kv) channels (62). The relaxation effects of H2S on vascular SMCs isolated from rats have been shown to rely on the KATP channel (21). The four K+ channels have also been detected in HCC (63). To examine the role of these K+ channels in H2S-mediated responses in CC, Jupiter et al. (38) injected tetraethylammonium (TEA, a non-selective K+ channel inhibitor), iberiotoxin (a large-conductance Ca2+-activated K+ [BKCa] channel inhibitor), and glybenclamide (GLB, a KATP channel inhibitor) into the CC of anesthetized rats and examined their effects on Na2S (0.03–1 mg/kg)-induced changes in ICP. The results showed that intracavernosal injection of Na2S induced an increase in ICP, which was attenuated by TEA and iberiotoxin but not by GLB. In an organ bath experiment, Abd Elmoneim et al. (41) treated rat CC tissues with TEA, GLB, 4-aminopyridine (4-AP, a Kv channel inhibitor), and barium chloride (BaCl2, a Kir channel inhibitor) to examine their effects on relaxation induced by L-Cys (1 μM–10 mM). The results showed that TEA and 4-AP remarkably attenuated relaxation, with the effects of TEA being stronger than those of 4-AP, whereas GLB and BaCl2 failed to reduce relaxation. These findings indicate that BKCa and Kv channels are involved in the H2S-induced relaxation of rat CC, whereas Kir and KATP channels may not participate in the process. In another study, GLB was found to attenuate the relaxation effects of NaHS on HCC strips (23); however, the dose of GLB used was 150 μM. When administered at a dose of >10 μM, GLB inhibits the Na+–K+ pump and L-type Ca2+ channel (64), which may interfere with the results. Consistently, studies have shown that treatment with GLB (10 μM) and NaHS does not suppress relaxation in rat (26, 41) or human (61) cavernosal tissue strips.
RhoA is a monomeric GTP enzyme that is activated upon binding to GTP, subsequently stimulating ROCK (a serine/threonine kinase) (65). ROCK phosphorylates the myosin-binding subunit of myosin light chain (MLC) phosphatase, which is responsible for the dephosphorylation of MLC, to inactivate the enzyme (66). In addition, it directly phosphorylates MLC, causing myosin to bind to β-actin, which promotes smooth muscle contraction (67). Chitaley et al. (68) used (R)-(+)-trans-N-(4-pyridyl)-4-(1-aminoethyl)-cyclohexanecarboxamide (Y-27632, a specific ROCK inhibitor) to examine the effects of ROCK on the cavernosal tone in rats. They found that inhibition of ROCK induced an increase in ICP, which stimulated penile erection in rats. This effect was found to be independent of the NO pathway. d’Emmanuele et al. (23) found that the relaxation effects of NaHS (1 μM–10 mM) were considerably stronger in HCC strips precontracted with U46619 or human endothelin-1 (two ROCK pathway modulators) that in those precontracted with phenylephrine (PE). This finding indicates that H2S regulates the ROCK pathway. Consistently, another study showed that fasudil (a ROCK inhibitor) reduced the relaxation response of mouse CC to exogenous H2S, suggesting that an interaction between H2S and ROCK is highly likely (69). Aydinoglu et al. (40) were the first to report that ROCK participates in the relaxation-inducing effects of H2S in mouse CC contracted with PE. They found that pre-treatment with Y-27632 remarkably reduced cavernosal muscle contraction induced by the PE-driven phosphorylation of the myosin phosphatase-targeting subunit 1 (MYPT1) at Thr696. However, PE-induced muscle contraction almost disappeared in the presence of L-Cys or NaHS. Correspondingly, the combination of Y-27632 and L-Cys (10 mM) or NaHS (1 mM) inhibited the expression of phosphorylated MYPT1, whereas PAG and AOAA reversed this change. Furthermore, Y-27632 increased the basal and L-Cys-induced production of H2S, which was attenuated by PAG and AOAA. These findings indicate that ROCK at least partly inhibits CSE/CBS in CCSMCs. In addition to regulating the phosphorylation of MLC, the RhoA/ROCK signaling pathway affects the phenotypic modulation of CCSMCs by regulating the downstream factors CDK2, Cyclin E1, and P27kip1, thereby promoting smooth muscle contraction (44). A study showed that NaHS (100 μmol/kg) inhibited the phenotypic transformation of CCSMCs induced by the upregulation of RhoA/ROCK signaling, thereby improving erectile function in rat models of bilateral cavernous nerve injury (BCNI) (44).
In addition to playing an important role in the abovementioned contraction mechanisms, H2S may be involved in other less investigated pathways of CC relaxation, such as the CO/heme oxygenase-1 (HO-1) pathway (43). Notably, the H2S donor used in a majority of the abovementioned studies is NaHS, whose H2S release rate may not be sufficient to accurately mimic endogenous H2S production. As a slow-H2S-releasing donor, GYY4137 is more suitable for investigating the effects of H2S on physiological and pathological processes. Qabazard et al. (45) found that the effects of GYY4137 on the relaxation of rat CC were at least partly mediated by the NO pathway and KATP channel. However, only a few studies have reported the use of GYY4137 to treat penile tissues. Moreover, GYY4137 produces CO, a by-product that acts in a similar way to H2S (70). Therefore, more experimental data are required to support the conclusion of existing studies. An in-depth understanding of the mechanisms through which H2S regulates penile erection may guide the development of novel therapeutic approaches for ED.
3.1.2 Therapeutic targets and future perspectives to treat ED
Penile erection involves the cooperation of nerves, blood vessels, and smooth muscle; consequently, lesions or damage in any part of these nerves, blood vessels, and smooth muscle may lead to ED. Neurovascular damage, diabetes, hypertension, side effects of drugs, and testosterone deficiency have been identified as causes of ED (71). PDE-5 inhibitors (PDE-5is) (such as sildenafil and tadalafil) are considered the first-line treatment for ED (72); however, some patients have poor outcomes. Before the erection-promoting effects of H2S were reported, a novel target, β3-adrenergic receptor, was identified. β3-adrenergic receptors are present in human CCSMCs and cause smooth muscle relaxation in HCC in a cGMP-dependent but NO-independent manner upon activation (73). Mitidieri et al. (74) found that activation of β3 receptors by BRL37344 (a β3-selective agonist) relaxed HCC and penile arterial rings in an H2S/cGMP-dependent manner, whereas inhibition of CSE notably reduced the relaxation. Treatment with BRL37344 considerably increased H2S production, whereas inhibition of CSE reduced the BRL37344-induced increase in cGMP expression in both tissues. Given that their function is independent of the endothelium, selective β3 agonists, such as mirabegron (75), may serve as alternative treatment agents for patients who do not respond to PDE-5is.
ED has been associated with defects in the L-Cys/H2S pathway (76). The levels of H2S synthases are decreased to varying degrees in the penile tissues of rats with ED caused by radical prostatectomy, hyperlipidemia, diabetes, or hypertension (27, 42, 44, 77). This phenomenon suggests that ED can be treated with exogenous H2S supplementation. The first drug developed was H2S-donating derivative of sildenafil (ACS6) (78). Although the muscle relaxation effects of ACS6 are similar to those of sildenafil citrate at the same concentration, ACS6 is more effective than sildenafil and NaHS in reducing superoxide formation and PDE5 expression. Theoretically, long-term use of ACS6 may improve ED by inhibiting oxidative stress and downregulating PDE5. Several natural plant extracts have been found to stimulate H2S synthesis in vivo. For example, resveratrol (RVT) (79), found in red wine, causes CC relaxation in a concentration-dependent manner in mice. This effect can be reversed by CBS inhibitors but not by L-NAME. Although RVT increases the basal and L-Cys-induced production of H2S, it does not affect NaHS-induced relaxation. These results suggest that RVT-induced relaxation is at least partly dependent on H2S production, does not interfere with the downstream mechanisms of H2S production, and is independent of NO. Sodium tanshinone IIA sulfonate (STS) (77), a water-soluble derivative of lipophilic diterpene isolated from the roots of Danshen plants, can reverse the high fat diet-induced decrease in CBS and CSE expression and H2S production in rats. In addition, it can preserve erectile function by activating the Nrf2/HO-1 pathway against high fat diet-induced oxidative stress.
Because H2S is primarily released by SMCs instead of endothelial cells, it may serve as a promising therapeutic target in patients with ED with endothelial dysfunction, such as those with metabolic syndrome and diabetes. A study showed that high-fructose diet-induced metabolic syndrome led to a reduction in the basal and L-Cys-induced production of H2S in rat penile tissues (80), whereas supplementation with exogenous H2S improved erectile function. GYY4137, an H2S donor with sustained release, has been shown to improve cavernosal vascular reactivity by inhibiting the TGF-β1/Smad/CTGF pathway in rats with STZ-induced diabetes (46). In addition, long-term treatment with GYY4137 can prevent or attenuate the reduction of H2S levels and improve cavernosal H2S production in diabetes (45, 46). The combination of H2S donors and PDE-5is holds great promise in the treatment of ED. A study showed that NaHS combined with tadalafil was more effective than NaHS alone in the treatment of ED in rats with partial bladder outlet obstruction (81). The reduced erectile response and H2S levels were only partially restored upon treatment with NaHS but completely restored upon treatment with both NaHS and tadalafil. In addition, the combined use of NaHS and tadalafil reversed the morphological and functional changes in the penis caused by ischemia after obstruction and had a positive effect on the erectile response. These results suggest that H2S can improve ED and restore spontaneous erection with long-term use.
3.2 Effects of H2S on the prostate
PCa, and particularly castration-resistant prostate cancer (CRPC), is the primary focus of research on H2S’ utilities on the prostate. Because the growing and progression of the prostate depend on androgens, androgen deprivation therapy is the mainstay of treatment for advanced PCa. However, most patients inevitably progress to androgen-independent castration resistance, which is a leading cause of death in patients with PCa (82). Given that the signals generated upon the biding of the androgen receptor (AR) to testosterone or 5α-dihydrotestosterone are closely related to the progression of PCa to CRPC, direct inhibition of AR is one of the widely used therapeutic strategies for PCa (82, 83). The highly conserved DNA-binding domain, one of the four domains of AR, contains two cysteine type 4 zinc fingers; of which, the second zinc finger is the binding site for homodimerization (83). According to Zhao et al. (30), H2S suppresses transactivation of AR by S-sulfhydrating cysteine Cys611 and Cys614 sites of its second zinc finger, thereby inhibiting the progression of antiandrogen-resistant PCa cells. Bicalutamide competes with AR for binding, making it an effective cancer treatment. It has been reported that PCa (LNCAP-B) cells resistant to bicalutamide expressed less of CSE than PCa (LNCaP) cells dependent on androgens. LNCaP-B cells overexpressing CSE or administered NaHS re-established sensitivity to bicalutamide, while CSE-deficient LNCaP cells persist in growing with the utilization of bicalutamide. On the contrary, changes in CBS expression under the same conditions did not have obvious effects on drug resistance. Therefore, it is possible to use the CSE/H2S system to assess prognosis and to treat early PCa and CRPC. However, Fukami et al. (47) showed that the effects of H2S were not beneficial to the treatment of PCa. They showed that androgen deprivation-induced enhancement of cytosolic cAMP elevated CSE expression and H2S production. A subsequent study found that H2S enhanced the activity of Cav3.2, which led to an increase in proliferation of tumors independent of androgen. Neuroendocrine phenotypes account for approximately 20–25% of all metastatic CRPC cases. In most cases, neuroendocrine differentiation is induced by androgen deprivation therapy (84). Differentiation of LNCaP cells is characterized by the upregulation of Ca2+-dependent secretion of mitogenic factors and the overexpression of Cav3.2 T-type Ca2+ channels that contribute to their secretion (85, 86). Fukami et al. showed that differentiated cells had increased expression of CSE and CBS and elevated T-type Ca2+ channel-dependent currents (T-currents). The T-currents were suppressed by CSE inhibitors but not by CBS inhibitors and were enhanced by H2S donors (NaHS, 0.1–1.5 mM; Na2S, 0.03–0.1 mM). These results indicate that in LNCaP cells undergoing neuroendocrine differentiation, H2S is able to stimulate T-type Ca2+ channels, leading to the development of neuroendocrine CRPC.
The abovementioned studies indicate that CSE/H2S is associated with the progression of PCa, and the contradictory conclusions could be attributed to the fact that PCa cells respond differently to H2S depending on their source, and dose and type of H2S donor (87). Research shows that drugs related to H2S are effective in treating cancer. Multi-cancer cell lines, including PCa cells, are inhibited 28–3000 times more effectively by H2S-releasing non-steroidal anti-inflammatory drugs (HS-NSAIDs) than by those of conventional NSAIDs (88). HS-ibuprofen is 200 times more potent than ibuprofen in LNCaP cells owing to its covalent attachment to the H2S-releasing moiety. The H2S moiety of H2S-releasing doxorubicin (H2SDox) exerts cardioprotective effects, reducing the cardiovascular side effects of doxorubicin (89). By releasing the SH2 group, disulfide bonds formation on P-gp would disrupt P-gp activity, ultimately improving tumor sensitivity to doxorubicin. A study showed that intracellular drug accumulation was substantially higher after H2SDox treatment than after doxorubicin treatment in DU-145 PCa cells resistant to androgen and doxorubicin. Zhou et al. (90) combined H2S with classical drugs and used nanotechnology to develop Zn2+-interference and H2S-induced therapeutics, which responds to the tumor microenvironment (TME) and is derived from tannic acid (TA)-altered zinc sulfide nanoparticles (ZnS@TA). ZnS@TA nanoparticles responded specifically to tumor cells based on the pH. H2S and Zn2+ were released in a small amount in a neutral environment (pH = 7.4) but had good degradation performance in a simulated TME. Consistently, ZnS@TA nanoparticles had no obvious effects on the viability of DU-145 cells under neutral conditions but considerably decreased cell viability in the TME at the same concentration. ZnS@TA nanoparticles attenuated the migratory and invasive abilities of PCa cells by increasing intracellular TA and Zn2+ levels. Subsequently, Zn2+ ions and H2S synergistically enhanced tumor cell apoptosis.
3.3 Effects of H2S on the testis
As a gaseous air pollutant, H2S may impair spermatogenesis (91) and inhibit sperm mobility via mechanisms related to AMPK/Akt (48) when combined with NH3, thereby disrupting male fertility. However, there are several investigations indicate that H2S can protect the testis and germ cells by inhibiting inflammation, oxidant activity, and apoptosis (Figure 2). The testis and sperm are highly susceptible to oxidative stress-induced damage. Studies have shown that physical stimulation (heat stress and restraint stress) (92), ischemia–reperfusion (I/R) injury, varicocele (testicular torsion), and ingestion of reproductive-toxic substances (cisplatin and nanoplastics) can increase the production of reactive oxygen species (ROS) in the testis, leading to testicular dysfunction and germ cell apoptosis. In addition, the aforementioned conditions may decrease the expression of CBS and CSE and production of H2S in the testis. Therefore, low levels of H2S in the testis may play a key role in male infertility.
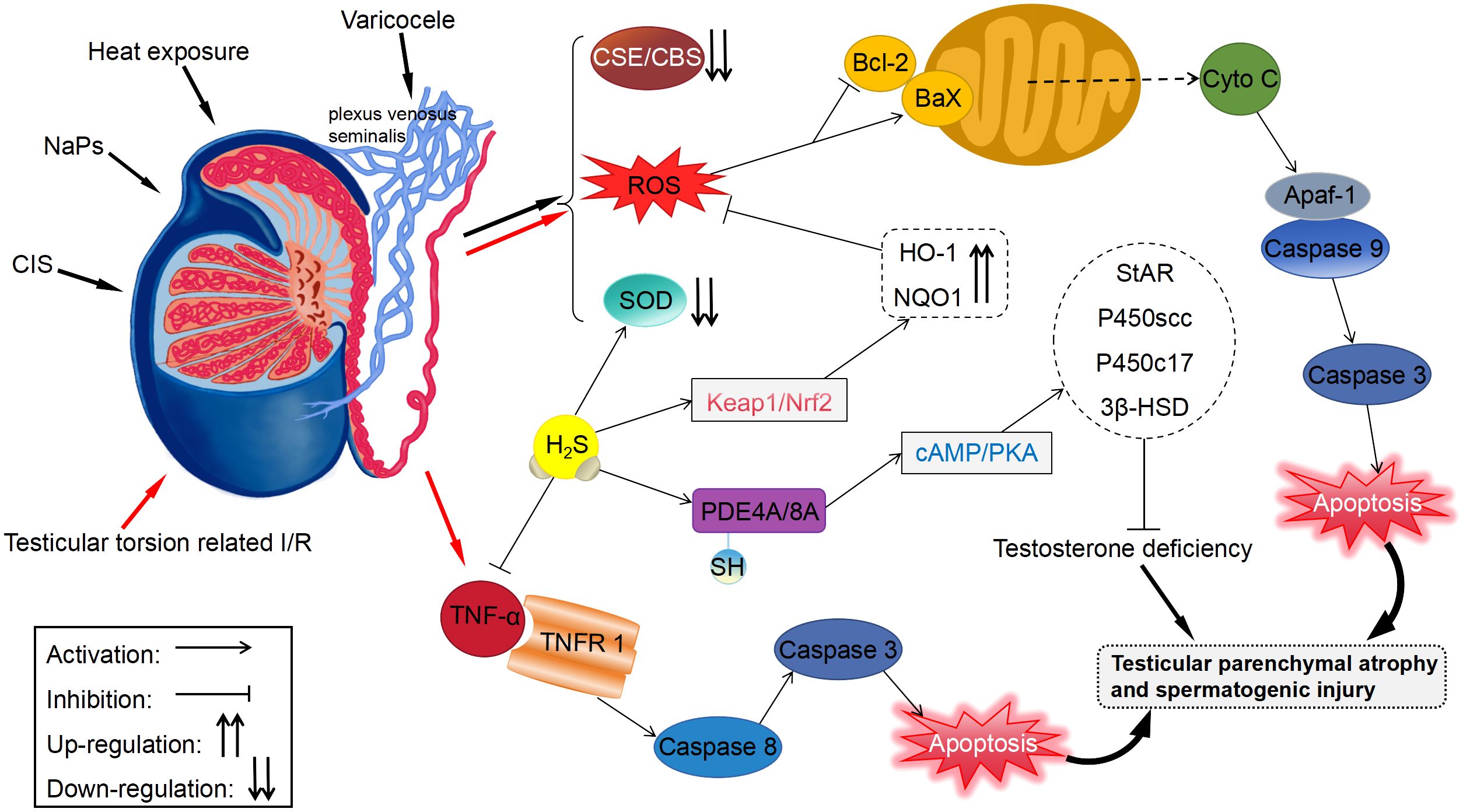
Figure 2 Protective effects of H2S on the testis and germ cells. Apaf-1, apoptosis protease-activating factor-1; HO-1, heme oxygenase-1; NQO1, NADPH dehydrogenase quinone 1.
Li et al. (32) stimulated the testis of mice with heat exposure (42°C, 30 minutes/day, 3 days) and found that heat stress remarkably elevated ROS generation and inhibited the SOD activity in germ cells. However, exogenous administration of NaHS stimulated SOD activity and reduced ROS generation. Mechanistically, H2S can inhibit cytochrome C release and Bax/Bcl-2 ratio, thus impeding the heat stress-induced testicular germ cells apoptosis. Bozkurt et al. (49) found that H2S alleviated excessive tissue detriment by reducing the levels of iNOS and the inflammatory cytokine TNF-α in a rat model of testicular torsion-induced I/R damage. Furthermore, H2S suppressed apoptosis through reducing apoptosis protease activating factor-1 level, thus protecting against testicular damage. Patients with varicocele are usually treated with surgery; however, the removal of the varicose veins (varicocelectomy) carries not only the risk of surgery but also the risk of postoperative side effects, including recurrence, hydrocele formation, atrophy, and bleeding (93). Rats with left varicocele exhibited significant reductions in left testicle and epididymis weights, as well as diameters and epithelial thicknesses of the seminiferous tubules. When NaHS was administered over a long period, it reduced oxidative stress and apoptosis in the testicles, restoring above results (94). According to the study by Xia et al. (54), GYY4137 had beneficial effects on rats with varicocele-induced ipsilateral epididymis damage and sperm injury through stimulating PI3K/Akt signaling. Additionally, Shafie et al. (95) reported that testosterone combined with NaHS alleviated varicocele-induced injury in rats. It was shorter in duration and required lower doses than using NaHS for a long period of time when testosterone was combined with NaHS.
Cisplatin (CIS), an antineoplastic drug increases the levels of free radicals and decreases those of antioxidant enzymes or non-enzymes in testicular tissue via oxidative stress, resulting in testicular toxicity (96). It was observed in rats testicular tissues that administration with CIS notably elevated the content of MDA, a direct indicator of ROS-induced lipid peroxidation, and inhibited the activity of SOD (97). Whereas, CIS-induced changes in biochemistry, histology, and morphology could be effectively reversed with NaHS treatment. Ingested polystyrene nanoparticles can accumulate in the testis and cause testicular atrophy, degeneration of spermatogenic tubules, and spermatogenesis disorders (98, 99). Using mouse spermatocytes, Li et al. (50) observed that nanoplastics caused autophagy and apoptosis dependent on ROS, leading to reproductive noxiousness. Conversely, H2S mitigated nanoplastic-mediated reproductive noxiousness via upregulating antioxidant enzymes level, including HO-1 and NQO1, through the Keap1/Nrf2 pathway. Additionally, testosterone inadequacy may adversely impact sperm morphology and vitality, potentially compromising male fertility (100). A mouse Leydig tumor cell line with H2O2 + LPS-induced testosterone deficits was overexpressed of CBS to restore testosterone levels by S-sulfhydrylating PDE4A and PDE8A and stimulating the cAMP/PKA signaling (51). Another study showed that animal models of deficient H2S production had lowered sperm vitality, which was alleviated upon exogenously administering H2S or overexpressing CBS (52). The stress-induced decrease in endogenous H2S generation was involved in damaged spermatogenesis and a defective blood–testis barrier.
It is possible that H2S may enhance sperm vitality in vitro, which could have implications for assisted reproduction. During the extraction of human sperm from a sperm bank for artificial insemination, freezing and thawing can produce ROS, resulting in oxidative stress-induced impairment to sperm (101). H2S donors are capable of maintaining sperm vitality, reducing acrosomal deprivation, and protecting sperm against oxidative stress (53). In particular, a high concentration of H2S attenuates sperm movement (102). In contrast, the simultaneous administration of low concentrations of H2S and NO donors has been shown to promote sperm frontward movement and safeguard plasma membrane against oxidative stress (103). According to Pintus et al. (104), short-term treatment with two N-thiocarboxyanhydrides derived from glycine and leucine enhanced the mitochondrial activity of pig sperm cells even in the presence of ROS. The two amino acid-based H2S-releasing drugs can mimic the physiological H2S release when carbonic anhydrase is present without causing cellular harm. Additionally, they can enhance sperm vitality after short-term treatments, consequently prolonging sperm survival.
3.4 Effects of H2S on other male reproductive organs
Studies investigating the effects of H2S on the epididymis and VD are limited. The contraction of the epididymis and VD contributes to the discharge of sperm. Studies have shown that the L-Cys/H2S pathway is involved in the regulation of VD tonic contractions and that H2S relaxes VD smooth muscle in a concentration-dependent manner (33, 55). Based on these findings, the mechanisms underlying VD relaxation have been investigated in some studies. Li et al. (55) treated VD bands with 2-APB, an inhibitor of transient receptor potential [TRP] channels, and apamin, an inhibitor of Ca2+-activated K+ [SKCa] channels, as well as L-NAME, TEA, iberiotoxin, GLB, and subsequently treated them with NaHS. According to the results, L-NAME, GLB, 2-APB, and apamin had no influence on the relaxation of VD to NaHS, while TEA and iberiotoxin considerably reduced it. These findings indicate that H2S may target BKCa channels in VD. Moreover, N-ethylmaleimide safeguards thiols against oxidation by alkylation, counteracting NaHS-mediated smooth muscle relaxation in VD; however, the strong reducing agent DTT, which can disrupt disulfide bonds in proteins, did not alter the effect of NaHS. The results indicate that H2S may impact the function of BKCa channels in VD smooth muscle by S-sulfhydration, consequently leading to muscle relaxation. In the aforementioned study, NaHS was used at a concentration of 1 mM, which exceeds the physiological concentration of H2S. Therefore, whether H2S regulates spontaneous contractions in VD in the physiological state remains unknown. In the epididymal lumen, the microenvironment supports sperm vitality, and mature sperm are stocked in the epididymis’ tail until ejaculation (105). Gao et al. (34) found that H2S induced remarkable K+ release from the epididymal epithelium in rats through stimulating KATP and BKCa channels. An increase in K+ concentration in the cauda epididymal intraluminal fluid inhibited sperm vitality regardless of pH. Consequently, H2S created a microenvironment with an elevated K+ concentration in the cauda epididymis lumen, which maintained epididymal sperm quiescence prior to ejaculation. According to these findings, H2S plays a significant role in ejaculation. It is possible that future studies will lead to the development of novel strategies for treating asthenospermia, spermatorrhea, as well as premature ejaculation.
4 Effects of H2S on other organs in the genitourinary system
In addition to the aforementioned components of the male reproductive system, the kidneys, ureters, bladder, and urethra are also impacted by H2S. Among these, the kidneys have been the subject of extensive research, particularly in relation to acute kidney injury, chronic kidney disease, kidney cancer, and other related conditions. Despite this, the bladder is often the primary organ considered in discussions of the genitourinary system due to its close association with the reproductive system. Specifically, the correlation between ED and lower urinary tract symptoms (LUTS) has been a topic of widespread examination.
H2S has been shown to potentially alleviate LUTS and associated ED by inducing relaxation of the smooth muscle in the bladder. Male LUTS encompasses a range of structural, functional, and sensory abnormalities affecting the lower urinary tract, including pelvic and pelvic floor organs such as the bladder, prostate, and urethra (106). The presentation of LUTS is multifaceted, with symptoms categorized into storage, voiding, and post-voiding symptoms, often occurring concurrently in affected individuals (107). The correlation between ED and LUTS has been extensively studied in recent years, with a growing body of evidence indicating a strong link, particularly in men with LUTS who are at a higher risk of developing ED (108, 109). For instance, a comprehensive population-based study examining the impact of overactive bladder, specifically urgency as a storage symptom, on male sexual health revealed a significant association with ED (110).
The primary mechanism of action for current first-line medications used in the treatment of LUTS involves the relaxation of bladder smooth muscle (111). The process of bladder contraction during urination is primarily regulated by cholinergic, adrenergic, and sensorimotor nerves (112). Early research indicated that the effect of H2S on the bladder mirrors that of capsaicin. In rats, NaHS prompted bladder contractions by stimulating primary afferent nerve (sensory nerve) terminals to release tachykinin, which subsequently activated NK1 and NK2 receptors (113). It is noteworthy that sensory nerves rapidly developed desensitization to H2S, leading to the cessation of contractions. Additionally, the application of H2S to desensitized sensory nerves resulted in the release of inhibitory neuropeptides and induced bladder relaxation (114). As the research progressed, Fernandes et al. (115) determined that GYY4137 activates L-type Cav channels in a concentration-dependent manner to enhance ACh release from guinea pig bladder neurons, thereby increasing the amplitude of phasic contraction of bladder smooth muscle. These experiments utilized isolated bladder strips with the urothelium removed, despite the presence of H2S not only in the nerve fiber and smooth muscle layers of the bladder but also in the urothelium (19, 25, 116, 117). Consistently, activation of M1/M3 receptors in the human urothelium leads to the phosphorylation of CBS at Ser227 through the cGMP/PKG pathway, resulting in elevated epithelial H2S production and bladder band relaxation (118). The excision of the urothelium and the use of CBS inhibitors both markedly enhanced carbachol-induced contractions in human bladder strips. Additionally, activation of β3 receptors in the human urothelium has been shown to stimulate H2S production and promote bladder relaxation. CSE, but not the CBS inhibitor, was found to increase BRL37344-induced relaxation, a response that was abolished following epithelial removal (117). These findings suggest that H2S may play a role in modulating bladder function as a neuromodulator.
In addition, H2S also causes relaxation of bladder smooth muscle by activating ion channels. It is now generally confirmed that H2S induces bladder relaxation by activating KATP channels and mechanisms that desensitize Ca2+ (25, 114, 119). On the contrary, the BKCa channel, another frequently researched ion channel, has sparked controversy. Fernandes et al. (115) demonstrated that GYY4137 directly inhibited BKCa channels activity and decreased BK channels open probability in guinea pig bladder smooth muscle, leading to an increase in spontaneous phasic and neurally evoked contractions. Conversely, inhibiting BKCa channels in pig bladder neck smooth muscle has been found to diminish the relaxant effects of rolipram, a PDE4 inhibitor, with the observed impact of rolipram being partially attributed to H2S released from neurons. Essentially, the relaxation of pig bladder neck smooth muscle may be facilitated by H2S activation of BKCa channels (119). The conflicting outcomes of the two studies underscore the necessity for additional research, particularly considering the absence of urothelium in the bladder tissues utilized. Factors such as species specificity and the quality of experimental reagents must also be taken into account. Recent advancements in the field have identified PDE inhibitors as promising therapeutic options for the management of LUTS and ED (120). Numerous investigations have demonstrated the involvement of H2S in the mechanism of action of these drugs, with rolipram exhibiting significantly greater efficacy compared to sildenafil (119, 121, 122).
In conclusion, the relaxation effect of H2S on bladder smooth muscle is beneficial to relieve LUTS inclusive of overactive bladder, thereby removing the primary cause of related ED and improving the life quality of patients in many ways.
5 Conclusion
H2S, the third gas signaling molecule after NO and CO, is involved in various pathophysiological processes in the male reproductive system. In particular, it promotes penile erectile function, protects testicular function, inhibits the progression of PCa, regulates the spontaneous contraction of VD, and maintains the hypomotility of sperm in the cauda epididymis before ejaculation. A complex mechanism by which H2S relaxes smooth muscles in the penis may involve activating BKCa and Kv channels, inhibiting RhoA/ROCK signaling pathways, and raising cGMP levels to bypass NO pathway. Recently, selective β3 agonists such as mirabegron (75) and natural extracts such as RVT (79) and STS (77) have been shown to improve ED, with both classes of compounds being at least partly dependent on H2S. Besides, the relaxation of bladder smooth muscle by H2S has been shown to improve LUTS and subsequent ED. Consequently, the multifaceted therapeutic benefits of H2S offer potential for reducing medication dosages and enhancing the quality of life for patients with both conditions. Therefore, H2S is a promising target for the clinical treatment of male reproductive system diseases.
The process of H2S promoting penile erection may involve a variety of mechanisms, however, a unanimous final conclusion has not yet been reached. There is a very interesting theory in the literature on ACS6 treatment of ED (78) and this theory (Figure 3) is as follows: Superoxides derived from NADPH oxidase (NOX) react with NO to form active nitrogen in CCSMCs and arterial SMCs, thereby reducing the bioavailability of NO and attenuating penile erection (123–126). NO inhibits the activity and expression of NOX via the cGMP/PKG pathway (127–129). However, in patients with ED with diseases that impair endothelial function, such as diabetes, decreased NO levels lead to a reduction in cGMP expression, which in turn attenuates the inhibitory effects of NO on NOX and increases the production of superoxides, eventually forming a vicious circle. These superoxides upregulate PDE5 expression, resulting in the increased metabolism of cGMP. Similar to NO, H2S inhibits the activity and expression of NOX via the cAMP/PKA pathway (130). NaHS can activate PKG dependent on cGMP (131). In addition, the superoxides derived from NOX can activate ROCK (132).
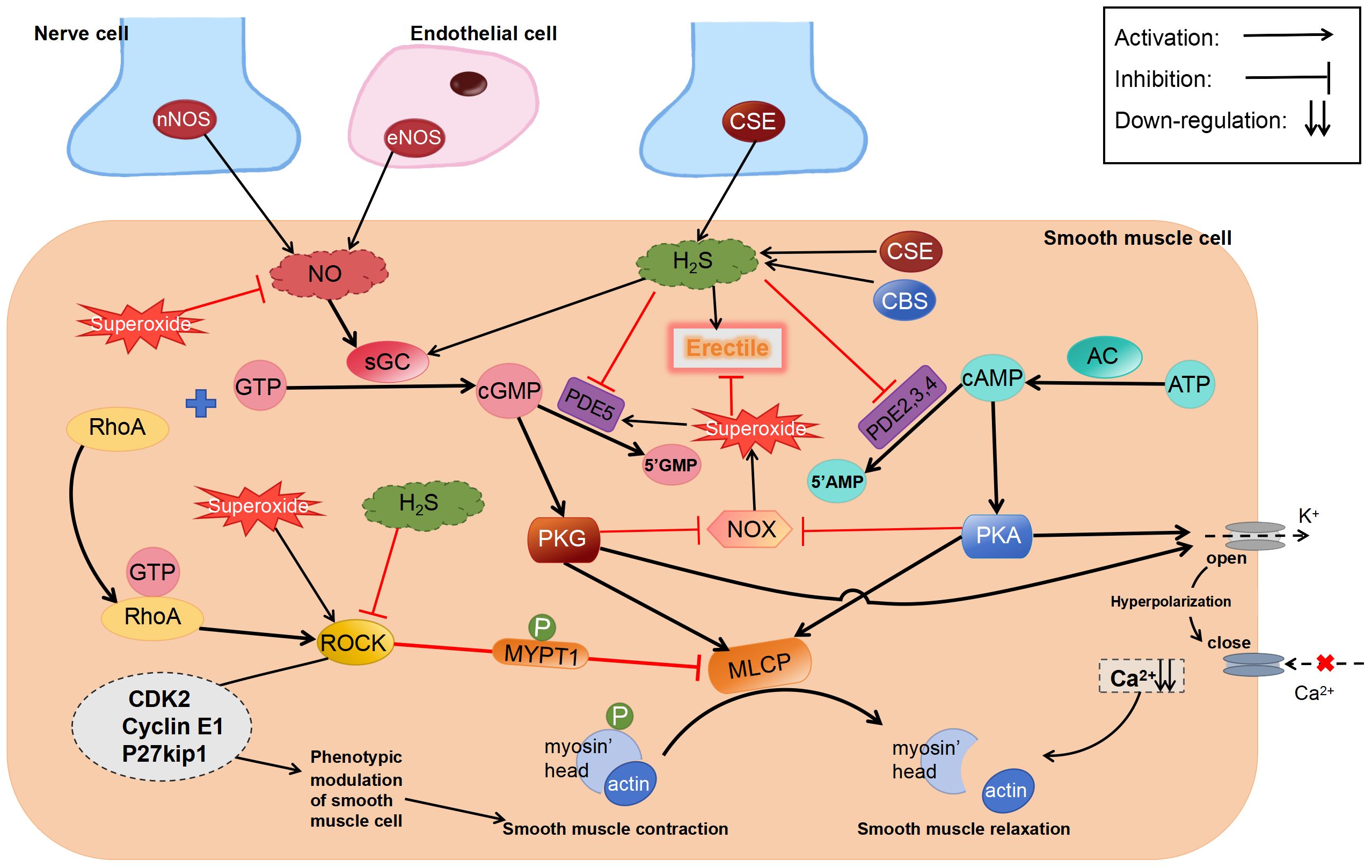
Figure 3 Regulatory effects of H2S on erectile dysfunction. ROCK, Rho-kinase; sGC, soluble guanylate cyclase; MYPT1, myosin phosphatase-targeting subunit 1; cGMP, cyclic guanosine monophosphate.
Although the abovementioned theory has not been comprehensively investigated, we speculate that “inhibition of superoxide production” is a more reasonable explanation for the contradictory results of existing studies. As well as activating PKA and PKG, H2S inhibits superoxide generation by regulating Nrf2 and downstream anti-oxidative stress proteins, such as SOD, NQO1, and HO-1, via S-sulfhydration of Keap1 (133). In addition to activating KATP (134) and BKCa (55) channels, H2S-mediated S-sulfhydration effect reduces PDE5A dimerization (135). Therefore, irrespective of the type of pathway inhibitors used, they can only partially prevent the relaxation induced by H2S.
Although exogenous H2S has been shown to alleviate ED, further investigation into the mechanisms through which H2S promotes erectile function may guide the development of targeted drugs. Moreover, the role of endogenous H2S in promoting penile erection remains uncertain. Ghasemi et al. (26) found that PAG increased NANC relaxation in rat CC, which may be attributed to the inhibition of NOS activity by H2S or the direct chemical reaction of H2S with endogenous nitrogen oxides, such as NO, NO+, and HNO. Based on this hypothesis, the authors divided rat CC strips precontracted with PE into three groups, which were treated with S-nitrosoglutathione, SNP, and Angeli’s salt (three nitrogen oxide donors), respectively. Subsequently, each group was treated with NaHS at doses of 30 nM, 300 nM, or 30 μM. The results showed that all three nitrogen oxide donors induced the relaxation of rat CC tissues in a concentration-dependent manner; however, treatment with NaHS remarkably inhibited the effects of Angeli’s salt. These findings indicate that NO participates in the regulation of erectile function mainly in the form of HNO and is inhibited by H2S. Some studies have suggested that the physiological concentration of H2S is at the nanomolar level (136). Ghasemi et al. showed that CC relaxation was observed only after treatment with NaHS. Therefore, endogenous H2S may inhibit nitrogenous relaxation in CC. However, to date, limited studies have focused on this topic, and further research is warranted to determine the regulatory effects of endogenous H2S on CC relaxation.
In addition to its effects on the penis, H2S has diverse effects on other male reproductive organs, which warrant an in-depth investigation. The expression of CBS is not considerably altered in hormone-dependent and drug-resistant human PCa tissues (30). Even if it is increased after neuroendocrine differentiation of PCa cells, it does not appear to be involved in the effects of H2S (47). However, overexpression of CBS in the testis can alleviate spermatogenesis disorders (51), and CBS/H2S can regulate testosterone synthesis (100) Therefore, the use of CBS as a therapeutic target for restoring testicular function may minimize the risk of side effects on the prostate. Given that successful ejaculation requires smooth muscle contraction in the VD, seminal vesicle, and prostate (137), drugs targeting H2S in the VD may be used to treat premature ejaculation. However, the effects of H2S on the VD suggest that the half-life of H2S should be controlled for a certain period to prevent ejaculation disorders and maintain normal sexual function and effective sexual behavior in men. In conclusion, H2S possesses great potential in the treatment of male reproductive system diseases.
Author contributions
YS: Writing – original draft. CM: Writing – original draft. QZ: Writing – original draft. RZ: Writing – original draft. DJ: Writing – review & editing. XS: Writing – review & editing.
Funding
The author(s) declare financial support was received for the research, authorship, and/or publication of this article. This study was supported by the China Postdoctoral Science Foundation (2022M711089), Heilongjiang Provincial Natural Science Foundation (YQ 2022H020), Postdoctoral Fund of Heilongjiang Province (LBHZ21080) and (LBHZ19035), Heilongjiang Provincial Health Commission Fund (20210202010178), and Doctor Innovation Fund of Heilongjiang University of Traditional Chinese Medicine (2019BS06).
Acknowledgments
We thank Bullet Edits Limited for the linguistic editing and proofreading of the manuscript.
Conflict of interest
The authors declare that the research was conducted in the absence of any commercial or financial relationships that could be construed as a potential conflict of interest.
Publisher’s note
All claims expressed in this article are solely those of the authors and do not necessarily represent those of their affiliated organizations, or those of the publisher, the editors and the reviewers. Any product that may be evaluated in this article, or claim that may be made by its manufacturer, is not guaranteed or endorsed by the publisher.
References
1. Miyaso H, Ogawa Y, Itoh M. Microenvironment for spermatogenesis and sperm maturation. Histochem Cell Biol. (2022) 157:273–85. doi: 10.1007/s00418-021-02071-z
2. Farsimadan M, Motamedifar M. Bacterial infection of the male reproductive system causing infertility. J Reprod Immunol. (2020) 142:103183. doi: 10.1016/j.jri.2020.103183
3. Fozooni R, Jafarzadeh Shirazi MR, Saedi S, Namavar Jahromi B, Khoradmehr A, Anvari M, et al. Male subfertility effects of sub-chronic ethanol exposure during stress in a rat model. Alcohol. (2021) 96:63–71. doi: 10.1016/j.alcohol.2021.08.003
4. Han X, Huang Q. Environmental pollutants exposure and male reproductive toxicity: The role of epigenetic modifications. Toxicology. (2021) 456:152780. doi: 10.1016/j.tox.2021.152780
5. He L, Gong H, You S, Zhang C, Zhong C, Li L. miRNA-138–5p suppresses cigarette smoke-induced apoptosis in testicular cells by targeting Caspase-3 through the Bcl-2 signaling pathway. J Biochem Mol Toxicol. (2021) 35:e22783. doi: 10.1002/jbt.22783
6. Castela A, Gomes P, Domingues VF, Paíga P, Costa R, Vendeira P, et al. Role of oxidative stress-induced systemic and cavernosal molecular alterations in the progression of diabetic erectile dysfunction. J Diabetes. (2015) 7:393–401. doi: 10.1111/1753-0407.12181
7. Kalinina EV, Gavriliuk LA, Pokrovsky VS. Oxidative stress and redox-dependent signaling in prostate cancer. Biochem (Mosc). (2022) 87:413–24. doi: 10.1134/S0006297922050030
8. Erfani MN, Sadeghi N, Tavalaee M, Tabandeh MR, Nasr-Esfahani MH. Evaluation of oxidative stress in testis and sperm of rat following induced varicocele. Urol. J. (2019) 16:300–6. doi: 10.22037/uj.v0i0.4740
9. Bisht S, Faiq M, Tolahunase M, Dada R. Oxidative stress and male infertility. Nat Rev Urol. (2017) 14:470–85. doi: 10.1038/nrurol.2017.69
10. Wang R. The gasotransmitter role of hydrogen sulfide. Antioxid Redox Signal. (2003) 5:493–501. doi: 10.1089/152308603768295249
11. Li J, Teng X, Jin S, Dong J, Guo Q, Tian D, et al. Hydrogen sulfide improves endothelial dysfunction by inhibiting the vicious cycle of NLRP3 inflammasome and oxidative stress in spontaneously hypertensive rats. J Hypertens. (2019) 37:1633–43. doi: 10.1097/HJH.0000000000002101
12. Shirazi MK, Azarnezhad A, Abazari MF, Poorebrahim M, Ghoraeian P, Sanadgol N, et al. The role of nitric oxide signaling in renoprotective effects of hydrogen sulfide against chronic kidney disease in rats: Involvement of oxidative stress, autophagy and apoptosis. J Cell Physiol. (2019) 234:11411–23. doi: 10.1002/jcp.27797
13. Sun X, Zhang R, Zhong Q, Song Y, Feng X. Regulatory effects of hydrogen sulfide on the female reproductive system. Eur J Pharmacol. (2024) 963:176265. doi: 10.1016/j.ejphar.2023.176265
14. Řimnáčová H, Moravec J, Štiavnická M, Havránková J, Monsef L, Hošek P, et al. Evidence of endogenously produced hydrogen sulfide (H2S) and persulfidation in male reproduction. Sci Rep. (2022) 12:11426. doi: 10.1038/s41598-022-15360-x
15. Mao Z, Li H, Zhao XL, Zeng XH. Hydrogen sulfide protects Sertoli cells against toxicant Acrolein-induced cell injury. Food Chem Toxicol. (2023) 176:113784. doi: 10.1016/j.fct.2023.113784
16. Olivencia MA, Esposito E, Brancaleone V, Castaldo S, Cirino G, Pérez-Vizcaino F, et al. Hydrogen sulfide regulates the redox state of soluble guanylate cyclase in CSE-/- mice corpus cavernosum microcirculation. Pharmacol Res. (2023) 194:106834. doi: 10.1016/j.phrs.2023.106834
17. Srilatha B, Muthulakshmi P, Adaikan PG, Moore PK. Endogenous hydrogen sulfide insufficiency as a predictor of sexual dysfunction in aging rats. Aging. Male. (2012) 15:153–8. doi: 10.3109/13685538.2012.668722
18. Kamoun P. Endogenous production of hydrogen sulfide in mammals. Amino. Acids. (2004) 26:243–54. doi: 10.1007/s00726-004-0072-x
19. Zou S, Shimizu T, Shimizu S, Higashi Y, Nakamura K, Ono H, et al. Possible role of hydrogen sulfide as an endogenous relaxation factor in the rat bladder and prostate. Neurourol. Urodyn. (2018) 37:2519–26. doi: 10.1002/nau.23788
20. Shibuya N, Koike S, Tanaka M, Ishigami-Yuasa M, Kimura Y, Ogasawara Y, et al. A novel pathway for the production of hydrogen sulfide from D-cysteine in mammalian cells. Nat Commun. (2013) 4:1366. doi: 10.1038/ncomms2371
21. Zhao W, Zhang J, Lu Y, Wang R. The vasorelaxant effect of H2S as a novel endogenous gaseous KATP channel opener. EMBO. J. (2001) 20:6008–16. doi: 10.1093/emboj/20.21.6008
22. Srilatha B, Adaikan PG, Li L, Moore PK. Hydrogen sulphide: a novel endogenous gasotransmitter facilitates erectile function. J Sex Med. (2007) 4:1304–11. doi: 10.1111/j.1743-6109.2007.00561.x
23. d’Emmanuele diVBR, Sorrentino R, Maffia P, Mirone V, Imbimbo C, Fusco F, et al. Hydrogen sulfide as a mediator of human corpus cavernosum smooth-muscle relaxation. Proc Natl Acad Sci U. S. A. (2009) 106:4513–8. doi: 10.1073/pnas.0807974105
24. Guo H, Gai JW, Wang Y, Jin HF, Du JB, Jin J. Characterization of hydrogen sulfide and its synthases, cystathionine β-synthase and cystathionine γ-lyase, in human prostatic tissue and cells. Urology. (2012) 79:483.e1–483.e4835. doi: 10.1016/j.urology.2011.10.013
25. Gai JW, Wahafu W, Guo H, Liu M, Wang XC, Xiao YX, et al. Further evidence of endogenous hydrogen sulphide as a mediator of relaxation in human and rat bladder. Asian. J Androl. (2013) 15:692–6. doi: 10.1038/aja.2013.32
26. Ghasemi M, Dehpour AR, Moore KP, Mani AR. Role of endogenous hydrogen sulfide in neurogenic relaxation of rat corpus cavernosum. Biochem Pharmacol. (2012) 83:1261–8. doi: 10.1016/j.bcp.2012.01.026
27. Zhang Y, Yang J, Wang T, Wang SG, Liu JH, Yin CP, et al. Decreased endogenous hydrogen sulfide generation in penile tissues of diabetic rats with erectile dysfunction. J Sex Med. (2016) 13:350–60. doi: 10.1016/j.jsxm.2016.01.002
28. Yetik-Anacak G, Dikmen A, Coletta C, Mitidieri E, Dereli M, Donnarumma E, et al. Hydrogen sulfide compensates nitric oxide deficiency in murine corpus cavernosum. Pharmacol Res. (2016) 113:38–43. doi: 10.1016/j.phrs.2016.08.015
29. Aydinoglu F, Dalkir FT, Demirbag HO, Ogulener N. The interaction of l-cysteine/H2S pathway and muscarinic acetylcholine receptors (mAChRs) in mouse corpus cavernosum. Nitric. Oxide. (2017) 70:51–8. doi: 10.1016/j.niox.2017.08.005
30. Zhao K, Li S, Wu L, Lai C, Yang G. Hydrogen sulfide represses androgen receptor transactivation by targeting at the second zinc finger module. J Biol Chem. (2014) 289:20824–35. doi: 10.1074/jbc.M114.559518
31. Sugiura Y, Kashiba M, Maruyama K, Hoshikawa K, Sasaki R, Saito K, et al. Cadmium exposure alters metabolomics of sulfur-containing amino acids in rat testes. Antioxid Redox Signal. (2005) 7:781–7. doi: 10.1089/ars.2005.7.781
32. Li G, Xie ZZ, Chua JM, Wong PC, Bian J. Hydrogen sulfide protects testicular germ cells against heat-induced injury. Nitric. Oxide. (2015) 46:165–71. doi: 10.1016/j.niox.2014.10.005
33. Li J, Li Y, Du Y, Mou K, Sun H, Zang Y, et al. Endogenous hydrogen sulfide as a mediator of vas deferens smooth muscle relaxation. Fertil. Steril. (2011) 95:1833–5. doi: 10.1016/j.fertnstert.2010.11.001
34. Gao DD, Xu JW, Qin WB, Peng L, Qiu ZE, Wang LL, et al. Cellular mechanism underlying hydrogen sulfide mediated epithelial K+ Secretion in rat epididymis. Front Physiol. (2019) 9:1886. doi: 10.3389/fphys.2018.01886
35. Dean RC, Lue TF. Physiology of penile erection and pathophysiology of erectile dysfunction. Urol. Clin North. Am. (2005) 32:379–v. doi: 10.1016/j.ucl.2005.08.007
36. Geng B, Chang L, Pan C, Qi Y, Zhao J, Pang Y, et al. Endogenous hydrogen sulfide regulation of myocardial injury induced by isoproterenol. Biochem Biophys Res Commun. (2004) 318:756–63. doi: 10.1016/j.bbrc.2004.04.094
37. Srilatha B, Adaikan PG, Moore PK. Possible role for the novel gasotransmitter hydrogen sulphide in erectile dysfunction–a pilot study. Eur J Pharmacol. (2006) 535:280–2. doi: 10.1016/j.ejphar.2006.02.001
38. Jupiter RC, Yoo D, Pankey EA, Reddy VV, Edward JA, Polhemus DJ, et al. Analysis of erectile responses to H2S donors in the anesthetized rat. Am J Physiol Heart. Circ Physiol. (2015) 309:H835–43. doi: 10.1152/ajpheart.00293.2015
39. Meng J, Ganesan Adaikan P, Srilatha B. Hydrogen sulfide promotes nitric oxide production in corpus cavernosum by enhancing expression of endothelial nitric oxide synthase. Int J Impot. Res. (2013) 25:86–90. doi: 10.1038/ijir.2012.39
40. Aydinoglu F, Adıbelli EÖ, Yılmaz-Oral D, Ogulener N. Involvement of RhoA/Rho-kinase in l-cysteine/H2S pathway-induced inhibition of agonist-mediated corpus cavernosal smooth muscle contraction. Nitric. Oxide. (2019) 85:54–60. doi: 10.1016/j.niox.2019.02.001
41. Abd Elmoneim H, Sharabi F, Mohy El Din M, Louedec L, Norel X, Senbel A. Potassium channels modulate the action but not the synthesis of hydrogen sulfide in rat corpus cavernosum. Life Sci. (2017) 189:39–43. doi: 10.1016/j.lfs.2017.09.013
42. Yilmaz E, Kaya-Sezginer E, Yilmaz-Oral D, Cengiz T, Bayatli N, Gur S. Effects of hydrogen sulphide donor, sodium hydrosulphide treatment on the erectile dysfunction in L-NAME-induced hypertensive rats. Andrologia. (2019) 51:e13240. doi: 10.1111/and.13240
43. Mostafa T, Rashed L, Nabil N, Abo-Sief AF, Mohamed MM, Omar MS. Cavernosal hydrogen sulfide levels are associated with nitric oxide and hemeoxygenase levels in diabetic rats. Int J Impot. Res. (2019) 31:105–10. doi: 10.1038/s41443-018-0084-9
44. Zeng Q, He S, Chen F, Wang L, Zhong L, Hui J, et al. Administration of H2S improves erectile dysfunction by inhibiting phenotypic modulation of corpus cavernosum smooth muscle in bilateral cavernous nerve injury rats. Nitric. Oxide. (2021) 107:1–10. doi: 10.1016/j.niox.2020.11.003
45. Qabazard B, Yousif MHM, Phillips OA. Alleviation of impaired reactivity in the corpus cavernosum of STZ-diabetic rats by slow-release H2S donor GYY4137. Int J Impot. Res. (2019) 31:111–8. doi: 10.1038/s41443-018-0083-x
46. Qabazard B, Yousif M, Mousa A, Phillips OA. GYY4137 attenuates functional impairment of corpus cavernosum and reduces fibrosis in rats with STZ-induced diabetes by inhibiting the TGF-β1/Smad/CTGF pathway. Biomed Pharmacother. (2021) 138:111486. doi: 10.1016/j.biopha.2021.111486
47. Fukami K, Sekiguchi F, Yasukawa M, Asano E, Kasamatsu R, Ueda M, et al. Functional upregulation of the H2S/Cav3.2 channel pathway accelerates secretory function in neuroendocrine-differentiated human prostate cancer cells. Biochem Pharmacol. (2015) 97:300–9. doi: 10.1016/j.bcp.2015.08.005
48. Zhao Y, Zhang WD, Liu XQ, Zhang PF, Hao YN, Li L, et al. Hydrogen Sulfide and/or Ammonia Reduces Spermatozoa Motility through AMPK/AKT Related Pathways. Sci Rep. (2016) 6:37884. doi: 10.1038/srep37884
49. Bozkurt M, Degirmentepe RB, Polat EC, Yildirim F, Sonmez K, Cekmen M, et al. Protective effect of hydrogen sulfide on experimental testicular ischemia reperfusion in rats. J Pediatr Urol. (2020) 16:40.e1–8. doi: 10.1016/j.jpurol.2019.10.006
50. Li S, Ma Y, Ye S, Su Y, Hu D, Xiao F. Endogenous hydrogen sulfide counteracts polystyrene nanoplastics-induced mitochondrial apoptosis and excessive autophagy via regulating Nrf2 and PGC-1α signaling pathway in mouse spermatocyte-derived GC-2spd(ts) cells. Food Chem Toxicol. (2022) 164:113071. doi: 10.1016/j.fct.2022.113071
51. Wang J, Wang J, Shen T, Hong R, Tang S, Zhao X. H2S catalysed by CBS regulates testosterone synthesis through affecting the sulfhydrylation of PDE. J Cell Mol Med. (2021) 25:3460–8. doi: 10.1111/jcmm.16428
52. Wang J, Wang W, Li S, Han Y, Zhang P, Meng G, et al. Hydrogen sulfide as a potential target in preventing spermatogenic failure and testicular dysfunction. Antioxid Redox Signal. (2018) 28:1447–62. doi: 10.1089/ars.2016.6968
53. Pintus E, Jovičić M, Kadlec M, Ros-Santaella JL. Divergent effect of fast- and slow-releasing HS donors on boar spermatozoa under oxidative stress. Sci Rep. (2020) 10:6508. doi: 10.1038/s41598-020-63489-4
54. Xia YQ, Ning JZ, Cheng F, Yu WM, Rao T, Ruan Y, et al. GYY4137 a H2S donor, attenuates ipsilateral epididymis injury in experimentally varicocele-induced rats via activation of the PI3K/Akt pathway. Iran. J Basic. Med Sci. (2019) 22:729–35. doi: 10.22038/ijbms.2019.30588.7372
55. Li Y, Zang Y, Fu S, Zhang H, Gao L, Li J. H2S relaxes vas deferens smooth muscle by modulating the large conductance Ca2+ -activated K+ (BKCa) channels via a redox mechanism. J Sex Med. (2012) 9:2806–13. doi: 10.1111/j.1743-6109.2012.02879.x
56. Sullivan ME, Thompson CS, Dashwood MR, Khan MA, Jeremy JY, Morgan RJ, et al. Nitric oxide and erection: is erectile dysfunction another manifestation of vascular disease? Cardiovasc Res. (1999) 43:6580–65. doi: 10.1016/S0008-6363(99)00135-2
57. Förstermann U, Gath I, Schwarz P, Closs EI, Kleinert H. Isoforms of nitric oxide synthase. Properties, cellular distribution and expressional control. Biochem Pharmacol. (1995) 50:1321–32. doi: 10.1016/0006-2952(95)00181-6
58. Murad F. Nitric oxide and cyclic guanosine monophosphate signaling in the eye. Can J Ophthalmol. (2008) 43:291–4. doi: 10.1139/I08-044
59. Hosoki R, Matsuki N, Kimura H. The possible role of hydrogen sulfide as an endogenous smooth muscle relaxant in synergy with nitric oxide. Biochem Biophys Res Commun. (1997) 237:527–31. doi: 10.1006/bbrc.1997.6878
60. Bucci M, Papapetropoulos A, Vellecco V, Zhou Z, Pyriochou A, Roussos C, et al. Hydrogen sulfide is an endogenous inhibitor of phosphodiesterase activity, Arterioscler. Thromb Vasc Biol. (2010) 30:1998–2004. doi: 10.1161/ATVBAHA.110.209783
61. La Fuente JM, Fernández A, Pepe-Cardoso AJ, Martínez-Salamanca JI, Louro N, Angulo J. L-cysteine/hydrogen sulfide pathway induces cGMP-dependent relaxation of corpus cavernosum and penile arteries from patients with erectile dysfunction and improves arterial vasodilation induced by PDE5 inhibition. Eur J Pharmacol. (2019) 863:172675. doi: 10.1016/j.ejphar.2019.172675
62. Nelson MT, Quayle JM. Physiological roles and properties of potassium channels in arterial smooth muscle. Am J Physiol. (1995) 268:C799–822. doi: 10.1152/ajpcell.1995.268.4.C799
63. Christ GJ, Spray DC, Brink PR. Characterization of K currents in cultured human corporal smooth muscle cells. J Androl. (1993) 14:319–28. doi: 10.1002/j.1939-4640.1993.tb00392.x
64. Lee SY, Lee CO. Inhibition of Na+–K+ pump and L-type Ca2+ channel by glibenclamide in Guinea pig ventricular myocytes. J Pharmacol Exp Ther. (2005) 312:61–8. doi: 10.1124/jpet.104.074369
65. Ridley AJ. Rho family proteins: coordinating cell responses. Trends. Cell Biol. (2001) 11:471–7. doi: 10.1016/S0962-8924(01)02153-5
66. Feng J, Ito M, Ichikawa K, Isaka N, Nishikawa M, Hartshorne DJ, et al. Inhibitory phosphorylation site for Rho-associated kinase on smooth muscle myosin phosphatase, J. Biol Chem. (1999) 274:37385–90. doi: 10.1074/jbc.274.52.37385
67. Somlyo AP, Somlyo AV. Signal transduction through the RhoA/Rho-kinase pathway in smooth muscle. J Muscle. Res Cell Motil. (2004) 25:613–5. doi: 10.1023/B:JURE.0000038362.84697.c0
68. Chitaley K, Wingard CJ, Clinton Webb R, Branam H, Stopper VS, Lewis RW, et al. Antagonism of Rho-kinase stimulates rat penile erection via a nitric oxide-independent pathway. Nat Med. (2001) 7:119–22. doi: 10.1038/83258
69. Aydinoglu F, Ogulener N. The role of arachidonic acid/cyclooxygenase cascade, phosphodiesterase IV and Rho-kinase in H2S-induced relaxation in the mouse corpus cavernosum, Pharmacol. Rep. (2017) 69:610–5. doi: 10.1016/j.pharep.2017.02.018
70. Powell CR, Dillon KM, Matson JB. A review of hydrogen sulfide (HS) donors: Chemistry and potential therapeutic applications. Biochem Pharmacol. (2018) 149:110–23. doi: 10.1016/j.bcp.2017.11.014
71. Besiroglu H, Otunctemur A, Ozbek E. The relationship between metabolic syndrome, its components, and erectile dysfunction: a systematic review and a meta-analysis of observational studies. J Sex Med. (2015) 12:1309–18. doi: 10.1111/jsm.12885
72. Salonia A, Burnett AL, Graefen M, Hatzimouratidis K, Montorsi F, Mulhall JP, et al. Prevention and management of postprostatectomy sexual dysfunctions part 2: recovery and preservation of erectile function, sexual desire, and orgasmic function. Eur Urol. (2012) 62:273–86. doi: 10.1016/j.eururo.2012.04.047
73. Cirino G, Sorrentino R, di Villa Bianca R.d, Popolo A, Palmieri A, Imbimbo C, et al. Involvement of beta 3-adrenergic receptor activation via cyclic GMP- but not NO-dependent mechanisms in human corpus cavernosum function. Proc Natl Acad Sci U. S. A. (2003) 100:5531–6. doi: 10.1073/pnas.0931347100
74. Mitidieri E, Tramontano T, Gurgone D, Imbimbo C, Mirone V, Fusco F, et al. β3 adrenergic receptor activation relaxes human corpus cavernosum and penile artery through a hydrogen sulfide/cGMP-dependent mechanism. Pharmacol Res. (2017) 124:100–4. doi: 10.1016/j.phrs.2017.07.025
75. Gur S, Peak T, Yafi FA, Kadowitz PJ, Sikka SC, Hellstrom WJ. Mirabegron causes relaxation of human and rat corpus cavernosum: could it be a potential therapy for erectile dysfunction? BJU. Int. (2016) 118:464–74. doi: 10.1111/bju.13515
76. La Fuente JM, Sevilleja-Ortiz A, García-Rojo E, El Assar M, Fernández A, Pepe-Cardoso AJ, et al. Erectile dysfunction is associated with defective L-cysteine/hydrogen sulfide pathway in human corpus cavernosum and penile arteries. Eur J Pharmacol. (2020) 884:173370. doi: 10.1016/j.ejphar.2020.173370
77. Zhong L, Ding W, Zeng Q, He B, Zhang H, Wang L, et al. Sodium tanshinone IIA sulfonate attenuates erectile dysfunction in rats with hyperlipidemia. Oxid Med Cell Longev. (2020) 2020:7286958. doi: 10.1155/2020/7286958
78. Shukla N, Rossoni G, Hotston M, Sparatore A, Del Soldato P, Tazzari V, et al. Effect of hydrogen sulphide-donating sildenafil (ACS6) on erectile function and oxidative stress in rabbit isolated corpus cavernosum and in hypertensive rats. BJU. Int. (2009) 103:1522–9. doi: 10.1111/j.1464-410X.2009.08415.x
79. Yetik-Anacak G, Dereli MV, Sevin G, Ozzayım O, Erac Y, Ahmed A. Resveratrol stimulates hydrogen sulfide (H2S) formation to relax murine corpus cavernosum. J Sex Med. (2015) 12:2004–12. doi: 10.1111/jsm.12993
80. Dayar E, Kara E, Yetik-Anacak G, Hocaoglu N, Bozkurt O, Gidener S, et al. Do penile haemodynamics change in the presence of hydrogen sulphide (H2 S) donor in metabolic syndrome-induced erectile dysfunction? Andrologia. (2018) 50(3):10.1111/and.12885. doi: 10.1111/and.12885
81. Yilmaz-Oral D, Kaya-Sezginer E, Oztekin CV, Bayatli N, Lokman U, Gur S. Evaluation of combined therapeutic effects of hydrogen sulfide donor sodium hydrogen sulfide and phosphodiesterase type-5 inhibitor tadalafil on erectile dysfunction in a partially bladder outlet obstructed rat model. Neurourol. Urodyn. (2020) 39:1087–97. doi: 10.1002/nau.24333
82. Desai K, McManus JM, Sharifi N. Hormonal therapy for prostate cancer. Endocr. Rev. (2021) 42:354–73. doi: 10.1210/endrev/bnab002
83. Lonergan PE, Tindall DJ. Androgen receptor signaling in prostate cancer development and progression. J Carcinog. (2011) 10:20. doi: 10.4103/1477-3163.83937
84. Liu S, Alabi BR, Yin Q, Stoyanova T. Molecular mechanisms underlying the development of neuroendocrine prostate cancer. Semin Cancer. Biol. (2022) 86:57–68. doi: 10.1016/j.semcancer.2022.05.007
85. Mariot P, Vanoverberghe K, Lalevee N, Rossier MF, Prevarskaya N. Prevarskaya, Overexpression of an alpha 1H (Cav3.2) T-type calcium channel during neuroendocrine differentiation of human prostate cancer cells. J Biol Chem. (2002) 277:10824–33. doi: 10.1074/jbc.M108754200
86. Gackière F, Bidaux G, Delcourt P, Van Coppenolle F, Katsogiannou M, Dewailly E, et al. CaV3.2 T-type calcium channels are involved in calciumdependent secretion of neuroendocrine prostate cancer cells. J Biol Chem. (2008) 283:10162–73. doi: 10.1074/jbc.M707159200
87. Ngowi EE, Afzal A, Sarfraz M, Khattak S, Zaman SU, Khan NH, et al. Role of hydrogen sulfide donors in cancer development and progression. Int J Biol Sci. (2021) 17:73–88. doi: 10.7150/ijbs.47850
88. Chattopadhyay M, Kodela R, Nath N, Dastagirzada YM, Velázquez-Martínez CA, Boring D, et al. Hydrogen sulfide-releasing NSAIDs inhibit the growth of human cancer cells: a general property and evidence of a tissue type-independent effect. Biochem Pharmacol. (2012) 83:715–22. doi: 10.1016/j.bcp.2011.12.018
89. Bigagli E, Luceri C, De Angioletti M, Chegaev K, D’Ambrosio M, Riganti C, et al. New NO- and H2S-releasing doxorubicins as targeted therapy against chemoresistance in castration-resistant prostate cancer: in vitro and in vivo evaluations. Invest. New. Drugs. (2018) 36:985–98. doi: 10.1007/s10637-018-0590-0
90. Zhou B, Jia BX, Zhang MJ, Tan YJ, Liang WY, Gan X, et al. Zn2+-interference and H2S-mediated gas therapy based on ZnS-tannic acid nanoparticles synergistic enhancement of cell apoptosis for specific treatment of prostate cancer. Colloids. Surf. B Biointerfaces. (2023) 226:113313. doi: 10.1016/j.colsurfb.2023.113313
91. Zhang W, Zhao Y, Zhang P, Hao Y, Yu S, Min L, et al. Decrease in male mouse fertility by hydrogen sulfide and/or ammonia can Be inheritable. Chemosphere. (2018) 194:147–57. doi: 10.1016/j.chemosphere.2017.11.164
92. Moustafa A. Changes in nitric oxide, carbon monoxide, hydrogen sulfide and male reproductive hormones in response to chronic restraint stress in rats. Free. Radic Biol Med. (2021) 162:353–66. doi: 10.1016/j.freeradbiomed.2020.10.315
93. Ameli M, Ahmadzadeh M, Khajavi A, Nabizadeh M. Evaluation of the success rate and complications of conventional varicocelectomy: Do we need microscopic surgery really? Urologia. (2019) 86:23–6. doi: 10.1177/0391560318758938
94. Lorian K, Kadkhodaee M, Kianian F, Abdi A, Ranjbaran M, Ashabi G, et al. Long-term NaHS administration reduces oxidative stress and apoptosis in a rat model of left-side varicocele. Andrologia. (2020) 52:e13496. doi: 10.1111/and.13496
95. Shafie A, Kianian F, Ashabi G, Kadkhodaee M, Ranjbaran M, Hajiaqaei M, et al. Beneficial effects of combination therapy with testosterone and hydrogen sulfide by reducing oxidative stress and apoptosis: Rat experimental varicocele model. Int J Reprod BioMed. (2022) 20:941–54. doi: 10.18502/ijrm.v20i11.12362
96. Fallahzadeh AR, Rezaei Z, Rahimi HR, Barmak MJ, Sadeghi H, Mehrabi S, et al. Evaluation of the effect of pentoxifylline on cisplatin-induced testicular toxicity in rats. Toxicol Res. (2017) 33:255–63. doi: 10.5487/TR.2017.33.3.255
97. Azarbarz N, Shafiei Seifabadi Z, Moaiedi MZ, Mansouri E. Assessment of the effect of sodium hydrogen sulfide (hydrogen sulfide donor) on cisplatin-induced testicular toxicity in rats. Environ Sci pollut Res Int. (2020) 27:8119–28. doi: 10.1007/s11356-019-07266-5
98. Amereh F, Babaei M, Eslami A, Fazelipour S, Rafiee M. The emerging risk of exposure to nano(micro)plastics on endocrine disturbance and reproductive toxicity: from a hypothetical scenario to a global public health challenge. Environ pollut. (2020) 261:114158. doi: 10.1016/j.envpol.2020.114158
99. Deng Y, Yan Z, Shen R, Huang Y, Ren H, Zhang Y. Enhanced reproductive toxicities induced by phthalates contaminated microplastics in male mice (Mus musculus). J Hazard. Mater. (2021) 406:124644. doi: 10.1016/j.jhazmat.2020.124644
100. Ohlander SJ, Lindgren MC, Lipshultz LI. Testosterone and male infertility. Urol. Clin North. Am. (2016) 43:195–202. doi: 10.1016/j.ucl.2016.01.006
101. Shi H, Li QY, Li H, Wang HY, Fan CX, Dong QY, et al. ROS-induced oxidative stress is a major contributor to sperm cryoinjury. Hum Reprod. (2023) 39(2):310–25. doi: 10.1093/humrep/dead250
102. Wiliński B, Wiliński J, Gajda M, Jasek E, Somogyi E, Głowacki M, et al. Sodium hydrosulfide exerts a transitional attenuating effect on spermatozoa migration in vitro. Folia. Biol (Krakow). (2015) 63:145–9. doi: 10.3409/fb63_2.145
103. Kadlec M, Pintus E, Ros-Santaella JL. The interaction of NO and H2S in boar spermatozoa under oxidative stress. Anim (Basel). (2022) 12:602. doi: 10.3390/ani12050602
104. Pintus E, Chinn AF, Kadlec M, García-Vázquez FA, Novy P, Matson JB, et al. N-thiocarboxyanhydrides, amino acid-derived enzyme-activated H2S donors, enhance sperm mitochondrial activity in presence and absence of oxidative stress. BMC. Vet Res. (2023) 19:52. doi: 10.1186/s12917-023-03593-5
105. Jones RC, Murdoch RN. Regulation ofthe motility and metabolism of spermatozoa for storage in the epididymis of eutherian and marsupial mammals. Reprod Fertil. Dev. (1996) 8:553–68. doi: 10.1071/RD9960553
106. D’Ancona C, Haylen B, Oelke M, Abranches-Monteiro L, Arnold E, Goldman H, et al. The International Continence Society (ICS) report on the terminology for adult male lower urinary tract and pelvic floor symptoms and dysfunction. Neurourol. Urodyn. (2019) 38:433–77. doi: 10.1002/nau.23897
107. Abdelmoteleb H, Jefferies ER, Drake MJ. Assessment and management of male lower urinary tract symptoms (LUTS). Int J Surg. (2016) 25:164–71. doi: 10.1016/j.ijsu.2015.11.043
108. De Nunzio C, Lombardo R, Tema G, Tubaro A. Erectile dysfunction and lower urinary tract symptoms. Curr Uro.l Rep. (2018) 19:61. doi: 10.1007/s11934-018-0817-9
109. Gkatzoudi C, Bouloukaki I, Mamoulakis C, Lionis C, Tsiligianni I. Evaluation of lower urinary tract symptoms in males and urinary incontinence in females in primary health care in Greece. Medicina (Kaunas). (2024) 60:389. doi: 10.3390/medicina60030389
110. Przydacz M, Osman N, De Cillis S, Guillot-Tantay C, Herve F, Hüsch T, et al. Overactive bladder negatively affects erectile function and promotes premature ejaculation: findings from large representative population-level study. World. J Urol. (2024) 42:139. doi: 10.1007/s00345-024-04841-5
111. Gomelsky A, Kelly EF, Dalton DC. Combination treatment for male lower urinary tract symptoms with anticholinergic and alpha-blockers. Curr Opin Urol. (2018) 28:277–83. doi: 10.1097/MOU.0000000000000489
112. de Groat WC, Griffiths D, Yoshimura N. Neural control of the lower urinary tract. Compr Physiol. (2015) 5:327–96. doi: 10.1002/cphy.c130056
113. Patacchini R, Santicioli P, Giuliani S, Maggi CA. Hydrogen sulfide (H2S) stimulates capsaicin-sensitive primary afferent neurons in the rat urinary bladder. Br J Pharmacol. (2004) 142:31–4. doi: 10.1038/sj.bjp.0705764
114. Fernandes VS, Ribeiro AS, Barahona MV, Orensanz LM, Martínez-Sáenz A, Recio P, et al. Hydrogen sulfide mediated inhibitory neurotransmission to the pig bladder neck: role of KATP channels, sensory nerves and calcium signaling. J Urol. (2013) 190:746–56. doi: 10.1016/j.juro.2013.02.103
115. Fernandes VS, Xin W, Petkov GV. Novel mechanism of hydrogen sulfide-induced Guinea pig urinary bladder smooth muscle contraction: role of BK channels and cholinergic neurotransmission. Am J Physiol Cell Physiol. (2015) 309:C107–16. doi: 10.1152/ajpcell.00021.2015
116. Fernandes VS, Ribeiro AS, Martínez MP, Orensanz LM, Barahona MV, Martínez-Sáenz A, et al. Endogenous hydrogen sulfide has a powerful role in inhibitory neurotransmission to the pig bladder neck. J Urol. (2013) 189:1567–73. doi: 10.1016/j.juro.2012.10.006
117. Mitidieri E, Pecoraro A, Esposito E, Brancaleone V, Turnaturi C, Napolitano L, et al. β3 relaxant effect in human bladder involves cystathionine γ-lyase-derived urothelial hydrogen sulfide. Antioxidants (Basel). (2022) 11:1480. doi: 10.3390/antiox11081480
118. d’Emmanuele diVBR, Mitidieri E, Fusco F, Russo A, Pagliara V, Tramontano T, et al. Urothelium muscarinic activation phosphorylates CBS(Ser227) via cGMP/PKG pathway causing human bladder relaxation through H2S production. Sci Rep. (2016) 6:31491. doi: 10.1038/srep31491
119. Ribeiro ASF, Fernandes VS, Martínez-Sáenz A, Martínez P, Barahona MV, Orensanz LM, et al. Powerful relaxation of phosphodiesterase type 4 inhibitor rolipram in the pig and human bladder neck. J Sex Med. (2014) 11:930–41. doi: 10.1111/jsm.12456
120. Fusco F, D’Anzeo G, Sessa A, Pace G, Rossi A, Capece M, et al. BPH/LUTS and ED: common pharmacological pathways for a common treatment. J Sex Med. (2013) 10:2382–93. doi: 10.1111/jsm.12261
121. Fusco F, di Villa Bianca R.d, Mitidieri E, Cirino G, Sorrentino R, Mirone V. Sildenafil effect on the human bladder involves the L-cysteine/hydrogen sulfide pathway: a novel mechanism of action of phosphodiesterase type 5 inhibitors. Eur Urol. (2012) 62:1174–80. doi: 10.1016/j.eururo.2012.07.025
122. Agis-Torres Á, Recio P, López-Oliva ME, Martínez MP, Barahona MV, Benedito S, et al. Phosphodiesterase type 4 inhibition enhances nitric oxide- and hydrogen sulfide-mediated bladder neck inhibitory neurotransmission. Sci Rep. (2018) 8:4711. doi: 10.1038/s41598-018-22934-1
123. Jeremy JY, Rowe D, Emsley AM, Newby AC. Nitric oxide and vascular smooth muscle cell proliferation. Cardiovasc Res. (1999) 43:580–94. doi: 10.1016/S0008-6363(99)00171-6
124. Koupparis AJ, Jeremy JY, Muzaffar S, Angelini GD, Shukla N. Sildenafil inhibits the formation of superoxide and the expression of gp47phox NADPH oxidase induced by the thromboxane A2 mimetic, U46619, in corpus cavernosal smooth muscle cells. BJU. Int. (2005) 96:423–7. doi: 10.1111/j.1464-410X.2005.05643.x
125. Shukla N, Jones R, Persad R, Angelini GD, Jeremy JY. Effect of sildenafil citrate and a nitric oxide donating sildenafil derivative, NCX 911, on cavernosal relaxation and superoxide formation in hypercholesterolaemic rabbits. Eur Pharmacol. (2005) 517:224–31. doi: 10.1016/j.ejphar.2005.05.012
126. Jeremy JY, Jones RA, Koupparis AJ, Hotston M, Persad R, Shukla N. Reactive oxygen species and erectile dysfunction: possible role of NADPH oxidase. Int J Impot. Res. (2007) 19:265–80. doi: 10.1038/sj.ijir.3901523
127. Muzaffar S, Jeremy JY, Angelini GD, Stuart Smith K, Shukla N. The role of the endothelium and nitric oxide synthases in modulating superoxide formation induced by endotoxin and cytokines in porcine pulmonary arteries. Thorax. (2003) 58:598–604. doi: 10.1136/thorax.58.7.598
128. Muzaffar S, Shukla N, Angelini GD, Jeremy JY. Nitroaspirins and SIN-1, but not aspirin, inhibit the expression of endotoxin- and cytokine- induced NADPH oxidase in vascular smooth muscle cells from pig pulmonary arteries. Circulation. (2004) 110:1140–7. doi: 10.1161/01.CIR.0000139851.50067.E4
129. Hotston M, Jeremy JY, Bloor J, Greaves NS, Persad R, Angelini G, et al. Homocysteine and copper interact to promote type 5 phosphodiesterase expression in rabbit cavernosal smooth muscle cells. Asian. J Androl. (2008) 10:905–13. doi: 10.1111/ajan.2008.10.issue-6
130. Muzaffar S, Shukla N, Bond M, Newby AC, Angelini GD, Sparatore A, et al. Exogenous hydrogen sulfide inhibits superoxide formation, NOX-1 expression and Rac (1) activity in human vascular smooth muscle cells. J Vasc Res. (2008) 45:521–8. doi: 10.1159/000129686
131. Bibli SI, Yang G, Zhou Z, Wang R, Topouzis S, Papapetropoulos A. Role of cGMP in hydrogen sulfide signaling. Nitric. Oxide. (2015) 46:7–13. doi: 10.1016/j.niox.2014.12.004
132. Muzaffar S, Shukla N, Bond M, Newby AC, Angelini GD, Jeremy JY. Acute inhibition of superoxide formation and Rac (1) activation by nitric oxide and iloprost in human vascular smooth muscle cells in response to the thromboxane A (2) analogue, U46619. Prostaglandins Leukotr. Essent Fatty Acids. (2008) 78:247–55. doi: 10.1016/j.plefa.2008.01.008
133. Song Y, Qu Y, Mao C, Zhang R, Jiang D, Sun X. Post-translational modifications of Keap1: the state of the art. Front Cell Dev Biol. (2024) 11:1332049. doi: 10.3389/fcell.2023.1332049
134. Mustafa AK, Sikka G, Gazi SK, Steppan J, Jung SM, Bhunia AK, et al. Hydrogen sulfide as endothelium-derived hyperpolarizing factor sulfhydrates potassium channels. Circulation. Res. (2011) 109:1259–68. doi: 10.1161/CIRCRESAHA.111.240242
135. Sun Y, Huang Y, Yu W, Chen S, Yao Q, Zhang C, et al. Sulfhydration-associated phosphodiesterase 5A dimerization mediates vasorelaxant effect of hydrogen sulfide. Oncotarget. (2017) 8:31888–900. doi: 10.18632/oncotarget.v8i19
136. Hughes MN, Centelles MN, Moore KP. Making and working with hydrogen sulfide: the chemistry and generation of hydrogen sulfide in vitro and its measurement in vivo: a review. Free. Radic Biol Med. (2009) 47:1346–53. doi: 10.1016/j.freeradbiomed.2009.09.018
Keywords: erectile dysfunction, H2S, testis, prostate cancer, oxidative stress, bladder
Citation: Song Y, Mao C, Zhong Q, Zhang R, Jiang D and Sun X (2024) Role of hydrogen sulfide in the male reproductive system. Front. Endocrinol. 15:1377090. doi: 10.3389/fendo.2024.1377090
Received: 26 January 2024; Accepted: 16 May 2024;
Published: 30 May 2024.
Edited by:
Guiming Liu, Northeast Ohio Medical University, United StatesReviewed by:
Emma Mitidieri, University of Naples Federico II, ItalyRaffaella Sorrentino, University of Naples Federico II, Italy
Copyright © 2024 Song, Mao, Zhong, Zhang, Jiang and Sun. This is an open-access article distributed under the terms of the Creative Commons Attribution License (CC BY). The use, distribution or reproduction in other forums is permitted, provided the original author(s) and the copyright owner(s) are credited and that the original publication in this journal is cited, in accordance with accepted academic practice. No use, distribution or reproduction is permitted which does not comply with these terms.
*Correspondence: Xutao Sun, c3VueHV0YW9AaGxqdWNtLm5ldA==; Deyou Jiang, amlhbmdkZXlvdUAxMjYuY29t
†These authors have contributed equally to this work