- 1Department of Anatomy and Cell Biology, University of Illinois at Chicago, Chicago, IL, United States
- 2Skaggs Pharmaceutical Sciences Center, University of Arizona, Tucson, AZ, United States
Increasing evidence suggests that female individuals have a higher Alzheimer’s disease (AD) risk associated with post-menopausal loss of circulating estradiol (E2). However, clinical data are conflicting on whether E2 lowers AD risk. One potential contributing factor is APOE. The greatest genetic risk factor for AD is APOE4, a factor that is pronounced in female individuals post-menopause. Clinical data suggests that APOE impacts the response of AD patients to E2 replacement therapy. However, whether APOE4 prevents, is neutral, or promotes any positive effects of E2 is unclear. Therefore, our goal was to determine whether APOE modulates the impact of E2 on behavior and AD pathology in vivo. To that end, mice that express human APOE3 (E3FAD) or APOE4 (E4FAD) and overproduce Aβ42 were ovariectomized at either 4 months (early) or 8 months (late) and treated with vehicle or E2 for 4 months. In E3FAD mice, we found that E2 mitigated the detrimental effect of ovariectomy on memory, with no effect on Aβ in the early paradigm and only improved learning in the late paradigm. Although E2 lowered Aβ in E4FAD mice in the early paradigm, there was no impact on learning or memory, possibly due to higher Aβ pathology compared to E3FAD mice. In the late paradigm, there was no effect on learning/memory and Aβ pathology in E4FAD mice. Collectively, these data support the idea that, in the presence of Aβ pathology, APOE impacts the response to E2 supplementation post-menopause.
1 Introduction
Sex is a major risk factor for Alzheimer’s disease (AD), with women accounting for ~60% of patients. Several pathways could contribute to the higher AD risk in female individuals, including sexual dimorphisms in brain function (1) and X chromosome-linked genes (2) that could impact vascular function (3) and neuroinflammation (3). In addition, the loss of sex hormones, particularly estrogen (E2), during menopause has emerged as a key component—for example, AD risk is highest in post-menopausal women (4, 5), oophorectomy increases dementia risk (6), and ovariectomy (OVX) disrupts behavior in mice that overproduce Aβ42 via familial AD (FAD) mutations (7–9). Estrogen replacement therapy (ERT) using E2 or other estrogens may lower AD risk or progression post-menopause. In support, observational studies identified that hormone therapy that results in high E2 is associated with a lower AD risk (10–14). In addition, E2 has been shown to mitigate the detrimental impact of OVX on behavior in FAD mice (7–9). However, clinical studies have produced conflicting data, i.e., whether E2 is beneficial or neutral or detrimental for cognition (15) and AD risk (16, 17). Potential confounding variables include timing, dose, and E2 formulation (18). Human APOE may play a key role in responses to E2.
APOE is the greatest genetic risk factor for AD, with APOE4 increasing AD risk up to 15-fold compared to APOE3. Importantly, AD risk is higher in female APOE4 carriers compared to male individuals (19, 20), which is particularly pronounced at older ages (21), suggesting a role of menopause. Those data seemingly support that E2 would be efficacious in APOE4 carriers. However, it is unclear whether E2 is beneficial or detrimental to APOE4 carriers vs. non-carriers (17). In vivo data are limited to findings that OVX lowered hippocampal spine density in APOE3-targeted replacement (TR) mice with no effect in APOE4-TR mice (22) and E2 modulated hippocampal plasticity in APOE4-TR mice (23). Thus, further research using transgenic models could aid in understanding the impact of APOE on both OVX and E2.
The goal of this study was to determine whether APOE modulates the impact of E2 on behavior and AD pathology in vivo. To address this, we used EFAD mice that overproduce Aβ42 and express human APOE3 (E3FAD) or APOE4 (E4FAD) (24). Behavior and Aβ pathology were assessed in mice that underwent either sham surgery or OVX with vehicle and E2 treatment: (1) at 8 months of age in mice treated for 4 months after OVX (early OVX) and (2) at 12 months of age in mice treated for 4 months after OVX (late OVX).
2 Materials and methods
2.1 Animals
All experiments followed the University of Illinois at Chicago Animal Care Committee protocols. EFAD mice express five familial AD (FAD) mutations and human APOE. Two groups of EFAD (5×FAD+/-/human APOE+/+) mice were used: female E3FAD and female E4FAD mice (24). The mice were ear-tagged during genotyping, and the investigators were blinded about APOE, treatment, and age. We initially designed this study to test the interactive effect of APOE and treatments (sham, OVX -/+ E2) on learning/memory and Aβ pathology in EFAD mice. However, due to the COVID-19 pandemic, we had to restructure the mouse enrollment for this study due to personnel restrictions and perform the surgeries and behavioral experiments for the E3FAD and E4FAD mice separately.
2.2 Surgery and treatments
EFAD mice were acclimatized to plain hydrogel in place of drinking water 3 days prior to OVX. Bilateral OVX or sham surgery was performed on female E3FAD and E4FAD mice as described previously (25, 26) at either 4 or 8 months of age. Immediately after OVX, the mice were treated with hydrogel with or without 13.9 μg/mL β-estradiol (E2). Each mouse consumes ~4.5 mL hydrogel/day, resulting in a dose of 2.5 mg/kg/day that was selected based on previous studies (27–29). The mice were treated from 4 to 8 months or from 8 to 12 months of age.
2.3 Morris water maze
In the week prior to sacrifice, mouse behavior was tested using a modified Morris water maze (MWM) protocol (30). A four-trial shaping procedure was conducted 1 day prior to testing, during which the mouse was placed in various locations within the pool area (i.e., on a platform, near the platform, between the platform and a ring, and near the edge of the ring) to habituate the mice. After the shaping trials, mouse behavior was tested in acquisition trials for five consecutive days consisting of 4 × 1 min trials/day with latency to the platform recorded for each trial. After the last acquisition on day 5, a single probe trial was run with the platform removed, and the readouts included latency to platform and latency to target quadrant (previously described (31–33)). Both acquisition and probe trials were recorded and analyzed with ANY-maze software (Stoelting Co., Wood Dale, IL, USA).
2.4 Estrous stage identification, brain tissue harvest, and processing
At the end of the study, vaginal smear was used to determine whether the mouse was in proestrus/estrus or metestrus/diestrus (34, 35). The mice were then anesthetized with ketamine (100 mg/kg) and xylazine (5 mg/kg) via intraperitoneal injection and perfused. Uterine horns were dissected from the mice and weighed. Then, the brains were removed and harvested for biochemical and immunohistochemical analysis as described previously (36, 37). For biochemical analysis, the cortex was dissected from the hemi-brain, flash-frozen in liquid nitrogen, and then stored at -80°C. The hemi for immunohistochemical analysis was drop-fixed in 4% paraformaldehyde for 24 h and then transferred to phosphate-buffered saline containing 0.01% sodium azide until ready to be sectioned on a sliding microtome.
2.5 Biochemical analysis (insoluble Aβ)
Frozen cortices dissected from the mouse hemi-brains were homogenized in 70% formic acid at 1 mL/150 mg brain tissue and mixed by end-over-end rotation for 2 h at room temperature with vortexing. The samples were then centrifuged (100,000 × g, 1 hour at 4°C), and the formic acid-soluble fraction was neutralized (with 20 volumes of 1 M Tris base), aliquoted, and frozen at −80°C (38). Total protein in the formic-acid-soluble extracts was quantified using the Bradford assay, and formic-acid-soluble Aβ42 was measured by ELISA following the manufacturer’s instructions (24, 38, 39). A list of all the antibodies used is provided in Supplementary Table S1.
2.6 Immunohistochemical analysis
Serial sagittal brain sections (35 μm thick, 280 μm apart, ~0.24–3.44 mm lateral) from EFAD mice were immunostained for Aβ deposition (24, 33, 40). The stained sections were imaged at ×10 magnification with a Zeiss fluorescence microscope and analyzed for cortical area covered by MOAB-2 in the cortex using ImageJ by investigators blinded to treatment. A list of all the antibodies used is provided in Supplementary Table S1.
2.7 Data and statistical analysis
Supplementary File S1 (Data Sheet 1) is a Word file containing one table and two figures. Supplementary File S2 (Data Sheet 2) is an Excel file containing all raw data including the number of mice and statistical analysis. MWM acquisition data was analyzed by repeated-measure univariate general linear model for within-subject effects (independent variable: day and treatment). All other statistical analyses were conducted using univariate general linear models for between-subjects effects with treatment as the independent variable. All statistical tests were followed with Bonferroni’s post-hoc tests in in SPSS (IBM SPSS Statistics for Macintosh, Version 29.0.1.1); p < 0.05 was considered significant. Data are presented as scatter plots with the mean and standard error of the mean (SEM).
3 Results
The goal of our study was to determine the extent to which APOE modulates the impact of E2 on Aβ pathology and behavior in vivo using EFAD mice that overproduce Aβ42 and express human APOE3 (E3FAD) or APOE4 (E4FAD). Previously, we demonstrated that after OVX, shorter-term E2 treatment increased Aβ deposition in E4FAD mice but decreased Aβ deposition in E3FAD mice (41). These data raised important questions including whether APOE interacts with OVX to impact Aβ levels/pathology and behavior in EFAD mice (i.e., comparison of OVX vs. sham surgery) and if any changes are mitigated by E2. Therefore, we performed OVX or sham surgery in E3FAD and E4FAD mice at 4 months (early OVX) and then treated with E2 until 8 months of age and evaluated the behavior and Aβ pathology. Natural menopause in humans typically occurs at mid-life (42), during which there may be early stages of Aβ pathology without any signs of cognitive impairment (6, 43, 44). Thus, our goal was to select an age with lower levels of Aβ pathology, without behavioral impairments. Moreover, 4 months of age is at an early stage of Aβ pathology in female EFAD mice with no behavioral impairments (32, 36). We also evaluated the impact of OVX at later ages, i.e., at 8 months and E2 treatment from 8 to 12 months of age in EFAD mice.
3.1 E3FAD (early OVX): OVX-induced memory deficits were mitigated by E2 treatment
APOE3 is often used as a control group in APOE research in comparison with APOE4. Thus, we initially focused on the impact of OVX and E2 treatment on behavior and pathology in E3FAD mice due to the important implications for a large proportion of AD patients (19).
OVX results in disruption of uterine horn weight and estrous stage/cycling in vivo (45–48). Therefore, we first confirmed that OVX induced uterine atrophy and the effect of E2. As expected, in E3FAD mice, OVX decreased uterine horn weights by 43% compared to sham (p = 0.07), and E2 increased uterine horn weights by ~100% and 250% compared to sham mice and the OVX group, respectively (Figure 1A). We next evaluated the impact of OVX and E2 on estrous stage distribution among the mice. In general, mice in proestrus and estrus stages are associated with higher levels of circulating E2 in the periphery compared to mice in metestrus and diestrus stages. We confirmed that OVX decreased the proportion of mice in proestrus/estrus compared to sham group, and E2 treatment after OVX increased the proportion of mice in proestrus/estrus compared to OVX and sham group (Figure 1B; Supplementary Figure S2).
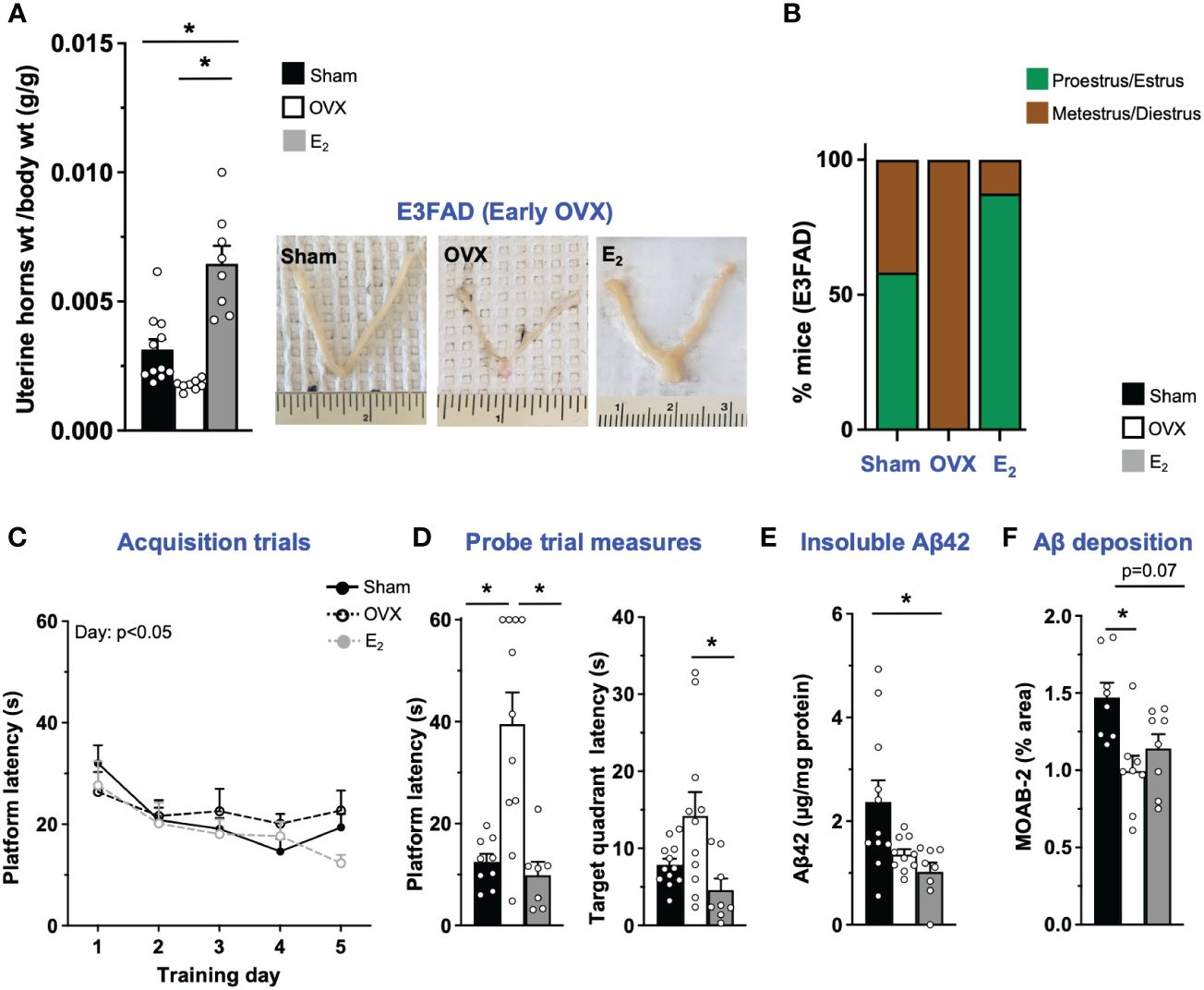
Figure 1 E3FAD (early OVX): OVX-induced memory deficits were mitigated by E2 treatment. Uterine horn weights were dissected from EFAD mice, and their weights were measured (A) to determine the effect of OVX and E2 treatment. (B) Estrous stages of E3FAD mice were determined before their sacrifice via vaginal cytology. Data was plotted as percentage of mice in proestrus/estrus or metestrus/diestrus. Learning and memory were assessed via Morris water maze. E3FAD mice were trained to determine the location of a platform over 5 days during the acquisition phase (C) and acquire the ability to remember the location of the platform (D) 24 h after the last training day probe trial. (E) Formic-acid-soluble Aβ42 was measured in cortical brain homogenates in E3FAD mice. Brain sections obtained from E3FAD mice were immunostained for Aβ using MOAB-2 and the percentage area quantified in the cortex (F). Data are expressed as mean ± SEM. Latency to platform during acquisition phase was analyzed by repeated-measure univariate general linear model for within-subject effects (independent variable: day and treatment). All other statistical analyses were conducted using univariate general linear models for between-subjects effects with treatment as independent variable. All statistical tests were followed with Bonferroni’s post-hoc tests (n = 8–12, *p < 0.05) (see Supplementary File S2 for detailed n sizes and statistical analysis).
Cognitive decline is one of main symptoms of AD (49). In FAD transgenic mouse models, including EFAD mice, learning and memory deficits are often assessed using the Morris water maze test (31, 32, 50, 51). In E3FAD mice, during acquisition phase, there was a main effect of training day but not treatments (Figure 1C). However, it appeared visually that E2-treated mice had better performance on day 5 compared to other groups. Indeed on day 5 the latency to platform was significantly lower in E2 group compared to the OVX group. Thus, although learning was not affected by OVX, E2 treatment marginally improved learning in E3FAD mice. Next, we evaluated memory in a single probe trial. We found that probe measures were impacted by both OVX and E2. Indeed both latency to platform and target quadrant followed the order: OVX > Sham ~ E2. Thus, in E3FAD mice, OVX resulted in memory deficits, which were mitigated by E2 (Figure 1D).
Extracellular Aβ is a major pathological hallmark and diagnostic criteria of AD in humans (52) and may be modulated by OVX and E2 treatment as found in FAD mice (8, 53). Therefore, we next evaluated the impact of OVX and E2 on Aβ levels in E3FAD mice using biochemistry and immunohistochemistry (Supplementary Figure S2). Surprisingly, we found that both OVX and E2 treatment mice had significantly decreased levels of formic-acid-soluble Aβ compared to sham mice in E3FAD (Figure 1C). Consistent with those results, OVX significantly decreased Aβ deposition compared to sham mice (Figure 1E), and E2 lowered cortical Aβ (p = 0.07) compared to sham (Figure 1F).
Collectively, these data demonstrate that, compared to sham surgery, OVX resulted in uterine horn atrophy, changes in estrous stage distribution, memory deficits, and lower Aβ levels in E3FAD mice. E2 mitigated the detrimental effect of OVX on the uterine horn, estrous stage distribution, and memory, with no effect on Aβ levels.
3.2 E4FAD (early OVX): E2 levels did not affect learning/memory but modulated uterine horn weights, estrous stage distribution, and Aβ levels in E4FAD female carriers
AD risk is higher in APOE4 carriers compared to APOE3 carriers, particularly female individuals (19, 20). However, there is limited in vivo data on how APOE4 modulates the effect of OVX and E2 on behavior and Aβ pathology. Therefore, we next investigated the impact of OVX and subsequent E2 treatment in E4FAD mice. OVX decreased uterine horn weight by ~32% in E4FAD mice compared to the sham group (Figure 2A). Furthermore, E2 treatment resulted in uterine hypertrophy with ~100% to 250% increase in uterine horn weight compared to mice that underwent either sham or OVX surgery, respectively (Figure 2A). We also confirmed that OVX decreased the proportion of mice in proestrus/estrus, compared to the sham group, and that E2 treatment increased the proportion of mice in proestrus/estrus after OVX (Figure 2B; Supplementary Figure S1). In terms of behavior, we found that, during acquisition phase, there were no main effects of either training day or treatments (Figure 2C). There was also no effect of OVX or E2 treatment in probe trial measures (Figure 2D). Although OVX and E2 did not impact insoluble Aβ42, OVX increased Aβ deposition (compared to sham mice), which was lowered by E2 (Figures 2E, F; Supplementary Figure S2).
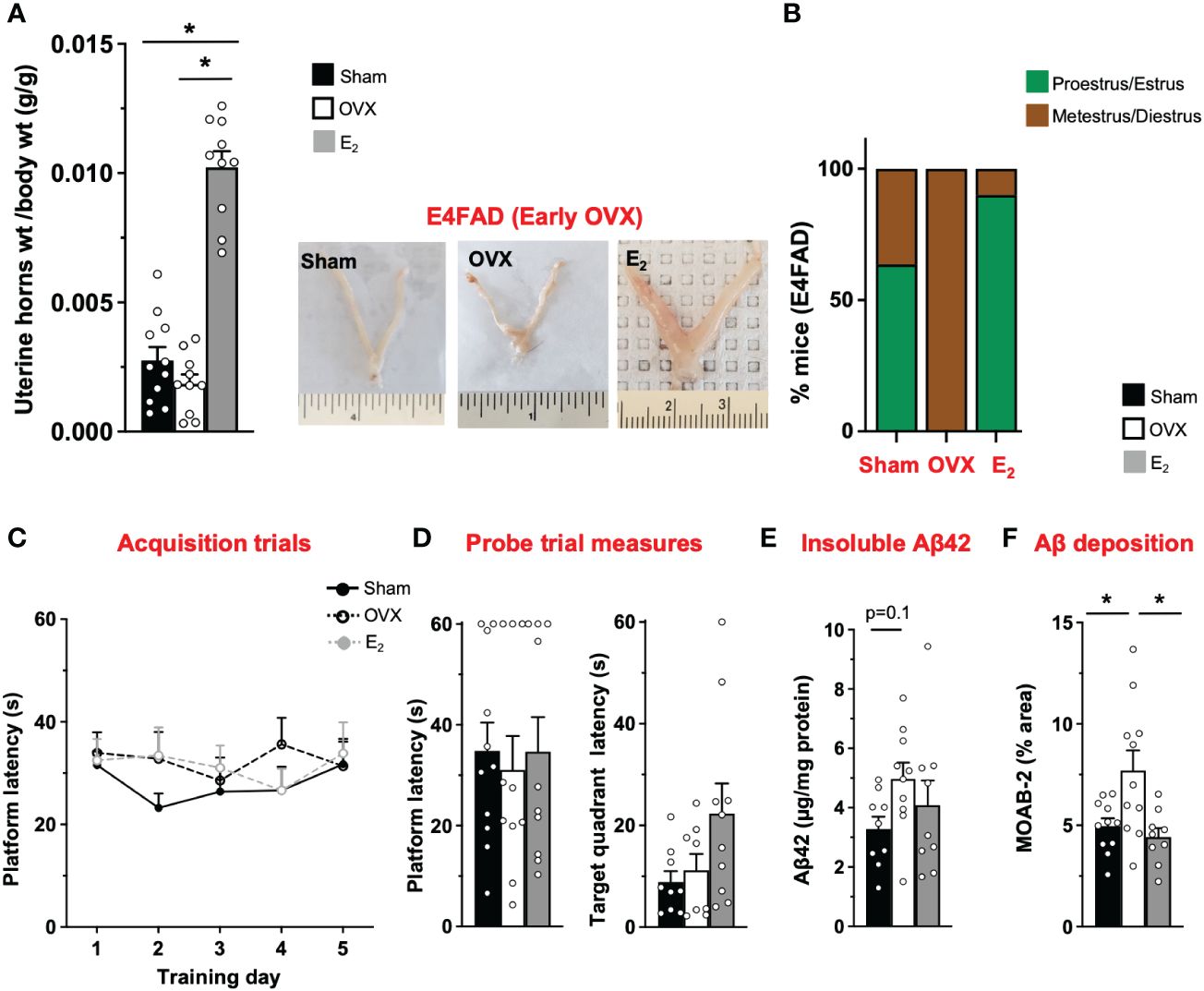
Figure 2 E4FAD (early OVX): E2 levels did not affect learning/memory but modulated uterine horn weights, estrous stage distribution, and Aβ levels in E4FAD females. Uterine horn weights were dissected from E4FAD mice, and their weights were measured (A) to determine the effect of OVX and E2 treatment. (B) Estrous stages of E4FAD mice were determined before their sacrifice via vaginal cytology. Data was plotted as percentage of mice in proestrus/estrus or metestrus/diestrus. Learning and memory were assessed via Morris water maze. E4FAD mice were trained to determine the location of a platform over 5 days during the acquisition phase (C) and acquire the ability to remember the location of the platform (D) 24 h after the last training day probe trial. (E) Formic-acid-soluble Aβ42 was measured in cortical brain homogenates in E4FAD mice. Brain sections obtained from E4FAD mice were immunostained for Aβ using MOAB-2 and the percentage area quantified in the cortex (F). Data are expressed as mean ± SEM. Latency to platform during acquisition phase was analyzed by repeated-measure univariate general linear model for within-subject effects (independent variable: day and treatment). All other statistical analyses were conducted using univariate general linear models for between-subjects effects with treatment as independent variable. All statistical tests were followed with Bonferroni’s post-hoc tests (n = 8–12, *p < 0.05) (see Supplementary File S2 for detailed n sizes and statistical analysis).
Taken together, OVX impacted uterine horn weights and estrous stage distribution and increased cortical Aβ deposition without affecting MWM readouts in E4FAD mice. E2 attenuated the effect of OVX on uterine horn weights and estrous stage distribution and decreased cortical Aβ deposition, with no effect on behavior.
3.3 E3FAD (late OVX): OVX did not impact learning/memory and Aβ, while E2 treatment improved learning
Our data demonstrated in E3FAD mice that OVX at 4 months of age is detrimental, and E2 treatment from 4 to 8 months of age may be protective for behavior. As described above, that paradigm was selected based on pathology. We next asked whether E2 would be beneficial if OVX was performed at an older age with greater Aβ pathology. Therefore, we focused on the effects of E2 treatment from 8–12 months of age (OVX at 8 months) on behavior and Aβ pathology in E3FAD mice.
In E3FAD mice, compared to sham, OVX at 8 months of age decreased uterine horn weights by 32% (Figure 3A) and increased the proportion of mice metestrus/diestrus. Furthermore, E2 increased uterine horn weights by ~200% and 400% compared to OVX and sham mice, respectively, and increased the proportion of mice in proestrus/estrus (Figure 3B; Supplementary Figure S1). However, we found 1/10 OVX mice in estrus stage. Although it seems impossible, neonatal treatment of female mice with estrogen or androgen has been demonstrated to induce ovary-independent persistent proliferation and cornification of vaginal epithelium that may result in the classification of the mice as under estrous phase (54). Therefore, it may be a one-off cytological presentation. Thus, E2 mitigated late OVX-induced changes in both uterine horn weight and estrous stage distribution in E3FAD mice. In terms of behavior in MWM, during acquisition trials, there was a main effect of treatments (Figure 3C). The post-hoc analysis revealed that latency to platform in acquisition trials was demonstrated by the sham ~ OVX > E2 group. However, both OVX and E2 did not affect the probe trial measures (Figure 3D). We also found that, after late OVX, neither OVX nor E2 impacted cortical insoluble Aβ42 (Figure 3E) or Aβ deposition (Figure 3F; Supplementary Figure S2). Overall, in E3FAD mice, OVX did not affect both learning/memory in MWM and Aβ pathology. E2 improved only learning in MWM without impacting the Aβ levels/pathology.
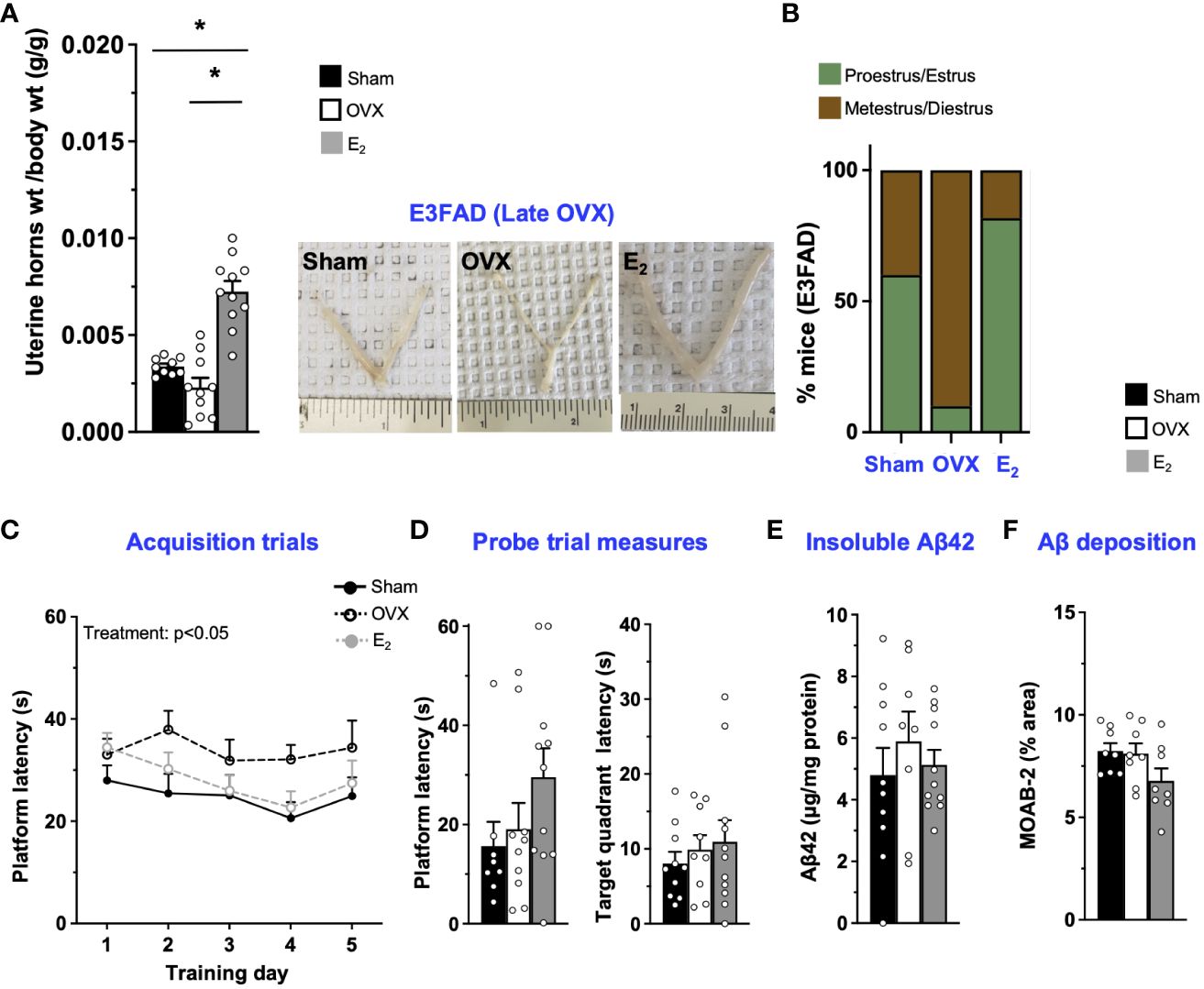
Figure 3 E3FAD (late OVX): OVX did not impact learning/memory and Aβ, while E2 treatment improved learning. Uterine horn weights were dissected from E3FAD mice, and their weights were measured (A) to determine the effect of OVX and E2 treatment. (B) Estrous stages of E3FAD mice were determined before their sacrifice via vaginal cytology. Data was plotted as percentage of mice in proestrus/estrus or metestrus/diestrus. Learning and memory were assessed via Morris water maze. E3FAD mice were trained to determine the location of a platform over 5 days during the acquisition phase (C) and acquire the ability to remember the location of the platform (D) 24 h after the last training day probe trial. (E) Formic-acid-soluble Aβ42 was measured in cortical brain homogenates in E3FAD mice. Brain sections obtained from E3FAD mice were immunostained for Aβ using MOAB-2 and the percentage area quantified in the cortex (F). Data are expressed as mean ± SEM. Latency to platform during acquisition phase was analyzed by repeated-measure univariate general linear model for within-subject effects (independent variable: day and treatment). All other statistical analyses were conducted using univariate general linear models for between-subjects effects with treatment as independent variable. All statistical tests were followed with Bonferroni’s post-hoc tests (n=8-12, * p<0.05). See Supplementary File S2 for detailed n sizes and statistical analysis.
Although E4FAD mice did not show any improvement in behavior but modulated Aβ pathology with E2 treatment after early OVX, we evaluated the effect of OVX and subsequent E2 treatment in older E4FAD mice (Supplementary Figure S3). There was no treatment effect on latency to platform during MWM acquisition trials/probe trials, latency to target quadrant during probe trials, and insoluble Aβ42 and Aβ deposition in the cortex (Supplementary Figures S3C–F). Overall, we found that both OVX and E2 treatment neither affected learning/memory nor Aβ pathology in E4FAD mice.
4 Discussion
4.1 Effect of OVX and E2 on behavior and Aβ pathology—modulation by APOE3
APOE3/3s account for ~30–50% of all AD patients (19). As female sex by itself is an AD risk factor, identifying pathways that could contribute to AD in APOE3/3 female individuals is important. One potential mechanism for higher AD risk is the loss of E2 during and after the menopausal transition. However, clinical data on the impact of menopause on AD risk in APOE3/3s are limited as the focus is typically on APOE4. In APOE3-TR mice, OVX reduced hippocampal spine density, long-term potentiation (22), and disrupted learning in MWM (55). Our data extends those findings to APOE3-FAD mice, as we found that OVX disrupted memory in MWM. Therefore, the loss of sex hormones may be a major contributing factor to AD risk for a large proportion of patients. Based on that idea, E2 would be predicted to protect against AD in APOE3 carrier. In fact, E2 treatment was associated with less cognitive decline or higher learning and memory performance in post-menopausal APOE4 non-carriers (56, 57), and we found that E2 improves memory in E3FAD mice (Early OVX). However, E2 only had a modest effect on learning in late OVX in E3FAD mice. These are consistent with data that early oophorectomy, where there is likely low Aβ pathology that increases AD risk, is lowered by ERT (58). Although there are caveats, E2 may be beneficial to prevent AD-associated neuronal dysfunction and cognitive decline in APOE3/3 if initiated early, before the accumulation of Aβ pathology.
Data from the current study and others raise the important discussion of the mechanism(s) that could underlie the effects of OVX and E2 on neuron function and learning/memory. Our findings suggest that neither OVX nor E2 modulate the Aβ levels in E3FAD mice, consistent with clinical data in APOE4 non-carriers (59). E2 is a potent agonist of the transcriptional response of the nuclear hormone, estrogen receptors (ERα and ERβ), and also activates extranuclear ERx signaling (60). The beneficial effect of E2 was likely mediated by ERs. ERs are expressed in multiple cell types throughout the brain (e.g., neurons, glia, and endothelial cells) and regulate signaling and gene expression that ultimately impact several functions—for instance, E2 is thought to impact neuron function directly (61) and indirectly via effects on inflammation (62, 63), metabolism (64, 65), neurovascular function (66, 67), oxidative stress (68, 69), and APOE levels (61, 70). Indeed E2 facilitated neurite outgrowth in APOE3 neurons (61) and suppressed inflammatory responses in APOE3 glia (71) in vitro. Linked to the question on how E2 works is whether the different functions become disrupted with age and high Aβ pathology, which could result in a lower activity. Future studies could focus on identifying the critical functions of E2 in APOE3/3 carriers.
4.2 Effect of OVX and of E2 on behavior and Aβ pathology—modulation by APOE4
AD risk is high in female APOE4 carriers (19–21), particularly at ages post-menopause. Therefore, it is logical to assume that APOE4 carriers should respond positively to E2, yet clinical studies are more conflicted than for APOE3 (17, 72). The type, timing, duration, and dose of E2 could contribute to discrepant results, along with APOE4-specific considerations. On the assumption that E2 should be beneficial, the timing of treatment in relation to pathology may be critical for APOE4. In APOE4-TR mice, E2 mitigated OVX-induced impairments in learning/memory (55). However, in the current study, E2 was not beneficial in E4FAD mice. Female E4FAD mice have high levels of Aβ, AD-relevant pathologies, and memory deficits by 6–8 months of age (36, 73). Therefore, the combination of female sex, APOE4, and OVX may have resulted in a severe phenotype that was not recoverable by E2. Thus, perhaps in less aggressive models that mimic gradual AD decline as found in humans, E2 could have been beneficial. Further studies in E4FAD to determine whether E2 by itself, administered earlier, may provide support to the “critical window” hypothesis.
An alternative explanation for the clinical data and our own is that, in the context of AD, APOE4/4s may be unresponsive to E2. Many AD patients are APOE3/4, whereas here we focused on APOE4/4 (see limitations). There is evidence that APOE4/4 modulates E2-dependent responses—for example, APOE4/4 neurons (61) and peritoneal macrophages from OVX APOE4-TR mice do not show a significant response to E2 in vitro (71). The impact of APEO4 is pleotropic but includes altering receptor signaling, gene transcription, and lipid transport (74–76). Through those effects, APOE4 may blunt E2-specific responses on multiple levels. In addition, the impact of OVX in the presence of APOE4 may also involve other sex steroid hormones and related hormones such as follicle-stimulating hormone (FSH)—for example, lowering FSH levels in APOE4/4 may mitigate the AD pathology and behavioral impairments associated with APOE4 (55). Thus, for APOE4/4s, it may be important to treat with other sex hormones such as progesterone as there is a link between APOE status and progesterone levels (77). Collectively, either initiating E2 therapy earlier or treatment with other hormones may be beneficial for APOE4 carriers.
4.3 Limitations
There are limitations in the extent to which we can conclude that E2 can mitigate OVX-induced memory impairments in E3FAD mice. As discussed, it is important to conduct additional experiments to identify potential mechanisms through which E2 induced a beneficial effect in E3FAD mice—for example, identifying whether these effects were mediated through ERα, Erβ, or ERx, cell-type specific effects, and downstream signaling pathways more proximal to behavior. Loss of circulating sex hormones in perimenopause is rapid within the context of a woman’s life, but rapid compared to surgical OVX, placing limitations on the model in both prevention and reversal paradigms (78). A less aggressive model of Aβ pathology than EFAD may also alter the response to E2. The dose, frequency, drug formulation, and alternative estrogens may also influence response.
We are also limited to the extent that we can conclude E2 is not beneficial for APOE4/4 carriers. As discussed, E4FAD mice are an aggressive model of Aβ pathology—for example, Aβ coverage in sham E4FAD mice is significantly greater than E3FAD mice that underwent sham surgery or OVX mice. Therefore, it is important to test the activity of E2 in a less aggressive model or even in models without any mutations in APP/PSEN1. Related to this is that the 5xFAD genes are expressed via the Thy-1 promoter in EFAD mice, which is reported to contain an estrogen response element (ERE). However, it is likely that the base mutation (T/C→A) on the core consensus sequence of the Thy-1 promoter at the position +6 would abolish the ER–ERE interaction (79). In addition, the flanking sequence does not contain a purine at -7 position that could potentially increase the binding affinity (80, 81). Furthermore, if E2 did bind to the ERE, then it would occur equally in E3FAD and E4FAD and would unlikely explain the APOE genotype differences in response in this study. However, further studies in additional APOE4 models are important. There were also some experimental limitations surrounding the way we induced hormonal loss. One aspect is how the extent of sex hormone loss induction (either OVX or chemical) in mice translates to humans is unclear with APOE4, especially as female E4FAD mice have disrupted behavior in the absence of OVX (36, 73). In fact, evaluating the activity of E2 in E4FAD mice or other mouse models in the absence of menopausal mimic may provide information of how E2 can impact brain function during aging. There is also the question of whether there are differences in cell-type-specific distribution of ERs, downstream signaling molecules (see “Discussion”), and functions in E3FAD compared to E4FAD mice after OVX. Therefore, conducting additional experiments is critical before discounting the potential of E2 as a therapeutic target for preventing/treating AD in female APOE4/4s individuals.
General limitations also include a lack of pharmacokinetic studies to determine brain and plasma levels after treatment. Although the effects of treatment are evident in estrogen-sensitive gynecological tissues, the sensitivity of tissues to estrogens may not reflect menopause. We selected the dose of E2 based on previous publications; however, levels in the plasma and brain may have been sub-optimal or even different between mice. In addition, continuous delivery of estrogen results in sustained estrogen levels (82–85), which does not mimic physiological fluctuations in hormonal levels, and therefore cyclic E2 treatments may provide greater neural protection. However, the efficacy of cyclic vs. continuous delivery of estrogen is controversial with studies showing improvement in learning/memory using continuous treatment (86–89), cyclic treatment (90), and continuous treatment when primed with repeated injections of E2 (91).
Another limitation of this study is that we are unable to directly compare data obtained in E3FAD and E4FAD mice, as the analysis of each APOE genotype was conducted separately due to the COVID-19 pandemic. In addition, it is also important to incorporate APOE3/4, additional hormones, and treatment windows in future studies.
4.4 Conclusions
Our data supports that the APOE differentially modulated the effect of OVX and E2 on behavior and Aβ pathology—specifically, that E2 may benefit APOE3/3s but not APOE4/4s after loss of sex hormones. Future studies are critical to the optimal treatment approaches for addressing the increased risk of AD after menopause for each APOE genotype.
Data availability statement
The original contributions presented in the study are included in the article/Supplementary Material. Further inquiries can be directed to the corresponding author.
Ethics statement
The animal study was approved by University of Illinois at Chicago Animal Care Committee. The study was conducted in accordance with the local legislation and institutional requirements.
Author contributions
DB: Data curation, Formal analysis, Methodology, Supervision, Writing – original draft, Writing – review & editing. AV-O: Data curation, Formal analysis, Methodology, Supervision, Writing – review & editing. AD: Methodology, Writing – review & editing. SN: Methodology, Writing – review & editing. SK: Methodology, Writing – review & editing. SP: Methodology, Writing – review & editing. JY: Methodology, Writing – review & editing. GT: Conceptualization, Funding acquisition, Writing – review & editing. ML: Conceptualization, Funding acquisition, Writing – review & editing. LT: Writing – original draft, Writing – review & editing.
Funding
The author(s) declare financial support was received for the research, authorship, and/or publication of this article. Previous funding to ML included National Institutes of Health (NIH) grants (R01 AG058068, R01AG057008, R21AG044682), University of Illinois at Chicago Institutional funds, and philanthropic support from Louis and Christine Friedrich. LT is supported by R01AG061114 (NIH) and University of Illinois at Chicago Institutional funds.
In memoriam
This article is dedicated in memory of Dr. LaDu, who tragically passed away last year. She will be missed by all.
Conflict of interest
The authors declare that the research was conducted in the absence of any commercial or financial relationships that could be construed as a potential conflict of interest.
Publisher’s note
All claims expressed in this article are solely those of the authors and do not necessarily represent those of their affiliated organizations, or those of the publisher, the editors and the reviewers. Any product that may be evaluated in this article, or claim that may be made by its manufacturer, is not guaranteed or endorsed by the publisher.
Supplementary material
The Supplementary Material for this article can be found online at: https://www.frontiersin.org/articles/10.3389/fendo.2024.1374825/full#supplementary-material
References
1. Toro CA, Zhang L, Cao J, Cai D. Sex differences in Alzheimer's disease: Understanding the molecular impact. Brain Res. (2019) 1719:194–207. doi: 10.1016/j.brainres.2019.05.031
2. Guo L, Zhong MB, Zhang L, Zhang B, Cai D. Sex differences in alzheimer's disease: Insights from the multiomics landscape. Biol Psychiatry. (2022) 91:61–71. doi: 10.1016/j.biopsych.2021.02.968
3. Zhu D, Montagne A, Zhao Z. Alzheimer's pathogenic mechanisms and underlying sex difference. Cell Mol Life Sci. (2021) 78:4907–20. doi: 10.1007/s00018-021-03830-w
4. Brinton RD. A women's health issue: Alzheimer's disease and strategies for maintaining cognitive health. Int J Fertility Womens Med. (1999) 44:174–85.
5. Riedel BC, Thompson PM, Brinton RD. Age, APOE and sex: Triad of risk of Alzheimer's disease. J Steroid Biochem Mol Biol. (2016) 160:134–47. doi: 10.1016/j.jsbmb.2016.03.012
6. Bove R, Secor E, Chibnik LB, Barnes LL, Schneider JA, Bennett DA, et al. Age at surgical menopause influences cognitive decline and Alzheimer pathology in older women. Neurology. (2014) 82:222–9. doi: 10.1212/WNL.0000000000000033
7. Simpkins JW, Green PS, Gridley KE, Singh M, de Fiebre NC, Rajakumar G. Role of estrogen replacement therapy in memory enhancement and the prevention of neuronal loss associated with Alzheimer's disease. Am J Med. (1997) 103:19S–25S. doi: 10.1016/S0002-9343(97)00260-X
8. Qin Y, An D, Xu W, Qi X, Wang X, Chen L, et al. Estradiol replacement at the critical period protects hippocampal neural stem cells to improve cognition in APP/PS1 mice. Front Aging Neurosci. (2020) 12:240. doi: 10.3389/fnagi.2020.00240
9. Kim JY, Mo H, Kim J, Kim JW, Nam Y, Rim YA, et al. Mitigating effect of estrogen in alzheimer's disease-mimicking cerebral organoid. Front Neurosci. (2022) 16:816174. doi: 10.3389/fnins.2022.816174
10. Paganini-Hill A, Henderson VW. Estrogen deficiency and risk of Alzheimer's disease in women. Am J Epidemiol. (1994) 140:256–61. doi: 10.1093/oxfordjournals.aje.a117244
11. Paganini-Hill A, Henderson VW. Estrogen replacement therapy and risk of Alzheimer disease. Arch Intern Med. (1996) 156:2213–7. doi: 10.1001/archinte.156.19.2213
12. Kawas C, Resnick S, Morrison A, Brookmeyer R, Corrada M, Zonderman A, et al. A prospective study of estrogen replacement therapy and the risk of developing Alzheimer's disease: the Baltimore Longitudinal Study of Aging. Neurology. (1997) 48:1517–21. doi: 10.1212/WNL.48.6.1517
13. Manly JJ, Merchant CA, Jacobs DM, Small SA, Bell K, Ferin M, et al. Endogenous estrogen levels and Alzheimer's disease among postmenopausal women. Neurology. (2000) 54:833–7. doi: 10.1212/wnl.54.4.833
14. Yue X, Lu M, Lancaster T, Cao P, Honda S, Staufenbiel M, et al. Brain estrogen deficiency accelerates Abeta plaque formation in an Alzheimer's disease animal model. Proc Natl Acad Sci U.S.A. (2005) 102:19198–203. doi: 10.1073/pnas.0505203102
15. Maki PM. Critical window hypothesis of hormone therapy and cognition: a scientific update on clinical studies. Menopause. (2013) 20:695–709. doi: 10.1097/GME.0b013e3182960cf8
16. Ali N, Sohail R, Jaffer SR, Siddique S, Kaya B, Atowoju I, et al. The role of estrogen therapy as a protective factor for alzheimer's disease and dementia in postmenopausal women: A comprehensive review of the literature. Cureus. (2023) 15:e43053. doi: 10.7759/cureus.43053
17. Valencia-Olver AC, Maldonado Weng J, Christensen A, LaDu MJ, Pike CJ. Role of estrogen in women's Alzheimer's disease risk as modified by APOE. J Neuroendocrinol. (2023) 35:e13209. doi: 10.1111/jne.13209
18. Nerattini M, Jett S, Andy C, Carlton C, Zarate C, Boneu C, et al. Systematic review and meta-analysis of the effects of menopause hormone therapy on risk of Alzheimer's disease and dementia. Front Aging Neurosci. (2023) 15:1260427. doi: 10.3389/fnagi.2023.1260427
19. Farrer LA, Cupples LA, Haines JL, Hyman B, Kukull WA, Mayeux R, et al. Effects of age, sex, and ethnicity on the association between apolipoprotein E genotype and Alzheimer disease. A meta-analysis. APOE and Alzheimer Disease Meta Analysis Consortium. JAMA. (1997) 278:1349–56. doi: 10.1001/jama.278.16.1349
20. Altmann A, Tian L, Henderson VW, Greicius MD. Sex modifies the APOE-related risk of developing Alzheimer disease. Ann Neurol. (2014) 75:563–73. doi: 10.1002/ana.24135
21. Neu SC, Pa J, Kukull W, Beekly D, Kuzma A, Gangadharan P, et al. Apolipoprotein E genotype and sex risk factors for alzheimer disease: A meta-analysis. JAMA Neurol. (2017) 74:1178–89. doi: 10.1001/jamaneurol.2017.2188
22. Klein RC, Saini S, Risher ML, Acheson SK, Fleming RL, Sexton HG, et al. Regional-specific effects of ovarian hormone loss on synaptic plasticity in adult human APOE targeted replacement mice. PLoS One. (2014) 9:e94071. doi: 10.1371/journal.pone.0094071
23. Yun SH, Park KA, Kwon S, Woolley CS, Sullivan PM, Pasternak JF, et al. Estradiol enhances long term potentiation in hippocampal slices from aged apoE4-TR mice. Hippocampus. (2007) 17:1153–7. doi: 10.1002/hipo.20357
24. Youmans KL, Tai LM, Nwabuisi-Heath E, Jungbauer L, Kanekiyo T, Gan M, et al. APOE4-specific changes in abeta accumulation in a new transgenic mouse model of alzheimer disease. J Biol Chem. (2012) 287:41774–86. doi: 10.1074/jbc.M112.407957
25. Frick KM, Berger-Sweeney J. Spatial reference memory and neocortical neurochemistry vary with the estrous cycle in C57BL/6 mice. Behav Neurosci. (2001) 115:229–37. doi: 10.1037/0735-7044.115.1.229
26. Rowe AA, Issioui Y, Johnny B, Wert KJ. Murine orchiectomy and ovariectomy to reduce sex hormone production. J Vis Exp. (2023) 201. doi: 10.3791/64379
27. Fernandez SM, Lewis MC, Pechenino AS, Harburger LL, Orr PT, Gresack JE, et al. Estradiol-induced enhancement of object memory consolidation involves hippocampal extracellular signal-regulated kinase activation and membrane-bound estrogen receptors. J Neurosci. (2008) 28:8660–7. doi: 10.1523/JNEUROSCI.1968-08.2008
28. Pechenino AS, Frick KM. The effects of acute 17beta-estradiol treatment on gene expression in the young female mouse hippocampus. Neurobiol Learn Mem. (2009) 91:315–22. doi: 10.1016/j.nlm.2008.09.017
29. Fleischer AW, Schalk JC, Wetzel EA, Hanson AM, Sem DS, Donaldson WA, et al. Long-term oral administration of a novel estrogen receptor beta agonist enhances memory and alleviates drug-induced vasodilation in young ovariectomized mice. Horm Behav. (2021) 130:104948. doi: 10.1016/j.yhbeh.2021.104948
30. Taxier LR, Philippi SM, York JM, LaDu MJ, Frick KM. The detrimental effects of APOE4 on risk for Alzheimer's disease may result from altered dendritic spine density, synaptic proteins, and estrogen receptor alpha. Neurobiol Aging. (2022) 112:74–86. doi: 10.1016/j.neurobiolaging.2021.12.006
31. Liu DS, Pan XD, Zhang J, Shen H, Collins NC, Cole AM, et al. APOE4 enhances age-dependent decline in cognitive function by down-regulating an NMDA receptor pathway in EFAD-Tg mice. Mol Neurodegener. (2015) 10:7. doi: 10.1186/s13024-015-0002-2
32. Thomas R, Morris AWJ, Tai LM. Epidermal growth factor prevents APOE4-induced cognitive and cerebrovascular deficits in female mice. Heliyon. (2017) 3:e00319. doi: 10.1016/j.heliyon.2017.e00319
33. Valencia-Olver AC, Balu D, Faulk N, Amiridis A, Wang Y, Pham C, et al. Inhibition of ACAT as a therapeutic target for alzheimer's disease is independent of apoE4 lipidation. Neurotherapeutics. (2023) 20(4). doi: 10.1007/s13311-023-01375-3
34. Byers SL, Wiles MV, Dunn SL, Taft RA. Mouse estrous cycle identification tool and images. PLoS One. (2012) 7:e35538. doi: 10.1371/journal.pone.0035538
35. Ajayi AF, Akhigbe RE. Staging of the estrous cycle and induction of estrus in experimental rodents: an update. Fertil Res Pract. (2020) 6:5. doi: 10.1186/s40738-020-00074-3
36. Balu D, Valencia-Olver AC, Islam Z, Mielczarek C, Hansen A, Perez Ramos TM, et al. APOE genotype and sex modulate Alzheimer's disease pathology in aged EFAD transgenic mice. Front Aging Neurosci. (2023) 15:1279343. doi: 10.3389/fnagi.2023.1279343
37. Balu D, Valencia-Olver AC, Nguyen A, Patnam M, York J, Peri F, et al. A small-molecule TLR4 antagonist reduced neuroinflammation in female E4FAD mice. Alzheimers Res Ther. (2023) 15:181. doi: 10.1186/s13195-023-01330-6
38. Youmans KL, Leung S, Zhang J, Maus E, Baysac K, Bu G, et al. Amyloid-beta42 alters apolipoprotein E solubility in brains of mice with five familial AD mutations. J Neurosci Methods. (2011) 196:51–9. doi: 10.1016/j.jneumeth.2010.12.025
39. Youmans KL, Tai LM, Kanekiyo T, Stine WB Jr., Michon SC, Nwabuisi-Heath E, et al. Intraneuronal Abeta detection in 5xFAD mice by a new Abeta-specific antibody. Mol Neurodegener. (2012) 7:8. doi: 10.1186/1750-1326-7-8
40. Rodriguez GA, Tai LM, LaDu MJ, Rebeck GW. Human APOE4 increases microglia reactivity at Abeta plaques in a mouse model of Abeta deposition. J Neuroinflamm. (2014) 11:111. doi: 10.1186/1742-2094-11-111
41. Kunzler J, Youmans KL, Yu C, Ladu MJ, Tai LM. APOE modulates the effect of estrogen therapy on Abeta accumulation EFAD-Tg mice. Neurosci Lett. (2014) 560:131–6. doi: 10.1016/j.neulet.2013.12.032
42. Dutta S, Sengupta P. Men and mice: Relating their ages. Life Sci. (2016) 152:244–8. doi: 10.1016/j.lfs.2015.10.025
43. Mosconi L, Berti V, Quinn C, McHugh P, Petrongolo G, Varsavsky I, et al. Sex differences in Alzheimer risk: Brain imaging of endocrine vs chronologic aging. Neurology. (2017) 89:1382–90. doi: 10.1212/WNL.0000000000004425
44. Mosconi L, Rahman A, Diaz I, Wu X, Scheyer O, Hristov HW, et al. Increased Alzheimer's risk during the menopause transition: A 3-year longitudinal brain imaging study. PLoS One. (2018) 13:e0207885. doi: 10.1371/journal.pone.0207885
45. Finch CE, Felicio LS, Mobbs CV, Nelson JF. Ovarian and steroidal influences on neuroendocrine aging processes in female rodents. Endocr Rev. (1984) 5:467–97. doi: 10.1210/edrv-5-4-467
46. Garcia-Perez MA, Del Val R, Noguera I, Hermenegildo C, Pineda B, Martinez-Romero A, et al. Estrogen receptor agonists and immune system in ovariectomized mice. Int J Immunopathol Pharmacol. (2006) 19:807–19. doi: 10.1177/039463200601900410
47. Lemini C, Jaimez R, Figueroa A, Martinez-Mota L, Avila ME, Medina M. Ovariectomy differential influence on some hemostatic markers of mice and rats. Exp Anim. (2015) 64:81–9. doi: 10.1538/expanim.14-0052
48. Sanchez K, Wu SL, Kakkar R, Darling JS, Harper CS, Fonken LK. Ovariectomy in mice primes hippocampal microglia to exacerbate behavioral sickness responses. Brain Behav Immun Health. (2023) 30:100638. doi: 10.1016/j.bbih.2023.100638
49. Rao RV, Subramaniam KG, Gregory J, Bredesen AL, Coward C, Okada S, et al. Rationale for a multi-factorial approach for the reversal of cognitive decline in alzheimer's disease and MCI: A review. Int J Mol Sci. (2023) 24. doi: 10.3390/ijms24021659
50. Webster SJ, Bachstetter AD, Nelson PT, Schmitt FA, Van Eldik LJ. Using mice to model Alzheimer's dementia: an overview of the clinical disease and the preclinical behavioral changes in 10 mouse models. Front Genet. (2014) 5:88. doi: 10.3389/fgene.2014.00088
51. Jankowsky JL, Zheng H. Practical considerations for choosing a mouse model of Alzheimer's disease. Mol Neurodegener. (2017) 12:89. doi: 10.1186/s13024-017-0231-7
52. Zhang Y, Chen H, Li R, Sterling K, Song W. Amyloid beta-based therapy for Alzheimer's disease: challenges, successes and future. Signal Transduct Target Ther. (2023) 8:248. doi: 10.1038/s41392-023-01484-7
53. Ding F, Yao J, Zhao L, Mao Z, Chen S, Brinton RD. Ovariectomy induces a shift in fuel availability and metabolism in the hippocampus of the female transgenic model of familial Alzheimer's. PLoS One. (2013) 8:e59825. doi: 10.1371/journal.pone.0059825
54. Takasugi N. Cytological basis for permanent vaginal changes in mice treated neonatally with steroid hormones. Int Rev Cytol. (1976) 44:193–224. doi: 10.1016/s0074-7696(08)61650-2
55. Xiong J, Kang SS, Wang M, Wang Z, Xia Y, Liao J, et al. FSH and ApoE4 contribute to Alzheimer's disease-like pathogenesis via C/EBPbeta/delta-secretase in female mice. Nat Commun. (2023) 14:6577. doi: 10.1038/s41467-023-42282-7
56. Yaffe K, Haan M, Byers A, Tangen C, Kuller L. Estrogen use, APOE, and cognitive decline: evidence of gene-environment interaction. Neurology. (2000) 54:1949–54. doi: 10.1212/WNL.54.10.1949
57. Burkhardt MS, Foster JK, Laws SM, Baker LD, Craft S, Gandy SE, et al. Oestrogen replacement therapy may improve memory functioning in the absence of APOE epsilon4. J Alzheimers Dis. (2004) 6:221–8. doi: 10.3233/jad-2004-6302
58. Rocca WA, Grossardt BR, Shuster LT. Oophorectomy, menopause, estrogen treatment, and cognitive aging: clinical evidence for a window of opportunity. Brain Res. (2011) 1379:188–98. doi: 10.1016/j.brainres.2010.10.031
59. Kantarci K, Tosakulwong N, Lesnick TG, Zuk SM, Lowe VJ, Fields JA, et al. Brain structure and cognition 3 years after the end of an early menopausal hormone therapy trial. Neurology. (2018) 90:e1404–12. doi: 10.1212/WNL.0000000000005325
60. Hara Y, Waters EM, McEwen BS, Morrison JH. Estrogen effects on cognitive and synaptic health over the lifecourse. Physiol Rev. (2015) 95:785–807. doi: 10.1152/physrev.00036.2014
61. Nathan BP, Barsukova AG, Shen F, McAsey M, Struble RG. Estrogen facilitates neurite extension via apolipoprotein E in cultured adult mouse cortical neurons. Endocrinology. (2004) 145:3065–73. doi: 10.1210/en.2003-1707
62. Au A, Feher A, McPhee L, Jessa A, Oh S, Einstein G. Estrogens, inflammation and cognition. Front Neuroendocrinol. (2016) 40:87–100. doi: 10.1016/j.yfrne.2016.01.002
63. Villa A, Vegeto E, Poletti A, Maggi A. Estrogens, neuroinflammation, and neurodegeneration. Endocr Rev. (2016) 37:372–402. doi: 10.1210/er.2016-1007
64. Rettberg JR, Yao J, Brinton RD. Estrogen: a master regulator of bioenergetic systems in the brain and body. Front Neuroendocrinol. (2014) 35:8–30. doi: 10.1016/j.yfrne.2013.08.001
65. Bustamante-Barrientos FA, Mendez-Ruette M, Ortloff A, Luz-Crawford P, Rivera FJ, Figueroa CD, et al. The impact of estrogen and estrogen-like molecules in neurogenesis and neurodegeneration: beneficial or harmful? Front Cell Neurosci. (2021) 15:636176. doi: 10.3389/fncel.2021.636176
66. Nguyen DH, Cunningham JT, Sumien N. Estrogen receptor involvement in vascular cognitive impairment and vascular dementia pathogenesis and treatment. Geroscience. (2021) 43:159–66. doi: 10.1007/s11357-020-00263-4
67. Goncalves FJ, Abrantes-Soares F, Pouso MR, Lorigo M, Cairrao E. Non-genomic effect of estradiol on the neurovascular unit and possible involvement in the cerebral vascular accident. Mol Neurobiol. (2023) 60:1964–85. doi: 10.1007/s12035-022-03178-7
68. White RE, Gerrity R, Barman SA, Han G. Estrogen and oxidative stress: A novel mechanism that may increase the risk for cardiovascular disease in women. Steroids. (2010) 75:788–93. doi: 10.1016/j.steroids.2009.12.007
69. Lagranha CJ, Silva TLA, Silva SCA, Braz GRF, da Silva AI, Fernandes MP, et al. Protective effects of estrogen against cardiovascular disease mediated via oxidative stress in the brain. Life Sci. (2018) 192:190–8. doi: 10.1016/j.lfs.2017.11.043
70. Wang JM, Irwin RW, Brinton RD. Activation of estrogen receptor alpha increases and estrogen receptor beta decreases apolipoprotein E expression in hippocampus in vitro and in vivo. Proc Natl Acad Sci U.S.A. (2006) 103:16983–8. doi: 10.1073/pnas.0608128103
71. Brown CM, Choi E, Xu Q, Vitek MP, Colton CA. The APOE4 genotype alters the response of microglia and macrophages to 17beta-estradiol. Neurobiol Aging. (2008) 29:1783–94. doi: 10.1016/j.neurobiolaging.2007.04.018
72. Wong GRM, Lee EJA, Liaw QY, Rajaram H. The role of oestrogen therapy in reducing risk of Alzheimer's disease: systematic review. BJPsych Open. (2023) 9:e194. doi: 10.1192/bjo.2023.579
73. Zaldua S, Damen FC, Pisharody R, Thomas R, Fan KD, Ekkurthi GK, et al. Epidermal growth factor treatment of female mice that express APOE4 at an age of advanced pathology mitigates behavioral and cerebrovascular dysfunction. Heliyon. (2020) 6:e03919. doi: 10.1016/j.heliyon.2020.e03919
74. Lane-Donovan C, Herz J. The ApoE receptors Vldlr and Apoer2 in central nervous system function and disease. J Lipid Res. (2017) 58:1036–43. doi: 10.1194/jlr.R075507
75. Theendakara V, Peters-Libeu CA, Bredesen DE, Rao RV. Transcriptional effects of apoE4: relevance to alzheimer's disease. Mol Neurobiol. (2018) 55:5243–54. doi: 10.1007/s12035-017-0757-2
76. Husain MA, Laurent B, Plourde M. APOE and alzheimer's disease: From lipid transport to physiopathology and therapeutics. Front Neurosci. (2021) 15:630502. doi: 10.3389/fnins.2021.630502
77. Jasienska G, Ellison PT, Galbarczyk A, Jasienski M, Kalemba-Drozdz M, Kapiszewska M, et al. Apolipoprotein E (ApoE) polymorphism is related to differences in potential fertility in women: a case of antagonistic pleiotropy? Proc Biol Sci. (2015) 282:20142395. doi: 10.1098/rspb.2014.2395
78. Diaz Brinton R. Minireview: translational animal models of human menopause: challenges and emerging opportunities. Endocrinology. (2012) 153:3571–8. doi: 10.1210/en.2012-1340
79. Driscoll MD, Sathya G, Muyan M, Klinge CM, Hilf R, Bambara RA. Sequence requirements for estrogen receptor binding to estrogen response elements. J Biol Chem. (1998) 273:29321–30. doi: 10.1074/jbc.273.45.29321
80. Klinge CM. Estrogen receptor interaction with estrogen response elements. Nucleic Acids Res. (2001) 29:2905–19. doi: 10.1093/nar/29.14.2905
81. Gruber CJ, Gruber DM, Gruber IM, Wieser F, Huber JC. Anatomy of the estrogen response element. Trends Endocrinol Metab. (2004) 15:73–8. doi: 10.1016/j.tem.2004.01.008
82. Shoupe D. HRT dosing regimens: continuous versus cyclic-pros and cons. Int J Fertil Womens Med. (2001) 46:7–15.
83. Resnick SM, Coker LH, Maki PM, Rapp SR, Espeland MA, Shumaker SA. The Women's Health Initiative Study of Cognitive Aging (WHISCA): a randomized clinical trial of the effects of hormone therapy on age-associated cognitive decline. Clin Trials. (2004) 1:440–50. doi: 10.1191/1740774504cn040oa
84. Coker LH, Espeland MA, Rapp SR, Legault C, Resnick SM, Hogan P, et al. Postmenopausal hormone therapy and cognitive outcomes: the Women's Health Initiative Memory Study (WHIMS). J Steroid Biochem Mol Biol. (2010) 118:304–10. doi: 10.1016/j.jsbmb.2009.11.007
85. Manson JE, Chlebowski RT, Stefanick ML, Aragaki AK, Rossouw JE, Prentice RL, et al. Menopausal hormone therapy and health outcomes during the intervention and extended poststopping phases of the Women's Health Initiative randomized trials. JAMA. (2013) 310:1353–68. doi: 10.1001/jama.2013.278040
86. Frick KM, Fernandez SM, Bulinski SC. Estrogen replacement improves spatial reference memory and increases hippocampal synaptophysin in aged female mice. Neuroscience. (2002) 115:547–58. doi: 10.1016/S0306-4522(02)00377-9
87. Markham JA, Pych JC, Juraska JM. Ovarian hormone replacement to aged ovariectomized female rats benefits acquisition of the morris water maze. Horm Behav. (2002) 42:284–93. doi: 10.1006/hbeh.2002.1819
88. Vaucher E, Reymond I, Najaffe R, Kar S, Quirion R, Miller MM, et al. Estrogen effects on object memory and cholinergic receptors in young and old female mice. Neurobiol Aging. (2002) 23:87–95. doi: 10.1016/S0197-4580(01)00250-0
89. Foster TC, Sharrow KM, Kumar A, Masse J. Interaction of age and chronic estradiol replacement on memory and markers of brain aging. Neurobiol Aging. (2003) 24:839–52. doi: 10.1016/S0197-4580(03)00014-9
90. Gresack JE, Frick KM. Effects of continuous and intermittent estrogen treatments on memory in aging female mice. Brain Res. (2006) 1115:135–47. doi: 10.1016/j.brainres.2006.07.067
Keywords: Alzheimer’s disease, ApoE4, female risk, transgenic mice, amyloid-beta
Citation: Balu D, Valencia-Olvera AC, Deshpande A, Narayanam S, Konasani S, Pattisapu S, York JM, Thatcher GRJ, LaDu MJ and Tai LM (2024) Estradiol improves behavior in FAD transgenic mice that express APOE3 but not APOE4 after ovariectomy. Front. Endocrinol. 15:1374825. doi: 10.3389/fendo.2024.1374825
Received: 22 January 2024; Accepted: 02 April 2024;
Published: 29 April 2024.
Edited by:
Yoshinori Okamoto, Meijo University, JapanReviewed by:
Roberta Marongiu, Cornell University, United StatesTal Nuriel, Columbia University, United States
Copyright © 2024 Balu, Valencia-Olvera, Deshpande, Narayanam, Konasani, Pattisapu, York, Thatcher, LaDu and Tai. This is an open-access article distributed under the terms of the Creative Commons Attribution License (CC BY). The use, distribution or reproduction in other forums is permitted, provided the original author(s) and the copyright owner(s) are credited and that the original publication in this journal is cited, in accordance with accepted academic practice. No use, distribution or reproduction is permitted which does not comply with these terms.
*Correspondence: Leon M. Tai, bGVvbnRhaUB1aWMuZWR1