- 1Research Group Molecular Nutritional Medicine and Department of Human Nutrition, German Institute of Human Nutrition Potsdam-Rehbruecke, Nuthetal, Germany
- 2German Center for Diabetes Research (DZD), München, Germany
- 3University of Lübeck, Lübeck, Germany
- 4Department of Endocrinology and Metabolism, Charité – Universitätsmedizin Berlin, Corporate Member of Freie Universität Berlin, and Humboldt-Universität zu Berlin, Berlin, Germany
Meal timing emerges as a crucial factor influencing metabolic health that can be explained by the tight interaction between the endogenous circadian clock and metabolic homeostasis. Mistimed food intake, such as delayed or nighttime consumption, leads to desynchronization of the internal circadian clock and is associated with an increased risk for obesity and associated metabolic disturbances such as type 2 diabetes and cardiovascular diseases. Conversely, meal timing aligned with cellular rhythms can optimize the performance of tissues and organs. In this review, we provide an overview of the metabolic effects of meal timing and discuss the underlying mechanisms. Additionally, we explore factors influencing meal timing, including internal determinants such as chronotype and genetics, as well as external influences like social factors, cultural aspects, and work schedules. This review could contribute to defining meal-timing-based recommendations for public health initiatives and developing guidelines for effective lifestyle modifications targeting the prevention and treatment of obesity and associated metabolic diseases. Furthermore, it sheds light on crucial factors that must be considered in the design of future food timing intervention trials.
Introduction
The circadian clock is an endogenous timing program that structures physiology and behavior according to the time of day, representing an adaptation to the 24-h light–dark cycle and corresponding environmental changes dictated by the Earth’s rotation (1). The circadian clock plays an essential role in metabolic processes. The organism’s ability to assimilate nutrients, mobilize these nutrients, and discard metabolic waste at specific times of the 24-h day is primed by daily rhythms in the function of numerous genes (2, 3). The desynchronization of the circadian clock associated with the modern 24/7 lifestyle increases the risk for several diseases, such as obesity, type 2 diabetes mellitus, and cardiovascular diseases (2, 4). Food intake is one of the most important zeitgebers (3, 5), and therefore, meal timing, together with food composition and calorie intake, is a crucial factor affecting metabolic health (4, 6). Nevertheless, eating times widely differ in the population, and time windows for food intake can be large, exceeding 14 h a day (7). Environmental and lifestyle factors, such as eating around the clock or shift work, which is accompanied by delayed or night eating, increase the risk of metabolic diseases (8).
Therefore, one can hypothesize that social structures such as planned break times at work and social obligations (e.g., regular family meal timing or religious fasting) can influence eating times from small families up to entire population groups. Additionally, with regard to different chronotypes in the population, meaning that each individual person has a circadian preference for performance and activity (9), fixed social structures and environmental conditions must be given more attention. In addition, precision nutrition, i.e., the individualization of eating times or the adaptation of meal plans to the individual circadian rhythms (chronotype), could be an approach to reduce or even eliminate desynchronization. Therefore, it is necessary to differentiate between hereditary factors and adaptable environmental factors linked to the internal circadian clock influencing meal timing.
The interplay between internal clock and metabolism
The function of the circadian clock in mammals has been previously described in several excellent reviews (10–12). This complex, interlinked hierarchical timing system is responsible for approximately 24-h rhythms in mammals, e.g., in humans, regulating corresponding day vs. night behavior, mainly through the central clock in the brain, but also via peripheral clocks in different tissues and organs (6). In this way, the circadian clock rhythmically coordinates biological processes to occur at the correct time to maximize the fitness of an individual. This enables the body to adapt to changes during the day and night, for example, by regulating eating rhythms or expression levels of certain genes, leading to corresponding circadian rhythms in metabolic function (4). Corresponding oscillations of processes or functions are designated as circadian or diurnal rhythms. The term “circadian rhythms” generally refers to ~24-h oscillations that occur in the absence of external timing cues (i.e., are endogenous). Diurnal (daily) rhythms observed during real-life conditions result from interactions between the internal clock and timing cues, which include light and food intake (2).
One crucial oscillator in the clock network is the so-called master clock or pacemaker, also known as the suprachiasmatic nucleus (SCN), located in the hypothalamus and entrained by light (4, 6) (Figure 1). The mechanism of the central molecular clock is based on transcriptional and post-translational feedback loops through the activation and repression of the gene’s own transcription (1, 3, 13). The master clock orchestrates the timing of physiological processes according to the 24-h light–dark cycles (14). Identical functioning peripheral clocks are present in almost every tissue (15). Metabolically active tissues and organs, including liver, gut, adipose tissue, and pancreas, are regulated by the circadian system and demonstrate oscillating rhythms underlying synchronization (16). A number of processes involved in digestion, absorption, utilization, and metabolization of food undergo daily rhythms (16). A growing body of evidence leads to the assumption that the maintenance of metabolic homeostasis is based on the interaction of the circadian clocks and metabolism, meaning that the central clock and peripheral clocks are well-coordinated (4).
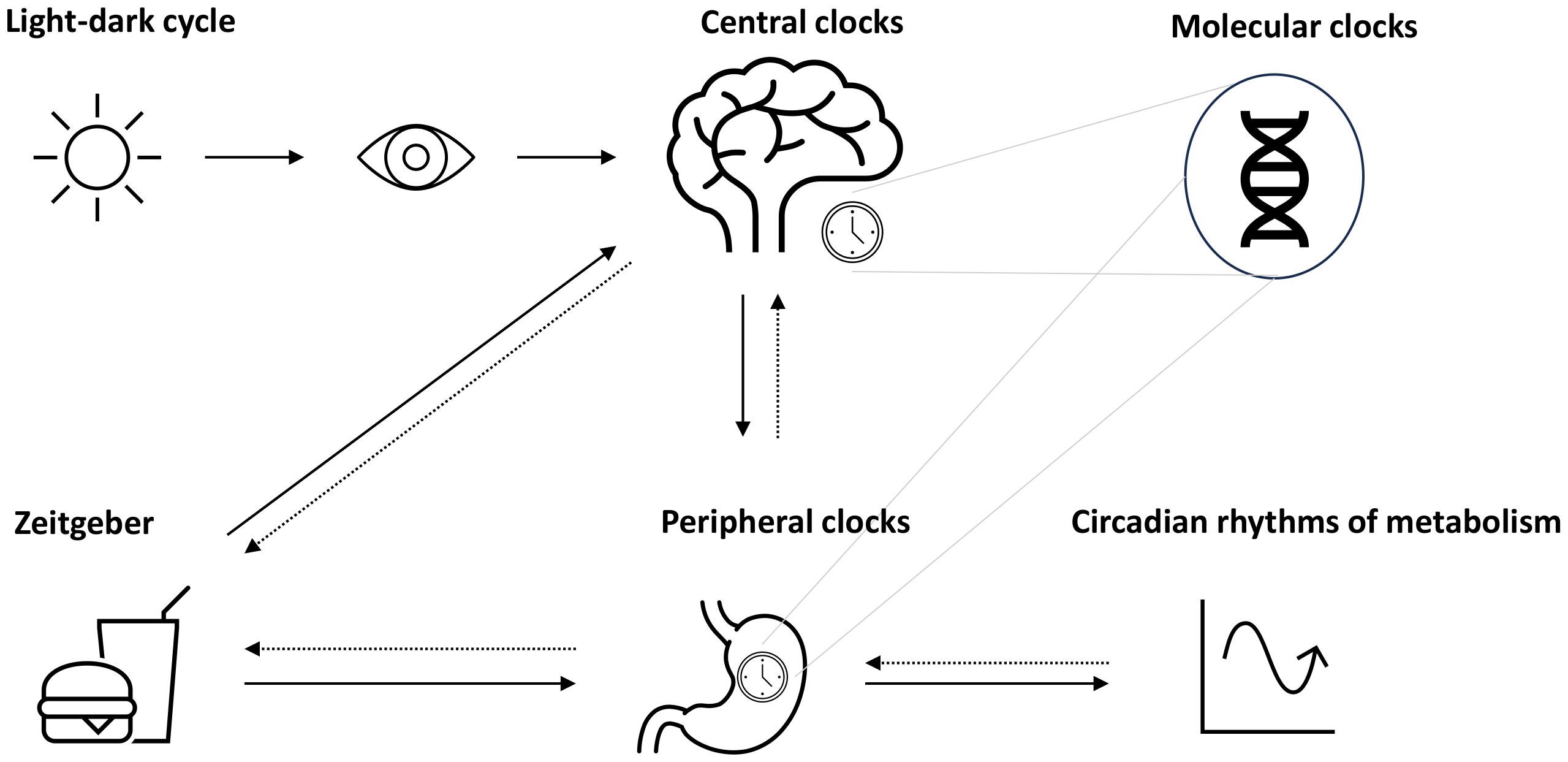
Figure 1 Circadian system and metabolic rhythms. The circadian system is coordinated by the suprachiasmatic nucleus (SCN) as the pacemaker, which is entrained by light (symbolized as sun). Together with further secondary clocks, the SCN influences the expression of gene making up molecular clocks in different tissues (peripheral clocks). Apart from light, further time cues (zeitgeber) such as food intake or physical activity can influence the circadian rhythms, e.g., through the modulation of metabolism, which may act as a feedback to adjust central and peripheral clocks.
Disruption of circadian clock machinery in rodents
Rodent studies have emphasized the crucial role of the internal clock in metabolic regulation on a systemic and organ- or tissue-specific level. Indeed, circadian clock mutant mice or knockout models of individual clock and clock-controlled genes have shown dysfunctions of the metabolic homeostasis (17). For instance, Turek et al. (18) highlighted weakened feeding rhythms, hyperphagia, obesity, hepatic steatosis, adverse changes in fat metabolism, and impaired glycemic control accompanied by hyperglycemia and hypoinsulinemia in Clock Δ19 mutant mice. Zuber et al. (19) demonstrated an adjustment of renal function up to a diabetes insipidus, accompanied by dysregulated sodium excretion rhythms in Clock knockout mice, emphasizing the importance of molecular clock for kidney function. The Per1 transcription factor, encoded by the Per1 gene in kidney, was identified as crucial for maintaining renal homeostasis (20). Additionally, Clock and Bmal1 transcription factors were recognized for their major role in the recovery regulation from insulin-induced, highlighting their importance in glucose homeostasis (21). Bmal1 showed specific relevance in the peripheral liver clock maintaining fasting glucose homeostasis and glucose clearance (22). Bmal1 and Clock were also found to be essential for pancreatic beta-cell clock function, influencing glucose metabolism, insulin signaling, and insulin secretion (23, 24). Clock protein Cry1 was identified as a regulator for gluconeogenesis in the liver (25). Moreover, the factor Per2 in mice was identified to play an important role in mechanisms related to adipogenesis, inflammation, and insulin sensitivity through direct repression of the nuclear receptor PPARγ (26). Cry1 and Cry2 gene deficiency in mice was accompanied by hypertension, leading to the hypothesis that both clock core components are involved in the regulation of blood pressure (27). Rev-erbα was identified as a regulator of lipid metabolism, repressing the transcription of gene coding Apolipoprotein C-III (28). Knockout of the clock gene Rorα resulted in severe atherosclerosis and reduced HDL levels in mice fed with an atherogenic diet (29).
Food intake and metabolic rhythms
To ensure synchronized circadian rhythms, corresponding time cues from the surrounding environment are needed, known as zeitgeber, such as light, physical activity, and food intake. Food intake, in particular, is a unique external clock synchronizer as it specifically affects the peripheral clocks of tissues and organs related to food intake, such as the liver, gut, and adipose tissue (15). Meal timing, therefore, entrains or, in other words, synchronizes peripheral circadian rhythms in metabolic tissues independently of the central clock (30). More precisely, in humans and animals, food intake has been proven to entrain the peripheral clocks of liver, heart, and pancreatic tissue (31–33). Under normal conditions, feeding rhythms align with the external light–dark cycle, contributing to the entrainment of peripheral oscillators with the central clock (34). However, food intake during the biological night can uncouple peripheral clocks from the SCN (31, 32), confirming that meal timing acts as a zeitgeber for circadian clocks.
Regulation of food intake and its feedback on metabolism
Historically, it has been thought that food intake is mainly regulated by physiological feedback mechanisms. This internal signaling system is primarily located in the hypothalamus, brain stem, and limbic system (35), where corresponding oropharyngeal, gastrointestinal, blood chemical, tissue metabolic, and other signals are integrated and translated into neuropeptide release (36). The idea is that this system stimulates and inhibits eating to maintain internal homeostasis. Signals can be categorized into short-term regulation, such as gastrointestinal filling, and long-term regulation, for example, feedback signals from adipose tissue (37). Indeed, the complexity of the system underlying food intake provides several new targets/strategies to control appetite, especially at a time when cardiovascular diseases, diabetes, and metabolic syndrome are showing increasing prevalence (38).
Food intake and circadian rhythms
Body weight control depends on various factors, such as the quantity and quality of food, as well as on meal timing (39). Understanding environmental factors that affect food intake is of great importance, as it seems to play a crucial role in the development of obesity (40). When a time cue, e.g., food intake, occurs at unusual times, this can lead to a conflict between the central and peripheral clocks, resulting in internal desynchronization (41). This occurs through metabolic feedback, which, in the case of food intake, can be the secretion of hormones involved in the regulation of metabolic processes, satiation, and appetite. For example, insulin, glucagon, adiponectin, corticosterone, leptin, chemerin, lipocalin, and visfatin demonstrate diurnal variations typically adapted to food intake throughout the active phase (which is at daytime for humans). Many of these hormones show marked postprandial changes, mirroring the pattern of the food intake (42, 43).
Another feedback mechanism involves microbiota oscillations, which coordinate the activity of diverse metabolic pathways before or after eating events in a 24-h rhythm when meal timing occurs regularly (44). A potential hypothesis for the circadian coordination of these mechanisms is the facilitation of overnight fasts (45). To be precise, hunger and corresponding appetite peaks in the biological evening and morning can be seen as a support for energy balance and, additionally, reduce the risk for awaking during sleep due to hunger (46). Consequently, when these complex interactions are disrupted through mistimed eating, traveling to another time zone, or shift work, so-called chronodisruption, desynchronization between the central and peripheral clocks can occur, leading to impaired metabolic homeostasis and adverse metabolic health effects.
Mistimed food intake and metabolic disorders
In recent years, several animal and human studies intensively investigated the effects of meal timing on metabolism, specifically examining the effects of mistimed food intake. Long-term desynchronization of circadian rhythms is associated with adverse metabolic health effects, including weight gain, increased body fat, obesity, impaired glucose tolerance, insulin resistance, higher blood pressure, and elevated cholesterol levels, as well as elevated risk for cardiovascular diseases, diabetes, and metabolic syndrome (18, 47–54). These health effects, with regards to meal timing, will be discussed in more detail below, starting with mouse studies followed by human studies.
In mouse studies, feeding animals only during the rest (light) phase resulted in greater weight gain compared to feeding them during the active (dark) phase (55). A replication and extrapolation of this study showed that mice gained even more weight under constant light conditions, but not when restricting the food intake to their “biological night” (56). Additionally, time-restricted feeding was found to compensate for the adverse effects of an unhealthy (high-fat) diet (57). Mistimed food intake in further animal studies has led to increased peripheral inflammation (58).
Similar findings are observed in humans, particularly in shift workers. A systematic review and meta-analysis indicated that despite no differences in total energy intake on dayshift vs. nightshift days, typical adverse effects of mistimed food intake, such as increased risk for obesity, were detected (52). This resulted in the hypothesis that other factors such as meal timing could explain the higher obesity and cardiometabolic disease prevalence among nightshift workers (59). Observational and experimental studies in humans confirm an association between meal timing, weight gain, glucose (in)tolerance, and diabetes (60). For example, later eating times are associated with the development of overweight (61). There are different biological explanations for these relationships. Their understanding could potentially open a new dimension in nutrition science, as most weight loss interventions currently focus on total energy intake rather than meal timing (62).
Further human studies have also focused on breakfast timing and meal regularity (63). Investigations in a female Swedish cohort revealed more food events and a shift towards a later meal timing in women with obesity (64). Along with this, another study stated a longer eating time during the day and quicker eating in women with obesity compared to women without obesity (65). A possible explanation for these associations is that night eating, defined as the intake of the majority of calories after 20:00, is linked to a higher body mass index (BMI), independently of sleep behavior (66–68). A study with 110 participants revealed an association of BMI and adiposity with food intake later in the “biological night”, but not the clock hours, emphasizing that meal timing should be considered relative to the inner clock (69). The latter could explain why some studies have not found associations between meal timing and metabolic outcomes, as the definition “night” is not always aligned with the biological night of an individual, which can vary depending on the chronotype (70).
These findings are supported by further clinical studies, suggesting that late main meal timing hinders intended weight loss (62) or even the therapeutic effect of bariatric surgery. Additionally, several randomized, crossover studies show that eating lunch at a later time reduces resting metabolic rate and equivalent energy expenditure (71, 72). Similar results, indicating an increased risk for weight gain, were observed when the main energy component was consumed in the evening instead of midday (73), showing a connection to impaired insulin sensitivity measured in insulin secretion and glucose tolerance (74). This potentially increased risk for developing a type 2 diabetes (T2D) was also reported when the same meal was consumed in the evening rather than the morning, leading to an increased glycemic/insulinemic response (72). Surprisingly, there was even a resemblance to the postprandial response of a (pre)diabetic patient, despite the subject being healthy (75). Furthermore, later dinner times showed a worsening of postprandial glucose profiles after breakfast on the next day (76). Overall, it is suggested that a low-energy first meal and a high-energy last meal are linked to difficulties in losing weight and impaired glucose metabolism (77). In contrast, the intake of the majority of calories in the morning, such as for breakfast, along with a minority of calories in the evening, has shown metabolically beneficial effects in terms of improving glucose tolerance, which is particularly relevant for T2D patients (78, 79).
Recently, Dashti et al. (80) summarized the adverse cardiometabolic effects of mistimed food intake including (1) development of obesity (42), (2) reduced diet-induced thermogenesis in the evening (81), (3) diminished glucose tolerance due to circadian misalignment resulting from lower insulin sensitivity (82, 83), (4) adverse effects of elevated melatonin on glucose metabolism (84), and (5) an increase in body fat (69). Furthermore, mistimed meal intake resulted in a phase shift in the peripheral clocks of the adipose tissue (30), changes in blood pressure (85), and an increase in inflammatory markers (86).
Over the last 40 years in the United States (US) (1971–2010), a shift in meal timing has been observed through the National Health and Nutrition Examination Surveys (NHANES) (87). In fact, a general later intake for all meals, except dinner and snacks after dinner, was detected (87). This development can be seen as negative, due to the adverse metabolic health effects discussed previously and in the scope of the current obesity pandemic. Therefore, understanding the underlying mechanisms is crucial to adjusting health in terms of diet and making recommendations for disease prevention.
To comprehend these effects, homeostatic and circadian mechanisms regulating food intake must be examined in more detail, e.g., how exactly the circadian rhythms influence hunger and energy expenditure (88). At night, energy expenditure is at its lowest (resting energy expenditure) in contrast to the morning and/or afternoons as shown using a forced desynchrony protocol (89). Hunger, appetite, and food preferences were investigated in another forced desynchrony study using special ratings, revealing a circadian rhythm for hunger with a peak in appetite in the biological morning around 08:00 and evening at 20:00 (46). These hunger rhythms appear to be robust, as they did not show any changes even when meal timing was manipulated (30) or when participants were sleep-deprived (45).
Finally, it is also important to consider the chronotype, which can be understood as a behavior resulting from the circadian system, influenced by genetics, the light–dark cycle, and other environmental factors such as social and personal surroundings. Chronotypes can range from morning to evening preferences, which are divided into early, intermediate, and late chronotypes (90). Extreme chronotypes, especially the late chronotype, might conflict with daily life structure, for example, due to work or school schedule. This applies not only to sleep behavior but also to meal timing preferences, which can vary depending on the individual chronotype. Furthermore, a late chronotype can lead to discrepancies in workday compared to non-workday behaviors, considered a so-called nutritional or meal jet lag, which may result in metabolically adverse events (90).
All in all, these findings suggest obesity as a chronobiological disease. However, the development of overweight is multifactorial, and regular food intake during the active phase needs to be considered in addition to known factors such as energy intake and/or dietary composition (60). Although we consider food intake as a “chronodisrupter” in the case of desynchronization, a change of paradigm towards a more positive term, such as “timekeeper”, offers the therapeutic chance of meal timing interventions, when considering that meal timing synchronized with the cellular activities can lead to an optimized performance of tissues and organs (91).
Possible mechanisms of meal timing effects
Despite this clear connection between meal timing and cardiometabolic health, the underlying mechanisms remain largely unknown. An important question is whether the effects are solely due to mistimed food intake or possibly due to other changes, such as energy intake, macronutrient composition of the meal, fasting duration, meal frequency, or changes in sleep behavior, accompanying mistimed meals.
It has been believed that individuals who do not eat early in the day tend to consume more energy in the evening; for example, people who skip breakfast tend to consume more calories and meals over the day (41, 92, 93). Therefore, explaining greater fat storage and the corresponding weight gain might be explained by changes in meal frequency. However, this aspect has not been carefully controlled in many studies.
These effects can also be explained through the disruption of the circadian system as it is highly interconnected with food intake (see also the Regulation of food intake and its feedback on metabolism section). In particular, circadian variations are mirrored in the energy expenditure and metabolic pattern of healthy individuals when comparing morning to evening. For example, food-induced thermogenesis is generally higher in the morning than in the evening and night (81). Additionally, when consuming the same meal in the evening instead of morning, lower resting metabolic rate as well as an increased glycemic and insulinemic responses occur (72). Beyond that, it has been shown that signaling pathways involving cAMP-response element binding protein (CREB), mechanistic target of rapamycin (mTOR), and adenosine monophosphate-activated protein kinase (AMPK) also play a role in energy expenditure. When restricting food intake to an 8-h period, CREB, mTOR, and AMPK pathway function and their circadian rhythms increase, leading to a higher expression pattern of certain liver genes and improved nutrient utilization (57).
Further mechanisms are the secretion of (an)orexigenic hormones, e.g., leptin, which is released from the adipose tissue proportional to lipid storage (35). Leptin decreases in terms of concentration as a consequence of mistimed eating. Mistimed eating leads to a decrease in leptin concentration, associated with increased food intake and lower energy expenditure, potentially explaining subsequent weight gain. Not only hormonal, but further digestive components required before food intake are controlled by the circadian clocks, which are diminished at night. For example, saliva flow in the mouth or in the gut, stomach acid, and intestinal motility flow decrease at night (94–96). Many of the digestive components, including peptide hormones of the gastrointestinal tract, not only underlie circadian rhythmicity, but also can affect the peripheral clocks and therefore metabolic rhythms, again providing feedback circuits (97).
Circadian clocks in the muscle and fat mass also play an important role in metabolism. For example, muscle protein synthesis rates are highest during the night, making protein intake, recommended for exercise recovery, most effective before sleeping (98). Regarding adipose tissue, mistimed food intake can increase the expression of the inflammatory markers tumor necrosis factor alpha (TNFα) and macrophage-1 antigen (MAC1) in white adipose tissue, similar to the upregulation seen with a high-fat diet (58). Mice lacking cryptochrome (Cry), a photoreceptor that regulates the entrainment by light of the circadian clock, are more susceptible to high-fat diet-induced obesity (99). Additionally, the expression of lipogenic genes is upregulated in the white adipose tissue of these mice, possibly explaining increased insulin secretion and lipid storage (48). Moreover, adipose tissue specific knockout (BMAL1) showed weight gain due to changes in energy regulation and a shift in food intake, emphasizing the interaction of meal timing and adipocyte clocks (100).
Finally, changes due to mistimed food intake have been observed in the diurnal salivary and gastrointestinal microbiota rhythms in terms of diversity and abundance, all underlying the circadian control (101). For example, eating a late main meal inverts the daily rhythm of salivary microbiota diversity, which may have an effect on the host metabolism (44). Furthermore, microbiota regulates body composition via the nuclear-factor, interleukin 3 regulated (NFIL3), which underlies the circadian control and affects the regulation of lipid absorption and export (102). Interestingly, microbial metabolites can affect the central and peripheral clocks and are linked to various processes in anabolism and catabolism of different macronutrients (103). Desynchronization of circadian rhythms due to mistimed food intake has been associated with gut dysbiosis and consequent metabolic and systemic disorders. In obese and elderly women, similar abnormalities in rhythmicity were observed (104).
Time-of-day-based nutritional strategy: time-restricted eating
An analysis of eating windows from an Indian cohort of 93 healthy individuals, who documented their food intake using phone cameras for 21 days, revealed that half of the cohort had daily eating windows longer than 15 h (105). Indeed, a decile of the cohort even showed eating windows around 20 h due to flexible working times or a partner engaged in shift work (105). The study demonstrated a caloric consumption of more than 30% of total calories in the evening and night, with less than 30% of total calories consumed in the morning (105). Previously, the identification of long eating periods in the population of American adults has shown benefits for people with overweight through a reduction in the length of the eating window, which manifested itself in weight loss, sleep improvement, and increased energy (7).
This concept of shortening meal times has been established for some time and is widely practiced in today’s society for weight management and modulation of eating patterns. In this regard, the approach of time-restricted eating (TRE) has been proven to shorten the eating window. TRE is characterized by intervals of 8 to 10 h during which food is consumed, with variations regarding time of day (106). In this respect, early TRE (eTRE), mid-day TRE (mTRE), and late TRE (lTRE) have been shown to be variations of TRE (106). The term “TRE” refers to a specific eating pattern or eating habit that relates to the timing of food intake (meal timing) and can be applied regardless of calorie restriction (106). Recent human trials on TRE have highlighted numerous beneficial metabolic effects of TRE, e.g., improved mean daily glucose levels (107, 108), fasting glucose levels (108–110), insulin resistance (108, 110, 111) or insulin sensitivity (112, 113), triglyceride levels (107, 109, 114, 115), total cholesterol (115, 116), and LDL cholesterol levels (116). However, results in TRE trials are highly variable, probably because of heterogeneous study designs, eating windows, study cohorts, and differences in calorie intake (117, 118). With regard to possible metabolic TRE effects that goes beyond a simple calorie restriction diets, recent studies investigated whether the combination of calorie restriction and TRE is more effective than a simple calorie restriction. Thus, Jamshed et al. (119) compared a weight loss treatment with energy restriction (ER), but eating longer than 12 h with a time-restricted weight loss treatment (eTRE+ER). The study revealed more positive effects of eTRE+ER regarding weight loss and blood pressure. However, another study by Liu et al. (120) comparing calorie restriction with or without TRE (CR or CR+TRE) in patients with obesity showed no significant differences in weight changes between the groups after 12 months.
Actually, there is little evidence for a recommendation of the TRE eating window, e.g., eTRE, mTRE, or lTRE. However, one randomized crossover trial in men who were at risk for T2D showed improved glycemic responses through TRE independently from clock time, but only demonstrated reductions of fasting glucose in continuous glucose monitoring when TRE was performed from 8 a.m. to 5 p.m (107).. In a recent published systematic review and meta-analysis of 15 TRE studies, the authors concluded that metabolic effects are dependent on the duration of the TRE eating window (121), with anthropometric and cardiometabolic changes being stronger with shorter eating windows (121).
Factors influencing meal timing
The modern lifestyle allows traveling across different time zones, fast food chains enable 24-h food access, and jobs with nighttime working hours open the possibility of working around the clock. Although this does not seem harmful at first and rather indicates possibilities than limitations, a closer look towards the influence of these factors on the circadian system is necessary. Indeed, jetlag through traveling, shift-working, and the constant availability of food, especially with high energy density, and artificial light have been previously described as environmental and lifestyle-associated factors that can lead to chronodisruption (122). A number of social and biological factors influencing meal timing (80) can be divided into different categories: (1) physiological factors (e.g., circadian clock, genetic factors, age, sex, body composition, and physical and mental disorders), (2) behavioral and personal preferences (e.g., daily calorie distribution, physical activity, artificial light exposure, chronotype, and sleep behavior), and (3) cultural and environmental factors (e.g., religion, tradition, economic status, social position, and work schedule) (Figure 2). Notably, many of these factors such as age, sex, chronotype, meal composition, physical activity, and others also influence metabolic outcomes, but it is difficult to separate meal-timing-mediated and meal-timing-independent effects on metabolism in free-living individuals. Some of the factors influencing meal timing are discussed in more detail below.
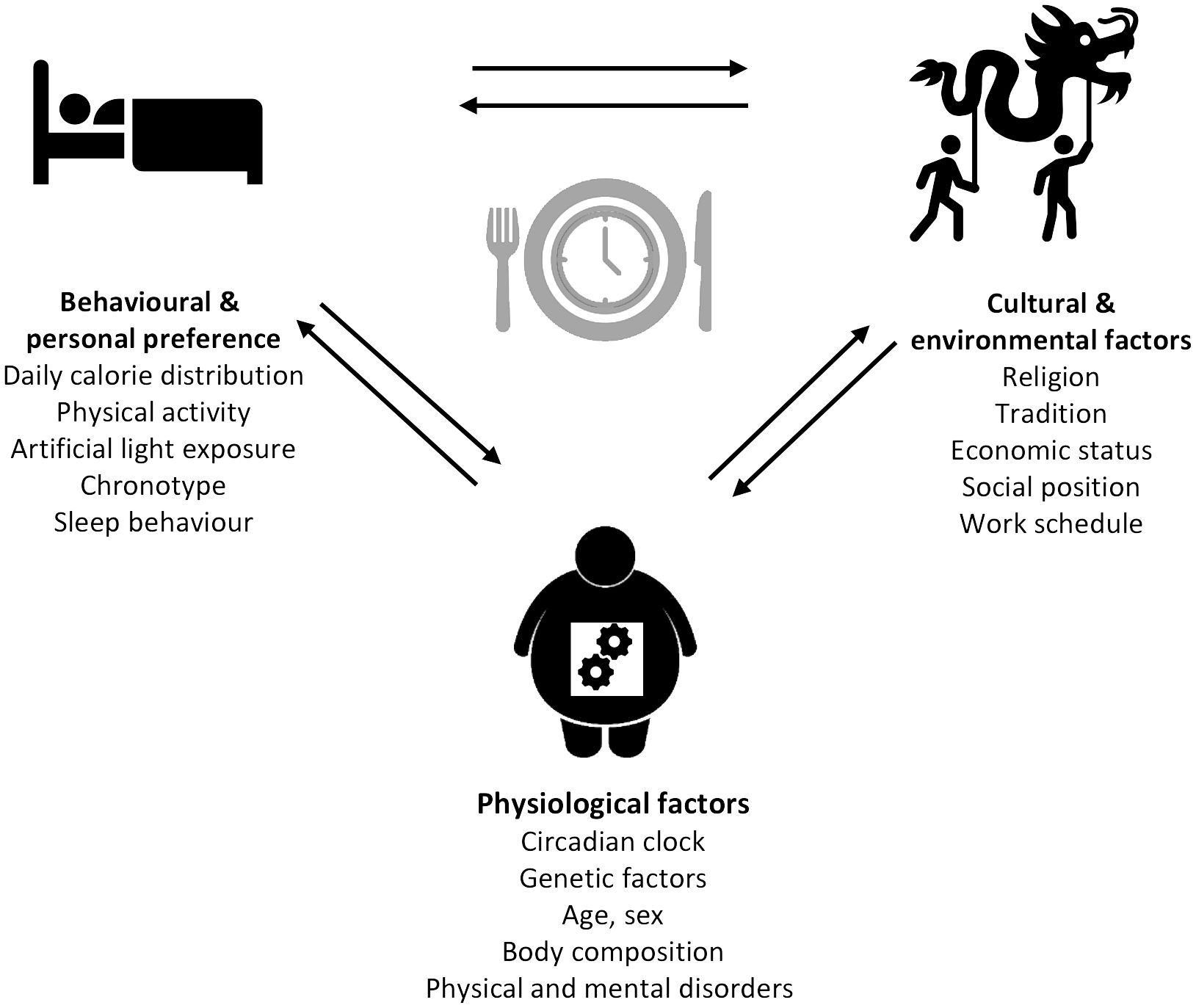
Figure 2 Determinants of meal timing. The factors influencing meal timing range from physiological to behavioral and personal preferences, as well as cultural and environmental factors each comprising different parameters.
Sex and age
Next to the circadian system and BMI, sex difference plays a crucial role in metabolic regulation (80). Thus, a meta-analysis in Iranian students including 24 studies revealed a higher prevalence of breakfast-skipping among girls than among boys (123). Sex differences in meal patterns were further described in a study performed in rural areas of Nepal, where the skipping of lunch and daytime-snacking were more frequently in men, than in women (124). Contrary to this, a study performed in 62,298 American adults reported that snacking behavior was more prevalent in women than in men (125). However, the authors also reported a trend in both sexes that a higher intake of snacks unrelated to main meals reduces the number of main meals (125). Moreover, differences in chronotypes (see the Chronotype section) dependent on age and sex were described previously in a large American cohort, inducing a higher prevalence of women having earlier chronotypes under the age of 40 years and later chronotypes above 40 years compared to men, possibly due to hormonal changes (126). In addition, there are more investigations pointing out an association of eating pattern in terms of meal timing and age. In fact, skipping breakfast was observed in the 2005–2010 NHANES survey, in which the 20–39 age group was the largest group to report no breakfast in both surveys (92). To conclude, adolescents were found to have the latest chronotypes, even if very early to very late chronotypes can be found in any age group (126).
Physical and mental disorders
Both physical and mental disorders can affect meal timing, and along with that, some diseases require adaptations of meal timing. One example is the night-eating syndrome where patients tend to suffer from morning anorexia as a result of excessive food consumption (hyperphagia) in the evening, leading to an increase of energy intake mainly in the second half of the day (127). Furthermore, a poorly controlled T2D can lead to a drop in blood glucose levels and may require meals outside of regular meal timing and therefore affecting the eating pattern. Another disease influencing meal timing is liver cirrhosis, where problems in the glycogen storage occur. In this case, patients should change their diet pattern towards a more frequent and therefore smaller food intake to prevent long fasting periods (128). Furthermore, psychiatric or mental disorders may influence dietary intake. Indeed, an association between depression symptoms and nocturnal eating has been described in young adults, indicating an effect on meal timing or vice versa (129). Moreover, psychiatric disorders, e.g., binge eating disorder or anorexia nervosa, and addictions, e.g., drug and alcohol addictions, are possible factors influencing meal time patterns.
Genetic factors
As meal timing can be seen as a potential new risk factor for metabolic diseases, the aim to modify it in order to prevent chronic diseases through weight reduction seems clear (130), but for this, we must gain a better understanding of this complex trait, starting with revealing the genetic and environmental contributions to the individual variability (131, 132). A necessary aim is to understand underlying biological mechanisms and to develop successful public health interventions or effective therapeutic approaches, most probably via personalization (133, 134).
There are limited data regarding the heritability contributing to the food intake and associated parameters such as body weight, which influence overall energy intake (135). However, not only these overarching variables seem to be influenced by genetic factors. There are also emerging data on the heredity of more subtle variables known as the microstructure of food intake, e.g., the frequency, composition, and meal timing, independent of the overall intake (131, 136). Additionally, further twin studies have shown that genetics influence different diet-related phenotypes such as energy and macronutrient intake, dietary patterns, and the intake of specific food items (137). There are even specific physiological variables, such as stomach filling before and after eating (138), or certain metabolic responses (139) that have been proven heritable. Finally, psychological variables such as the subjective rating of hunger and palatability of food have also been found to be influenced by genetic factors (140, 141).
To our knowledge, there have been only two studies that have investigated the heritability of meal timing. One involved 265 identical and fraternal adult twin pairs who recorded 7-day food diaries (142). The other study included 52 Spanish twin pairs who also recorded 7-day food intake and additional sleep diaries (143). Both studies demonstrated highest heritability for breakfast: 24% by De Castro (142) and 56% by Lopez-Minguez et al. (143). This was contrasted by lunch ranging from 18% by De Castro (142) to 38% by Lopez-Minguez et al. (143), whereas the latter team did not detect any heritability for dinner, despite 22% heritability for dinner timing detected by De Castro (142). Furthermore, a high heritability of 64% was shown for the caloric midpoint by Lopez-Minguez et al. (143) with a similar heritability for other phenotypically related behavioral traits such as wake (55%) and bedtimes (38%). These factors even correlated with 85% of co-variance between midpoint and chronotype as well as 90% to wake and 75% to bedtime, implying a common genetic variation. These findings suggest that the heritability of the later meals matches the heritability of evening behavior and is lower than the corresponding heritability for early meals and behavior. This also implies that health interventions should be geared more towards the easier, more modifiable, later meal intake (143).
Genetic variants within core clock genes, which control circadian rhythms of transcription and translation in different cells in the body, are a possible explanation for the heritability of meal timing. In particular, they control the temporality of hunger and appetite, independent of other behaviors (46). Polymorphisms within core clock genes such as PER, CRY, BMAL1, and CLOCK may lead to individual differences in their expression (144). For example, single-gene mutations in the clock gene CRY1 have been shown to confer extreme early or late chronotypes (145).
Furthermore, taking a closer look at the CLOCK gene rs4580704 variant, obese participants carrying the minor G allele had their lunch significantly later than participants carrying major alleles (62). Furthermore, the obesity-related perilipin-1 (PLIN1) gene seems to influence the interaction between meal timing and weight loss: people with rs1052700 amino acid (AA) show less weight loss when eating late compared to TT carriers, where eating late does not influence weight loss at all (146). Genetic influences have been suggested for the night eating syndrome (NES) and sleep-related eating disorders (SRED), which are defined as eating disorders with a preference towards late night eating (147). In addition, the microbiome and its composition, known as the microbiome phenotype, have been shown to account for 97% heritability and could therefore constitute a further important component for the heritability of meal timing (148). Interestingly, the risk allele within melatonin receptor MTNR1B provides a link between late dinner eating and impaired glucose tolerance, whereas in non-risk carriers, this relation was less pronounced (149).
Contrary to these findings, the genome-wide association study (TwinUK) with 2006 participants did not reveal any genetic variant associated with breakfast-skipping possibly because of the modest sample size or incomplete genetic coverage (150). Therefore, it is tempting to elucidate the genes associated with mistimed food intake as these may help to understand the biological mechanisms underlying the regulation of meal timing. Revealing these genes could enable further testing of the relation between meal timing and other related traits, especially towards chronic diseases, in order to identify individuals who are at higher risk of mistimed eating or, on the other side, those who are resistant to timing interventions. Ideally, this could lead to the development of individual diet recommendations in line with genetic preferences.
Meal composition
Xiao et al. (151) revealed clear associations between carbohydrate and protein intake with obesity, which indicates variations in nutrient response and nutrient requirement around the circadian cycle and therefore reflects health status. Beyond that, the nutrient composition could be a possible factor influencing meal timing. Thus, a study in students who were offered a walnut or an isocaloric gummy candy snack 90 min before eating dinner in the university cafeteria found that they had less food desire compared to the no-snack control group (152). Indeed, the walnut snack with a low glycemic index, low protein, and low polyunsaturated fats showed more positive effects than the gummy candy snack. Moreover, a recent study by Braden et al. (153) showed a lower appetite and higher satiety through micellar casein and pea protein isolate consumption in healthy subjects with a corresponding increase of peptide YY levels when compared with soy protein, but did not show effects on subsequent food intake. With regard to fiber, there is a steady assumption that fiber intake increases satiety. However, a systematic review of 44 publications examined that approximately 61% of the acute fiber treatments did not lead to an enhanced satiety, and beyond that, a relation between fiber type and dose satiety and food intake was not found (154). Since it is not yet clear how nutrients affect meal timing, further future studies on the effects of nutrient timing are needed.
Chronotype
The chronotype can be described as a complex phenotype representing the individual preference of a person regarding his or her active and sleep time (155). Thus, chronotyping requires the allocation to morning, midday, and evening type (155). However, the chronotype seems to be dependent on environmental factors, which again is affected by mechanisms of innate homeostasis of sleep and circadian rhythms, as shown by a genome-wide association analysis, identifying genomic loci associated to chronotype (156). Owing to a normal distribution of chronotypes, the assumption that common genetic variants in population affect the phenotype arises (157). Indeed, it has been stated that being a night owl or a morning lark is dependent on the individual genetic makeup (157). Regarding meal timing, it has been shown that the choice of food timing underlies the influence of chronotype (158). Thus, late sleepers, i.e. late chronotypes, consume the more calories later in the day than normal sleepers (67). Furthermore, a recent study that was conducted among Italians during COVID-19 lockdown revealed that evening chronotypes had a higher vulnerability regarding late meal timing, breakfast-skipping, and unhealthy habits in relation to their chrononutrition profiles (158). Along with that, the Sleep Extension Study with 119 participants found that later chronotypes eat breakfast approximately 1.3 h later than early chronotypes and consumed twice as many calories after 20:00 (159). In addition, it has been reported that breakfast-skipping is associated with a poorer glycemic control in patients with T2D and is more present in late chronotypes (160). Furthermore, it has been shown that late chronotypes also have a higher frequency of meals with the largest intake at 19:00 to 21:00 compared to early chronotypes, who have their calories more equally distributed over the day (161).
Individual lifestyle factors and daily routines
The preferences of people regarding their lifestyle vary widely. Depending on preferences, individuals distribute their daily calorie intake in different ways. Beyond that, personal preferences for a specific hobby can affect eating habits and especially eating times. Thus, the optimal performance and recovery in sports may require an implementation of a special meal timing and composition based on recommendations (98, 162). Along with that, the individuals’ desire for body weight optimization can lead to personal preferences regarding the choice of a specific diet. Indeed, there are several diets, interacting with the natural meal timing as intermittent fasting or TRE, which lay the ground for informed meal timing interventions (80). Moreover, the personal preference for specific foods or beverages can affect meal timing. Thus, it has been shown that energy intake is reduced for obese participants with moderate coffee intake (163), emphasizing caffeine’s effect on appetite and on meal timing. However, another study showed that caffeine intake leads to less energy consumption at breakfast (10.0%), but also demonstrated a shift towards later intake in the day (164). Furthermore, medications and supplements for certain diseases can influence meal timing as they must be taken before a meal, affecting the medication itself on the one hand, but also appetite, which may result in further changes in meal timing (165). Next to the lifestyle factor of caffeine intake, alcohol is a complex dietary component with the potential to affect food intake (166). Thus, the possible short-term appetite-stimulating effects of alcohol can induce a change in meal timing (167). Indeed, the authors of a study involving 282 students concluded that the likeliness towards night eating is higher for those consuming alcohol more frequently (168).
Artificial light exposure and sleep–wake pattern
In pre-industrial societies, the sleeping routine of people was in harmony with natural light (169). However, in the modern era, light conditions changed with the development of artificial lighting, enabling activities around the clock, e.g., socializing via light-emitting devices, or the possibility of commerce, production, and access to 24-h services, and therefore also leading to shifts in meal timing (170). A possible consequence of this is that light at night and the corresponding longer active compared to rest phase intervals have led to an increase in food intake and therefore excessive weight gain, as shown in rodent studies (56). A significant interaction between food and meal timing was previously described due to an influence of meal timing on sleep timing, and even the sleep timing influence was stronger than the meal timing influence regarding parameters of food intake (171). Apart from wake and sleep timing, long wake periods during the night may lead to a greater possibility for food intake and later eating time (172). Thus, sleep duration influences meal timing and the pattern of food intake (173–175). Social jetlag is a result of sleep depth due to a discrepancy between social and biological times, or rather work and free days (176). Indeed, sleep deprivation of 4 h per night over 5 days leads to an increase in energy consumption of around 533 ± 296 kcal between 22:00 and 03:59 and a reduction of energy intake during 14:59–20:00 compared to baseline (177). Similar findings were reported in another study with sleep restriction, with a 0.8-kg weight gain due to smaller breakfast and a corresponding higher energy intake of 42% after dinner during the nighttime (178). Along with that, another study showed a decrease in the feeling of satiety and fullness in participants who underwent a four-night intervention with 5 h of sleeping (179). This leads to the assumption that sleep deprivation affects meal timing. In contrast, longer sleep episodes correlate with an earlier intake of dinner/the last meal (83) as well as a decreased consumption of free sugars (173).
Geography, religion, and social status
Geography, ethnicity, and culture seem to affect meal timing. Thus, a survey of 36,020 people in 10 countries across central/northern Europe and the Mediterranean revealed a trend for fewer eating events in Mediterranean countries, with a higher energy intake at lunch (40.0% of daily energy) compared to 20.0% in the central/northern areas (180). In addition, snacking, which has become a prevalent eating style, has been identified as a factor influencing cardiometabolic health (130). In this respect, Mediterranean countries indicate a more mindful handling towards lower snacking behavior due to about approximately 15% snacking compared to 25% to 30% in central/northern European countries (180).
Tradition and religion have been shown to influence meal timing throughout history as well as recently; for example, in the Islamic month of Ramadan, meal timing is restricted to the hours between sunrise and sunset for 1 month (181). Interestingly, overnight fasting has also been shown to vary across Europe, with the shortest period being 09:12 in the Netherlands and 10:54 in the Czech Republic (182). Indeed, the change in meal timing towards eating before and after sunset is associated with changes in circadian rhythms and therefore affects cortisol, melatonin, leptin, or ghrelin levels (183).
Moreover, for centuries, social class has influenced meal timing; for example the former middle class, such as tradesmen and merchants, could eat their meals later because of longer work hours. It has also been shown that people with lower income in the US are more likely to skip breakfast (92) and have fewer eating occasions in general (184).
Work schedules and social obligations
Work schedules, school times, and opening hours, e.g., from restaurants or supermarkets, are environmental factors that can lead to meal timing adjustment, change in dietary composition, and the amount of eating events. Indeed, it has been shown that weekday and weekend variabilities can influence the number of meals and snacks, which are decreased on weekends in German (68) and US populations (185). The NHANES study (2003–2012), based on data of 11,646 U.S. adults, showed that people consumed more calories and had a poorer diet quality during the weekend, especially on Saturdays, resulting in higher energy, fat, and protein intake; and confirmed previous data from NHANES (1999–2004) that already revealed associations between temporal dietary patters and diet quality (186, 187). However, the differences between weekends and weekdays also occur between (un)employed participants, implying that not only workspace but also cultural and social factors seem to influence food intake (80). Beyond that, the timing factor was identified to be linked to diet quality (187). Furthermore, a cross-sectional analysis of 428 children, assessing differences in eating habits between weekdays and weekends, revealed a more adequate eating habit regarding vegetables, lunch, and snacks on weekdays, therefore indicating the influence of school canteens in promoting health (188). This is another example of the influence of environmental factors on eating behavior. According to this, social meal timing, e.g., the common meal of family members, has recently been discussed as an important factor for metabolic health (189). Moreover, weekday–weekend variability was demonstrated by Gill and Panda (7) who observed a delay of 1 h of breakfast on weekends compared to weekdays, contrary to the mean last caloric intake, most probably dinner, which was more commonly advanced. Moreover, a trend towards later breakfast as well as earlier dinner and less frequent meals with a longer eating window on weekends compared to weekdays has been shown by other smaller studies from the US (159, 161).
In modern society, not everyone has a 9 a.m.-to-5 p.m. job with regular free weekends. Indeed, differences in meal timing can be caused by shift work, 24-h services, or irregular part-time work. Children are already subjected to external influences, e.g., the holiday season. Regarding this, Mohd Azmi et al. (190) discuss several abnormalities of eating behavior in shift workers, e.g., the NES (191), increased food cravings (192), and the difficulty to follow typical eating patterns within rotational shifts (193).
Conclusion and outlook
In this review, we provided a comprehensive overview of the crucial role of meal timing for metabolic health and the development of obesity and associated diseases. Factors influencing meal timing are very complex and include both internal genetic and external environmental and behavioral factors. They often influence each other directly or indirectly, thus presenting a challenge for the application of meal timing targeting strategies. Nevertheless, environmental factors, e.g., lifestyle, working schedules, and social obligations, are modifiable and can change during a life span. Thus, a precision nutrition approach taking as many internal and external meal time factors as possible into account could be feasible and effective. However, the genetic component needs further investigation in order to understand the metabolic consequences of mistimed food intake.
Author contributions
JV: Writing – original draft, Visualization. BP: Writing – review & editing, Writing – original draft. OP-R: Writing – review & editing, Writing – original draft, Supervision, Resources, Project administration, Funding acquisition, Conceptualization.
Funding
The author(s) declare financial support was received for the research, authorship, and/or publication of this article. The study is funded by the European Association for the Study of Diabetes (Morgagni Prize 2020, OP-R) and by the Deutsche Forschungsgemeinschaft (DFG, German Research Foundation) – 491394008. Funders were not involved in preparation of study design and implementation of the study; data collection, management, data analyzation, and interpretation; preparation, review, or approval of the manuscript; and decision to submit the manuscript for publication.
Conflict of interest
The authors declare that the research was conducted in the absence of any commercial or financial relationships that could be construed as a potential conflict of interest.
The author(s) declared that they were an editorial board member of Frontiers, at the time of submission. This had no impact on the peer review process and the final decision.
Publisher’s note
All claims expressed in this article are solely those of the authors and do not necessarily represent those of their affiliated organizations, or those of the publisher, the editors and the reviewers. Any product that may be evaluated in this article, or claim that may be made by its manufacturer, is not guaranteed or endorsed by the publisher.
References
1. Finger AM, Kramer A. Mammalian circadian systems: Organization and modern life challenges. Acta Physiol (Oxf). (2021) 231:e13548. doi: 10.1111/apha.13548
2. Panda S. Circadian physiology of metabolism. Science. (2016) 354:1008–15. doi: 10.1126/science.aah4967
3. Asher G, Sassone-Corsi P. Time for food: the intimate interplay between nutrition, metabolism, and the circadian clock. Cell. (2015) 161:84–92. doi: 10.1016/j.cell.2015.03.015
4. Chamorro R, Jouffe C, Oster H, Uhlenhaut NH, Meyhöfer SM. When should I eat: A circadian view on food intake and metabolic regulation. Acta Physiol (Oxf). (2023) 237:e13936. doi: 10.1111/apha.13936
5. Lewis P, Oster H, Korf HW, Foster RG, Erren TC. Food as a circadian time cue - evidence from human studies. Nat Rev Endocrinol. (2020) 16:213–23. doi: 10.1038/s41574-020-0318-z
6. Kessler K, Pivovarova-Ramich O. Meal timing, aging, and metabolic health. Int J Mol Sci. (2019) 20:1911. doi: 10.3390/ijms20081911
7. Gill S, Panda S. A smartphone app reveals erratic diurnal eating patterns in humans that can be modulated for health benefits. Cell Metab. (2015) 22:789–98. doi: 10.1016/j.cmet.2015.09.005
8. Rogers M, Coates AM, Banks S. Meal timing, sleep, and cardiometabolic outcomes. Curr Opin Endocrine Metab Res. (2021) 18:128–32. doi: 10.1016/j.coemr.2021.03.006
9. Zou H, Zhou H, Yan R, Yao Z, Lu Q. Chronotype, circadian rhythm, and psychiatric disorders: Recent evidence and potential mechanisms. Front Neurosci. (2022) 16:811771. doi: 10.3389/fnins.2022.811771
10. Hastings MH, Maywood ES, Brancaccio M. The mammalian circadian timing system and the suprachiasmatic nucleus as its pacemaker. Biol (Basel). (2019) 8:13. doi: 10.3390/biology8010013
11. Mohawk JA, Green CB, Takahashi JS. Central and peripheral circadian clocks in mammals. Annu Rev Neurosci. (2012) 35:445–62. doi: 10.1146/annurev-neuro-060909-153128
12. Dibner C, Schibler U, Albrecht U. The mammalian circadian timing system: organization and coordination of central and peripheral clocks. Annu Rev Physiol. (2010) 72:517–49. doi: 10.1146/annurev-physiol-021909-135821
13. Hastings MH, Maywood ES, Brancaccio M. Generation of circadian rhythms in the suprachiasmatic nucleus. Nat Rev Neurosci. (2018) 19:453–69. doi: 10.1038/s41583-018-0026-z
14. Lekkas D, Paschos GK. The circadian clock control of adipose tissue physiology and metabolism. Auton Neurosci. (2019) 219:66–70. doi: 10.1016/j.autneu.2019.05.001
15. Oosterman JE, Kalsbeek A, la Fleur SE, Belsham DD. Impact of nutrients on circadian rhythmicity. Am J Physiol Regul Integr Comp Physiol. (2015) 308:R337–50. doi: 10.1152/ajpregu.00322.2014
16. Lopez-Minguez J, Gomez-Abellan P, Garaulet M. Timing of breakfast, lunch, and dinner. Effects on Obesity and Metabolic Risk. Nutrients. (2019) 11:2624. doi: 10.3390/nu11112624
17. Sahar S, Sassone-Corsi P. Regulation of metabolism: the circadian clock dictates the time. Trends Endocrinol metabolism: TEM. (2012) 23:1–8. doi: 10.1016/j.tem.2011.10.005
18. Turek FW, Joshu C, Kohsaka A, Lin E, Ivanova G, McDearmon E, et al. Obesity and metabolic syndrome in circadian Clock mutant mice. Science. (2005) 308:1043–5. doi: 10.1126/science.1108750
19. Zuber AM, Centeno G, Pradervand S, Nikolaeva S, Maquelin L, Cardinaux L, et al. Molecular clock is involved in predictive circadian adjustment of renal function. Proc Natl Acad Sci U.S.A. (2009) 106:16523–8. doi: 10.1073/pnas.0904890106
20. Douma LG, Costello HM, Crislip GR, Cheng KY, Lynch IJ, Juffre A, et al. Kidney-specific KO of the circadian clock protein PER1 alters renal Na(+) handling, aldosterone levels, and kidney/adrenal gene expression. Am J Physiol Renal Physiol. (2022) 322:F449–f459. doi: 10.1152/ajprenal.00385.2021
21. Rudic RD, McNamara P, Curtis AM, Boston RC, Panda S, Hogenesch JB, et al. BMAL1 and CLOCK, two essential components of the circadian clock, are involved in glucose homeostasis. PloS Biol. (2004) 2:e377. doi: 10.1371/journal.pbio.0020377
22. Lamia KA, Storch KF, Weitz CJ. Physiological significance of a peripheral tissue circadian clock. Proc Natl Acad Sci U.S.A. (2008) 105:15172–7. doi: 10.1073/pnas.0806717105
23. Marcheva B, Ramsey KM, Buhr ED, Kobayashi Y, Su H, Ko CH, et al. Disruption of the clock components CLOCK and BMAL1 leads to hypoinsulinaemia and diabetes. Nature. (2010) 466:627–31. doi: 10.1038/nature09253
24. Sadacca LA, Lamia KA, deLemos AS, Blum B, Weitz CJ. An intrinsic circadian clock of the pancreas is required for normal insulin release and glucose homeostasis in mice. Diabetologia. (2011) 54:120–4. doi: 10.1007/s00125-010-1920-8
25. Zhang EE, Liu Y, Dentin R, Pongsawakul PY, Liu AC, Hirota T, et al. Cryptochrome mediates circadian regulation of cAMP signaling and hepatic gluconeogenesis. Nat Med. (2010) 16:1152–6. doi: 10.1038/nm.2214
26. Grimaldi B, Bellet MM, Katada S, Astarita G, Hirayama J, Amin RH, et al. PER2 controls lipid metabolism by direct regulation of PPARγ. Cell Metab. (2010) 12:509–20. doi: 10.1016/j.cmet.2010.10.005
27. Doi M, Takahashi Y, Komatsu R, Yamazaki F, Yamada H, Haraguchi S, et al. Salt-sensitive hypertension in circadian clock-deficient Cry-null mice involves dysregulated adrenal Hsd3b6. Nat Med. (2010) 16:67–74. doi: 10.1038/nm.2061
28. Raspé E, Duez H, Mansén A, Fontaine C, Fiévet C, Fruchart JC, et al. Identification of Rev-erbalpha as a physiological repressor of apoC-III gene transcription. J Lipid Res. (2002) 43:2172–9. doi: 10.1194/jlr.m200386-jlr200
29. Mamontova A, Séguret-Macé S, Esposito B, Chaniale C, Bouly M, Delhaye-Bouchaud N, et al. Severe atherosclerosis and hypoalphalipoproteinemia in the staggerer mouse, a mutant of the nuclear receptor RORalpha. Circulation. (1998) 98:2738–43. doi: 10.1161/01.CIR.98.24.2738
30. Wehrens SMT, Christou S, Isherwood C, Middleton B, Gibbs MA, Archer SN, et al. Meal timing regulates the human circadian system. Curr Biol. (2017) 27:1768–1775.e3. doi: 10.1016/j.cub.2017.04.059
31. Stokkan KA, Yamazaki S, Tei H, Sakaki Y, Menaker M. Entrainment of the circadian clock in the liver by feeding. Science. (2001) 291:490–3. doi: 10.1126/science.291.5503.490
32. Damiola F, Le Minh N, Preitner N, Kornmann B, Fleury-Olela F, Schibler U. Restricted feeding uncouples circadian oscillators in peripheral tissues from the central pacemaker in the suprachiasmatic nucleus. Genes Dev. (2000) 14:2950–61. doi: 10.1101/gad.183500
33. Gamble KL, Young ME. Metabolism as an integral cog in the mammalian circadian clockwork. Crit Rev Biochem Mol Biol. (2013) 48:317–31. doi: 10.3109/10409238.2013.786672
34. Challet E. The circadian regulation of food intake. Nat Rev Endocrinol. (2019) 15:393–405. doi: 10.1038/s41574-019-0210-x
35. Stanley S, Wynne K, McGowan B, Bloom S. Hormonal regulation of food intake. Physiol Rev. (2005) 85:1131–58. doi: 10.1152/physrev.00015.2004
36. Grossman MI. The physiology of symptoms. II. Regulation of food intake. Am J Dig Dis. (1958) 3:659–68. doi: 10.1007/BF02231238
37. Rakha A, Mehak F, Shabbir MA, Arslan M, Ranjha M, Ahmed W, et al. Insights into the constellating drivers of satiety impacting dietary patterns and lifestyle. Front Nutr. (2022) 9:1002619. doi: 10.3389/fnut.2022.1002619
38. Cifuentes L, Acosta A. Homeostatic regulation of food intake. Clin Res Hepatol Gastroenterol. (2022) 46:101794. doi: 10.1016/j.clinre.2021.101794
39. Kim JY. Optimal diet strategies for weight loss and weight loss maintenance. J Obes Metab Syndr. (2021) 30:20–31. doi: 10.7570/jomes20065
40. Hopkins M, Beaulieu K, Gibbons C, Halford JCG, Blundell J, Stubbs J, et al. The Control of Food Intake in Humans. In: Feingold KR, Anawalt B, Blackman MR, Boyce A, Chrousos G, Corpas E, de Herder WW, Dhatariya K, Dungan K, Hofland J, Kalra S, Kaltsas G, Kapoor N, Koch C, Kopp P, Korbonits M, Kovacs CS, Kuohung W, Laferrère B, Levy M, McGee EA, McLachlan R, New M, Purnell J, Sahay R, Shah AS, Singer F, Sperling MA, Stratakis CA, Trence DL, Wilson DP, editors. Endotext. MDText.com, Inc, South Dartmouth (MA (2000).
41. Bellisle F. Impact of the daily meal pattern on energy balance. Scandinavian J Food Nutr. (2004) 48:114–8. doi: 10.3402/fnr.v48i3.1637
42. Garaulet M, Gomez-Abellan P. Timing of food intake and obesity: a novel association. Physiol Behav. (2014) 134:44–50. doi: 10.1016/j.physbeh.2014.01.001
43. Qian J, Morris CJ, Caputo R, Garaulet M, Scheer F. Ghrelin is impacted by the endogenous circadian system and by circadian misalignment in humans. Int J Obes (Lond). (2019) 43:1644–9. doi: 10.1038/s41366-018-0208-9
44. Collado MC, Engen PA, Bandin C, Cabrera-Rubio R, Voigt RM, Green SJ, et al. Timing of food intake impacts daily rhythms of human salivary microbiota: a randomized, crossover study. FASEB J Off Publ Fed Am Societies Exp Biol. (2018) 32:2060–72. doi: 10.1096/fj.201700697RR
45. McHill AW, Hull JT, McMullan CJ, Klerman EB. Chronic insufficient sleep has a limited impact on circadian rhythmicity of subjective hunger and awakening fasted metabolic hormones. Front Endocrinol. (2018) 9. doi: 10.3389/fendo.2018.00319
46. Scheer FA, Morris CJ, Shea SA. The internal circadian clock increases hunger and appetite in the evening independent of food intake and other behaviors. Obes (Silver Spring). (2013) 21:421–3. doi: 10.1002/oby.20351
47. Antunes LC, Levandovski R, Dantas G, Caumo W, Hidalgo MP. Obesity and shift work: chronobiological aspects. Nutr Res Rev. (2010) 23:155–68. doi: 10.1017/S0954422410000016
48. Barclay JL, Shostak A, Leliavski A, Tsang AH, Johren O, Muller-Fielitz H, et al. High-fat diet-induced hyperinsulinemia and tissue-specific insulin resistance in Cry-deficient mice. American journal of physiology. Endocrinol Metab. (2013) 304:E1053–63. doi: 10.1152/ajpendo.00512.2012
49. Di Lorenzo L, De Pergola G, Zocchetti C, L'Abbate N, Basso A, Pannacciulli N, et al. Effect of shift work on body mass index: results of a study performed in 319 glucose-tolerant men working in a Southern Italian industry. Int J Obes Relat Metab Disord. (2003) 27:1353–8. doi: 10.1038/sj.ijo.0802419
50. Morris CJ, Purvis TE, Mistretta J, Scheer FA. Effects of the internal circadian system and circadian misalignment on glucose tolerance in chronic shift workers. J Clin Endocrinol Metab. (2016) 101:1066–74. doi: 10.1210/jc.2015-3924
51. Shi SQ, Ansari TS, McGuinness OP, Wasserman DH, Johnson CH. Circadian disruption leads to insulin resistance and obesity. Curr Biol. (2013) 23:372–81. doi: 10.1016/j.cub.2013.01.048
52. Sun M, Feng W, Wang F, Li P, Li Z, Li M, et al. Meta-analysis on shift work and risks of specific obesity types. Obes Rev. (2018) 19:28–40. doi: 10.1111/obr.12621
53. Vetter C, Devore EE, Wegrzyn LR, Massa J, Speizer FE, Kawachi I, et al. Association between rotating night shift work and risk of coronary heart disease among women. JAMA J Am Med Assoc. (2016) 315:1726–34. doi: 10.1001/jama.2016.4454
54. Zarrinpar A, Chaix A, Panda S. Daily eating patterns and their impact on health and disease. Trends Endocrinol Metab. (2016) 27:69–83. doi: 10.1016/j.tem.2015.11.007
55. Arble DM, Bass J, Laposky AD, Vitaterna MH, Turek FW. Circadian timing of food intake contributes to weight gain. Obes (Silver Spring). (2009) 17:2100–2. doi: 10.1038/oby.2009.264
56. Fonken LK, Workman JL, Walton JC, Weil ZM, Morris JS, Haim A, et al. Light at night increases body mass by shifting the time of food intake. Proc Natl Acad Sci United States America. (2010) 107:18664–9. doi: 10.1073/pnas.1008734107
57. Hatori M, Vollmers C, Zarrinpar A, DiTacchio L, Bushong EA, Gill S, et al. Time-restricted feeding without reducing caloric intake prevents metabolic diseases in mice fed a high-fat diet. Cell Metab. (2012) 15:848–60. doi: 10.1016/j.cmet.2012.04.019
58. Fonken LK, Lieberman RA, Weil ZM, Nelson RJ. Dim light at night exaggerates weight gain and inflammation associated with a high-fat diet in male mice. Endocrinology. (2013) 154:3817–25. doi: 10.1210/en.2013-1121
59. Bonham MP, Bonnell EK, Huggins CE. Energy intake of shift workers compared to fixed day workers: A systematic review and meta-analysis. Chronobiol Int. (2016) 33:1086–100. doi: 10.1080/07420528.2016.1192188
60. Beccuti G, Monagheddu C, Evangelista A, Ciccone G, Broglio F, Soldati L, et al. Timing of food intake: Sounding the alarm about metabolic impairments? A systematic review. Pharmacol Res. (2017) 125:132–41. doi: 10.1016/j.phrs.2017.09.005
61. Martinez-Lozano N, Tvarijonaviciute A, Rios R, Baron I, Scheer F, Garaulet M. Late eating is associated with obesity, inflammatory markers and circadian-related disturbances in school-aged children. Nutrients. (2020) 12:2881. doi: 10.3390/nu12092881
62. Garaulet M, Gomez-Abellan P, Alburquerque-Bejar JJ, Lee YC, Ordovas JM, Scheer FA. Timing of food intake predicts weight loss effectiveness. Int J Obes (Lond). (2013) 37:604–11. doi: 10.1038/ijo.2012.229
63. Mesas AE, Muñoz-Pareja M, López-García E, Rodríguez-Artalejo F. Selected eating behaviours and excess body weight: a systematic review. Obes Rev. (2012) 13:106–35. doi: 10.1111/j.1467-789X.2011.00936.x
64. Bertéus Forslund H, Lindroos AK, Sjöström L, Lissner L. Meal patterns and obesity in Swedish women-a simple instrument describing usual meal types, frequency and temporal distribution. Eur J Clin Nutr. (2002) 56:740–7. doi: 10.1038/sj.ejcn.1601387
65. Corbalán-Tutau MD, Madrid JA, Garaulet M. Timing and duration of sleep and meals in obese and normal weight women. Association with increase blood pressure. Appetite. (2012) 59:9–16. doi: 10.1016/j.appet.2012.03.015
66. Aronoff NJ, Geliebter A, Zammit G. Gender and body mass index as related to the night-eating syndrome in obese outpatients. J Am Diet Assoc. (2001) 101:102–4. doi: 10.1016/S0002-8223(01)00022-0
67. Baron KG, Reid KJ, Kern AS, Zee PC. Role of sleep timing in caloric intake and BMI. Obes (Silver Spring). (2011) 19:1374–81. doi: 10.1038/oby.2011.100
68. Winkler G, Döring A, Keil U. Meal patterns in middle-aged men in Southern Germany: results from the MONICA Augsburg dietary survey 1984/85. Appetite. (1999) 32:33–7. doi: 10.1006/appe.1998.0193
69. McHill AW, Phillips AJ, Czeisler CA, Keating L, Yee K, Barger LK, et al. Later circadian timing of food intake is associated with increased body fat. Am J Clin Nutr. (2017) 106:1213–9. doi: 10.3945/ajcn.117.161588
70. Reid KJ, Baron KG, Zee PC. Meal timing influences daily caloric intake in healthy adults. Nutr Res. (2014) 34:930–5. doi: 10.1016/j.nutres.2014.09.010
71. Bandin C, Scheer FA, Luque AJ, Avila-Gandia V, Zamora S, Madrid JA, et al. Meal timing affects glucose tolerance, substrate oxidation and circadian-related variables: A randomized, crossover trial. Int J Obes (Lond). (2015) 39:828–33. doi: 10.1038/ijo.2014.182
72. Bo S, Fadda M, Castiglione A, Ciccone G, De Francesco A, Fedele D, et al. Is the timing of caloric intake associated with variation in diet-induced thermogenesis and in the metabolic pattern? A randomized cross-over study. Int J Obes (Lond). (2015) 39:1689–95. doi: 10.1038/ijo.2015.138
73. Wang JB, Patterson RE, Ang A, Emond JA, Shetty N, Arab L. Timing of energy intake during the day is associated with the risk of obesity in adults. J Hum Nutr Diet. (2014) 27 Suppl 2:255–62. doi: 10.1111/jhn.12141
74. Morgan LM, Shi JW, Hampton SM, Frost G. Effect of meal timing and glycaemic index on glucose control and insulin secretion in healthy volunteers. Br J Nutr. (2012) 108:1286–91. doi: 10.1017/S0007114511006507
75. Scheer FA, Hilton MF, Mantzoros CS, Shea SA. Adverse metabolic and cardiovascular consequences of circadian misalignment. Proc Natl Acad Sci U.S.A. (2009) 106:4453–8. doi: 10.1073/pnas.0808180106
76. Tsuchida Y, Hata S, Sone Y. Effects of a late supper on digestion and the absorption of dietary carbohydrates in the following morning. J Physiol Anthropol. (2013) 32:9. doi: 10.1186/1880-6805-32-9
77. Jakubowicz D, Barnea M, Wainstein J, Froy O. High caloric intake at breakfast vs. dinner differentially influences weight loss of overweight and obese women. Obes (Silver Spring). (2013) 21:2504–12. doi: 10.1002/oby.20460
78. Jakubowicz D, Wainstein J, Ahren B, Bar-Dayan Y, Landau Z, Rabinovitz HR, et al. High-energy breakfast with low-energy dinner decreases overall daily hyperglycaemia in type 2 diabetic patients: a randomised clinical trial. Diabetologia. (2015) 58:912–9. doi: 10.1007/s00125-015-3524-9
79. Qin LQ, Li J, Wang Y, Wang J, Xu JY, Kaneko T. The effects of nocturnal life on endocrine circadian patterns in healthy adults. Life Sci. (2003) 73:2467–75. doi: 10.1016/S0024-3205(03)00628-3
80. Dashti HS, Scheer F, Saxena R, Garaulet M. Timing of food intake: identifying contributing factors to design effective interventions. Adv Nutr. (2019) 10:606–20. doi: 10.1093/advances/nmy131
81. Morris CJ, Garcia JI, Myers S, Yang JN, Trienekens N, Scheer FA. The human circadian system has a dominating role in causing the morning/evening difference in diet-induced thermogenesis. Obes (Silver Spring). (2015) 23:2053–8. doi: 10.1002/oby.21189
82. Morris CJ, Yang JN, Garcia JI, Myers S, Bozzi I, Wang W, et al. Endogenous circadian system and circadian misalignment impact glucose tolerance via separate mechanisms in humans. Proc Natl Acad Sci United States America. (2015) 112:E2225–34. doi: 10.1073/pnas.1418955112
83. Qian J, Dalla Man C, Morris CJ, Cobelli C, Scheer F. Differential effects of the circadian system and circadian misalignment on insulin sensitivity and insulin secretion in humans. Diabetes Obes Metab. (2018) 20:2481–5. doi: 10.1111/dom.13391
84. Garaulet M, Gómez-Abellán P, Rubio-Sastre P, Madrid JA, Saxena R, Scheer FA. Common type 2 diabetes risk variant in MTNR1B worsens the deleterious effect of melatonin on glucose tolerance in humans. Metabolism. (2015) 64:1650–7. doi: 10.1016/j.metabol.2015.08.003
85. Grimaldi D, Carter JR, Van Cauter E, Leproult R. Adverse impact of sleep restriction and circadian misalignment on autonomic function in healthy young adults. Hypertension. (2016) 68:243–50. doi: 10.1161/HYPERTENSIONAHA.115.06847
86. Morris CJ, Purvis TE, Hu K, Scheer FA. Circadian misalignment increases cardiovascular disease risk factors in humans. Proc Natl Acad Sci United States America. (2016) 113:E1402–11. doi: 10.1073/pnas.1516953113
87. Kant AK, Graubard BI. Association of self-reported sleep duration with eating behaviors of American adults: NHANES 2005-2010. Am J Clin Nutr. (2014) 100:938–47. doi: 10.3945/ajcn.114.085191
88. Ruddick-Collins LC, Johnston JD, Morgan PJ, Johnstone AM. The Big Breakfast Study: Chrono-nutrition influence on energy expenditure and bodyweight. Nutr Bull. (2018) 43:174–83. doi: 10.1111/nbu.12323
89. Zitting KM, Vujovic N, Yuan RK, Isherwood CM, Medina JE, Wang W, et al. Human resting energy expenditure varies with circadian phase. Curr Biol. (2018) 28:3685–3690.e3. doi: 10.1016/j.cub.2018.10.005
90. Roenneberg T, Allebrandt KV, Merrow M, Vetter C. Social jetlag and obesity. Curr Biol. (2012) 22:939–43. doi: 10.1016/j.cub.2012.03.038
91. Cox KH, Takahashi JS. Circadian clock genes and the transcriptional architecture of the clock mechanism. J Mol Endocrinol. (2019) 63:R93–R102. doi: 10.1530/JME-19-0153
92. Kant AK, Graubard BI. Within-person comparison of eating behaviors, time of eating, and dietary intake on days with and without breakfast: NHANES 2005-2010. Am J Clin Nutr. (2015) 102:661–70. doi: 10.3945/ajcn.115.110262
93. Stanton JL Jr., Keast DR. Serum cholesterol, fat intake, and breakfast consumption in the United States adult population. J Am Coll Nutr. (1989) 8:567–72. doi: 10.1080/07315724.1989.10720328
94. Cummings DE, Purnell JQ, Frayo RS, Schmidova K, Wisse BE, Weigle DS. A preprandial rise in plasma ghrelin levels suggests a role in meal initiation in humans. Diabetes. (2001) 50:1714–9. doi: 10.2337/diabetes.50.8.1714
95. Natalucci G, Riedl S, Gleiss A, Zidek T, Frisch H. Spontaneous 24-h ghrelin secretion pattern in fasting subjects: maintenance of a meal-related pattern. Eur J Endocrinol. (2005) 152:845–50. doi: 10.1530/eje.1.01919
96. Scheving LA. Biological clocks and the digestive system. Gastroenterology. (2000) 119:536–49. doi: 10.1053/gast.2000.9305
97. Landgraf D, Neumann AM, Oster H. Circadian clock-gastrointestinal peptide interaction in peripheral tissues and the brain. Best Pract Res Clin Endocrinol Metab. (2017) 31:561–71. doi: 10.1016/j.beem.2017.10.007
98. Trommelen J, van Loon LJ. Pre-sleep protein ingestion to improve the skeletal muscle adaptive response to exercise training. Nutrients. (2016) 8:763. doi: 10.3390/nu8120763
100. Paschos GK, Ibrahim S, Song WL, Kunieda T, Grant G, Reyes TM, et al. Obesity in mice with adipocyte-specific deletion of clock component Arntl. Nat Med. (2012) 18:1768–77. doi: 10.1038/nm.2979
101. Kuang Z, Wang Y, Li Y, Ye C, Ruhn KA, Behrendt CL, et al. The intestinal microbiota programs diurnal rhythms in host metabolism through histone deacetylase 3. Science. (2019) 365:1428–34. doi: 10.1126/science.aaw3134
102. Wang Y, Kuang Z, Yu X, Ruhn KA, Kubo M, Hooper LV. The intestinal microbiota regulates body composition through NFIL3 and the circadian clock. Science. (2017) 357:912–6. doi: 10.1126/science.aan0677
103. Mashaqi S, Gozal D. "Circadian misalignment and the gut microbiome. A bidirectional relationship triggering inflammation and metabolic disorders"- a literature review. Sleep Med. (2020) 72:93–108. doi: 10.1016/j.sleep.2020.03.020
104. Leung GKW, Huggins CE, Bonham MP. Effect of meal timing on postprandial glucose responses to a low glycemic index meal: A crossover trial in healthy volunteers. Clin Nutr. (2019) 38:465–71. doi: 10.1016/j.clnu.2017.11.010
105. Gupta NJ, Kumar V, Panda S. A camera-phone based study reveals erratic eating pattern and disrupted daily eating-fasting cycle among adults in India. PloS One. (2017) 12:e0172852. doi: 10.1371/journal.pone.0172852
106. Rynders CA, Thomas EA, Zaman A, Pan Z, Catenacci VA, Melanson EL. Effectiveness of intermittent fasting and time-restricted feeding compared to continuous energy restriction for weight loss. Nutrients. (2019) 11:2442. doi: 10.3390/nu11102442
107. Hutchison AT, Regmi P, Manoogian ENC, Fleischer JG, Wittert GA, Panda S, et al. Time-restricted feeding improves glucose tolerance in men at risk for type 2 diabetes: A randomized crossover trial. Obes (Silver Spring). (2019) 27:724–32. doi: 10.1002/oby.22449
108. Jamshed H, Beyl RA, Della Manna DL, Yang ES, Ravussin E, Peterson CM. Early time-restricted feeding improves 24-hour glucose levels and affects markers of the circadian clock, aging, and autophagy in humans. Nutrients. (2019) 11:1234. doi: 10.3390/nu11061234
109. Chow LS, Manoogian ENC, Alvear A, Fleischer JG, Thor H, Dietsche K, et al. Time-restricted eating effects on body composition and metabolic measures in humans who are overweight: A feasibility study. Obes (Silver Spring). (2020) 28:860–9. doi: 10.1002/oby.22756
110. Moro T, Tinsley G, Bianco A, Marcolin G, Pacelli QF, Battaglia G, et al. Effects of eight weeks of time-restricted feeding (16/8) on basal metabolism, maximal strength, body composition, inflammation, and cardiovascular risk factors in resistance-trained males. J Trans Med. (2016) 14:290. doi: 10.1186/s12967-016-1044-0
111. Cienfuegos S, Gabel K, Kalam F, Ezpeleta M, Wiseman E, Pavlou V, et al. Effects of 4- and 6-h time-restricted feeding on weight and cardiometabolic health: A randomized controlled trial in adults with obesity. Cell Metab. (2020) 32:366–378.e3. doi: 10.1016/j.cmet.2020.06.018
112. Jones R, Pabla P, Mallinson J, Nixon A, Taylor T, Bennett A, et al. Two weeks of early time-restricted feeding (eTRF) improves skeletal muscle insulin and anabolic sensitivity in healthy men. Am J Clin Nutr. (2020) 112:1015–28. doi: 10.1093/ajcn/nqaa192
113. Sutton EF, Beyl R, Early KS, Cefalu WT, Ravussin E, Peterson CM. Early time-restricted feeding improves insulin sensitivity, blood pressure, and oxidative stress even without weight loss in men with prediabetes. Cell Metab. (2018) 27:1212–1221.e3. doi: 10.1016/j.cmet.2018.04.010
114. Cai H, Qin YL, Shi ZY, Chen JH, Zeng MJ, Zhou W, et al. Effects of alternate-day fasting on body weight and dyslipidaemia in patients with non-alcoholic fatty liver disease: a randomised controlled trial. BMC Gastroenterol. (2019) 19:219. doi: 10.1186/s12876-019-1132-8
115. Zeb F, Wu X, Chen L, Fatima S, Haq IU, Chen A, et al. Effect of time-restricted feeding on metabolic risk and circadian rhythm associated with gut microbiome in healthy males. Br J Nutr. (2020) 123:1216–26. doi: 10.1017/S0007114519003428
116. Wilkinson MJ, Manoogian ENC, Zadourian A, Lo H, Fakhouri S, Shoghi A, et al. Ten-hour time-restricted eating reduces weight, blood pressure, and atherogenic lipids in patients with metabolic syndrome. Cell Metab. (2020) 31:92–104.e5. doi: 10.1016/j.cmet.2019.11.004
117. Peters B, Koppold-Liebscher DA, Schuppelius B, Steckhan N, Pfeiffer AFH, Kramer A, et al. Effects of early vs. Late time-restricted eating on cardiometabolic health, inflammation, and sleep in overweight and obese women: A study protocol for the chronoFast trial. Front Nutr. (2021) 8:765543. doi: 10.3389/fnut.2021.765543
118. Schuppelius B, Peters B, Ottawa A, Pivovarova-Ramich O. Time restricted eating: A dietary strategy to prevent and treat metabolic disturbances. Front Endocrinol (Lausanne). (2021) 12:683140. doi: 10.3389/fendo.2021.683140
119. Jamshed H, Steger FL, Bryan DR, Richman JS, Warriner AH, Hanick CJ, et al. Effectiveness of early time-restricted eating for weight loss, fat loss, and cardiometabolic health in adults with obesity: A randomized clinical trial. JAMA Intern Med. (2022) 182:953–62. doi: 10.1001/jamainternmed.2022.3050
120. Liu D, Huang Y, Huang C, Yang S, Wei X, Zhang P, et al. Calorie restriction with or without time-restricted eating in weight loss. N Engl J Med. (2022) 386:1495–504. doi: 10.1056/NEJMoa2114833
121. Kamarul Zaman M, Teng N, Kasim SS, Juliana N, Alshawsh MA. Effects of time-restricted eating with different eating duration on anthropometrics and cardiometabolic health: A systematic review and meta-analysis. World J Cardiol. (2023) 15:354–74. doi: 10.4330/wjc.v15.i7.354
122. Franzago M, Alessandrelli E, Notarangelo S, Stuppia L, Vitacolonna E. Chrono-nutrition: circadian rhythm and personalized nutrition. Int J Mol Sci. (2023) 24:2571. doi: 10.3390/ijms24032571
123. Ghafari M, Doosti-Irani A, Amiri M, Cheraghi Z. Prevalence of the skipping breakfast among the Iranian students: A review article. Iran J Public Health. (2017) 46:882–9.
124. Sudo N, Sekiyama M, Ohtsuka R, Maharjan M. Gender differences in "luxury food intake" owing to temporal distribution of eating occasions among adults of Hindu communities in lowland Nepal. Asia Pac J Clin Nutr. (2009) 18:441–6.
125. Kant AK, Graubard BI. 40-year trends in meal and snack eating behaviors of American adults. J Acad Nutr Diet. (2015) 115:50–63. doi: 10.1016/j.jand.2014.06.354
126. Fischer D, Lombardi DA, Marucci-Wellman H, Roenneberg T. Chronotypes in the US - Influence of age and sex. PloS One. (2017) 12:e0178782. doi: 10.1371/journal.pone.0178782
127. O'Reardon JP, Peshek A, Allison KC. Night eating syndrome : diagnosis, epidemiology and management. CNS Drugs. (2005) 19:997–1008. doi: 10.2165/00023210-200519120-00003
128. Yamanaka-Okumura H, Nakamura T, Takeuchi H, Miyake H, Katayama T, Arai H, et al. Effect of late evening snack with rice ball on energy metabolism in liver cirrhosis. Eur J Clin Nutr. (2006) 60:1067–72. doi: 10.1038/sj.ejcn.1602420
129. Guentcheva I, Dugas EN, Hanusaik N, Drapeau V, Sylvestre MP, O'Loughlin J. Depression symptoms and night eating in young adulthood. Eat Weight Disord. (2020) 25:1593–600. doi: 10.1007/s40519-019-00796-4
130. St-Onge MP, Ard J, Baskin ML, Chiuve SE, Johnson HM, Kris-Etherton P, et al. Meal timing and frequency: implications for cardiovascular disease prevention: A scientific statement from the american heart association. Circulation. (2017) 135:e96–e121. doi: 10.1161/CIR.0000000000000476
131. de Castro JM. Behavioral genetics of food intake regulation in free-living humans. Nutrition. (1999) 15:550–4. doi: 10.1016/S0899-9007(99)00114-8
132. Tanaka T, Ngwa JS, van Rooij FJ, Zillikens MC, Wojczynski MK, Frazier-Wood AC, et al. Genome-wide meta-analysis of observational studies shows common genetic variants associated with macronutrient intake. Am J Clin Nutr. (2013) 97:1395–402. doi: 10.3945/ajcn.112.052183
133. Danilenko KV, Plisov IL, Hébert M, Kräuchi K, Wirz-Justice A. Influence of timed nutrient diet on depression and light sensitivity in seasonal affective disorder. Chronobiol Int. (2008) 25:51–64. doi: 10.1080/07420520801903976
134. Nohara K, Yoo SH, Chen ZJ. Manipulating the circadian and sleep cycles to protect against metabolic disease. Front Endocrinol (Lausanne). (2015) 6:35. doi: 10.3389/fendo.2015.00035
135. Grimm ER, Steinle NI. Genetics of eating behavior: established and emerging concepts. Nutr Rev. (2011) 69:52–60. doi: 10.1111/nure.2011.69.issue-1
136. de Castro JM. Genetic influences on daily intake and meal patterns of humans. Physiol Behav. (1993) 53:777–82. doi: 10.1016/0031-9384(93)90188-L
137. Pallister T, Spector TD, Menni C. Twin studies advance the understanding of gene-environment interplay in human nutrigenomics. Nutr Res Rev. (2014) 27:242–51. doi: 10.1017/S095442241400016X
138. Hewitt JK, Stunkard AJ, Carroll D, Sims J, Turner JR. A twin study approach towards understanding genetic contributions to body size and metabolic rate. Acta Genet Med Gemellol (Roma). (1991) 40:133–46. doi: 10.1017/S0001566000002567
139. Frahnow T, Osterhoff MA, Hornemann S, Kruse M, Surma MA, Klose C, et al. Heritability and responses to high fat diet of plasma lipidomics in a twin study. Sci Rep. (2017) 7:3750. doi: 10.1038/s41598-017-03965-6
140. Keskitalo K, Tuorila H, Spector TD, Cherkas LF, Knaapila A, Kaprio J, et al. The Three-Factor Eating Questionnaire, body mass index, and responses to sweet and salty fatty foods: a twin study of genetic and environmental associations. Am J Clin Nutr. (2008) 88:263–71. doi: 10.1093/ajcn/88.2.263
141. Tholin S, Rasmussen F, Tynelius P, Karlsson J. Genetic and environmental influences on eating behavior: the Swedish Young Male Twins Study. Am J Clin Nutr. (2005) 81:564–9. doi: 10.1093/ajcn/81.3.564
142. de Castro JM. Heritability of diurnal changes in food intake in free-living humans. Nutrition. (2001) 17:713–20. doi: 10.1016/S0899-9007(01)00611-6
143. Lopez-Minguez J, Dashti HS, Madrid-Valero JJ, Madrid JA, Saxena R, Scheer F, et al. Heritability of the timing of food intake. Clin Nutr. (2019) 38:767–73. doi: 10.1016/j.clnu.2018.03.002
144. Vitaterna MH, Shimomura K, Jiang P. Genetics of circadian rhythms. Neurol Clin. (2019) 37:487–504. doi: 10.1016/j.ncl.2019.05.002
145. Ashbrook LH, Krystal AD, Fu YH, Ptáček LJ. Genetics of the human circadian clock and sleep homeostat. Neuropsychopharmacology. (2020) 45:45–54. doi: 10.1038/s41386-019-0476-7
146. Garaulet M, Vera B, Bonnet-Rubio G, Gomez-Abellan P, Lee YC, Ordovas JM. Lunch eating predicts weight-loss effectiveness in carriers of the common allele at PERILIPIN1: the ONTIME (Obesity, Nutrigenetics, Timing, Mediterranean) study. Am J Clin Nutr. (2016) 104:1160–6. doi: 10.3945/ajcn.116.134528
147. Lundgren JD, Allison KC, Stunkard AJ. Familial aggregation in the night eating syndrome. Int J Eat Disord. (2006) 39:516–8. doi: 10.1002/eat.20269
148. Grieneisen L, Dasari M, Gould TJ, Björk JR, Grenier JC, Yotova V, et al. Gut microbiome heritability is nearly universal but environmentally contingent. Science. (2021) 373:181–6. doi: 10.1126/science.aba5483
149. Lopez-Minguez J, Saxena R, Bandin C, Scheer FA, Garaulet M. Late dinner impairs glucose tolerance in MTNR1B risk allele carriers: A randomized, cross-over study. Clin Nutr. (2018) 37:1133–40. doi: 10.1016/j.clnu.2017.04.003
150. Boraska V, Davis OS, Cherkas LF, Helder SG, Harris J, Krug I, et al. Genome-wide association analysis of eating disorder-related symptoms, behaviors, and personality traits. Am J Med Genet B Neuropsychiatr Genet. (2012) 159B:803–11. doi: 10.1002/ajmg.b.32087
151. Xiao Q, Garaulet M, Scheer F. Meal timing and obesity: interactions with macronutrient intake and chronotype. Int J Obes (Lond). (2019) 43:1701–11. doi: 10.1038/s41366-018-0284-x
152. Wilson T, DeVaan LS, LaCasse ME, Gile EM, Weis MJ, Ahmann MD, et al. Effect of walnut predinner snack on mealtime hunger and nutrient intake among university students. J Med Food. (2022) 25:89–96. doi: 10.1089/jmf.2021.0092
153. Braden ML, Gwin JA, Leidy HJ. Protein source influences acute appetite and satiety but not subsequent food intake in healthy adults. J Nutr. (2023) 153:1825–33. doi: 10.1016/j.tjnut.2023.04.001
154. Clark MJ, Slavin JL. The effect of fiber on satiety and food intake: a systematic review. J Am Coll Nutr. (2013) 32:200–11. doi: 10.1080/07315724.2013.791194
155. Montaruli A, Castelli L, Mulè A, Scurati R, Esposito F, Galasso L, et al. Biological rhythm and chronotype: new perspectives in health. Biomolecules. (2021) 11:487. doi: 10.3390/biom11040487
156. Jones SE, Lane JM, Wood AR, van Hees VT, Tyrrell J, Beaumont RN, et al. Genome-wide association analyses of chronotype in 697,828 individuals provides insights into circadian rhythms. Nat Commun. (2019) 10:343. doi: 10.1038/s41467-018-08259-7
157. Kalmbach DA, Schneider LD, Cheung J, Bertrand SJ, Kariharan T, Pack AI, et al. Genetic basis of chronotype in humans: insights from three landmark GWAS. Sleep. (2017) 40:zsw048. doi: 10.1093/sleep/zsw048
158. Bazzani A, Marantonio S, Andreozzi G, Lorenzoni V, Bruno S, Cruz-Sanabria F, et al. Late chronotypes, late mealtimes. Chrononutrition and sleep habits during the COVID-19 lockdown in Italy. Appetite. (2022) 172:105951. doi: 10.1016/j.appet.2022.105951
159. Lucassen EA, Zhao X, Rother KI, Mattingly MS, Courville AB, de Jonge L, et al. Evening chronotype is associated with changes in eating behavior, more sleep apnea, and increased stress hormones in short sleeping obese individuals. PloS One. (2013) 8:e56519. doi: 10.1371/journal.pone.0056519
160. Reutrakul S, Hood MM, Crowley SJ, Morgan MK, Teodori M, Knutson KL. The relationship between breakfast skipping, chronotype, and glycemic control in type 2 diabetes. Chronobiol Int. (2014) 31:64–71. doi: 10.3109/07420528.2013.821614
161. Maukonen M, Kanerva N, Partonen T, Kronholm E, Tapanainen H, Kontto J, et al. Chronotype differences in timing of energy and macronutrient intakes: A population-based study in adults. Obes (Silver Spring). (2017) 25:608–15. doi: 10.1002/oby.21747
162. Kerksick CM, Arent S, Schoenfeld BJ, Stout JR, Campbell B, Wilborn CD, et al. International society of sports nutrition position stand: nutrient timing. J Int Soc Sports Nutr. (2017) 14:33. doi: 10.1186/s12970-017-0189-4
163. Gavrieli A, Karfopoulou E, Kardatou E, Spyreli E, Fragopoulou E, Mantzoros CS, et al. Effect of different amounts of coffee on dietary intake and appetite of normal-weight and overweight/obese individuals. Obes (Silver Spring). (2013) 21:1127–32. doi: 10.1002/oby.20190
164. Panek-Shirley LM, DeNysschen C, O'Brien E, Temple JL. Caffeine transiently affects food intake at breakfast. J Acad Nutr Diet. (2018) 118:1832–43. doi: 10.1016/j.jand.2018.05.015
165. Shimada H, Nilsson C, Noda Y, Kim H, Lundström T, Yajima T. Effects of food on the pharmacokinetics of omega-3-carboxylic acids in healthy Japanese male subjects: A phase I, randomized, open-label, three-period, crossover trial. J Atheroscler Thromb. (2017) 24:980–7. doi: 10.5551/jat.38737
166. Yeomans MR, Caton S, Hetherington MM. Alcohol and food intake. Curr Opin Clin Nutr Metab Care. (2003) 6:639–44. doi: 10.1097/00075197-200311000-00006
167. Caton SJ, Ball M, Ahern A, Hetherington MM. Dose-dependent effects of alcohol on appetite and food intake. Physiol Behav. (2004) 81:51–8. doi: 10.1016/j.physbeh.2003.12.017
168. Lloyd-Richardson EE, Lucero ML, Dibello JR, Jacobson AE, Wing RR. The relationship between alcohol use, eating habits and weight change in college freshmen. Eat Behav. (2008) 9:504–8. doi: 10.1016/j.eatbeh.2008.06.005
169. Yetish G, Kaplan H, Gurven M, Wood B, Pontzer H, Manger PR, et al. Natural sleep and its seasonal variations in three pre-industrial societies. Curr Biol. (2015) 25:2862–8. doi: 10.1016/j.cub.2015.09.046
170. Meléndez-Fernández OH, Liu JA, Nelson RJ. Circadian rhythms disrupted by light at night and mistimed food intake alter hormonal rhythms and metabolism. Int J Mol Sci. (2023) 24:3392. doi: 10.3390/ijms24043392
171. St-Onge MP, Pizinger T, Kovtun K, RoyChoudhury A. Sleep and meal timing influence food intake and its hormonal regulation in healthy adults with overweight/obesity. Eur J Clin Nutr. (2019) 72:76–82. doi: 10.1038/s41430-018-0312-x
172. Hsieh SD, Muto T, Murase T, Tsuji H, Arase Y. Association of short sleep duration with obesity, diabetes, fatty liver and behavioral factors in Japanese men. Intern Med. (2011) 50:2499–502. doi: 10.2169/internalmedicine.50.5844
173. Al Khatib HK, Hall WL, Creedon A, Ooi E, Masri T, McGowan L, et al. Sleep extension is a feasible lifestyle intervention in free-living adults who are habitually short sleepers: a potential strategy for decreasing intake of free sugars? A randomized controlled pilot study. Am J Clin Nutr. (2018) 107:43–53. doi: 10.1093/ajcn/nqx030
174. Dashti HS, Scheer FA, Jacques PF, Lamon-Fava S, Ordovás JM. Short sleep duration and dietary intake: epidemiologic evidence, mechanisms, and health implications. Adv Nutr. (2015) 6:648–59. doi: 10.3945/an.115.008623
175. Tasali E, Chapotot F, Wroblewski K, Schoeller D. The effects of extended bedtimes on sleep duration and food desire in overweight young adults: a home-based intervention. Appetite. (2014) 80:220–4. doi: 10.1016/j.appet.2014.05.021
176. Wittmann M, Dinich J, Merrow M, Roenneberg T. Social jetlag: misalignment of biological and social time. Chronobiol Int. (2006) 23:497–509. doi: 10.1080/07420520500545979
177. Spaeth AM, Dinges DF, Goel N. Sex and race differences in caloric intake during sleep restriction in healthy adults. Am J Clin Nutr. (2014) 100:559–66. doi: 10.3945/ajcn.114.086579
178. Markwald RR, Melanson EL, Smith MR, Higgins J, Perreault L, Eckel RH, et al. Impact of insufficient sleep on total daily energy expenditure, food intake, and weight gain. Proc Natl Acad Sci U.S.A. (2013) 110:5695–700. doi: 10.1073/pnas.1216951110
179. Ness KM, Strayer SM, Nahmod NG, SChade MM, Chang AM, Shearer GC, et al. Four nights of sleep restriction suppress the postprandial lipemic response and decrease satiety. J Lipid Res. (2019) 60:1935–45. doi: 10.1194/jlr.P094375
180. Huseinovic E, Winkvist A, Freisling H, Slimani N, Boeing H, Buckland G, et al. Timing of eating across ten European countries - results from the European Prospective Investigation into Cancer and Nutrition (EPIC) calibration study. Public Health Nutr. (2019) 22:324–35. doi: 10.1017/S1368980018002288
181. Shariatpanahi ZV, Shariatpanahi MV, Shahbazi S, Hossaini A, Abadi A. Effect of Ramadan fasting on some indices of insulin resistance and components of the metabolic syndrome in healthy male adults. Br J Nutr. (2008) 100:147–51. doi: 10.1017/S000711450787231X
182. Park MK, Freisling H, Huseinovic E, Winkvist A, Huybrechts I, Crispim SP, et al. Comparison of meal patterns across five European countries using standardized 24-h recall (GloboDiet) data from the EFCOVAL project. Eur J Nutr. (2018) 57:1045–57. doi: 10.1007/s00394-017-1388-0
183. Ali T, Lessan N. Chrononutrition in the context of Ramadan: Potential implications. Diabetes Metab Res Rev. (2023) 40:e3728. doi: 10.1002/dmrr.3728
184. Kant AK, Graubard BI. Family income and education were related with 30-year time trends in dietary and meal behaviors of American children and adolescents. J Nutr. (2013) 143:690–700. doi: 10.3945/jn.112.165258
185. Thompson FE, Larkin FA, Brown MB. Weekend-weekday differences in reported dietary intake: The nationwide food consumption survey, 1977–78. Nutr Res. (1986) 6:647–62. doi: 10.1016/S0271-5317(86)80006-9
186. An R. Weekend-weekday differences in diet among U.S. adults, 2003-2012. Ann Epidemiol. (2016) 26:57–65. doi: 10.1016/j.annepidem.2015.10.010
187. Eicher-Miller HA, Khanna N, Boushey CJ, Gelfand SB, Delp EJ. Temporal dietary patterns derived among the adult participants of the national health and nutrition examination survey 1999-2004 are associated with diet quality. J Acad Nutr Diet. (2016) 116:283–91. doi: 10.1016/j.jand.2015.05.014
188. Esposito F, Sanmarchi F, Marini S, Masini A, Scrimaglia S, Adorno E, et al. Weekday and weekend differences in eating habits, physical activity and screen time behavior among a sample of primary school children: the "Seven days for my health" Project. Int J Environ Res Public Health. (2022) 19:4215. doi: 10.3390/ijerph19074215
189. Scander H, Lennernäs Wiklund M, Yngve A. Assessing time of eating in commensality research. Int J Environ Res Public Health. (2021) 18:2941. doi: 10.3390/ijerph18062941
190. Mohd Azmi NAS, Juliana N, Mohd Fahmi Teng NI, Azmani S, Das S, Effendy N. Consequences of circadian disruption in shift workers on chrononutrition and their psychosocial well-being. Int J Environ Res Public Health. (2020) 17:2043. doi: 10.3390/ijerph17062043
191. Haus E, Reinberg A, Mauvieux B, Le Floc'h N, Sackett-Lundeen L, Touitou Y. Risk of obesity in male shift workers: A chronophysiological approach. Chronobiol Int. (2016) 33:1018–36. doi: 10.3109/07420528.2016.1167079
192. Gifkins J, Johnston A, Loudoun R. The impact of shift work on eating patterns and self-care strategies utilised by experienced and inexperienced nurses. Chronobiol Int. (2018) 35:811–20. doi: 10.1080/07420528.2018.1466790
Keywords: meal timing, circadian clock, chrononutrition, precision nutrition, obesity, metabolic diseases
Citation: Peters B, Vahlhaus J and Pivovarova-Ramich O (2024) Meal timing and its role in obesity and associated diseases. Front. Endocrinol. 15:1359772. doi: 10.3389/fendo.2024.1359772
Received: 21 December 2023; Accepted: 01 March 2024;
Published: 22 March 2024.
Edited by:
Mengliu Yang, Chongqing Medical University, ChinaReviewed by:
Erin C. Hanlon, The University of Chicago, United StatesHenrik Oster, University of Lübeck, Germany
Copyright © 2024 Peters, Vahlhaus and Pivovarova-Ramich. This is an open-access article distributed under the terms of the Creative Commons Attribution License (CC BY). The use, distribution or reproduction in other forums is permitted, provided the original author(s) and the copyright owner(s) are credited and that the original publication in this journal is cited, in accordance with accepted academic practice. No use, distribution or reproduction is permitted which does not comply with these terms.
*Correspondence: Olga Pivovarova-Ramich, b2xnYS5yYW1pY2hAZGlmZS5kZQ==
†These authors have contributed equally to this work