- 1Department of Orthopedics, Tongji Hospital, Tongji Medical College, Huazhong University of Science and Technology, Wuhan, China
- 2Clinical Innovation and Research Center (CIRC), Shenzhen Hospital, Southern Medical University, Shenzhen, China
- 3The First Clinical Medical College, Southern Medical University, Guangzhou, China
- 4Department of Rehabilitation, Tongji Hospital, Tongji Medical College, Huazhong University of Science and Technology, Wuhan, China
Objective: Thyroid hormones have actions on cartilage, whereas the association between thyroid hormone related diseases and osteoarthritis (OA) are unclear. This study aims to investigate the association between thyrotoxicosis and OA.
Methods: Summary-level genetic data of thyrotoxicosis were obtained from FinnGen cohorts (nCase = 10,569, nControl = 762,037). Summary-level data of OA were obtained from a large-scale genome-wide association study of UK Biobank (nCase = 40,659, nControl = 756,338). Single nucleotide polymorphisms (SNPs) robustly associated with thyrotoxicosis or OA were used as genetic instruments. A two-sample bidirectional Mendelian randomization (MR) analysis was designed to assess the effect of genetic predisposition of thyrotoxicosis on OA risk, as well as the reverse their relationship. The causal effect was estimated by Inverse-variance weighted method, with weighted median and MR-Egger as supplementary methods.
Results: Genetic predisposition of thyrotoxicosis was associated with the onset of knee OA (autoimmune hyperthyroidism: odds ratio [OR]: 1.05, 95% confidence interval [CI]: 1.03-1.07, FDR < 0.001; thyrotoxicosis: OR: 1.05, 95% CI: 1.02-1.08, FDR = 0.016; thyrotoxicosis with diffuse goitre: OR: 1.04, 95% CI: 1.02-1.07, FDR = 0.003; other and/or unspecified thyrotoxicosis: OR: 1.05, 95% CI: 1.02-1.09, FDR = 0.003), whereas thyrotoxicosis was not associated with hip OA. In reverse MR analysis, genetic predisposition to OA was not associated with thyrotoxicosis. No pleiotropy was identified in the MR analyses. Sensitivity analyses indicated the robustness of the MR estimates.
Conclusion: This study provides MR evidence supporting causal association of thyrotoxicosis with knee OA in European population, whereas OA may have no causal effects on thyrotoxicosis.
Introduction
Osteoarthritis (OA) is a widespread chronic degenerative joint condition which mainly involves knee joint (1). OA is characterized by the progressive degeneration of articular tissues throughout the entire joint, encompassing not only the cartilage, synovial membrane, and subchondral bone, but also the menisci, infrapatellar fat pad, and the tendons and ligaments (2). In the progression of OA, cartilage degradation (3), synovitis (4), subchondral bone lesions (5), meniscal degeneration (6), inflammation and fibrosis of the infrapatellar fat pad (7), and instability of ligaments (8) not only occur independently but also collaboratively and interactively, either concurrently or sequentially. These pathological changes are interconnected, jointly driving the pathological process of OA, underscoring the systemic nature of this complex disease (2). OA causes chronic pain, swelling, stiffness, and restricted joint movement of patients (9). An epidemiological study from 2020 reported that roughly 3.8% of the global population, equating to 250 million people, are affected by OA (10). Among those over 60 years old, 18% of females and 9.6% of males experience symptomatic OA (10). Beyond the huge health burden on patients, OA also contributes prominently to escalating medical expenses in United States (11). Therefore, early detection and effective intervention are crucial to curtail its prevalence (12, 13). Although some risk factors such as aging (14), trauma (15), obesity (16), and metabolic disorders (17) has been linked to OA, the etiology and pathogenesis have not yet been fully clarified.
The thyroid is a vital endocrine organ responsible for regulating physiological processes through the synthesis and secretion of calcitonin and thyroid hormones (THs), including triiodothyronine (T3) and thyroxine (T4) (18). These hormones are crucial for the normal growth, differentiation, and functioning of various tissues. Insufficient TH levels can result in fatigue, constipation, and weight gain, while excessive levels may heighten the risk of cardiovascular diseases and osteoporosis (19). Thyrotoxicosis refers the clinical manifestation of excessive TH action at the tissue level due to inappropriately elevated concentrations of circulating THs (20). As a subset of thyrotoxicosis, hyperthyroidism specifically denotes the excessive synthesis and secretion of THs by the thyroid gland (20). Graves’ disease, accounting for 70% of cases, is the primary etiology of hyperthyroidism and is defined as autoimmune hyperthyroidism (21). Other causes include toxic nodular hyperthyroidism, constituting 16%, which stems from one or more autonomously functioning thyroid nodules; subacute granulomatous thyroiditis, making up 3%, induced by inflammation; and medications, comprising 9%, notably amiodarone, tyrosine kinase inhibitors, and immune checkpoint inhibitors (22, 23).
While THs are well-established regulators of skeleton development, growth, and maintenance, recent studies also highlight their potential significance in articular cartilage and OA (24). Iodothyronine deiodinase 2 and 3, encoded by DIO2 and DIO3, regulate the conversion between prohormone thyroxine T4 and the bioactive thyroid hormone T3, which were identified as disease susceptibility loci in OA (25, 26). Hyperthyroid states were associated with increased joint effusion and musculoskeletal ultrasound abnormalities (27). Moreover, eprotirome (KB2115), a thyroid hormone receptor (TR) agonist, can cause dose-related articular cartilage damage (28, 29). These studies suggest the potential role of thyroid hormones in OA pathogenesis. However, the association between thyrotoxicosis and the risk of OA is unclear due to no epidemiology evidence.
Mendelian randomization (MR) is an effective method used to elucidate the relationships between exposures and health outcomes (30). By employing genetic variants as proxies for exposures, MR leverages the random allocation of genes at conception. These variants remain unaltered by disease progression, enabling MR to address confounding variables and minimize issues of reverse causation effectively (31, 32). Previous study has suggested the susceptibility loci of thyrotoxicosis (33), which is the basis for using genetic variants as a proxy of thyrotoxicosis. In this study, a bidirectional MR design was utilized to investigate the association between thyrotoxicosis and OA. Genetic variants were used as instrumental proxy of genetic predisposition to thyrotoxicosis, and MR analyses can infer whether OA risk is increased with increased genetic predisposition to thyrotoxicosis. Due to the strength of MR studies, this study offers a causative inference on the causal association between thyrotoxicosis and OA.
Methods
Study design
A two-sample MR analysis to assess the causal impact of thyrotoxicosis on hip or knee OA, utilizing summary-level data of genome-wide association studies (GWAS) from MRC IEU OpenGWAS database (https://gwas.mrcieu.ac.uk/). Three assumptions were satisfied in the MR design: (i) the genetic instruments used as the proxy of genetic predisposition must be robustly correlated with thyrotoxicosis; (ii) the genetic instruments have no association with potential confounders; and (iii) the genetic instruments affect hip or knee OA only through thyrotoxicosis (Figure 1). Subsequently, a reverse MR analysis was performed to investigate whether hip or knee OA has a reverse effect on the risk of thyrotoxicosis.
Data sources for exposure and outcome
Both data sources for exposure and outcome were obtain from MRC IEU OpenGWAS database with GWAS IDs. Summary-level data of thyrotoxicosis-related traits were originally from the FinnGen consortium R5 release, including autoimmune hyperthyroidism (962 cases and 172,976 controls; GWAS ID: “finn-b-AUTOIMMUNE_HYPERTHYROIDISM”), thyrotoxicosis (4,142 cases and 213,693 controls; GWAS ID: “finn-b-THYROTOXICOSIS”), thyrotoxicosis with diffuse goitre (2,350 cases and 187,684 controls; GWAS ID: “finn-b-E4_THYTOXGOITDIF”), and other and/or unspecified thyrotoxicosis (3,115 cases and 187,684 controls; GWAS ID: “finn-b-E4_THYTOXNAS”). Summary-level data of OA-related traits were originally from a genome-wide meta-analysis of UK Biobank (34), including knee OA (2,4955 cases and 378,169 controls; GWAS ID: “ebi-a-GCST007090”) and hip OA (15,704 cases and 378,169 controls; GWAS ID: “ebi-a-GCST007091”). Since the traits of thyrotoxicosis and OA are based on Finland and UK individuals, respectively, there was no overlap between exposure and outcome data sources. All the summary-level data was derived from on both genders of European ancestry. Original studies included in the cited GWASs and consortia received ethical approval from their respective institutional review boards, and all participants had given informed consent. Detailed information on data sources was summarized in Table 1.
Genetic instrument selection
The proxy instruments of genetic predisposition of thyrotoxicosis or OA were selected by rigorous filtering steps to guarantee the assumptions of MR analysis. To select independent and significant genetic instruments, single nucleotide polymorphisms (SNPs) were selected at the genome-wide significance level (P < 5.0×10-8) and at linkage disequilibrium (LD) r2 < 0.001 within a 10,000-kilobase window based on European ancestry reference data from the 1000 Genomes Project. Then, the selected SNPs were checked by the PhenoScanner platform (https://www.phenoscanner.medschl.cam.ac.uk) to exclude the SNPs associated with potential risk factors of outcome. Third, the SNPs associated with the outcome at the genome-wide significance level (P < 5×10-5) were discarded to guarantee the third assumption. Fourth, ambiguous and palindromic SNPs were excluded by harmonizing the SNPs extracted from exposure and outcome data. Fifth, to quantify the strength of the selected genetic instruments, F-statistics was calculated and the SNPs with an F-statistic less than 10 were excluded to avoid weak instrument bias. Finally, Setiger test was performed to remove the SNPs with a reverse causality between the exposure and the outcome. Finally, MR analyses were performed if more than three SNPs were retained.
Mendelian randomization
The MR analyses were performed with R software (version 4.2.2), TwoSampleMR (version 0.5.6), Mendelian Randomization (version 0.5.0), and MR-PRESSO package. Four MR methods, including inverse variance weighting (IVW), weighted median, weighted mode, and MR-Egger, were used as the previous study descripted (32). IVW method under the random-effect model was used as the primary analysis method, since IVW provides the most precise estimation of causal association. Other MR analyses, such as weighted median and MR-Egger, have been implemented as a complement to IVW. The IVW estimates were compared with weighted median and MR-Egger to check whether the results of different methods were consistent. Potential heterogeneity of SNPs was evaluated using Cochrane’s Q-statistics. MR-Egger intercept was used to identify possible pleiotropic effects, and the MR estimates is invalid if pleiotropy exists. Finally, the robustness of MR estimates was assessed by leave-one-out sensitivity analysis to check whether the MR estimates were driven by any single SNP. After adjusted by Benjamini and Hochberg method for multiple tests, the MR estimates with a FDR < 0.05 was considered statistically significant.
Results
After the rigorous screening, 5, 9, 10, and 8 SNPs with P < 5.0×10-8 and F > 10 were selected to instrument autoimmune hyperthyroidism, thyrotoxicosis, thyrotoxicosis with diffuse goitre, and other and/or unspecified thyrotoxicosis. Moreover, 5 and 23 SNPs with P < 5.0×10-8 and F > 10 were selected to instrument knee and hip OA. In the MR analysis of the effect of knee OA on autoimmune hyperthyroidism, one SNP (rs1078301) was excluded by Setiger test. Similarly, two SNPs (rs12040949 and rs4252548) were excluded by Setiger test in the analysis of effect of hip OA on autoimmune hyperthyroidism. The detailed information on genetic instruments selected for thyrotoxicosis and OA-related traits was summarized in Supplementary Tables 1–3.
As shown in Figure 2 and Supplementary Figure 1, the MR estimates of IVW method indicates that the genetic predisposition of thyrotoxicosis was causally associated with the increased risk of knee OA (autoimmune hyperthyroidism: odds ratio [OR]: 1.05, 95% confidence interval [CI]: 1.03-1.07, P < 0.001, FDR < 0.001; thyrotoxicosis: OR: 1.05, 95% CI: 1.02-1.08, P = 0.004, FDR = 0.016; thyrotoxicosis with diffuse goitre: OR: 1.04, 95% CI: 1.02-1.07, P < 0.001, FDR = 0.003; other and/or unspecified thyrotoxicosis: OR: 1.05, 95% CI: 1.02-1.09, P < 0.001, FDR = 0.003). In the other MR methods, most of MR estimates were statistically significant with a OR more than 1. Since all the P values of Egger intercept were large than 0.05 (Table 2), no horizontal pleiotropy was identified in the MR analyses. Thus, the significant MR estimates were valid. Moreover, all P-heterogeneity values of heterogeneity test were not statistically significant, thus no heterogeneity was identified in the IVW and MR Egger analyses (Table 2), in line with the funnel plots (Supplementary Figure 3). Of note, the P-heterogeneity value of MR Egger is 0.05 in the analysis of thyrotoxicosis and hip OA (Table 2). Leave-one-out sensitivity analysis showed that the pooled effect sizes of thyrotoxicosis-OA were all statistically significant after removing a SNP in turn, which indicates the robustness of these associations (Supplementary Figure 5). Together, these results suggest a causal association of thyrotoxicosis with knee OA, while no association between thyrotoxicosis and hip OA was identified since all the MR estimates was not significant.
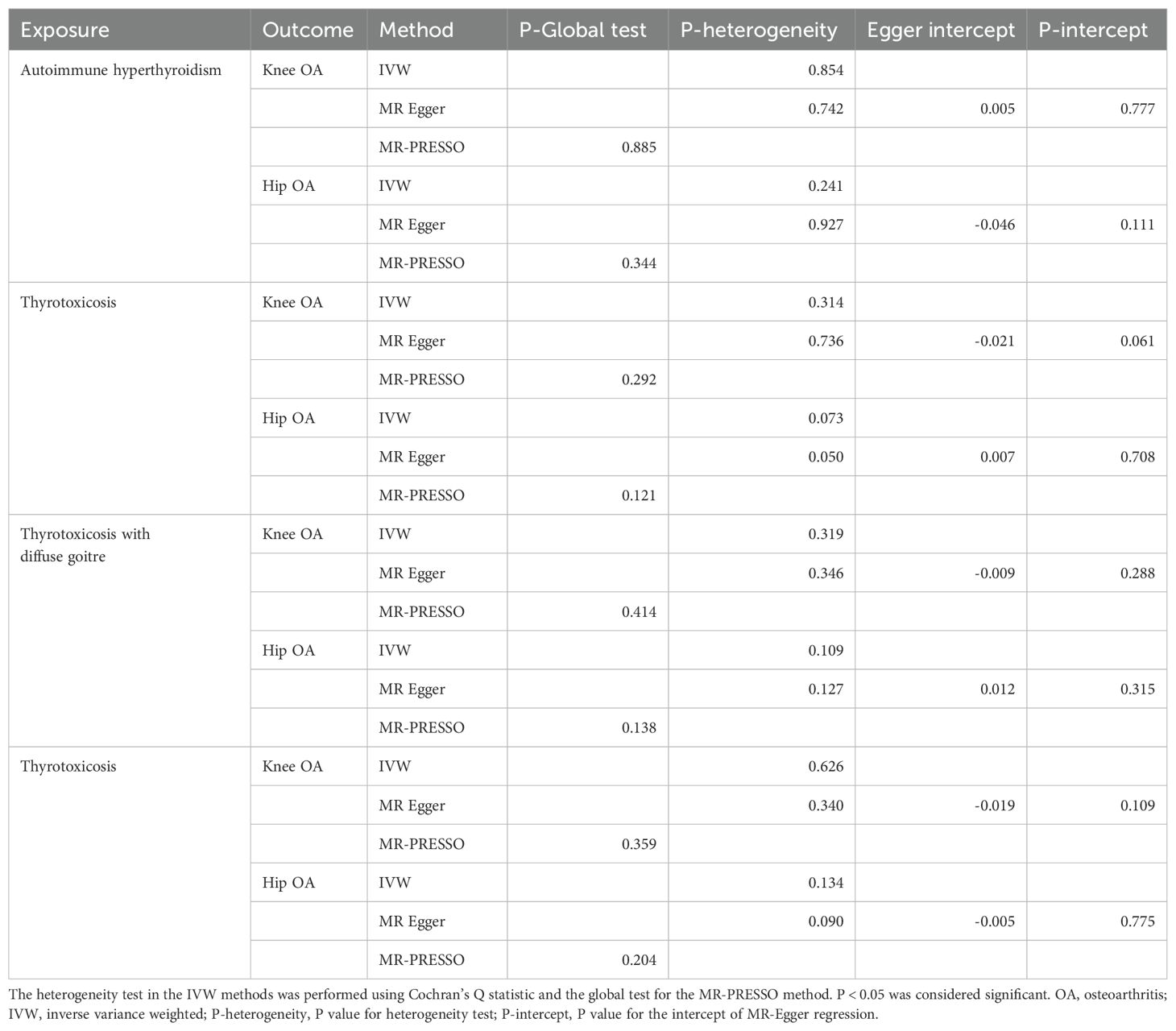
Table 2. Thyrotoxicosis-OA MR analysis of for outliers, heterogeneity, and pleiotropy of genetic instruments.
The reverse MR analyses indicates that genetic predisposition of OA was not causally associated with thyrotoxicosis (Figure 3 and Supplementary Figure 2). In the MR analysis of knee OA, the results of IVW method showed that knee OA may be associated with thyrotoxicosis (thyrotoxicosis: OR: 0.71, 95% CI: 0.52-0.96, P = 0.028, FDR = 0.441; other and/or unspecified thyrotoxicosis: OR: 0.67, 95% CI: 0.47-0.96, P = 0.027, FDR = 0.441) and the other MR methods also showed a consistent OR with a compromised P value (Figure 3). Since all P-intercept are larger than 0.05, no horizontal pleiotropy was identified (Table 3). P-Global test and P-heterogeneity of heterogeneity test were not statistically significant, indicating no heterogeneity in the MR analyses (Table 3), although some of funnel plots were asymmetry (Supplementary Figure 4). Leave-one-out sensitivity analysis indicated the robustness of the associations of knee OA with thyrotoxicosis (Supplementary Figure 6). However, since all FDR did not pass the multiple tests, OA appears not associated with thyrotoxicosis.
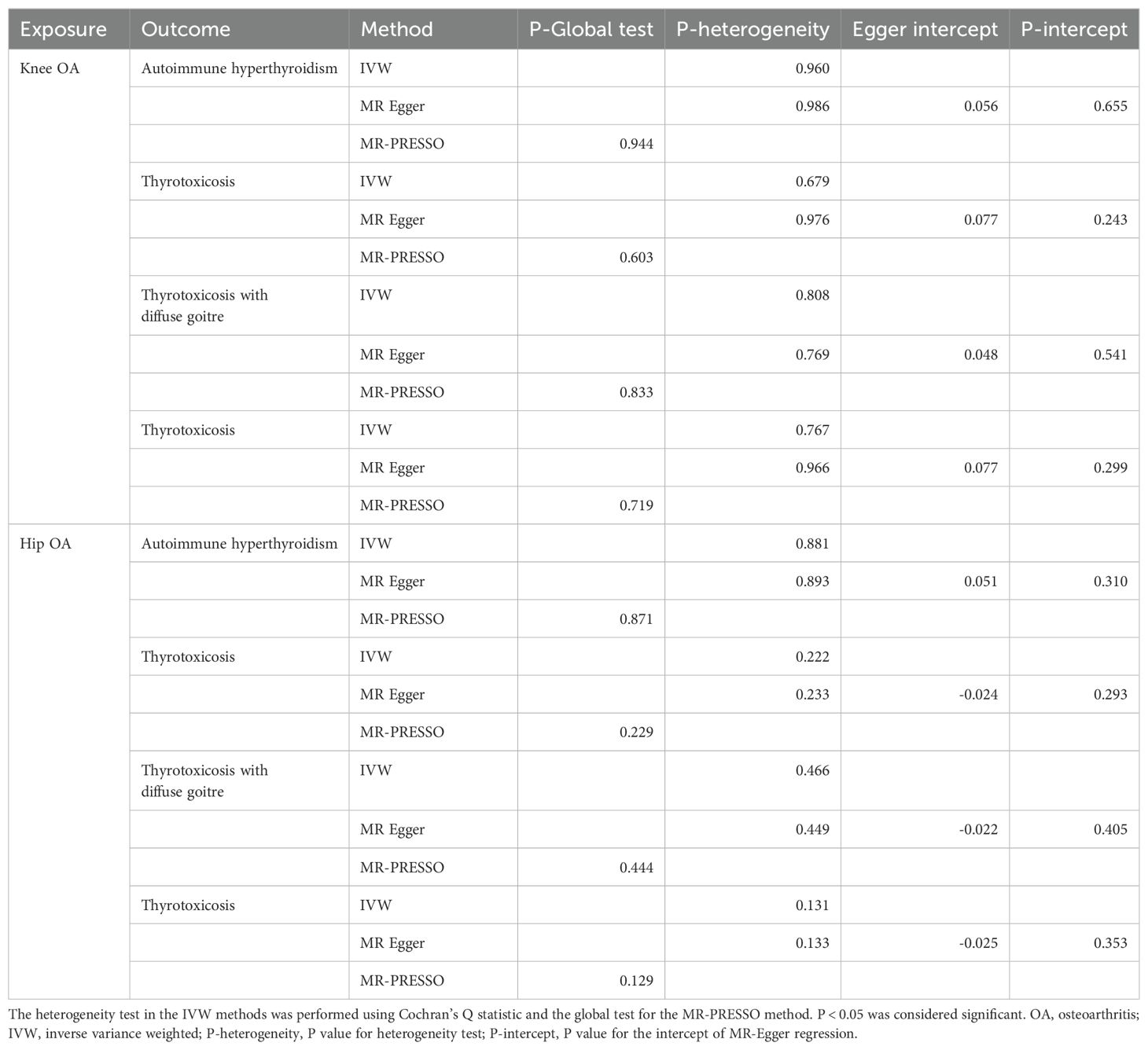
Table 3. OA-thyrotoxicosis MR analysis for outliers, heterogeneity, and pleiotropy of genetic instruments.
Discussion
The present study employed a two-sample bidirectional MR design to investigate the association between thyrotoxicosis and OA. We found that genetic predisposition of thyrotoxicosis is causally associated with the increased risk of knee OA but not hip OA, while no sufficient evidence support that OA has a reverse effect on thyrotoxicosis onset. These findings provide new insights into thyrotoxicosis and OA.
The role of THs in the development, growth, and maintenance of the skeleton is well-established. The actions of THs are mediated through their interactions with thyroid hormone receptors (TRs), including TRα1, TRβ1 and TRβ2 (35). TRα1 and TRβ1 are present in nearly all tissues, such as cartilage and bone, whereas TRβ2 is primarily expressed in the hypothalamus and pituitary (36, 37). Thyroid hormone transporters (THTs), including monocarboxylate transporters 8 and 10 (MCT8 and MCT10), organic acid transporter protein-1c1 (OATP1c1), and the non-specific L-type amino acid transporters 1 and 2 (LAT1 and LAT2), exhibit varied tissue distribution. Specifically, in the skeleton, MCT10 is predominantly expressed in the growth plate, while MCT8 is found in chondrocytes, osteoblasts, and osteoclasts (24, 38). Additionally, DIO2, essential for converting T4 to T3 in thyroid hormone metabolism, is expressed in chondrocytes and osteoblasts (39, 40). Consequently, the skeleton is a target organ for TH, indicating a potential link between thyroid-related diseases and skeletal disorders, including OA.
The epidemiological evidence linking OA and hyperthyroidism is limited. However, some lines of evidence have indicated their association. DIO2 and DIO3 were identified as OA susceptibility loci (25, 26, 41). DIO2 protein is upregulated in OA cartilage, and the mutation rs225014 of DIO2 gene may be associated with increased OA risk (42). This suggests that DIO2 may be a therapeutic target of OA. Nevertheless, DIO2 also acts as an anti-inflammatory regulator in chondrocytes (40). Inhibiting DIO2 markedly intensifies IL-1β-induced inflammatory responses (40). Therefore, the detailed role of DIO2 remains elucidated and understanding DIO2 levels in the articular cartilage of hyperthyroidism patients is crucial for establishing the connection between DIO2 and OA. Additionally, the TR agonist eprotirome (KB2115) has been shown to induce dose-related articular cartilage damage (28, 29), suggesting that hyperthyroidism-related OA risk might be contingent on the activation of TR downstream signaling.
The potential biological connection between two diseases is subchondral bone lesion. One critical musculoskeletal complication of thyrotoxicosis is osteoporosis. Thyrotoxicosis-related bone loss is primarily attributed to accelerated resorption, which is not offset by a corresponding increase in bone formation. This phenomenon has been predominantly linked to elevated thyroid hormone levels (43). The bone loss associated with high bone turnover may extend to the subchondral bone of joints as well, which is closely linked with the early bone loss and OA onset (5, 44). Elevated TR expression and enhanced nuclear translocation were observed in the osteoblasts of human OA knee (45), indicating that TR signaling is activated in OA osteoblasts. The TR signaling has positive regulation on VEGF expression, a critical factor of angiogenesis inducing OA subchondral bone lesions, since silencing TRα expression decrease VEGF in osteoblasts (45). MMP13, a well-document catabolic factor degrading cartilage, also decreased in chondrocytes when co-cultured with TRα-silenced osteoblasts (45). Thus, treatment with siRNA of TRα succeeded in attenuating subchondral bone loss and cartilage degeneration in OA mouse model (45). Although these lines of evidence suggest a critical role of TR signaling in OA subchondral bone lesions while a more comprehensive study that employs the TH administration into health joint is needed to check whether TH could induce subchondral bone remodeling and OA onset which supports a direction contribution of TH to OA by the actions on joint. Other musculoskeletal symptoms, such as muscular weakness, may contribute to OA. Muscular weakness can increase joint instability, leading to abnormal mechanical loading and subsequent cartilage damage (46).
This study has several limitations. Firstly, the findings of this study are only limited to the European populations due to all the data sources are based on European ancestry. Secondly, since this study is based on summary-level data, the details of the population such as age and sex and the disease-related traits such as duration and treatment are analyzed and the stratification analyses of this factors were not conducted. Thirdly, the associations between THs and OA is not included in this study. It is necessary to treat THs as continuous variables and use nonlinear Mendelian randomization (MR) analysis to explore the relationship between circulating TH concentrations and OA. However, our data source is limited to summary-level data, which restricts our ability to achieve this goal. Finally, while statistically significant in indicating a causal relationship, the association between thyrotoxicosis and OA may be modest. The presence of causal yet small effect sizes underscores the necessity to delve into the underlying mechanisms, offering valuable insights for future research directions. Furthermore, these small effect sizes could bear practical implications, particularly when viewed within a multifactorial framework and considering their cumulative impact. Grasping the role of these factors alongside others is crucial for a comprehensive understanding of OA development or progression.
Conclusions
Genetic predisposition of thyrotoxicosis is associated with the onset of knee OA but not hip OA in European population, while no sufficient evidence support that OA has a reverse effect on thyrotoxicosis onset. Patients with thyrotoxicosis should be vigilant about joint health and be aware of the potential risk of developing OA.
Data availability statement
The original contributions presented in the study are included in the article/Supplementary Material. Further inquiries can be directed to the corresponding authors.
Ethics statement
The requirement of ethical approval was waived by Ethics Committee of Southern Medical University for the studies involving humans because this study is based on the summary-level data of open-access public databases of GWAS. All GWASs acquired appropriate consent from participants and obtained ethical approval in accordance with the national legislation and the institutional requirements in the original studies.
Author contributions
ZH: Writing – original draft, Visualization, Investigation, Formal analysis, Data curation, Conceptualization. ZG: Data curation, Formal analysis, Writing – review & editing. SJ: Data curation, Validation, Writing – review & editing. WX: Writing – review & editing, Software, Data curation. XH: Writing – original draft, Software, Data curation. JC: Writing – review & editing, Writing – original draft, Software, Data curation. JZ: Writing – review & editing, Writing – original draft, Investigation, Data curation, Conceptualization.
Funding
The author(s) declare financial support was received for the research, authorship, and/or publication of this article. This work was supported by the Startup Fund for Distinguished Scholars of Shenzhen Hospital, Southern Medical University (Grant No. KYQD-2024-01, JZ).
Conflict of interest
The authors declare that the research was conducted in the absence of any commercial or financial relationships that could be construed as a potential conflict of interest.
Publisher’s note
All claims expressed in this article are solely those of the authors and do not necessarily represent those of their affiliated organizations, or those of the publisher, the editors and the reviewers. Any product that may be evaluated in this article, or claim that may be made by its manufacturer, is not guaranteed or endorsed by the publisher.
Supplementary material
The Supplementary Material for this article can be found online at: https://www.frontiersin.org/articles/10.3389/fendo.2024.1364027/full#supplementary-material
Supplementary Table 1 | Selection procedures of genetic instruments.
Supplementary Table 2 | Genetic instruments proxying genetic predisposition of thyrotoxicosis.
Supplementary Table 3 | Genetic instruments proxying genetic predisposition of OA.
References
1. Collaborators G.B.D.O. Global, regional, and national burden of osteoarthritis 1990-2020 and projections to 2050: a systematic analysis for the Global Burden of Disease Study 2021. Lancet Rheumatol. (2023) 5:e508–22. doi: 10.1016/S2665-9913(23)00163-7
2. Yao Q, Wu X, Tao C, Gong W, Chen M, Qu M, et al. Osteoarthritis: pathogenic signaling pathways and therapeutic targets. Signal Transduct Target Ther. (2023) 8:56. doi: 10.1038/s41392-023-01330-w
3. Wang W, Ma Z, Feng X, Ren J, Sun S, Shao Y, et al. TfR1 mediated iron metabolism dysfunction as a potential therapeutic target for osteoarthritis. Arthritis Res Ther. (2024) 26:71. doi: 10.1186/s13075-024-03304-x
4. Olivotto E, Trisolino G, Belluzzi E, Lazzaro A, Strazzari A, Pozzuoli A, et al. Macroscopic synovial inflammation correlates with symptoms and cartilage lesions in patients undergoing arthroscopic partial meniscectomy: A clinical study. J Clin Med. (2022) 11:4330. doi: 10.3390/jcm11154330
5. Wan J, He Z, Peng R, Wu X, Zhu Z, Cui J, et al. Injectable photocrosslinking spherical hydrogel-encapsulated targeting peptide-modified engineered exosomes for osteoarthritis therapy. J Nanobiotechnol. (2023) 21:284. doi: 10.1186/s12951-023-02050-7
6. Ghouri A, Muzumdar S, Barr AJ, Robinson E, Murdoch C, Kingsbury SR, et al. The relationship between meniscal pathologies, cartilage loss, joint replacement and pain in knee osteoarthritis: a systematic review. Osteoarthritis Cartilage. (2022) 30:1287–327. doi: 10.1016/j.joca.2022.08.002
7. Tang S, Yao L, Ruan J, Kang J, Cao Y, Nie X, et al. Single-cell atlas of human infrapatellar fat pad and synovium implicates APOE signaling in osteoarthritis pathology. Sci Transl Med. (2024) 16:eadf4590. doi: 10.1126/scitranslmed.adf4590
8. Wallace DT, Riches PE, Picard F. The assessment of instability in the osteoarthritic knee. EFORT Open Rev. (2019) 4:70–6. doi: 10.1302/2058-5241.4.170079
9. Neogi T. The epidemiology and impact of pain in osteoarthritis. Osteoarthritis Cartilage. (2013) 21:1145–53. doi: 10.1016/j.joca.2013.03.018
10. Hunter DJ, March L, Chew M. Osteoarthritis in 2020 and beyond: a lancet commission. Lancet. (2020) 396:1711–2. doi: 10.1016/S0140-6736(20)32230-3
11. Vina ER, Kwoh CK. Epidemiology of osteoarthritis: literature update. Curr Opin Rheumatol. (2018) 30:160–7. doi: 10.1097/BOR.0000000000000479
12. Roos EM, Arden NK. Strategies for the prevention of knee osteoarthritis. Nat Rev Rheumatol. (2016) 12:92–101. doi: 10.1038/nrrheum.2015.135
13. Im GI. The concept of early osteoarthritis and its significance in regenerative medicine. Tissue Eng Regener Med. (2022) 19:431–6. doi: 10.1007/s13770-022-00436-6
14. Rahmati M, Nalesso G, Mobasheri A, Mozafari M. Aging and osteoarthritis: Central role of the extracellular matrix. Ageing Res Rev. (2017) 40:20–30. doi: 10.1016/j.arr.2017.07.004
15. Wang LJ, Zeng N, Yan ZP, Li JT, Ni GX. Post-traumatic osteoarthritis following ACL injury. Arthritis Res Ther. (2020) 22:57. doi: 10.1186/s13075-020-02156-5
16. Nedunchezhiyan U, Varughese I, Sun AR, Wu X, Crawford R, Prasadam I. Obesity, inflammation, and immune system in osteoarthritis. Front Immunol. (2022) 13:907750. doi: 10.3389/fimmu.2022.907750
17. Sampath SJP, Venkatesan V, Ghosh S, Kotikalapudi N. Obesity, metabolic syndrome, and osteoarthritis-an updated review. Curr Obes Rep. (2023) 12:308–31. doi: 10.1007/s13679-023-00520-5
18. Mullur R, Liu YY, Brent GA. Thyroid hormone regulation of metabolism. Physiol Rev. (2014) 94:355–82. doi: 10.1152/physrev.00030.2013
19. Ogundipe VML, Groen AH, Hosper N, Nagle PWK, Hess J, Faber H, et al. Generation and differentiation of adult tissue-derived human thyroid organoids. Stem Cell Rep. (2021) 16:913–25. doi: 10.1016/j.stemcr.2021.02.011
20. Sharma A, Stan MN. Thyrotoxicosis: diagnosis and management. Mayo Clin Proc. (2019) 94:1048–64. doi: 10.1016/j.mayocp.2018.10.011
21. Menconi F, Marcocci C, Marino M. Diagnosis and classification of Graves’ disease. Autoimmun Rev. (2014) 13:398–402. doi: 10.1016/j.autrev.2014.01.013
22. Franklyn JA, Boelaert K. Thyrotoxicosis. Lancet. (2012) 379:1155–66. doi: 10.1016/S0140-6736(11)60782-4
23. Wiersinga WM, Poppe KG, Effraimidis G. Hyperthyroidism: aetiology, pathogenesis, diagnosis, management, complications, and prognosis. Lancet Diabetes Endocrinol. (2023) 11:282–98. doi: 10.1016/S2213-8587(23)00005-0
24. Williams GR. Thyroid hormone actions in cartilage and bone. Eur Thyroid J. (2013) 2:3–13. doi: 10.1016/S2665-9913(23)00163-7
25. Kerkhof HJ, Lories RJ, Meulenbelt I, Jonsdottir I, Valdes AM, Arp P, et al. A genome-wide association study identifies an osteoarthritis susceptibility locus on chromosome 7q22. Arthritis Rheum. (2010) 62:499–510. doi: 10.1002/art.27184
26. Meulenbelt I, Bos SD, Chapman K, van der Breggen R, Houwing-Duistermaat JJ, Kremer D, et al. Meta-analyses of genes modulating intracellular T3 bio-availability reveal a possible role for the DIO3 gene in osteoarthritis susceptibility. Ann Rheum Dis. (2011) 70:164–7. doi: 10.1136/ard.2010.133660
27. Kim BY, Kim SS, Park HK, Kim HS. Assessment of the relationship between knee ultrasound and clinical symptoms in patients with thyroid dysfunction. J Int Med Res. (2020) 48:300060519897701. doi: 10.1177/0300060519897701
28. Ladenson PW, Kristensen JD, Ridgway EC, Olsson AG, Carlsson B, Klein I, et al. Use of the thyroid hormone analogue eprotirome in statin-treated dyslipidemia. N Engl J Med. (2010) 362:906–16. doi: 10.1056/NEJMoa0905633
29. Sjouke B, Langslet G, Ceska R, Nicholls SJ, Nissen SE, Ohlander M, et al. Eprotirome in patients with familial hypercholesterolaemia (the AKKA trial): a randomised, double-blind, placebo-controlled phase 3 study. Lancet Diabetes Endocrinol. (2014) 2:455–63. doi: 10.1016/S2213-8587(14)70006-3
30. Sekula P, Del Greco MF, Pattaro C, Kottgen A. Mendelian randomization as an approach to assess causality using observational data. J Am Soc Nephrol. (2016) 27:3253–65. doi: 10.1681/ASN.2016010098
31. Lv Z, Cui J, Zhang J, He L. Lifestyle factors and subacromial impingement syndrome of the shoulder: potential associations in finnish participants. BMC Musculoskelet Disord. (2024) 25:220. doi: 10.1186/s12891-024-07345-w
32. Lv Z, Zhao Y, Cui J, Zhang J. Genetically proxied sarcopenia-related muscle traits and depression: evidence from the finnGen cohort. Am J Geriatr Psychiatry. (2024) 32:32–41. doi: 10.1016/j.jagp.2023.08.001
33. Cheung CL, Lau KS, Ho AY, Lee KK, Tiu SC, Lau EY, et al. Genome-wide association study identifies a susceptibility locus for thyrotoxic periodic paralysis at 17q24.3. Nat Genet. (2012) 44:1026–9. doi: 10.1038/ng.2367
34. Tachmazidou I, Hatzikotoulas K, Southam L, Esparza-Gordillo J, Haberland V, Zheng J, et al. Identification of new therapeutic targets for osteoarthritis through genome-wide analyses of UK Biobank data. Nat Genet. (2019) 51:230–6. doi: 10.1038/s41588-018-0327-1
35. Ortiga-Carvalho TM, Sidhaye AR, Wondisford FE. Thyroid hormone receptors and resistance to thyroid hormone disorders. Nat Rev Endocrinol. (2014) 10:582–91. doi: 10.1038/nrendo.2014.143
36. Forrest D, Sjoberg M, Vennstrom B. Contrasting developmental and tissue-specific expression of alpha and beta thyroid hormone receptor genes. EMBO J. (1990) 9:1519–28. doi: 10.1002/embj.1990.9.issue-5
37. Abel ED, Boers ME, Pazos-Moura C, Moura E, Kaulbach H, Zakaria M, et al. Divergent roles for thyroid hormone receptor beta isoforms in the endocrine axis and auditory system. J Clin Invest. (1999) 104:291–300. doi: 10.1172/JCI6397
38. Abe S, Namba N, Abe M, Fujiwara M, Aikawa T, Kogo M, et al. Monocarboxylate transporter 10 functions as a thyroid hormone transporter in chondrocytes. Endocrinology. (2012) 153:4049–58. doi: 10.1210/en.2011-1713
39. Bassett JH, Boyde A, Howell PG, Bassett RH, Galliford TM, Archanco M, et al. Optimal bone strength and mineralization requires the type 2 iodothyronine deiodinase in osteoblasts. Proc Natl Acad Sci USA. (2010) 107:7604–9. doi: 10.1073/pnas.0911346107
40. Cheng AWM, Bolognesi M, Kraus VB. DIO2 modifies inflammatory responses in chondrocytes. Osteoarthritis Cartilage. (2012) 20:440–5. doi: 10.1016/j.joca.2012.02.006
41. Bomer N, Den Hollander W, Ramos YF, Bos SD, van der Breggen R, Lakenberg N, et al. Underlying molecular mechanisms of DIO2 susceptibility in symptomatic osteoarthritis. Ann Rheum Dis. (2015) 74:1571–9. doi: 10.1136/annrheumdis-2013-204739
42. Bos SD, Bovee JV, Duijnisveld BJ, Raine EV, Van Dalen WJ, Ramos YF, et al. Increased type II deiodinase protein in OA-affected cartilage and allelic imbalance of OA risk polymorphism rs225014 at DIO2 in human OA joint tissues. Ann Rheum Dis. (2012) 71:1254–8. doi: 10.1136/annrheumdis-2011-200981
43. Zaidi M, Davies TF, Zallone A, Blair HC, Iqbal J, Moonga SS, et al. Thyroid-stimulating hormone, thyroid hormones, and bone loss. Curr Osteoporos Rep. (2009) 7:47–52. doi: 10.1007/s11914-009-0009-0
44. Wan J, He Z, Zhao Y, Hao X, Cui J, Chen A, et al. Novel strategy of senescence elimination via toxicity-exempted kinome perturbations by nanoliposome-based thermosensitive hydrogel for osteoarthritis therapy. Adv Composites Hybrid Mater. (2023) 6:104. doi: 10.1007/s42114-023-00673-w
45. Li L, Li M, Pang Y, Wang J, Wan Y, Zhu C, et al. Abnormal thyroid hormone receptor signaling in osteoarthritic osteoblasts regulates microangiogenesis in subchondral bone. Life Sci. (2019) 239:116975. doi: 10.1016/j.lfs.2019.116975
Keywords: thyrotoxicosis, hyperthyroidism, Mendelian randomization, risk, osteoarthritis
Citation: He Z, Gong Z, Jiao S, Xiong W, Hao X, Cui J and Zhang J (2024) Genetic predisposition to thyrotoxicosis and onset of knee osteoarthritis. Front. Endocrinol. 15:1364027. doi: 10.3389/fendo.2024.1364027
Received: 01 January 2024; Accepted: 16 August 2024;
Published: 02 October 2024.
Edited by:
Erik Fink Eriksen, Spesialistsenteret Pilestredet Park, NorwayReviewed by:
Dina Keumala Sari, Universitas Sumatera Utara, IndonesiaLu Liu, Temple University, United States
Assunta Pozzuoli, University of Padua, Italy
Copyright © 2024 He, Gong, Jiao, Xiong, Hao, Cui and Zhang. This is an open-access article distributed under the terms of the Creative Commons Attribution License (CC BY). The use, distribution or reproduction in other forums is permitted, provided the original author(s) and the copyright owner(s) are credited and that the original publication in this journal is cited, in accordance with accepted academic practice. No use, distribution or reproduction is permitted which does not comply with these terms.
*Correspondence: Jiaming Zhang, amlhbWluZ196aGFuZ3RqbWNAaWNsb3VkLmNvbQ==; Jiarui Cui, anJmZmZhaXJ5YmFiaUAxNjMuY29t; Xiaoxia Hao, eHhoYW90am11QDE2My5jb20=
†These authors have contributed equally to this work