- 1Centro de Investigaciones Endocrinológicas “Dr. César Bergadá” (CEDIE), CONICET – FEI – División de Endocrinología, Hospital de Niños Ricardo Gutiérrez, Buenos Aires, Argentina
- 2Universidad de Buenos Aires, Facultad de Medicina, Departamento de Biología Celular, Histología, Embriología y Genética, Buenos Aires, Argentina
- 3Instituto de Investigaciones Biomédicas, Santa Fe, Argentina
Anti-Müllerian hormone (AMH) is a Sertoli cell-secreted glycoprotein involved in male fetal sex differentiation: it provokes the regression of Müllerian ducts, which otherwise give rise to the Fallopian tubes, the uterus and the upper part of the vagina. In the first trimester of fetal life, AMH is expressed independently of gonadotropins, whereas from the second trimester onwards AMH testicular production is stimulated by FSH and oestrogens; at puberty, AMH expression is inhibited by androgens. AMH has also been suggested to participate in testicular descent during fetal life, but its role remains unclear. Serum AMH is a well-recognized biomarker of testicular function from birth to the first stages of puberty. Especially in boys with nonpalpable gonads, serum AMH is the most useful marker of the existence of testicular tissue. In boys with cryptorchidism, serum AMH levels reflect the mass of functional Sertoli cells: they are lower in patients with bilateral than in those with unilateral cryptorchidism. Interestingly, serum AMH increases after testis relocation to the scrotum, suggesting that the ectopic position result in testicular dysfunction, which may be at least partially reversible. In boys with cryptorchidism associated with micropenis, low AMH and FSH are indicative of central hypogonadism, and serum AMH is a good marker of effective FSH treatment. In patients with cryptorchidism in the context of disorders of sex development, low serum AMH is suggestive of gonadal dysgenesis, whereas normal or high AMH is found in patients with isolated androgen synthesis defects or with androgen insensitivity. In syndromic disorders, assessment of serum AMH has shown that Sertoli cell function is preserved in boys with Klinefelter syndrome until mid-puberty, while it is affected in patients with Noonan, Prader-Willi or Down syndromes.
1 Introduction
The existence of a second testicular factor, in addition to testosterone, involved in fetal male sex differentiation was first suggested by the pioneering work carried out by the French scientist Alfred Jost in the 1940’s and 1950’s (1, 2). He called this factor “the Müllerian inhibitor” because it provoked the regression of the Müllerian ducts, the anlagen of the Fallopian tubes, the uterus and the upper portion of the vagina (Figure 1A). One of his trainees, the Parisian pediatrician Nathalie Josso, subsequently led the group that demonstrated that the “Müllerian inhibitor” −also called Müllerian inhibiting substance (MIS) or factor (MIF)− was a glycoprotein (7) secreted by the immature Sertoli cell (8), and named it anti-Müllerian hormone (AMH) (9).
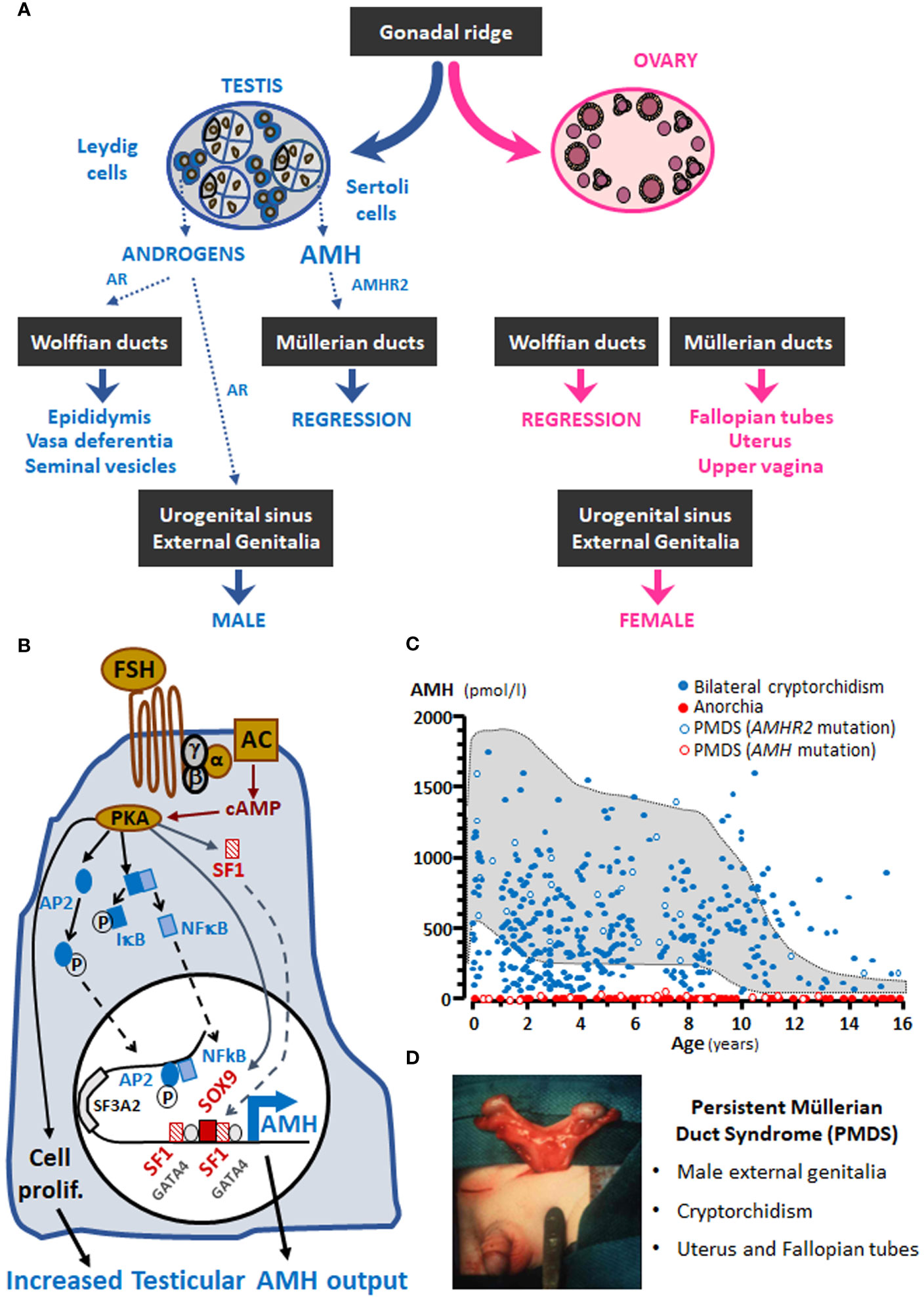
Figure 1 (A) AMH involvement in male fetal sex differentiation. (B) Regulation of testicular AMH production by FSH. (C) Serum AMH levels in boys with nonpalpable gonads; the shaded area represents normal levels. (D) PMDS: persistent Müllerian duct syndrome. α, β and γ, subunits of the Gs protein involved in FSH receptor signalling; AC, adenylyl cyclase; AP2, GATA4, IκB, NFκB, SF1 and SOX9, transcription factors involved in AMH expression regulation; AR, androgen receptor; cAMP, cyclic adenosine monophosphate; P, phosphorylated proteins; PKA, protein kinase A; prolif, proliferation. Modified with permission from: Rey and Grinspon (2) © 2010 Elsevier Ltd. (part A), Lasala et al. (3) © 2011 American Physiological Society (part B), and Josso et al. (4) © 2013 Hindawi Publishing Corporation (part C). Data in part C were obtained from Grinspon et al. (5) and Grinspon et al. (6).
1.1 AMH: the protein and the gene
AMH is a 560-aminoacid glycoprotein that contains a 24-aminoacid signal sequence. It is synthesized by the fetal Sertoli cell as a precursor homodimer of approximately 140 kDa, composed of a 110-kDa N-terminal pro-region and a 25-kDa C-terminal region. After post-translational modifications due to proteolysis (10), the N-terminal and C-terminal dimers stay associated forming a biologically active non-covalent complex (11, 12). When AMH binds to its receptor through its C-terminal domain, the N-terminal pro-region is displaced from the non-covalent complex (13). A cysteine knot motif in the C-terminal region of AMH and its post-translational processing are typical of the transforming growth factor beta (TGFβ) superfamily, to which AMH belongs (14).
The human AMH gene is approximately 2.75-kb long and consists of 5 exons (14). It maps to the short arm of chromosome 19 at position 13.3 (15), between SF3A2, encoding splicing factor 3A subunit 2 [also known as SAP62 (16)] and JSRP1, coding for the junctional sarcoplasmic reticulum protein 1. Interestingly, both genes are less than 400 bp distant from AMH. The major transcription initiation site in the male gonad is 10 bp upstream of the ATG codon (17), the biologically active C-terminal domain is encoded by the 3’ end of the 5th exon, and the polyadenylation signal is 90 nucleotides downstream of the TGA codon (14). A functional initiator (Inr) element in the human AMH gene is specifically recognized by transcription factor TFII-I (18). The proximal promoter has binding sites for SOX9, the main transactivating factor in the fetal Sertoli cell (3, 19), and for SF1, which is also a major regulator of AMH expression in humans (20) and rodents (3, 21–23). Response elements for GATA factors (22, 24, 25), SP1 (17) and AP1 (3) have also been found in the proximal 500 bp of the human AMH promoter (Figure 1B). Initially, the finding of SF3A2/SAP62 less than 800 bp upstream of the ATG codon in the human AMH gene and less than 500 bp upstream in the mouse gene (16) abridged the attention to the proximal promoter. However, in vivo experimental studies in mice showed that more distant sequences are necessary for the maintenance of Amh expression after birth (26). Subsequent studies identified the physiological relevance of binding sites for AP2, NFκB (27) and the oestrogen receptor α (ERα) (28), present more than 1700 bp upstream of the ATG codon.
1.2 AMH expression
The testes are by far the most important source of AMH. The ovaries also produce AMH, though in much lesser amounts. In agonadal individuals, e.g. pure gonadal dysgenesis, bilateral anorchia, complete primary ovarian failure or post-castration, AMH is undetectable in serum (Figure 1C). Very tiny quantities of AMH have been detected in other organs, as discussed below.
1.2.1 AMH expression in the testis and its regulation
AMH activity and its best known function are related to its testicular origin (1). AMH begins to be expressed in the fetal male gonad as soon as Sertoli cells differentiate, i.e. in the 6th embryonic week (8 weeks of amenorrhea) in humans (29). Thus, AMH is one of the earliest markers of Sertoli cell differentiation and function. AMH protein expression is limited to the rough endoplasmic reticulum and the Golgi apparatus of Sertoli cells (30, 31). Although its best characterized action, provoking the regression of Müllerian ducts, is completed by the 10th fetal week (32), AMH continues to be secreted by the testis throughout fetal and postnatal life.
Due to its time-restricted and sex-specific action during early fetal life, AMH synthesis and secretion is tightly regulated. The initiation of AMH expression in fetal Sertoli cells is independent of gonadotropins. The SRY-family transcription factor SOX9 triggers AMH gene transcription after binding to a specific response element present in the proximal promoter (19, 33, 34), 151 bp upstream of translation initiation site ATG, according to the most recent consensus nomenclature (35). Subsequently, SF1 upregulates AMH expression (21, 34) after binding to specific response elements at -102 and -228 of the human AMH promoter (20). Therefore, Müllerian duct regression occurs without any need for gonadotropin regulation of testicular function.
Sertoli cells continue to express high amounts of AMH during fetal life, infancy and childhood. FSH plays a role in the increase of AMH production by the testes, through two mechanisms (Figure 1B): it induces Sertoli cell proliferation and upregulates AMH gene expression (27, 36). FSH action is transduced by the classical G protein-coupled FSH receptor pathway involving the Gsα subunit (37) and cyclic AMP (27, 38). Three downstream kinase-mediated mechanisms are involved in AMH transcriptional upregulation through proximal promoter sequences: PKA increases Sertoli cell expression of SOX9, SF1 and GATA4 as well as nuclear translocation of SF1; PI3K/PKB action increases the effects of SF1 and GATA4; and MEK1/2 and p38 MAPK enhance GATA4-mediated AMH upregulation (3). SF1 cooperates with GATA4 (39) and WT1 (40) to upregulate AMH transcription, while DAX1 counteracts the SF1/GATA4 action on AMH expression (41). On the other hand, PKA also boosts distal AMH promoter activity mediated by transcription factors AP2 and NFκB (27).
Finally, FSH induces aromatization of androgens to oestrogens, which upregulate AMH transcription involving ERα binding to distal AMH promoter sequences and, to a lesser extent, the G-coupled oestrogen receptor GPER, also called GPER1 or GPR30 (28).
During pubertal development, Sertoli cells undergo maturation, which is characterized by their proliferation arrest, a progressive decrease in AMH expression (42) and a switch of AMH directional secretion from the basal compartment, driving AMH to blood vessels in the interstitial tissue, to the adluminal compartment, directing AMH to the seminal fluid (43). Androgens are the major regulators of Sertoli cell maturation (44) and of AMH downregulation. Indeed, in Tfm mice with a natural androgen receptor (AR) defect (36) and in genetically modified mice lacking the AR in Sertoli cells (45), AMH expression persists high at pubertal age. Testosterone and dihydrotestosterone (DHT) downregulate the activity of the human AMH promoter despite the absence of canonical androgen response elements (ARE). The inhibitory effect of androgens on AMH expression is mediated either through blockage by interaction, i.e. an interaction between the AR and SF1, or through blockage by competition due to a direct binding of the AR on SF1 response elements in the proximal AMH promoter (23). The inhibitory effect of androgens prevails over the stimulating effect of FSH and oestrogens during pubertal maturation and adulthood (Figure 1C). The downregulation of testicular AMH production is coincident with other androgen-dependent processes, such as the establishment of the blood-testis barrier and the onset of adult spermatogenesis (36, 46, 47). Although intratesticular testosterone concentrations are high in the fetus and neonate, AMH is not downregulated owing to the lack of expression of the AR in Sertoli cells in those periods of life (48–50). In the adult male, AMH levels are 10- to 20-fold lower as compared to childhood (51), yet 2-fold higher than in females (52).
1.2.2 AMH expression in other organs
In the ovary, AMH begins to be expressed in the 25th fetal week (53), when Müllerian ducts are no longer sensitive to its action (32, 54). In humans, like shown in other mammals in the 1980´s (55, 56), AMH is produced mainly by granulosa cells of primary and small antral follicles, and decreases in large follicles; no AMH expression is seen in the corpus luteum, corpus albicans or atretic follicles (57, 58). As compared to the testis, ovarian AMH secretion is much lower and more stable throughout life, with a moderate peak during puberty or early adulthood and a decrease from the age of 25-30 years until menopause, when it becomes undetectable in serum (52, 59–61). The regulation of AMH expression in the ovary has received less attention than in the testis. FOXL2 and WNT4 are believed to trigger AMH expression in granulosa cells, with SF1 and GATA involved as upregulators. FSH and cyclic AMP, as well as members of the BMP family, have been shown to enhance AMH production in the ovary, whereas the effects of oestrogens and androgens remain unclear, probably depending on follicular stage [for review, see ref. (62)].
AMH mRNA has been found in other organs, such as neurons and gonadotrophs, using ultrasensitive techniques, which has prompted the hypothesis that AMH has other functions in the central nervous system (62–64). The fact that AMH cannot be detected in circulation in individuals lacking gonadal tissue and that patients with biallelic pathogenic variants in AMH or its specific receptor AMHR2 only show the persistence of the uterus, with no neurologic symptoms (35) challenge these new findings or, at least, indicate that the proposed actions for AMH beyond fetal development are redundantly assured by other factors.
1.3 AMH action in target tissues
In concordance with members of the TGFβ family, AMH signals through two membrane-bound receptors with serine-threonine kinase activity. AMH must be cleaved −although the dissociation of the N-terminal and C-terminal fragments is not absolutely necessary− to bind to the specific AMH type 2 receptor (AMHR2). Subsequently, a nonspecific type 1 receptor is recruited by AMHR2 to transduce its signal (65). The type 1 receptor used varies according to the target tissue: in the mesenchymal cells that surround the Müllerian duct epithelium, AMHR2 recruits type 1 receptors ACVR1 and BMPR1A to phosphorylate intracellular proteins SMAD1/5/8 (62). A few target genes have been identified in rodents, including Osx, Mmp2 and Wif1 (66). AMH induces apoptosis and epithelial-mesenchymal transformation, finally leading to Müllerian duct regression (67). In the female fetus, lacking AMH expression during the critical period, and in male fetuses with abolished AMH production, owing to AMH gene mutations, or action, due to AMHR2 mutations, Müllerian ducts are maintained and differentiate into the Fallopian tubes, the uterus and the upper third of the vagina. This condition, replicated in male mice with Amh (68) or Amhr2 (69) gene knockouts, is known as the persistent Müllerian duct syndrome (PMDS, Figure 1D) (65). Müllerian derivatives also persist in individuals with dysgenetic gonads leading to insufficient testicular hormone production and resulting in disorders of sex development (DSD) (70).
2 AMH and testicular descent during fetal life
The biphasic model of fetal testicular descent is the most accepted proposal to explain how the testes descend from their original intra-abdominal position, when they differentiate from the urogenital ridge in the 7th week, to the scrotum a few weeks before birth (71–74). The three hormones produced by the differentiating testis, AMH, androgens and insulin-like peptide 3 (INSL3), have been suggested to promote testis descent (Figure 2). The fetal testis is attached to the abdominal wall by the cranial suspensory ligament at its upper pole and the gubernaculum at its lower pole together with the epididymis. The initial or transabdominal phase of testicular descent is characterized by the regression of the cranial ligament and the thickening of the gubernaculum attaching to the inguinal region. In the second or inguino-scrotal phase, the testis descends along the inguinal canal into the scrotum.
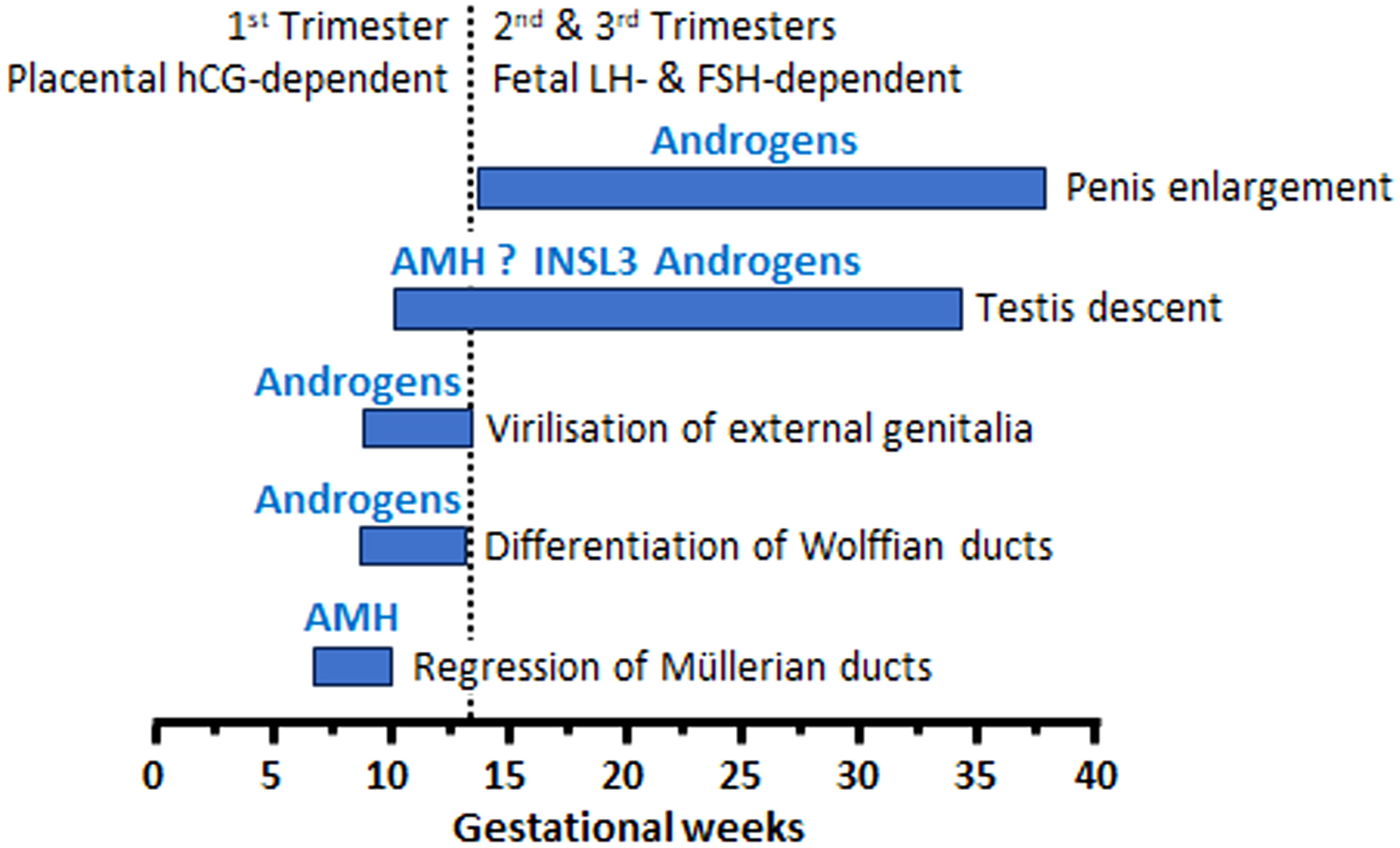
Figure 2 Hormones involved in male sex differentiation, including testicular descent during fetal life.
INSL3 is the main testicular factor that controls the enlargement of the gubernaculum (73, 74), whereas androgens play some role in the gubernaculum swelling reaction. Androgens also provoke the regression of the cranial suspensory ligament (71). Both INSL3 and androgens are secreted by Leydig cells under the stimulus of human chorionic gonadotrophin (hCG) in the first 10-20 weeks of fetal life and LH in the second part of gestation.
The role of AMH in testis descent remains controversial. Until the 1990´s AMH was proposed as a candidate for the swelling reaction of the gubernaculum (75), but INSL3 was later recognized as the main responsible (71). Further observations in mice provided experimental evidence against an action for AMH on the gubernaculum (68, 76). However, in vitro studies showed that AMH might enhance the effect of INSL3 on the gubernaculum (77). Furthermore, in patients with PMDS due to AMH or AMHR2 inactivating mutations, testicular descent does not occur normally. There are 3 presentations of cryptorchidism in patients with PMDS (35, 71): in the first one, both testes remain in intraabdominal position attached to the uterus and Fallopian tubes (similar to the normal ovarian position); in the second presentation, one testis is scrotal tracking the homolateral Fallopian tube and the contralateral testis remains intraabdominal (this is known as “hernia uteri inguinalis”); finally, the third situation is characterized by the presence of both testes, Fallopian tubes and uterus in one hemi-scrotum (known as “transverse testicular ectopia”). In all conditions the gubernaculum is long, indicating a lack of the swelling reaction, which could be explained by AMH signaling failure (71). The unusually long gubernaculum allows abnormal mobility of the testes, which are at increased risk of torsion (78). Alternatively, cryptorchidism in patients with PMDS could be explained by the retention of Müllerian derivatives which prevent testicular descent owing to a mechanical obstruction (35). In summary, there is no clear evidence that AMH is involved in testicular descent: although clinical observations in patients with PMDS seem to suggest a role for AMH in the INSL3-mediated swelling of the gubernaculum, experimental evidence could not be obtained from rodent models, probably due to species differences as regard the physiology of testicular descent between rodents and humans (71). In fact, in the human the gubernacular cord shortens in mid gestation explaining in part that testicular descent is usually complete by birth, whereas rodents have a long gubernacular cord that enables the testis inside the abdomen or the inguinal canal until puberty. Therefore, AMH may have a more impactful effect on INSL3-mediated control of the gubernacular swelling reaction in humans than in rodents (71). The demonstration of a timely expression of AMHR2 and its downstream signaling pathway in the gubernaculum is essential to support the hypothesis of AMH involvement in testicular descent during fetal life.
3 AMH as a biomarker of testicular function
Beyond its physiological role in Müllerian duct regression during the first trimester of fetal life and its potential roles in the physiology of the ovary and the hypothalamic-gonadotroph axis, AMH has proved to be a useful biomarker of testicular function and, especially, of its most conspicuous cell population, the immature Sertoli cell, in pediatric ages (4, 79–81).
The circulating AMH levels are commensurate with the mass of functional Sertoli cells in the fetus (29), newborns (82), infants and children (51, 83). In males of pubertal age, serum AMH is indicative of the maturation status of Sertoli cells, reflecting testosterone concentration and action within the testis (84, 85). This is especially useful in the early stages of pubertal development, i.e. Tanner stages 2 and 3 (86), when circulating testosterone may still be very low or undetectable (51, 87, 88).
Measurement of serum AMH is informative of the amount of functional testicular tissue in patients with DSD (89). Serum AMH is undetectable, indicating a complete absence of functional testicular tissue, in patients with complete gonadal dysgenesis, while it is below the male range −and probably above the female range− in patients with partial testicular dysgenesis. Conversely, serum AMH is in the male range or above in patients with isolated steroidogenic defects or androgen insensitivity. In 46,XX virilized patients, serum AMH is within the female range, indicating absence of testicular tissue, when the etiology is aromatase deficiency, androgen-secreting tumors or congenital adrenal hyperplasia. Conversely, it is above the female range in the case of 46,XX ovotesticular or testicular DSD (90).
In normally virilized 46,XY boys of prepubertal age with enlarged testes, low serum AMH is suggestive of precocious puberty, either central (87, 91) or peripheral (87), and its increase after treatment is indicative of successful reduction of intratesticular hormone concentration (87, 91). On the other hand, normal or high AMH levels rule out precocious maturation and suggest prepubertal macroorchidism in boys under 9 years with testicular volume ≥4 ml. High AMH may also reflect the existence of hypoestrogenic states (28), excessive FSH downstream signaling (37) or Sertoli cell tumors (92). The usefulness of serum AMH as a biomarker in patients with conditions that may present with cryptorchidism will be addressed in detail below.
4 AMH in boys with cryptorchidism
Cryptorchidism is the clinical sign resulting from an impaired testicular descent during fetal life (93), or from the re-ascent occurring later in life, when the cremasteric reflex is established (94). Cryptorchidism may be the consequence of a primary testicular dysfunction, of a central disorder affecting the GnRH-gonadotroph axis, or of an anatomic malformation independent of any endocrine disorder (Table 1) (72). Whichever the case may be, cryptorchidism is a frequent complaint in infants and children brought to the pediatrician at an age when the LH-Leydig cell axis is quiescent. Therefore, the assessment of the existence and the mass of functional testicular tissue relies mainly on the assessment of the Sertoli cell population. The clinical utility of measuring serum AMH levels in boys with cryptorchidism will be discussed.
4.1 Boys with nonpalpable gonads: cryptorchidism vs anorchia
When the gonads are not in the scrotum and cannot be detected in the inguinal regions by palpation or by ultrasonography, the first step in the diagnostic process is to establish the existence of functional testicular tissue. Basal serum gonadotropins and testosterone do not prove useful: testosterone is usually undetectable in serum after the age of 3-6 months (51, 95) and gonadotrophin levels may be normal even in the absence of testicular tissue (5). Testosterone levels measured after hCG stimulation were used in the past searching for the existence of functional testicular tissue; however, it is no longer a choice except when there is a specific interest in assessing Leydig cell function. Direct markers of the existence of prepubertal testicular tissue, such as AMH or inhibin B, have become the first choice. Indeed, basal serum AMH proves to be more informative than testosterone post-hCG both in boys with abdominal testes and in boys with anorchia (96). The predictive value of detectable serum AMH for the presence of testes was 98% and that of undetectable serum AMH for anorchia was 92% (Figure 3); the only false negative result was observed in a boy with PMDS due to an AMH mutation who had testes but serum AMH was undetectable.
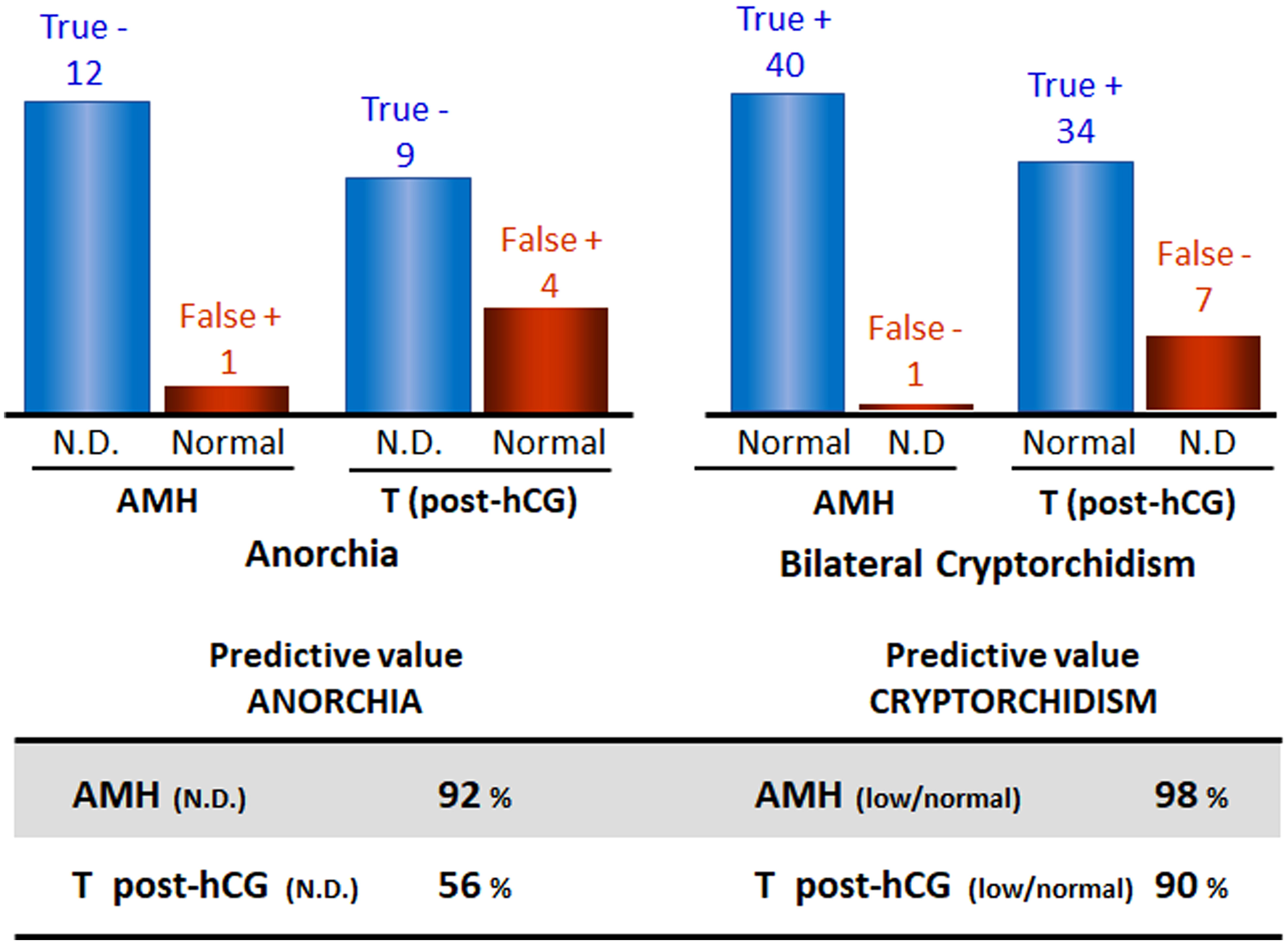
Figure 3 Predictive values of serum AMH and testosterone (T) post-hCG in boys with nonpalpable gonads for the existence of functional testicular tissue. Data from: Lee et al. (96). N.D., non-detectable.
Furthermore, serum AMH assessment has better predictive values than imaging studies for abdominal testes (97, 98) and may be more sensitive than surgical procedures for the identification of the existence of testicular tissue (99).
4.2 Assessment of testicular function in boys with isolated cryptorchidism
For decades, the treatment of cryptorchidism has focused on bringing the testes to the scrotum in an adequate and timely manner, as if placing the gonads in their proper position solved everything, without much attention having been paid to their functional status. However, it seems clear that assessing testicular function at the time of diagnosis can help to understand the pathophysiology of cryptorchidism in each patient and its potential fertility prognosis, as well as to appraise the effect of treatment.
Serum AMH levels are commensurate with the amount of functional testicular tissue. Most studies have found that serum AMH is overall lower in boys with cryptorchidism than in the general population (100, 101). However, AMH levels are not systematically decreased in all boys with cryptorchidism, and a few studies could not detect a significant difference as compared to control boys (102, 103). In boys with cryptorchidism, low serum AMH is suggestive of hypogonadism, reflecting a decreased Sertoli cell mass and, therefore, a reduced testicular size and/or an impaired Sertoli cell function (6, 104–112). Low AMH levels have also been suggested to predict a decreased number of germ cells in the testes (113, 114)
4.2.1 Unilateral vs bilateral cryptorchidism
Since cryptorchidism is a sign of heterogeneous disorders (Table 1) (72), a wide range of serum AMH levels can be found in patients with undescended testes. As expected, testicular AMH output is more affected in boys with bilateral undescended testes than in those with unilateral cryptorchidism (Figure 4A). Mean or median serum AMH is significantly lower (approximately -420 to -560 pmol/l, equivalent to -60 to -80 ng/ml) in boys with bilateral cryptorchidism than in age-matched controls, while a significant difference is not always evident between boys with unilateral cryptorchidism and controls (6, 104, 113, 115). Nevertheless, a large study showed that serum AMH was clearly below the normal range in 16% of boys with unilateral cryptorchidism aged 1-6 months and in 7% of older infants and prepubertal boys. In patients with bilaterally undescended testes, serum AMH was abnormally low in 14% of infants < 6 months-old and in 19-37% of older infants and prepubertal children (6). Another study showed that serum AMH was normal in only half of the boys with cryptorchidism, without distinguishing between unilateral and bilateral forms (105). Preterm birth does not seem to have an influence on testicular function in cryptorchid boys before puberty (Figure 4B) (6).
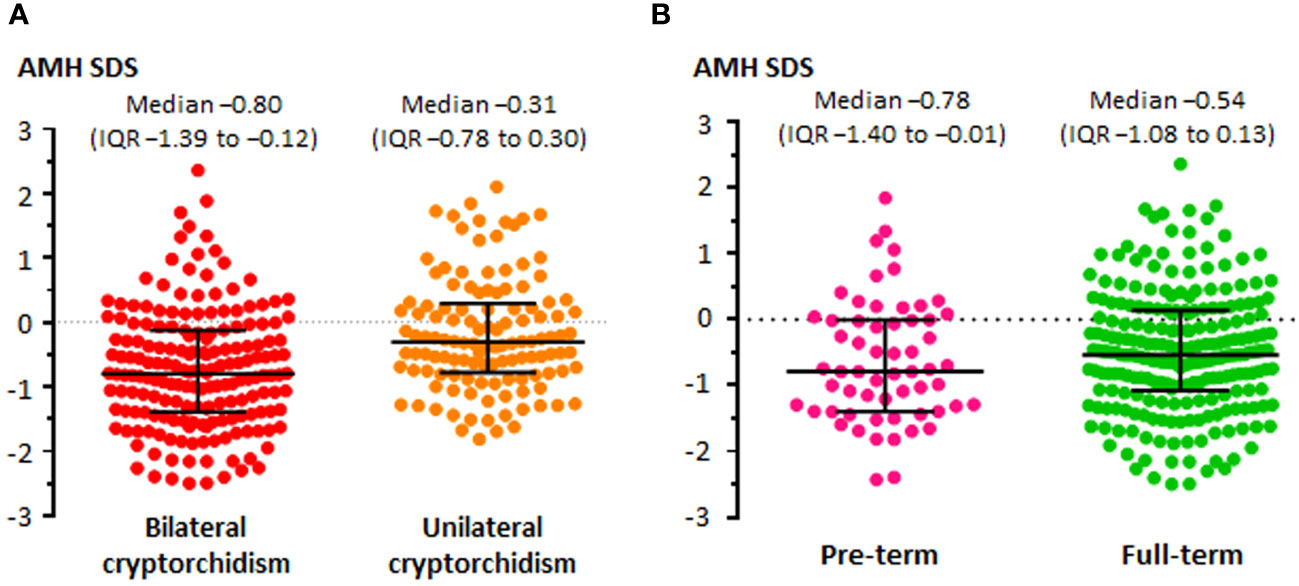
Figure 4 Levels of serum AMH, expressed in standard deviation scores (SDS), in boys with unilateral or bilateral cryptorchidism (A), and in boys with unilateral or bilateral cryptorchidism born pre-term or full-term (B). Modified with permission, from Grinspon et al. (6).
4.2.2 Before and after orchiopexy
The impaired testicular function in patients with cryptorchidism may have two explanations: a primary gonadal dysfunction that results in a disordered testicular descent to the scrotum and, on the other hand, the longstanding stay of the gonad in an ectopic position that affects its normal function. In boys with cryptorchidism, serum AMH increases after successful orchiopexy (6, 106, 111), indicating that the correction of the abnormal position may have a positive influence, even if partial, on testicular function (Figure 5).
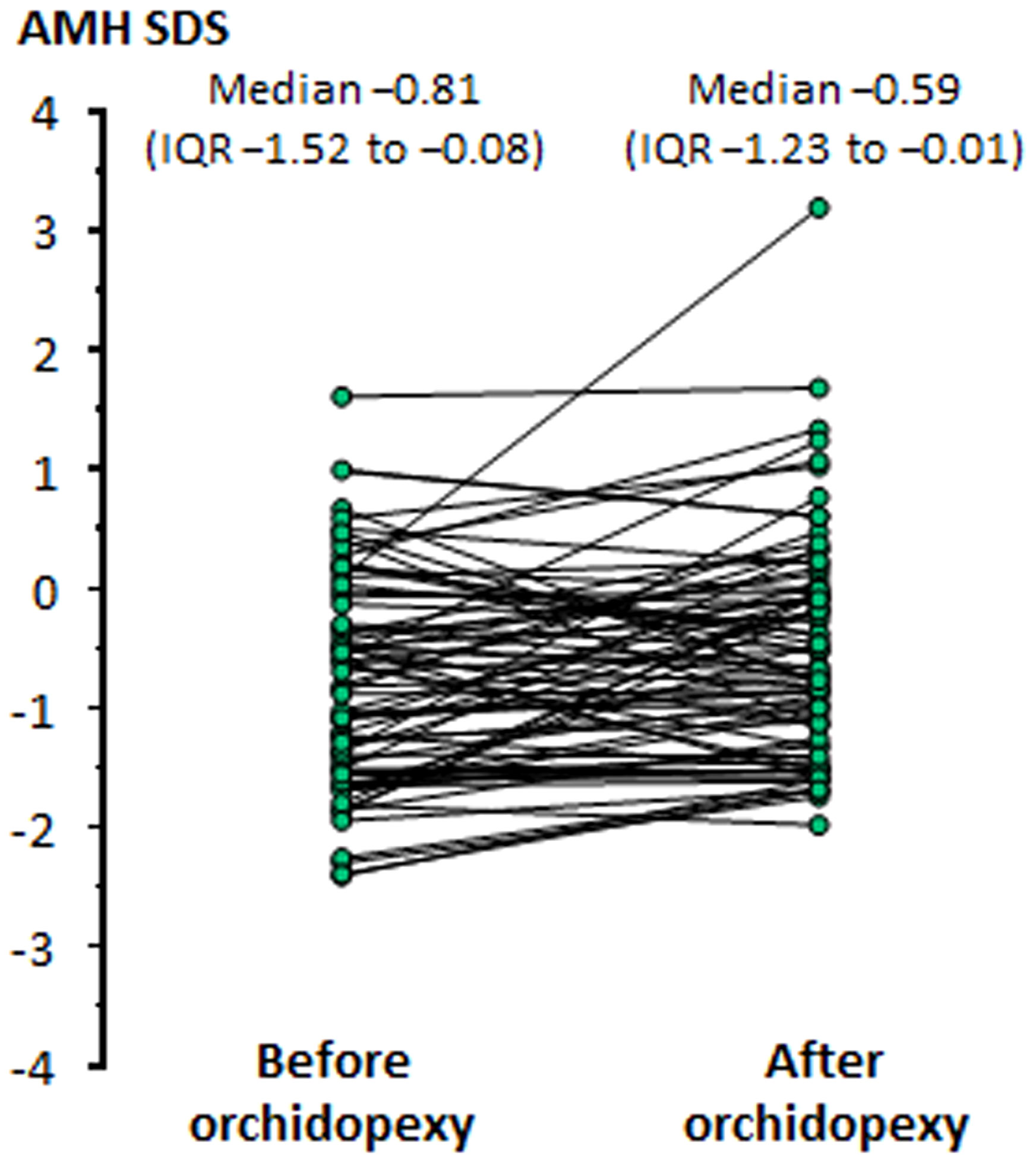
Figure 5 Levels of serum AMH, expressed in standard deviation scores (SDS), in boys before and after orchiopexy Modified with permission, from Grinspon et al. (6).
4.3 Assessment of testicular function in boys with cryptorchidism and associated genital anomalies
The co-occurrence of cryptorchidism with other genital anomalies, such as micropenis, hypospadias or ambiguous genitalia, or with syndromic features increases the risk of testicular dysfunction.
4.3.1 Cryptorchidism and micropenis
The existence of micropenis may be a sign of defective androgen action during the second half of fetal life, which can be due to a primary testicular dysfunction such as testicular regression syndrome or to central (hypogonadotrophic) hypogonadism (116). In a newborn or infant <6 months-old with cryptorchidism and micropenis, low serum AMH associated with low testosterone and gonadotrophins are strongly suggestive of congenital central hypogonadism (108, 117). Treatment with gonadotrophins results in an increase in serum AMH and testis volume, reflecting FSH action on Sertoli cells, as well as a rise in serum testosterone, reflecting LH action on Leydig cells and leading to penile enlargement and testis descent (117–120). Testicular regression results in very low or undetectable AMH levels, together with low or undetectable levels of the other testicular hormones and elevated gonadotrophins.
4.3.2 Cryptorchidism and hypospadias or ambiguous genitalia
The coexistence of cryptorchidism with hypospadias and incompletely fused labioscrotal swellings is indicative of insufficient androgen action in the first trimester of fetal life in a 46,XY individual resulting in disorders of sex development (DSD) (70). This can be due to a disorder of gonadal development (testicular dysgenesis), or to a disorder of androgen biosynthesis (Leydig cell hypoplasia, steroidogenic enzyme defects) or action (androgen insensitivity). Serum testosterone is below the male range in both disorders of gonadal development and of androgen biosynthesis. Serum AMH determination can be useful to distinguish between them (Figure 6), since it is below the male range in patients with testicular dysgenesis but within the normal range or above in patients with defects of androgen synthesis (112, 121–126). The coexistence of testosterone and AMH levels in the male range is suggestive of partial androgen insensitivity (121, 124, 126).
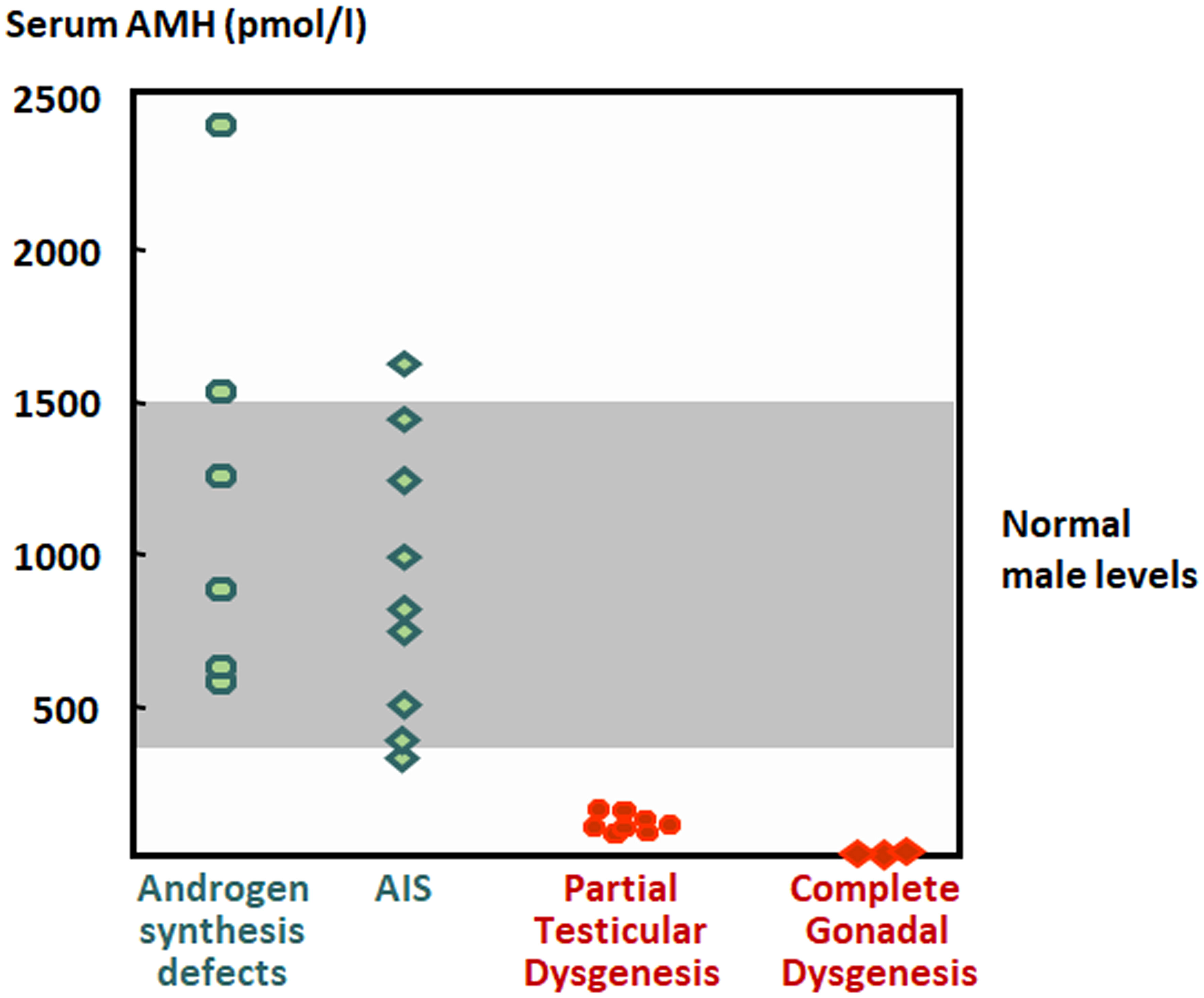
Figure 6 Levels of serum AMH in boys with disorders of sex development. The shaded area represents normal levels. AIS, androgen insensitivity syndrome. Modified with permission, from Rey et al. (121). © 1999 The Endocrine Society.
4.3.3 Cryptorchidism in genetic syndromes
Cryptorchidism is a relatively common feature of genetic syndromes resulting from sex chromosome (47,XXY Klinefelter syndrome) or autosome (Trisomy 21, Down syndrome) aneuploidies, or from single locus disorders, such as Noonan and Prader-Willi syndromes (72).
In patients with Klinefelter syndrome, serum AMH, as well as other reproductive hormones, are within the normal range in infants and children, indicating that endocrine testis function is not affected before puberty (127–130).
Trisomy 21 is characterized by an early establishment of primary hypogonadism, as reflected by lower serum AMH levels, as compared to age-matched controls, during infancy and childhood. Sertoli cell dysfunction was independent from the occurrence of cryptorchidism in patients with Down syndrome (51).
In boys with Noonan syndrome associated with PTPN11 gene variants, serum AMH is low as compared to the general population, which suggests a Sertoli cell dysfunction; conversely, normal AMH levels have been found in boys with Noonan syndrome with pathogenic variants in SOS1 (131).
Boys with Prader-Willi syndrome show a mild Sertoli cell dysfunction, resulting in serum AMH levels in the lower half of the reference range, with a mild increase in gonadotrophins, from early infancy (132) throughout puberty (133, 134)
5 Concluding remarks
AMH is a well-established biomarker of the immature Sertoli cell, from the fetal stage until mid-puberty. Its unequivocal role is to induce the regression of Müllerian ducts in the male fetus. Other physiological roles of AMH, such as its involvement in testicular descent, remain to be ascertained. Nonetheless, serum AMH determination is a useful tool to assess the existence and functional capacity of testicular tissue during infancy, childhood and early puberty in boys with cryptorchidism, either isolated or associated with micropenis or with other signs of fetal undervirilization.
Author contributions
RR: Conceptualization, Writing – original draft, Writing – review & editing. RG: Writing – review & editing.
Funding
The author(s) declare that no financial support was received for the research, authorship, and/or publication of this article.
Conflict of interest
Until 2020, RR received royalties for the development of an AMH ELISA kit and both authors received honoraria for technology services using the AMH ELISA.
The author(s) declared that they were an editorial board member of Frontiers, at the time of submission. This had no impact on the peer review process and the final decision.
Publisher’s note
All claims expressed in this article are solely those of the authors and do not necessarily represent those of their affiliated organizations, or those of the publisher, the editors and the reviewers. Any product that may be evaluated in this article, or claim that may be made by its manufacturer, is not guaranteed or endorsed by the publisher.
Glossary
References
1. Jost A. Problems of fetal endocrinology: the gonadal and hypophyseal hormones. Recent Prog Hormone Res. (1953) 8:379–418.
2. Rey RA, Grinspon RP. Normal male sexual differentiation and aetiology of disorders of sex development. Best Pract Res Clin Endocrinol Metab. (2011) 25:221–38. doi: 10.1016/j.beem.2010.08.013
3. Lasala C, Schteingart HF, Arouche N, Bedecarrás P, Grinspon RP, Picard JY, et al. SOX9 and SF1 are involved in cyclic AMP-mediated upregulation of anti-Mullerian gene expression in the testicular prepubertal Sertoli cell line SMAT1. Am J Physiol Endocrinol Metab. (2011) 301:E539–547. doi: 10.1152/ajpendo.00187.2011
4. Josso N, Rey RA, Picard JY. Anti-müllerian hormone: a valuable addition to the toolbox of the pediatric endocrinologist. Int J Endocrinol. (2013) 2013:674105. doi: 10.1155/2013/674105
5. Grinspon RP, Ropelato MG, Bedecarrás P, Loreti N, Ballerini MG, Gottlieb S, et al. Gonadotrophin secretion pattern in anorchid boys from birth to pubertal age: pathophysiological aspects and diagnostic usefulness. Clin Endocrinol (Oxf). (2012) 76:698–705. doi: 10.1111/j.1365-2265.2011.04297.x
6. Grinspon RP, Gottlieb S, Bedecarras P, Rey RA. Anti-müllerian hormone and testicular function in prepubertal boys with cryptorchidism. Front Endocrinol (Lausanne). (2018) 9:182. doi: 10.3389/fendo.2018.00182
7. Picard JY, Tran D, Josso N. Biosynthesis of labelled anti-Müllerian hormone by fetal testes: evidence for the glycoprotein nature of the hormone and for its disulfide-bonded structure. Mol Cell Endocrinology. (1978) 12:17–30. doi: 10.1016/0303-7207(78)90098-9
8. Tran D, Meusy-Dessolle N, Josso N. Anti-Müllerian hormone is a functional marker of foetal Sertoli cells. Nature. (1977) 269:411–2. doi: 10.1038/269411a0
9. Josso N. WOMEN IN REPRODUCTIVE SCIENCE: Anti-Mullerian hormone: a look back and ahead. Reproduction. (2019) 158:F81–9. doi: 10.1530/REP-18-0602
10. Pepinsky RB, Sinclair LK, Chow EP, Mattaliano RJ, Manganaro TF, Donahoe PK, et al. Proteolytic processing of mullerian inhibiting substance produces a transforming growth factor-beta-like fragment. J Biol Chem. (1988) 263:18961–4. doi: 10.1016/S0021-9258(18)37375-7
11. Wilson CA, di Clemente N, Ehrenfels C, Pepinsky RB, Josso N, Vigier B, et al. Müllerian inhibiting substance requires its N-terminal domain for maintenance of biological activity, a novel finding within the transforming growth factor-beta superfamily. Mol Endocrinol. (1993) 7:247–57. doi: 10.1210/mend.7.2.8469238
12. Nachtigal MW, Ingraham HA. Bioactivation of Mullerian inhibiting substance during gonadal development by a kex2/subtilisin-like endoprotease. Proc Natl Acad Sci United States America. (1996) 93:7711–6. doi: 10.1073/pnas.93.15.7711
13. Cate RL, di Clemente N, Racine C, Groome NP, Pepinsky RB, Whitty A. The anti-Mullerian hormone prodomain is displaced from the hormone/prodomain complex upon bivalent binding to the hormone receptor. J Biol Chem. (2022) 298:101429. doi: 10.1016/j.jbc.2021.101429
14. Cate RL, Mattaliano RJ, Hession C, Tizard R, Farber NM, Cheung A, et al. Isolation of the bovine and human genes for Müllerian inhibiting substance and expression of the human gene in animal cells. Cell. (1986) 45:685–98. doi: 10.1016/0092-8674(86)90783-X
15. Cohen-Haguenauer O, Picard JY, Mattei MG, Serero S, Nguyen VC, de Tand MF, et al. Mapping of the gene for anti-Müllerian hormone to the short arm of human chromosome 19. Cytogenet Cell Genet. (1987) 44:2–6.
16. Dresser DW, Hacker A, Lovell-Badge R, Guerrier D. The genes for a spliceosome protein (SAP62) and the anti-Müllerian hormone (AMH) are contiguous. Hum Mol Genet. (1995) 4:1613–8. doi: 10.1093/hmg/4.9.1613
17. Guerrier D, Boussin L, Mader S, Josso N, Kahn A, Picard JY. Expression of the gene for anti-Müllerian hormone. JReprodFertil. (1990) 88:695–706. doi: 10.1530/jrf.0.0880695
18. Morikawa N, Clarke TR, Novina CD, Watanabe K, Haqq C, Weiss M, et al. Human Mullerian-inhibiting substance promoter contains a functional TFII-I-binding initiator. BiolReprod. (2000) 63:1075–83. doi: 10.1095/biolreprod63.4.1075
19. de Santa Barbara P, Bonneaud N, Boizet B, Desclozeaux M, Moniot B, Sudbeck P, et al. Direct interaction of SRY-related protein SOX9 and steroidogenic factor 1 regulates transcription of the human anti-Müllerian hormone gene. Mol Cell Biol. (1998) 18:6653–65. doi: 10.1128/mcb.18.11.6653
20. Schteingart HF, Picard JY, Valeri C, Marshall I, Treton D, di Clemente N, et al. A mutation inactivating the distal SF1 binding site on the human anti-Mullerian hormone promoter causes persistent Mullerian duct syndrome. Hum Mol Genet. (2019) 28:3211–8. doi: 10.1093/hmg/ddz147
21. Shen WH, Moore CC, Ikeda Y, Parker KL, Ingraham HA. Nuclear receptor steroidogenic factor 1 regulates the Müllerian inhibiting substance gene: a link to the sex determination cascade. Cell. (1994) 77:651–61. doi: 10.1016/0092-8674(94)90050-7
22. Watanabe K, Clarke TR, Lane AH, Wang X, Donahoe PK. Endogenous expression of Mullerian inhibiting substance in early postnatal rat Sertoli cells requires multiple steroidogenic factor-1 and GATA-4-binding sites. Proc Natl Acad Sci USA. (2000) 97:1624–9. doi: 10.1073/pnas.97.4.1624
23. Edelsztein NY, Racine C, di Clemente N, Schteingart HF, Rey RA. Androgens downregulate anti-Mullerian hormone promoter activity in the Sertoli cell through the androgen receptor and intact SF1 sites. Biol Reprod. (2018) 99:1303–12. doi: 10.1093/biolre/ioy152
24. Tremblay JJ, Viger RS. Transcription factor GATA-4 enhances Mullerian inhibiting substance gene transcription through a direct interaction with the nuclear receptor SF-1. Mol Endocrinol. (1999) 13:1388–401. doi: 10.1210/me.13.8.1388
25. Beau C, Rauch M, Joulin V, Jégou B, Guerrier D. GATA-1 is a potential repressor of anti-Müllerian hormone expression during the establishment of puberty in the mouse. Mol Reprod Dev. (2000) 56:124–38. doi: 10.1002/(sici)1098-2795(200006)56:2<124::aid-mrd2>3.0.co;2-j
26. Beau C, Vivian N, Münsterberg A, Dresser DW, Lovell-Badge R, Guerrier D. In vivo analysis of the regulation of the anti-Müllerian hormone, as a marker of Sertoli cell differentiation during testicular development, reveals a multi-step process. Mol Reprod Dev. (2001) 59:256–64. doi: 10.1002/mrd.1030
27. Lukas-Croisier C, Lasala C, Nicaud J, Bedecarrás P, Kumar TR, Dutertre M, et al. Follicle-stimulating hormone increases testicular Anti-Müllerian hormone (AMH) production through Sertoli cell proliferation and a nonclassical cyclic adenosine 5'-monophosphate-mediated activation of the AMH gene. Mol Endocrinol. (2003) 17:550–61. doi: 10.1210/me.2002-0186
28. Valeri C, Lovaisa MM, Racine C, Edelsztein NY, Riggio M, Giulianelli S, et al. Molecular mechanisms underlying AMH elevation in hyperoestrogenic states in males. Sci Rep. (2020) 10:15062. doi: 10.1038/s41598-020-71675-7
29. Josso N, Lamarre I, Picard JY, Berta P, Davies N, Morichon N, et al. Anti-Müllerian hormone in early human development. Early Hum Dev. (1993) 33:91–9. doi: 10.1016/0378-3782(93)90204-8
30. Tran D, Josso N. Localization of anti-Müllerian hormone in the rough endoplasmic reticulum of the developing bovine Sertoli cell using immunocytochemistry with a monoclonal antibody. Endocrinology. (1982) 111:1562–7. doi: 10.1210/endo-111-5-1562
31. Hayashi M, Shima H, Hayashi K, Trelstad RL, Donahoe PK. Immunocytochemical localization of Mullerian inhibiting substance in the rough endoplasmic reticulum and Golgi apparatus in Sertoli cells of the neonatal calf testis using a monoclonal antibody. J HistochemCytochem. (1984) 32:649–54. doi: 10.1177/32.6.6373916
32. Taguchi O, Cunha GR, Lawrence WD, Robboy SJ. Timing and irreversibility of Müllerian duct inhibition in the embryonic reproductive tract of the human male. DevBiol. (1984) 106:394–8. doi: 10.1016/0012-1606(84)90238-0
33. Morais da Silva S, Hacker A, Harley V, Goodfellow P, Swain A, Lovell-Badge R. Sox9 expression during gonadal development implies a conserved role for the gene in testis differentiation in mammals and birds. Nat Genet. (1996) 14:62–8. doi: 10.1038/ng0996-62
34. Arango NA, Lovell-Badge R, Behringer RR. Targeted mutagenesis of the endogenous mouse Mis gene promoter: in vivo definition of genetic pathways of vertebrate sexual development. Cell. (1999) 99:409–19. doi: 10.1016/s0092-8674(00)81527-5
35. Picard JY, Cate RL, Racine C, Josso N. The persistent mullerian duct syndrome: an update based upon a personal experience of 157 cases. Sex Dev. (2017) 11:109–25. doi: 10.1159/000475516
36. Al-Attar L, Noël K, Dutertre M, Belville C, Forest MG, Burgoyne PS, et al. Hormonal and cellular regulation of Sertoli cell anti-Müllerian hormone production in the postnatal mouse. J Clin Invest. (1997) 100:1335–43. doi: 10.1172/JCI119653
37. Rey RA, Venara M, Coutant R, Trabut JB, Rouleau S, Lahlou N, et al. Unexpected mosaicism of R201H-GNAS1 mutant-bearing cells in the testes underlie macro-orchidism without sexual precocity in McCune-Albright syndrome. Hum Mol Genet. (2006) 15:3538–43. doi: 10.1093/hmg/ddl430
38. Voutilainen R, Miller WL. Human Müllerian inhibitory factor messenger ribonucleic acid is hormonally regulated in the fetal testis and in adult granulosa cells. Mol Endocrinol. (1987) 1:604–8. doi: 10.1210/mend-1-9-604
39. Viger RS, Mertineit C, Trasler JM, Nemer M. Transcription factor GATA-4 is expressed in a sexually dimorphic pattern during mouse gonadal development and is a potent activator of the Müllerian inhibiting substance promoter. Development. (1998) 125:2665–75. doi: 10.1242/dev.125.14.2665
40. Nachtigal MW, Hirokawa Y, Enyeart-VanHouten DL, Flanagan JN, Hammer GD, Ingraham HA. Wilms' tumor 1 and Dax-1 modulate the orphan nuclear receptor SF-1 in sex-specific gene expression. Cell. (1998) 93:445–54. doi: 10.1016/S0092-8674(00)81172-1
41. Tremblay JJ, Viger RS. Nuclear receptor Dax-1 represses the transcriptional cooperation between GATA-4 and SF-1 in Sertoli cells. Biol Reprod. (2001) 64:1191–9. doi: 10.1095/biolreprod64.4.1191
42. Tran D, Meusy-Dessolle N, Josso N. Waning of anti-Müllerian activity: an early sign of Sertoli cell maturation in the developing pig. Biol Reprod. (1981) 24:923–31. doi: 10.1095/biolreprod24.4.923
43. Josso N, Picard JY, Dacheux JL, Courot M. Detection of anti-Müllerian activity in boar rete testis fluid. J Reprod Fertil. (1979) 57:397–400. doi: 10.1530/jrf.0.0570397
44. Rey RA. The role of androgen signaling in male sexual development at puberty. Endocrinology. (2021) 162:bqaa215. doi: 10.1210/endocr/bqaa215
45. Chang C, Chen YT, Yeh SD, Xu Q, Wang RS, Guillou F, et al. Infertility with defective spermatogenesis and hypotestosteronemia in male mice lacking the androgen receptor in Sertoli cells. Proc Natl Acad Sci USA. (2004) 101:6876–81. doi: 10.1073/pnas.0307306101
46. Hirobe S, He WW, Lee MM, Donahoe PK. Mullerian inhibiting substance messenger ribonucleic acid expression in granulosa and Sertoli cells coincides with their mitotic activity. Endocrinology. (1992) 131:854–62. doi: 10.1210/endo.131.2.1639028
47. Rey R, Al-Attar L, Louis F, Jaubert F, Barbet P, Nihoul-Fékété C, et al. Testicular dysgenesis does not affect expression of anti-mullerian hormone by Sertoli cells in premeiotic seminiferous tubules. Am J Pathology. (1996) 148:1689–98.
48. Berensztein EB, Baquedano MS, González CR, Saraco NI, Rodriguez J, Ponzio R, et al. Expression of aromatase, estrogen receptor alpha and beta, androgen receptor, and cytochrome P-450scc in the human early prepubertal testis. Pediatr Res. (2006) 60:740–4. doi: 10.1203/01.pdr.0000246072.04663.bb
49. Chemes HE, Rey RA, Nistal M, Regadera J, Musse M, González-Peramato P, et al. Physiological androgen insensitivity of the fetal, neonatal, and early infantile testis is explained by the ontogeny of the androgen receptor expression in Sertoli cells. J Clin Endocrinol Metab. (2008) 93:4408–12. doi: 10.1210/jc.2008-0915
50. Boukari K, Meduri G, Brailly-Tabard S, Guibourdenche J, Ciampi ML, Massin N, et al. Lack of androgen receptor expression in Sertoli cells accounts for the absence of anti-Mullerian hormone repression during early human testis development. J Clin Endocrinol Metab. (2009) 94:1818–25. doi: 10.1210/jc.2008-1909
51. Grinspon RP, Bedecarrás P, Ballerini MG, Iñíguez G, Rocha A, Mantovani Rodrigues Resende EA, et al. Early onset of primary hypogonadism revealed by serum anti-Müllerian hormone determination during infancy and childhood in trisomy 21. Int J Androl. (2011) 34:e487–98. doi: 10.1111/j.1365-2605.2011.01210.x
52. Hagen CP, Aksglæde L, Sorensen K, Main KM, Boas M, Cleemann L, et al. Serum levels of anti-Mullerian hormone as a marker of ovarian function in 926 healthy females from birth to adulthood and in 172 Turner syndrome patients. J Clin Endocrinol Metab. (2010) 95:5003–10. doi: 10.1210/jc.2010-0930
53. Kuiri-Hänninen T, Kallio S, Seuri R, Tyrvainen E, Liakka A, Tapanainen J, et al. Postnatal developmental changes in the pituitary-ovarian axis in preterm and term infant girls. J Clin Endocrinol Metab. (2011) 96:3432–9. doi: 10.1210/jc.2011-1502
54. Picon R. Action du testicule foetal sur le développement in vitro des canaux de Müller chez le rat. ArchAnatMicroscMorphExp. (1969) 58:1–19.
55. Vigier B, Picard JY, Tran D, Legeai L, Josso N. Production of anti-Müllerian hormone: another homology between Sertoli and granulosa cells. Endocrinology. (1984) 114:1315–20. doi: 10.1210/endo-114-4-1315
56. Bézard J, Vigier B, Tran D, Mauleon P, Josso N. Immunocytochemical study of anti-Müllerian hormone in sheep ovarian follicles during fetal and post-natal development. J Reprod Fertil. (1987) 80:509–16. doi: 10.1530/jrf.0.0800509
57. Rajpert-De Meyts E, Jørgensen N, Græm N, Müller J, Cate RL, Skakkebæk NE. Expression of anti-Müllerian hormone during normal and pathological gonadal development: association with differentiation of Sertoli and granulosa cells. J Clin Endocrinol Metab. (1999) 84:3836–44. doi: 10.1210/jcem.84.10.6047
58. Rey R, Sabourin JC, Venara M, Long WQ, Jaubert F, Zeller WP, et al. Anti-Mullerian hormone is a specific marker of Sertoli- and granulosa-cell origin in gonadal tumors. Hum Pathol. (2000) 31:1202–8. doi: 10.1053/hupa.2000.18498
59. Rey RA, Lhommé C, Marcillac I, Lahlou N, Duvillard P, Josso N, et al. Antimüllerian hormone as a serum marker of granulosa cell tumors of the ovary: comparative study with serum alpha-inhibin and estradiol. AmJObstetGynecol. (1996) 174:958–65. doi: 10.1016/s0002-9378(96)70333-2
60. Long WQ, Ranchin V, Pautier P, Belville C, Denizot P, Cailla H, et al. Detection of minimal levels of serum anti-Müllerian hormone during follow-up of patients with ovarian granulosa cell tumor by means of a highly sensitive enzyme-linked immunosorbent assay. J Clin Endocrinol Metab. (2000) 85:540–4. doi: 10.1210/jcem.85.2.6378
61. Kelsey TW, Wright P, Nelson SM, Anderson RA, Wallace WH. A validated model of serum anti-mullerian hormone from conception to menopause. PloS One. (2011) 6:e22024. doi: 10.1371/journal.pone.0022024
62. di Clemente N, Racine C, Pierre A, Taieb J. Anti-müllerian hormone in female reproduction. Endocr Rev. (2021) 42:753–82. doi: 10.1210/endrev/bnab012
63. Wang PY, Koishi K, McGeachie AB, Kimber M, Maclaughlin DT, Donahoe PK, et al. Mullerian inhibiting substance acts as a motor neuron survival factor in vitro. Proc Natl Acad Sci U S A. (2005) 102:16421–5. doi: 10.1073/pnas.0508304102
64. Kereilwe O, Kadokawa H. Bovine gonadotrophs express anti-Mullerian hormone (AMH): comparison of AMH mRNA and protein expression levels between old Holsteins and young and old Japanese Black females. Reprod Fertil Dev. (2019) 31:810–9. doi: 10.1071/RD18341
65. Josso N, Picard JY. Genetics of anti-Mullerian hormone and its signaling pathway. Best Pract Res Clin Endocrinol Metab. (2022) 36:101634. doi: 10.1016/j.beem.2022.101634
66. Moses MM, Behringer RR. A gene regulatory network for Mullerian duct regression. Environm Epigenet. (2019) 5:dvz017. doi: 10.1093/eep/dvz017
67. Allard S, Adin P, Gouédard L, di Clemente N, Josso N, Orgebin-Crist MC, et al. Molecular mechanisms of hormone-mediated Mullerian duct regression: involvement of beta-catenin. Development. (2000) 127:3349–60. doi: 10.1242/dev.127.15.3349
68. Behringer RR, Finegold MJ, Cate RL. Müllerian-inhibiting substance function during mammalian sexual development. Cell. (1994) 79:415–25. doi: 10.1016/0092-8674(94)90251-8
69. Mishina Y, Rey R, Finegold MJ, Matzuk MM, Josso N, Cate RL, et al. Genetic analysis of the Müllerian-inhibiting substance signal transduction pathway in mammalian sexual differentiation. Genes Dev. (1996) 10:2577–87. doi: 10.1101/gad.10.20.2577
70. Grinspon RP, Bergadá I, Rey RA. Male hypogonadism and disorders of sex development. Front Endocrinol (Lausanne). (2020) 11:211. doi: 10.3389/fendo.2020.00211
71. Hutson JM, Lopez-Marambio FA. The possible role of AMH in shortening the gubernacular cord in testicular descent: A reappraisal of the evidence. J Pediatr Surg. (2017) 52:1656–60. doi: 10.1016/j.jpedsurg.2017.05.021
72. Rodprasert W, Virtanen HE, Makela JA, Toppari J. Hypogonadism and cryptorchidism. Front Endocrinol (Lausanne). (2019) 10:906. doi: 10.3389/fendo.2019.00906
73. Ivanova E, Vincel B, Verkauskas G, Hadziselimovic F. Gubernaculum and epididymo-testicular descent: review of the literature. Acta Med Litu. (2022) 29:201–10. doi: 10.15388/Amed.2022.29.2.6
74. Ivell R, Mamsen LS, Andersen CY, Anand-Ivell R. Expression and role of INSL3 in the fetal testis. Front Endocrinol (Lausanne). (2022) 13:868313. doi: 10.3389/fendo.2022.868313
75. Hutson JM, Baker M, Terada M, Zhou B, Paxton G. Hormonal control of testicular descent and the cause of cryptorchidism. Reprod FertilDev. (1994) 6:151–6. doi: 10.1071/RD9940151
76. Lyet L, Vigier B, van der Schoot P. Anti-mullerian hormone in relation to the growth and differentiation of the gubernacular primordia in mice. J Reprod Fertil. (1996) 108:281–8. doi: 10.1530/jrf.0.1080281
77. Kubota Y, Temelcos C, Bathgate RA, Smith KJ, Scott D, Zhao C, et al. The role of insulin 3, testosterone, M++llerian inhibiting substance and relaxin in rat gubernacular growth. Mol Hum Reproduction. (2002) 8:900–5. doi: 10.1093/molehr/8.10.900
78. Imbeaud S, Rey R, Berta P, Chaussain J-L, Wit J-M, Lustig RH, et al. Testicular degeneration in three patients with the persistent müllerian duct syndrome. Eur J Pediatr. (1995) 154:187–90. doi: 10.1007/BF01954268
79. Condorelli RA, Cannarella R, Calogero AE, La Vignera S. Evaluation of testicular function in prepubertal children. Endocrine. (2018) 62:274–80. doi: 10.1007/s12020-018-1670-9
80. Rey RA. Biomarkers of male hypogonadism in childhood and adolescence. Adv Lab Med. (2020) 2020:24. doi: 10.1515/almed-2020-0024
81. Kanakatti Shankar R, Dowlut-McElroy T, Dauber A, Gomez-Lobo V. Clinical utility of anti-mullerian hormone in pediatrics. J Clin Endocrinol Metab. (2022) 107:309–23. doi: 10.1210/clinem/dgab687
82. Bergadá I, Milani C, Bedecarrás P, Andreone L, Ropelato MG, Gottlieb S, et al. Time course of the serum gonadotropin surge, inhibins, and anti-Mullerian hormone in normal newborn males during the first month of life. J Clin Endocrinol Metab. (2006) 91:4092–8. doi: 10.1210/jc.2006-1079
83. Aksglæde L, Sorensen K, Boas M, Mouritsen A, Hagen CP, Jensen RB, et al. Changes in anti-Mullerian hormone (AMH) throughout the life span: a population-based study of 1027 healthy males from birth (cord blood) to the age of 69 years. J Clin Endocrinol Metab. (2010) 95:5357–64. doi: 10.1210/jc.2010-1207
84. Edelsztein NY, Rey RA. Importance of the androgen receptor signaling in gene transactivation and transrepression for pubertal maturation of the testis. Cells. (2019) 8:1–17. doi: 10.3390/cells8080861
85. Edelsztein NY, Valeri C, Lovaisa MM, Schteingart HF, Rey RA. AMH regulation by steroids in the mammalian testis: underlying mechanisms and clinical implications. Front Endocrinol (Lausanne). (2022) 13:906381. doi: 10.3389/fendo.2022.906381
86. Marshall WA, Tanner JM. Variations in the pattern of pubertal changes in boys. Arch Dis Child. (1970) 45:13–23. doi: 10.1136/adc.45.239.13
87. Rey R, Lordereau-Richard I, Carel JC, Barbet P, Cate RL, Roger M, et al. Anti-müllerian hormone and testosterone serum levels are inversely related during normal and precocious pubertal development. J Clin Endocrinol Metab. (1993) 77:1220–6. doi: 10.1210/jc.2005-2564
88. Rey R, Mebarki F, Forest MG, Mowszowicz I, Cate RL, Morel Y, et al. Anti-müllerian hormone in children with androgen insensitivity. J Clin Endocrinol Metab. (1994) 79:960–4. doi: 10.1210/jcem.79.4.7962305
89. Josso N, Rey RA. What does AMH tell us in pediatric disorders of sex development? Front Endocrinol (Lausanne). (2020) 11:619. doi: 10.3389/fendo.2020.00619
90. Grinspon RP, Rey RA. Molecular characterization of XX maleness. Int J Mol Sci. (2019) 20:6089. doi: 10.3390/ijms20236089
91. Grinspon RP, Andreone L, Bedecarrás P, Ropelato MG, Rey RA, Campo SM, et al. Male Central Precocious Puberty: Serum Profile of Anti-Mullerian Hormone and Inhibin B before, during, and after Treatment with GnRH Analogue. Int J Endocrinol. (2013) 2013:823064. doi: 10.1155/2013/823064
92. Venara M, Rey R, Bergadá I, Mendilaharzu H, Campo SM, Chemes H. Sertoli cell proliferations of the infantile testis: an intratubular form of Sertoli cell tumor? Am J Surg Pathol. (2001) 25:1237–44. doi: 10.1097/00000478-200110000-00003
93. Sijstermans K, Hack WW, Meijer RW, van der Voort-Doedens LM. The frequency of undescended testis from birth to adulthood: a review. Int J Androl. (2008) 31:1–11. doi: 10.1111/j.1365-2605.2007.00770.x
94. Hack WW, Goede J, van der Voort-Doedens LM, Meijer RW. Acquired undescended testis: putting the pieces together. Int J Androl. (2012) 35:41–5. doi: 10.1111/j.1365-2605.2011.01155.x
95. Busch AS, Ljubicic ML, Upners EN, Fischer MB, Raket LL, Frederiksen H, et al. Dynamic changes of reproductive hormones in male minipuberty: Temporal dissociation of Leydig- and Sertoli-cell activity. J Clin Endocrinol Metab. (2022) 107:1560–8. doi: 10.1210/clinem/dgac115
96. Lee MM, Donahoe PK, Silverman BL, Hasegawa T, Hasegawa Y, Gustafson ML, et al. Measurements of serum Müllerian inhibiting substance in the evaluation of children with nonpalpable gonads. New Engl J Med. (1997) 336:1480–6. doi: 10.1056/NEJM199705223362102
97. Maghnie M, Vanzulli A, Paesano P, Bragheri R, Palladini G, Preti P, et al. The accuracy of magnetic resonance imaging and ultrasonography compared with surgical findings in the localization of the undescended testis. Arch Pediatr Adolesc Med. (1994) 148:699–703. doi: 10.1001/archpedi.1994.02170070037006
98. Jespersen K, Ljubicic ML, Johannsen TH, Christiansen P, Skakkebaek NE, Juul A. Distinguishing between hidden testes and anorchia: the role of endocrine evaluation in infancy and childhood. Eur J Endocrinol. (2020) 183:107–17. doi: 10.1530/EJE-20-0041
99. McEachern R, Houle AM, Garel L, Van Vliet G. Lost and found testes: the importance of the hCG stimulation test and other testicular markers to confirm a surgical declaration of anorchia. Hormone Res. (2004) 62:124–8. doi: 10.1159/000080018
100. Kuiri-Hanninen T, Koskenniemi J, Dunkel L, Toppari J, Sankilampi U. Postnatal testicular activity in healthy boys and boys with cryptorchidism. Front Endocrinol (Lausanne). (2019) 10:489. doi: 10.3389/fendo.2019.00489
101. Lopez Dacal J, Castro S, Suco S, Correa Brito L, Grinspon RP, Rey RA. Assessment of testicular function in boys and adolescents. Clin Endocrinol (Oxf). (2023). doi: 10.1111/cen.14979
102. Pierik FH, Deddens JA, Burdorf A, de Muinck Keizer-Schrama SM, Jong FH, Weber RF. The hypothalamus-pituitary-testis axis in boys during the first six months of life: a comparison of cryptorchidism and hypospadias cases with controls Int J Androl. (2009) 32:453–61. doi: 10.1111/j.1365-2605.2008.00877.x
103. Sinopidis X, Kostopoulou E, Rojas-Gil AP, Panagidis A, Kourea E, Skiadopoulos S, et al. Association of antimullerian hormone with the size of the appendix testis, the androgen and estrogen receptors and their expression in the appendix testis, in congenital cryptorchidism. J Pediatr Endocrinol Metab. (2021) 34:1247–55. doi: 10.1515/jpem-2021-0240
104. Yamanaka J, Baker M, Metcalfe S, Hutson JM. Serum levels of Mullerian inhibiting substance in boys with cryptorchidism. JPediatrSurg. (1991) 26:621–3. doi: 10.1016/0022-3468(91)90723-7
105. Misra M, MacLaughlin DT, Donahoe PK, Lee MM. Measurement of Mullerian inhibiting substance facilitates management of boys with microphallus and cryptorchidism. J Clin Endocrinol Metab. (2002) 87:3598–602. doi: 10.1210/jcem.87.8.8742
106. Demircan M, Akinci A, Mutus M. The effects of orchiopexy on serum anti-Mullerian hormone levels in unilateral cryptorchid infants. Pediatr Surg Int. (2006) 22:271–3. doi: 10.1007/s00383-006-1646-3
107. Matuszczak E, Hermanowicz A, Debek W, Oksiuta M, Dzienis-Koronkiewicz E, Zelazowska-Rutkowska B. Serum AMH concentration as a marker evaluating gonadal function in boys operated on for unilateral cryptorchidism between 1st and 4th year of life. Endocrine. (2012) 41:334–7. doi: 10.1007/s12020-011-9551-5
108. Braslavsky D, Grinspon RP, Ballerini MG, Bedecarrás P, Loreti N, Bastida G, et al. Hypogonadotropic hypogonadism in infants with congenital hypopituitarism: A challenge to diagnose at an early stage. Horm Res Paediatr. (2015) 84:289–97. doi: 10.1159/000439051
109. Cortes D, Clasen-Linde E, Hutson JM, Li R, Thorup J. The Sertoli cell hormones inhibin-B and anti Mullerian hormone have different patterns of secretion in prepubertal cryptorchid boys. J Pediatr Surg. (2016) 51:475–80. doi: 10.1016/j.jpedsurg.2015.08.059
110. van Brakel J, de Muinck Keizer-Schrama S, Hazebroek FWJ, Dohle GR, de Jong FH. INSL3 and AMH in patients with previously congenital or acquired undescended testes. J Pediatr Surg. (2017) 52:1327–31. doi: 10.1016/j.jpedsurg.2017.03.064
111. Huang W-H, Xu L-Y, Chen S-S, Chen Z-Q, Cui X, Zhou C-M. The effect of scrotal versus inguinal orchiopexy on the testicular function of children with clinically palpable, inguinal undescended testis: a randomized controlled trial. Asian J Andrology. (2023) 25:745–9. doi: 10.4103/aja202314
112. Lucas-Herald AK, Alkanhal KI, Caney E, Malik I, Alimussina M, McNeilly JD, et al. Gonadal function in boys with bilateral undescended testes. J Endocrine Society. (2024) 8(2):bvad153. doi: 10.1210/jendso/bvad153
113. Hamdi SM, Almont T, Galinier P, Mieusset R, Thonneau P. Altered secretion of Sertoli cells hormones in 2-year-old prepubertal cryptorchid boys: a cross-sectional study. Andrology. (2017) 5:783–9. doi: 10.1111/andr.12373
114. Kato T, Mizuno K, Matsumoto D, Nishio H, Nakane A, Kurokawa S, et al. Low serum inhibin B/follicle-stimulating hormones and anti-mullerian hormone/follicle-stimulating hormones ratios as markers of decreased germ cells in infants with bilateral cryptorchidism. J Urol. (2022) 207:701–9. doi: 10.1097/JU.0000000000002344
115. Komarowska MD, Milewski R, Charkiewicz R, Matuszczak E, Sulewska A, Zelazowska-Rutkowska B, et al. Are anti-Mullerian hormone and its receptor polymorphism associated with the hormonal condition of undescended testes? Adv Med Sci. (2016) 61:288–92. doi: 10.1016/j.advms.2016.03.004
116. Rey RA, Grinspon RP, Gottlieb S, Pasqualini T, Knoblovits P, Aszpis S, et al. Male hypogonadism: an extended classification based on a developmental, endocrine physiology-based approach. Andrology. (2013) 1:3–16. doi: 10.1111/j.2047-2927.2012.00008.x
117. Bougnères P, François M, Pantalone L, Rodrigue D, Bouvattier C, Demesteere E, et al. Effects of an early postnatal treatment of hypogonadotropic hypogonadism with a continuous subcutaneous infusion of recombinant follicle-stimulating hormone and luteinizing hormone. J Clin Endocrinol Metab. (2008) 93:2202–5. doi: 10.1210/jc.2008-0121
118. Main KM, Schmidt IM, Toppari J, Skakkebæk NE. Early postnatal treatment of hypogonadotropic hypogonadism with recombinant human FSH and LH. Eur J Endocrinol. (2002) 146:75–9. doi: 10.1530/eje.0.1460075
119. Sarfati J, Bouvattier C, Bry-Gauillard H, Cartes A, Bouligand J, Young J. Kallmann syndrome with FGFR1 and KAL1 mutations detected during fetal life. Orphanet J Rare Dis. (2015) 10:71. doi: 10.1186/s13023-015-0287-9
120. Lambert AS, Bougnères P. Growth and descent of the testes in infants with hypogonadotropic hypogonadism receiving subcutaneous gonadotropin infusion. Int J Pediatr Endocrinol. (2016) 2016:13. doi: 10.1186/s13633-016-0031-9
121. Rey RA, Belville C, Nihoul-Fékété C, Michel-Calemard L, Forest MG, Lahlou N, et al. Evaluation of gonadal function in 107 intersex patients by means of serum antimüllerian hormone measurement. J Clin Endocrinol Metab. (1999) 84:627–31. doi: 10.1210/jcem.84.2.5507
122. Lee MM, Misra M, Donahoe PK, MacLaughlin DT. MIS/AMH in the assessment of cryptorchidism and intersex conditions. Mol Cell Endocrinology. (2003) 211:91–8. doi: 10.1016/j.mce.2003.09.014
123. Juniarto AZ, van der Zwan YG, Santosa A, Ariani MD, Eggers S, Hersmus R, et al. Hormonal evaluation in relation to phenotype and genotype in 286 patients with a disorder of sex development from Indonesia. Clin Endocrinol (Oxf). (2016) 85:247–57. doi: 10.1111/cen.13051
124. Johannsen TH, Main KM, Ljubicic ML, Jensen TK, Andersen HR, Andersen MS, et al. Sex differences in reproductive hormones during mini-puberty in infants with normal and disordered sex development. J Clin Endocrinol Metab. (2018) 103:3028–37. doi: 10.1210/jc.2018-00482
125. Lucas-Herald AK, Kyriakou A, Alimussina M, Guaragna-Filho G, Diver LA, McGowan R, et al. Serum anti-mullerian hormone in the prediction of response to hCG stimulation in children with DSD. J Clin Endocrinol Metab. (2020) 105:1608–16. doi: 10.1210/clinem/dgaa052
126. Abdelghaffar S, AbdelMoneam EN, Hassanein SA, Radwan NA, Mira MF. Categorization of differences of sex development among Egyptian children and the role of antimullerian hormone and inhibin B. Front Endocrinol (Lausanne). (2023) 13:1072399. doi: 10.3389/fendo.2022.1072399
127. Bastida MG, Rey RA, Bergadá I, Bedecarrás P, Andreone L, del Rey G, et al. Establishment of testicular endocrine function impairment during childhood and puberty in boys with Klinefelter syndrome. Clin Endocrinol (Oxf). (2007) 67:863–70. doi: 10.1111/j.1365-2265.2007.02977.x
128. Aksglæde L, Christiansen P, Sorensen K, Boas M, Linneberg A, Main KM, et al. Serum concentrations of Anti-Mullerian Hormone (AMH) in 95 patients with Klinefelter syndrome with or without cryptorchidism. Acta Paediatr. (2011) 100:839–45. doi: 10.1111/j.1651-2227.2011.02148.x
129. Cabrol S, Ross JL, Fennoy I, Bouvattier C, Roger M, Lahlou N. Assessment of Leydig and Sertoli cell functions in infants with nonmosaic Klinefelter syndrome: insulin-like peptide 3 levels are normal and positively correlated with LH levels. J Clin Endocrinol Metab. (2011) 96:E746–753. doi: 10.1210/jc.2010-2103
130. Spaziani M, Granato S, Liberati N, Rossi FM, Tahani N, Pozza C, et al. From mini-puberty to pre-puberty: early impairment of the hypothalamus-pituitary-gonadal axis with normal testicular function in children with non-mosaic Klinefelter syndrome. J Endocrinol Invest. (2021) 44:127–38. doi: 10.1007/s40618-020-01281-x
131. Moniez S, Pienkowski C, Lepage B, Hamdi S, Daudin M, Oliver I, et al. Noonan syndrome males display Sertoli cell-specific primary testicular insufficiency. Eur J Endocrinol. (2018) 179:409–18. doi: 10.1530/EJE-18-0582
132. Hirsch HJ, Eldar-Geva T, Erlichman M, Pollak Y, Gross-Tsur V. Characterization of minipuberty in infants with Prader-Willi syndrome. Horm Res Paediatr. (2014) 82:230–7. doi: 10.1159/000365047
133. Hirsch HJ, Eldar-Geva T, Benarroch F, Rubinstein O, Gross-Tsur V. Primary testicular dysfunction is a major contributor to abnormal pubertal development in males with prader-willi syndrome. J Clin Endocrinol Metab. (2009) 94:2262–8. doi: 10.1210/jc.2008-2760
Keywords: androgens, cryptorchidism, AMH, anti-Müllerian hormone, testis, testicular descent
Citation: Rey RA and Grinspon RP (2024) Anti-Müllerian hormone, testicular descent and cryptorchidism. Front. Endocrinol. 15:1361032. doi: 10.3389/fendo.2024.1361032
Received: 24 December 2023; Accepted: 22 February 2024;
Published: 04 March 2024.
Edited by:
Helena Virtanen, University of Turku, FinlandReviewed by:
Suresh Yenugu, University of Hyderabad, IndiaChrystele Racine, Université Paris Cité, France
Copyright © 2024 Rey and Grinspon. This is an open-access article distributed under the terms of the Creative Commons Attribution License (CC BY). The use, distribution or reproduction in other forums is permitted, provided the original author(s) and the copyright owner(s) are credited and that the original publication in this journal is cited, in accordance with accepted academic practice. No use, distribution or reproduction is permitted which does not comply with these terms.
*Correspondence: Rodolfo A. Rey, cm9kb2xmb3JleUBjZWRpZS5vcmcuYXI=; Romina P. Grinspon, cmdyaW5zcG9uQGNlZGllLm9yZy5hcg==