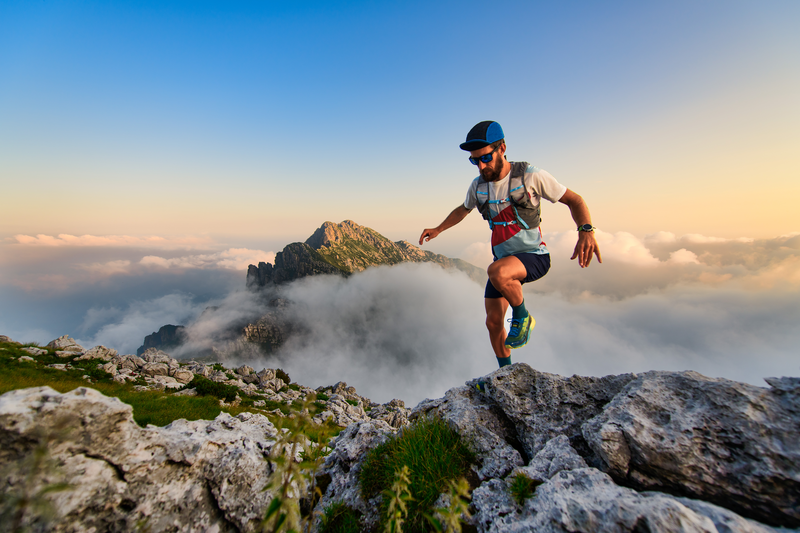
95% of researchers rate our articles as excellent or good
Learn more about the work of our research integrity team to safeguard the quality of each article we publish.
Find out more
REVIEW article
Front. Endocrinol. , 20 February 2024
Sec. Cancer Endocrinology
Volume 15 - 2024 | https://doi.org/10.3389/fendo.2024.1328960
Lung cancer is responsible for the highest number of cancer-related deaths, with non-small cell lung cancer (NSCLC) being the most prevalent subtype. A critical aspect of managing lung cancer is reducing morbidity and mortality rates among NSCLC patients. Identifying high-risk factors for lung cancer and facilitating early diagnosis are invaluable in achieving this objective. Recent research has highlighted the association between insulin resistance and the development of NSCLC, further emphasizing its significance in the context of lung cancer. It has been discovered that improving insulin resistance can potentially inhibit the progression of lung cancer. Consequently, this paper aims to delve into the occurrence of insulin resistance, the mechanisms underlying its involvement in lung cancer development, as well as its potential value in predicting, assessing, and treating lung cancer.
Based on the fact that early symptoms of lung cancer are atypical, leading to late-stage diagnosis for the majority of patients, there is a missed opportunity for surgical treatment (1). Although individualized, biomarker-driven therapy, screening for high-risk groups, and surgical treatment have improved survival rates, the results remain unsatisfactory (2). Therefore, it is imperative to identify and screen high-risk groups in order to improve the mortality rate of lung cancer. Current recognized risk factors for lung cancer include smoking, passive smoking, history of chronic obstructive pulmonary disease (COPD), occupational exposure, and genetic susceptibility (1). Recent research has also indicated that insulin resistance is a contributing factor in various cancers (3, 4). While there is growing evidence linking insulin resistance to non-small cell lung cancer (NSCLC), there is currently no clinically accepted threshold to assess insulin resistance (5, 6). Insulin resistance promotes tumor cell proliferation, invasion, and metastasis through mechanisms affecting insulin-like growth factor (IGF)-related pathways, inflammatory response, tumor microenvironment, and reactive oxygen species (ROS) (7–9). Pharmacological interventions, lifestyle changes, and weight loss are believed to reduce insulin resistance and decrease the risk of lung cancer development (10–12). Furthermore, targeted therapy utilizing insulin resistance-related signaling pathways may improve insulin resistance and inhibit the growth and invasion of lung cancer cells (13). In summary, this paper aims to explore the mechanism of insulin resistance in lung cancer, provide an overview of research progress, and deepen understanding of the relationship between insulin resistance and lung cancer to guide the development of new prediction tools and treatment strategies.
Insulin resistance, initially proposed by Harold Himsworth in the early 1930s (14), is a metabolic disorder closely associated with obesity and type 2 diabetes. It is characterized by a diminished cellular response to insulin, leading to a reduction in insulin bioactivity (15). This condition has significant implications for overall metabolic health and contributes to the development of various diseases.
The insulin receptor serves as the central receptor responsible for insulin’s actions, and any abnormalities in its signaling pathway can result in impaired insulin signaling (16). Evidence suggests that both the expression level and function of the insulin receptor are closely linked to insulin resistance (17, 18). For instance, mutations in the insulin receptor gene can diminish receptor activity, leading to disruptions in insulin binding and subsequent signaling events (19).
Adipose tissue plays a crucial role in the development of insulin resistance. Excessive accumulation of adipose tissue triggers an inflammatory response in adipocytes, which in turn results in the production of various inflammatory factors (20). These factors disrupt insulin signaling pathways and contribute to the onset of insulin resistance. Examples of such inflammatory factors include tumor necrosis factor-α (TNF-α) and interleukin-6 (IL-6) (21).
Insulin resistance is characterized by a diminished ability of cells to respond to insulin signaling, resulting in impaired glucose utilization and disrupted glucose metabolism (22). As a consequence, glucose is unable to enter cells efficiently, leading to elevated blood glucose levels (23, 24). The increased blood glucose levels, in turn, stimulate the secretion of insulin, establishing a detrimental cycle of insulin and glucose interplay (25, 26). To break this cycle, it is crucial to address the underlying insulin resistance and restore proper glucose metabolism.
Insulin resistance has been shown to have significant effects on fatty acid metabolism (27, 28). It disrupts the balance of fatty acid synthesis, storage, oxidation, and utilization. In the presence of insulin resistance, there is an increased synthesis and storage of fatty acids in adipose tissue. Simultaneously, fatty acid oxidation and utilization are inhibited, causing an accumulation of fatty acids in non-adipose tissues. This abnormal accumulation contributes to the development of disorders in fatty acid metabolism and further exacerbates insulin resistance (29, 30).
Insulin resistance often triggers a chronic state of low-grade inflammation in the body (31, 32). The activation of inflammatory response due to insulin resistance results in the infiltration of inflammatory cells and the release of inflammatory factors. These factors can disrupt insulin signaling pathways, further aggravating the condition of insulin resistance (33, 34).
Early and timely recognition of insulin resistance is crucial in preventing the development of related diseases. Clinically, insulin resistance indexes such as the normoglycemic-hyperinsulinic clamp, insulin resistance index (HOMA-IR), and triglyceride glucose index (TyG) are used for assessment. Initially, the normoglycemic-hyperinsulinemic clamp was considered the gold standard for assessing insulin resistance. However, its high cost and complexity limit its use in monitoring the general population (35). As a result, HOMA-IR became a commonly used method to assess insulin resistance, as it does not require routine measurement of insulin levels in clinical settings. However, HOMA-IR has certain limitations (36). In recent years, the TyG index has gained popularity due to its reliability and simplicity and has started to replace HOMA-IR in assessing insulin resistance (37). Studies have shown that the TyG index has a higher predictive value for insulin resistance than HOMA-IR (38). Additionally, a triglyceride glucose body mass index (TyG-BMI) based on the TyG index has been proposed as an alternative index for assessing insulin resistance (6). However, there is still a lack of specific cut-off values to predict insulin resistance, which requires further investigation.
Lung cancer remains the second most common malignant tumor in the world, following breast cancer (39). Over the years, numerous studies have investigated the association between insulin resistance and NSCLC by examining a large number of cases. A cross-sectional survey of the Chinese population conducted by Xin Yan et al. found that the TyG index was independently associated with the risk of NSCLC (5). Similarly, Feifei Wang et al. utilized the TyG-BMI index to assess insulin resistance and found it to be a risk factor for NSCLC in the Chinese population (6). Additionally, their study demonstrated that the BMI index was also a risk factor for NSCLC (6). Another prospective study involving 29,133 Finnish men showed that elevated levels of insulin resistance were linked to an increased risk of lung cancer (40). Similarly, Petridou et al. conducted a study on an Athenian population and concluded that insulin resistance was an independent risk factor for NSCLC (41). However, some studies provide contradictory findings. A prospective study conducted by Lijie Wang et al. on a British population showed no significant correlation between the TyG index and the risk of NSCLC (42). These conflicting results may be attributed to differences in lifestyle, dietary habits, geography, and genetic factors among different races. Regardless of insulin intake or impaired insulin utilization in insulin-resistant states, elevated insulin levels may increase the risk of NSCLC. A study on diabetes mellitus and lung cancer in postmenopausal women, conducted by Juhua Luo et al., reported a significant increase in the risk of lung cancer among postmenopausal women with diabetes mellitus after insulin treatment (43). Additionally, Ren Qiu et al.’s study on the correlation between insulin and the non-small cell lung cancer cell line (A549) found that insulin could contribute to the proliferation of lung cancer cells (44). This suggests that, in the state of insulin resistance, excessive insulin accumulates extracellularly and promotes the development of lung cancer. In conclusion, a substantial body of research supports the association between insulin resistance and the development of NSCLC.
Insulin resistance is a condition characterized by reduced biological activity of insulin, leading to an increase in insulin secretion to compensate for its diminished function. However, this increased insulin secretion is often accompanied by elevated secretion of insulin-like growth factors (IGFs) (45). IGFs, which include IGF-1 and IGF-2, are a group of peptide hormones that can promote various cellular processes such as cell proliferation, migration, invasion, and epithelial-mesenchymal transition (EMT). This promotion is achieved through the activation of the phosphatidylinositol-3 kinase (PI3K) and mitogen-activated protein kinase (MAPK) pathways. Structurally similar to insulin, IGF-1 and IGF-2 can bind to both the insulin receptor and the IGF-1 receptor. The binding of IGF-1 and IGF-2 to the IGF-1 receptor induces phosphorylation of the receptor itself, which in turn phosphorylates its binding proteins, including insulin receptor substrate-1 (IRS-1) and Src homologous collagen (SHC). This phosphorylation activates the PI3K/Akt and Ras/MAPK signaling pathways, respectively, resulting in enhanced cell growth, proliferation, invasion, and inhibition of apoptosis (46, 47). Figure 1 illustrates the molecular mechanisms involved. Numerous studies have demonstrated increased expression levels of IGF-1 and IGF-2 in NSCLC tumor tissues (48, 49). Interestingly, a study by Kim et al. discovered that low expression of IGF-1 was associated with longer overall survival (OS) in NSCLC patients. This finding emphasizes the significance of IGF-1 as a prognostic indicator and suggests the potential for risk stratification in NSCLC based on IGF-1 levels (50).
The IGF axis plays a crucial role in the growth, invasion, and metastasis of NSCLC, with molecular proteins such as IGF-1R, IGF-2R, and insulin-like growth factor binding protein (IGFBP) being implicated (51). The expression of IGF-1R in NSCLC tissues is closely associated with that of IGF-1 and serves as an independent negative predictor of NSCLC prognosis (49, 52). This negative effect may be linked to the co-expression of IL-6 and EGFR (53). A meta-analysis revealed that high expression of IGF-1R predicts a lower likelihood of disease-free survival (DFS) in NSCLC patients (54). Moreover, elevated levels of IGF-1 have been found to be a negative prognostic indicator and associated with poor survival in NSCLC patients (55). Consequently, targeting IGF-1R shows promise as a therapeutic approach and prognostic biomarker for NSCLC. Similarly, low expression of IGF-2R is associated with a worse prognosis in advanced NSCLC (56). Additionally, IGFBP competitively binds to IGF, preventing it from binding to the IGF receptor, and demonstrates anti-metastatic, anti-angiogenic, and anti-tumor activity in NSCLC cells (57). Several studies have explored intervention strategies targeting the IGF axis for lung cancer progression. For instance, IGF-1R inhibitors have been investigated for lung cancer treatment, blocking the activation of the IGF-1R signaling pathway and inhibiting the proliferation and invasion of lung cancer cells (58, 59). In addition, modulation of IGF activity by regulating the level of IGFBP has been explored (60, 61).
Insulin resistance has a significant impact not only on the biological properties of tumor cells but also on the tumor microenvironment, thereby promoting the development of lung cancer (62). In the state of insulin resistance, there are aberrations in the insulin receptor signaling pathway, specifically a reduction in tyrosine phosphorylation of IRS1 and inhibition of the PI3K/Akt signaling pathway (63). Consequently, cellular response to insulin is compromised, affecting glucose metabolism and cell proliferation. Due to the inability of cells to effectively utilize glucose as an energy source, they increase their demand for alternative nutrients, such as fatty acids and amino acids, to compensate for the lack of energy. This metabolic shift creates a more favorable environment for tumor cell survival, growth, and metastasis (64, 65). Furthermore, it has been observed that insulin-resistant tumor tissues exhibit enhanced inflammatory responses and increased secretion of inflammatory factors, such as TNF-α and interleukin-6 (IL-6) (66). These inflammatory factors stimulate the production of growth factors and angiogenic factors within tumor cells, further facilitating tumor growth, invasion, and metastasis (67).
Enhanced inflammatory responses in insulin-resistant states have been associated with the progression of lung cancer. It has been observed that insulin resistance can stimulate the production and release of inflammatory factors by activating inflammation-related signaling pathways, such as the NF-κB-related pathway. These inflammatory factors contribute to the promotion of tumor cell growth, invasion, and metastasis (8, 68). Moreover, insulin resistance is also implicated in the progression of lung cancer through its influence on tumor immune escape. In insulin-resistant states, T cell function is suppressed, leading to a reduced ability to recognize and eliminate tumor cells. Consequently, this creates a favorable environment for tumor cell growth and metastasis (9, 69). Additionally, insulin resistance can alter the interaction between tumor cells and the surrounding stroma by affecting the expression of cell adhesion molecules and matrix metalloproteinases. This, in turn, facilitates cell invasion and metastasis (70).
Insulin resistance is known to be associated with the increased production of ROS, although the exact mechanisms underlying this association are not yet fully understood. Current research suggests several potential pathways through which insulin resistance can lead to ROS production. One possible mechanism is the impairment of mitochondrial function within the cell. Mitochondria are responsible for energy production, and when their function is compromised, they may generate more ROS as a byproduct of the oxidative phosphorylation process (71). Hence, insulin resistance may contribute to mitochondrial dysfunction, resulting in elevated ROS production. Another factor linking insulin resistance to ROS production is chronic low-grade inflammation. Research indicates that insulin resistance can promote inflammatory responses, which, in turn, can increase the production of ROS (72). The activation of inflammatory pathways due to insulin resistance may contribute to an amplified intracellular inflammatory response, leading to higher levels of ROS. Additionally, insulin resistance has been found to increase the activity of an enzyme system known as NADPH oxidase. This enzyme system is responsible for reducing oxygen to reactive oxygen species, thereby generating ROS within the cell (73). When NADPH oxidase activity is elevated, there is a corresponding increase in ROS production. The excess production of ROS overwhelms the cell’s antioxidant defense mechanisms, ultimately resulting in cellular damage. It has been proposed that ROS can induce DNA damage, gene mutations, and apoptosis, which are all significant factors in the development of cancer (74). Furthermore, there is evidence suggesting that ROS can impact the progression and prognosis of lung cancer (75).
Several studies have demonstrated that dietary factors can impact the association between insulin resistance and lung cancer. For instance, incorporating high-fiber diets, reducing saturated fats, and consuming foods rich in antioxidants may potentially enhance insulin resistance and lower the risk of developing lung cancer (10, 11). Moreover, natural products like caffeine and tea polyphenols have been indicated to ameliorate insulin resistance and inhibit the progression of lung cancer (76). These findings highlight the potential benefits of dietary modifications and the use of natural compounds in reducing the risk of lung cancer by improving insulin resistance.
Physical activity and weight management play crucial roles in regulating insulin resistance and reducing the risk of lung cancer. Research has demonstrated that engaging in moderate exercise can enhance insulin sensitivity, lower insulin resistance, and subsequently decrease the likelihood of developing lung cancer (77). Moreover, maintaining a healthy weight is particularly vital in combating insulin resistance since obesity is closely associated with this condition. By achieving and sustaining a healthy weight, individuals can effectively mitigate the risk of both insulin resistance and lung cancer (78).
Numerous studies have investigated the potential of pharmacological interventions in improving insulin resistance and preventing lung cancer development. Metformin, an insulin sensitizer, has been extensively studied for its ability to inhibit the proliferation and invasion of lung cancer cells by enhancing insulin resistance and influencing metabolic pathways (12). Molecularly targeted therapies have also been explored to modulate insulin resistance and impede lung cancer progression. For instance, targeting the PI3K/Akt/mTOR signaling pathway is believed to ameliorate insulin resistance, thus inhibiting the proliferation and invasion of lung cancer cells (13, 79). Furthermore, other targeted drugs, such as IGF-1R inhibitors and AMPK activators, have demonstrated potential in modulating insulin resistance and lung cancer (80, 81).
There is a strong connection between insulin resistance and the development of lung cancer. In individuals with insulin resistance, elevated insulin levels play a significant role in the initiation and progression of lung cancer. This association between insulin resistance and lung cancer involves various pathways. Firstly, insulin resistance promotes the proliferation, invasion, and metastasis of tumor cells. Additionally, insulin resistance activates inflammatory responses, inhibits apoptosis, and impacts immune escape mechanisms. These findings are illustrated in Figure 2.
Figure 2 Association between insulin resistance and NSCLC development (This image was drawn by Figdraw).
Insulin resistance has emerged as a potential therapeutic target in the clinical setting, particularly for the treatment of lung cancer. Numerous studies have explored the use of insulin resistance modulators to intervene in the development of this disease. Specifically, insulin-sensitizing agents and inhibitors of insulin-like growth factor-1 receptor (IGF-1R) have been investigated as potential treatments for lung cancer, aiming to inhibit aberrant signaling and cell proliferation associated with insulin resistance. Furthermore, drugs that can modulate insulin resistance, such as insulin sensitizers and anti-inflammatory agents, may also hold promise in the treatment of lung cancer.
However, there are still numerous areas that remain uncharted and require further research regarding the relationship between insulin resistance and lung cancer. Firstly, the accurate assessment of the extent of insulin resistance needs to be addressed. Secondly, the causal relationship between insulin resistance and lung cancer needs to be explored in depth. Finally, it is crucial to investigate the molecular mechanisms underlying insulin resistance. In order to gain a better understanding of these aspects, more comprehensive clinical studies are warranted. These studies will play a vital role in validating the efficacy and safety of insulin resistance modulators for the treatment of lung cancer.
A comprehensive investigation into the connection between insulin resistance and lung cancer can enhance our understanding of the mechanisms involved in the development of lung cancer. This study can also provide a solid foundation for the development of targeted therapeutic strategies. By effectively regulating insulin resistance, we aim to offer improved treatment options for lung cancer patients, ultimately leading to enhanced survival rates and improved quality of life.
SZ: Conceptualization, Writing – original draft. LW: Resources, Writing – original draft. WW: Project administration, Writing – original draft. RL: Conceptualization, Writing – review & editing.
The author(s) declare that no financial support was received for the research, authorship, and/or publication of this article.
The authors declare that the research was conducted in the absence of any commercial or financial relationships that could be construed as a potential conflict of interest.
All claims expressed in this article are solely those of the authors and do not necessarily represent those of their affiliated organizations, or those of the publisher, the editors and the reviewers. Any product that may be evaluated in this article, or claim that may be made by its manufacturer, is not guaranteed or endorsed by the publisher.
1. Bade BC, Dela Cruz CS. Lung cancer 2020: epidemiology, etiology, and prevention. Clin Chest Med (2020) 41:1–24. doi: 10.1016/j.ccm.2019.10.001.
2. The Lancet. Lung cancer: some progress, but still a lot more to do. Lancet (2019) 394:1880. doi: 10.1016/S0140-6736(19)32795-3.
3. Fritz J, Bjørge T, Nagel G, Manjer J, Engeland A, Häggström C, et al. The triglyceride-glucose index as a measure of insulin resistance and risk of obesity-related cancers. Int J Epidemiol (2020) 49:193–204. doi: 10.1093/ije/dyz053.
4. Yin DT, He H, Yu K, Xie J, Lei M, Ma R, et al. The association between thyroid cancer and insulin resistance, metabolic syndrome and its components: a systematic review and meta-analysis. Int J Surg (2018) 57:66–75. doi: 10.1016/j.ijsu.2018.07.013.
5. Yan X, Gao Y, Tong J, Tian M, Dai J, Zhuang Y. Association between triglyceride glucose index and non-small cell lung cancer risk in Chinese population. Front Oncol (2021) 11:585388. doi: 10.3389/fonc.2021.585388.
6. Wang F, He T, Wang G, Han T, Yao Z. Association of triglyceride glucose-body mass index with non-small cell lung cancer risk: A case-control study on Chinese adults. Front Nutr (2022) 9:1004179. doi: 10.3389/fnut.2022.1004179
7. Chen YM, Qi S, Perrino S, Hashimoto M, Brodt P. Targeting the IGF-axis for cancer therapy: development and validation of an IGF-trap as a potential drug. Cells (2020) 9:1098. doi: 10.3390/cells9051098.
8. Iyengar NM, Gucalp A, Dannenberg AJ, Hudis CA. Obesity and cancer mechanisms: tumor microenvironment and inflammation. J Clin Oncol (2016) 34:4270–6. doi: 10.1200/JCO.2016.67.4283.
9. Djiogue S, Nwabo Kamdje AH, Vecchio L, Kipanyula MJ, Farahna M, Aldebasi Y, et al. Insulin resistance and cancer: the role of insulin and IGFs. Endocr Relat Cancer (2013) 20:R1–R17. doi: 10.1530/ERC-12-0324.
10. Cai H, Sobue T, Kitamura T, Ishihara J, Murai U, Sawada N, et al. Dietary fibre intake is associated with reduced risk of lung cancer: a Japan public health centre-based prospective study (JPHC). Int J Epidemiol (2022) 51:1142–52. doi: 10.1093/ije/dyac054.
11. Yang JJ, Yu D, Xiang YB, Blot W, White E, Robien K, et al. Association of dietary fiber and yogurt consumption with lung cancer risk: A pooled analysis. JAMA Oncol (2020) 6:e194107. doi: 10.1001/jamaoncol.2019.4107.
12. Fatehi Hassanabad A, MacQueen KT. Molecular mechanisms underlining the role of metformin as a therapeutic agent in lung cancer. Cell Oncol (Dordr) (2021) 44:1–18. doi: 10.1007/s13402-020-00570-0.
13. Tan AC. Targeting the PI3K/Akt/mTOR pathway in non-small cell lung cancer (NSCLC). Thorac Cancer (2020) 11:511–8. doi: 10.1111/1759-7714.13328.
14. Kim SH. Measurement of insulin action: a tribute to Sir Harold Himsworth. Diabetes Med (2011) 28:1487–93. doi: 10.1111/j.1464-5491.2011.03409.x.
15. Samuel VT, Shulman GI. Mechanisms for insulin resistance: common threads and missing links. Cell (2012) 148:852–71. doi: 10.1016/j.cell.2012.02.017.
16. Boucher J, Tseng YH, Kahn CR. Insulin and insulin-like growth factor-1 receptors act as ligand-specific amplitude modulators of a common pathway regulating gene transcription. J Biol Chem (2010) 285:17235–45. doi: 10.1074/jbc.M110.118620.
17. Semple RK, Sleigh A, Murgatroyd PR, Adams CA, Bluck L, Jackson S, et al. Postreceptor insulin resistance contributes to human dyslipidemia and hepatic steatosis. J Clin Invest (2009) 119:315–22. doi: 10.1172/JCI37432.
18. Flier JS. Insulin receptors and insulin resistance. Annu Rev Med (1983) 34:145–60. doi: 10.1146/annurev.me.34.020183.001045.
19. Hosoe J, Kadowaki H, Miya F, Aizu K, Kawamura T, Miyata I, et al. Structural basis and genotype-phenotype correlations of INSR mutations causing severe insulin resistance. Diabetes (2017) 66:2713–23. doi: 10.2337/db17-0301.
20. Weisberg SP, McCann D, Desai M, Rosenbaum M, Leibel RL, Ferrante AW Jr. Obesity is associated with macrophage accumulation in adipose tissue. J Clin Invest (2003) 112:1796–808. doi: 10.1172/JCI19246.
21. Lumeng CN, Saltiel AR. Inflammatory links between obesity and metabolic disease. J Clin Invest (2011) 121:2111–7. doi: 10.1172/JCI57132.
22. Petersen MC, Shulman GI. Mechanisms of insulin action and insulin resistance. Physiol Rev (2018) 98:2133–223. doi: 10.1152/physrev.00063.2017.
23. DeFronzo RA, Ferrannini E, Groop L, Henry RR, Herman WH, Holst JJ, et al. Type 2 diabetes mellitus. Nat Rev Dis Primers (2015) 1:15019. doi: 10.1038/nrdp.2015.19.
24. Perry RJ, Camporez JP, Kursawe R, Titchenell PM, Zhang D, Perry CJ, et al. Hepatic acetyl CoA links adipose tissue inflammation to hepatic insulin resistance and type 2 diabetes. Cell (2015) 160:745–58. doi: 10.1016/j.cell.2015.01.012.
25. Campbell JE, Newgard CB. Mechanisms controlling pancreatic islet cell function in insulin secretion. Nat Rev Mol Cell Biol (2021) 22(2)::142–58. doi: 10.1038/s41580-020-00317-7
26. Nauck MA, Meier JJ. Incretin hormones: their role in health and disease. Diabetes Obes Metab (2018) 20 Suppl 1:5–21. doi: 10.1111/dom.13129.
27. Perry RJ, Samuel VT, Petersen KF, Shulman GI. The role of hepatic lipids in hepatic insulin resistance and type 2 diabetes. Nature (2014) 510:84–91. doi: 10.1038/nature13478.
28. Samuel VT, Shulman GI. The pathogenesis of insulin resistance: integrating signaling pathways and substrate flux. J Clin Invest (2016) 126:12–22. doi: 10.1172/JCI77812.
29. Randle PJ, Garland PB, Hales CN, Newsholme EA. The glucose fattyacid cycle. Its role in insulin sensitivity and the metabolic disturbances of diabetes mellitus. Lancet (1963) 1(7285):785–9. doi: 10.1016/s0140-6736(63)91500-9
30. Samuel VT, Shulman GI. Nonalcoholic fatty liver disease as a nexus of metabolic and hepatic diseases. Cell Metab (2018) 27:22–41. doi: 10.1016/j.cmet.2017.08.002.
31. Hotamisligil GS. Inflammation, metaflammation and immunometabolic disorders. Nature (2017) 542:177–85. doi: 10.1038/nature21363.
32. Shoelson SE, Herrero L, Naaz A. Obesity, inflammation, and insulin resistance. Gastroenterology (2007) 132:2169–80. doi: 10.1053/j.gastro.2007.03.059.
33. Shoelson SE, Lee J, Goldfine AB. Inflammation and insulin resistance. J Clin Invest (2006) 116:1793–801. doi: 10.1172/JCI29069.
34. Donath MY, Shoelson SE. Type 2 diabetes as an inflammatory disease. Nat Rev Immunol (2011) 11:98–107. doi: 10.1038/nri2925.
35. Bonora E, Targher G, Alberiche M, Bonadonna RC, Saggiani F, Zenere MB, et al. Homeostasis model assessment closely mirrors the glucose clamp technique in the assessment of insulin sensitivity: studies in subjects with various degrees of glucose tolerance and insulin sensitivity. Diabetes Care (2000) 23:57–63. doi: 10.2337/diacare.23.1.57.
36. Locateli JC, Lopes WA, Simoes CF, de Oliveira GH, Oltramari K, Bim RH, et al. Triglyceride/glucose index is a reliable alternative marker for insulin resistance in South American overweight and obese children and adolescents. J Pediatr Endocrinol Metab (2019) 32:1163–70. doi: 10.1515/jpem-2019-0037.
37. Lee SB, Kim MK, Kang S, Park K, Kim JH, Baik SJ, et al. Triglyceride glucose index is superior to the homeostasis model assessment of insulin resistance for predicting nonalcoholic fatty liver disease in Korean adults. Endocrinol Metab (2019) 34:179–86. doi: 10.3803/EnM.2019.34.2.179
38. Guo W, Zhu W, Wu J, Li X, Lu J, Qin P, et al. Triglyceride glucose index is associated with arterial stiffness and 10-year cardiovascular disease risk in a chinese population. Front Cardiovasc Med (2021) 8:585776. doi: 10.3389/fcvm.2021.585776.
39. Sung H, Ferlay J, Siegel RL, Laversanne M, Soerjomataram I, Jemal A, et al. Global cancer statistics 2020: GLOBOCAN estimates of incidence and mortality worldwide for 36 cancers in 185 countries. CA Cancer J Clin (2021) 71:209–49. doi: 10.3322/caac.21660.
40. Argirion I, Weinstein SJ, Männistö S, Albanes D, Mondul AM. Serum insulin, glucose, indices of insulin resistance, and risk of lung cancer. Cancer Epidemiol Biomarkers Prev (2017) 26:1519–24. doi: 10.1158/1055-9965.EPI-17-0293.
41. Petridou ET, Sergentanis TN, Antonopoulos CN, Dessypris N, Matsoukis IL, Aronis K, et al. Insulin resistance: an independent risk factor for lung cancer? Metabolism (2011) 60:1100–6. doi: 10.1016/j.metabol.2010.12.002.
42. Wang L, Si S, Li J, Li Y, Chen X, Xue F, et al. Triglyceride-glucose index is not associated with lung cancer risk: A prospective cohort study in the UK biobank. Front Oncol (2021) 11:774937. doi: 10.3389/fonc.2021.774937.
43. Luo J, Chlebowski R, Wactawski-Wende J, Schlecht NF, Tinker L, Margolis KL. Diabetes and lung cancer among postmenopausal women. Diabetes Care (2012) 35:1485–91. doi: 10.2337/dc11-2108.
44. Qiu R, Miao T, Lin L, Wei Z. Biological functions of nuclear-localized insulin receptor (IR) on A549 lung cancer cells. Endokrynol Pol (2022) 73(1):121–30. doi: 10.5603/EP.a2021.0099
45. LeRoith D, Yakar S. Mechanisms of disease: metabolic effects of growth hormone and insulin-like growth factor 1. Nat Clin Pract Endocrinol Metab (2007) 3:302–10. doi: 10.1038/ncpendmet0427.
46. Siddle K. Signalling by insulin and IGF receptors: supporting acts and new players. J Mol Endocrinol (2011) 47:R1–R10. doi: 10.1530/JME-11-0022.
47. Pollak MN, Schernhammer ES, Hankinson SE. Insulin-like growth factors and neoplasia. Nat Rev Cancer (2004) 4:505–18. doi: 10.1038/nrc1387.
48. Qi L, Liu F, Zhang F, Zhang S, Lv L, Bi Y, et al. lncRNA NEAT1 competes against let-7a to contribute to non-small cell lung cancer proliferation and metastasis. BioMed Pharmacother (2018) 103:1507–15. doi: 10.1016/j.biopha.2018.04.053.
49. Tang H, Bai Y, Pan G, Wang X, Wei Y, Yang Z, et al. Interleukin-6 and insulin-like growth factor-1 synergistically promote the progression of NSCLC. Autoimmunity (2018) 51:399–407. doi: 10.1080/08916934.2018.1550079.
50. Kim JS, Kim ES, Liu D, Lee JJ, Solis L, Behrens C, et al. Prognostic implications of tumoral expression of insulin like growth factors 1 and 2 in patients with non-small-cell lung cancer. Clin Lung Cancer (2014) 15:213–21. doi: 10.1016/j.cllc.2013.12.006.
51. Xu X, Qiu Y, Chen S, Wang S, Yang R, Liu B, et al. Different roles of the insulin-like growth factor (IGF) axis in non-small cell lung cancer. Curr Pharm Des (2022) 28:2052–64. doi: 10.2174/1381612828666220608122934
52. Agulló-Ortuño MT, Díaz-García CV, Agudo-López A, Pérez C, Cortijo A, Paz-Ares L, et al. Relevance of insulin-like growth factor 1 receptor gene expression as a prognostic factor in non-small-cell lung cancer. J Cancer Res Clin Oncol (2015) 141:43–53. doi: 10.1007/s00432-014-1787-z.
53. Gately K, Forde L, Cuffe S, Cummins R, Kay EW, Feuerhake F, et al. High coexpression of both EGFR and IGF1R correlates with poor patient prognosis in resected non-small-cell lung cancer. Clin Lung Cancer (2014) 15:58–66. doi: 10.1016/j.cllc.2013.08.005.
54. Xu J, Bie F, Wang Y, Chen X, Yan T, Du J. Prognostic value of IGF-1R in lung cancer: A PRISMA-compliant meta-analysis. Med (Baltimore) (2019) 98:e15467. doi: 10.1097/MD.0000000000015467.
55. Al-Saad S, Richardsen E, Kilvaer TK, Donnem T, Andersen S, Khanehkenari M, et al. The impact of MET, IGF-1, IGF1R expression and EGFR mutations on survival of patients with non-small-cell lung cancer. PloS One (2017) 12:e0181527. doi: 10.1371/journal.pone.0181527.
56. Tian Z, Yao G, Song H, Zhou Y, Geng J. IGF2R expression is associated with the chemotherapy response and prognosis of patients with advanced NSCLC. Cell Physiol Biochem (2014) 34:1578–88. doi: 10.1159/000366361.
57. Kelley KM, Oh Y, Gargosky SE, Gucev Z, Matsumoto T, Hwa V, et al. Insulin-like growth factor-binding proteins (IGFBPs) and their regulatory dynamics. Int J Biochem Cell Biol (1996) 28:619–37. doi: 10.1016/1357-2725(96)00005-2.
58. Iams WT, Lovly CM. Molecular pathways: clinical applications and future direction of insulin-like growth factor-1 receptor pathway blockade. Clin Cancer Res (2015) 21:4270–7. doi: 10.1158/1078-0432.CCR-14-2518.
59. Tognon CE, Sorensen PH. Targeting the insulin-like growth factor 1 receptor (IGF1R) signaling pathway for cancer therapy. Expert Opin Ther Targets (2012) 16:33–48. doi: 10.1517/14728222.2011.638626.
60. Takahashi SI, Perks CM. Editorial: the role of the IGF/insulin-IGFBP axis in normal physiology and disease. Front Endocrinol (Lausanne) (2022) 13:892140. doi: 10.3389/fendo.2022.892140.
61. Hu Q, Zhou Y, Ying K, Ruan W. IGFBP, a novel target of lung cancer? Clin Chim Acta (2017) 466:172–7. doi: 10.1016/j.cca.2017.01.017.
62. Remsing Rix LL, Sumi NJ, Hu Q, Desai B, Bryant AT, Li X, et al. IGF-binding proteins secreted by cancer-associated fibroblasts induce context-dependent drug sensitization of lung cancer cells. Sci Signal (2022) 15:eabj5879. doi: 10.1126/scisignal.abj5879.
63. Kahn CR. The molecular mechanism of insulin action. Annu Rev Med (1985) 36:429–51. doi: 10.1146/annurev.me.36.020185.002241.
64. Li Z, Zhang H. Reprogramming of glucose, fatty acid and amino acid metabolism for cancer progression. Cell Mol Life Sci (2016) 73:377–92. doi: 10.1007/s00018-015-2070-4.
65. Masi T, Patel BM. Altered glucose metabolism and insulin resistance in cancer-induced cachexia: a sweet poison. Pharmacol Rep (2021) 73:17–30. doi: 10.1007/s43440-020-00179-y
66. Jiang X, Wang J, Deng X, Xiong F, Zhang S, Gong Z, et al. The role of microenvironment in tumor angiogenesis. J Exp Clin Cancer Res (2020) 39:204. doi: 10.1186/s13046-020-01709-5.
67. Detaille D, Guigas B, Chauvin C, Batandier C, Fontaine E, Wiernsperger N, et al. Metformin prevents high-glucose-induced endothelial cell death through a mitochondrial permeability transition-dependent process. Diabetes (2005) 54:2179–87. doi: 10.2337/diabetes.54.7.2179.
68. Yoshikawa T, Noguchi Y, Doi C, Makino T, Nomura K. Insulin resistance in patients with cancer: relationships with tumor site, tumor stage, body-weight loss, acute-phase response, and energy expenditure. Nutrition (2001) 17:590–3. doi: 10.1016/S0899-9007(01)00561-5.
69. Sunahara KK, Martins JO. Alveolar macrophages in diabetes: friends or foes? J Leukoc Biol (2012) 91:871–6. doi: 10.1189/jlb.0911488.
70. Dali-Youcef N, Hnia K, Blaise S, Messaddeq N, Blanc S, Postic C, et al. Matrix metalloproteinase 11 protects from diabesity and promotes metabolic switch. Sci Rep (2016) 6:25140. doi: 10.1038/srep25140.
71. Kasai S, Kokubu D, Mizukami H, Itoh K. Mitochondrial reactive oxygen species, insulin resistance, and nrf2-mediated oxidative stress response-toward an actionable strategy for anti-aging. Biomolecules (2023) 13:1544. doi: 10.3390/biom13101544.
72. Wei Y, Chen K, Whaley-Connell AT, Stump CS, Ibdah JA, Sowers JR. Skeletal muscle insulin resistance: role of inflammatory cytokines and reactive oxygen species. Am J Physiol Regul Integr Comp Physiol (2008) 294:R673–80. doi: 10.1152/ajpregu.00561.2007.
73. Tiganis T. Reactive oxygen species and insulin resistance: the good, the bad and the ugly. Trends Pharmacol Sci (2011) 32:82–9. doi: 10.1016/j.tips.2010.11.006.
74. Aredia F, Czaplinski S, Fulda S, Scovassi AI. Molecular features of the cytotoxicity of an NHE inhibitor: Evidence of mitochondrial alterations, ROS overproduction and DNA damage. BMC Cancer (2016) 16:851. doi: 10.1186/s12885-016-2878-9.
75. ArulJothi KN, Kumaran K, Senthil S, Nidhu AB, Munaff N, Janitri VB, et al. Implications of reactive oxygen species in lung cancer and exploiting it for therapeutic interventions. Med Oncol (2022) 40:43. doi: 10.1007/s12032-022-01900-y.
76. Gao X, Xie Q, Kong P, Liu L, Sun S, Xiong B, et al. Polyphenol- and caffeine-rich postfermented pu-erh tea improves diet-induced metabolic syndrome by remodeling intestinal homeostasis in mice. Infect Immun (2017) 86:e00601–17. doi: 10.1128/IAI.00601-17.
77. Stocks T, Rapp K, Bjørge T, Manjer J, Ulmer H, Selmer R, et al. Blood glucose and risk of incident and fatal cancer in the metabolic syndrome and cancer project (me-can): analysis of six prospective cohorts. PloS Med (2009) 6:e1000201. doi: 10.1371/journal.pmed.1000201.
78. Stocks T, Bjørge T, Ulmer H, Manjer J, Häggström C, Nagel G, et al. Metabolic risk score and cancer risk: pooled analysis of seven cohorts. Int J Epidemiol (2015) 44:1353–63. doi: 10.1093/ije/dyv001.
79. Fumarola C, Bonelli MA, Petronini PG, Alfieri RR. Targeting PI3K/AKT/mTOR pathway in non small cell lung cancer. Biochem Pharmacol (2014) 90(3):197–207. doi: 10.1016/j.bcp.2014.05.011.
80. Yu J, Zhang L, Peng J, Ward R, Hao P, Wang J, et al. Dictamnine, a novel c-Met inhibitor, suppresses the proliferation of lung cancer cells by downregulating the PI3K/AKT/mTOR and MAPK signaling pathways. Biochem Pharmacol (2022) 195:114864. doi: 10.1016/j.bcp.2021.114864.
Keywords: NSCLC, insulin resistance, development, diagnosis, treatment
Citation: Zhan S, Wang L, Wang W and Li R (2024) Insulin resistance in NSCLC: unraveling the link between development, diagnosis, and treatment. Front. Endocrinol. 15:1328960. doi: 10.3389/fendo.2024.1328960
Received: 06 November 2023; Accepted: 07 February 2024;
Published: 20 February 2024.
Edited by:
Eva Surmacz, Allysta Pharmaceuticals, Inc., United StatesReviewed by:
Cesar Miguel Momesso Santos, Faculty Enau, BrazilCopyright © 2024 Zhan, Wang, Wang and Li. This is an open-access article distributed under the terms of the Creative Commons Attribution License (CC BY). The use, distribution or reproduction in other forums is permitted, provided the original author(s) and the copyright owner(s) are credited and that the original publication in this journal is cited, in accordance with accepted academic practice. No use, distribution or reproduction is permitted which does not comply with these terms.
*Correspondence: Ruoran Li, bXVuaTIwMDcwN0AxNjMuY29t
†ORCID: Ruoran Li, orcid.org/0009-0007-9574-0376
Liu Wang, orcid.org/0009-0004-3884-5815
Wenping Wang, orcid.org/0009-0001-7516-2552
Disclaimer: All claims expressed in this article are solely those of the authors and do not necessarily represent those of their affiliated organizations, or those of the publisher, the editors and the reviewers. Any product that may be evaluated in this article or claim that may be made by its manufacturer is not guaranteed or endorsed by the publisher.
Research integrity at Frontiers
Learn more about the work of our research integrity team to safeguard the quality of each article we publish.