- 1Department of Radiology, The Fourth Affiliated Hospital of Harbin Medical University, Harbin, Heilongjiang, China
- 2The Magnetic Resonance Imaging Room, First Affiliated Hospital of Harbin Medical University, Harbin, Heilongjiang, China
Background: The triglyceride glucose (TyG) index is an effective method for determining insulin resistance (IR). Limited research has explored the connection between the TyG index and functionally significant stenosis in hypertensive patients. Furthermore, the connections between the TyG index, fat attenuation index (FAI) and atherosclerotic plaque characteristics are also worth exploring.
Methods: The study screened 1622 hypertensive participants without coronary artery disease history who underwent coronary computed tomography angiography. The TyG index was calculated as ln (fasting glucose [mg/dL] * fasting TG [mg/dL]/2). Adverse plaque characteristics (HRPCs), high-risk plaques (HRPs), FAI, and CT-derived fractional flow reserve (FFRCT) were analyzed and measured for all patients. Functionally significant stenosis causing ischemia is defined as FFRCT ≤ 0.80. Two patient groups were created based on the FFRCT: the FFRCT < 0.80 group and the FFRCT > 0.80 group. In hypertensive patients, the association between the TyG index and FFRCT was examined applying a logistic regression model.
Results: The TyG index was higher for people with FFRCT ≤ 0.80 contrast to those with FFRCT > 0.80. After controlling for additional confounding factors, the logistic regression model revealed a clear connection between the TyG index and FFRCT ≤ 0.80 (OR = 1.718, 95% CI 1.097–2.690, p = 0.018). The restricted cubic spline analysis displayed a nonlinear connection between the TyG index and FFRCT ≤ 0.80 (p for nonlinear = 0.001). The TyG index increased the fraction of individuals with HRPs and HRPCs, FAI raised, and FFRCT decreased (p < 0.05). The multivariate linear regression analysis illustrated a powerfulcorrelation between high TyG index levels and FAI, FFRCT, positive remodeling (PR), and low-attenuation plaque (LAPs) (standardized regression coefficients: 0.029 [p = 0.007], -0.051 [p < 0.001], 0.029 [p = 0.027], and 0.026 [p = 0.046], separately).
Conclusion: In hypertensive patients, the TyG index showed an excellent association with a risk of FFRCT ≤ 0.80. Additionally, the TyG index was also linked to FAI, FFRCT, PR, and LAPs.
Introduction
A major public health concern, hypertension has a morbidity incidence of 23.2% in China (1). A significant predictor of atherosclerosis is hypertension, which often coexists with coronary heart disease (CHD) (2). Coronary artery disease (CAD) is more common in those with hypertension. However, a sizable fraction of individuals do not have CAD (3). In addition, studies have shown that disconnection between the presence of ischemia and the degree of coronary artery stenosis (CS) is common in CHD subjects. Only approximately half of obstructive lesions will lead to ischemia (4). Consequently, developing a low-cost, reliable, and broadly applicable biomarker to identify myocardial ischemia in hypertensive patients is crucial.
The seriousness and type of any coronary artery atherosclerosis, as well as the coronary anatomy, may all be assessed by computed tomography coronary angiography (CCTA) (5). CT-derived fractional flow reserve (FFRCT) can be used to accurately locate lesions that lead to decreased blood flow function and detect lesion-specific ischemia, and the gold standard for invasive coronary angiography (ICA) with fractional flow reserve (FFR) has confirmed it (6, 7). Functionally significant stenosis causing ischemia with significant hemodynamic changes is defined as FFRCT ≤ 0.80 at each patient level (8, 9). An FFR threshold of 0.80 helps identify people who can benefit from coronary revascularization, according to several randomized trials. FFR is a commonly utilized metric for figuring out the functional value of CAD in a lesion-specific way (10).
The triglyceride glucose (TyG) index has been put forward as a simple and economical method to assess insulin resistance (IR) (11). TyG index and the following variables have been proven to be significantly correlated in numerous studies: the prevalence of cardiovascular disease, myocardial infarction risk, the prognosis for chronic heart failure, and in-stent stenosis (12–14). The heightened TyG index is a notable independent risk factor for coronary heart disease, linked to a higher chance of major adverse cardiovascular and cerebral events (15, 16). Additionally, there is a correlation between the rising TyG index and the increasing prevalence of hypertension, and the TyG index can be useful for forecasting the potential for hypertension in ordinary people (17, 18). Our previous investigation revealed an excellent correlation between the TyG index and CS > 50% in hypertensive subjects (3). The TyG index can be applied to forecast the adverse consequences for individuals with coronary heart disease or hypertension (2). However, the current study is limited to exploring the connection between anatomical CS and the TyG index in hypertensive individuals, and research has indicated that coronary artery function outweighs the effect of anatomy on clinical outcomes (10). In the literature, there is a lack of research that pays attention to the association between the TyG index and functional significant coronary artery stenosis in hypertensive individuals. Furthermore, no research has been done on the correlation between the TyG index, the fat attenuation index (FAI) of pericoronary adipose tissue (PCAT) and traditional plaque features in hypertensive patients.
Therefore, in this study our goal consists of: (1) exploring the connection between functional significant stenosis and TyG index in hypertensive individuals; (2) studying the association between pericoronary adipose tissue index, hemodynamics, plaque characteristics, and TyG index in patients affected by hypertension.
Materials and methods
Study population
The research was cross-sectional and observational. This research included consecutive subjects with hypertension who met the 2017 ACC/AHA guidelines (3) and underwent CCTA in the Fourth Affiliated Hospital of Harbin Medical University during January 2022 and January 2023 due to typical or atypical chest pain. Exclusion criteria included inaccessible fasting glucose and triglyceride (TG) values, malignant disease, uncontrolled hyperthyroidism or hypothyroidism, and significant hepatic or renal failure. Moreover, those on low-triglyceride medications were excluded. Finally, 1622 eligible participants were enrolled for analysis in our research (a detailed flow chart is displayed in Figure 1).
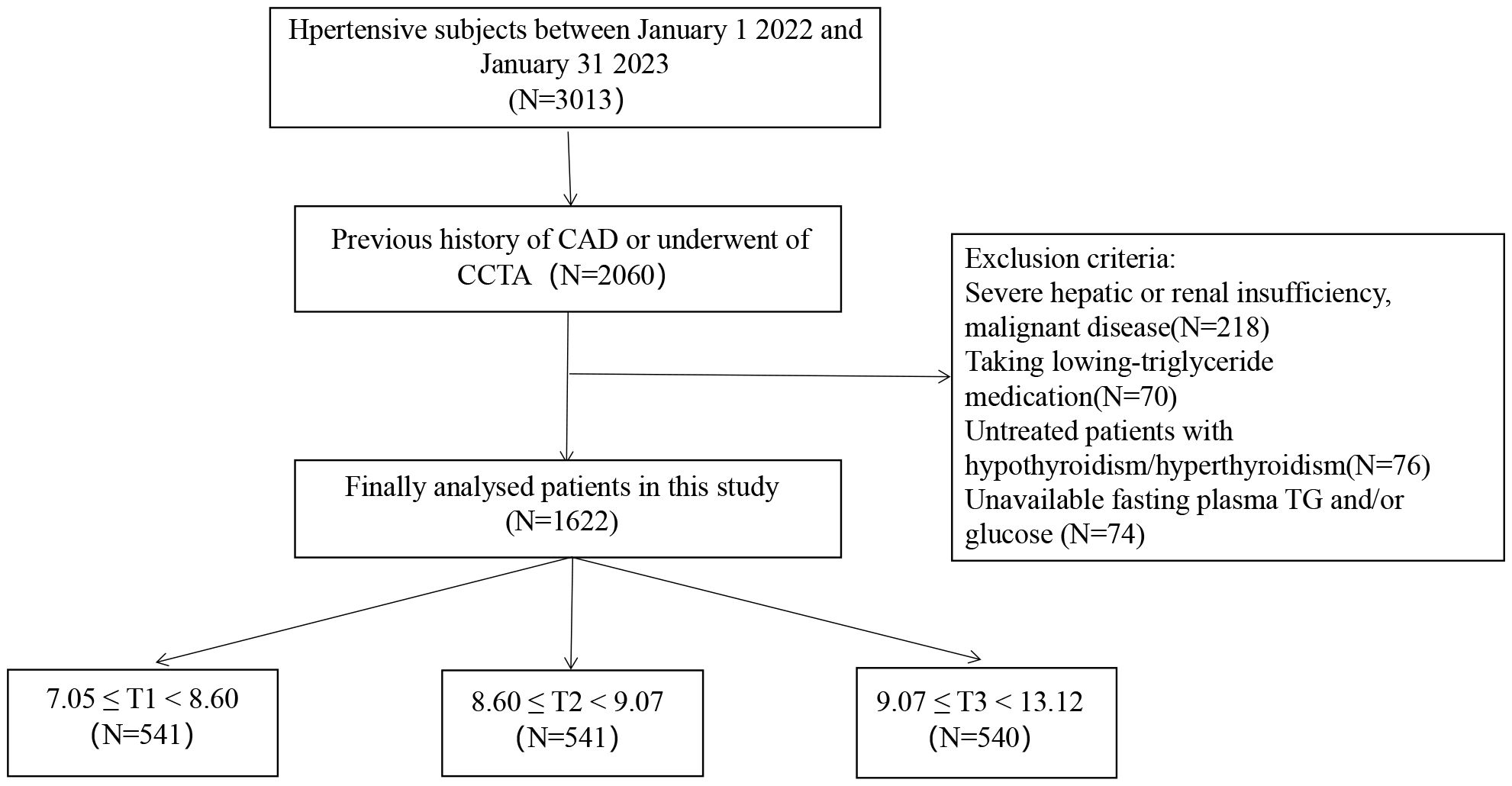
Figure 1 Study flow chart. CAD, coronary artery disease; CCTA, coronary computed tomography angiography; TG, triglyceride; T, tertile.
This study program is executed pursuant to the principles delineated in the Helsinki Declaration, and it has been endorsed by our institutional committee in conformity with local ethical norms (Approved No. of Ethics Committee: 2023–41). Given that this is a retroactive inquiry, the patient’s informed consent was discarded.
Data acquisition
From each patient’s medical history, the following details were gathered: age, sex, smoking, drinking, and history of prescription use. All participants had their fasting venous blood samples taken early in the morning. Laboratory analysis included measurements of creatinine, uric acid (UA), fasting blood glucose (FBG), total cholesterol (TC), high density lipoprotein (HDL-C), low density lipoprotein (LDL-C), triglyceride (TG) and other biochemical indices. The TyG index was calculated by ln (fasting TG [mg/dL] * fasting glucose [mg/dL]/2) (11). The formula for calculating body mass index (BMI) was weight divided by square height (19). Hyperlipidemia was characterized as TG ≥ 2.3 mmol/L, LDL-C ≥ 4.1 mmol/L, HDL-C ≤ 1.0 mmol/L or TC ≥ 6.2 mmol/L (20). Glycosylated hemoglobin ≥ 6.5% and FBG ≥ 7.0 mmol/L or random blood glucose > 11.1 mmol/L were the diagnostic criteria for diabetic mellitus (DM) (21).
CT image acquisition
Every scan was conducted by a 320-detector-row CT scanner (Aquilion ONE, Toshiba, Tokyo, Japan), with noncontrast chest CT scans at admission and coronary CTA scans during hospital stays. Fast heart rate individuals were given oral metoprolol (25–75 mg) at baseline one hour before the test.
It was necessary to use a dual-channel high-pressure syringe to inject 60–80 mL of nonionic iodine contrast agent. Coronary CTA images were taken using prospective ECG triggering. The settings used in our experiment for acquisition and reconstruction were the rack rotation speed at 275 ms/time, tube potential at 120 kV, automatic tube current modification based on patient BMI, and 0.5 mm slice increments. Two expert investigators in cardiovascular imaging reviewed the collected imaging data.
Visual recognition of adverse plaque characteristics
Segment analysis was utilized to detect adverse plaque characteristics (HRPCs) consisting of spotty calcification, positive remodeling (PR), low-attenuation plaque (LAPs), and the napkin ring sign (NRS). Low-attenuation plaque has an attenuation density of less than 30 Hounsfield Units (HU), while coronary artery wall calcification with a diameter of less than 3 mm was classified as spotty calcification. An outer vessel diameter greater than 10% of the mean diameter of the segments immediately proximal and distal to the plaque was considered positive remodeling. The ‘napkin ring’ sign, which consists of a high-attenuation outer rim surrounding a low-attenuation core region (22). High-risk plaques (HRPs) were defined as those that met two or more of those criteria (23).
Pericoronary fat attenuation index acquisition
The PCAT was quantitatively quantified using specialized software (Shukun Technology).
For all voxels in the range of -190 to -30 Hounsfield units (HU), PCAT is defined as the radial distance from the vessel wall equal to the vessel diameter. The average CT attenuation of PCAT (HU) is quantified by the FAI (24). We analyzed PCAT in the 10- to 50-mm segment proximal to the right coronary artery (RCA). The CT measurement of FAI is fully automated and requires a small amount of additional manual optimization.
Analysis of hemodynamic parameters of CCTA
A computational fluid dynamics-based software model (Shukun Technology) was utilized for the hemodynamic study. The software is used to segment the three-dimensional coronary artery model semiautomatically, and the FFRCT value of any stenosis at the distal end of each vessel is recorded. When there are numerous stenoses, the distal FFRCT value of the most severe lesion is used. If there was no stenosis, the FFRCT value of the middle segment of the vessel was recorded.
Statistical analyses
Datasets were examined using R version 4.2.2 and SPSS (25.0, IBM Corp, New York).
Normally distributed data are represented by the means ± standard deviations and were contrasted with an analysis of variance, or T test. Nonparametric data, which are presented as medians (interquartile ranges), were analyzed by the Mann−Whitney U test or the Kruskal−Wallis H test. Categorical variables were examined with the Chi-squared test and are described as percentages. The study utilized logistic regression analysis to examine the connection between FFRCT and the TyG index, incorporating confounding variables into the regression equation to account for potential baseline data impacts. Model 1 was modified for sex, BMI, and age, and Model 2 was modified for drinking, smoking, hyperlipidemia, DM, creatine, UA, TG, FBG, ALT, HDL-C, and ApoA, which rested on Model 1. Model 3 was modified for NRS, LAPs, PR, spotty calcification, HRPs, coronary artery calcium score (CACS), and FAI, which rested on Model 2. The nonlinear connection with the TyG index and FFRCT ≤ 0.80 was investigated using restricted cubic splines. The independent variables causing the increase in the TyG index were found using both univariate and multivariate linear regression. A p value of < 0.05 is deemed statistically significant.
Results
Baseline characteristics of the patient cohort
This study examined 1622 hypertensive patients, revealing that males constituted 45.07% of the population and 53.58% had hyperlipidemia. In all, 554 (34.16%) individuals had myocardial ischemia (FFRCT ≤ 0.80). Within the study population, the patients’ average age was 62.43 ± 10.12 years. The median TyG index was 8.84 (IQR 8.48-9.22). The TyG index tertile was utilized to divide the available participants into three groups in Table 1.
Individuals with the highest TyG index were male, younger, had a higher BMI, smoking, and had a history of diabetes and hyperlipidemia (p < 0.05). The TyG index seemed to have an inverse relationship with HDL-C, apolipoprotein A (ApoA), lipoprotein (A) [Lp (A)], FFRCT and a positive relationship with TG, FBG, TC, apolipoprotein B (ApoB), UA, alanine aminotransferase (ALT), and CACS (p < 0.05). Likewise, we noticed a noticeable rise in the incidence of FFRCT ≤ 0.80 as the TyG index rose. (p < 0.001).
There were notable variations across the three groups, and the FAI rose as the TyG index value increased (p < 0.001). Group T3 had a greater occurrence of HRPs and HRPCs, which comprise spotty calcification, PR, LAPs, and NRS, in comparison to Groups T1 or T2 (p < 0.05).
Baseline characteristics of groups categorized with FFRCT
The baseline features of the subjects are illustrated in Table 2. Compared with the control group, the majority of the subjects with FFRCT ≤ 0.80 were described as follows: old, male, smoking, drinking, and a history of hyperlipidemia and DM (p < 0.05). The FFRCT ≤ 0.80 group displayed a greater TG, ApoB, FBG, creatine, UA, and TyG index but a lower ApoA and HDL-C. The median FFRCT values for the FFRCT > 0.80 and FFRCT ≤ 0.80 groups were 0.90 and 0.71, correspondingly. HRPs and HRPCs, including spotty calcification, PR, NRS, and LAPs, were more likely to occur in individuals with an FFRCT ≤ 0.80 (p < 0.05).
Moreover, in contrast to the FFRCT > 0.80 group, the FFRCT ≤ 0.80 group had a significantly greater proportion of HRPCs (Additional Table 1). The percentage of HRPCs varied significantly between the two groups (p < 0.001; Additional Figure 1). Lesions with ≥ 2 HRPCs appeared in 26.71% and 11.80% of the two groups, respectfully (Additional Table 1, Additional Figure 1). In contrast to the FFRCT > 0.80 group, the FAI and CACS values in the FFRCT < 0.80 group were greater (p = 0.002, p < 0.001).
Association between the TyG index and FFRCT
The FFRCT < 0.80 and the TyG index showed a positive connection in an unadjusted logistic regression model. Following the adjustment for other variables, there was still a separate correlation between the TyG index and FFRCT ≤ 0.80 in Model 1, Model 2, and Model 3 (OR = 2.537, 95% CI 2.100–3.065, p < 0.001; OR = 2.135, 95% CI 1.403–3.247, p < 0.001; OR = 1.718, 95% CI 1.097–2.690, p = 0.018) (Table 3). The TyG index exhibited a nonlinear correlation with an FFRCT ≤ 0.80 in its continuous range (p for nonlinear = 0.001) (Figure 2).
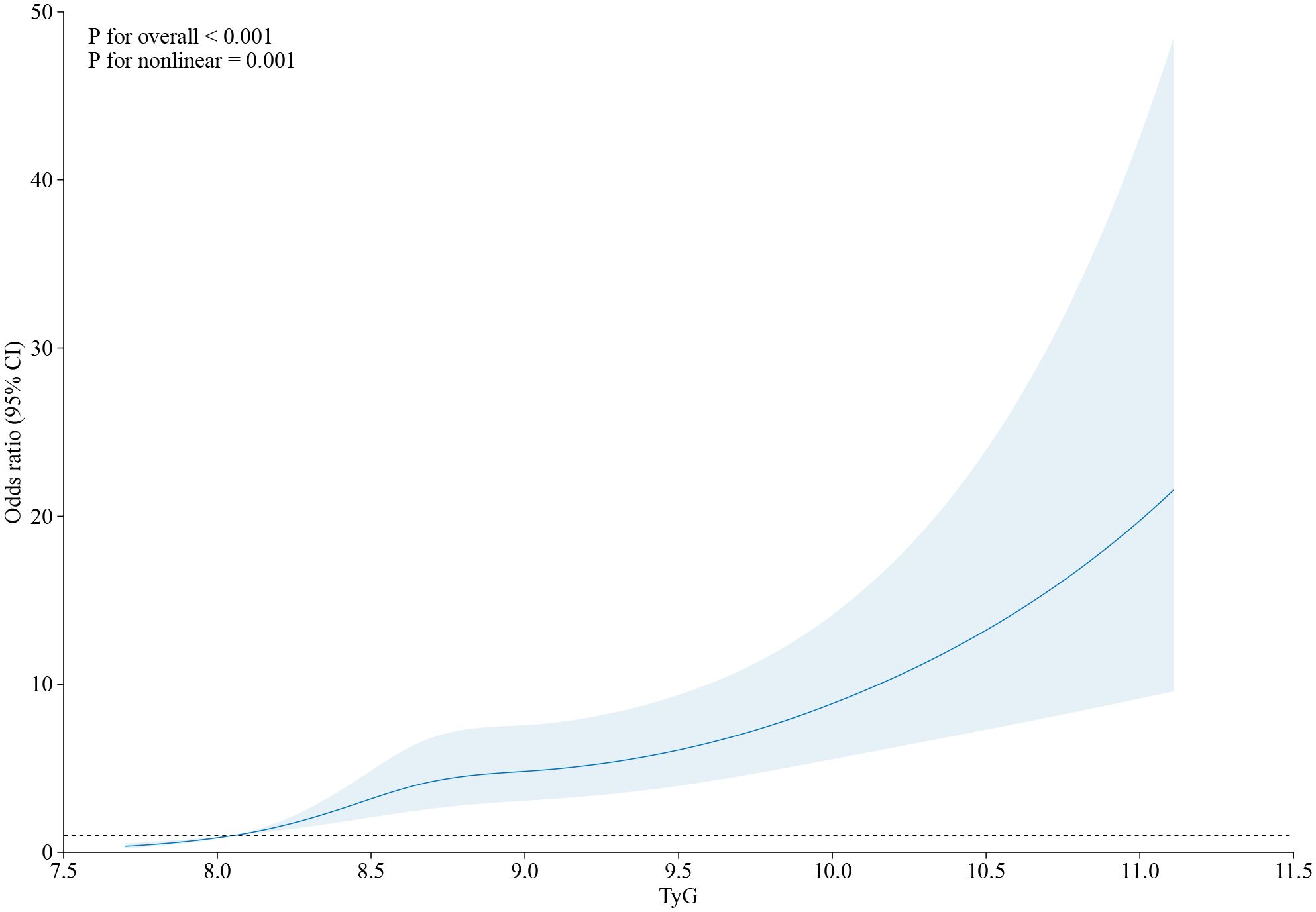
Figure 2 The restricted cubic spline of TyG index and the incidence of FFRCT ≤ 0.80. Odds ratios and 95% CIs derived from restricted cubic spline regression, with knots placed at the 5th, 35th, 65th, and 95th percentiles of the distribution of TyG index. OR, odds ratio; CI, confidence interval; CAD, coronary artery disease; TyG index, triglyceride glucose index; RCS, restricted cubic spline.
TyG index and FFRCT relationships in specific subgroups
The correlation between FFRCT ≤ 0.80 and the TyG index was evaluated across several subgroups (Figure 3). In the subgroups of males, BMI < 24 kg/m2, no drinking, no PR, and no LAPs, the positive correlation across FFRCT ≤ 0.80 and the TyG index was more notable after controlling for other variables.
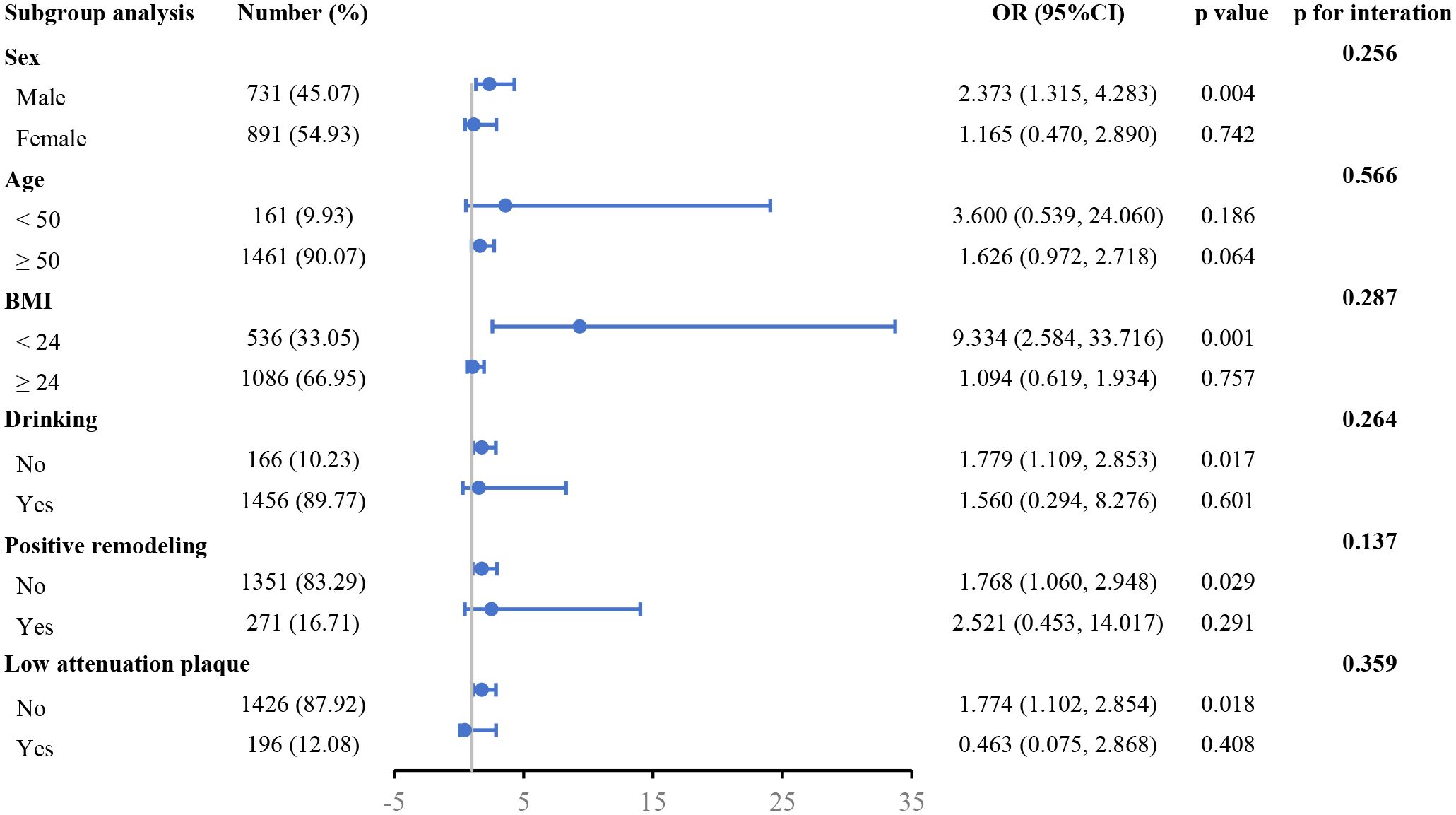
Figure 3 Forest plot investigating the association between the TyG index and with the risk of FFRCT ≤ 0.80 in different subgroups. TyG, triglyceride glucose; FFRCT, CT-derived fractional flow reserve; BMI, body mass index; OR, odds ratio; CI, confidence interval.
CCTA imaging characteristics are linked to elevated TyG index levels
The variables linked to elevated TyG index levels were identified through the use of univariate and multivariate analyses (Table 4). Within the univariate analysis, a greater TyG index was closely linked with PR, spotty calcification, LAPs, NRS, HRPs, FAI, and FFRCT. A greater TyG index value was independently linked with FFRCT, FAI, PR, and LAPs in the multivariate analysis (standardized regression coefficients: -0.051 [p < 0.001], 0.029 [p = 0.007], 0.029 [p = 0.027], and 0.026 [p = 0.046], separately).
Discussion
The following were the study’s main points: (1) In individuals with hypertension, the TyG index significantly affects the incidence of functionally significant stenosis. It implies that in identifying hypertensive patients at greater risk of myocardial ischemia and directing further detection and more intensive care, the TyG index may be useful. (2) The TyG index showed independent correlations with CT-derived fractional flow reserve (FFRCT), the fat attenuation index (FAI), low-attenuation plaque and positive remodeling in hypertensive patients.
The prominent risk cause for cardiovascular disease, hypertension is associated with worse outcomes. Compared to those without hypertension, subjects with hypertension exhibited more advanced coronary atherosclerosis by CCTA and a greater chance for major adverse cardiac events in the future (25). Therefore, identifying hypertensive individuals at increased risk for CAD is essential for guiding further diagnosis and early treatment. However, standard CCTA results related to coronary stenosis often overstate the degree of lesion-specific ischemia in CAD patients with severe grade stenosis (26, 27). FFRCT is a noninvasive physiological detection method that is useful for precisely assessing lesion-specific ischemia and is superior to stenosis on coronary CT angiograms of both 50% or greater and greater than 70% in predicting clinical outcomes (28). Currently, a positive index of myocardial ischemia with FFRCT < 0.80 has been utilized to direct interventional treatment and assess prognosis (28, 29). Therefore, it is of clinical value to determine the risk factors influencing FFRCT ≤ 0.80; they may have a bearing on early intervention.
It is commonly accepted that insulin resistance raises the risk of oxidative stress, inflammation, atherosclerosis, and endothelial dysfunction—all of which are prevalent in hypertension (30). The TyG index has already shown to be a faithful easy IR estimate indicator lately and is comparable to the gold standard for assessing IR, the euglycemic-hyperinsulinemic clamp technique (31, 32). Research has demonstrated that when predicting insulin resistance using the hyperglycemic clamp test, the TyG index outperformed the homeostasis model assessment of insulin resistance (HOMA-IR) index (11). Multiple analyses reported a clear connection between the onset of atherosclerotic cardiovascular disease and the TyG index (19, 31, 33). A correlation between the number and severity of coronary artery stenotic lesions and the TyG index was demonstrated by cohort studies (31). Furthermore, the TyG index is one method for forecasting the severity of coronary heart disease, independent of glucose metabolism (34). New research indicates that the brachia-ankle pulse wave velocity and the severity of coronary heart disease are strongly connected with the TyG index, which also relates to the risk of subclinical arteriosclerosis in hypertensive individuals (3, 33). However, no research has explored the connection between FFRCT ≤ 0.80 and the TyG index in hypertensive patients. The TyG index and myocardial ischemia were shown to be correlated in this study, with a greater TyG index level indicating a better chance of FFRCT ≤ 0.80. Treatment approaches that reduce the TyG index is possible helpful to reduce future myocardial ischemia in hypertensive patients. Furthermore, subgroup analysis findings indicated that FFRCT ≤ 0.80 and the TyG index were likewise stable within various subgroups. Surprisingly, the subgroups of patients classified as men, BMI < 24 kg/m2, no drinking, no PR, and no LAPs showed a greater association with this connection. Although the precise process is unknown, it is a consideration that we must all take into account. Therefore, further exploration and research are needed in the future.
The TyG index and FFRCT may be linked in hypertensive patients according to their assessment of IR status, although the precise mechanism behind this relationship is yet unknown. First, through oxidative stress, IR can impair the function of the coronary endothelium and cause inflammation, which leads to coronary artery dysfunction (21, 34). Second, IR is characterized by impaired vasodilation, microvascular disease, and atherosclerotic disease. Hemodynamic damage reduces the blood flow of the epicardial coronary artery (21, 35). Future research may be conducted to elucidate the connection between FFRCT and the TyG index.
Additionally, this research also evaluated the correlation between the TyG index and adverse plaque characteristics (HRPCs) and the FAI in hypertensive patients. Damage to vascular endothelial cells brought on by IR can trigger an inflammatory reaction (23). It has been demonstrated that coronary artery inflammation inhibits fat formation in perivascular fat, thereby altering the attenuation of perivascular fat to CCTA (36). It has been demonstrated that the FAI index, which is based on the attenuation of pericoronary adipose tissue (PCAT) on CCTA, indicates the inflammation of the coronary artery wall. Around the proximal RCA, PCAT attenuation is a biomarker of worldwide coronary inflammation (29). This might explain why FAI and the TyG index are correlated. Simultaneously, our research demonstrates that individuals with an increased serum TyG index also frequently have high-risk plaques (HRPs) and typical HRPCs. This result is in line with other research (23, 37), showing a relationship between plaque stability and the TyG index. Thus, we hypothesize that serum TyG index level and plaque instability may be related.
Our research examined the correlation between FFRCT ≤ 0.80 and the TyG index in hypertensive individuals and further investigated the links between the TyG index and FAI and HRPs. This research holds significant clinical utility. First, our research demonstrates that the TyG index is a risk indicator for FFRCT ≤ 0.80 and that reducing the index may protect hypertensive patients from myocardial ischemia. Therefore, more interventional research is needed to ascertain if therapy targeted at lowering the quantity of IR as indicated by the TyG index improves myocardial ischemia in hypertensive individuals. Secondly, the TyG index is a blood indicator that may be used in real time to assess a patient’s coronary physiological function during follow-up since it is readily measured, accessible, and repeatable. In addition, we observed that an increased TyG index is more probably to be associated with HRPs and correspond to a higher FAI; this consideration will be helpful in further exploration of the effects of IR on plaque instability and coronary artery wall inflammation in hypertensive patients.
This study is restricted by some limitations. First, the study was cross-sectional and retrospective observational. We screened subjects in order. Some intrinsic variances do exist, though. Second, it has been reported that FFRCT is consistent with fractional flow reserve (FFR) in invasive coronary angiography (ICA). However, FFRCT is still not the best method for evaluating coronary artery disease-specific ischemia. Furthermore, our research did not include any quantitative evaluation of coronary artery plaque, which is an aspect that warrants analysis in future research.
Conclusion
This study provides some proof that in hypertensive patients, the TyG index is independently connection with an elevated chance of FFRCT ≤ 0.80. This will give useful data for early risk assessment and direct further detection efforts, and steer more focused treatment plans. Simultaneously, hypertensive patients who had a greater TyG index also had greater odds of HRPs, a lower FFRCT, and a higher FAI. Additionally, a separate correlation was seen between the TyG index and positive remodeling, low-attenuation plaque, FAI, and FFRCT.
Data availability statement
The raw data supporting the conclusions of this article will be made available by the authors, without undue reservation.
Ethics statement
The study protocol was approved by the Medical Ethics Committee of The Fourth Affiliated Hospital of Harbin Medical University (Approved No. of Ethics Committee: 2023–41) and conducted in accordance with the principles contained within the Declaration of Helsinki, but patient informed consent was waived because it was a retrospective study.
Author contributions
SW: Conceptualization, Data curation, Formal analysis, Investigation, Methodology, Visualization, Writing – original draft, Writing – review & editing. ZS: Conceptualization, Data curation, Formal analysis, Investigation, Methodology, Visualization, Writing – original draft. HP: Conceptualization, Methodology, Project administration, Writing – original draft. TY: Data curation, Investigation, Project administration, Writing – original draft. LL: Conceptualization, Data curation, Methodology, Writing – original draft. JX: Conceptualization, Methodology, Writing – original draft. WW: Data curation, Investigation, Project administration, Visualization, Writing – review & editing. TZ: Conceptualization, Formal analysis, Funding acquisition, Investigation, Methodology, Project administration, Software, Supervision, Writing – original draft, Writing – review & editing.
Funding
The author(s) declare that financial support was received for the research, authorship, and/or publication of this article. The Beijing Cihua Medical Development Foundation Project (Research on CT-assisted diagnosis of coronary heart disease based on artificial intelligence), the important research and development projects in Heilongjiang province (Grant NO. JD22C007); National Natural Science Foundation of China (No.62376078).
Acknowledgments
We thank Jinquan Bai from the Department of Radiology, The Fourth Affiliated Hospital of Harbin Medical University, China, for his guidance and help in revising the article. We thank Min Zhao from Pharmaceutical Diagnostics, GE Healthcare, Beijing, China, for our statistical analysis.
Conflict of interest
The authors declare that the research was conducted in the absence of any commercial or financial relationships that could be construed as a potential conflict of interest.
Publisher’s note
All claims expressed in this article are solely those of the authors and do not necessarily represent those of their affiliated organizations, or those of the publisher, the editors and the reviewers. Any product that may be evaluated in this article, or claim that may be made by its manufacturer, is not guaranteed or endorsed by the publisher.
Supplementary material
The Supplementary Material for this article can be found online at: https://www.frontiersin.org/articles/10.3389/fendo.2024.1323722/full#supplementary-material
Abbreviations
TyG index, Triglyceride glucose index; IR, Insulin resistance; FAI, Fat attenuation index; CAD, Coronary artery disease; CCTA, Computed tomography coronary angiography; FFRCT, CT-derived fractional flow reserve; HRPCs, Adverse plaque characteristics; PR, Positive remodeling; LAPs, Low-attenuation plaque; NRS, The napkin ring sign; HRPs, High-risk plaques; CHD, Coronary heart disease; CS, Coronary artery stenosis; ICA, Invasive coronary angiography; FFR, Fractional flow reserve; PCAT, Pericoronary adipose tissue; ALT, Alanine aminotransferase; AST, Aspartate aminotransferase; UA, Uric acid; TC, Total cholesterol; TG, Triglyceride; HDL-C, High density lipoprotein; LDL-C, Low density lipoprotein; FBG, Fasting blood glucose; ApoA, Apolipoprotein A; ApoB, Apolipoprotein B; Lp (A), Lipoprotein (A); BMI, Body mass index; DM, Diabetes mellitus; RCA, Right coronary artery; OR, Odds ratio; CI, Confidence interval; CACS, Coronary artery calcium score; HOMA-IR, Homeostasis model assessment insulin resistance.
References
1. Zhang F, Kadierding R, Zhang S, Yang H, Ren L, Ren M, et al. Triglyceride glucose index for predicting major adverse cardiovascular events in Chinese patients with hypertension. Angiology. (2022) 74:259–67. doi: 10.1177/00033197221104559
2. Liu Y, Zhu B, Zhou W, Du Y, Qi D, Wang C, et al. Triglyceride–glucose index as a marker of adverse cardiovascular prognosis in patients with coronary heart disease and hypertension. Cardiovasc Diabetol. (2023) 22:133. doi: 10.1186/s12933-023-01866-9
3. Pan W, Ren Y, Yang F, Wang M, Li X, Yin D. Triglyceride glucose index is associated with obstructive coronary artery disease in hypertensive patients. Cardiovasc Diabetol. (2023) 22:9. doi: 10.1186/s12933-023-01739-1
4. Gaur S, Øvrehus KA, Dey D, Leipsic J, Bøtker HE, Jensen JM, et al. Coronary plaque quantification and fractional flow reserve by coronary computed tomography angiography identify ischaemia-causing lesions. Eur Heart J. (2016) 37:1220–7. doi: 10.1093/eurheartj/ehv690
5. Venuraju SM, Lahiri A, Jeevarethinam A, Cohen M, Darko D, Nair D, et al. Duration of type 2 diabetes mellitus and systolic blood pressure as determinants of severity of coronary stenosis and adverse events in an asymptomatic diabetic population: PROCEED study. Cardiovasc Diabetol. (2019) 18:51. doi: 10.1186/s12933-019-0855-8
6. Xie Z, Zhu J, Li W, Liu L, Zhuo K, Yang R, et al. Relationship of epicardial fat volume with coronary plaque characteristics, coronary artery calcification score, coronary stenosis, and CT-FFR for lesion-specific ischemia in patients with known or suspected coronary artery disease. Int J Cardiol. (2021) 332:8–14. doi: 10.1016/j.ijcard.2021.03.052
7. Yoon YE, Koo B-K. Non-invasive functional assessment using computed tomography: when will they be ready for clinical use? Cardiovasc Diagnosis And Ther. (2012) 2:106–12. doi: 10.3978/j.issn.2223-3652.2012.04.02
8. Rabkin SW, Waheed A, Poulter RS, Wood D. Myocardial perfusion pressure in patients with hypertension and coronary artery disease: implications for DBP targets in hypertension management. J Hypertens. (2013) 31:975–82. doi: 10.1097/HJH.0b013e32835e831c
9. Wu M, Feng J, Zhang Z, Zhang N, Yang F, Li R, et al. Sex-specific associations of cardiovascular risk factors and coronary plaque composition for hemodynamically significant coronary artery stenosis: a coronary computed tomography angiography study. BMC Cardiovasc Disord. (2023) 23:423. doi: 10.1186/s12872-023-03438-x
10. Nørgaard BL, Leipsic J, Gaur S, Seneviratne S, Ko BS, Ito H, et al. Diagnostic performance of noninvasive fractional flow reserve derived from coronary computed tomography angiography in suspected coronary artery disease the NXT trial (Analysis of coronary blood flow using CT angiography: next steps). J Am Coll Cardiol. (2014) 63:1145–55. doi: 10.1016/j.jacc.2013.11.043
11. Hong S, Han K, Park C-Y. The triglyceride glucose index is a simple and low-cost marker associated with atherosclerotic cardiovascular disease: a population-based study. BMC Med. (2020) 18:361. doi: 10.1186/s12916-020-01824-2
12. Tao S, Yu L, Li J, Huang Li, Huang X, Zhang W, et al. Association between the triglyceride-glucose index and 1-year major adverse cardiovascular events in patients with coronary heart disease and hypertension. Cardiovasc Diabetol. (2023) 22:305. doi: 10.1186/s12933-023-02018-9
13. Dou J, Guo C, Wang Y, Peng Z, Wu R, Li Q, et al. Association between triglyceride glucosebody mass and one-year all-cause mortality of patients with heart failure: a retrospective study utilizing the MIMIC-IV database. Cardiovasc Diabetol. (2023) 22:309. doi: 10.1186/s12933-023-02047-4
14. Yang Yu, Ma M, Zhang J, Jin S, Zhang D, Lin X. Triglyceride-glucose index in the prediction of clinical outcomes after successful recanalization for coronary chronic total occlusions. Cardiovasc Diabetol. (2023) 22:304. doi: 10.1186/s12933-023-02037-6
15. Tao L-C, Xu J-N, Wang T-T, Hua F, Li J-J. Triglyceride-glucose index as a marker in cardiovascular diseases: landscape and limitations. Cardiovasc Diabetol. (2022) 21:68. doi: 10.1186/s12933-022-01511-x
16. Li H, Zuo Y, Qian F, Chen S, Tian X, Wang P, et al. Triglyceride-glucose index variability and incident cardiovascular disease: a prospective cohort study. Cardiovasc Diabetol. (2022) 21:105. doi: 10.1186/s12933-022-01541-5
17. Jian S, Su-Mei N, Xue C, Jie Z, Xue-sen W. Association and interaction between triglyceride–glucose index and obesity on risk of hypertension in middle-aged and elderly adults. Clin Exp Hypertension. (2017) 39:732–9. doi: 10.1080/10641963.2017.1324477
18. Zhao Y, Yang X, Wu Y, Huang H, Hu F, Zhang M, et al. Association of triglyceride–glucose index and its 6-year change with risk of hypertension: A prospective cohort study. Nutrition Metab Cardiovasc Dis. (2023) 33:568–76. doi: 10.1016/j.numecd.2022.12.001
19. Su J, Li Z, Huang M, Wang Y, Yang T, Ma M, et al. Triglyceride glucose index for the detection of the severity of coronary artery disease in diferent glucose metabolic states in patients with coronary heart disease: a RCSCD-TCM study in China. Cardiovasc Diabetol. (2022) 21:96. doi: 10.1186/s12933-022-01523-7
20. Li Z, He Y, Wang S, Li L, Yang R, Liu Y, et al. Association between triglyceride glucose index and carotid artery plaque in diferent glucose metabolic states in patients with coronary heart disease: a RCSCD-TCM study in China. Cardiovasc Diabetol. (2022) 21:38. doi: 10.1186/s12933-022-01470-3
21. Yu B, Mo Y, Hu X, Wang W, Liu J, Jin J, et al. Triglyceride-glucose index is associated with quantitative flow ratio in patients with acute ST-elevation myocardial infarction after percutaneous coronary intervention. Front Cardiovasc Med. (2022) 9:1002030. doi: 10.3389/fcvm.2022.1002030
22. Osborne-Grinter M, Kwiecinski J, Doris M, McElhinney P, Cadet S, Adamson PD, et al. Association of coronary artery calcium score with qualitatively and quantitatively assessed adverse plaque on coronary CT angiography in the SCOT-HEART trial. Eur Heart Journal-cardiovascular Imaging. (2022) 23:1210–21. doi: 10.1093/ehjci/jeab135
23. Ma Y, Zhen Y, Wang M, Gao L, Dang Y, Shang J, et al. Associations between the serum triglyceride-glucose index and pericoronary adipose tissue attenuation and plaque features using dual-layer spectral detector computed tomography: a cross-sectional study. Front Endocrinol. (2023) 14:1166117. doi: 10.3389/fendo.2023.1166117
24. Dong X, Zhu C, Li Na, Shi Ke, Si N, Wang Y, et al. Identification of patients with acute coronary syndrome and representation of their degree of inflammation: application of pericoronary adipose tissue within different radial distances of the proximal coronary arteries. Quantitative Imaging Med And Surg. (2023) 13:3644–59. doi: 10.21037/qims-22-864
25. Nakanishi R, Baskaran L, Gransar H, Budoff MJ, Achenbach S, Al-Mallah M, et al. Relationship of hypertension to coronary atherosclerosis and cardiac events in patients with coronary computed tomographic angiography. Hypertension. (2017) 70:293–9. doi: 10.1161/HYPERTENSIONAHA.117.09402
26. Yang F, Pang Z, Cui S, Ma Y, Li Y, Wang Y, et al. Predictive value of coronary stenosis degree combined with CT-FFR and resting-state CTP for major adverse cardiac events in obstructive coronary artery disease. Medicine. (2023) 102:e34438. doi: 10.1097/MD.0000000000034438
27. Patel MR, Nørgaard BL, Fairbairn TA, Nieman K, Akasaka T, Berman DS, et al. 1-year impact on medical practice and clinical outcomes of FFRCT. JACC: Cardiovasc Imaging. (2020) 13:97–105. doi: 10.1016/j.jcmg.2019.03.003
28. Ihdayhid AR, Norgaard BL, Gaur S, Leipsic J, Nerlekar N, Osawa K, et al. Prognostic value and risk continuum of noninvasive fractional flow reserve derived from coronary CT angiography. Radiology. (2019) 292:343–51. doi: 10.1148/radiol.2019182264
29. Zhou Ke, Shang J, Guo Y, Ma S, Lv B, Zhao Na, et al. Incremental diagnostic value of radiomics signature of pericoronary adipose tissue for detecting functional myocardial ischemia: a multicenter study. Eur Radiol. (2023) 33:3007–19. doi: 10.1007/s00330-022-09377-z
30. Mone P, Gennaro SDe, Moriello D, Frullone S, D’Amelio R, Ferrante MNV, et al. Insulin resistance drives cognitive impairment in hypertensive pre-diabetic frail elders: the CENTENNIAL study. Eur J Prev Cardiol. (2023) 30:1283–8. doi: 10.1093/eurjpc/zwad173
31. Wang X, Xu W, Song Q, Zhao Z, Meng X, Xia C, et al. Association between the triglyceride–glucose index and severity of coronary artery disease. Cardiovasc Diabetol. (2022) 21:168. doi: 10.1186/s12933-022-01606-5
32. Varzideh F, Kansakar U, Jankauskas SS, Gambardella J, Santulli G. Cardiovascular endocrinology: evolving concepts and updated epidemiology of relevant diseases. Front Endocrinol. (2021) 12:772876. doi: 10.3389/fendo.2021.772876
33. Li M, Zhan A, Huang X, Hu L, Zhou W, Wang T, et al. Positive association between triglyceride glucose index and arterial stifness in hypertensive patients: the China H-type Hypertension Registry Study. Cardiovasc Diabetol. (2020) 19:139. doi: 10.1186/s12933-020-01124-2
34. Knudson JD, Dincer UD, Bratz IN, Sturek M, Dick GM, Tune JD. Mechanisms of coronary dysfunction in obesity and insulin resistance. Microcirculation. (2010) 14:317–38. doi: 10.1080/10739680701282887
35. Schulman IH, Zhou M-S. Vascular insulin resistance: A potential link between cardiovascular and metabolic diseases. Curr Hypertension Rep. (2009) 11:48–55. doi: 10.1007/s11906-009-0010-0
36. Oikonomou EK, Marwan M, Desai MY, Mancio J, Alashi A, Centeno EH, et al. Non-invasive detection of coronary inflammation using computed tomography and prediction of residual cardiovascular risk (the CRISP CT study): a post-hoc analysis of prospective outcome data. Lancet. (2018) 392:929–39. doi: 10.1016/S0140-6736(18)31114-0.;392(10151):929-939
Keywords: TyG index, fat attenuation index, CT-derived fractional flow reserve, hypertension, insulin resistance, computed tomography coronary angiography
Citation: Wang S, Shi Z, Pan H, Yan T, Liu L, Xu J, Wang W and Zhang T (2024) Triglyceride glucose index is associated with functional coronary artery stenosis in hypertensive patients. Front. Endocrinol. 15:1323722. doi: 10.3389/fendo.2024.1323722
Received: 18 October 2023; Accepted: 12 March 2024;
Published: 25 March 2024.
Edited by:
Pasquale Mone, University of Molise, ItalyReviewed by:
Fahimeh Varzideh, Albert Einstein College of Medicine, United StatesImma Forzano, University of Naples Federico II, Italy
Antonio de Donato, BioGeM Institute, Italy
Copyright © 2024 Wang, Shi, Pan, Yan, Liu, Xu, Wang and Zhang. This is an open-access article distributed under the terms of the Creative Commons Attribution License (CC BY). The use, distribution or reproduction in other forums is permitted, provided the original author(s) and the copyright owner(s) are credited and that the original publication in this journal is cited, in accordance with accepted academic practice. No use, distribution or reproduction is permitted which does not comply with these terms.
*Correspondence: Wei Wang, 2447@hrbmu.edu.cn; Tong Zhang, yingxiang939@163.com
†These authors have contributed equally to this work