- Department of Medical Imaging, The First Affiliated Hospital of Kunming Medical University, Kunming, China
More than 619 million people in the world suffer from low back pain (LBP). As two potential inducers of LBP, intervertebral disc degeneration (IVDD) and fat infiltration of paraspinal muscles (PSMs) have attracted extensive attention in recent years. So far, only one review has been presented to summarize their relationship and relevant mechanisms. Nevertheless, it has several noticeable drawbacks, such as incomplete categorization and discussion, lack of practical proposals, etc. Consequently, this paper aims to systematically summarize and classify the interaction between IVDD and fat infiltration of PSMs, thus providing a one-stop search handbook for future studies. As a result, four mechanisms of IVDD leading to fat infiltration of PSMs and three mechanisms of fat infiltration in PSMs causing IVDD are thoroughly analyzed and summarized. The typical reseaches are tabulated and evaluated from four aspects, i.e., methods, conclusions, benefits, and drawbacks. We find that IVDD and fat infiltration of PSMs is a vicious cycle that can promote the occurrence and development of each other, ultimately leading to LBP and disability. Finally, eight perspectives are proposed for future in-depth research.
1 Introduction
Low back pain (LBP) has been identified as a major global public health problem (1) and the leading cause of the disease burden worldwide (2). According to statistics, up to 2020, approximately 619 million people around the world suffer from LBP (3). Given its prevalence and burden rise with age (4), as well as the increasing population aging and the modern lifestyle changes, LBP should be urgently addressed in the 21st century.
The pathogenesis of LBP is diverse, including intervertebral disc degeneration (IVDD), radicular pain, facet arthropathy, myofascial pain, sacroiliac joint pain, spondyloarthropathies, etc. More details are available in the review by Knezevic et al. (5). Among them, IVDD is the main pathogenic factor of LBP (6, 7). It generally exhibits disc cell apoptosis, annulus fibrosus (AF) tears, and nucleus pulposus (NP) protrusion, structural changes in the cartilaginous endplates (CEP), disc collapse, and metabolic changes (8, 9). These degenerative changes contribute to the loss of normal structure and function of the intervertebral disc (IVD), giving rise to disc-related pain and inflammation, along with decreased spinal stability (10, 11). Additionally, during disc degeneration, structure remodeling of paraspinal muscles (PSMs) usually occurs, e.g., fat infiltration, muscle asymmetry, and size reduction (12). Notably, fat infiltration of PSMs increases with the progression of IVDD and is actively associated with the severity and dysfunction of LBP (13, 14).
In fact, there is a close interaction between fat infiltration in PSMs and IVDD (12, 15). On the one hand, IVDD can induce fatty infiltration of PSMs by affecting their loading (16) and innervation (17, 18), as well as releasing inflammatory mediators (19, 20). On the other hand, fat-infiltrated PSMs can promote IVDD via increasing mechanical stress on the disc (21) and releasing inflammatory (22) and adipokine factors (23).
In recent years, a growing number of studies have reported the interaction between IVDD and fat infiltration of PSMs. To further understand the mechanism of their interaction, relevant literature from 2014 to Apr. 2024 is investigated and counted based on the search keywords of ‘intervertebral disc degeneration, paraspinal muscles, and fatty infiltration’. The specific search process and results are shown in Figure 1. From Figure 1B, it can be seen that the research on their interaction is generally at a relatively high level, especially in the recent three years.
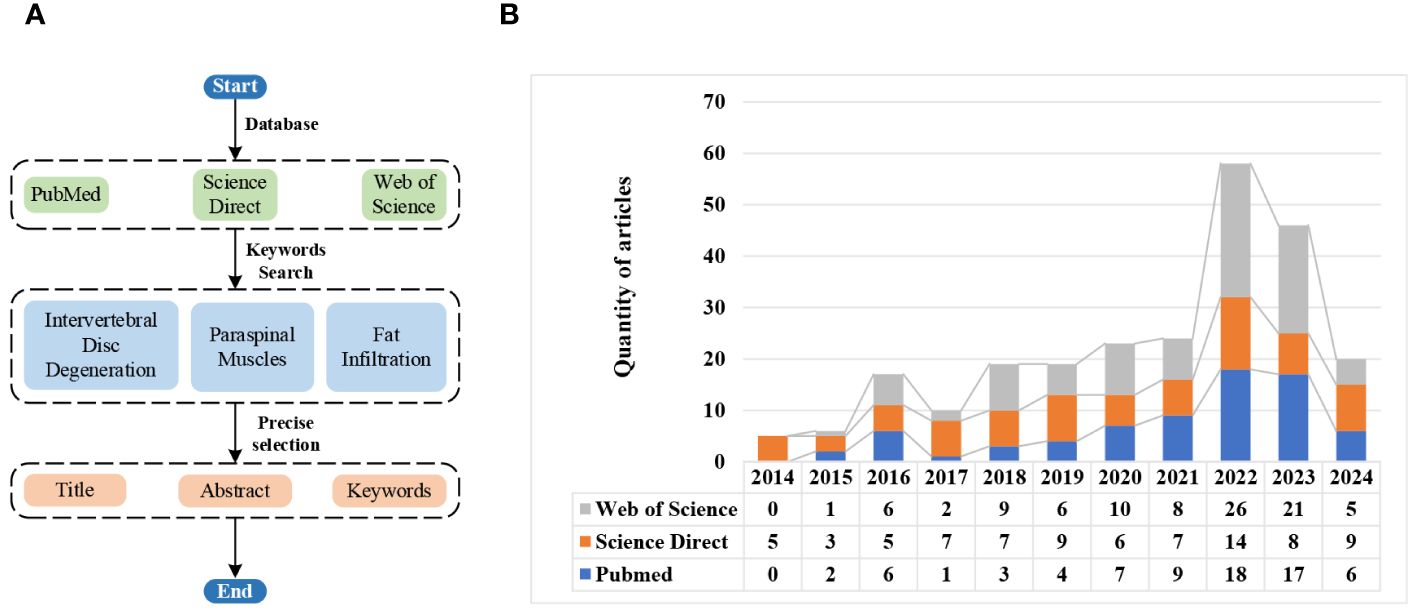
Figure 1 Literature search flowchart and results via different databases. (A) Search flowchart. (B) Search results.
Until now, only Wang et al. (24) have published a review on the relationship between IVDD and fat infiltration of PSMs, which has the following shortcomings: (i) Incomplete investigation and analysis on the interaction between IVDD and fat infiltration of PSMs; (ii) Lack of detailed summaries and perspectives. In order to overcome the above shortcomings, this paper offers a comprehensive and in-depth investigation of the interaction between IVDD and fat infiltration of PSMs, aiming to offer a state-of-the-art, one-stop manual for present and further research. Then, the screening process for the review is depicted in Figure 2. Inclusion criteria encompassed studies in English focusing on the IVDD and PSMs relationship, with excluded material such as reviews, case reports, and conference abstracts. Finally, a total of 72 papers were included in the investigation. Its main contributions are summarized as follows:
● Four mechanisms of IVDD involved in the fatty infiltration of PSMs and three mechanisms of fatty infiltration of PSMs engaged in IVDD are systematically classified and summarized, upon which the related studies are systematically tabulated and evaluated from four aspects, i.e., methods, conclusions, benefits, and drawbacks.
● In the part of summarizations and discussions, five key findings are put forward from three aspects: research content, methods and results, and their reasons are analyzed.
● Eight perspectives in four main aspects (i.e., promising research, experimental methods, models and samples) are proposed for future research.
The rest of this article is organized as follows: Section 2 summarizes four mechanisms of IVDD involved in fat infiltration of PSMs. Three mechanisms of fat infiltration of PSMs participated in IVDD are concluded in Section 3. Section 4 gives comprehensive summarizations and discussions of some findings. In Section 5, four conclusions are presented. Finally, Section 6 offers several proposals and perspectives for further research.
2 Mechanisms of IVDD involved in fat infiltration of PSMs
There are four main mechanisms of IVDD causing fatty infiltration of PSMs, particularly the multifidus muscle (MM): inflammatory response, muscle denervation, muscle chronic disuse, and biomechanical imbalance.
2.1 Inflammatory response
Both human and animal studies have consistently demonstrated that dysregulation of local inflammatory activity is a significant contributing factor to the fatty infiltration of PSMs associated with IVDD (12, 15, 19, 20, 25). The summarizations of recent studies exploring this mechanism are presented in Table 1. Inflammatory factors play a crucial role in the occurrence and development of IVDD (26, 27). Specifically, tumor necrosis factor-α (TNF-α)and interleukin-1beta (IL-1β), as the most pivotal inflammatory cytokines, exhibit strong pro-inflammatory activity and can stimulate the release of various proinflammatory mediators. Their upregulated expression in degenerative IVD is closely linked to multiple pathological processes of IVDD (28).
IL-1β mediates the production of reactive oxygen species (ROS), and the excessive accumulation of ROS can cause oxidative stress (28). Increased production of ROS is associated with the differentiation of preadipocytes to adipocytes and the accumulation of adipose tissue (29). Persistent oxidative stress can lead to muscle damage, significantly affecting the contractility of skeletal muscle and inducing fibrotic degeneration as well as muscle fatty changes (30). TNF-α is a key player in muscle atrophy, which can induce the upregulation of inflammatory cytokine gene expression and activate the nuclear factor-kappa B (NF-κB) pathway in muscle atrophy (31). Furthermore, TNF-α is related to the disorder of lipid metabolism, and it is potentially an important factor in promoting white adipogenesis in skeletal muscle (22, 28, 32). Interestingly, the relationship between adiposity and pro-inflammatory cytokines is two-way; TNF-α is produced not only by adipose tissue but also regulates adipogenesis (19, 20). In animal models of IVDD or injury, despite no muscle damage, higher expression of proinflammatory cytokine genes (e.g., TNF and IL-1β) and fat infiltration were observed in MM (25, 33–35). The mechanism of fat infiltration in MM is related to the local inflammatory response induced by TNF, which originates from the degenerated disc rather than the PSMs (12). In addition, TNF expression in MM after IVDD is mediated by M1 macrophages in adipose tissue (25).
Fibroblasts, preadipocytes, and satellite cells are existent in the perimuscular connective tissue. These cells can transdifferentiate into adipose tissue under the stimulation of pro-inflammatory cytokines, ultimately leading to adipocyte proliferation (30). A large number of fibro-adipogenic progenitors (FAPs) and satellite cells (SCs) were found in the MM of patients with lumbar disc herniation (LDH) (36). FAPs are an inherent muscle stem cell population that differentiate into adipocytes and fibroblasts and contribute significantly to muscle fat infiltration and fibrosis (37, 38). FAPs appear as a brown/beige fat (BAT) phenotype, whereas white adipose tissue makes up the majority of muscle fat infiltration (39, 40). BAT has demonstrated positive effects on myogenesis (41). Therefore, a potential avenue for mitigating fat infiltration (FI) and muscle atrophy in the future involves directing FAPs toward a BAT phenotype.
Figure 3 shows the current lineage of research on inflammatory response.
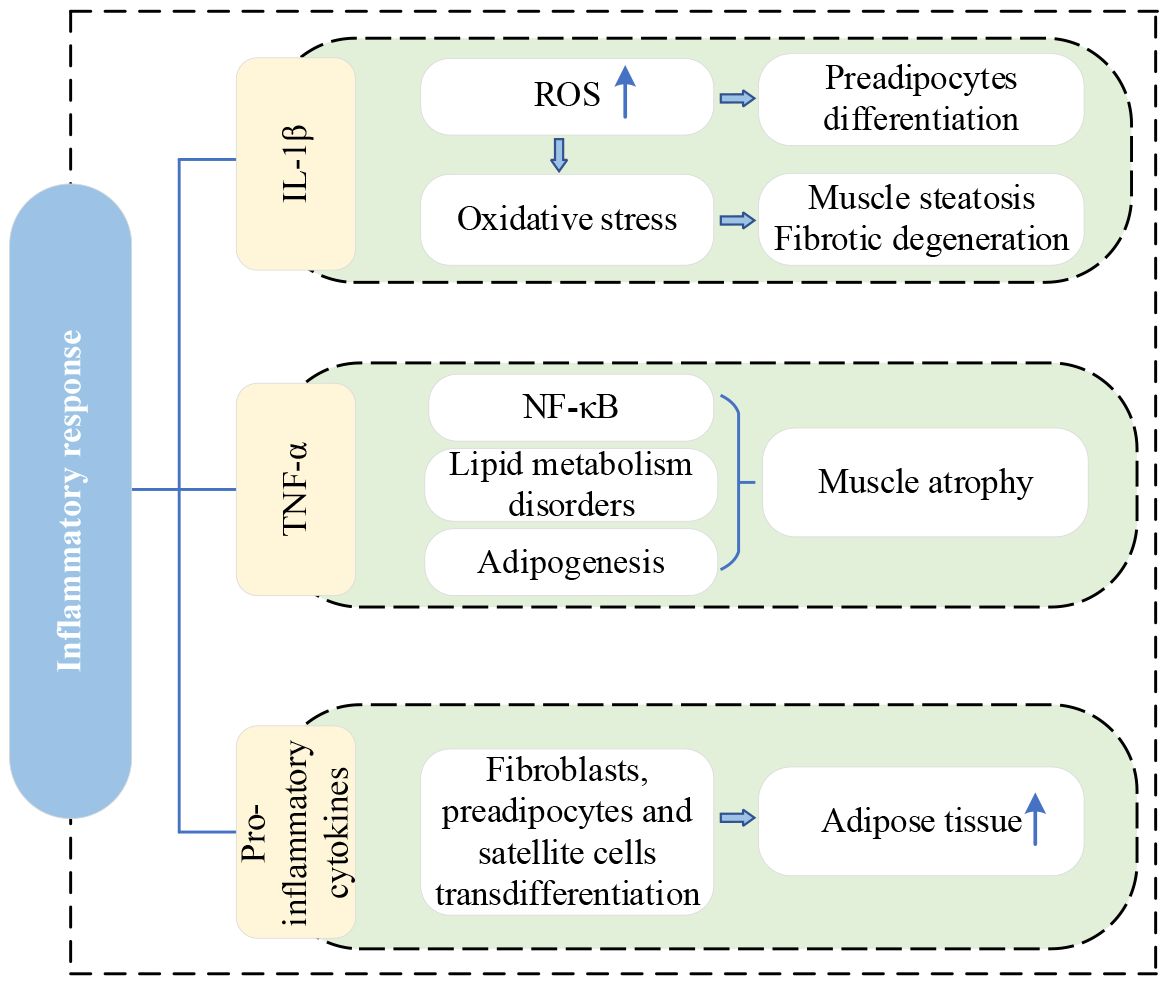
Figure 3 Research lineage of inflammatory response. IL-1β, interleukin-1beta; ROS, reactive oxygen species; TNF-α, tumor necrosis factor-α; NF-κB, nuclear factor-kappa B.
2.2 Muscle denervation
IVDD is a complex process involving molecular and structural alterations, which causes the compression of both the dorsal root ganglia and the nerve root (42), with atrophy and fat infiltration in the PSMs they innervate (17, 18, 43, 44). Recent studies on this mechanism are summarized in Table 2. Muscle denervation usually undergoes three stages: 1) immediate loss of function, followed by rapid weight loss and muscle fiber atrophy, 2) more severe muscle atrophy, including loss of most sarcomeric tissue, and 3) interstitial fibrosis and fat deposition (45). Additionally, denervated muscles experience alterations in glucose metabolism and protein hydrolysis, which are associated with reduced muscle fiber diameter and increased fat content. A review of the MRI appearance of muscle denervation by Kamath et al. reveals that progressive muscle atrophy and fat infiltration can be observed a few weeks after denervation (46). Consequently, in the absence of neural innervation, skeletal muscles inevitably undergo a process of atrophy, eventually largely replaced by fibrous connective and adipose tissue. Similarly, continuous compression of a nerve root by disc herniation also leads to muscle atrophy and fat replacement in muscles supplied by that nerve (47, 48). The MM is particularly susceptible because of being innervated only by the medial branch of the dorsal root of the spinal nerve, with no intersegmental nerve supply like other PSMs (44). Early studies demonstrated that denervated rabbit muscles can transform into white adipose tissue and express specific genes, especially the adipose obese (ob) gene (49). The expression of the ob gene is specific to adipose tissue in various animal species, including humans. Moreover, denervated skeletal muscles of most animals exhibit a dramatic increase in DNA synthesis, since the proliferation of all kinds of cells such as satellite cells, macrophages, mast cells, etc. Most of these cells secrete various cytokines to stimulate the proliferation and differentiation of macrophages, mast cells, fibroblasts, preadipocytes, and muscle precursor cells, thereby promoting muscle repair or fat degeneration (49). Additionally, it has been shown that TNF-α influences myoblast differentiation and fiber degradation and its expression increases with disc injury and affects axon conduction (50).
Figure 4 illustrates the current vein of research on muscle denervation.
2.3 Muscle disuse
IVDD is the main cause of LBP and significantly affects the stability and motor function of the spine. Pain and discomfort from IVDD often make individuals reduce exercise and activity, causing the disuse of PSMs – a lack of exercise. Short-term disuse promotes fatty acid infiltration into skeletal muscle cells and is associated with the development of intermuscular adipose tissue(IMAT) (51). Table 3 provides a summary of recent studies on this mechanism. When PSMs lack exercise, their capillary reactivity decreases, resulting in the muscle blood supply being inadequate and leaving the muscle in a hypoxic state (56). Persistent hypoxia causes incomplete utilization of muscle glycogen and accumulation of lactic acid and various metabolites, which induces muscle edema, stimulating or exacerbating nerve pain (57, 58). The long-term effect makes the muscle gradually thin and degenerate, eventually replaced by adipose tissue (59). Previous studies have indicated a higher accumulation of adipose tissue in PSMs is related to physical inactivity (54, 60). Movement and gravitational loading are essential for maintaining a healthy spine. When the axial load of the human body decreases, such as during prolonged bedrest or spaceflight, the active and passive elements of the lumbar spine are affected (61). In response to decreased use, the lumbar spine undergoes adaptive remodeling (62). Adverse effects include lumbopelvic muscle atrophy, fat accumulation in PSMs, reduced lumbar lordosis, and altered disc hydration status (54, 61). Surprisingly, Hides et al. (63) observed a significant increase in the CSA of the MM during the last magnetic resonance scan of bed rest and the first scan of re-loading. They suggested that this alteration might be attributed to fluid transfer of the atrophic muscle during reloading or fiber and connective tissue damage (subsequent swelling) after walking to mask muscle atrophy. The double S-curve of the spine enables the body to slow down the vertical gravitational loads and spread forces horizontally. However, this requires the action of muscles in the lumbopelvic region to maintain the anterior kyphosis of the spine, especially the MM (64). Bed rest eliminates axial gravitational loading from the spine, potentially depriving the necessary stimulus for the normal functioning of the MM (63). In addition, disc degeneration itself can cause the physiological curve of the spine to become shallower and disappear.
Brisk walking (65), aerobic training (66), resistance training (67–69), and other forms of exercise can effectively prevent fat infiltration or delay its progression speed. Additionally, exercise not only prevents but also improves the inflammatory response in muscles secondary to IVDD (52). This positive effect is attributed to exercise promoting the release of immunomodulatory factors in the blood, which exerts anti-inflammatory effects. Interestingly, Wesselink et al.’s review shows that fat infiltration of PSMs in patients with LBP is irreversible through exercise (55). However, it’s important to note that their conclusions are based on studies with low statistical power and methodological quality, and only provide moderate-quality evidence. In the future, more and higher quality research is needed to draw definitive conclusions.
Figure 5 provides the current research network on muscle disuse.
2.4 Biomechanical imbalance
The IVD and PSMs are not only surface adjacent but also biomechanical and biochemical interconnected (70). Serving as a crucial structure in the spine, the IVD offers buffering and support between adjacent vertebrae but is susceptible to degeneration influenced by factors such as age, lifestyle, and genetics. After degeneration, IVD loses its normal structure and function, which leads to the narrowing of the disc space and settling of the motion segment (71). Subsequently, the ligamentum flavum buckles, eventually leading to instability of the lumbar spine. The main function of PSMs is to maintain the stability and movement of the spine. Under normal circumstances, the load on PSMs in humans is in balance. However, when IVDD causes changes in disc height and vertebral instability, the tension load on PSMs increases to maintain spinal stability. If instability persists or becomes severe, surpassing the stress limits of the PSMs, it can result in atrophy and fat infiltration (16, 71, 72).
The current context of research on biomechanical imbalances is shown in Figure 6.
3 Mechanisms of fat infiltration of PSMs involved in IVDD
Similar to the impact of IVDD on PSMs, fat-infiltrated PSMs also affect IVD. However, there are few studies on the impact of fat infiltration of PSMs in the IVDD. Here we summarize three mechanisms, inflammation, lipid metabolism disorders, and abnormal mechanical load.
3.1 Inflammation mechanism
Adipose tissue is a primary source of numerous inflammatory cytokines, and an excess of adipocytes contributes to a state of systemic chronic low-grade inflammation. Previous studies have established a link between proinflammatory cytokines derived from adipose tissue(including TNF and IL-Iβ) and both obesity and osteoarthritis (73, 74). Obesity can cause fatty infiltration of PSMs (75). Furthermore, in obesity, paraspinal adipose tissue is considered to be a source of systemic pro-inflammatory cytokines (19). The fat infiltration of PSMs primarily consists of white adipose tissue, and the abnormal accumulation of fat leads to functional impairment of white adipose tissue, characterized by elevated levels of specific pro-inflammatory cytokines such as TNF (22). In addition, adipocytes in white adipose tissue recruit macrophages from the blood by synthesizing and secreting lactic acid, and the macrophages are polarized after infiltrating into fat, forming CD11c+ M1 type, highly expressing pro-inflammatory cytokines such as IL-1β and TNF (76), which are key driving factors of IVDD and closely associated with various pathological processes of IVDD (28).
TNF-α primarily exerts its effects through activating the NF-κB signaling pathway, which causes the expression of type II collagen and proteoglycan to be downregulated, and the expression of a disintegrin and metalloproteinase with thrombospondin motifs (ADAMTs)-4,5, matrix metalloproteinases (MMPs) to be upregulated. This cascade induces matrix degradation, cellular apoptosis, and precipitating degenerative changes in the lumbar vertebral discs (77). Simultaneously, the death domain of TNF receptors binds to other intracellular signaling proteins containing death domains, such as TNF receptor-associated death domain protein (TRADD) and Fas-associated death domain protein (FADD). This interaction triggers the cysteinyl-aspartate-specific protease (caspase) family of cascade reactions, which leads to caspase-3 activation, ultimately causing cell apoptosis (78). Additionally, TNF-α also directly inhibits NP cell activity and reduces the expression of growth differentiation factor 5(GDF-5) to accelerate IVDD.
IL-1β mainly activates mitogen-activated protein kinase(MAPK) and nuclear factor-kappa B(NF-κB) signaling pathways, upregulating MMPs-1, 2, 3, 4, 13, and ADAMTs-4, 5 (79). This leads to the degradation of type II collagen and proteoglycans, thereby accelerating the development of IVDD. Furthermore, IL-1β stimulates the expression of vascular endothelial growth factor(VEGF) and nerve growth factor(NGF), promoting the invasion of nerves and blood vessels into degenerated discs (80), eventually inducing LBP. Beyond these effects, IL-1β amplifies the inflammatory cascade by increasing the secretion of inflammatory cytokines (IL-6, 8, 17) and C-C Motif Chemokine Ligand chemokines (CCL-2, 3, 5, 7). This, in turn, attracts immune cells to accumulate at the site of inflammation, releasing inflammatory mediators that exacerbate IVDD (81–83).
Figure 7 portrays the present state of research on the inflammation mechanism.
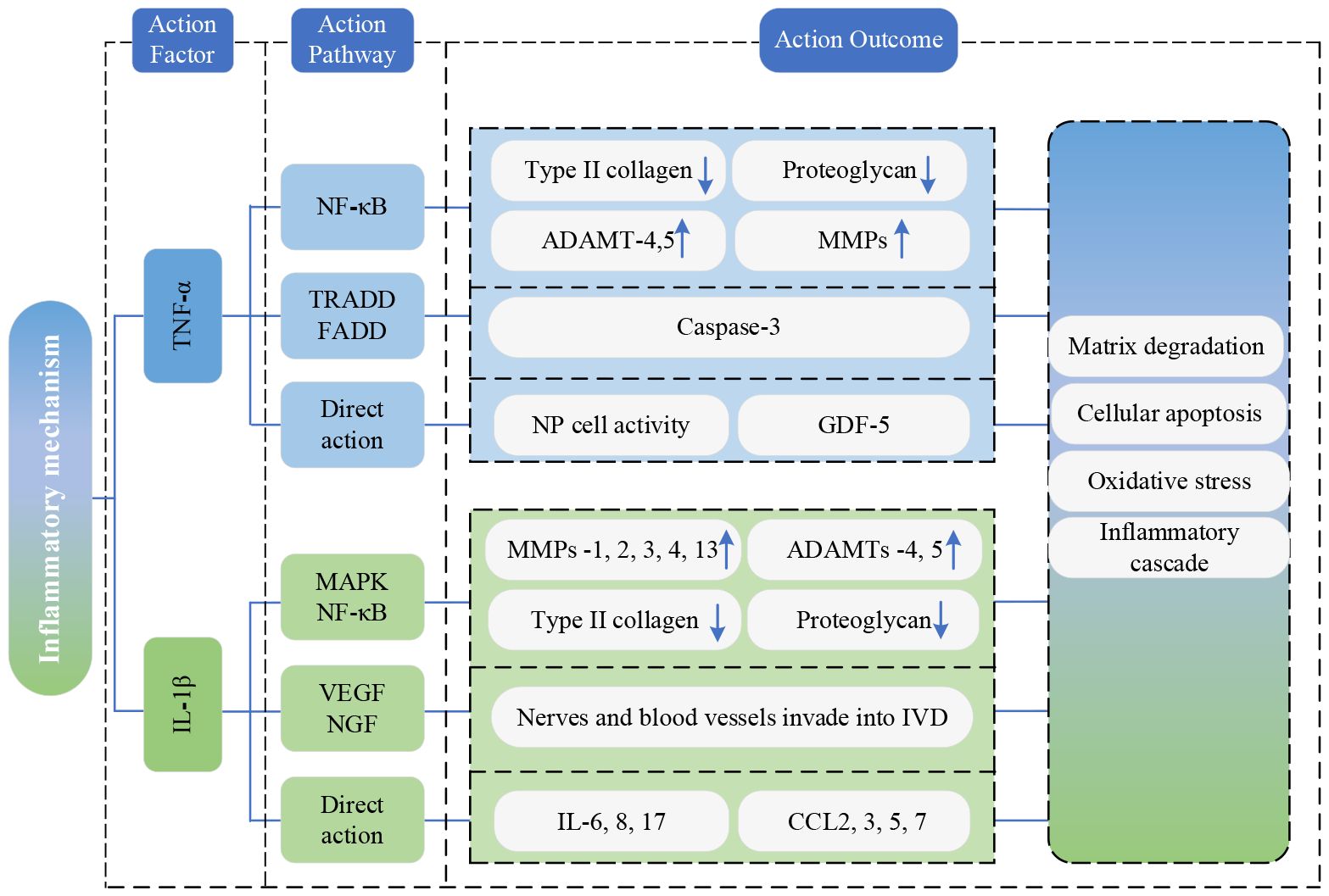
Figure 7 Research state of Inflammation mechanism. TNF-α, tumor necrosis factor-α; NF-κB, nuclear factor-kappa B; IL-1β, interleukin-1beta; ADAMTs, a disintegrin and metalloproteinase with thrombospondin motifs; MMP, matrix metalloproteinases; TRADD, TNF receptor-associated death domain protein; FADD, Fas-associated death domain protein; NP, nucleus pulposus; GDF-5, growth differentiation factor 5; MAPK, mitogen-activated protein kinase; NF-κB, nuclear factor-kappa B; MMPs, matrix metalloproteinases; ADAMTs, a disintegrin and metalloproteinase with thrombospondin motifs; VEGF, vascular endothelial growth factor; NGF, nerve growth factor; IVD, Intervertebral disc; IL, interleukin; CCL, C-C Motif Chemokine Ligand.
3.2 Lipid metabolism disorder
Adipocyte-secreted adipokines, as biologically active substances with metabolic and immunomodulatory functions, play a significant role in the occurrence and progression of IVDD (23).
Leptin can stimulate the proliferation of AF and NP cells by inducing the expression of cell cycle protein D1 and activating JAK-STAT3, MEK-ERK and PI3K-Akt signaling pathways (84). However, despite this proliferative effect, the increased expression of extracellular matrix-degrading enzymes prevents the proper synthesis of extracellular matrix components by the proliferating cells. This imbalance promotes catabolism and contributes to disc cell senescence, eventually leading to IVDD (84, 85). Additionally, when leptin acts alone or in synergy with TNF-α, IL-1β, or IL-6 in NP cells, it significantly increases the production of NO and expression of inflammatory cytokines and MMPs, speeding up the process of IVDD (86). Leptin can also promote calcification of the CEP, interfering with the transport of nutrients to disc cells, and ultimately contributing to IVDD (87).
Adiponectin levels are related to IVDD, nevertheless, conflicting results have been reported in published literature. Khabour et al. discovered higher levels of circulating adiponectin in IVDD patients compared to healthy controls (88), while Yuan et al. verified a downregulation of adiponectin expression in IVDD patients, which is negatively correlated with IVDD severity (89). In addition, adiponectin performs anti-inflammatory effects by inhibiting the expression of pro-inflammatory mediators (89). Therefore, the downregulation of adiponectin in IVD may lead to an imbalanced inflammatory response and contribute to disc degeneration (84).
Resistin is upregulated in IVDD and positively correlates with the Pfirrmann grade (90). Through activating p38-MAPK and NF-κB signaling pathways, resistin could bind to Toll-like receptor 4(TLR4) and augment the expression of CCL4 in NP cells, promoting macrophage infiltration and inflammatory response in IVD tissue (90). Furthermore, resistin, by activating the p38-MAPK signaling pathway, induces metabolic disruption in NP cells and accelerates the progression of IVDD (91).
Visfatin is associated with cell differentiation, stress, apoptosis, and inflammation processes (92). In NP cells, IL-1β stimulates the expression of visfatin, increasing degradation-related proteins ADAMTs-4,5 and MMPs-3,13 and decreasing type II collagen and aggrecan. This process prompts the degradation of the extracellular matrix (22). Moreover, the expression level of visfatin is positively correlated with the degree of IVDD (93).
The current framework of research on adipokines is depicted in Figure 8.
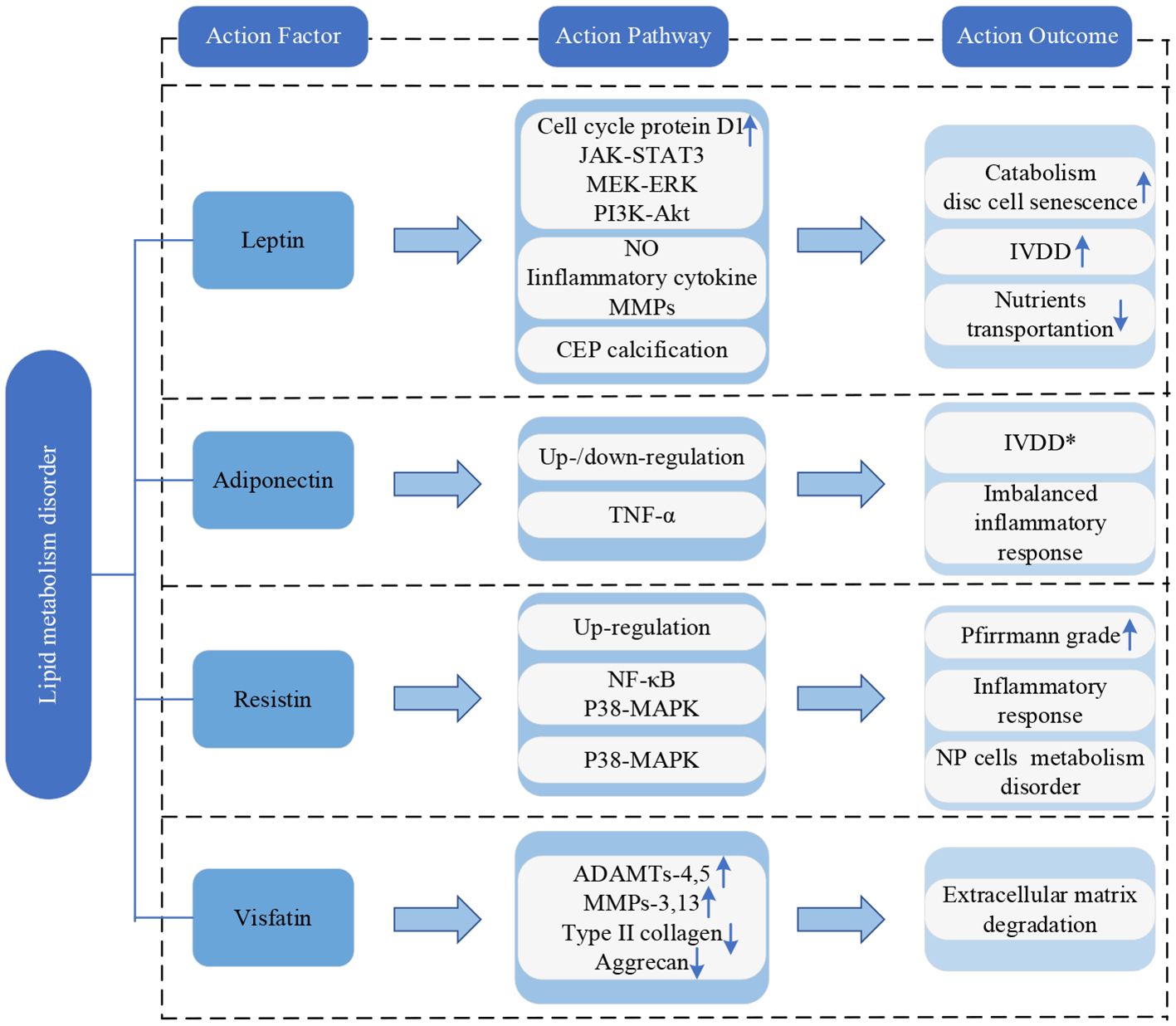
Figure 8 Research framework of lipid metabolism disorder. IVDD, intervertebral disc degeneration; CEP, cartilaginous endplate; TNF-α, tumor necrosis factor-α; NP, nucleus pulposus; NF-κB, nuclear factor-kappa B; MAPK, mitogen-activated protein kinase; ADAMTs, a disintegrin and metalloproteinase with thrombospondin motifs; MMPs, matrix metalloproteinases. * indicates controversy.
3.3 Abnormal mechanical stress
Spinal instability is the premise of the main pathological mechanism leading to IVDD (94). PSMs are a major source of load-bearing for the spine and play a crucial role in its movement and stability. When the PSMs are replaced by fatty tissue, the contractility and load-bearing capacity of muscles are weakened. Consequently, more pressure is transferred to the discs and small joints (21). If the pressure load is excessively high or exits for too long, NP cells will age, apoptosis, and necrosis, resulting in lower activity and decreased numbers, which is one of the most important pathological processes in IVDD (95). Abnormal pressure load can also accelerate the calcification and vascular degeneration of the CEP, leading to a significant decrease in permeability coefficients and transport rates (42). This makes it more difficult for sugar, oxygen, and other macromolecular nutrients IVD cells require and metabolites such as lactic acid to diffuse or excrete. In the acidic and hypoxic microenvironment, the activity and numbers of NP cells decreased, with weakened extracellular matrix (ECM) anabolism capacity, as a result promoting the progress of IVDD (96, 97). Additionally, abnormal mechanical loads can induce increased expression of MMPs and ADAMTs genes, so that the catabolism of ECM exceeds the anabolism. This results in the content of type II collagen and proteoglycan decreasing significantly, leading to dehydration and fibrosis of NP tissue, a reduction in intervertebral space height, instability of the IVD structure, and an accelerated progression of IVDD (98).
The current lineage of research on abnormal mechanical stress is depicted in Figure 9.
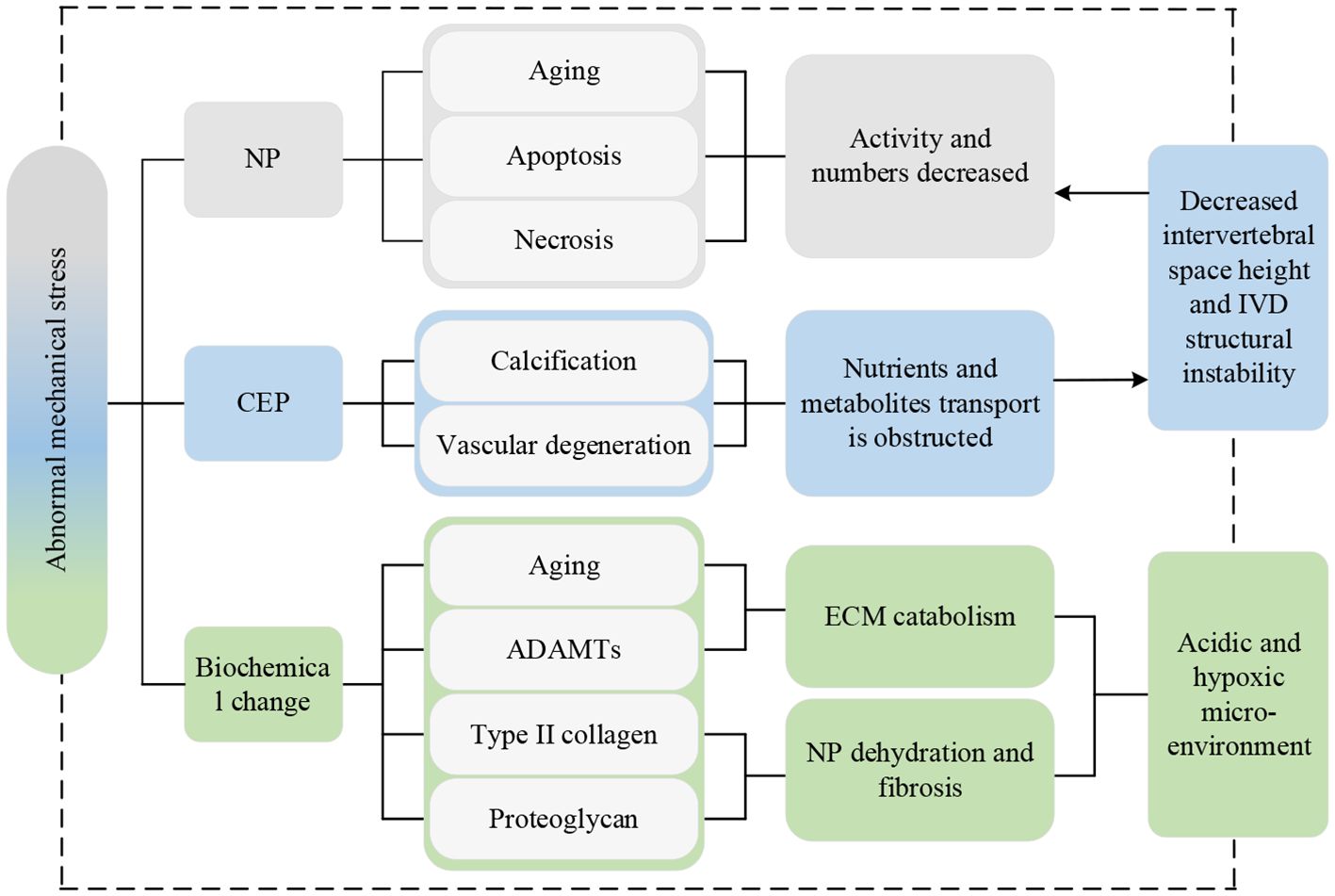
Figure 9 Research lineage of abnormal mechanical stress. Np, nucleus pulposus; CEP, cartilaginous endplate; IVD, intervertebral disc; ADAMTs, a disintegrin and metalloproteinase with thrombospondin motifs; ECM, extracellular matrix.
4 Summary of the evidence and discussion
Figure 10 depicts the above mechanisms intuitively, illustrating more distinctly and visually the interaction between IVDD and fat infiltration of PSMs. Here, several summaries and discussions are stated as follows:
a) Among all the above mechanisms, inflammation is the most extensively studied, because of the universality of the inflammatory response and the numerous types and functions of inflammatory factors.
b) Most studies concentrate on fatty infiltration of the MM. The reason is that MM has the largest attachment area and is only innervated by a single nerve, making it more susceptible and simple to study (99–101).
c) The degree and incidence of IVDD are higher at the L4-L5 and L5-S1 levels, since the proximity of the distal lumbar segments to the static sacrum, and their mobility and stresses are greater than the sacral segments, which is conducive to degeneration (102).
d) The research methods mainly focus on animal experiments and cross-sectional studies, it may be a choice that strategically considers factors such as time efficiency, cost-effectiveness, and the feasibility of repetition.
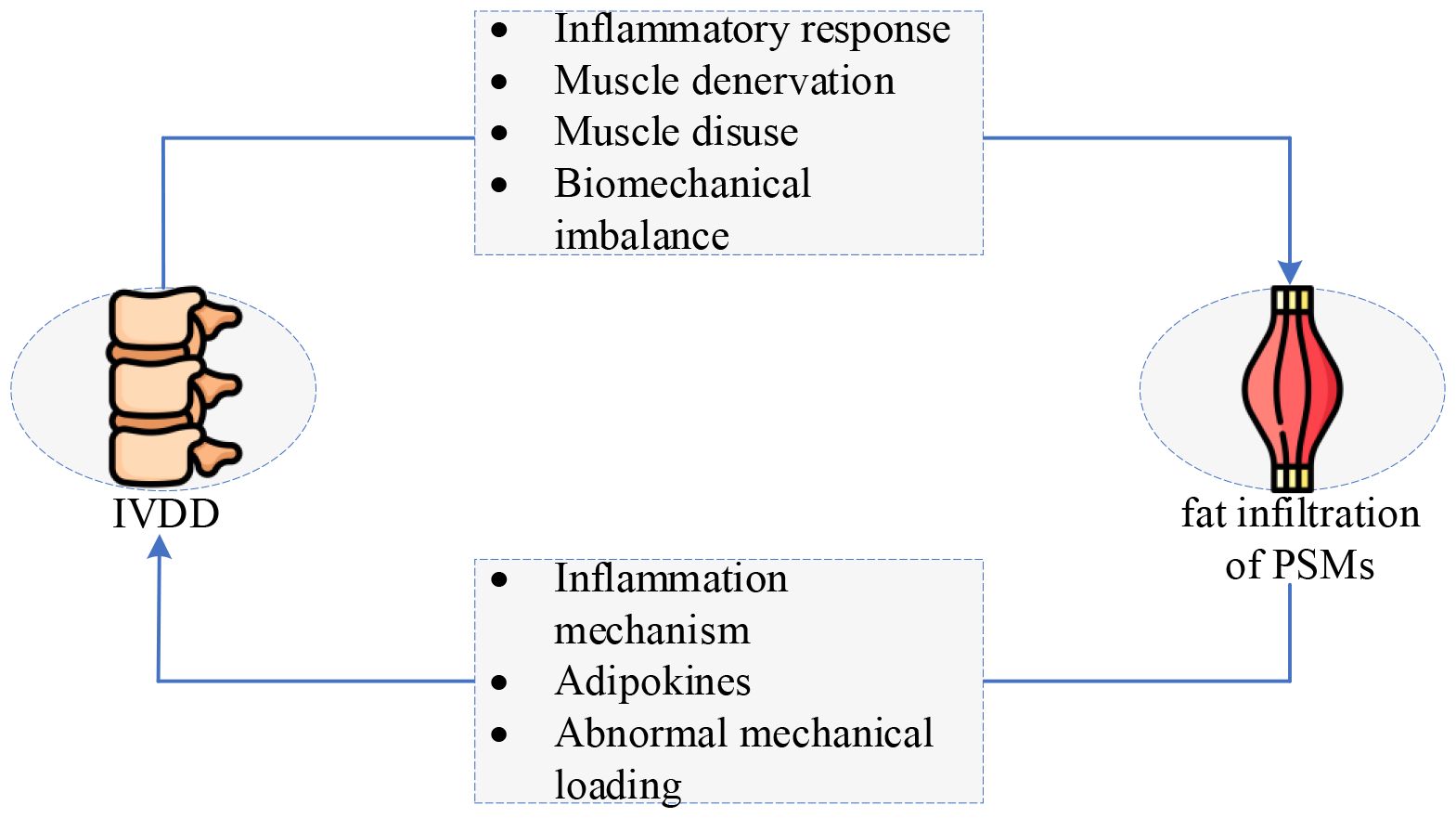
Figure 10 The interaction between IVDD and fat infiltration of PSMs: a vicious circle. IVDD, intervertebral disc degeneration. (The original image is from https://www.freepik.com/pricing.).
5 Conclusions
This paper systematically reviews the interaction between IVDD and fat infiltration of PSMs and classifies its mechanisms. Here, four main conclusions are drawn as follows:
a) IVDD and fat infiltration of PSMs can promote the occurrence and development of each other, forming a vicious cycle that ultimately leads to LBP and disability.
b) Four mechanisms of IVDD causing fatty infiltration of PSMs are summarized, i.e., inflammatory response, muscle denervation, muscle disuse, and biomechanical imbalance.
c) Three mechanisms of IVDD caused by fatty infiltration in PSMs are systematically reviewed: inflammation mechanism, lipid metabolism disorder, and abnormal mechanical stress.
d) Inflammation functions as a bridge between IVDD and fat infiltration of PSMs. Exercise can prevent and improve the inflammatory response.
6 Perspectives
Eight proposals and perspectives in four main aspects (i.e., promising research, experimental methods, models and samples) are proposed as follows:
a) The molecular-level interaction between intervertebral disc tissue and paraspinal muscle fat is not yet fully understood. Specifically, the molecular mechanisms of signal transduction and cytokine release between intervertebral disc cells and paraspinal muscle adipocytes still need to be further studied.
b) Muscles at a single spinal level do not represent the whole lumbar spine muscles, so future studies should measure the amount of fat infiltration in each spine-level muscle and collect its tissue samples.
c) Differences in the segmentation method of fat infiltration in PSMs (in- or excluding epimuscular fat) in different studies, thus clear quantification of lipid levels within and out of muscle cells is recommended for future research, ensuring more consistent and comparable outcomes in the studies assessing fat infiltration of PSMs.
d) Although rats are a commonly used experimental animal model, they cannot fully simulate humans due to the huge differences between them and humans. Hence, animal models closer to humans should be explored, or high-quality clinical cohort studies could be conducted.
e) Given the complexity, time, and cost of research, numerous studies, both animal experiments and clinical investigations often have relatively small sample sizes. Therefore, future studies could increase the sample size to obtain more powerful and reliable results.
f) Considering the pivotal role of PSMs’ health in spinal stability and functional outcomes, it is recommended to integrate information concerning the extent of fat infiltration and atrophy of PSMs into decision-making algorithms for Early Recovery After Surgery (ERAS) protocols. According to a systematic review by Zaed et al. (103), the importance of ERAS in spinal surgery is emphasized but still needs to be supported by high-quality clinical randomized controlled trials (RCT). Therefore, it is also advised that fat infiltration be taken into account in future RCT.
g) The treatment of LBP should pay more attention to the relationship between IVD and PSMs. Given the complex interplay between IVDD and fat infiltration of PSMs, a multimodal treatment targeting multiple mechanisms can be adopted in the future. Combining pharmacotherapy, exercise interventions, and neuromodulation techniques may offer synergistic benefits in managing LBP.
h) Considering that inflammation is a critical bridge between IVDD and fatty infiltration of PSMs, it may be possible in the future to disrupt the vicious cycle of IVDD and fatty infiltration and halt the progression of LBP with targeted anti-inflammatory drug therapy.
Author contributions
JJ: Writing – original draft, Writing – review & editing, Formal analysis, Investigation, Visualization. YH: Funding acquisition, Conceptualization, Writing – review & editing. BH: Funding acquisition, Supervision, Validation, Writing – review & editing.
Funding
The author(s) declare financial support was received for the research, authorship, and/or publication of this article. The authors gratefully acknowledge the support of the National Natural Science Foundation of China (No. 82302131, 82260338); and the Yunnan Fundamental Research Projects (No. 202301AY070001203, 202301AU70163, 202301AS070016).
Conflict of interest
The authors declare that the research was conducted in the absence of any commercial or financial relationships that could be construed as a potential conflict of interest.
Publisher’s note
All claims expressed in this article are solely those of the authors and do not necessarily represent those of their affiliated organizations, or those of the publisher, the editors and the reviewers. Any product that may be evaluated in this article, or claim that may be made by its manufacturer, is not guaranteed or endorsed by the publisher.
References
1. Buchbinder R, Van Tulder M, Öberg B, Costa LM, Woolf A, Schoene M, et al. Low back pain: a call for action. Lancet. (2018) 391:2384–8. doi: 10.1016/S0140–6736(18)30488–4
2. Cieza A, Causey K, Kamenov K, Hanson SW, Chatterji S, Vos T. Global estimates of the need for rehabilitation based on the Global Burden of Disease study 2019: a systematic analysis for the Global Burden of Disease Study 2019. Lancet. (2020) 396:2006–17. doi: 10.1016/S0140–6736(20)32340–0
3. Ferreira ML, De Luca K, Haile LM, Steinmetz JD, Culbreth GT, Cross M, et al. Global, regional, and national burden of low back pain, 1990–2020, its attributable risk factors, and projections to 2050: a systematic analysis of the Global Burden of Disease Study 2021. Lancet Rheumatol. (2023) 5:e316–29. doi: 10.2139/ssrn.4318392
4. Hartvigsen J, Hancock MJ, Kongsted A, Louw Q, Ferreira ML, Genevay S, et al. What low back pain is and why we need to pay attention. Lancet. (2018) 391:2356–67. doi: 10.1016/S0140-6736(18)30480-X
5. Knezevic NN, Candido KD, Vlaeyen JWS, Van Zundert J, Cohen SP. Low back pain. Lancet. (2021) 398:78–92. doi: 10.1016/S0140–6736(21)00733–9
6. Russo F, Ambrosio L, Giannarelli E, Vorini F, Mallio CA, Quattrocchi CC, et al. Innovative quantitative magnetic resonance tools to detect early intervertebral disc degeneration changes: a systematic review. Spine J. (2023) 23:1435–50. doi: 10.1016/j.spinee.2023.05.011
7. Sakai D, Grad S. Advancing the cellular and molecular therapy for intervertebral disc disease. Advanced Drug Delivery Rev. (2015) 84:159–71. doi: 10.1016/j.addr.2014.06.009
8. Guo W, Li BL, Zhao JY, Li XM, Wang LF. Causal associations between modifiable risk factors and intervertebral disc degeneration. Spine J. (2023) 0:S1529943023034873. doi: 10.1016/j.spinee.2023.10.021
9. Gullbrand SE, Ashinsky BG, Bonnevie ED, Kim DH, Engiles JB, Smith LJ, et al. Long term mechanical function and integration of an implanted tissue engineered intervertebral disc. Sci Transl Med. (2018) 10:eaau0670. doi: 10.1126/scitranslmed.aau0670
10. Yu J, Taylor W, Verrills P, Porter LR, Gavino A, Young JF, et al. Results of a pilot study on the safety and early efficacy of novel polymethyl methacrylate microspheres and a hyaluronic acid device for the treatment of low back pain caused by degenerative and diseased intervertebral discs of the lumbar spine. Cureus. (2021) 13:e16308. doi: 10.7759/cureus.16308
11. Vergroesen PP, Kingma I, Emanuel KS, Hoogendoorn RJ, Welting TJ, van Royen BJ, et al. Mechanics and biology in intervertebral disc degeneration: a vicious circle. Osteoarthritis Cartilage. (2015) 23:1057–70. doi: 10.1016/j.joca.2015.03.028
12. Shi L, Yan B, Jiao Y, Chen Z, Zheng Y, Lin Y, et al. Correlation between the fatty infiltration of paraspinal muscles and disc degeneration and the underlying mechanism. BMC Musculoskelet Disord. (2022) 23:509. doi: 10.1186/s12891–022-05466–8
13. Teichtahl AJ, Urquhart DM, Wang Y, Wluka AE, Wijethilake P, O’Sullivan R, et al. Fat infiltration of paraspinal muscles is associated with low back pain, disability, and structural abnormalities in community-based adults. Spine J. (2015) 15:1593–601. doi: 10.1016/j.spinee.2015.03.039
14. Hildebrandt M, Fankhauser G, Meichtry A, Luomajoki H. Correlation between lumbar dysfunction and fat infiltration in lumbar multifidus muscles in patients with low back pain. BMC Musculoskelet Disord. (2017) 18:12. doi: 10.1186/s12891–016-1376–1
15. Huang Y, Wang L, Luo B, Yang K, Zeng X, Chen J, et al. Associations of lumber disc degeneration with paraspinal muscles myosteatosis in discogenic low back pain. Front Endocrinol. (2022) 13:891088. doi: 10.3389/fendo.2022.891088
16. Guan J, Zhao D, Liu T, Yu X, Feng N, Jiang G, et al. Correlation between surgical segment mobility and paravertebral muscle fatty infiltration of upper adjacent segment in single-segment LDD patients: retrospective study at a minimum 2 years’ follow-up. BMC Musculoskelet Disord. (2023) 24:28. doi: 10.1186/s12891-023-06137-y
17. Hodges P, Holm AK, Hansson T, Holm S. Rapid atrophy of the lumbar multifidus follows experimental disc or nerve root injury. Spine (2006) 31:2926–33. doi: 10.1097/01.brs.0000248453.51165.0b
18. Boström AF, Parzefall B, Blutke A, Davies ES. Epaxial muscle atrophy is more evident in large dogs with intervertebral disc disease than in dogs with ischaemic myelopathy. Res Veterinary Sci. (2022) 146:60–9. doi: 10.1016/j.rvsc.2022.03.011
19. Chen X, Li Y, Wang W, Cui P, Wang Y, Lu S. Correlation between inflammatory cytokine expression in paraspinal tissues and severity of disc degeneration in individuals with lumbar disc herniation. BMC Musculoskelet Disord. (2023) 24:193. doi: 10.1186/s12891-023-06295-z
20. James G, Chen X, Diwan A, Hodges PW. Fat infiltration in the multifidus muscle is related to inflammatory cytokine expression in the muscle and epidural adipose tissue in individuals undergoing surgery for intervertebral disc herniation. Eur Spine J. (2021) 30:837–45. doi: 10.1007/s00586–020-06514–4
21. Sudhir G, Jayabalan V, Sellayee S, Gadde S, Kailash K. Is there an interdependence between paraspinal muscle mass and lumbar disc degeneration? A MRI based study at 2520 levels in 504 patients. J Clin Orthopaedics Trauma. (2021) 22:101576. doi: 10.1016/j.jcot.2021.101576
22. Francisco V, Pino J, González-Gay MÁ, Lago F, Karppinen J, Tervonen O, et al. A new immunometabolic perspective of intervertebral disc degeneration. Nat Rev Rheumatol. (2022) 18:47–60. doi: 10.1038/s41584-021-00713-z
23. MacDonald IJ, Liu SC, Huang CC, Kuo SJ, Tsai CH, Tang CH. Associations between adipokines in arthritic disease and implications for obesity. IJMS. (2019) 20:1505. doi: 10.3390/ijms20061505
24. Wang Z, Zhao Z, Han S, Hu X, Ye L, Li Y, et al. Advances in research on fat infiltration and lumbar intervertebral disc degeneration. Front Endocrinol. (2022) 13:1067373. doi: 10.3389/fendo.2022.1067373
25. James G, Sluka KA, Blomster L, Hall L, Schmid AB, Shu CC, et al. Macrophage polarization contributes to local inflammation and structural change in the multifidus muscle after intervertebral disc injury. Eur Spine J. (2018) 27:1744–56. doi: 10.1007/s00586–018-5652–7
26. Lyu FJ, Cui H, Pan H, Mc Cheung K, Cao X, Iatridis JC, et al. Painful intervertebral disc degeneration and inflammation: from laboratory evidence to clinical interventions. Bone Res. (2021) 9:7. doi: 10.1038/s41413-020-00125-x
27. Khan AN, Jacobsen HE, Khan J, Filippi CG, Levine M, Lehman RA, et al. Inflammatory biomarkers of low back pain and disc degeneration: a review. Ann NY Acad Sci. (2017) 1410:68–84. doi: 10.1111/nyas.13551
28. Wang Y, Che M, Xin J, Zheng Z, Li J, Zhang S. The role of IL-1β and TNF-α in intervertebral disc degeneration. Biomedicine Pharmacotherapy. (2020) 131:110660. doi: 10.1016/j.biopha.2020.110660
29. Furukawa S, Fujita T, Shimabukuro M, Iwaki M, Yamada Y, Nakajima Y, et al. Increased oxidative stress in obesity and its impact on metabolic syndrome. J Clin Invest. (2004) 114:1752–61. doi: 10.1172/JCI21625
30. Elliott J, Pedler A, Kenardy J, Galloway G, Jull G, Sterling M. The temporal development of fatty infiltrates in the neck muscles following whiplash injury: an association with pain and posttraumatic stress. PLoS One. (2011) 6:e21194. doi: 10.1371/journal.pone.0021194
31. Thoma A, Lightfoot AP. NF-kB and inflammatory cytokine signalling: role in skeletal muscle atrophy. Adv Exp Med Biol. (2018) 1088:267–79. doi: 10.1007/978–981-13–1435-3_12
32. Valerio A, Cardile A, Cozzi V, Bracale R, Tedesco L, Pisconti A, et al. TNF- downregulates eNOS expression and mitochondrial biogenesis in fat and muscle of obese rodents. J Clin Invest. (2006) 116:2791–8. doi: 10.1172/JCI28570
33. Hodges PW, James G, Blomster L, Hall L, Schmid AB, Shu C, et al. Can proinflammatory cytokine gene expression explain multifidus muscle fiber changes after an intervertebral disc lesion? Spine. (2014) 39:1010–7. doi: 10.1097/BRS.0000000000000318
34. James G, Blomster L, Hall L, Schmid AB, Shu CC, Little CB, et al. Mesenchymal Stem Cell Treatment of Intervertebral Disc Lesion Prevents Fatty Infiltration and Fibrosis of the Multifidus Muscle, but not Cytokine and Muscle Fiber Changes. Spine. (2016) 41:1208–17. doi: 10.1097/BRS.0000000000001669
35. Hodges PW, James G, Blomster L, Hall L, Schmid A, Shu C, et al. Multifidus muscle changes after back injury are characterized by structural remodeling of muscle, adipose and connective tissue, but not muscle atrophy: molecular and morphological evidence. Spine. (2015) 40:1057–71. doi: 10.1097/BRS.0000000000000972
36. Agha O, Mueller-Immergluck A, Liu M, Zhang H, Theologis AA, Clark A, et al. Intervertebral disc herniation effects on multifidus muscle composition and resident stem cell populations. JOR Spine. (2020) 3:e1091. doi: 10.1002/jsp2.1091
37. Uezumi A, Ito T, Morikawa D, Shimizu N, Yoneda T, Segawa M, et al. Fibrosis and adipogenesis originate from a common mesenchymal progenitor in skeletal muscle. J Cell Sci. (2011) 124:3654–64. doi: 10.1242/jcs.086629
38. Uezumi A, Fukada S, Yamamoto N, Takeda S, Tsuchida K. Mesenchymal progenitors distinct from satellite cells contribute to ectopic fat cell formation in skeletal muscle. Nat Cell Biol. (2010) 12:143–52. doi: 10.1038/ncb2014
39. Özcan-Ekşi EE, Ekşi MŞ, Turgut VU, Canbolat Ç, Pamir MN. Reciprocal relationship between multifidus and psoas at L4-L5 level in women with low back pain. Br J Neurosurg. (2021) 35:220–8. doi: 10.1080/02688697.2020.1783434
40. Gorski T, Mathes S, Krützfeldt J. Uncoupling protein 1 expression in adipocytes derived from skeletal muscle fibro/adipogenic progenitors is under genetic and hormonal control: UCP1 expression in skeletal muscle FAPs. J Cachexia Sarcopenia Muscle. (2018) 9:384–99. doi: 10.1002/jcsm.12277
41. Kong X, Yao T, Zhou P, Kazak L, Tenen D, Lyubetskaya A, et al. Brown adipose tissue controls skeletal muscle function via the secretion of myostatin. Cell Metab. (2018) 28:631–43.e3. doi: 10.1016/j.cmet.2018.07.004
42. Yeh C-H, Jin L, Shen F, Balian G, Li X. MiR-221 attenuates the osteogenic differentiation of human annulus fibrosus cells. Spine J. (2016) 16:896–904. doi: 10.1016/j.spinee.2016.03.026
43. Battié MC, Niemelainen R, Gibbons LE, Dhillon S. Is level- and side-specific multifidus asymmetry a marker for lumbar disc pathology? Spine J. (2012) 12:932–9. doi: 10.1016/j.spinee.2012.08.020
44. Yaltırık K, Güdü BO, Işık Y, Altunok Ç, Tipi U, Atalay B. Volumetric muscle measurements indicate significant muscle degeneration in single-level disc herniation patients. World Neurosurg. (2018) 116:e500–4. doi: 10.1016/j.wneu.2018.05.019
45. Carlson BM. The biology of long-term denervated skeletal muscle. Eur J Transl Myol. (2014) 24:5–11. doi: 10.4081/ejtm.2014.3293
46. Kamath S, Venkatanarasimha N, Walsh MA, Hughes PM. MRI appearance of muscle denervation. Skeletal Radiol. (2008) 37:397–404. doi: 10.1007/s00256–007-0409–0
47. Cooley JR, Walker BF M, Ardakani E, Kjaer P, Jensen TS, Hebert JJ. Relationships between paraspinal muscle morphology and neurocompressive conditions of the lumbar spine: a systematic review with meta-analysis. BMC Musculoskelet Disord. (2018) 19:351. doi: 10.1186/s12891–018-2266–5
48. Carvalho V, Santos J, Santos Silva P, Vaz R, Pereira P. Relationship between fatty infiltration of paraspinal muscles and clinical outcome after lumbar discectomy. Brain Spine. (2022) 2:101697. doi: 10.1016/j.bas.2022.101697
49. Dulor JP, Cambon B, Vigneron P, Reyne Y, Nouguès J, Casteilla L, et al. Expression of specific white adipose tissue genes in denervation-induced skeletal muscle fatty degeneration. FEBS Lett. (1998) 439:89–92. doi: 10.1016/S0014–5793(98)01216–2
50. Wang X, Jia R, Li J, Zhu Y, Liu H, Wang W, et al. Research progress on the mechanism of lumbarmultifidus injury and degeneration. Oxid Med Cell Longevity. (2021) 2021:1–9. doi: 10.1155/2021/6629037
51. Pagano AF, Brioche T, Arc-Chagnaud C, Demangel R, Chopard A, Py G. Short-term disuse promotes fatty acid infiltration into skeletal muscle: Short term muscle disuse promotes IMAT development. J Cachexia Sarcopenia Muscle. (2018) 9:335–47. doi: 10.1002/jcsm.12259
52. James G, Millecamps M, Stone LS, Hodges PW. Dysregulation of the inflammatory mediators in the multifidus muscle after spontaneous intervertebral disc degeneration SPARC-null mice is ameliorated by physical activity. Spine. (2018) 43:E1184–94. doi: 10.1097/BRS.0000000000002656
53. Maurer E, Klinger C, Lorbeer R, Rathmann W, Peters A, Schlett CL, et al. Long-term effect of physical inactivity on thoracic and lumbar disc degeneration-an MRI-based analysis of 385 individuals from the general population. Spine J. (2020) 20:1386–96. doi: 10.1016/j.spinee.2020.04.016
54. De Martino E, Hides J, Elliott JM, Hoggarth MA, Zange J, Lindsay K, et al. Intramuscular lipid concentration increased in localized regions of the lumbar muscles following 60 day bedrest. Spine J. (2022) 22:616–28. doi: 10.1016/j.spinee.2021.11.007
55. Wesselink EO, Pool JJM, Mollema J, Weber KA, Elliott JM, Coppieters MW, et al. Is fatty infiltration in paraspinal muscles reversible with exercise in people with low back pain? A systematic review. Eur Spine J. (2023) 32:787–96. doi: 10.1007/s00586-022-07471-w
56. Valdivieso P, Franchi MV, Gerber C, Flück M. Does a better perfusion of deconditioned muscle tissue release chronic low back pain? Front Med (Lausanne). (2018) 5:77. doi: 10.3389/fmed.2018.00077
57. Tang Y, Yang S, Chen C, Luo K, Chen Y, Wang D, et al. Assessment of the association between paraspinal muscle degeneration and quality of life in patients with degenerative lumbar scoliosis. Exp Ther Med. (2020) 20:505–11. doi: 10.3892/etm.2020.8682
58. Zhao X, Liang H, Hua Z, Li W, Li J, Wang L, et al. The morphological characteristics of paraspinal muscles in young patients with unilateral neurological symptoms of lumbar disc herniation. BMC Musculoskelet Disord. (2022) 23:994. doi: 10.1186/s12891–022-05968–5
59. Liu C, Xue J, Liu J, Ma G, Moro A, Liang T, et al. Is there a correlation between upper lumbar disc herniation and multifidus muscle degeneration? A retrospective study of MRI morphology. BMC Musculoskelet Disord. (2021) 22:92. doi: 10.1186/s12891-021-03970-x
60. Sions JM, Elliott JM, Pohlig RT, Hicks GE. Trunk muscle characteristics of the multifidi, erector spinae, psoas, and quadratus lumborum in older adults with and without chronic low back pain. J Orthop Sports Phys Ther. (2017) 47:173–9. doi: 10.2519/jospt.2017.7002
61. De Martino E, Hides J, Elliott JM, Hoggarth MA, Zange J, Lindsay K, et al. The effects of reconditioning exercises following prolonged bed rest on lumbopelvic muscle volume and accumulation of paraspinal muscle fat. Front Physiol. (2022) 13:862793. doi: 10.3389/fphys.2022.862793
62. Stein TP, Wade CE. Nutritional consequences of critical illness myopathies. J Nutr. (2005) 135:1803S–5S. doi: 10.1093/jn/135.7.1803S
63. Hides JA, Belavý DL, Stanton W, Wilson SJ, Rittweger J, Felsenberg D, et al. Magnetic resonance imaging assessment of trunk muscles during prolonged bed rest. Spine (2007) 32:1687–92. doi: 10.1097/BRS.0b013e318074c386
64. Bailey JF, Miller SL, Khieu K, O’Neill CW, Healey RM, Coughlin DG, et al. From the international space station to the clinic: how prolonged unloading may disrupt lumbar spine stability. Spine J. (2018) 18:7–14. doi: 10.1016/j.spinee.2017.08.261
65. Goodpaster BH, Chomentowski P, Ward BK, Rossi A, Glynn NW, Delmonico MJ, et al. Effects of physical activity on strength and skeletal muscle fat infiltration in older adults: a randomized controlled trial. J Appl Physiol. (2008) 105:1498–503. doi: 10.1152/japplphysiol.90425.2008
66. Nakagawa Study Group, Ikenaga M, Yamada Y, Kose Y, Morimura K, Higaki Y, et al. Effects of a 12-week, short-interval, intermittent, low-intensity, slow-jogging program on skeletal muscle, fat infiltration, and fitness in older adults: randomized controlled trial. Eur J Appl Physiol. (2017) 117:7–15. doi: 10.1007/s00421–016-3493–9
67. Ghasemikaram M, Chaudry O, Nagel AM, Uder M, Jakob F, Kemmler W, et al. Effects of 16 months of high intensity resistance training on thigh muscle fat infiltration in elderly men with osteosarcopenia. GeroScience. (2021) 43:607–17. doi: 10.1007/s11357–020-00316–8
68. Flor-Rufino C, BarraChina-Igual J, Pérez-Ros P, Pablos-Monzó A, Sanz-Requena R, Martínez-Arnau FM. Fat infiltration and muscle hydration improve after high-intensity resistance training in women with sarcopenia. A randomized Clin trial Maturitas. (2023) 168:29–36. doi: 10.1016/j.maturitas.2022.09.001
69. Marcus RL, Addison O, Kidde JP, Dibble LE, Lastayo PC. Skeletal muscle fat infiltration: impact of age, inactivity, and exercise. J Nutr Health Aging. (2013) 14:362–6. doi: 10.1007/s12603–010-0081–2
70. Zhao Y, Huang M, Serrano Sosa M, Cattell R, Fan W, Li M, et al. Fatty infiltration of paraspinal muscles is associated with bone mineral density of the lumbar spine. Arch Osteoporos. (2019) 14:99. doi: 10.1007/s11657–019-0639–5
71. Khattab K, Dziesinski LK, Crawford R, Ballatori A, Nyayapati P, Krug R, et al. Spatial distribution of fat infiltration within the paraspinal muscles: implications for chronic low back pain. Eur Spine J. (2022) 31:2875–83. doi: 10.1007/s00586–022-07296–7
72. Kalichman L, Carmeli E, Been E. The association between imaging parameters of the paraspinal muscles, spinal degeneration, and low back pain. BioMed Res Int. (2017) 2017:1–14. doi: 10.1155/2017/2562957
73. Pecchi E, Priam S, Gosset M, Pigenet A, Sudre L, Laiguillon MC, et al. Induction of nerve growth factor expression and release by mechanical and inflammatory stimuli in chondrocytes: possible involvement in osteoarthritis pain. Arthritis Res Ther. (2014) 16:R16. doi: 10.1186/ar4443
74. De Boer TN, Van Spil WE, Huisman AM, Polak AA, Bijlsma JWJ, Lafeber FPJG, et al. Serum adipokines in osteoarthritis; comparison with controls and relationship with local parameters of synovial inflammation and cartilage damage. Osteoarthritis Cartilage. (2012) 20:846–53. doi: 10.1016/j.joca.2012.05.002
75. Özcan-Ekşi EE, Turgut VU, Küçüksüleymanoğlu D, Ekşi MŞ. Obesity could be associated with poor paraspinal muscle quality at upper lumbar levels and degenerated spine at lower lumbar levels: Is this a domino effect? J Clin Neurosci. (2021) 94:120–7. doi: 10.1016/j.jocn.2021.10.005
76. Feng T, Zhao X, Gu P, Yang W, Wang C, Guo Q, et al. Adipocyte-derived lactate is a signalling metabolite that potentiates adipose macrophage inflammation via targeting PHD2. Nat Commun. (2022) 13:5208. doi: 10.1038/s41467–022-32871–3
77. Cabal-Hierro L, Lazo PS. Signal transduction by tumor necrosis factor receptors. Cell Signalling. (2012) 24:1297–305. doi: 10.1016/j.cellsig.2012.02.006
78. Füllsack S, Rosenthal A, Wajant H, Siegmund D. Redundant and receptor-specific activities of TRADD, RIPK1 and FADD in death receptor signaling. Cell Death Dis. (2019) 10:122. doi: 10.1038/s41419–019-1396–5
79. Wang WJ, Yu XH, Wang C, Yang W, He WS, Zhang SJ, et al. MMPs and ADAMTSs in intervertebral disc degeneration. Clinica Chimica Acta. (2015) 448:238–46. doi: 10.1016/j.cca.2015.06.023
80. La Binch A, Cole AA, Breakwell LM, Michael AL, Chiverton N, Cross AK, et al. Expression and regulation of neurotrophic and angiogenic factors during human intervertebral disc degeneration. Arthritis Res Ther. (2014) 16:416. doi: 10.1186/s13075–014-0416–1
81. Pattappa G, Peroglio M, Sakai D, Mochida J, Benneker LM, Alini M, et al. CCL5/RANTES is a key chemoattractant released by degenerative intervertebral discs in organ culture. eCM. (2014) 27:124–36. doi: 10.22203/eCM.v027a10
82. Gruber H, Hoelscher G, Ingram J, Norton H, Hanley E. Increased IL-17 expression in degenerated human discs and increased production in cultured annulus cells exposed to IL-1ß and TNF-α. Biotechnic Histochem. (2013) 88:302–10. doi: 10.3109/10520295.2013.783235
83. Wang J, Tian Y, Phillips KLE, Chiverton N, Haddock G, Bunning RA, et al. Tumor necrosis factor α- and interleukin-1β-dependent induction of CCL3 expression by nucleus pulposus cells promotes macrophage migration through CCR1. Arthritis Rheumatism. (2013) 65:832–42. doi: 10.1002/art.37819
84. Ruiz-Fernández C, Francisco V, Pino J, Mera A, González-Gay MA, Gómez R, et al. Molecular relationships among obesity, inflammation and intervertebral disc degeneration: are adipokines the common link? IJMS. (2019) 20:2030. doi: 10.3390/ijms20082030
85. Zhao C-Q, Liu D, Li H, Jiang L-S, Dai L-Y. Expression of leptin and its functional receptor on disc cells: contribution to cell proliferation. Spine. (2008) 33:E858–64. doi: 10.1097/BRS.0b013e31818338e5
86. Segar AH, Fairbank JCT, Urban J. Leptin and the intervertebral disc: a biochemical link exists between obesity, intervertebral disc degeneration and low back pain—an in vitro study in a bovine model. Eur Spine J. (2019) 28:214–23. doi: 10.1007/s00586–018-5778–7
87. Han Y, Ma B, Guo S, Yang M, Li L, Wang S, et al. Leptin regulates disc cartilage endplate degeneration and ossification through activation of the MAPK-ERK signalling pathway in vivo and in vitro. J Cell Mol Medi. (2018) 22:2098–109. doi: 10.1111/jcmm.13398
88. Khabour OF, Abu-Rumeh L, Al-Jarrah M, Jamous M, Alhashimi F. Association of adiponectin protein and ADIPOQ gene variants with lumbar disc degeneration. Exp Ther Med. (2014) 8:1340–4. doi: 10.3892/etm.2014.1909
89. Yuan B, Huang L, Yan M, Zhang S, Zhang Y, Jin B, et al. Adiponectin downregulates TNF-α Expression in degenerated intervertebral discs. Spine. (2018) 43:E381–9. doi: 10.1097/BRS.0000000000002364
90. Li Z, Wang X, Pan H, Yang H, Li X, Zhang K, et al. Resistin promotes CCL4 expression through toll-like receptor-4 and activation of the p38-MAPK and NF-κB signaling pathways: implications for intervertebral disc degeneration. Osteoarthritis Cartilage. (2017) 25:341–50. doi: 10.1016/j.joca.2016.10.002
91. Liu C, Yang H, Gao F, Li X, An Y, Wang J, et al. Resistin promotes intervertebral disc degeneration by upregulation of ADAMTS-5 through p38 MAPK signaling pathway. Spine. (2016) 41:1414–20. doi: 10.1097/BRS.0000000000001556
92. Zhang Z, Xiao K, Wang S, Ansari AR, Niu X, Yang W, et al. Visfatin is a multifaceted molecule that exerts regulation effects on inflammation and apoptosis in RAW264.7 cells and mice immune organs. Front Immunol. (2022) 13:1018973. doi: 10.3389/fimmu.2022.1018973
93. Shi C, Wu H, Chen H, Wang X, Liu Y, Cao P, et al. Nicotinamide phosphoribosyl transferase inhibitor APO866 prevents IL-1β-induced human nucleus pulpo⁃ sus cell degeneration via autophagy. Cell Physiol Biochem. (2018) 49:2463–82. doi: 10.1159/000493843
94. Musa G, Abakirov MD, Chmutin GE, Mamyrbaev ST, Ramirez MDJE, Sichizya K, et al. Advancing insights into recurrent lumbar disc herniation: A comparative analysis of surgical approaches and a new classification. J Craniovertebral Junction Spine. (2024) 15:66–73. doi: 10.4103/jcvjs.jcvjs_177_23
95. Yurube T, Hirata H, Kakutani K, Maeno K, Takada T, Zhang Z, et al. Notochordal cell disappearance and modes of apoptotic cell death in a rat tail static compression-induced disc degeneration model. Arthritis Res Ther. (2014) 16:R31. doi: 10.1186/ar4460
96. Arpinar VE, Rand SD, Klein AP, Maiman DJ, Muftuler LT. Changes in perfusion and diffusion in the endplate regions of degenerating intervertebral discs: a DCE-MRI study. Eur Spine J. (2015) 24:2458–67. doi: 10.1007/s00586-015-4172-y
97. Tang X, Jing L, Richardson WJ, Isaacs RE, Fitch RD, Brown CR, et al. Identifying molecular phenotype of nucleus pulposus cells in human intervertebral disc with aging and degeneration. J Orthop Res. (2016) 34:1316–26. doi: 10.1002/jor.23244
98. Bonnevie ED, Gullbrand SE, Ashinsky BG, Tsinman TK, Elliott DM, Chao PG, et al. Aberrant mechanosensing in injured intervertebral discs as a result of boundary-constraint disruption and residual-strain loss. Nat BioMed Eng. (2019) 3:998–1008. doi: 10.1038/s41551–019-0458–4
99. Sun D, Liu P, Cheng J, Ma Z, Liu J, Qin T. Correlation between intervertebral disc degeneration, paraspinal muscle atrophy, and lumbar facet joints degeneration in patients with lumbar disc herniation. BMC Musculoskelet Disord. (2017) 18:167. doi: 10.1186/s12891–017-1522–4
100. Cheng Z, Li Y, Li M, Huang J, Huang J, Liang Y, et al. Correlation between posterior paraspinal muscle atrophy and lumbar intervertebral disc degeneration in patients with chronic low back pain. Int Orthopaedics (SICOT). (2023) 47:793–801. doi: 10.1007/s00264–022-05621–9
101. Freeman MD, Woodham MA, Woodham AW. The role of the lumbar multifidus in chronic low back pain: A review. PM&R. (2010) 2:142–6. doi: 10.1016/j.pmrj.2009.11.006
102. Saleem S, Aslam HM, Rehmani MAK, Raees A, Alvi AA, Ashraf J. Lumbar disc degenerative disease: disc degeneration symptoms and magnetic resonance image findings. Asian Spine J. (2013) 7:322. doi: 10.4184/asj.2013.7.4.322
Keywords: low back pain, intervertebral disc degeneration, paraspinal muscles, fat infiltration, interplay mechanisms
Citation: Jiang J, Huang Y and He B (2024) Advances in the interaction between lumbar intervertebral disc degeneration and fat infiltration of paraspinal muscles: critical summarization, classification, and perspectives. Front. Endocrinol. 15:1353087. doi: 10.3389/fendo.2024.1353087
Received: 09 December 2023; Accepted: 10 June 2024;
Published: 24 June 2024.
Edited by:
Changjun Li, Central South University, ChinaReviewed by:
Mario Ganau, Oxford University Hospitals NHS Trust, United KingdomManuel De Jesus Encarnacion Ramirez, Peoples’ Friendship University of Russia, Russia
Andrei Tica, University of Craiova, Romania
Copyright © 2024 Jiang, Huang and He. This is an open-access article distributed under the terms of the Creative Commons Attribution License (CC BY). The use, distribution or reproduction in other forums is permitted, provided the original author(s) and the copyright owner(s) are credited and that the original publication in this journal is cited, in accordance with accepted academic practice. No use, distribution or reproduction is permitted which does not comply with these terms.
*Correspondence: Bo He, hebo_ydyy@qq.com