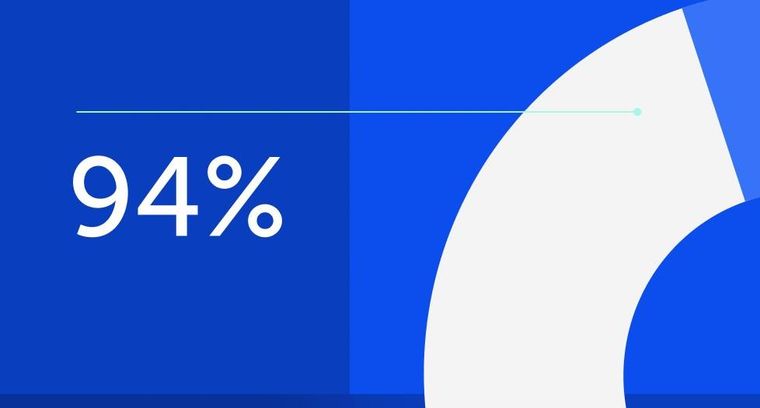
94% of researchers rate our articles as excellent or good
Learn more about the work of our research integrity team to safeguard the quality of each article we publish.
Find out more
REVIEW article
Front. Endocrinol., 12 March 2024
Sec. Bone Research
Volume 15 - 2024 | https://doi.org/10.3389/fendo.2024.1340625
This article is part of the Research TopicOrgan System Crosstalk in Degenerative Musculoskeletal DiseasesView all 11 articles
The intervertebral disc is not isolated from other tissues. Recently, abundant research has linked intervertebral disc homeostasis and degeneration to various systemic diseases, including obesity, metabolic syndrome, and diabetes. Organokines are a group of diverse factors named for the tissue of origin, including adipokines, osteokines, myokines, cardiokines, gastrointestinal hormones, and hepatokines. Through endocrine, paracrine, and autocrine mechanisms, organokines modulate energy homeostasis, oxidative stress, and metabolic balance in various tissues to mediate cross-organ communication. These molecules are involved in the regulation of cellular behavior, inflammation, and matrix metabolism under physiological and pathological conditions. In this review, we aimed to summarize the impact of organokines on disc homeostasis and degeneration and the underlying signaling mechanism. We focused on the regulatory mechanisms of organokines to provide a basis for the development of early diagnostic and therapeutic strategies for disc degeneration.
Intervertebral disc degeneration (IVDD) is the main contributor to the development of low back pain, leading to a remarkable loss of disability-adjusted life years as well as a substantial economic burden on society (1, 2). Healthy discs are cartilaginous structures that contribute one-third of the spine height and act as “elastic cushions” providing essential support, absorbing mechanical stress through compression, and providing flexibility. At the core of discs lies the gel-like nucleus pulposus (NP), which is surrounded by a concentric layer-arranged annulus fibrosus (AF) and two semi-rigid thin cartilage endplates (CEPs) that lie beneath the adjacent vertebrae. Various biological processes, including inflammation modulation, prevention of neovascularization, cell homeostasis, and matrix metabolism balance, are essential for preserving the disc homeostasis (3). IVDD, which is characterized mainly by persistent inflammation and matrix metabolism imbalance, refers to the progressive deterioration of the disc structure, leading to disc herniation, disc height loss, and nerve compression (3). The therapeutic options for IVDD are limited due to a poor understanding of the underlying mechanisms.
The healthy disc is not isolated from other tissues, despite having long been known as a unique organ without blood vessels, nerves, or immune cell infiltration (4). An increasing number of studies have shown that organokines, the bioactive factors secreted by diverse tissues, may have a vital impact on disc homeostasis. The expression of organokines can be induced by several factors, including physical activity, diet, aging, and metabolic alterations like obesity and diabetes (5, 6). Through autocrine, paracrine, or endocrine mechanisms, organokines have been linked to several inflammatory diseases, such as rheumatoid arthritis (7, 8). However, the role of organokines in IVDD is not completely understood.
In this review, we aimed to summarize the molecular and biochemical characteristics of organokines from specific tissues and their association with disc homeostasis and degeneration. The organokines treated in this review include adipokines, osteokines, myokines, cardiokines, gastrointestinal hormones, and hepatokines. Common hormones, growth factors, cytokines, and chemokines are excluded (Figure 1). Organokines play regulatory roles in cellular behavior, inflammation, and matrix metabolism in intervertebral disc homeostasis by binding to their receptors and activating downstream signaling pathways. We hope that this review will deepen the understanding of IVDD in the view of organ crosstalk and pave the way for the development of novel therapeutic interventions.
Figure 1 Diagrams illustrate that organokines, including adipokines, osteokines, myokines, cardiokines, gastrointestinal hormones and hepatokines, mediates the cross-organ regulation of disc homeostasis under physiological and pathological conditions from the major tissues of endocrinory ability. OPG, osteoprotegerin; DKK-1, dickkopf-1; PTHrP, Parathyroid Hormone-Related Protein; SPARC, Secreted protein acidic and rich in cysteine; BMPs, Bone morphogenetic proteins; ANP, atrial natriuretic peptide; GLP-1, Glucagon-like peptide-1; ANGPTLs, angiopoietin-like proteins; FSTL-1, Follistatin-like-1; LCN-2, lipocalin-2. Graphic elements were created using biorender.com.
Obesity, characterized by excessive adipose tissue, has been recognized as a significant risk factor for disc degeneration (9). In recent decades, adipose tissue has been considered as an endocrine organ that secretes various bioactive factors named adipokines (10) (Figure 2, Table 1). The cell-signaling proteins, such as leptin, adiponectin, and progranulin (PGRN) are secreted from adipose tissues and act like cytokines in the obesity-related impact on non-adipose tissues (33, 34). Research suggests that adipokine signaling is involved in the regulation of intervertebral disc homeostasis by several conditions, including disc tissue disruption by vertebral osteomyelitis, disc inflammation by ectopic adipose tissue infiltration, and osteonectin deletion-induced disc degeneration in mice (35–38).
Figure 2 Schematic plots illustrate the signaling mechanism of various adipokines in intervertebral disc cells. Leptin, binding to LepR, activates JAK-2/STAT-3, MAPK, ERK1/2, RhoA/ROCK/LIMK/Cofilin-2 pathways, promoting disc degeneration. Adiponectin, binding to AdipoR1/2, activates AMPK, inhibiting the NF-κB pathway to exert a protective effect. Progranulin activates TNFR1/2, inhibiting NF-κB, ERK1/2, and Wnt/β-catenin pathways, providing a protective effect. Resistin, binding to TLR-4, inhibits NF-κB or MAPK pathways, exhibiting a protective effect. Chemerin, binding to CMKLR1 or TLR-4, activates the NF-κB pathway, promoting disc degeneration. Visfatin, binding to Insulin receptors (InsulinR) or TLR-4, activates JNK, MAPK, ERK1/2, and NF-κB pathways, promoting disc degeneration. However, the intracellular NAMPT activity of Visfatin delivered by Adipo-sEV could promote NAD+ biogenesis and SIRT activity, exerting a protective effect. Omentin-1 activates PI3K/Akt and SIRT1 pathways, providing a protective effect, though its receptor remains incompletely understood. Graphic elements were created using biorender.com.
Leptin is a peptide hormone that is mainly synthesized in white adipose tissue and plays a regulatory role in energy metabolism and body weight. Beyond enhancing energy consumption in target cells, leptin can promote the production of pro-inflammatory cytokines, underlying the inflammatory and painful impacts of obesity. Both the leptin protein and the leptin receptor (LepR) have been detected in discs and are positively correlated with age and degeneration severity (11). While leptin can induce osteogenic differentiation in CEPs (17), the levels of leptin and its receptors increase with matrix metalloproteinase (MMP) and cytokine levels in the AF and NP of degenerative discs (12, 16, 39). Mechanistically, leptin can drive matrix catabolism via the Janus kinase-2 (JAK-2)/signal transducer and activator of transcription-3 (STAT-3) and mitogen-activated protein kinase (MAPK) pathways (13, 40). Additionally, leptin activates the ras homolog gene family member A (RhoA)/rho-associated coiled-coil containing protein kinase (ROCK) pathway and cytoskeletal remodeling in response to mechanical signals (15, 41). Although these findings suggest leptin has detrimental effects, whole-body leptin receptor knockout mice display delayed cellular proliferation and differentiation, elevated MMP-3 levels, and higher apoptosis rates, leading to IVDD (14, 18). Moreover, LepR has been identified as a lineage marker and fate modulator of notochord-derived cells at perinatal stages (42). Therefore, the potential fundamental role of leptin–LepR interactions in IVDD requires further exploration.
Adiponectin, a glycoprotein that is uniquely expressed by adipocytes, could maintain energy balance and suppress inflammation or apoptosis in various tissues by binding to adiponectin receptors (AdipoR1/2). However, the role of adiponectin and AdipoRs in IVDD is unclear. Previous studies showed that adiponectin expression in degenerative discs was decreased or absent while AdipoR1 and AdipoR2 expression increased or decreased with the Pfirrmann grade of degenerative discs (22, 43). However, plasma adiponectin levels were found to be increased in IVDD patients (44). Recently, administration of the AdipoR agonist AdipoRon was found to effectively reduce the levels of the pro-inflammatory factor tumor necrosis factor α (TNF-α) and mitigate disc degeneration (21). In future research, the exact role of adiponectin–AdipoR interactions in IVDD needs to be clarified.
PGRN, a secreted glycoprotein that can be cleaved into granulins by enzymes like elastase, exerts anti-inflammatory effects and plays protective roles by enhancing cell proliferation and through interacting with TNF receptors (TNFRs) or other receptors. Although higher PGRN levels are associated with higher degeneration severity in IVDD patients, current evidence suggests a protective role for PGRN in disc degeneration and aging (3, 45). Knockdown of PGRN in aged mice accelerates disc degeneration by promoting matrix catabolism and cellular dysfunction in AF and CEPs (29). Mechanistically, PGRN competitively binds to TNFR-1, thereby inhibiting the expression of the pro-inflammatory factor interleukin-17 (IL-17) and inflammatory and catabolic pathways (30, 45). Moreover, PGRN promotes anabolism and the production of the anti-inflammatory factor IL-10 via binding to TNFR-2 (28, 30). Additionally, PGRN and its derivatives, like atsttrin, inhibit epoxide synthase-2, IL-6, IL-17, and MMP-13 production, thereby inhibiting IVDD progression (29, 30).
Resistin is a cysteine-rich polypeptide that is secreted by white adipocytes and is involved in insulin resistance. In agreement with the devastating effect of diabetes on IVDs, recent research indicates resistin’s involvement in IVDD for its pro-inflammatory properties (46). By targeting Toll-like receptor-4 (TLR-4), resistin activates the nuclear factor kappa-light-chain-enhancer of activated B cells (NF-κB) signaling to increase the expression of the macrophage inflammatory protein chemokine C-C motif ligand 4 (CCL4), thereby fostering macrophage infiltration into discs (19). In addition, resistin triggers inflammatory cascades through the activation of the MAPK and NF-κB pathways, which increases the NLR family pyrin domain containing 3 protein (NLRP3) inflammasomes and the expression levels of IL-1β, IL-6, IL-8, and MMPs in discs (20, 47).
Chemerin is an obesity-associated adipokine and is involved in various processes including inflammation by interacting with chemokine-like receptor 1 (CMKLR1). The expression levels of chemerin and CMKLR1 are increased in degenerative NP tissues, especially those of obese individuals (31). Furthermore, the administration of chemerin results in inflammation and tissue degeneration, while CMKLR1 knockdown could slow the progression of needle-induced disc degeneration in rats (31). Furthermore, chemerin exerts pro-senescent and pro-inflammatory effects on NP cells through binding to TLR-4, a well-known receptor activating the NF-κB signaling cascade (31, 48).
Visfatin, identified as the extracellular form of nicotinamide-phosphate ribosyl transferase (NAMPT), has been known to mediate insulin resistance and inflammation via binding to the insulin receptor or the innate immune receptor TLR-4. Visfatin could induce IL-6 expression and disc degeneration by activating the MAPK pathway, which participates in the inflammatory response (26). In addition, pharmacological inhibition or knockdown of visfatin resulted in the maintenance of metabolism balance by enhancing autophagy in the presence of IL-1β (24). Interestingly, a recent study showed that NAMPT was delivered in small extracellular vesicles derived from adipocytes (Adipo-sMV) and mediated the protective impact of Adipo-sMV through increased nicotinamide adenine dinucleotide (NAD) and NAD-dependent deacetylase sirtuin-1 (SIRT1) activity in senescent NP and CEP cells (23). Considering the lack of a secretion signal sequence, visfatin/NAMPT may play a multifaceted role dependent on its location: serving as the rate‐limiting enzyme for NAD+ biosynthesis in the cytosol or binding receptors on the cellular surface after leakage into the extracellular space.
Omentin-1, an anti-inflammatory adipokine, exhibits anti-inflammatory and antioxidant properties. Its expression level is inversely correlated with the progression of various diseases, including diabetes, obesity, and osteoarthritis (49, 50). Recent studies showed that omentin-1 could protect NP cells from ongoing senescence, inflammation, apoptosis, or matrix metabolism imbalance in the presence of IL-1β through activating SIRT1 or the phosphoinositide 3-kinase (PI3K)/protein kinase B (PKB, also known as Akt) signaling pathway (32, 49). Therefore, it is valuable to further investigate its in vivo therapeutic potential in IVDD treatment.
Osteokines are a category of proteins predominantly secreted in bone and can have a significant influence on the homeostasis of bone and extraosseous organs (51–53). The interplay between bone homeostasis regulation and disc degeneration is becoming increasingly recognized. Indeed, the osteogenic potential of discs increases with the progression of degeneration, evidenced by elevated osteogenic differentiation of AF and CEP cells (54, 55). Then, intradiscal ectopic ossifications or calcifications can result in increased tissue stiffness, thereby provoking inflammation, disc degeneration, and low back pain (56–59). Additionally, structural alterations of vertebral bone, such as Modic changes (also known as magnetic resonance imaging [MRI] signal intensity changes in vertebral bone marrow) and vertebral osteoporosis, have been identified as associated with the development of IVDD (60, 61). Therefore, it is imperative to investigate the precise role of these osteokines (Figure 3, Table 2) in the pathophysiology of disc degeneration.
Figure 3 Schematic plots illustrate the signaling mechanism of various osteokines in intervertebral disc cells. PTHrP, binding to PTHR, could activate MAPK or PKA/CREB/Hedgehog pathways to protect disc from degeneration. Sclerostin binds to LRPs to activate Wnt/β-catenin pathway and matrix anabolism, while inhibits the expression of DKK-1 that inhibits Wnt/β-catenin pathway. Osteoprotegerin inhibits the RANK-RANKL interactions to protect disc from degeneration, while the intracellular signaling pathway is unknown. BMP-2,4,7,14(GDF5) binds BMPR-I/II to activate PI3K/Akt, Smads pathway and inhibit PUMA expression to protect disc from degeneration. Periostin could interact with Integrin receptors, NF-κB pathway and Wnt/β-catenin pathway to promote disc degeneration. Osteonectin/SPARC could modulate TLR-4, CXCR1/2, or GFs-GFRs interactions to protect disc from degeneration. Graphic elements were created using biorender.com.
OPG is a typical osteokine recognized as the regulator of bone mass and the receptor activator of NF-κB (RANK)/RANK ligand (RANKL) pathway, while it exists in various extraosseous tissues including discs. Various studies have shown a significant correlation between the levels of OPG in serum or disc samples and degeneration severity (62, 63, 81, 82). Further, OPG gene polymorphisms and increased OPG expression levels may contribute to IVDD development (81). OPG and RANK/RANKL expression could be upregulated with increased catabolism in AF, NP, or CEP cells exposed to acidic microenvironments or the inflammatory factor IL-1β (63, 64). However, OPG knockout results in osteoclast-mediated cartilage erosion, leading to disorganized alignment of CEPs, enhanced bone formation or neovascularization, and elevated inflammatory factors in mice (65–67). Thus, the multifaceted role of OPG in disc homeostasis highlights that further research is needed to elucidate its mechanism in IVD biology.
Sclerostin and DKK-1 are a pair of physical activity-related osteokines that competitively bind to the Wnt coreceptors lipoprotein receptor-related proteins (LRPs) and mediate the crosstalk between bone and other organs. Recently, sclerostin and DKK-1 have been shown to be involved in spinal pathological conditions, including spinal ligament ossification, spondylarthritis, and disc calcification (66, 67, 83). A recent study illustrated the compensatory increase in DKK-1 levels and the suppression of the Wnt/β-catenin pathway in sclerostin-depleted murine discs, and the administration of antibodies against sclerostin or DKK-1 exhibited beneficial effects on proteoglycan content, disc hydration, and height (84). Considering the complex role of Wnt signaling in disc development and degeneration, it is needed to clarify the exact roles and determinants of these Wnt inhibitors in IVDD (85).
PTHrP, first discovered in malignancy-associated hypercalcemia, has been recognized as an osteokine acting in a paracrine manner on bone and other tissues through binding to the PTH-1 receptor (PTH-1R). PTHrP is involved in intervertebral disc maturation and calcification, delays cellular mineralization and hypertrophy in Col IX knockout mice, and inhibits progressive kyphoscoliosis in fibroblast growth factor receptor-3 (FGFR-3) knockout mice (83–85). By enhancing Hedgehog, transforming growth factor beta (TGF-β), Wnt/β-catenin, mammalian target of rapamycin (mTOR), and MAPK/protein kinase A (PKA) signaling, PTH-1R activation by PTH administration plays a protective role in NP cell activity and disc homeostasis (69–72, 74). Considering the elevated PTH-1R expression in NP cells, the role of PTHrP–PTH-1R interactions in IVDD ought to be elucidated in future research (73, 86).
BMPs are osteokines participating in the formation and maintenance of bone and various non-bone tissues, including cartilage (51, 87). Various studies confirmed the presence of BMPs, including BMP-2, 4, 7, and 14 (also known as growth differentiation factor -5 [GDF-5]), with their receptors (BMPR-I/II) in the IVD (88–92). Mechanistically, BMP-2 and BMP-7 activate various signaling pathways, including the Smad/Puma and PI3K/Akt signaling pathways, to inhibit NP cell apoptosis or senescence (75, 76). Additionally, GDF-5 deficiency in mice results in notable matrix abnormalities and disc degeneration, which could be substantially restored by treatment with recombinant human GDF5 (93, 94). Due to their anti-inflammatory and pro-regenerative effects, recombinant human BMPs are used for bone grafting in vertebral fusion surgery as well as disc tissue engineering (95, 96).
Osteonectin, also known as secreted protein acidic and rich in cysteine (SPARC), is one of the most abundantly expressed non-collagenous proteins in mineralized tissues as well as non-mineralized tissues and orchestrates inflammation and tissue remodeling through binding to TLR-4, BMPRs, integrin receptors, and various growth factors. SPARC expression in human disc cells decreases with age and disc degeneration (97). Moreover, SPARC-deficient mice exhibit spontaneous disc degeneration and lower back pain, evidenced by an age-dependent increase in neuron markers like calcitonin gene-related peptide and Neuropeptide-Y within the discs and peripheral nerves (78, 98, 99). Additionally, these mice demonstrate a diminished lumbar neutral zone, increased spinal stiffness, and reduced spinal mobility (100). SPARC knockout results in elevated levels of inflammatory mediators and vascular endothelial growth factor, which can be mitigated by interventions like exercise and treatment with TAK-242 (a TLR-4 antagonist) or reparixin (an inhibitor of CXC chemokine receptors [CXCR1/2]) (80, 101–103). Therefore, SPARC is a promising target for preventing IVDD in modulating cell–matrix interactions and governing neural, immune, and inflammatory pathways (79, 104, 105).
Periostin is a bone turnover-related osteokine that is highly expressed in collagen-rich tissue—including periosteum—and mediates tissue remodeling through binding to integrin receptors and proteoglycans. In human and rat discs, periostin levels gradually decrease from the outer AF to the central NP and increase with degeneration development (106–108). Mechanistically, periostin promotes NP cell apoptosis via the Wnt/β-catenin signaling pathway and cellular senescence via the NF-κB pathway, contributing to the development of IVDD (109, 110). Considering its role as a matricellular protein, further investigation is needed to elucidate whether periostin participates in the regulation of disc cell–matrix interactions (111).
Lipocalin-2 (LCN-2), a glycoprotein secreted by osteoblasts and adipocytes, functions as a pro-inflammatory factor in obesity-related metabolic disorders, despite our limited understanding of the potential LCN-2 receptors (112, 113). A recent study suggested a correlation between LCN-2 and the expression of inflammation-related genes in human discs (114). Moreover, upregulated expression of LCN-2 has been validated to increase MMP-9 activity in AF cells (115). Considering that LCN-2 could function as a biomechanical and inflammatory sensor in bone–cartilage crosstalk, its specific role in IVDD needs to be elucidated (9).
Fibroblast growth factor-23 (FGF-23) is the first identified osteokine that can bind to the tyrosine kinase FGF receptors (FGFRs) to regulate phosphate and vitamin D metabolism (116). However, direct evidence linking FGF-23 to IVDD is currently lacking. Klotho, a crucial cofactor for FGF-23 in the activation of FGFRs, mitigates inflammation in NP cells and counteracts extracellular matrix degradation in IVDD (117, 118). Accordingly, the role of FGF-23 in IVD homeostasis, potentially analogous to that of Klotho, presents an intriguing avenue for further investigation.
Similar to adipose tissue and bone, skeletal muscle and cardiac muscle can function as endocrine organs and secrete tissue-specific hormones, termed myokines and cardiokines, respectively (119) (Figure 4, Table 3). It is well recognized that these molecules mediate cross-organ crosstalk beyond the muscle tissue itself and orchestrate the multi-tissue response to physical activity and other stress (112, 113, 119, 136). Given the emerging link between muscle activity and IVDD progression, the roles of myokines and cardiokines in IVDD deserve more attention and in-depth investigation.
Figure 4 Schematic plots illustrate the signaling mechanism of other organokines in intervertebral disc cells. Irisin binds Integrin receptors to activate autophagy and inhibit LATS/YAP pathway to protect disc from degeneration. Apelin, binding APJ receptors to activate PI3K/Akt pathway and induce TFEB-mediated autophagy to protect disc from degeneration. FSTL-1, binding TLR-4, DIP2A, BMPRs or Wnt receptors, could activate MAPK, JNK, ERK1/2, NF-κB and Smad pathway to promote disc degeneration. Additionally, the FSTL-1 deficiency inhibit the maintenance of disc homeostasis. ANP binds NPR to protect cell from apoptosis and oxidative damage by activating NRF2/HO-1 pathway. Ghrelin binds GHSR to activate Akt and inhibit NF-κB pathways to protect disc from degeneration. GLP-1 or its analogs, binding to GLP-1R, activate PI3K/Akt/mTOR, PI3K/Akt/GSK3β, as well as inhibiting MAPK/AP-1 pathways to protect disc from degeneration. Amylin binds RAMPs or CTR to activate PI3K/Akt/mTOR pathway and inhibits MAPK/AP-1 pathways to protect disc from degeneration. Meanwhile, Amylin aggregates could inhibit IL-1β/IL1Ra interactions. ANGPTL4/8 could bind Integrin receptors or Ig-like receptors to activate NF-κB pathway to promote disc degeneration. Graphic elements were created using biorender.com.
Irisin is a well-characterized myokine derived from fibronectin type III domain-containing protein 5 (FNDC5). It mediates the health benefits of exercise by binding with integrins. Exercise elevated irisin levels in plasma and NP tissue and FNDC5/irisin knockout abolished the protective effects of exercise against IVDD in a murine model (114). By activating autophagy or large tumor suppressor kinase (LATS)/yes-associated protein (YAP)/connective tissue growth factor (CTGF, also known as CCN2) signaling, irisin can help maintain cellular activity and matrix metabolism balance and inhibit inflammatory effects, thereby decelerating the progression of IVDD (115, 117).
Myostatin (also known as GDF8) functions as a negative regulator of skeletal muscle growth. It binds to activin receptors (ACVRs) and can be expressed in back muscles after IVD injury (118, 137). However, the role of myostatin in IVDD is incompletely understood. Myostatin plays an inhibitory role in cartilage formation and chondrocyte proliferation, and its serum levels exhibit a positive correlation with the severity of conditions such as osteoarthritis and rheumatoid arthritis (121, 138). Additionally, ACVR1 silencing reversed lipopolysaccharide-induced inflammation and matrix degradation in NP cells, implying the potential unfavorable impacts of ACVR1 activation by myostatin upon discs (120). However, earlier studies indicated the fundamental role of myostatin in disc homeostasis. Myostatin deficiency in mice resulted in increased muscle weight, accompanied by endplates ossification at the L4–L5 level and a notable reduction in proteoglycan content in the endplates and inner AF (122, 139). Therefore, more comprehensive research is needed to elucidate the potential mechanism underlying the multi-faceted role of myostatin–ACVRs interactions in IVDD.
Apelin, identified as the endogenous ligand for the G-protein coupled receptor APJ, plays a regulatory role across diverse tissues including skeletal muscle and the cardiovascular system. Apelin and its receptor APJ are downregulated in degenerative NP tissue (123, 124). Moreover, administration of apelin results in suppressed matrix degradation, apoptosis, and inflammation in the presence of IL-1β and increased matrix anabolism in the presence of the oxidative stress inducer H2O2 (123, 124). Mechanistically, apelin enhances the PI3K/Akt pathway and transcription factor EB (TFEB)-mediated autophagy flux in NP cells (123, 124). Considering the significant role of apelin in exercise-induced benefits, exploring whether and how apelin participates in muscle–disc crosstalk is valuable (140).
Follistatin-like-1(FSTL-1) is a kind of myokine and cardiokine modulating immune responses, cell proliferation, and differentiation through binding to TLR-4, Wnt receptors, and various growth factors. FSTL-1 has an adverse effect on disc homeostasis, accompanied by increased concentrations in the serum of IVDD patients, discs of rats with IVDD, and the cerebrospinal fluid of dogs with IVDD (125, 141). Mechanistically, FSTL-1 promotes NP cell inflammation by activating the MAPK, Smads, or NF-κB signaling pathway (125, 141). Interestingly, the knockout of FSTL-1 during embryonic development leads to a decrease in vertebral cartilage and matrix anabolism, indicating its fundamental role in early IVD formation (142). Moreover, FSTL-1 may play diverse roles in disc development and maturation, given that it could mediate the differentiation of pre-cartilaginous stem cells into NP-like cells (143).
As a typical cardiokine, ANP binds to natriuretic peptide receptors (NPRs) to induce diuretic, natriuretic, and vasodilating effects and regulate the renin–angiotensin–aldosterone system (144). NPR mutations can result in impaired cartilage development, potentially leading to secondary degenerative changes and suboptimal joint development (145, 146). Recent studies indicated that ANP inhibited oxidative damage and cell death in endplates by activating the nuclear factor erythroid 2-related factor 2 (Nrf2)/Heme oxygenase-1 (HO-1) signaling pathway (128). Additionally, given the presence and adverse effects of the local tissue renin–angiotensin system (tRAS) in discs, whether ANP has a protective impact via suppressing the tRAS in IVDD is an interesting avenue for future research (147–149).
In recent years, the interplay between the digestive system and disc homeostasis has received increasing attention, with a special focus on the role of the gut microbiota in the gastrointestinal endocrine system (150–153). It is worth noting that the endocrine functions of the digestive system facilitate complex inter-organ communication through various gastrointestinal hormones (such as Ghrelin and Amylin) and hepatokines (Figure 4, Table 3) (154, 155). Insight into how these endocrine factors influence disc physiology can expand our understanding of IVDD.
Ghrelin is a circulating brain–gut peptide hormone that promotes growth hormone secretion via binding to the growth hormone secretagogue receptor (GHSR) and participates in the regulation of insulin resistance, obesity, and inflammation. Ghrelin was found present in the NP tissue, and ghrelin administration demonstrated a protective effect in a rabbit IVDD model (132). Mechanistically, ghrelin suppresses IL-1β-induced catabolism and inflammatory cytokine production by inhibiting the NF-κB pathway, while promoting anabolism via Akt signaling (132).
Glucagon-like peptide-1(GLP-1), a peptide hormone secreted by intestinal L-cells, has broad pharmacological potential for managing type 2 diabetes mellitus and metabolic syndrome-related disorders by binding to its receptor -GLP-1R. GLP-1R activation leads to the inhibition of inflammation and apoptosis through downstream pathways including the PKA, PKC, and extracellular signal-regulated kinase 1/2 (ERK1/2) signaling pathways (156, 157). Notably, GLP-1R activation by liraglutide (a long-acting GLP-1 analog) has been shown to protect NP cells against hyperglycemia-induced apoptosis via the PI3K/Akt signaling pathway (134, 135). Administration of another GLP-1R agonist, exenatide, in discs promotes matrix synthesis and mitigates oxidative stress-induced matrix catabolism via inhibiting the activation of MAPK and activator protein-1 (AP-1) activity (133). Considering the therapeutic potential of GLP-1 activation, there is a need to further elucidate the role of endogenous GLP-1 in IVDD.
Amylin, also known as islet amyloid polypeptide (IAPP), is a peptide that is predominantly secreted by pancreatic islet β-cells and participates in the development of diabetes through receptor activity-modifying proteins (RAMPs) or the calcitonin receptor (CTR) to inhibit insulin and glucagon secretion. During IVDD progression, amylin and its receptors are downregulated in the NP and AF cells, while amylin aggregates accumulate in NP tissues (129–131). Amylin overexpression in NP cells can maintain matrix metabolism balance and control the autophagy–apoptosis crosstalk by the PI3K/Akt/mTOR and MAPK signaling pathways (130). Meanwhile, these protective effects could be augmented by neutralizing IL-1β/IL-1 receptor antagonist (IL-1Ra) signaling induced by amylin aggregation (129). Furthermore, the amylin analog pramlintide showed the ability to relieve matrix metabolism impairment and enhance cell survival via a mitochondrial-dependent apoptotic pathway in NP cells (158). Additionally, amylin activates Akt/mTOR signaling to protect AF cells from death through the death receptor Fas/FasL and the mitochondrial-dependent apoptotic pathway (131).
Hepatokines, such as angiopoietin-like proteins (ANGPTLs) and fetuin-A (also known as α2-HS-glycoprotein), are hormone-like proteins secreted by hepatocytes (159). ANGPTLs act as modulators of lipid metabolism, angiogenesis, and inflammation via binding to integrin receptors and immunoglobulin-like receptors. However, their roles in regulating disc homeostasis are poorly understood. Recent research illustrated the correlation between the upregulation of ANGPTL4/8 and the severity of disc degeneration (160, 161). Mechanistically, ANGPTL4/8 appears to promote matrix degradation and the production of inflammatory cytokines like TNF-α through the activation of the NF-κB signaling pathway (161, 162). Fetuin-A functions as an indirect inhibitor of ectopic mineralization and inflammation. Recent studies demonstrated that intra-articular injection of fetuin-A derivatives leads to improved osteoarthritis scores and mobility in a rat osteoarthritis model (163). Thus, it is worthwhile to explore the role of fetuin-A in IVDD.
Organokines, serving as the potential communicator between the extra discal tissues and the disc, participate in pathological processes such as cell death, inflammation, and matrix loss, thereby contributing to IVDD onset and progression. Current evidence suggests that the release and interactions of organokines could be regulated by multiple factors, making their impact challenging to quantify. Lifestyle factors such as exercise, diet, stress, sleep, and microbiome profoundly influence organokines production, affecting disc homeostasis and susceptibility to IVDD-related diseases (6, 164–166). Exercise, known as beneficial for IVD homeostasis, can modulate the release and activity of organ factors like irisin, ANGPTL4, osteocalcin, and adiponectin (113, 167). Notedly, acute exercise can fast change levels of myokines, hepatokines, osteokines, and immune cytokines, while long-term training alters baseline adipokines (113, 167). For instance, exercise normalizes leptin and lowers resistin, reducing inflammation and insulin resistance, which may help protect against IVDD (113). Considering the individual variability in response, further research is essential to explore pharmacological mimics of exercise on organokines modulation for IVDD treatments.
Diet type or pattern have potential protective effects on disc homeostasis and degeneration, taking Dietary supplements such as n-3 fatty acids (FAs) and bioactive dietary polyphenol preparations (BDPP) for example (168, 169). Interestingly, recent studies indicate that dietary patterns and types are closely related to adipokine secretion (170). Mediterranean, low-fat, and low-carbohydrate diets have been found associated with decreased levels of leptin and vaspin and increased adiponectin (170). Leptin and vaspin may adversely affect disc homeostasis maintenance, while the role of adiponectin remains controversial. Therefore, future research may focus on identifying whether the secretion type, quantity, and activity of organokines underly the links between diet types, patterns, or nutritional supplements and disc homeostasis.
A variety of organokines from adipose, bone, muscle, or digestive tissues play an adverse or protective role in intervertebral disc homeostasis. Most studies have focused on the impact on cells or tissue of single origin and have not considered overall disc or extra discal dynamics. Functional studies using cell cultures and animal models are encouraged to comprehensively evaluate the role of organokines in IVDD, especially cross-organ communication. The impact and detailed mechanisms of organokines-mediated interactions warrant further investigation under both physiological and pathological conditions.
Future research should prioritize developing pharmacological agents or biologics designed to modulate organokines activity, agonists or antagonists for receptors of organokines and inhibitors for organokines signaling pathways for potential clinical applications. Current investigations into the regulation of organokines by exercise, diet, and stress predominantly rely on in vitro or animal models. Moreover, it is essential to elucidate which organokines paly dominant roles on disc cell homeostasis and matrix metabolism balance. Consequently, future studies need to be more holistic, examining the impact of specific lifestyle choices on the entire spectrum of organokines, ideally assessing both local disc tissue and systemic levels. Given that many aspects of these molecules in humans remain under-explored or contentious—such as their in vivo half-life, protein binding in circulation, effective concentration in disc tissues, receptor interactions, and overall impact on disc health—clinical trials face a considerable journey ahead.
YH: Data curation, Investigation, Visualization, Writing – original draft, Writing – review & editing. SL: Investigation, Visualization, Writing – original draft, Writing – review & editing. HL: Funding acquisition, Investigation, Resources, Writing – review & editing. FD: Conceptualization, Funding acquisition, Supervision, Writing – review & editing, Project administration. ZS: Conceptualization, Project administration, Resources, Supervision, Writing – review & editing. LX: Conceptualization, Project administration, Resources, Supervision, Writing – review & editing.
The author(s) declare financial support was received for the research, authorship, and/or publication of this article. The current work was partially funded by the National Natural Science Foundation of China (82202765, 82160434) and the National Natural Science Foundation of Hubei Province (2022CFB343).
The authors declare that the research was conducted in the absence of any commercial or financial relationships that could be construed as a potential conflict of interest.
All claims expressed in this article are solely those of the authors and do not necessarily represent those of their affiliated organizations, or those of the publisher, the editors and the reviewers. Any product that may be evaluated in this article, or claim that may be made by its manufacturer, is not guaranteed or endorsed by the publisher.
1. Global burden of 369 diseases and injuries in 204 countries and territories, 1990-2019: A systematic analysis for the global burden of disease study 2019. Lancet. (2020) 396(10258):1204–22. doi: 10.1016/s0140-6736(20)30925-9
2. Hartvigsen J, Hancock MJ, Kongsted A, Louw Q, Ferreira ML, Genevay S, et al. What low back pain is and why we need to pay attention. Lancet. (2018) 391(10137):2356–67. doi: 10.1016/S0140-6736(18)30480-X
3. Francisco V, Pino J, González-Gay M, Lago F, Karppinen J, Tervonen O, et al. A new immunometabolic perspective of intervertebral disc degeneration. Nat Rev Rheumatol. (2022) 18(1):47–60. doi: 10.1038/s41584-021-00713-z
4. Feng P, Che Y, Gao C, Zhu L, Gao J, Vo NV. Immune exposure: How macrophages interact with the nucleus pulposus. Front Immunol. (2023) 14:1155746. doi: 10.3389/fimmu.2023.1155746
5. Lőrincz H, Somodi S, Ratku B, Harangi M, Paragh G. Crucial regulatory role of organokines in relation to metabolic changes in non-diabetic obesity. Metabolites. (2023) 13(2). doi: 10.3390/metabo13020270
6. Lim JY, Kim E. The role of organokines in obesity and type 2 diabetes and their functions as molecular transducers of nutrition and exercise. Metabolites. (2023) 13(9). doi: 10.3390/metabo13090979
7. Chung HS, Choi KM. Organokines in disease. Adv Clin Chem. (2020) 94:261–321. doi: 10.1016/bs.acc.2019.07.012
8. Laurindo LF, de Maio MC, Barbalho SM, Guiguer EL, Araújo AC, de Alvares Goulart R, et al. Organokines in rheumatoid arthritis: A critical review. Int J Mol Sci. (2022) 23(11). doi: 10.3390/ijms23116193
9. Ruiz-Fernández C, Francisco V, Pino J, Mera A, González-Gay MA, Gómez R, et al. Molecular relationships among obesity, inflammation and intervertebral disc degeneration: Are adipokines the common link? Int J Mol Sci. (2019) 20(8):2030. doi: 10.3390/ijms20082030
10. Scherer PE. Adipose tissue: From lipid storage compartment to endocrine organ. Diabetes. (2006) 55(6):1537–45. doi: 10.2337/db06-0263
11. Chen HH, Hsu HT, Liao MH, Teng MS. Effects of sex and obesity on lep variant and leptin level associations in intervertebral disc degeneration. . Int J Mol Sci. (2022) 23(20). doi: 10.3390/ijms232012275
12. Segar AH, Fairbank JCT, Urban J. Leptin and the intervertebral disc: A biochemical link exists between obesity, intervertebral disc degeneration and low back pain-an in vitro study in a bovine model. Eur Spine J. (2019) 28(2):214–23. doi: 10.1007/s00586-018-5778-7
13. Miao D, Zhang L. Leptin modulates the expression of catabolic genes in rat nucleus pulposus cells through the mitogen-activated protein kinase and janus kinase 2/Signal transducer and activator of transcription 3 pathways. Mol Med Rep. (2015) 12(2):1761–8. doi: 10.3892/mmr.2015.3646
14. Li X, Liu X, Wang Y, Cao F, Chen Z, Hu Z, et al. Intervertebral disc degeneration in mice with type II diabetes induced by leptin receptor deficiency. BMC Musculoskelet Disord. (2020) 21(1):77. doi: 10.1186/s12891-020-3091-1
15. Li Z, Liang J, Wu WK, Yu X, Yu J, Weng X, et al. Leptin activates Rhoa/Rock pathway to induce cytoskeleton remodeling in nucleus pulposus cells. Int J Mol Sci. (2014) 15(1):1176–88. doi: 10.3390/ijms15011176
16. Ding W, Zhao C, Cao L, Zhang K, Sun W, Xie Y, et al. Leptin induces terminal differentiation of rat annulus fibrosus cells Via activation of mapk signaling. Anat Rec (Hoboken). (2013) 296(12):1806–12. doi: 10.1002/ar.22806
17. Song Y, Li S, Geng W, Luo R, Liu W, Tu J, et al. Sirtuin 3-dependent mitochondrial redox homeostasis protects against ages-induced intervertebral disc degeneration. Redox Biol. (2018) 19:339–53. doi: 10.1016/j.redox.2018.09.006
18. Natelson DM, Lai A, Krishnamoorthy D, Hoy RC, Iatridis JC, Illien-Jünger S. Leptin signaling and the intervertebral disc: Sex dependent effects of leptin receptor deficiency and western diet on the spine in a type 2 diabetes mouse model. PloS One. (2020) 15(5):e0227527. doi: 10.1371/journal.pone.0227527
19. Li Z, Wang X, Pan H, Yang H, Li X, Zhang K, et al. Resistin promotes Ccl4 expression through toll-like receptor-4 and activation of the P38-mapk and nf-κb signaling pathways: Implications for intervertebral disc degeneration. Osteoarthritis Cartilage. (2017) 25(2):341–50. doi: 10.1016/j.joca.2016.10.002
20. Shin JH, Park S, Cho H, Kim JH, Choi H. Adipokine human resistin promotes obesity-associated inflammatory intervertebral disc degeneration Via pro-inflammatory cytokine cascade activation. Sci Rep. (2022) 12(1):8936. doi: 10.1038/s41598-022-12793-2
21. Ohnishi H, Zhang Z, Yurube T, Takeoka Y, Kanda Y, Tsujimoto R, et al. Anti-inflammatory effects of adiponectin receptor agonist adiporon against intervertebral disc degeneration. Int J Mol Sci. (2023) 24(10):8566. doi: 10.3390/ijms24108566
22. Terashima Y, Kakutani K, Yurube T, Takada T, Maeno K, Hirata H, et al. Expression of adiponectin receptors in human and rat intervertebral disc cells and changes in receptor expression during disc degeneration using a rat tail temporary static compression model. J Orthop Surg Res. (2016) 11(1):147. doi: 10.1186/s13018-016-0481-z
23. Sun Y, Li X, Yang X, Chen B, Zhang W. Small extracellular vesicles derived from adipocytes attenuate intervertebral disc degeneration in rats by rejuvenating senescent nucleus pulposus cells and endplate cells by delivering exogenous nampt. Oxid Med Cell Longevity. (2021) 2021:9955448. doi: 10.1155/2021/9955448
24. Shi C, Wu H, Du D, Im HJ, Zhang Y, Hu B, et al. Nicotinamide phosphoribosyltransferase inhibitor Apo866 prevents IL-1β-Induced human nucleus pulposus cell degeneration Via autophagy. Cell Physiol Biochem. (2018) 49(6):2463–82. doi: 10.1159/000493843
25. Huang Y, Peng Y, Sun J, Li S, Hong J, Zhou J, et al. Nicotinamide phosphoribosyl transferase controls Nlrp3 inflammasome activity through mapk and nf-κb signaling in nucleus pulposus cells, as suppressed by melatonin. Inflammation. (2020) 43(3):796–809. doi: 10.1007/s10753-019-01166-z
26. Cui H, Du X, Liu C, Chen S, Cui H, Liu H, et al. Visfatin promotes intervertebral disc degeneration by inducing IL-6 expression through the Erk/Jnk/P38 signalling pathways. Adipocyte. (2021) 10(1):201–15. doi: 10.1080/21623945.2021.1910155
27. Ding H, Wei J, Zhao Y, Liu Y, Liu L, Cheng L. Progranulin derived engineered protein atsttrin suppresses tnf-α-Mediated inflammation in intervertebral disc degenerative disease. Oncotarget. (2017) 8(65):109692–702. doi: 10.18632/oncotarget.22766
28. Wang S, Sun G, Fan P, Huang L, Chen Y, Chen C. Distinctive roles of tumor necrosis factor receptor type 1 and type 2 in a mouse disc degeneration model. J Orthop Translat. (2021) 31:62–72. doi: 10.1016/j.jot.2021.11.003
29. Zhao Y-P, Tian Q-Y, Liu B, Cuellar J, Richbourgh B, Jia T-H, et al. Progranulin knockout accelerates intervertebral disc degeneration in aging mice. Sci Rep. (2015) 5(1):9102. doi: 10.1038/srep09102
30. Wang S, Wei J, Fan Y, Ding H, Tian H, Zhou X, et al. Progranulin is positively associated with intervertebral disc degeneration by interaction with IL-10 and IL-17 through tnf pathways. Inflammation. (2018) 41:1852–63. doi: 10.1007/s10753-018-0828-1
31. Hu S, Shao Z, Zhang C, Chen L, Mamun AA, Zhao N, et al. Chemerin facilitates intervertebral disc degeneration Via Tlr4 and Cmklr1 and activation of nf-kb signaling pathway. Aging (Albany NY). (2020) 12(12):11732–53. doi: 10.18632/aging.103339
32. Huang X, Chen C, Chen Y, Xu J, Liu L. Omentin-1 alleviate interleukin-1β (IL-1β)-Induced nucleus pulposus cells senescence. Bioengineered. (2022) 13(5):13849–59. doi: 10.1080/21655979.2022.2084495
33. Sharma A. The role of adipokines in intervertebral disc degeneration. Med Sci. (2018) 6(2):34. doi: 10.3390/medsci6020034
34. Clemente-Suárez VJ, Redondo-Flórez L, Beltrán-Velasco AI, Martín-Rodríguez A, Martínez-Guardado I, Navarro-Jiménez E, et al. The role of adipokines in health and disease. Biomedicines. (2023) 11(5):1290. doi: 10.3390/biomedicines11051290
35. Lang S, Loibl M, Gläsner J, Simon M, Rupp M, Grad S, et al. Vertebral osteomyelitis is characterised by increased Rank/Opg and Rankl/Opg expression ratios in vertebral bodies and intervertebral discs. Eur Cells Materials. (2021) 2021(42):438–51. doi: 10.22203/eCM.v042a27
36. Wang Z, Zhao Z, Han S, Hu X, Ye L, Li Y, et al. Advances in research on fat infiltration and lumbar intervertebral disc degeneration. Front Endocrinol (Lausanne). (2022) 13:1067373. doi: 10.3389/fendo.2022.1067373
37. Kos K, Wilding JP. Sparc: A key player in the pathologies associated with obesity and diabetes. Nat Rev Endocrinol. (2010) 6(4):225–35. doi: 10.1038/nrendo.2010.18
38. James G, Millecamps M, Stone LS, Hodges PW. Dysregulation of the inflammatory mediators in the multifidus muscle after spontaneous intervertebral disc degeneration sparc-null mice is ameliorated by physical activity. Spine (Phila Pa 1976). (2018) 43(20):E1184–e94. doi: 10.1097/brs.0000000000002656
39. Hsu HT, Yue CT, Teng MS, Tzeng IS, Li TC, Tai PA, et al. Immuohistochemical score of matrix metalloproteinase-1 may indicate the severity of symptomatic cervical and lumbar disc degeneration. Spine J. (2020) 20(1):124–37. doi: 10.1016/j.spinee.2019.08.004
40. Li Z, Yu X, Liang J, Wu WK, Yu J, Shen J. Leptin downregulates aggrecan through the P38-adamst pathway in human nucleus pulposus cells. PloS One. (2014) 9(10):e109595. doi: 10.1371/journal.pone.0109595
41. Li Z, Shen J, Wu WK, Yu X, Liang J, Qiu G, et al. The role of leptin on the organization and expression of cytoskeleton elements in nucleus pulposus cells. J Orthop Res. (2013) 31(6):847–57. doi: 10.1002/jor.22308
42. Gao B, Yin J, Xu X, Fan J, Wang D, Zheng C, et al. Leptin receptor-expressing cells represent a distinct subpopulation of notochord-derived cells and are essential for disc homoeostasis. J Orthop Translat. (2020) 21:91–9. doi: 10.1016/j.jot.2019.11.005
43. Yuan B, Huang L, Yan M, Zhang S, Zhang Y, Jin B, et al. Adiponectin downregulates tnf-α expression in degenerated intervertebral discs. Spine. (2018) 43(7):E381–E9. doi: 10.1097/brs.0000000000002364
44. Khabour OF, Abu−Rumeh L, Al−Jarrah M, Jamous M, Alhashimi F. Association of adiponectin protein and adipoq gene variants with lumbar disc degeneration. Exp Ther Med. (2014) 8(4):1340–4. doi: 10.3892/etm.2014.1909
45. Toh H, Chitramuthu BP, Bennett HPJ, Bateman A. Structure, function, and mechanism of progranulin; the brain and beyond. J Mol Neurosci. (2011) 45(3):538–48. doi: 10.1007/s12031-011-9569-4
46. Mahmoud M, Kokozidou M, Auffarth A, Schulze-Tanzil G. The relationship between diabetes mellitus type II and intervertebral disc degeneration in diabetic rodent models: A systematic and comprehensive review. Cells. (2020) 9(10). doi: 10.3390/cells9102208
47. Liu C, Yang H, Gao F, Li X, An Y, Wang J, et al. Resistin promotes intervertebral disc degeneration by upregulation of adamts-5 through P38 mapk signaling pathway. Spine (Phila Pa 1976). (2016) 41(18):1414–20. doi: 10.1097/brs.0000000000001556
48. Zhang GZ, Liu MQ, Chen HW, Wu ZL, Gao YC, Ma ZJ, et al. Nf-κb signalling pathways in nucleus pulposus cell function and intervertebral disc degeneration. Cell Prolif. (2021) 54(7):e13057. doi: 10.1111/cpr.13057
49. Cabral VLF, Wang F, Peng X, Gao J, Zhou Z, Sun R, et al. Omentin-1 promoted proliferation and ameliorated inflammation, apoptosis, and degeneration in human nucleus pulposus cells. Arch Gerontology Geriatrics. (2022) 102:104748. doi: 10.1016/j.archger.2022.104748
50. Recinella L, Orlando G, Ferrante C, Chiavaroli A, Brunetti L, Leone S. Adipokines: New potential therapeutic target for obesity and metabolic, rheumatic, and cardiovascular diseases. Front Physiol. (2020) 11:578966. doi: 10.3389/fphys.2020.578966
51. Shimonty A, Bonewald LF, Huot JR. Metabolic health and disease: A role of osteokines? Calcif Tissue Int. (2023) 113(1):21–38. doi: 10.1007/s00223-023-01093-0
52. Han Y, You X, Xing W, Zhang Z, Zou W. Paracrine and endocrine actions of bone–the functions of secretory proteins from osteoblasts, osteocytes, and osteoclasts. Bone Res. (2018) 6(1):16. doi: 10.1038/s41413-018-0019-6
53. Kirk B, Feehan J, Lombardi G, Duque G. Muscle, bone, and fat crosstalk: The biological role of myokines, osteokines, and adipokines. Curr Osteoporosis Rep. (2020) 18(4):388–400. doi: 10.1007/s11914-020-00599-y
54. Jin L, Liu Q, Scott P, Zhang D, Shen F, Balian G, et al. Annulus fibrosus cell characteristics are a potential source of intervertebral disc pathogenesis. PloS One. (2014) 9(5):e96519. doi: 10.1371/journal.pone.0096519
55. Yuan FL, Xu RS, Ye JX, Zhao MD, Ren LJ, Li X. Apoptotic bodies from endplate chondrocytes enhance the oxidative stress-induced mineralization by regulating ppi metabolism. J Cell Mol Med. (2019) 23(5):3665–75. doi: 10.1111/jcmm.14268
56. Shao J, Yu M, Jiang L, Wei F, Wu F, Liu Z, et al. Differences in calcification and osteogenic potential of herniated discs according to the severity of degeneration based on pfirrmann grade: A cross-sectional study. BMC Musculoskelet Disord. (2016) 17:191. doi: 10.1186/s12891-016-1015-x
57. Illien-Jünger S, Torre OM, Kindschuh WF, Chen X, Laudier DM, Iatridis JC. Ages induce ectopic endochondral ossification in intervertebral discs. Eur Cell Mater. (2016) 32:257–70. doi: 10.22203/eCM.v032a17
58. Shao J, Yu M, Jiang L, Wu F, Liu X. Sequencing and bioinformatics analysis of the differentially expressed genes in herniated discs with or without calcification. Int J Mol Med. (2017) 39(1):81–90. doi: 10.3892/ijmm.2016.2821
59. Zehra U, Tryfonidou M, Iatridis JC, Illien-Jünger S, Mwale F, Samartzis D. Mechanisms and clinical implications of intervertebral disc calcification. Nat Rev Rheumatol. (2022) 18(6):352–62. doi: 10.1038/s41584-022-00783-7
60. Xiao ZF, He JB, Su GY, Chen MH, Hou Y, Chen SD, et al. Osteoporosis of the vertebra and osteochondral remodeling of the endplate causes intervertebral disc degeneration in ovariectomized mice. Arthritis Res Ther. (2018) 20(1):207. doi: 10.1186/s13075-018-1701-1
61. Li W, Zhao H, Zhou S, Xiong Z, Zhong W, Guan J, et al. Does vertebral osteoporosis delay or accelerate lumbar disc degeneration? a systematic review. Osteoporos Int. (2023). doi: 10.1007/s00198-023-06880-x
62. Takegami N, Akeda K, Yamada J, Sano T, Murata K, Huang J, et al. Rank/Rankl/Opg system in the intervertebral disc. Arthritis Res Ther. (2017) 19(1):121. doi: 10.1186/s13075-017-1332-y
63. Sano T, Akeda K, Yamada J, Takegami N, Sudo T, Sudo A. Expression of the Rank/Rankl/Opg system in the human intervertebral disc: Implication for the pathogenesis of intervertebral disc degeneration. BMC Musculoskeletal Disord. (2019) 20(1):225. doi: 10.1186/s12891-019-2609-x
64. Li H, Liu H, Zhang N, Zhu Z. Involvement of the g-Protein-Coupled receptor 4 in the increased expression of Rank/Rankl/Opg system and neurotrophins by nucleus pulposus cells under the degenerated intervertebral disc-like acidic microenvironment. BioMed Res Int. (2020) 2020:1328436. doi: 10.1155/2020/1328436
65. Liang Q-Q, Li X-F, Zhou Q, Xing L, Cheng S-D, Ding D-F, et al. The expression of osteoprotegerin is required for maintaining the intervertebral disc endplate of aged mice. Bone. (2011) 48(6):1362–9. doi: 10.1016/j.bone.2011.03.773
66. Li X-F, Xue C-C, Zhao Y-J, Cheng S-D, Zhao D-F, Liang Q-Q, et al. Deletion of opg leads to increased neovascularization and expression of inflammatory cytokines in the lumbar intervertebral disc of mice. Spine. (2017) 42(1):E8–E14. doi: 10.1097/BRS.0000000000001701
67. Hao X-d, Jiang L-s, Jiang S-d, Guo Z, Dai L-y. Relationship between lumbar intervertebral disc degeneration and vertebral osteoporosis in osteoprotegerin gene knock-out mice. Chin J Tissue Eng Res. (2012) 16:4402–7. doi: 10.3969/j.issn.1673-8225.2012.24.007
68. Kroon T, Bhadouria N, Niziolek P, Edwards IIID, Choi R, Clinkenbeard EL, et al. Suppression of Sost/Sclerostin and dickkopf-1 augment intervertebral disc structure in mice. J Bone Miner Res. (2022) 37(6):1156–69. doi: 10.1002/jbmr.4546
69. Madiraju P, Gawri R, Wang H, Antoniou J, Mwale F. Mechanism of parathyroid hormone-mediated suppression of calcification markers in human intervertebral disc cells. Eur Cell Mater. (2013) 25:268–83. doi: 10.22203/ecm.v025a19
70. Wang XY, Jiao LY, He JL, Fu ZA, Guo RJ. Parathyroid hormone 1−34 inhibits senescence in rat nucleus pulposus cells by activating autophagy Via the M−Tor pathway. Mol Med Rep. (2018) 18(3):2681–8. doi: 10.3892/mmr.2018.9229
71. Jia H, Ma J, Lv J, Ma X, Xu W, Yang Y, et al. Oestrogen and parathyroid hormone alleviate lumbar intervertebral disc degeneration in ovariectomized rats and enhance wnt/β-catenin pathway activity. Sci Rep. (2016) 6:27521. doi: 10.1038/srep27521
72. Zhou Z, Tian FM, Gou Y, Wang P, Zhang H, Song HP, et al. Enhancement of lumbar fusion and alleviation of adjacent segment disc degeneration by intermittent Pth(1-34) in ovariectomized rats. J Bone Miner Res. (2016) 31(4):828–38. doi: 10.1002/jbmr.2736
73. Li Y, Wei Y, Li H, Che H, Miao D, Ma C, et al. Exogenous parathyroid hormone alleviates intervertebral disc degeneration through the sonic hedgehog signalling pathway mediated by creb. Oxid Med Cell Longev. (2022) 2022:9955677. doi: 10.1155/2022/9955677
74. Zheng L, Cao Y, Ni S, Qi H, Ling Z, Xu X, et al. Ciliary parathyroid hormone signaling activates transforming growth factor-β to maintain intervertebral disc homeostasis during aging. Bone Res. (2018) 6:21. doi: 10.1038/s41413-018-0022-y
75. Xie S, Zhao C, Chen W, Li G, Xiong Z, Tang X, et al. Recombinant human bone morphogenetic protein 2 and 7 inhibit the degeneration of intervertebral discs by blocking the puma-dependent apoptotic signaling. Int J Biol Sci. (2021) 17(9):2367. doi: 10.7150/ijbs.56823
76. Gong C, Pan W, Hu W, Chen L. Bone morphogenetic protein-7 retards cell subculture-induced senescence of human nucleus pulposus cells through activating the Pi3k/Akt pathway. Biosci Rep. (2019) 39(3). doi: 10.1042/bsr20182312
77. Tan Y, Yao X, Dai Z, Wang Y, Lv G. Bone morphogenetic protein 2 alleviated intervertebral disc degeneration through mediating the degradation of ecm and apoptosis of nucleus pulposus cells Via the Pi3k/Akt pathway. Int J Mol Med. (2019) 43(1):583–92. doi: 10.3892/ijmm.2018.3972
78. Millecamps M, Tajerian M, Naso L, Sage EH, Stone LS. Lumbar intervertebral disc degeneration associated with axial and radiating low back pain in ageing sparc-null mice. PAIN. (2012) 153(6):1167–79. doi: 10.1016/j.pain.2012.01.027
79. Lee S, Jang SH, Suzuki-Narita M, Gregoire S, Millecamps M, Stone LS. Voluntary running attenuates behavioural signs of low back pain: Dimorphic regulation of intervertebral disc inflammation in male and female sparc-null mice. Osteoarthritis Cartilage. (2022) 30(1):110–23. doi: 10.1016/j.joca.2021.06.014
80. Whittal MC, Poynter SJ, Samms K, Briar KJ, Sinopoli SI, Millecamps M, et al. Tak-242 treatment and its effect on mechanical properties and gene expression associated with ivd degeneration in sparc-null mice. Eur Spine J. (2022) 31(10):2801–11. doi: 10.1007/s00586-022-07310-y
81. Xue JB, Zhan XL, Wang WJ, Yan YG, Liu C. Opg Rs2073617 polymorphism is associated with upregulated opg protein expression and an increased risk of intervertebral disc degeneration. Exp Ther Med. (2016) 12(2):702–10. doi: 10.3892/etm.2016.3342
82. Chen S, Bai R-j, Wang L-s. Relationship between osteoprotegerin level in the degenerated intervertebral disc and schneiderman’s classification. Chin J Tissue Eng Res. (2012) 16(4):691.
83. Bach FC, de Rooij KM, Riemers FM, Snuggs JW, de Jong WAM, Zhang Y, et al. Hedgehog proteins and parathyroid hormone-related protein are involved in intervertebral disc maturation, degeneration, and calcification. JOR Spine. (2019) 2(4):e1071. doi: 10.1002/jsp2.1071
84. Kamper M, Paulsson M, Zaucke F. Absence of collagen ix accelerates hypertrophic differentiation in the embryonic mouse spine through a disturbance of the ihh-pthrp feedback loop. Cell Tissue Res. (2017) 367(2):359–67. doi: 10.1007/s00441-016-2501-z
85. Gao C, Chen BP, Sullivan MB, Hui J, Ouellet JA, Henderson JE, et al. Micro ct analysis of spine architecture in a mouse model of scoliosis. Front Endocrinol (Lausanne). (2015) 6:38. doi: 10.3389/fendo.2015.00038
86. Ekşi M, Orhun Ö, Demir YN, Kara M, Berikol G, Özcan-Ekşi EE. Are serum thyroid hormone, parathormone, calcium, and vitamin d levels associated with lumbar spine degeneration? a cross-sectional observational clinical study. Eur Spine J. (2023) 32(5):1561–74. doi: 10.1007/s00586-023-07673-w
87. Rider CC, Mulloy B. Bone morphogenetic protein and growth differentiation factor cytokine families and their protein antagonists. Biochem J. (2010) 429(1):1–12. doi: 10.1042/BJ20100305
88. Li YF, Tang XZ, Liang CG, Hui YM, Ji YH, Xu W, et al. Role of growth differentiation factor-5 and bone morphogenetic protein type II receptor in the development of lumbar intervertebral disc degeneration. Int J Clin Exp Pathol. (2015) 8(1):719–26.
89. Feng C, Liu H, Yang Y, Huang B, Zhou Y. Growth and differentiation factor-5 contributes to the structural and functional maintenance of the intervertebral disc. Cell Physiol Biochem. (2015) 35(1):1–16. doi: 10.1159/000369670
90. Nakamura Y, Nakaya H, Saito N, Wakitani S. Coordinate expression of bmp-2, bmp receptors and noggin in normal mouse spine. J Clin Neurosci. (2006) 13(2):250–6. doi: 10.1016/j.jocn.2005.05.011
91. Li J, Yoon ST, Hutton WC. Effect of bone morphogenetic protein-2 (Bmp-2) on matrix production, other bmps, and bmp receptors in rat intervertebral disc cells. J Spinal Disord Tech. (2004) 17(5):423–8. doi: 10.1097/01.bsd.0000112084.85112.5d
92. Hollenberg AM, Maqsoodi N, Phan A, Huber A, Jubril A, Baldwin AL, et al. Bone morphogenic protein-2 signaling in human disc degeneration and correlation to the pfirrmann mri grading system. Spine J. (2021) 21(7):1205–16. doi: 10.1016/j.spinee.2021.03.002
93. Guo S, Cui L, Xiao C, Wang C, Zhu B, Liu X, et al. The mechanisms and functions of gdf-5 in intervertebral disc degeneration. Orthopaedic Surg. (2021) 13(3):734–41. doi: 10.1111/os.12942
94. Li X, Leo BM, Beck G, Balian G, Anderson GD. Collagen and proteoglycan abnormalities in the GDF-5- deficient mice and molecular changes when treating disk cells with recombinant growth factor. Spine. (2004) 29(20):2229–34. doi: 10.1097/01.brs.0000142427.82605
95. Krouwels A, Iljas JD, Kragten AHM, Dhert WJA, Öner FC, Tryfonidou MA, et al. Bone morphogenetic proteins for nucleus pulposus regeneration. Int J Mol Sci. (2020) 21(8):2720. doi: 10.3390/ijms21082720
96. Gillman CE, Jayasuriya AC. Fda-approved bone grafts and bone graft substitute devices in bone regeneration. Mater Sci Eng C Mater Biol Appl. (2021) 130:112466. doi: 10.1016/j.msec.2021.112466
97. Gruber HE, Ingram JA, Leslie K, Hanley ENJ. Cellular, but not matrix, immunolocalization of sparc in the human intervertebral disc: Decreasing localization with aging and disc degeneration. Spine. (2004) 29(20):2223–8. doi: 10.1097/01.brs.0000142225.07927.29
98. Millecamps I, Tajerian M, Sage EH, Stone LS. Behavioral signs of chronic back pain in the sparc-null mouse. Spine. (2011) 36(2):95–102. doi: 10.1097/BRS.0b013e3181cd9d75
99. Miyagi M, Millecamps M, Danco AT, Ohtori S, Takahashi K, Stone LS. Issls prize winner: Increased innervation and sensory nervous system plasticity in a mouse model of low back pain due to intervertebral disc degeneration. Spine. (2014) 39(17). doi: 10.1097/BRS.0000000000000334
100. Whittal MC, Molladavoodi S, Zwambag DP, Millecamps M, Stone LS, Gregory DE. Mechanical consequence of induced intervertebral disc degeneration in the sparc-null mouse. J Biomechanical Eng. (2020) 143(2). doi: 10.1115/1.4047995
101. Kawarai Y, Jang SH, Lee S, Millecamps M, Kang H, Gregoire S, et al. Exercise attenuates low back pain and alters epigenetic regulation in intervertebral discs in a mouse model. Spine J. (2021) 21(11):1938–49. doi: 10.1016/j.spinee.2021.06.002
102. Millecamps M, Czerminski JT, Mathieu AP, Stone LS. Behavioral signs of axial low back pain and motor impairment correlate with the severity of intervertebral disc degeneration in a mouse model. Spine J. (2015) 15(12):2524–37. doi: 10.1016/j.spinee.2015.08.055
103. Krock E, Millecamps M, Anderson KM, Srivastava A, Reihsen TE, Hari P, et al. Interleukin-8 as a therapeutic target for chronic low back pain: Upregulation in human cerebrospinal fluid and pre-clinical validation with chronic reparixin in the sparc-null mouse model. EBioMedicine. (2019) 43:487–500. doi: 10.1016/j.ebiom.2019.04.032
104. Lyu FJ, Cui H, Pan H, Mc Cheung K, Cao X, Iatridis JC, et al. Painful intervertebral disc degeneration and inflammation: From laboratory evidence to clinical interventions. Bone Res. (2021) 9(1):7. doi: 10.1038/s41413-020-00125-x
105. Li ZY, Zhou AF, Li G, Zhou LY, Pu PM, Zhu K, et al. Chronic spinal cord compression associated with intervertebral disc degeneration in sparc-null mice. Neural Regener Res. (2023) 18(3):634–42. doi: 10.4103/1673-5374.350210
106. Gruber HE, Norris RA, Kern MJ, Hoelscher GL, Ingram JA, Zinchenko N, et al. Periostin is expressed by cells of the human and sand rat intervertebral discs. Biotechnic Histochem. (2011) 86(3):199–206. doi: 10.3109/10520291003722774
107. Tsai T-T, Lai P-L, Liao J-C, Fu T-S, Niu C-C, Chen L-H, et al. Increased periostin gene expression in degenerative intervertebral disc cells. Spine J. (2013) 13(3):289–98. doi: 10.1016/j.spinee.2013.01.040
108. Zhu D, Zhou W, Wang Z, Wang Y, Liu M, Zhang G, et al. Periostin: An emerging molecule with a potential role in spinal degenerative diseases. Front Med. (2021) 8:694800. doi: 10.3389/fmed.2021.694800
109. Wu J, Chen Y, Liao Z, Liu H, Zhang S, Zhong D, et al. Self-amplifying loop of nf-κb and periostin initiated by Piezo1 accelerates mechano-induced senescence of nucleus pulposus cells and intervertebral disc degeneration. Mol Ther. (2022) 30(10):3241–56. doi: 10.1016/j.ymthe.2022.05.021
110. Zhu D, Wang Z, Zhang G, Ma C, Qiu X, Wang Y, et al. Periostin promotes nucleus pulposus cells apoptosis by activating the Wnt/β-catenin signaling pathway. FASEB J. (2022) 36(7):e22369. doi: 10.1096/fj.202200123R
111. Nikoloudaki G, Snider P, Simmons O, Conway SJ, Hamilton DW. Periostin and matrix stiffness combine to regulate myofibroblast differentiation and fibronectin synthesis during palatal healing. Matrix Biol. (2020) 94:31–56. doi: 10.1016/j.matbio.2020.07.002
112. Maurer E, Klinger C, Lorbeer R, Hefferman G, Schlett CL, Peters A, et al. Association between cardiovascular risk factors and degenerative disc disease of the thoracolumbar spine in the general population: Results from the kora mri study. Acta Radiol. (2022) 63(6):750–9. doi: 10.1177/02841851211010391
113. Belavý DL, Albracht K, Bruggemann GP, Vergroesen PP, van Dieën JH. Can exercise positively influence the intervertebral disc? Sports Med. (2016) 46(4):473–85. doi: 10.1007/s40279-015-0444-2
114. Zhou W, Shi Y, Wang H, Chen L, Yu C, Zhang X, et al. Exercise-induced Fndc5/Irisin protects nucleus pulposus cells against senescence and apoptosis by activating autophagy. Exp Mol Med. (2022) 54(7):1038–48. doi: 10.1038/s12276-022-00811-2
115. Chen T, Lin Y, Wu Z, Shi H, Hu W, Li S, et al. Irisin ameliorates intervertebral disc degeneration by activating Lats/Yap/Ctgf signaling. Oxid Med Cell Longev. (2022) 2022:9684062. doi: 10.1155/2022/9684062
116. Touchberry CD, Green TM, Tchikrizov V, Mannix JE, Mao TF, Carney BW, et al. FGF23 is a novel regulator of intracellular calcium and cardiac contractility in addition to cardiac hypertrophy. Am J Physiology-Endocrinology Metab. (2013) 304(8):E863–E73. doi: 10.1152/ajpendo.00596.2012
117. Vadalà G, Di Giacomo G, Ambrosio L, Cicione C, Tilotta V, Russo F, et al. Effect of irisin on human nucleus pulposus cells: New insights into the biological cross-talk between muscle and intervertebral disk. Spine (Phila Pa 1976). (2023) 48(7):468–75. doi: 10.1097/brs.0000000000004488
118. Hodges PW, James G, Blomster L, Hall L, Schmid A, Shu C, et al. Multifidus muscle changes after back injury are characterized by structural remodeling of muscle, adipose and connective tissue, but not muscle atrophy: Molecular and morphological evidence. Spine (Phila Pa 1976). (2015) 40(14):1057–71. doi: 10.1097/brs.0000000000000972
119. Severinsen MCK, Pedersen BK. Muscle-organ crosstalk: The emerging roles of myokines. Endocr Rev. (2020) 41(4):594–609. doi: 10.1210/endrev/bnaa016
120. Yu B, Zhu Z, Shen B, Lu J, Guo K, Zhao W, et al. Microrna-137 inhibits the inflammatory response and extracellular matrix degradation in lipopolysaccharide-stimulated human nucleus pulposus cells by targeting activin a receptor type I. Bioengineered. (2022) 13(3):6396–408. doi: 10.1080/21655979.2022.2042987
121. Elkasrawy M, Fulzele S, Bowser M, Wenger K, Hamrick M. Myostatin (Gdf-8) inhibits chondrogenesis and chondrocyte proliferation in vitro by suppressing sox-9 expression. Growth Factors. (2011) 29(6):253–62. doi: 10.3109/08977194.2011.599324
122. Hamrick MW, Pennington C, Byron CD. Bone architecture and disc degeneration in the lumbar spine of mice lacking gdf-8 (Myostatin). J Orthop Res. (2003) 21(6):1025–32. doi: 10.1016/S0736-0266(03)00105-0
123. Jiang W, Zhao P, Zhang X. Apelin promotes ecm synthesis by enhancing autophagy flux Via tfeb in human degenerative np cells under oxidative stress. BioMed Res Int. (2020) 2020:4897170. doi: 10.1155/2020/4897170
124. Liu W, Niu F, Sha H, Liu L-D, Lv Z-S, Gong W-Q, et al. Apelin-13/Apj system delays intervertebral disc degeneration by activating the Pi3k/Akt signaling pathway. Eur Rev Med Pharmacol Sci. (2020) 24(6):2820–8. doi: 10.26355/eurrev_202003_20643
125. Liu Y, Wei J, Zhao Y, Zhang Y, Han Y, Chen B, et al. Follistatin-like protein 1 promotes inflammatory reactions in nucleus pulposus cells by interacting with the mapk and nfκb signaling pathways. Oncotarget. (2017) 8(26):43023–34. doi: 10.18632/oncotarget.17400
126. Shi J, Wang S, He Q, Liu K, Zhao W, Xie Q, et al. Tnf-α induces up-regulation of microrna-27a Via the P38 signalling pathway, which inhibits intervertebral disc degeneration by targeting Fstl1. J Cell Mol Med. (2021) 25(15):7146–56. doi: 10.1111/jcmm.16745
127. Wang S, Wei J, Shi J, He Q, Zhou X, Gao X, et al. Follistatin-like 1 attenuation suppresses intervertebral disc degeneration in mice through interacting with tnf-α and smad signaling pathway. Oxid Med Cell Longev. (2021) 2021:6640751. doi: 10.1155/2021/6640751
128. He F, Gai J, Wang J, Tang L, Liu Y, Feng Q. Atrial natriuretic peptide protects vertebral endplate chondrocytes against H(2)O(2)−Induced apoptosis and oxidative stress through activation of the Nrf2/Ho−1 signaling pathway. Mol Med Rep. (2021) 24(5). doi: 10.3892/mmr.2021.12394
129. Wu X, Liao Z, Wang K, Hua W, Liu X, Song Y, et al. Targeting the IL-1β/IL-1ra pathways for the aggregation of human islet amyloid polypeptide in an ex vivo organ culture system of the intervertebral disc. Exp Mol Med. (2019) 51(9):1–16. doi: 10.1038/s12276-019-0310-7
130. Wu X, Song Y, Liu W, Wang K, Gao Y, Li S, et al. Iapp modulates cellular autophagy, apoptosis, and extracellular matrix metabolism in human intervertebral disc cells. Cell Death Discovery. (2017) 3(1):16107. doi: 10.1038/cddiscovery.2016.107
131. Wu X, Wang K, Hua W, Li S, Liu X, Liu W, et al. Down-regulation of islet amyloid polypeptide expression induces death of human annulus fibrosus cells Via mitochondrial and death receptor pathways. Biochim Biophys Acta Mol Basis Dis. (2017) 1863(6):1479–91. doi: 10.1016/j.bbadis.2017.04.016
132. Li W, Wu X, Qu R, Wang W, Chen X, Cheng L, et al. Ghrelin protects against nucleus pulposus degeneration through inhibition of nf-κb signaling pathway and activation of akt signaling pathway. Oncotarget. (2017) 8(54):91887–901. doi: 10.18632/oncotarget.19695
133. Peng Y, Lin H, Tian S, Liu S, Li J, Lv X, et al. Glucagon-like peptide-1 receptor activation maintains extracellular matrix integrity by inhibiting the activity of mitogen-activated protein kinases and activator protein-1. Free Radic Biol Med. (2021) 177:247–59. doi: 10.1016/j.freeradbiomed.2021.10.034
134. Ming-Yan Y, Jing Z, Shu-Qin G, Xiao-Liang B, Zhi-Hong L, Xue Z. Liraglutide inhibits the apoptosis of human nucleus pulposus cells induced by high glucose through Pi3k/Akt/Caspase-3 signaling pathway. Biosci Rep. (2019) 39(8). doi: 10.1042/bsr20190109
135. Yao M, Zhang J, Li Z, Bai X, Ma J, Li Y. Liraglutide protects nucleus pulposus cells against high-glucose induced apoptosis by activating Pi3k/Akt/ Mtor/Caspase-3 and Pi3k/Akt/Gsk3β/Caspase-3 signaling pathways. Front Med (Lausanne). (2021) 8:630962. doi: 10.3389/fmed.2021.630962
136. Das DK, Graham ZA, Cardozo CP. Myokines in skeletal muscle physiology and metabolism: Recent advances and future perspectives. Acta Physiol (Oxf). (2020) 228(2):e13367. doi: 10.1111/apha.13367
137. Rodgers BD, Ward CW. Myostatin/Activin receptor ligands in muscle and the development status of attenuating drugs. Endocr Rev. (2022) 43(2):329–65. doi: 10.1210/endrev/bnab030
138. Dankbar B, Fennen M, Brunert D, Hayer S, Frank S, Wehmeyer C, et al. Myostatin is a direct regulator of osteoclast differentiation and its inhibition reduces inflammatory joint destruction in mice. Nat Med. (2015) 21(9):1085–90. doi: 10.1038/nm.3917
139. Gentry BA, Ferreira JA, Phillips CL, Brown M. Hindlimb skeletal muscle function in myostatin-deficient mice. Muscle Nerve. (2011) 43(1):49–57. doi: 10.1002/mus.21796
140. Vinel C, Lukjanenko L, Batut A, Deleruyelle S, Pradère JP, Le Gonidec S, et al. The exerkine apelin reverses age-associated sarcopenia. Nat Med. (2018) 24(9):1360–71. doi: 10.1038/s41591-018-0131-6
141. Horvatić A, Gelemanović A, Pirkić B, Smolec O, Beer Ljubić B, Rubić I, et al. Multi-omics approach to elucidate cerebrospinal fluid changes in dogs with intervertebral disc herniation. Int J Mol Sci. (2021) 22(21):11678. doi: 10.3390/ijms222111678
142. Chaly Y, Blair HC, Smith SM, Bushnell DS, Marinov AD, Campfield BT, et al. Follistatin-like protein 1 regulates chondrocyte proliferation and chondrogenic differentiation of mesenchymal stem cells. Ann Rheum Dis. (2015) 74(7):1467–73. doi: 10.1136/annrheumdis-2013-204822
143. Wang Q, Wang J, Gu X, Feng D, Li D, Jiang T. Microrna-124-3p inhibits the differentiation of precartilaginous stem cells into nucleus pulposus-like cells Via targeting Fstl1. Exp Ther Med. (2021) 22(1):725. doi: 10.3892/etm.2021.10157
144. Suda M, Ogawa Y, Tanaka K, Tamura N, Yasoda A, Takigawa T, et al. Skeletal overgrowth in transgenic mice that overexpress brain Natriuretic Peptide. Proc Natl Acad Sci. (1998) 95(5):2337–42. doi: 10.1073/pnas.95.5.2337
145. Yotsumoto T, Morozumi N, Furuya M, Fujii T, Hirota K, Ueda Y, et al. Foramen magnum stenosis and midface hypoplasia in C-type natriuretic peptide-deficient rats and restoration by the administration of human C-type natriuretic peptide with 53 amino acids. PloS One. (2019) 14(5):e0216340. doi: 10.1371/journal.pone.0216340
146. Hannema SE, van Duyvenvoorde HA, Premsler T, Yang RB, Mueller TD, Gassner B, et al. An activating mutation in the kinase homology domain of the natriuretic peptide receptor-2 causes extremely tall stature without skeletal deformities. J Clin Endocrinol Metab. (2013) 98(12):E1988–98. doi: 10.1210/jc.2013-2358
147. Morimoto R, Akeda K, Iida R, Nishimura A, Tsujii M, Obata S, et al. Tissue renin-angiotensin system in the intervertebral disc. Spine (Phila Pa 1976). (2013) 38(3):E129–36. doi: 10.1097/BRS.0b013e31827b8c89
148. Sun K, Sun X, Sun J, Jiang Y, Lin F, Kong F, et al. Tissue renin-angiotensin system (Tras) induce intervertebral disc degeneration by activating oxidative stress and inflammatory reaction. Oxid Med Cell Longev. (2021) 2021:3225439. doi: 10.1155/2021/3225439
149. Li Z, Wystrach L, Bernstein A, Grad S, Alini M, Richards RG, et al. The tissue-Renin-Angiotensin-System of the human intervertebral disc. Eur Cell Mater. (2020) 40:115–32. doi: 10.22203/eCM.v040a07
150. Li W, Lai K, Chopra N, Zheng Z, Das A, Diwan AD. Gut-disc axis: A cause of intervertebral disc degeneration and low back pain? Eur Spine J. (2022) 31(4):917–25. doi: 10.1007/s00586-022-07152-8
151. Geng Z, Wang J, Chen G, Liu J, Lan J, Zhang Z, et al. Gut microbiota and intervertebral disc degeneration: A bidirectional two-sample mendelian randomization study. J Orthop Surg Res. (2023) 18(1):601. doi: 10.1186/s13018-023-04081-0
152. Ratna HV, Jeyaraman M, Yadav S, Jeyaraman N, Nallakumarasamy A. Is dysbiotic gut the cause of low back pain? Cureus. (2023) 15(7):e42496. doi: 10.7759/cureus.42496
153. Freidin M, Wells P, Stalteri M, Williams F. Pilot analysis of association between gut microbiome and modic changes. Orthopaedic Proc. (2019) 101-B(SUPP_10):1–1. doi: 10.1302/1358-992X.2019.10.001
154. Bany Bakar R, Reimann F, Gribble FM. The intestine as an endocrine organ and the role of gut hormones in metabolic regulation. Nat Rev Gastroenterol Hepatol. (2023) 20(12):784–96. doi: 10.1038/s41575-023-00830-y
155. Zhao X, Wang M, Wen Z, Lu Z, Cui L, Fu C, et al. Glp-1 receptor agonists: Beyond their pancreatic effects. Front Endocrinol. (2021) 12:1040. doi: 10.3389/fendo.2021.721135
156. Mehdi SF, Pusapati S, Anwar MS, Lohana D, Kumar P, Nandula SA, et al. Glucagon-like peptide-1: A multi-faceted anti-inflammatory agent. Front Immunol. (2023) 14:1148209. doi: 10.3389/fimmu.2023.1148209
157. Drucker DJ. Mechanisms of action and therapeutic application of glucagon-like peptide-1. Cell Metab. (2018) 27(4):740–56. doi: 10.1016/j.cmet.2018.03.001
158. Wu X, Song Y, Li S, Liu X, Hua W, Wang K, et al. Pramlintide regulation of extracellular matrix (Ecm) and apoptosis through mitochondrial-dependent pathways in human nucleus pulposus cells. Int J Immunopathol Pharmacol. (2018) 31:394632017747500. doi: 10.1177/0394632017747500
159. Chow LS, Gerszten RE, Taylor JM, Pedersen BK, Van Praag H, Trappe S, et al. Exerkines in health, resilience and disease. Nat Rev Endocrinol. (2022) 18(5):273–89. doi: 10.1038/s41574-022-00641-2
160. Liu F-J, Xie L-Y, Li H-Z, Cao S-N, Chen Y-Z, Bin S, et al. Expression of Angptl4 in nucleus pulposus tissues is associated with intervertebral disc degeneration. Dis Markers. (2021) 2021:3532716. doi: 10.1155/2021/3532716
161. Liao Z, Wu X, Song Y, Luo R, Yin H, Zhan S, et al. Angiopoietin-like protein 8 expression and association with extracellular matrix metabolism and inflammation during intervertebral disc degeneration. J Cell Mol Med. (2019) 23(8):5737–50. doi: 10.1111/jcmm.14488
162. Jia C, Li X, Pan J, Ma H, Wu D, Lu H, et al. Silencing of angiopoietin-like protein 4 (Angptl4) decreases inflammation, extracellular matrix degradation, and apoptosis in osteoarthritis via the sirtuin 1/NF-κB pathway. Oxid Med Cell Longevity. (2022) 2022:1135827. doi: 10.1155/2022/1135827
163. Van den Akker GGH, Steijns JSJJ, Stassen RHMJ, Wasilewski GB, Peeters LCW, Wijnands KAP, et al. Development of a cyclic-inverso Ahsg/Fetuin a-based peptide for inhibition of calcification in osteoarthritis. Osteoarthritis Cartilage. (2023) 31(6):727–40. doi: 10.1016/j.joca.2022.11.007
164. Schumann B, Bolm-Audorff U, Bergmann A, Ellegast R, Elsner G, Grifka J, et al. Lifestyle factors and lumbar disc disease: Results of a german multi-center case-control study (Epilift). Arthritis Res Ther. (2010) 12(5):1–8. doi: 10.1186/ar3164
165. Ambrosio L, Mazzuca G, Maguolo A, Russo F, Cannata F, Vadalà G, et al. The burden of low back pain in children and adolescents with overweight and obesity: From pathophysiology to prevention and treatment strategies. Ther Adv Musculoskeletal Dis. (2023) 15:1759720X231188831. doi: 10.1177/1759720X231188831
166. Chen Y, Yang L, Gao X, Tang A, He H, Xiong C, et al. The impact of diabetes mellitus on patient-reported outcomes of chronic low back pain with modic changes at one year: A prospective cohort study. Global Spine J. (2023) 12:21925682231206962. doi: 10.1177/21925682231206962
167. Belavý DL, Quittner MJ, Ridgers N, Ling Y, Connell D, Rantalainen T. Running exercise strengthens the intervertebral disc. Sci Rep. (2017) 7(1):45975. doi: 10.1038/srep45975
168. Lai A, Ho L, Evashwick-Rogler TW, Watanabe H, Salandra J, Winkelstein BA, et al. Dietary polyphenols as a safe and novel intervention for modulating pain associated with intervertebral disc degeneration in an in-vivo rat model. PloS One. (2019) 14(10):e0223435. doi: 10.1371/journal.pone.0223435
169. Staszkiewicz R, Sobański D, Ulasavets U, Wieczorek J, Golec E, Marcol W, et al. Evaluation of the concentration of selected elements in serum patients with intervertebral disc degeneration. J Trace Elements Med Biol. (2023) 77:127145. doi: 10.1016/j.jtemb.2023.127145
Keywords: intervertebral disc homeostasis, intervertebral disc degeneration, organokines, organ crosstalk, signaling pathway
Citation: He Y, Liu S, Lin H, Ding F, Shao Z and Xiong L (2024) Roles of organokines in intervertebral disc homeostasis and degeneration. Front. Endocrinol. 15:1340625. doi: 10.3389/fendo.2024.1340625
Received: 18 November 2023; Accepted: 19 February 2024;
Published: 12 March 2024.
Edited by:
Changjun Li, Central South University, ChinaReviewed by:
Qiaoyue Guo, University of Maryland, Baltimore, United StatesCopyright © 2024 He, Liu, Lin, Ding, Shao and Xiong. This is an open-access article distributed under the terms of the Creative Commons Attribution License (CC BY). The use, distribution or reproduction in other forums is permitted, provided the original author(s) and the copyright owner(s) are credited and that the original publication in this journal is cited, in accordance with accepted academic practice. No use, distribution or reproduction is permitted which does not comply with these terms.
*Correspondence: Liming Xiong, eGlvbmdsaW1pbmdAaHVzdC5lZHUuY24=; Zengwu Shao, c3p3cHJvQDE2My5jb20=; Fan Ding, am15eWRmQDE2My5jb20=
†These authors have contributed equally to this work and share first authorship
Disclaimer: All claims expressed in this article are solely those of the authors and do not necessarily represent those of their affiliated organizations, or those of the publisher, the editors and the reviewers. Any product that may be evaluated in this article or claim that may be made by its manufacturer is not guaranteed or endorsed by the publisher.
Research integrity at Frontiers
Learn more about the work of our research integrity team to safeguard the quality of each article we publish.