- 1College of Veterinary Medicine, South China Agricultural University, Guangzhou, China
- 2Key Laboratory of Animal Genetics, Breeding and Reproduction in the Plateau Mountain Region, College of Animal Science, Guizhou University, Guiyang, China
- 3College of Veterinary Medicine, Shanxi Agricultural University, Jinzhong, China
Introduction: Nutritional deficiency occurs frequently during pregnancy and breastfeeding. Tryptophan (Trp), an essential amino acid which is critical for protein synthesis, serves as the precursor for serotonin, melatonin, and kynurenine (Kyn). The imbalance between serotonin and kynurenine pathways in Trp metabolism is closely related to inflammation and depression. This study assessed the effects of Trp deficiency on mouse early pregnancy.
Methods: Embryo implantation and decidualization were analyzed after female mice had been fed diets containing 0.2% Trp (for the control group), 0.062% Trp (for the low Trp group) and 0% Trp (for the Trp-free group) for two months. The uteri of the mice were collected on days 4, 5, and 8 of pregnancy for further analysis.
Results: On day 8 of pregnancy, the number of implantation sites were found to be similar between the control and the low Trp groups. However, no implantation sites were detected in the Trp-free group. On day 5 of pregnancy, plane polarity- and decidualization-related molecules showed abnormal expression pattern in the Trp-free group. On day 4 of pregnancy, there was no significant difference in uterine receptivity molecules between the low-Trp group and the control group, but uterine receptivity was abnormal in the Trp-free group. At implantation sites of the Trp-free group, IDO and AHR levels were markedly elevated. This potentially increased levels of Kyn, 2-hydroxy estradiol, and 4-hydroxy estradiol to affect decidualization.
Conclusions: Trp-free diet may impair decidualization via the IDO-KYN-AHR pathway.
1 Introduction
Embryo implantation involves the interaction between the active blastocyst and a receptive uterus, and this even occurs only when the uterine environment is favorable for blastocyst implantation. Decidualization is the process by which endometrial stromal fibroblasts differentiate into decidual cells. Both embryo implantation and decidualization are essential for the establishment and maintenance of pregnancy in rodents and primates (1, 2).
Essential amino acids are required for pregnancy. Poor placental amino acid transport results in reduced fetal growth (3, 4). Tryptophan (Trp), an essential amino acid, can be only obtained through the diet. Adults need 3.5 mg of L-Trp/kg body weight/day to maintain nitrogen balance (5). The amount of Trp in corn and sorghum is low, so sufficient Trp is often lacking in poor areas or areas where corn and sorghum are staple foods (6, 7). Under-absorption of Trp can also cause Trp deficiency in Hartnup disease which causes under-absorption of neutral amino acids (including Trp) in the renal tubules and malabsorption of these amino acids in the gastrointestinal tract (8). Crohn’s disease is also associated with insufficient Trp absorption (9). Trp and its metabolites are involved in the production of environmental pollutants and affect body development (10, 11). Air pollutants may cause Trp metabolic disorders through oxidative stress and inflammation (12). Due to the increased needs of both females and fetuses for growth and development, Trp is particularly crucial during pregnancy (13, 14). Although Trp can be metabolized through the kynurenine (Kyn), 5-hydroxytryptamine, and indole pathways, more than 95% of Trp is metabolized via the Kyn pathway. The main rate-limiting enzymes in the Kyn pathway are Trp-2,3-dioxygenase (TDO), indoleamine-2,3-dioxygenase 1 (IDO1), and idoleamine-2,3-dioxygenase 2 (IDO2) (15, 16). IDO is essential for pregnancy because inhibition of IDO results in fetal loss in pregnant mice (17). TDO2 is highly expressed in mouse decidua. Inhibition of TDO2 leads to decreased expression of Cox2 and Vegf in decidual cells (18). Trp hydroxylase (TPH) can convert Trp to serotonin, a crucial neurotransmitter involved in the control of adaptive responses and reactions to environmental changes (19). Pups of Tph1-deficient mice have major developmental abnormalities in the brain and other tissues (20).
Dioxins and similar compounds are chlorinated organic pollutants that affect cell proliferation and differentiation by binding to the aryl hydrocarbon receptor (AHR). These chemicals also stimulate tumor growth and cause strong immunological, developmental, and reproductive damage through mechanisms unrelated to cytotoxicity (21, 22). Trp metabolites can bind to AHR (23). Ahr-/- mice have an impaired ability to support embryo-fetal development (24). Abnormal activation of AHR and the aryl hydrocarbon receptor nuclear translocator (ARNT) is common in endometriosis and uterine leiomyoma (25).
For growth and reproduction, the National Research Council recommended a dietary Trp dose of 2.0 g/kg for mice (16). The data from studies of diets containing high levels of Trp are contradictory. Although one study reported that L- Trp at 3.3 g/kg body weight/day during mouse pregnancy has no obvious effect on fetal growth (26), another study showed that a high L- Trp diet leads to a lower fetal weight (27). A Trp-deficient diet can prolong the lifespan by slowing physiological ageing (28, 29). Maternal Trp deficiency is associated with adverse offspring outcomes (17, 30). However, the effects of Trp deficiency on embryo implantation and decidualization remain to be defined.
Therefore, female mice were fed Trp-deficient, low-Trp or control diets for two months to evaluate the impact of these conditions on implantation and decidualization. The findings demonstrated that implantation and decidualization continued to occur normally even when the Trp deficit level reached 0.062%. Meanwhile, we found that a Trp -free diet can activate AHR and that a Trp-deficient diet results in pregnancy failure through activation of the IDO-KYN-AHR pathway in mice.
2 Results
2.1 Complete deficiency of Trp results in abnormal decidualization
The body weights of mice in the control group, low-Trp group and Trp-free group were comparable at the beginning of feeding (Figure 1A). The body weights of mice in the control group were significantly higher than that those of mice in the low-Trp and Trp-free groups after 2 months of feeding (Figure 1A).
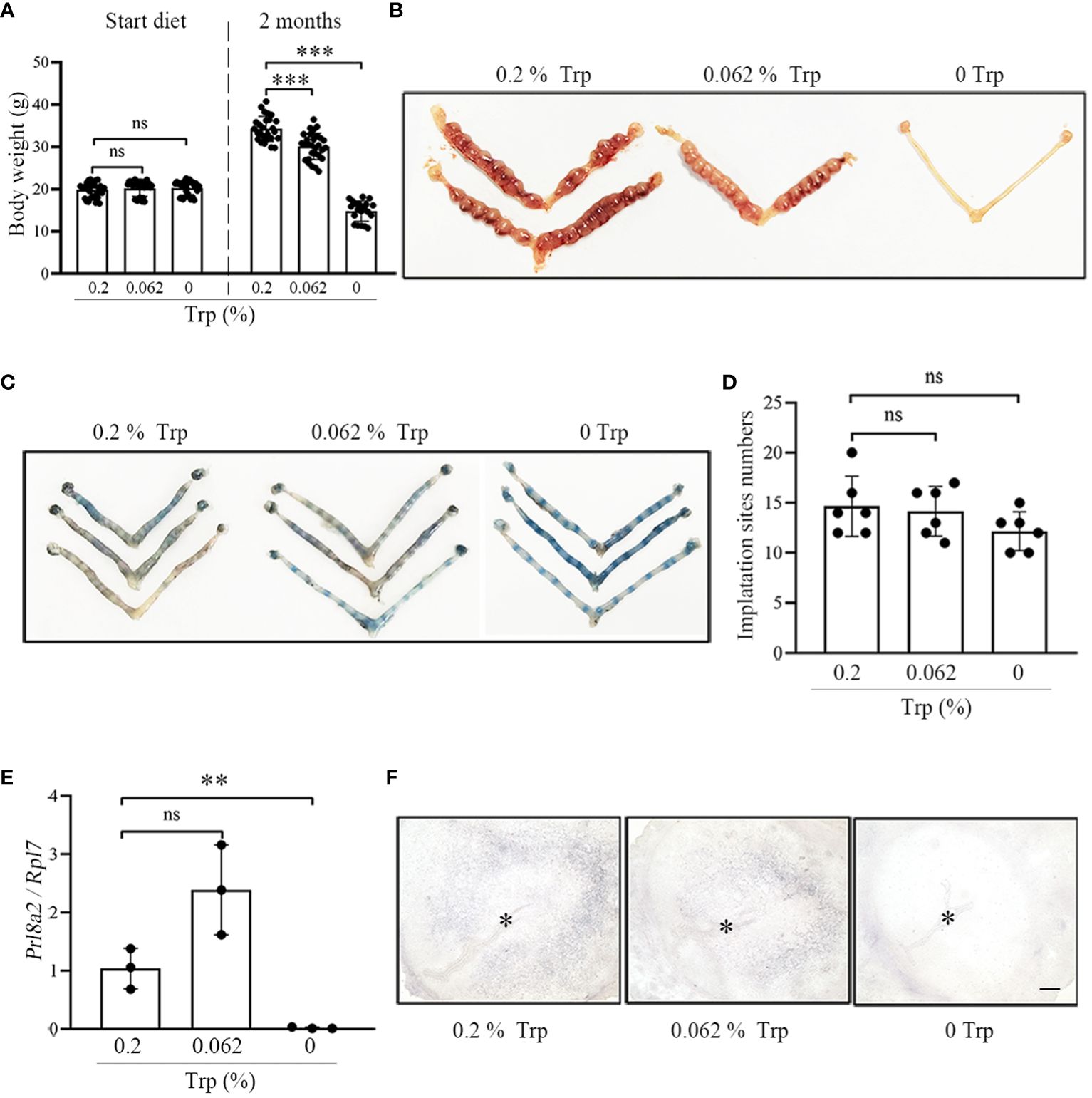
Figure 1 Complete Trp deficiency results in abnormal decidualization. (A) The body weight of female mice after feeding with different diet for two months. (B) A representative photograph showing the number of implantation sites on day 8 of pregnancy in each group (N=5 mice). (C) A representative photograph showing the number of implantation sites on day 5 of pregnancy in each group (N=5 mice). (D) Statistical analysis of the number of implantation sites on day 5 of pregnancy in each group. (E) qPCR analysis of Prl8a2 mRNA level in mouse uteri at implantation sites on day 5 in each group. (F) Alkaline phosphatase staining in mouse uteri on day 5 of implantation sites in each group. * Embryo. Scale bar, 50 μm. **, p < 0.01; ***, p < 0.001, ns, not significant.
To investigate the effects of Trp deficiency on early pregnancy, we examined mouse uteri in each group on day 8 of pregnancy. There was no obvious difference in the implantation sites between the low-Trp group and the control group on day 8 of pregnancy. In the Trp-free group, no implantation sites were observed in the uterus (Figure 1B). To explore the cause of pregnancy abnormalities on day 8 of pregnancy in the Trp-free group, we analyzed mouse uteri on day 5 of pregnancy. However, implantation sites were identified in each group, and the numbers of implantation sites were similar among the three groups (Figures 1C, D). We further examined the levels of decidualization-related markers.
Decidualization is critical for establishing pregnancy in mice (2). The prolactin family 8 subfamily A member 2 (Prl8a2) is a reliable marker for in vitro mouse decidualization (31). Compared with that in the control group, the expression of Prl8a2 in the low-Trp group was significantly higher, while Prl8a2 expression in the Trp-free group was significantly lower (Figure 1E). An increase in ALP activity is a marker for mouse decidualization (32). The density of alkaline phosphatase staining in the control group was higher than that in the low-Trp group, while alkaline phosphatase staining was not observed in the Trp-free group (Figure 1F). These results suggest that there was a decidualization abnormality in the Trp-free group.
2.2 Implantation chamber and planar cell polarity
A unique crypt (implantation chamber) is established when luminal epithelial evaginations move towards the antimesometrial pole and are usually “spear shaped” (33, 34). On day 5 of pregnancy, the morphology of the implantation chamber was similar between the control group and the low-Trp group. Compared to that in the control group, the morphology of the implantation chamber in the Trp-free group was abnormal. There were significantly more abnormal implantation chambers in the Trp-free group than in both the control and low-Trp groups (Figures 2A–C).
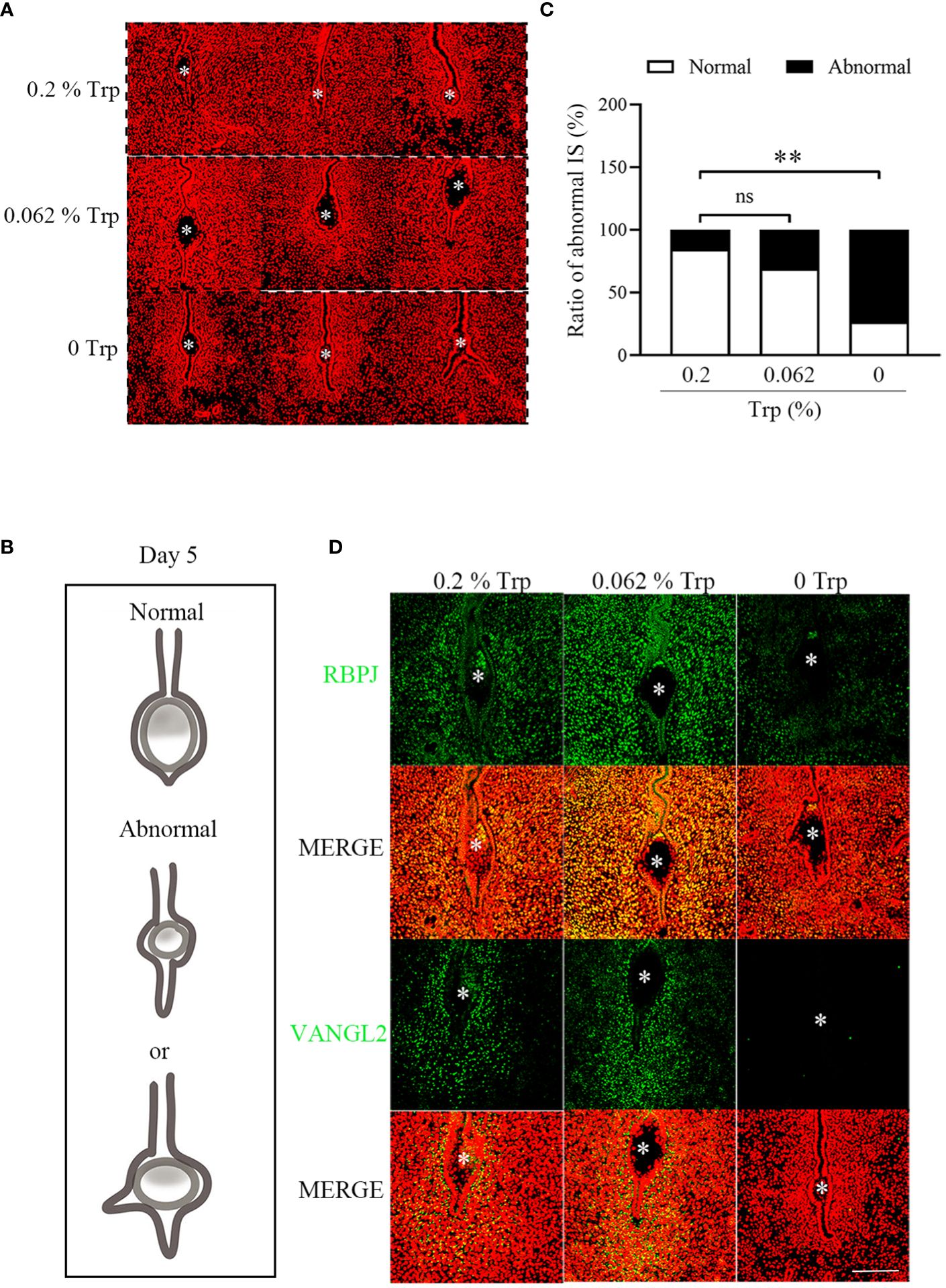
Figure 2 The morphology of implantation chambers and planar cell polarity signaling on day 5 of pregnancy. (A) The morphology of implantation chambers in each group. There were abnormal luminal closure and increased epithelial branching in Trp-free group. (B) A diagram showing normal and abnormal implantation chamber at implantation sites. (C) The number of abnormal implantation chambers and statistical analysis by the chi-square test (N=15 mice). IS: implantation sites. (D) Immunofluorescence of RBPJ and VANGL2 at implantation sites. * Embryo. Scale bar, 50 μm. **, p < 0.01, ns, not significant.
Planar cell polarity (PCP) signaling is necessary for crypt development (35). Van Gogh-Like Protein 2 (VANGL2) and Rbpsuh (RBPJ) are key PCP components. Epithelial Vangl2 null mice exhibit shallower luminal epithelial eversions and defects in crypt shape and size (36). In Rbpj-deficient mice, a seriously deviated uterus embryo axis at the implantation site, abnormal decidual morphology, and impaired embryonic development were observed (37). Therefore, we examined the expression of PCP signaling molecules in the uterus on day 5 of pregnancy in each group. The immunofluorescence intensity of VANGL2 and RBPJ in the uterus of the Trp-free group was lower than that in the control group, while the immunofluorescence signal in the low-Trp group was slightly greater than that in the control group (Figure 2D). These results suggest that PCP signaling is impaired in the Trp-free group.
2.3 Uterine receptivity
A successful pregnancy requires proper interaction between the activated embryo and the receptive uterus. On day 4 of pregnancy, uterine epithelial cells stop proliferating and begin to differentiate into the receptive phase (38, 39). Compared to that in the control group, immunostaining for Ki67, a marker of cell proliferation, was increased in epithelial cells on day 4 of pregnancy in the Trp-free group, while Ki67 immunostaining in the low-Trp group was similar to that in the control group (Figure 3A). Compared to that in the control and low-Trp groups, the number of subluminal stromal cells immunostained for Hand2 was obviously lower in the Trp-free group (Figure 3A). Lactoferrin (LTF) and complement C3 (C3) are target genes of oestrogen signaling (40, 41). Compared to that in the control group, Ltf and C3 expression in the Trp-free group was significantly higher. In the low-Trp group, the expression of Ltf was similar to that in the control group, but C3 expression was significantly higher than that in the control group (Figures 3B, C). Suppression of oestrogen-mediated uterine epithelial proliferation by progesterone is a prerequisite for successful implantation. HAND2, a progesterone-stimulated gene, is expressed in the uterine stroma during the peri-implantation period and is essential for mouse embryo implantation (39). These results suggest that a Trp-free diet for two months might have adverse effects on uterine receptivity.
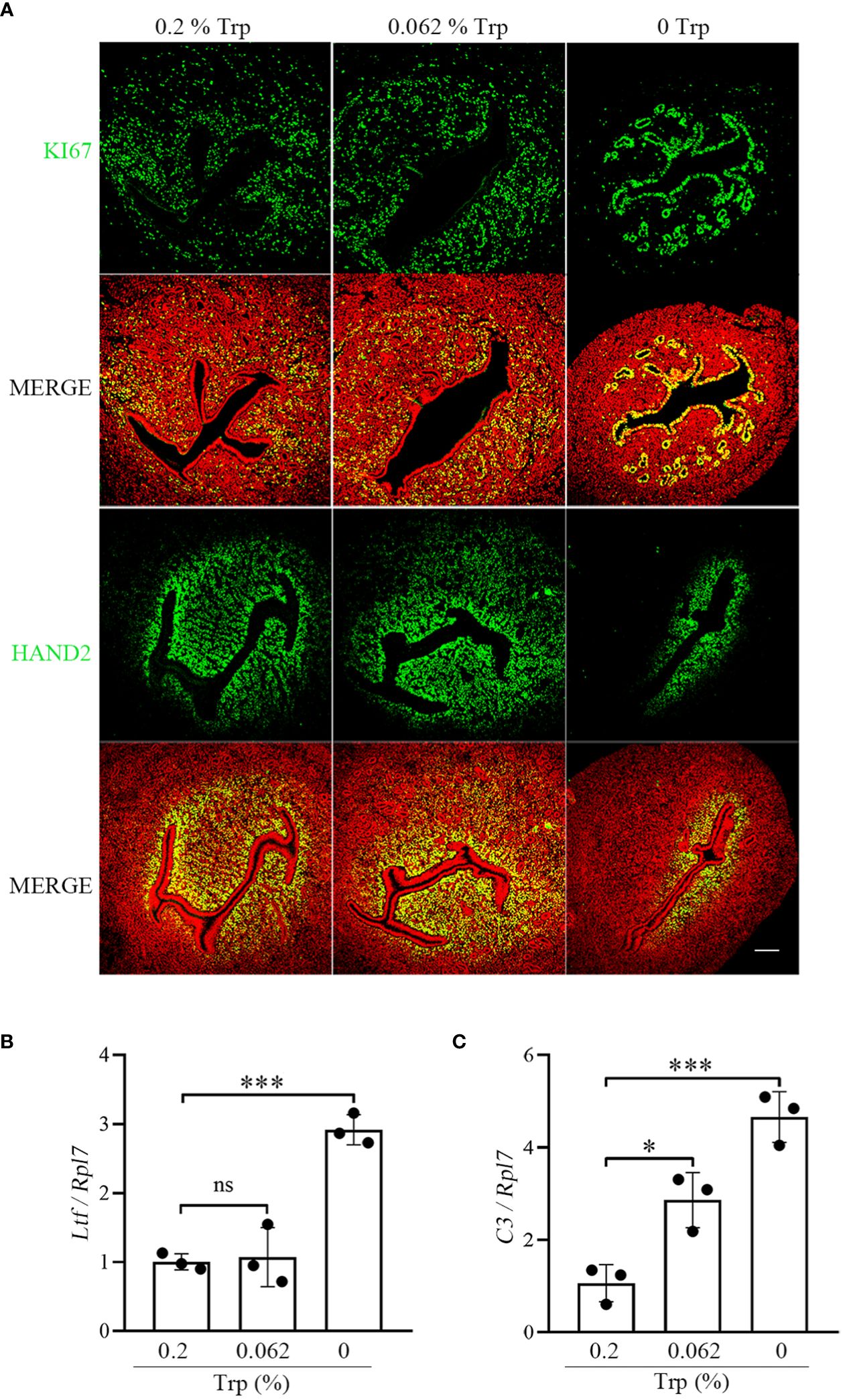
Figure 3 Uterine receptivity on day 4 of pregnancy. (A) Ki67 immunostaining and HAND2 immunofluorescence. * Embryo. Scale bar, 50 μm. (B) qPCR analysis of Ltf mRNA level. (C) qPCR analysis of C3 mRNA level. *, p < 0.05; ***, p < 0.001; ns, not significant.
2.4 The IDO-kynurenine pathway of Trp metabolism at the implantation site
Although Trp is metabolized to Kyn mainly via IDO1 and Tdo2, Trp is also used to synthesize serotonin via the TPH1 enzyme (13). To further explore the reasons for the adverse effects of Trp deficiency on early pregnancy, we examined the key enzymes and their products involved in Trp metabolism. Compared to those in the control and low-Trp groups, there was an increase in IDO1 immunofluorescence and a decrease in TPH1 immunofluorescence in the Trp-free group (Figure 4A). The uterine Kyn concentrations in the Trp-free and low-Trp groups were significantly higher than those in the control group (Figure 4B). However, the uterine serotonin concentration in the Trp-free group was significantly lower than that in the control and low-Trp groups (Figure 4C). These data suggest that Trp is metabolized mainly into Kyn. Under in vitro decidualization, the expression of Prl8a2, a marker of mouse in vitro decidualization (42), was significantly increased, which was significantly inhibited by either 0.5 or 1 mM Kyn (Figure 4D). In addition, treatment of mouse stromal cells with 5-HT under in vitro decidualization conditions revealed that low concentrations of 5-HT had minimal effects on Prl8a2 expression, while higher concentrations of 5-HT suppressed Prl8a2 expression (Figure 4E). Therefore, the high uterine Kyn concentration resulting from strong IDO1 expression impaired mouse decidualization in the Trp-free group.
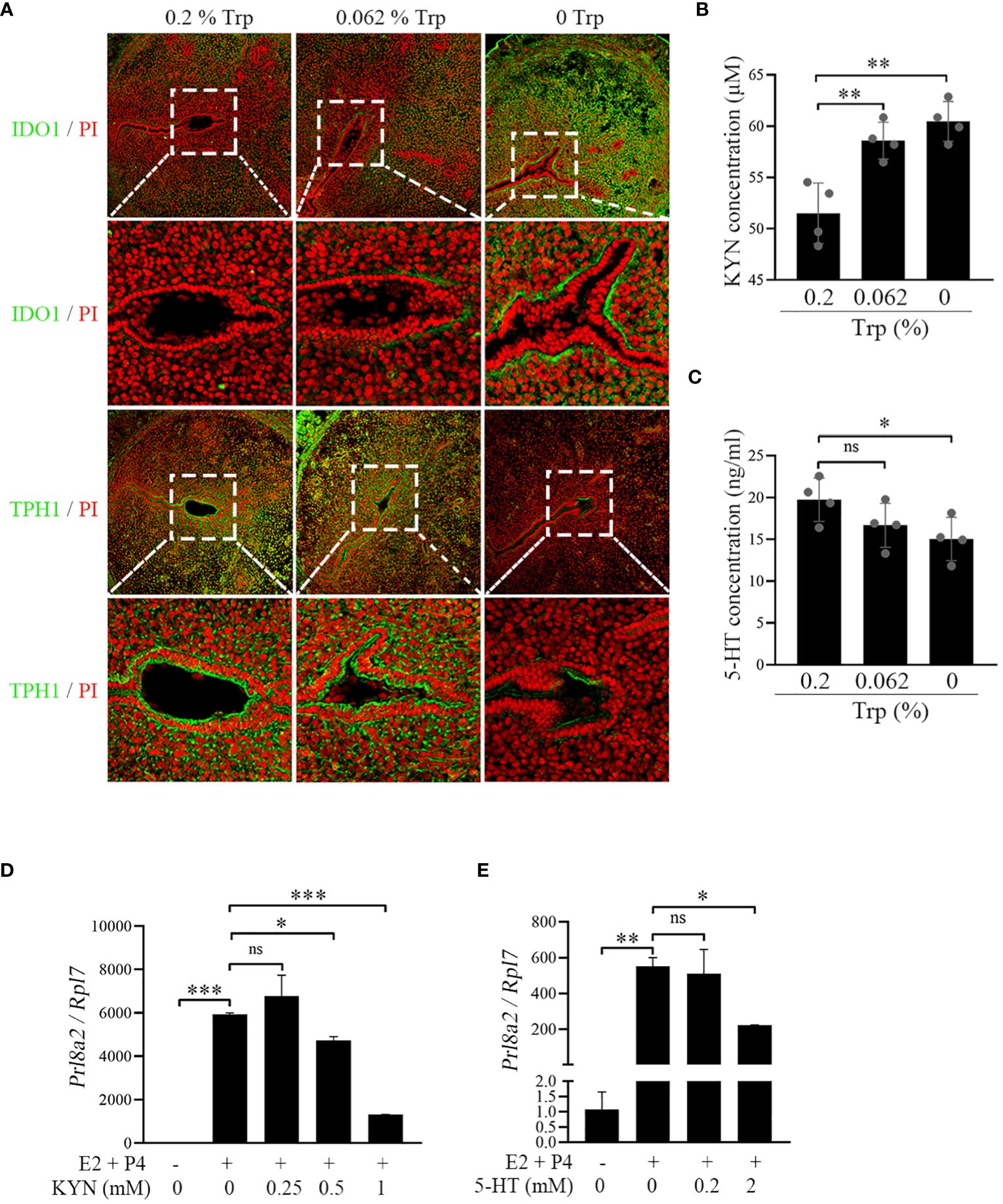
Figure 4 IDO-kynurenine pathway at implantation site on day 5 of pregnancy. (A) Immunofluorescence of IDO1 and TPH1. * Embryo. Scale bar, 50 μm. (B) Uterine Kyn concentration at implantation site. (C) Uterine 5-HT concentration at implantation site. (D) qPCR analysis of Prl8a2 mRNA level after mouse stromal cells were treated with Kyn for 48 h under in vitro decidualization. (E) qPCR analysis of Prl8a2 mRNA level after mouse stromal cells were treated with 5-HT for 48 h under in vitro decidualization. *, p < 0.05; **, p < 0.01; ***, p < 0.001, ns, not significant.
2.5 Kynurenine-induced AhR signaling
Because Trp and Kyn can activate AHR (43) and the AHR target genes CYP1A1 and CYP1B1 (44, 45), the expression of AHR and its target genes was examined. Compared with that in the low-Trp and control groups, AHR immunofluorescence was strongly detected in the subluminal stromal cells at the implantation site in the Trp-free group (Figure 5A). Additionally, the expression of CYP1A1 and CYP1B1 was significantly higher in the Trp-free and low-Trp groups than in the control group (Figures 5B, C). Cytochrome CYP1A1 and CYP1B1 catalyze the oxidative metabolism of oestradiol to produce the catechol oestrogens 2-hydroxy oestradiol (2OE-E2) and 4-hydroxy oestradiol (4OE-E2), respectively (46, 47). Under in vitro decidualization, Prl8a2 expression was significantly increased, which was suppressed by 2OE-E2 (20 μM) and 4OE-E2 (2 and 20 μM) (Figures 5D, E). Treatment of mouse stromal cells with Kyn under in vitro decidualization significantly increased Cyp1a1 and Cyp1b1 expression. CH223191, an AHR inhibitor, abrogated Kyn-induced Cyp1a1 and Cyp1b1 upregulation (Figures 5F, G). Moreover, treatment of mouse stromal cells with Kyn under in vitro decidualization significantly downregulated Prl8a2 expression, while CH223191 could improve the inhibition of decidualization by Kyn (Figure 5H). Taken together, these results suggest that activated AHR and downstream genes contribute to abnormal decidualization in the Trp-free group.
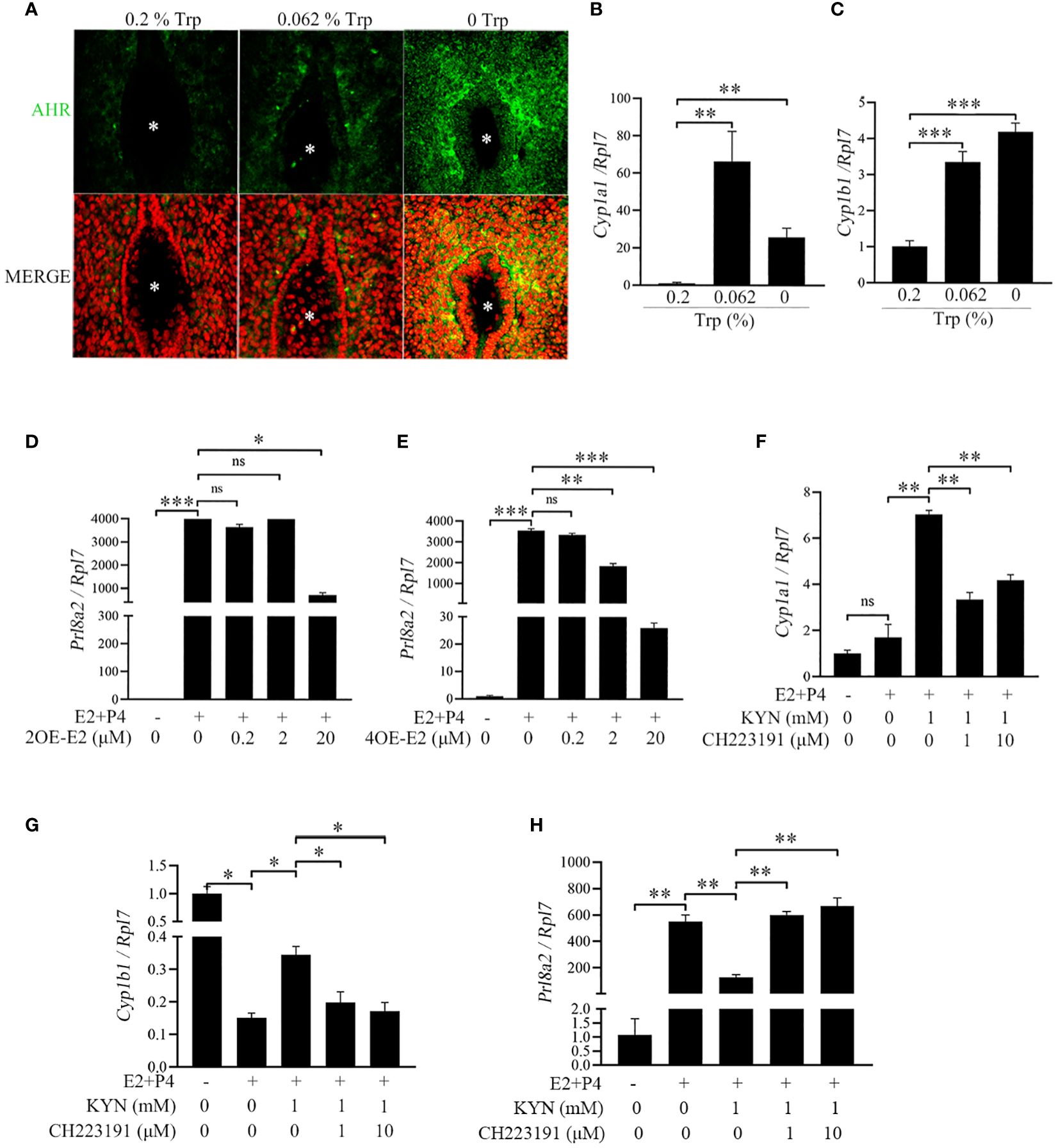
Figure 5 AHR signaling and its effects on mouse decidualization. (A) AhR immunofluorescence at implantation site on day 5 of pregnancy. * Embryo. Scale bar, 50 μm. (B) qPCR analysis of Cyp1a1 mRNA level in mouse uterus at implantation sites on day 5 of pregnancy. (C) qPCR analysis of Cyp1b1 mRNA level in mouse uterus at implantation site on day 5 of pregnancy. (D) qPCR analysis of Prl8a2 mRNA level after mouse stromal cells were treated with 2OE-E2 for 48 h under in vitro decidualization. (E) qPCR analysis of Prl8a2 mRNA level after mouse stromal cells were treated with 4OE-E2 for 48 h under in vitro decidualization. (F) qPCR analysis of Cypla1 mRNA level after mouse stromal cells were treated with CH223191 (AHR inhibitor) for 48 h in the presence or absence of Kyn under in vitro decidualization. (G) qPCR analysis of Cyplb1 mRNA level after mouse stromal cells were treated with CH223191 for 48 h in the presence or absence of Kyn under in vitro decidualization. (H) qPCR analysis of Prl8a2 mRNA level after mouse stromal cells were treated with CH223191 for 48 h in the presence or absence of Kyn under in vitro decidualization. *, p < 0.05; **, p < 0.01; ***, p < 0.001, ns, not significant.
3 Discussion
In our study, mice fed a Trip-free diet for two months had abnormalities in uterine receptivity, the implantation chamber and decidualization. However, a diet in which the Trp content was reduced to 0.062% had little effect on early pregnancy. Trp is an essential amino acid necessary for protein synthesis and can be obtained only from the diet (48, 49). Although Trp deficiency has been extensively investigated in different animal species (50, 51), its effects on embryo implantation and decidualization are still unclear.
In our study, the diet containing 0.062% Trp had no obvious negative effects on uterine receptivity, implantation, or decidualization. A previous study showed that mice fed 0.08% Trp had greater survival and lower growth than did fully fed control mice (29). Symptoms of Trp insufficiency may manifest when the consumption level falls short by a mere 25% of the recommended intake. These symptoms include diminished food consumption, decelerated growth, compromised bone formation and aberrant behavior (52). Trp deficiency leads to an increase in climbing behavior in male rats but has no obvious effect on sexual behavior in female rats (53, 54). Maternal Trp deficiency is associated with adverse offspring outcomes, including decreased body weight and growth retardation (30, 55).
In our study, although implantation sites were detected on day 5 of pregnancy in the Trp-free group, no implantation sites were observed on day 8 of pregnancy. In the Trp-free group, most of the crypts at the implantation site had an abnormal “spear shape”. RBPJ and VANGL2 are two key components of the PCP signaling pathway that are essential for crypt formation (35). In our study, the levels of RBPJ and VANGL2 were obviously lower in the Trp-free group than in the control group. Because defects in crypt shape and size have adverse effects throughout pregnancy (36), abnormalities in PCP signaling may result in pregnancy failure.
Our results may indicate that early pregnancy in mice fed a Trp-free diet may be affected through the IDO-Kyn pathway. IDO is the major rate-limiting enzyme for metabolizing Trp to Kyn (56). In our study, IDO1 protein levels increased at the uterine implantation sites in mice fed a Trp-free diet and Kyn concentrations also increased significantly compared to control mice. During normal pregnancy, IDO1 expression was highest on day 4 of pregnancy and decreased on days 5-8 of pregnancy. IDO1 overexpression significantly decreased the expression of the decidualization marker Prl8a2 (57). In a human study, Kyn promoted decidualization of human endometrial stromal cells (58). However, mouse in vitro decidualization was significantly decreased by Kyn, which is opposite to what occurs during human decidualization. This difference may be attributed to differences in species and Kyn concentrations. Changes in kynurenine pathway metabolite concentrations may play a role in the pathophysiology of pregnancy complications (59). TPH1 is the first and rate-limiting step in serotonin biosynthesis (60). TPH1 protein levels were significantly reduced in mice fed a Trp-free diet than that in the control group, and the concentration of serotonin was also significantly lower in mice fed a Trp-free diet than that in the control group. Inducing serotonergic dysfunction in rats using a Trp-free diet is a quick, painless, efficient, and very precise way to reduce serum serotonin concentration (61). Moreover, Tph1-deficient female mice have abnormal central nervous system development (62). Adequate serotonin is essential for fetal development and contraction of the myometrium (63, 64).
Kyn may control decidualization via AHR. CYP1A1 and CYP1B1, two AhR target genes, catalyze the oxidative metabolism of oestradiol to produce 2OE-E2 and 4OE-E2, respectively. Both 2OE-E2 and 4OE-E2 can promote human in vitro decidualization (57). However, in our study, mouse in vitro decidualization was inhibited by either 2OE-E2 or 4OE-E2. Kyn, an endogenous agonist of AHR, controls several immunological and physiological processes (58). AHR plays an important role as a sensor of environmental pollutants and is closely related to multiple signaling pathways involved in early development and pathogenesis (65). AHR mediates the effects of many environmental endocrine disruptors and leads to the loss of normal ovarian function in polluted environments (66). Cyp1a1 and Cyp1b1 catalyze functional reactions in biological metabolism and are of toxicological importance (22). The AHR signaling cascade is involved in oestrogen regulation in the female reproductive system (67). On day 4 of pregnancy, Cyp1b1 mRNA was strongly expressed in subluminal stromal cells and weakly expressed in epithelial cells. Oestrogen may induce its own degradation of toxic 4OH E2 by increasing the expression of CYP1B1 in the uterus. Delayed implantation in mice can be initiated by 4OH E2 (68, 69). In conclusion, a diet devoid of Trp may impair decidualization via the IDO-KYN-AHR pathway.
4 Conclusion
In our study, a low-Trp diet (0.062% Trp) had no obvious impact on implantation or decidualization. However, a complete lack of Trp led to abnormal decidualization via the IDO-KYN-AHR pathway in mice.
5 Materials and methods
5.1 Animals and diet
Female CD-1 mice (6 weeks old) were bought from Hunan Slaike Jingda Laboratory Animal Co., Ltd. (Chaina, Hunan) and kept in a temperature controlled environment with a 12 h light photoperiod. The animals were given free food and water. All animal protocols were approved by the Animal Care and Use Committee of South China Agricultural University (No. 2021f085). Female mice were co-caged with fertile or vasectomized males to induce pregnancy or pseudopregnancy. The day when vaginal plug was observed was defined as day 1 of pregnancy. From days 1 to 4, pregnancy was confirmed by collecting embryos from oviducts or uterus. Implantation sites on days 5 and 6 of pregnancy were visualized by intravenous injection of 0.1 ml of 1% Chicago blue dye (Sigma-Aldrich). Artificial decidualization was induced by intraluminal injection of 10 μl of sesame oil (Sigma-Aldrich). The uninjected contralateral uterine horn served as control.
According to the nutrient requirements of laboratory animals, the Trp content in the maintenance feed for mice is 0.1%, and the Trp content in the breeding feed is 0.2% (70). In a previous study, Trp amount in a Trp-limited diet is 0.062% (52). Because we mainly studied the effects of Trp content on early pregnancy, female mice were fed different diets containing 0.2% Trp (for control group, N=35 mice), 0.062% Trp (for low-Trp group, N=35 mice) and 0% Trp (for Trp-free group, N=35 mice), respectively. Mouse diets containing different concentrations of Trp were purchased from Jiangsu Xietong Pharmaceutical Bio-engineering Co., Ltd. (Jiangsu, China),. All proteins in the mouse diets were replaced with amino acid premixes in the process of making feed to ensure that the Trp content in the food was 0.2%, 0.062% and 0 of the total feed (see Table 1 for specific feed formulations). In vivo experiments with dietary for Trp addition or deficiency are generally a long-term process for 12 weeks, 8 weeks, or 70 days in previous studies (71–74). Therefore, we first fed female mice with different amounts of Trp for 2 months and then performed different treatments. Pregnant or pseudopregnant mice were also fed with the diets containing different concentrations of Trp until they were sacrificed by cervical dislocation. Female body weights were comparable in three groups at the beginning of feeding. The uteri and serum were collected on days 4, 5 and 8 of pregnancy for further analysis.
5.2 Alkaline phosphatase staining
Alkaline phosphatase (ALP) staining was performed as previously described (75). Frozen sections (10 μm) of mouse uteri were quickly fixed for 10 min in 4% paraformaldehyde (158127, Sigma Aldrich, St. Louis, MO). After three washes in PBS, sections were incubated with nitro blue tetrazolium (0885, Life Science) and 5-bromo-4-chloro-3-indolyl phosphate (0329, Life Science, St. Louis, MO) in PBS solution for 10 min to show alkaline phosphatase activity. The staining density was examined under a microscope. Each experiment was repeated at least three times.
5.3 Immunofluorescence
The immunofluorescence method was performed as previously described (76, 77). Frozen sections (10 μm) were fixed with 4% paraformaldehyde (158127, Sigma Aldrich) for 10 min. Paraffin sections (5 μm) were dewaxed, hydrated and antigen-retrieved by boiling in 10 mM citric acid buffer for 10 min. After permeabilized with 0.1% Triton 100 and blocked with 10% horse serum at 37° C for 1 h, sections were incubated with appropriate dilutions of primary antibodies overnight at 4° C. Primary antibodies used in this study included anti-KI67 (1:200, GB111141, Exilon, Guangzhou), anti-RBPJ (1:200, 14613, Proteintech, Wuhan, China), anti-VANGL2 (1:200, 21492, Proteintech), anti-HAND2 (1:200, sc-9409, Santa Cruz Biotechnogoly, Santa Cruz, USA), anti-IDO1 (1:200, 66528, Proteintech), anti-TPH1 (1:100, CSB-PA024100LA01HU, Cusabio, Wuhan, China). After washing with PBS, sections were incubated with 488-conjugated secondary antibodies (2.5 μg/ml, G21234, Invitrogen, Carlsbad, CA) for 40 min, counterstained with propidium iodide (5 μg/ml, PI, P4170, Sigma-Aldrich) and mounted with Extended™ Diamond Antifade Mountant (Thermo Fisher, Waltham, MA). Images were taken with a laser scanning confocal microscope (Leica, Germany). Each experiment was repeated at least three times.
5.4 Kynurenine assay
Kyn concentration was determined as previously described (78). In short, after the mouse uterus was collected at implantation sites on day 5 of pregnancy, the endometrium was isolated from the myometrium and homogenized in sample diluent. After centrifugated at 5000×g for 10 min, a total of 360 μl supernatant was mixed with 180 μl of 30% trichloroacetic acid (TCA) (T6399, Sigma) and incubated at 50° C for 30 min. After centrifugation at 3000×g for 10 min, the supernatant was mixed with equal volume of current Ehrlich reagent (2% p-dimethylaminobenzaldehyde, D109644, Aladdin, Shanghai, China) dissolved in glacial acetic acid) thoroughly, and incubated at room temperature for 12-30 min. Absorption was measured at 492 nm and compared with the standard curve of L-Kyn (HY-104026, MedChemExpress, NJ, USA). Each experiment was repeated at least three times.
5.5 ELISA assay
After removing myometrium, the endometrium at implantation sites on day 5 of pregnancy was homogenized for serotonin measurement according to the manufacturer’s instructions (Elabscience, E-EL-0033c, Wuhan, China). This kit’s sensitivity is greater than 9.38 ng/ml. The standard working solution or samples of 50 μL were mixed with 50 μL biotinylated antibody in working solution and incubated at 37° C for 45 min. Following washing 3 times, 100 μL HRP enzyme conjugate working solution was added to each well and incubated at 37° C for 30 min. After washing 5 times, 90 μL of substrate solution was added to each well and incubated at 37° C for 15 min. Absorbance at 450 nm wavelength was measured following adding 50 μL of termination solution to each well. Each experiment was repeated at least three times.
5.6 Isolation and treatment of mouse endometrial stromal cells
Mouse endometrial stromal cells were isolated as previously mentioned (77). Mouse uteri on day 4 of pseudopregnancy were longitudinally cut, washed in HBSS, and incubated with 1% (W/V) trypsin and 6 mg/ml dispase in 3.5 mL HBSS for 1 h at 4°C, 1 h at room temperature, and 10 min at 37°C. The uterine tissues were washed with Hanks’ balanced salt solution and incubated with 6 ml of HBSS with 0.15 mg/ml Collagenase I (Invitrogen, 17100-017) at 37°C for 35 min. Primary endometrial stromal cells were cultured in DMEM/F12 with 10% FBS.
Mouse endometrial stromal cells were induced for in vitro decidualization as previously described (79). Endometrial stromal cells were treated with 10 nM of estradiol-17 β and 1 μM of P4 in DMEM/F12 containing 2% charcoal-treated FBS (cFBS, Biological Industries) to induce decidualization in vitro for 72 h. Stromal cells were treated with L- Kyn (0.25, 0.5 and 1 mM, HY-104026, MedChemExpress), 4-Hydroxyestradiol (0.2, 2 or 20 mM, GC19552, Glpbio, Shanghai, China), 2-Hydroxyestradiol (0.2, 2 or 20 mM, GC13460, Glpbio) in DMEM/F12 containing 2% cFBS, respectively. Each experiment was repeated at least three times.
5.7 Real-time RT-PCR
RT-qPCR was performed as previously described (80). Total RNAs were digested with RQ1 deoxyribonuclease I (Promega, Fitchburg, WI) to remove DNA, and reverse-transcribed into cDNA with Prime Script Reverse Transcriptase Reagent Kit (Takara, Japan). Real-time PCR was performed using a SYBR Premix Ex Taq Kit (TaKaRa) on the CFX96 TouchTM Real-Time System (Bio-Rad) The 2-△△Ct method was used to calculate the data, which were standardized to the RPL7 (mouse) or RPL7 (human) level. The primer sequences for each gene were provided in Table 2. Each experiment was repeated at least three times.
5.8 Statistical analysis
Data were processed using GraphPad Prism 8.0. The student’s t test was employed to compare the difference between the two groups. The ANOVA test was utilized to compare the difference of more than two groups. At least three independent replications of each experiment were conducted. Each group in the mouse study contained a minimum of three mice. Standard deviation (SD) and mean were used to present the data. A result with a p-value of 0.05 was deemed significant.
Data availability statement
The original contributions presented in the study are included in the article/supplementary material. Further inquiries can be directed to the corresponding author.
Ethics statement
The animal study was approved by Animal Care and Use Committee of South China Agricultural University. The study was conducted in accordance with the local legislation and institutional requirements.
Author contributions
STC: Writing – review & editing, Data curation, Investigation, Validation, Writing – original draft. FR: Investigation, Writing – review & editing. WWS: Investigation, Writing – review & editing. CKL: Investigation, Writing – review & editing. PCW: Investigation, Writing – review & editing. HNL: Investigation, Writing – review & editing. ZMY: Writing – review & editing, Conceptualization, Funding acquisition, Project administration, Supervision.
Funding
The author(s) declare financial support was received for the research, authorship, and/or publication of this article. This study was supported by National Natural Science Foundation of China (32171114 and 31871511).
Conflict of interest
The authors declare that the research was conducted in the absence of any commercial or financial relationships that could be construed as a potential conflict of interest.
Publisher’s note
All claims expressed in this article are solely those of the authors and do not necessarily represent those of their affiliated organizations, or those of the publisher, the editors and the reviewers. Any product that may be evaluated in this article, or claim that may be made by its manufacturer, is not guaranteed or endorsed by the publisher.
Abbreviations
Trp, Tryptophan; Kyn, Kynurenine; IDO1, Indoleamine-2, 3-dioxygenase 1; TPH1, Tryptophan hydroxylase 1; 5-HT, 5-hydroxytryptamine; AHR, Aryl hydrocarbon receptor; 2OE E2, 2-hydroxy estradiol; 4OE E2, 4-hydroxy estradiol; VANGL2, Van Gogh-Like Protein 2; RBPJ, Rbpsuh.
References
1. Gellersen B, Brosens JJ. Cyclic decidualization of the human endometrium in reproductive health and failure. Endocrine Rev. (2014) 35:851–905. doi: 10.1210/er.2014-1045
2. Tan Y, Li M, Cox S, Davis MK, Tawfik O, Paria BC, et al. HB-EGF directs stromal cell polyploidy and decidualization via cyclin D3 during implantation. Dev Biol. (2004) 265:181–95. doi: 10.1016/j.ydbio.2003.09.019
3. Hussain T, Tan B, Murtaza G, Metwally E, Yang H, Kalhoro MS, et al. Role of dietary amino acids and nutrient sensing system in pregnancy associated disorders. Front Pharmacol. (2020) 11:586979. doi: 10.3389/fphar.2020.586979
4. Cleal JK, Lofthouse EM, Sengers BG, Lewis RM. A systems perspective on placental amino acid transport. J Physiol. (2018) 596:5511–22. doi: 10.1113/JP274883
5. Sainio EL, Pulkki K, Young SN. L-Tryptophan: Biochemical, nutritional, and pharmacological aspects. Amino Acids. (1996) 10:21–47. doi: 10.1007/BF00806091
7. Goldberger J, Wheeler GA. Experimental pellagra in the human subject brought about by a restricted diet. Nutrition. (1990) 6:357–62. doi: 10.2307/4572984
8. Patel AB, Prabhu AS. Hartnup disease. Indian J Dermatol. (2008) 53:31–2. doi: 10.4103/0019-5154.39740
9. Beeken WL. Serum tryptophan in Crohn's disease. Scandinavian J Gastroenterol. (1976) 11:735–40. doi: 10.1080/00365521.1976.12097180
10. Zhu X, Gong W, Li W, Zhang C. Fixating lead in coal gangue with phosphate using phosphate-dissolving bacteria: Phosphorus dissolving characteristics of bacteria and adsorption mechanism of extracellular polymer. J Hazardous Materials. (2023) 458:131923. doi: 10.1016/j.jhazmat.2023.131923
11. Kim S, Li H, Jin Y, Armad J, Gu H, Mani S, et al. Maternal PBDE exposure disrupts gut microbiome and promotes hepatic proinflammatory signaling in humanized PXR-transgenic mouse offspring over time. Toxicological Sciences: an Off J Soc Toxicol. (2023) 194:209–25. doi: 10.1093/toxsci/kfad056
12. Liang D, Li Z, Vlaanderen J, Tang Z, Jones DP, Vermeulen R, et al. A state-of-the-science review on high-resolution metabolomics application in air pollution health research: current progress, analytical challenges, and recommendations for future direction. Environ Health Perspectives. (2023) 131:56002. doi: 10.1289/EHP11851
13. Badawy AA. Tryptophan metabolism, disposition and utilization in pregnancy. Bioscience Rep. (2015) 35:e00261. doi: 10.1042/BSR20150197
14. Badawy AA, Namboodiri AM, Moffett JR. The end of the road for the tryptophan depletion concept in pregnancy and infection. Clin Sci. (2016) 130:1327–33. doi: 10.1042/CS20160153
15. Xue C, Li G, Zheng Q, Gu X, Shi Q, Su Y, et al. Tryptophan metabolism in health and disease. Cell Metab. (2023) 35:1304–26. doi: 10.1016/j.cmet.2023.06.004
16. Hoang VH, Trang NTK, Minh TC, Long LTB, Lan TH, Hue NT, et al. Design, synthesis, and evaluation the bioactivities of novel 1,3-dimethyl-6-amino-1H-indazole derivatives as anticancer agents. Bioorganic Medicinal Chem. (2023) 90:117377. doi: 10.1016/j.bmc.2023.117377
17. Munn DH, Zhou M, Attwood JT, Bondarev I, Conway SJ, Marshall B, et al. Prevention of allogeneic fetal rejection by tryptophan catabolism. Science. (1998) 281:1191–3. doi: 10.1126/science.281.5380.1191
18. Li DD, Gao YJ, Tian XC, Yang ZQ, Cao H, Zhang QL, et al. Differential expression and regulation of Tdo2 during mouse decidualization. J Endocrinol. (2014) 220:73–83. doi: 10.1530/JOE-13-0429
19. Correia AS, Vale N. Tryptophan metabolism in depression: A narrative review with a focus on serotonin and kynurenine pathways. Int J Mol Sci. (2022) 23:8493. doi: 10.3390/ijms23158493
20. Laurent L, Deroy K, St-Pierre J, Côté F, Sanderson JT, Vaillancourt C. Human placenta expresses both peripheral and neuronal isoform of tryptophan hydroxylase. Biochimie. (2017) 140:159–65. doi: 10.1016/j.biochi.2017.07.008
21. Schmidt JV, Bradfield CA. Ah receptor signaling pathways. Annu Rev Cell Dev Biol. (1996) 12:55–89. doi: 10.1146/annurev.cellbio.12.1.55
22. Hankinson O. The aryl hydrocarbon receptor complex. Annu Rev Pharmacol Toxicol. (1995) 35:307–40. doi: 10.1146/annurev.pa.35.040195.001515
23. Grifka-Walk HM, Jenkins BR, Kominsky DJ. Amino Acid Trp: The far out impacts of host and commensal tryptophan metabolism. Front Immunol. (2021) 12:653208. doi: 10.3389/fimmu.2021.653208
24. Abbott BD, Schmid JE, Pitt JA, Buckalew AR, Wood CR, Held GA, et al. Adverse reproductive outcomes in the transgenic Ah receptor-deficient mouse. Toxicologyand Appl Pharmacol. (1999) 155:62–70. doi: 10.1006/taap.1998.8601
25. Khorram O, Garthwaite M, Golos T. Uterine and ovarian aryl hydrocarbon receptor (AHR) and aryl hydrocarbon receptor nuclear translocator (ARNT) mRNA expression in benign and Malignant gynaecological conditions. Mol Hum Reproduction. (2002) 8:75–80. doi: 10.1093/molehr/8.1.75
26. Tsuji A, Nakata C, Sano M, Fukuwatari T, Shibata K. L-Tryptophan metabolism in pregnant mice fed a high L-tryptophan diet and the effect on maternal, placental, and fetal growth. Int J Tryptophan Res. (2013) 6:21–33. doi: 10.4137/IJTR.S12715
27. Matsueda S, Niiyama Y. The effects of excess amino acids on maintenance of pregnancy and fetal growth in rats. J Nutr Sci Vitaminol. (1982) 28:557–73. doi: 10.3177/jnsv.28.557
28. Segall P. Long-term tryptophan restriction and aging in the rat. Aktuelle Gerontologie. (1977) 7:535–8.
29. Segall PE, Timiras PS. Patho-physiologic findings after chronic tryptophan deficiency in rats: a model for delayed growth and aging. Mech Ageing Dev. (1976) 5:109–24. doi: 10.1016/0047-6374(76)90012-9
30. Penatti EM, Barina AE, Raju S, Li A, Kinney HC, Commons KG, et al. Maternal dietary tryptophan deficiency alters cardiorespiratory control in rat pups. J Appl Physiol. (2011) 110:318–28. doi: 10.1152/japplphysiol.00788.2010
31. Orwig KE, Soares, M. J. Transcriptional activation of the decidual/trophoblast prolactin-related protein gene. Endocrinology. (1999) 140:4032–9. doi: 10.1210/en.140.9.4032
32. Yee GM, Kennedy TG. Stimulatory effects of prostaglandins upon endometrial alkaline phosphatase activity during the decidual cell reaction in the rat. Biol Reproduction. (1988) 38:1129–36. doi: 10.1095/biolreprod38.5.1129
33. Cha J, Sun X, Dey SK. Mechanisms of implantation: strategies for successful pregnancy. Nat Med. (2012) 18:1754–67. doi: 10.1038/nm.3012
34. Cha J, Bartos A, Park C, Sun X, Li Y, Cha SW, et al. Appropriate crypt formation in the uterus for embryo homing and implantation requires Wnt5a-ROR signaling. Cell Rep. (2014) 8:382–92. doi: 10.1016/j.celrep.2014.06.027
35. Yuan J, Cha J, Deng W, Bartos A, Sun X, Ho HH, et al. Planar cell polarity signaling in the uterus directs appropriate positioning of the crypt for embryo implantation. Proc Natl Acad Sci U S A. (2016) 113:E8079–e8088. doi: 10.1073/pnas.1614946113
36. Yuan J, Deng W, Cha J, Sun X, Borg JP, Dey SK. Tridimensional visualization reveals direct communication between the embryo and glands critical for implantation. Nat Communication. (2018) 9:603. doi: 10.1038/s41467-018-03092-4
37. Zhang S, Kong S, Wang B, Cheng X, Chen Y, Wu W, et al. Uterine Rbpj is required for embryonic-uterine orientation and decidual remodeling via Notch pathway-independent and -dependent mechanisms. Cell Res. (2014) 24:925–42. doi: 10.1038/cr.2014.82
38. Finn CA, Martin L. The role of the oestrogen secreted before oestrus in the preparation of the uterus for implantation in the mouse. J Endocrinol. (1970) 47:431–8. doi: 10.1677/joe.0.0470431
39. Li Q, Kannan A, DeMayo FJ, Lydon JP, Cooke PS, Yamagishi H, et al. The antiproliferative action of progesterone in uterine epithelium is mediated by Hand2. Science. (2011) 331:912–6. doi: 10.1126/science.1197454
40. Griffiths RM, Pru CA, Behura SK, Cronrath AR, McCallum ML, Kelp NC, et al. AMPK is required for uterine receptivity and normal responses to steroid hormones. Reproduction. (2020) 159:707–17. doi: 10.1530/REP-19-0402
41. Sundstrom SA, Komm BS, Ponce-de-Leon H, Yi Z, Teuscher C, Lyttle CR. Estrogen regulation of tissue-specific expression of complement C3. J Biol Chem. (1989) 264:16941–7. doi: 10.1016/S0021-9258(19)84798-1
42. Gu Y, Soares MJ, Srivastava RK, Gibori G. Expression of decidual prolactin-related protein in the rat decidua. Endocrinology. (1994) 135:1422–7. doi: 10.1210/endo.135.4.7925104
43. Mezrich JD, Fechner JH, Zhang X, Johnson BP, Burlingham WJ, Bradfield CA. An interaction between kynurenine and the aryl hydrocarbon receptor can generate regulatory T cells. J Immunol. (2010) 185:3190–8. doi: 10.4049/jimmunol.0903670
44. Spink DC, Eugster HP, Lincoln DW 2nd, Schuetz JD, Schuetz EG, Johnson JA, et al. 17 beta-estradiol hydroxylation catalyzed by human cytochrome P450 1A1: a comparison of the activities induced by 2,3,7,8-tetrachlorodibenzo-p-dioxin in MCF-7 cells with those from heterologous expression of the cDNA. Arch Biochem Biophysics. (1992) 293:342–8. doi: 10.1016/0003-9861(92)90404-K
45. Hayes CL, Spink DC, Spink BC, Cao JQ, Walker NJ, Sutter TR. 17 beta-estradiol hydroxylation catalyzed by human cytochrome P450 1B1. Proc Natl Acad Sci U S A. (1996) 93:9776–81. doi: 10.1073/pnas.93.18.9776
46. Hanna IH, Dawling S, Roodi N, Guengerich FP, Parl FF. Cytochrome P450 1B1 (CYP1B1) pharmacogenetics: association of polymorphisms with functional differences in estrogen hydroxylation activity. Cancer Res. (2000) 60:3440–4.
47. Dawling S, Roodi N, Parl FF. Methoxyestrogens exert feedback inhibition on cytochrome P450 1A1 and 1B1. Cancer Res. (2003) 63:3127–32.
48. Friedman M. Analysis, nutrition, and health benefits of tryptophan. Int J Tryptophan Res. (2018) 11:1–12. doi: 10.1177/1178646918802282
49. Kaluzna-Czaplinska J, Gatarek P, Chirumbolo S, Chartrand MS, Bjorklund G. How important is tryptophan in human health. Crit Rev Food Sci Nutr. (2019) 59:72–88. doi: 10.1080/10408398.2017.1357534
50. Rankin LC, Kaiser KA, de Los Santos-Alexis K, Park H, Uhlemann AC, Gray DHD, et al. Dietary tryptophan deficiency promotes gut RORγt(+) Treg cells at the expense of Gata3(+) Treg cells and alters commensal microbiota metabolism. Cell Rep. (2023) 42:112135. doi: 10.1016/j.celrep.2023.112135
51. Yusufu I, Ding K, Smith K, Wankhade UD, Sahay B, Patterson GT, et al. A tryptophan-deficient diet induces gut microbiota dysbiosis and increases systemic inflammation in aged mice. Int J Mol Sci. (2021) 22:5005. doi: 10.3390/ijms22095005
52. Moehn S, Pencharz PB, Ball RO. Lessons learned regarding symptoms of tryptophan deficiency and excess from animal requirement studies. J Nutr. (2012) 142:2231s–5s. doi: 10.3945/jn.112.159061
53. Carruba MO, Picotti GB, Genovese E, Mantegazza P. Stimulatory effect of a maize diet on sexual behaviour of male rats. Life Sci. (1977) 20:159–64. doi: 10.1016/0024-3205(77)90143-6
54. Benedetti F, Moja EA. Failure of a tryptophan-free amino acid mixture to modify sexual behavior in the female rat. Physiol Behavior. (1993) 54:1235–7. doi: 10.1016/0031-9384(93)90354-I
55. Omstedt PT, von der Decken A. Dietary amino acids: effect of depletion and recovery on protein synthesis in vitro in rat skeletal muscle and liver. Br J Nutr. (1974) 31:67–76. doi: 10.1079/BJN19740009
56. Stone TW, Darlington LG. Endogenous kynurenines as targets for drug discovery and development. Nat Rev Drug Discov. (2002) 1:609–20. doi: 10.1038/nrd870
57. Li DD, Yin YH, Wu JY, Yang ZQ, Cao H, Zhang QL, et al. Effects of Ido1 on mouse decidualization. Mol Biol. (2015) 49:649–57. doi: 10.7868/S002689841503012X
58. Wang PC, Chen ST, Hong ZK, Li SY, Yang ZS, Quan S, et al. Tryptophan and kynurenine stimulate human decidualization via activating Aryl hydrocarbon receptor: Short title: Kynurenine action on human decidualization. Reprod Toxicol. (2020) 96:282–92. doi: 10.1016/j.reprotox.2020.07.011
59. van Zundert SK, Broekhuizen M, Smit AJ, van Rossem L, Mirzaian M, Willemsen SP, et al. The role of the kynurenine pathway in the (Patho) physiology of maternal pregnancy and fetal outcomes: A systematic review. Int J Tryptophan Res. (2022) 15:1–19. doi: 10.1177/11786469221135545
60. Walther DJ, Peter JU, Bashammakh S, Hortnagl H, Voits M, Fink H, et al. Synthesis of serotonin by a second tryptophan hydroxylase isoform. Science. (2003) 29:76. doi: 10.1126/science.1078197
61. Kot M, Daujat-Chavanieu M. The impact of serotonergic system dysfunction on the regulation of P4501A isoforms during liver insufficiency and consequences for thyroid hormone homeostasis. Food Chem Toxicol. (2016) 97:70–81. doi: 10.1016/j.fct.2016.08.027
62. Côté F, Fligny C, Bayard E, Launay JM, Gershon MD, Mallet J, et al. Maternal serotonin is crucial for murine embryonic development. Proc Natl Acad Sci U S A. (2007) 104:329–34. doi: 10.1073/pnas.0606722104
63. Doherty LF, Kwon HE, Taylor HS. Regulation of tryptophan 2,3-dioxygenase by HOXA10 enhances embryo viability through serotonin signaling. Am J Physiol Endocrinol Metab. (2011) 300:E86–93. doi: 10.1152/ajpendo.00439.2010
64. Cordeaux Y, Pasupathy D, Bacon J, Charnock-Jones DS, Smith GC. Characterization of serotonin receptors in pregnant human myometrium. J Pharmacol Exp Ther. (2009) 328:682–91. doi: 10.1124/jpet.108.143040
65. Nebert DW. Aryl hydrocarbon receptor (AHR): "pioneer member" of the basic-helix/loop/helix per-Arnt-sim (bHLH/PAS) family of "sensors" of foreign and endogenous signals. Prog Lipid Res. (2017) 67:38–57. doi: 10.1016/j.plipres.2017.06.001
66. Bidgoli SA, Khorasani H, Keihan H, Sadeghipour A, Mehdizadeh A. Role of endocrine disrupting chemicals in the occurrence of benign uterine leiomyomata: special emphasis on AhR tissue levels. Asian Pacific J Cancer Prev. (2012) 13:5445–50. doi: 10.7314/APJCP.2012.13.11.5445
67. Rataj F, Möller FJ, Jähne M, Hönscheid P, Zierau O, Vollmer G, et al. Progesterone, as well as 17β-estradiol, is important for regulating AHR battery homoeostasis in the rat uterus. Arch Toxicol. (2015) 89:393–404. doi: 10.1007/s00204-014-1261-3
68. Rataj F, Möller FJ, Jähne M, Zierau O, Diel P, Vollmer G, et al. Regulation of uterine AHR battery gene expression by 17β-Estradiol is predominantly mediated by estrogen receptor α. Arch Toxicol. (2012) 86:1603–12. doi: 10.1007/s00204-012-0870-y
69. Paria BC, Lim H, Wang XN, Liehr J, Das SK, Dey SK. Coordination of differential effects of primary estrogen and catecholestrogen on two distinct targets mediates embryo implantation in the mouse. Endocrinology. (1998) 139:5235–46. doi: 10.1210/en.139.12.5235
70. National Research Council (US) Subcommittee on Laboratory Animal Nutrition. Nutrient requirements of laboratory animals: Fourth revised edition. Washington (DC: National Academies Press (US (1995).
71. Chojnacki C, Gąsiorowska A, Popławski T, Błońska A, Konrad P, Zajdler R, et al. Reduced intake of dietary tryptophan improves beneficial action of budesonide in patients with lymphocytic colitis and mood disorders. Nutrients. (2023) 15:1674. doi: 10.3390/nu15071674
72. Hu D, Liu J, Yu W, Li C, Huang L, Mao W, et al. Tryptophan intake, not always the more the better. Front Nutr. (2023) 10:1140054. doi: 10.3389/fnut.2023.1140054
73. Yusuke S, Hideki M, Yoko M, Takumi O, Tsutomu F, Katsumi S, et al. Thirteen week toxicity study of dietary l-tryptophan in rats with a recovery period of 5 weeks. J Appl Toxicol. (2018) 38:552–63. doi: 10.1002/jat.3562
74. Zhang X, Wang A, Chang E, Han B, Xu J, Fu Y, et al. Effects of dietary tryptophan on the antioxidant capacity and immune response associated with TOR and TLRs/MyD88/NF-κB signaling pathways in northern snakehead, Channa argus (Cantor, 1842). Front Immunol. (2023) 14:1149151. doi: 10.3389/fimmu.2023.1149151
75. Fujioka-Kobayashi M, Sawada K, Kobayashi E, Schaller B, Zhang Y, Miron RJ. Osteogenic potential of rhBMP9 combined with a bovine-derived natural bone mineral scaffold compared to rhBMP2. Clin Oral Implants Res. (2017) 28:381–7. doi: 10.1111/clr.12804
76. Li SY, Song Z, Yan YP, Li B, Song MJ, Liu YF, et al. Aldosterone from endometrial glands is benefit for human decidualization. Cell Death Dis. (2020) 11:679. doi: 10.1038/s41419-020-02844-9
77. Zheng HT, Zhang HY, Chen ST, Li MY, Fu T, Yang ZM. The detrimental effects of stress-induced glucocorticoid exposure on mouse uterine receptivity and decidualization. FASEB J. (2020) 34:14200–16. doi: 10.1096/fj.201902911RR
78. Wu X, Xiong E, Wang W, Scali M, Cresti M. Universal sample preparation method integrating trichloroacetic acid/acetone precipitation with phenol extraction for crop proteomic analysis. Nat Protoc. (2014) 9:362–74. doi: 10.1038/nprot.2014.022
79. Liang XH, Deng WB, Li M, Zhao ZA, Wang TS, Feng XH, et al. Egr1 protein acts downstream of estrogen-leukemia inhibitory factor (LIF)-STAT3 pathway and plays a role during implantation through targeting Wnt4. J Biol Chem. (2014) 289:23534–45. doi: 10.1074/jbc.M114.588897
Keywords: tryptophan deficiency, decidualization, uterine receptivity, aryl hydrocarbon receptor, uterus
Citation: Chen ST, Ran F, Shi WW, Liu CK, Wang PC, Luo HN and Yang ZM (2024) Tryptophan in the mouse diet is essential for embryo implantation and decidualization. Front. Endocrinol. 15:1356914. doi: 10.3389/fendo.2024.1356914
Received: 16 December 2023; Accepted: 02 April 2024;
Published: 01 May 2024.
Edited by:
Richard Ivell, University of Nottingham, United KingdomReviewed by:
Enoch Appiah Adu Gyamfi, The State University of New York (SUNY), United StatesXuan Shao, Chinese Academy of Sciences (CAS), China
Copyright © 2024 Chen, Ran, Shi, Liu, Wang, Luo and Yang. This is an open-access article distributed under the terms of the Creative Commons Attribution License (CC BY). The use, distribution or reproduction in other forums is permitted, provided the original author(s) and the copyright owner(s) are credited and that the original publication in this journal is cited, in accordance with accepted academic practice. No use, distribution or reproduction is permitted which does not comply with these terms.
*Correspondence: Zeng-Ming Yang, eWFuZ3ptQGd6dS5lZHUuY24=