- 1Department of Nephrology, West China Hospital, Sichuan University, Chengdu, China
- 2Division of Nephrology, Kidney Research Institute, West China Hospital, Sichuan University, Chengdu, China
- 3West China Biomedical Big Data Center, West China Hospital, Sichuan University, Chengdu, China
Obesity and Type 2 Diabetes (T2D) are two highly prevalent diseases that exhibit a complex interplay between them. Obesity serves as a primary risk factor for the development of T2D, and conversely, individuals with T2D often exhibit comorbid obesity. Renal dysfunction emerges as a critical consequence of the convergence of obesity and Type 2 Diabetes, contributing significantly to the overall burden of complications associated with these conditions. Recognizing the profound implications of renal dysfunction in individuals contending with both obesity and Type 2 Diabetes, interventions targeting weight loss have gained prominence as potential therapeutic avenues. Weight loss not only addresses the primary risk factor of obesity but also holds the promise of mitigating the progression of Type 2 Diabetes and its associated renal complications. This comprehensive review aims to explore the impact of weight loss on renal function in individuals contending with the convergence of obesity and T2D.
1 Association between obesity, type 2 diabetes, and chronic kidney disease
The global prevalence of obesity and T2D has reached alarming levels. As of 2021, approximately 529 million individuals were living with diabetes globally, with a standardized prevalence rate of 6.1% (5.8–6.5%) (1). Concurrently, obesity’s prevalence and severity continue to rise within the T2D and general populations. Startling statistics from the “National Diabetes Statistics Report (2017)” indicate that an overwhelming 87.5% of adult diabetic patients are either overweight or obese (2).
Obesity is unequivocally established as a critical risk factor for various diseases, including diabetes, hypertension, and cardiovascular disorders (3), all of which have significant adverse effects on kidney health, leading to conditions such as diabetic kidney disease (DKD) (4, 5) and end-stage renal disease (ESRD) (6). A study by Kamel et al. found that the risk of DKD among individuals with diabetes rises with increasing BMI, ranging from 0.91 for overweight individuals (BMI > 25 kg/m2) to 2.16 for severely obese individuals (BMI > 40 kg/m2) (7). These effects are mediated by several factors, including alterations in glomerular hemodynamics, heightened sympathetic nervous activity, hypertension, chronic inflammation, endothelial dysfunction, and so on (8). Intriguingly, even in the absence of diabetes, obese individuals may experience a heightened frequency and severity of proteinuria, with obesity independently contributing to the development of glomerulopathy. Obesity also negatively impacts key Chronic kidney disease(CKD)-associated risk factors, encompassing blood lipid levels, blood pressure, blood glucose control, and the promotion of insulin resistance (9). Notably, CKD tends to be more prevalent and progresses more rapidly in obese individuals with T2D compared to those with normal weight (2).
Given the intricate intertwining of obesity, T2D, and CKD, weight loss interventions have emerged as prospective strategies to enhance renal function and overall health in patients grappling with obesity and T2D (10). Extensive researches underscored the profound benefits of weight loss on kidney health (11–16). Rebecca O’Brien et al. discovered that among patients aged 19–79 with T2DM who underwent bariatric surgery, bariatric surgery was associated with a lower cumulative incidence of diabetic nephropathy(DN) at 5 years (17). Weight loss interventions, including lifestyle modifications, pharmaceutical interventions, and bariatric surgery, have the potential to enhance insulin sensitivity, regulate glucose levels, and control blood pressure (18, 19). Additionally, weight loss has demonstrated efficacy in reducing oxidative stress and inflammation, key factors in kidney injury development (20).
This review aims to provide a comprehensive analysis of the effects of weight loss interventions on renal function in patients with obesity and T2D. By examining the existing evidence, we seek to contribute valuable insights into the potential of weight loss strategies as renoprotective measures and to advocate for the adoption of personalized and multidisciplinary approaches in managing obesity, T2D, and CKD.
2 Obesity and diabetes: primary mechanisms leading to renal dysfunction
2.1 Diabetic kidney disease
Hyperglycemia-induced metabolic dysregulation is a widely recognized primary contributor to the onset and progression of DKD (21). The pathophysiology of DKD is multifaceted, encompassing hemodynamic (RAAS activation, endothelial dysfunction), metabolic (accumulation of advanced glycation end-products), pro-inflammatory (reactive oxygen species generation, tumor necrosis factor-α activation), and pro-fibrotic (stimulation of transforming growth factor-β signaling), but in reality these elements interact locally and systemically to form a complex and dynamic interplay resulting in functional and structural changes to the kidney (22). The Renin-Angiotensin-Aldosterone System (RAAS) plays a pivotal role in the pathogenesis of DKD, partly through its promotion of efferent arteriolar constriction and intraglomerular hypertension, as well as its activation of inflammatory and fibrotic pathways (23). Furthermore, factors such as Protein Kinase C-β, oxidative stress mediators, Advanced Glycation End-products (AGE), as well as various cytokines and chemokines, play significant roles in driving DKD progression in the context of hyperglycemic conditions (21, 22).
Obesity, which often co-occurs with diabetes, poses a substantial risk to renal function (24, 25). Accumulation of visceral fat triggers an adipocyte stress response and pro-inflammatory signaling, leading to metabolic dysregulation. Ectopic lipid deposition within the kidneys and the presence of intracellular lipid metabolites, such as ceramides, contribute to oxidative stress and podocyte insulin resistance, resulting in impairment of the glomerular barrier (26). Obesity also exacerbates conditions like hypertension, insulin resistance, Type 2 diabetes, and atherosclerosis, all of which contribute to renal injury and endothelial dysfunction (24, 26). Recent research indicates that obesity may exert an influence on the composition of gut microbiota, potentially impacting the development of diabetic kidney disease (27). Alterations in gut microbiota associated with obesity, including reduced microbial diversity and shifts in microbial species composition, have the potential to compromise gut barrier integrity and promote inflammation, ultimately affecting renal health (28).
2.2 Obesity-related glomerulopathy
Recent studies identify obesity as an independent risk factor for renal dysfunction, apart from diabetes and hypertension (29). ORG has emerged as a distinctive subtype of CKD, characterized by proteinuria, enlarged glomeruli, and a gradual decline in renal function (30). Obesity-induced alterations in adipose tissue impact renal health through diverse mechanisms. Adipose tissue stress affects adipokine secretion, altering the adiponectin-to-leptin ratio, associated with renal impairment (26). Obesity also impacts renal sodium handling and hypertension development, with elevated leptin levels stimulating the sympathetic nervous system and activating the renin-angiotensin system (26, 30). Mechanical effects of adipose tissue deposition, like perirenal and renal sinus fat accumulation, may also contribute to hypertension and renal injury (31). These changes may slow peritubular capillary blood flow, promote sodium retention, and lead to chronic renal function decline.
The systemic and local disruptions observed in ORG exhibit similarities to the pathogenesis of DKD. These two diseases interact with each other, leading to progressive renal damage.
3 Impact of weight loss on renal function in diabetic patients
Numerous high-quality clinical studies have provided substantial evidence supporting the role of weight loss in alleviating the progression of existing DKD and reducing the long-term incidence of diabetes-related renal complications. As previously discussed, O’Brien et al. conducted a retrospective observational cohort study of 2,205 type 2 diabetes patients who underwent metabolic surgery. A comparison with 11,059 matched non-surgical control subjects revealed a remarkable reduction in the incidence of kidney disease over a 6-year follow-up period. Specifically, metabolic surgery demonstrated a 6.4% incidence in surgical patients compared to 14% in non-surgical controls, with an adjusted hazard ratio of 0.45 [95% CI 0.29-0.71] (17). A matched cohort study by Madsen et al. showed that RYGB surgery led to a significant reduction in T2DM microvascular complications (HR 0.53 [95% CI 0.38–0.73]) and the incidence of type 2 DKD(incidence rate ratio 0.54 [95%CI 0.31–0.94]) (32). Additionally, Glucagon-Like Peptide-1(GLP-1), an incretin hormone, induces weight loss by reducing appetite, delaying gastric emptying, and optimizing insulin and glucagon secretion timing. Studies have demonstrated that Liraglutide not only leads to weight loss but also improves blood sugar control, enhances various cardiovascular markers, and reduces albuminuria. The LEADER RCT (33) confirmed that Liraglutide can slow the occurrence and progression of DKD and alleviate the recurrence of type 2 diabetes after metabolic surgery. Therefore, the use of Liraglutide after metabolic surgery may provide additional renal protective benefits (34). Some medications may potentiate weight loss but are not FDA approved for obesity. Sodium glucose co-transporter 2 inhibitors (SGLT2 inhibitors) also have significant weight-reducing effects. Although their primary purpose is to lower blood sugar by inhibiting renal sodium-glucose co-transporter-2, rather than being a weight loss drug, it has been observed that these agents can substantially improve body weight and exert a potent protective effect on kidney function. A comprehensive meta-analysis has underscored the multifaceted benefits of SGLT-2 inhibitors in patients with T2DM and CKD. These inhibitors not only lowered glycated hemoglobin (–0.29%, 95% CI –0.39 to –0.19) but also demonstrated reductions in blood pressure, body weight, and albuminuria. Furthermore, they mitigated the annual decline in eGFR slope (placebo-subtracted difference of 1.35 mL/1.73 m2/year, 95% CI 0.78-1.93) and decreased the risk of the composite renal outcome, encompassing doubling of serum creatinine, end-stage kidney disease, or renal death (HR 0.71, 95% CI 0.53-0.95) (35).
3.1 Weight loss interventions primarily alleviate renal damage by improving the following conditions
3.1.1 Diabetes emission
As mentioned earlier, elevated blood glucose levels are a crucial factor in the progression of diabetes patients to diabetic kidney disease. Numerous studies have demonstrated that weight loss interventions can significantly enhance insulin sensitivity, thereby improving blood sugar control and alleviating kidney damage (36). Evidence indicates that early weight loss surgical interventions in T2DM patients can lead to diabetes remission and substantially reduce the residual complications of the disease (36). A notable 10-year follow-up study revealed that 37.5% of patients who underwent surgical treatment sustained diabetes remission throughout the decade. This rate contrasts with 5.5% for medical therapy, 50.0% for biliopancreatic diversion (BPD), and 25.0% for Roux-en-Y gastric bypass (RYGB) (37). Mechanistic research suggests that weight loss primarily reduces intra-abdominal, intramyocellular, and intrahepatocellular lipids under hypocaloric conditions, rather than generalized body fat (38). This loss reverses crucial pathophysiological processes in diabetes, such as enhancing peripheral insulin sensitivity, improving cellular insulin signal transduction, boosting insulin secretion, and decreasing hepatic glucose production (38). The resulting improved insulin sensitivity optimizes glycemic control, thereby preserving glomerular integrity and function, reducing oxidative stress and inflammation, and preserving renal unit structure (39).
3.1.2 Reducing proteinuria and improving kidney structure
Weight loss interventions, particularly surgical procedures, demonstrate sustained remission of albuminuria post-surgery, with significant reductions in albuminuria observed across all baseline albumin-creatinine ratio tertiles, with remission occurring in 78% of patients and parallel studies in Zucker diabetic fatty rats revealed that weight loss and improvements in glycemia following RYGB surgery were accompanied by the normalization of glomerular tuft size, reduced podocyte expression of desmin, and preservation of podocyte foot process morphology (40). Notably, RYGB attenuated podocyte stress and dedifferentiation in the ZDF rat model, likely due to enhanced metabolic control, especially its potent glucose-lowering effect post-surgery (40). Similarly, researchers observed a reduction in urinary protein and the restoration of glomerular injury, attributed to improved glomerular filtration membrane ultrastructure and increased nephrin protein expression (41). Additionally, studies in obese type 2 diabetic patients revealed that calorie restriction also improved glomerular hyperfiltration and various cardiovascular risk factors, while also reducing serum angiotensin II levels, suggesting reduced RAS activity (14). Furthermore, Glucagon-Like Peptide-1 Receptor Agonists (GLP1-RA) like liraglutide can inhibit sodium-hydrogen exchanger 3 in proximal tubular cells, increasing natriuresis and diuresis (42).
3.1.3 Alleviating inflammatory status
Chronic low-grade inflammation is a hallmark of diabetic nephropathy and obesity (43). Researchers have uncovered that the reduction in body weight observed in RYGB rats is associated with a diminished fibrotic Transforming Growth Factor β (TGFβ) signal (44). TGFβ is recognized as a major driver of fibrosis and a key mediator of the hypertrophic and prosclerotic changes in diabetic nephropathy. Consequently, its downregulation appears to be a potential favorable effect in renal damage in patients with obesity and type 2 diabetes (45). Similarly, Bariatric surgery in mouse models activated Peroxisome Proliferator-Activated Receptor Alpha (PPARα), leading to the inhibition of Reactive Oxygen Species (ROS) generation. This action mitigates oxidative stress and reduces renal apoptosis, showcasing the protective effect of bariatric surgery on kidneys affected by diabetic nephropathy (46). Furthermore, Dietary intervention in mouse models attenuate progressive urinary protein excretion and renal inflammation, suggesting that adiposity drives renal inflammation in DKD (47–51). Reduced expression levels of inflammatory factors such as C-Reactive Protein (CRP) and C-C Motif Chemokine Ligand 2 (CCL2) enhance kidney function, decrease renal fibrosis, and improve inflammation control (52). Furthermore, GLP1-RA have been shown to stimulate pathways that reduce reactive oxygen species in the kidneys. Additionally, they contribute to lowering inflammation by decreasing cytokine production and immune cell infiltration (42). This evidence collectively highlights the intricate interplay between inflammation, weight loss interventions, and the potential protective effects on renal health in the context of diabetic nephropathy and obesity.
3.1.4 Gut microbiota changes
Alterations in gut microbiota following weight loss have been associated with improvements in energy balance, enhanced intestinal insulin release, and weight reduction. Recent meta-analyses have revealed significant alterations in gut microbiota and microbial metabolites following weight loss surgery. These changes are closely associated with improved glucose homeostasis, weight reduction, and modifications in gastrointestinal intake and exercise behaviors (53). A detailed investigation focused on diabetic patients undergoing metabolic surgery has unveiled intricate connections among serum metabolomics, gut microbiota composition, and hormonal profiles, particularly concerning improvements in diabetes and metabolic syndrome. Noteworthy correlations between specific gut bacteria, such as the Eubacterium eligens group, and metabolites like lacosamide glucuronide and UDP-L-arabinose were identified. Positive correlations were observed between Enterococcus and metabolites like glutamic acid and vindoline (54). Furthermore, research has shown that medical treatments also significantly alter intestinal microbiota, with observable changes like increased Proteobacteria and variable Bacteroidetes. These shifts correlate with changes in patient weight, and glucose metabolism (55). Additionally, the dysbiotic state induced by a high-fat diet was ameliorated by transitioning to a lower-fat, higher-fiber control diet, especially when combined with sleeve gastrectomy. This led to increased microbial diversity and shifting relative abundances (56). Moreover, the role of short-chain fatty acids (SCFAs), generated through bacterial fermentation of dietary fiber in the colon, has been crucial in protecting mice against the clinical and histologic manifestations of diabetic nephropathy by activating G Protein-Coupled Receptors GPR43 and GPR109 (57). Targeted dietary fiber supplementation to increase SCFA levels has also been found to contribute to better improvements in hemoglobin A1c levels, partly attributed to increased production of glucagon-like peptide-1 (GLP-1) (58). Hence, alterations in gut microbiota composition following weight loss may contribute to improved blood glucose and blood pressure control by enhancing colonic L cell secretion of GLP-1.
4 Impact of weight reduction on renal function in obese patients
A growing body of evidence suggests that weight reduction yields positive outcomes in non-diabetic patients with obesity, Several longitudinal studies, including a large cohort study (59), observed a substantial decrease in the risk of CKD in obese patients after weight reduction surgery. Notably, improvements in CKD risk categories are observed at 1 and 7 years after surgery, particularly in patients with moderate to high baseline CKD risk. It demonstrated similarities in the effects of weight reduction on renal function in both diabetic and non-diabetic obese patients. These effects encompass reduced adipose tissue, improved lipid metabolism, and ameliorated inflammatory states.
4.1 The benefit of adipose tissue reduction
Post-weight loss, there is a notable decrease in visceral adipose tissue and renal sinus fat. This reduction contributes to a favorable adipokine profile, diminished inflammatory cytokines, and lowered ROS production, potentially resulting in reduced RAAS activation levels (60). Obesity-induced metabolic syndrome disrupts lipid metabolism, leading to abnormal lipid profiles. Weight loss interventions have consistently proven effective in decreasing blood lipid levels and fat deposition, while increasing adiponectin levels, which play a key role in metabolic regulation (15, 61). Adiponectin plays a multifaceted role in metabolic control, promoting fatty acid oxidation through AMP-activated protein kinase (AMPK) activation. AMPK activation enhances insulin sensitivity in crucial insulin-target tissues like skeletal muscle and white adipose tissue, vital for blood glucose control. By stimulating fatty acid oxidation and ceramidase activity, adiponectin counteracts lipotoxicity and oxidative stress (62). Moreover, both intensive lifestyle interventions and bariatric surgery-induced weight loss have been found to reduce lipid accumulation and decrease local and systemic inflammatory microenvironments (60). Additionally, beyond bodyweight reduction, the improvement of the metabolic kidney milieu and restoration of endothelial function could also contribute to the renoprotective effects of regular exercise in chronic diabetic diseases (63). Furthermore, several longitudinal studies exploring the reduction of ectopic renal fat post-weight loss surgery have demonstrated a correlation with improved renal function. Renal sinus fat (RSF), a fat depot at the hilum of the kidney, has been studied for its association with hypertension, and its reduction post-weight loss surgery has been observed. In comparison to the lean control group, obese patients accumulated more RSF (2.3 [1.7-3.1] vs. 1.8 [1.4-2.5] cm2). Hypertensive patients, when compared to normotensive subjects, had a larger RSF depot (2.6 [2.0-3.3] vs. 2.0 [1.4-2.5] cm2), even after considering BMI. In combined data, RSF showed a negative correlation with estimated glomerular filtration rate (eGFR) but had no association with systolic or diastolic blood pressure. After weight loss surgery, RSF decreased along with other obesity markers. The magnitude of RSF reduction was greater in patients who experienced hypertension relief compared to those who still had hypertension (-0.68 [-0.74, -0.44] vs. -0.28 [-0.59, 0] cm2, p = 0.009). The accumulation of RSF appears to be linked to the pathogenesis of obesity-related hypertension, and post-surgery, a significant decrease in RSF was observed, correlating with relief from hypertension (31). Similarly, in a study involving dietary-induced weight loss over an 18-month period, a correlation was observed between RSF and baseline eGFR as well as microalbuminuria. After 8 months, RSF decreased by 6.18%, and this reduction was associated with overall body weight loss. The decrease in RSF was also linked to improvements in lipid profiles and blood glucose control (37).
4.2 Weight reduction and glomerular filtration rate
Studies, including one by Lin et al., demonstrate that bariatric surgery was associated with eGFR preservation in all obese patients and, particularly, in those with moderate-to-high CKD risks. The study revealed a significant negative correlation was evident between an increased eGFR and a reduced BMI (Spearman’s correlation -0.229, P < 0.001),and the bariatric surgery group had a significantly lower risk of an eGFR decline ≥25% at 12 months [adjusted HR (aHR) 0.47, P = 0.03). After BS, obese patients with hypertension or albuminuria had significantly lower risks of eGFR declines ≥25% (aHR 0.37, P = 0.02 and aHR 0.13, P = 0.0018, respectively) (64). In a prospective cohort study, researchers observed analogous trends. Twenty-five individuals, comprising both obese and non-diabetic subjects, exhibited considerable stability in unadjusted mean glomerular filtration rate (mGFR) and a noteworthy improvement in adjusted mGFR (65). Caloric restriction-based dietary interventions also exhibit positive effects. Individuals with eGFR < 120 mL experience an overall increase in GFR with weight loss, while those with hyperfiltration witness a substantial decline in GFR, indicating a favorable reduction in obesity-associated glomerular hyperfiltration (66–68). Moreover, utilizing advanced imaging techniques, researchers provided compelling evidence that obesity induces structural, metabolic, and hemodynamic changes in the kidneys. Obese subjects showed higher renal volume but lower radiodensity, suggestive of potential water and/or lipid accumulation. Cardiac output and eGFR were increased by approximately 25% in obese individuals. Total renal blood flow was higher in the obese, and FFA uptake was about 50% higher due to elevated circulating FFA levels. Importantly, following weight loss (26 ± 8 kg), these changes in eGFR, total renal blood flow, kidney volume, FFA uptake, and renal density were partially reversed, thereby mitigating the risk of obesity-induced progression of chronic kidney disease (69).
In conclusion, weight reduction offers multifaceted benefits for both diabetes and obesity, including improved metabolic disturbances, reduced adipose tissue deposition, alleviated chronic inflammation, and restored renal structure and function. For patients with obesity and concurrent diabetes, weight reduction interventions are beneficial for renal health, potentially delaying or preventing renal function impairment (70).
In Figure 1, we elaborate on the specific mechanisms illustrating the impact of weight loss intervention on renal function in obese and diabetic patients.
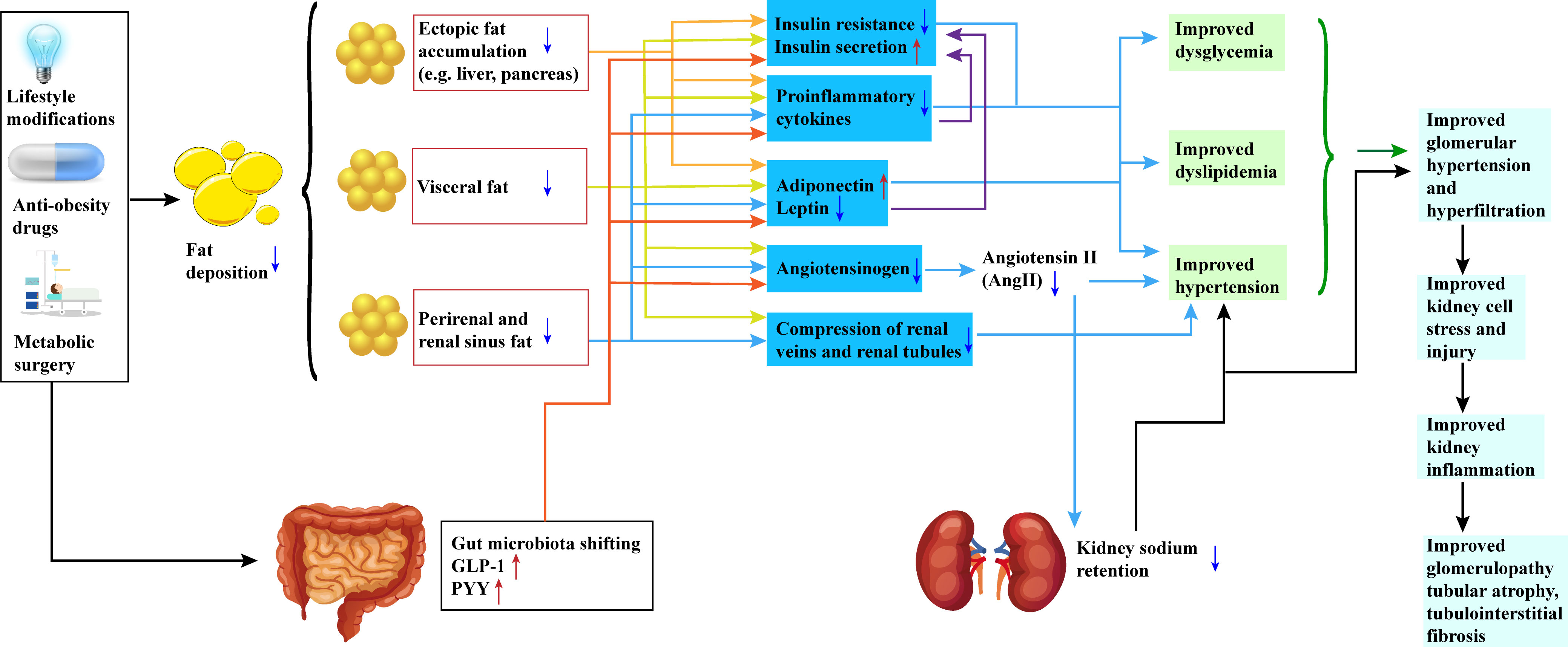
Figure 1 Potential Mechanisms of Weight Loss Intervention in Preserving Renal Function in Obesity and Diabetic Patients. All three approaches—lifestyle modifications, anti-obesity medications, and metabolic surgeries—result in a reduction in patient body weight. This reduction is characterized by a decrease in fat deposition, encompassing visceral fat, renal sinus fat, and ectopic adipose tissue. The reduction of fat in these specific regions promotes the restoration of insulin sensitivity, elevation of peripheral insulin levels, suppression of pro-inflammatory factors, and modulation of adipokine release. Simultaneously, alterations in the release patterns of adipokines are observed, marked by an increase in adiponectin levels and a decrease in leptin levels. Furthermore, the renin-angiotensin-aldosterone system (RAS) is inhibited. Additionally, shifts in gut microbiota composition and increased secretion of hormones such as GLP-1 and PYY contribute to these effects. Collectively, these changes culminate in enhanced regulation of blood glucose, blood lipids, and blood pressure. The inhibition of the RAS system also leads to reduced urinary sodium retention, providing a safeguard for the kidneys. These interventions exert a favorable impact on renal function, including the amelioration of glomerular hyperfiltration, preservation of renal cell integrity, and reduction in renal inflammation. Ultimately, these mechanisms translate into enhancements in kidney structure, including the attenuation of glomerular and renal tubular atrophy, as well as a reduction in interstitial fibrosis. GLP-1(Glucagon-Like Peptide-1); PYY(Peptide YY). Red arrows represent an increase, and blue arrows represent a decrease.
5 Perspectives and conclusion
Obesity combined with diabetes poses a significant health challenge globally, with the associated renal issues posing a serious threat to patient health. Weight loss interventions show promising potential in improving renal function, and current research has made some encouraging progress. However, future research directions and key issues still require further exploration to enhance our understanding of this crucial area and ultimately improve patients’ quality of life. Firstly, mechanistic studies will continue to be a focal point. Understanding how weight loss interventions impact renal function through biochemical pathways, and delving into the molecular and cellular mechanisms between obesity, diabetes, and renal diseases, is crucial for developing more targeted intervention strategies. These studies are expected to unveil new therapeutic targets and opportunities for drug development. Secondly, long-term effects and safety assessments will be a critical area for future research. Over time, the long-term impact of weight loss interventions on renal function needs more attention, especially regarding their role in the progression of renal diseases. Personalized and comprehensive interventions represent another important future direction. Different patients have diverse genetic backgrounds, lifestyles, and disease stages, making personalized intervention plans crucial. Integrating drug therapy, lifestyle adjustments, even metabolic surgery to optimize renal function improvement will be a key goal of future research and clinical practice.
In conclusion, the impact of weight loss interventions on renal function in patients with obesity and diabetes is a research area of significant clinical importance. With further research and technological advancements, we can not only better comprehend its mechanisms but also formulate more effective treatment strategies, ultimately offering diabetic and obesity patients improved health and quality of life.
Author contributions
XG: Conceptualization, Data curation, Methodology, Writing – original draft. XZ: Conceptualization, Methodology, Supervision, Validation, Writing – review & editing. PF: Conceptualization, Methodology, Supervision, Validation, Writing – review & editing.
Funding
The author(s) declare that no financial support was received for the research, authorship, and/or publication of this article.
Conflict of interest
The authors declare that the research was conducted in the absence of any commercial or financial relationships that could be construed as a potential conflict of interest.
Publisher’s note
All claims expressed in this article are solely those of the authors and do not necessarily represent those of their affiliated organizations, or those of the publisher, the editors and the reviewers. Any product that may be evaluated in this article, or claim that may be made by its manufacturer, is not guaranteed or endorsed by the publisher.
References
1. GBD 2021 Diabetes Collaborators. Global, regional, and national burden of diabetes from 1990 to 2021, with projections of prevalence to 2050: a systematic analysis for the Global Burden of Disease Study 2021. Lancet (2023) 402(10397):203–34. doi: 10.1016/S0140-6736(23)01301-6
2. Control and Prevention, C. National Diabetes Statistics Report, 2017-Estimates of Diabetes and Its Burden in the United States Background. (2017). Available at: https://policycommons.net/artifacts/3490914/national-diabetes-statistics-report-2017/4291734.
3. Afshin A, Forouzanfar MH, Reitsma MB, Sur P, Estep K, Lee A, et al. Health effects of overweight and obesity in 195 countries over 25 years. N Engl J Med (2017) 377(1):13–27. doi: 10.1056/NEJMoa1614362
4. Wu Z, Yu S, Kang X, Liu Y, Xu Z, Li Z, et al. Association of visceral adiposity index with incident nephropathy and retinopathy: a cohort study in the diabetic population. Cardiovasc Diabetol (2022) 21(1):32. doi: 10.1186/s12933-022-01464-1
5. Ou Y-L, Lee M-Y, Lin IT, Wen W-L, Hsu W-H, Chen S-C. Obesity-related indices are associated with albuminuria and advanced kidney disease in type 2 diabetes mellitus. Ren Fail (2021) 43(1):1250–8. doi: 10.1080/0886022X.2021.1969247
6. Hsu C-Y, McCulloch CE, Iribarren C, Darbinian J, Go AS. Body mass index and risk for end-stage renal disease. Ann Intern Med (2006) 144(1):21–8. doi: 10.7326/0003-4819-144-1-200601030-00006
7. Mohammedi K, Chalmers J, Herrington W, Li Q, Mancia G, Marre M, et al. Associations between body mass index and the risk of renal events in patients with type 2 diabetes. Nutr Diabetes (2018) 8(1):7. doi: 10.1038/s41387-017-0012-y
8. Kreiner FF, Schytz PA, Heerspink HJL, von Scholten BJ, Idorn T. Obesity-related kidney disease: current understanding and future perspectives. Biomedicines (2023) 11(9):2498. doi: 10.3390/biomedicines11092498
9. Maric-Bilkan C. Obesity and diabetic kidney disease. Med Clin North Am (2013) 97(1):59–74. doi: 10.1016/j.mcna.2012.10.010
10. Jacob P, McCafferty K. Assessment and management of chronic kidney disease in people living with obesity. Clin Med (Lond) (2023) 23(4):353–6. doi: 10.7861/clinmed.2023-0195
11. Bjornstad P, Hughan K, Kelsey MM, Shah AS, Lynch J, Nehus E, et al. Effect of surgical versus medical therapy on diabetic kidney disease over 5 years in severely obese adolescents with type 2 diabetes. Diabetes Care (2020) 43(1):187–95. doi: 10.2337/dc19-0708
12. Chao AT, Chee Fang S, Lam BC, Cheng AK, Low SK, Su Chi L. Effect of bariatric surgery on diabetic nephropathy in obese type 2 diabetes patients in a retrospective 2-year study: A local pilot. Diabetes Vasc Dis Res (2018) 15(2):139–44. doi: 10.1177/1479164117742315
13. Dong L, Li J, Lian Y, Tang ZX, Zen Z, Yu P, et al. Long-term intensive lifestyle intervention promotes improvement of stage III diabetic nephropathy. Med Sci Monit (2019) 25:3061–8. doi: 10.12659/msm.913512
14. Ruggenenti P, Cortinovis M, Trillini M, Parvanova A, Abbate M, Satriano C, et al. Long-term kidney and systemic effects of calorie restriction in overweight or obese type 2 diabetic patients (C.Re.S.O. 2 randomized controlled trial). Diabetes Res Clin Pract (2022) 185:109804. doi: 10.1016/j.diabres.2022.109804
15. Ruggenenti P, Abbate M, Ruggiero B, Rota S, Trillini M, Aparicio C, et al. Renal and systemic effects of calorie restriction in patients with type 2 diabetes with abdominal obesity: A randomized controlled trial. Diabetes (2017) 66(1):75–86. doi: 10.2337/db16-0607
16. Cohen RV, Pereira TV, Aboud CM, Zanata Petry TB, Lopes Correa JL, Schiavon CA, et al. Gastric bypass versus best medical treatment for diabetic kidney disease: 5 years follow up of a single-centre open label randomised controlled trial. E Clin Med (2022) 53:101725. doi: 10.1016/j.eclinm.2022.101725
17. O’Brien R, Johnson E, Haneuse S, Coleman KJ, O’Connor PJ, Fisher DP, et al. Microvascular outcomes in patients with diabetes after bariatric surgery versus usual care: A matched cohort study. Ann Intern Med (2018) 169(5):300–10. doi: 10.7326/m17-2383
18. Nair M, le Roux CW, Docherty NG. Mechanisms underpinning remission of albuminuria following bariatric surgery. Curr Opin Endocrinol Diabetes Obes (2016) 23(5):366–72. doi: 10.1097/med.0000000000000279
19. Martin WP, White J, López-Hernández FJ, Docherty NG, le Roux CW. Metabolic surgery to treat obesity in diabetic kidney disease, chronic kidney disease, and end-stage kidney disease; what are the unanswered questions? Front Endocrinol (Lausanne) (2020) 11:289. doi: 10.3389/fendo.2020.00289
20. Serra A, Granada ML, Romero R, Bayés B, Cantón A, Bonet J, et al. The effect of bariatric surgery on adipocytokines, renal parameters and other cardiovascular risk factors in severe and very severe obesity: 1-year follow-up. Clin Nutr (2006) 25(3):400–8. doi: 10.1016/j.clnu.2005.11.014
21. Alicic RZ, Rooney MT, Tuttle KR. Diabetic kidney disease: challenges, progress, and possibilities. Clin J Am Soc Nephrol (2017) 12(12):2032–45. doi: 10.2215/CJN.11491116
22. Lytvyn Y, Bjornstad P, van Raalte DH, Heerspink HL, Cherney DZI. The new biology of diabetic kidney disease-mechanisms and therapeutic implications. Endocr Rev (2020) 41(2):202–31. doi: 10.1210/endrev/bnz010
23. Warren AM, Knudsen ST, Cooper ME. Diabetic nephropathy: an insight into molecular mechanisms and emerging therapies. Expert Opin Ther Targets (2019) 23(7):579–91. doi: 10.1080/14728222.2019.1624721
24. Bhupathiraju SN, Hu FB. Epidemiology of obesity and diabetes and their cardiovascular complications. Circ Res (2016) 118(11):1723–35. doi: 10.1161/CIRCRESAHA.115.306825
25. Todd JN, Dahlström EH, Salem RM, Sandholm N, Forsblom C, McKnight AJ, et al. Genetic evidence for a causal role of obesity in diabetic kidney disease. Diabetes (2015) 64(12):4238–46. doi: 10.2337/db15-0254
26. Chait A, den Hartigh LJ. Adipose tissue distribution, inflammation and its metabolic consequences, including diabetes and cardiovascular disease. Front Cardiovasc Med (2020) 7:22. doi: 10.3389/fcvm.2020.00022
27. Zaky A, Glastras SJ, Wong MYW, Pollock CA, Saad S. The role of the gut microbiome in diabetes and obesity-related kidney disease. Int J Mol Sci (2021) 22(17):9641. doi: 10.3390/ijms22179641
28. Fang Q, Liu N, Zheng B, Guo F, Zeng X, Huang X, et al. Roles of gut microbial metabolites in diabetic kidney disease. Front Endocrinol (Lausanne) (2021) 12:636175. doi: 10.3389/fendo.2021.636175
29. Kjaergaard AD, Teumer A, Witte DR, Stanzick K-J, Winkler TW, Burgess S, et al. Obesity and kidney function: A two-sample Mendelian randomization study. Clin Chem (2022) 68(3):461–72. doi: 10.1093/clinchem/hvab249
30. Xu T, Sheng Z, Yao L. Obesity-related glomerulopathy: pathogenesis, pathologic, clinical characteristics and treatment. Front Med (2017) 11(3):340–8. doi: 10.1007/s11684-017-0570-3
31. Moritz E, Dadson P, Saukko E, Honka M-J, Koskensalo K, Seppälä K, et al. Renal sinus fat is expanded in patients with obesity and/or hypertension and reduced by bariatric surgery associated with hypertension remission. Metabolites (2022) 12(7):617. doi: 10.3390/metabo12070617
32. Madsen LR, Baggesen LM, Richelsen B, Thomsen RW. Effect of Roux-en-Y gastric bypass surgery on diabetes remission and complications in individuals with type 2 diabetes: a Danish population-based matched cohort study. Diabetologia (2019) 62(4):611–20. doi: 10.1007/s00125-019-4816-2
33. Mann JFE, Ørsted DD, Brown-Frandsen K, Marso SP, Poulter NR, Rasmussen S, et al. Liraglutide and renal outcomes in type 2 diabetes. N Engl J Med (2017) 377(9):839–48. doi: 10.1056/NEJMoa1616011
34. Parvathareddy VP, Ella KM, Shah M, Navaneethan SD. Treatment options for managing obesity in chronic kidney disease. Curr Opin Nephrol Hypertens (2021) 30(5):516–23. doi: 10.1097/MNH.0000000000000727
35. Toyama T, Neuen BL, Jun M, Ohkuma T, Neal B, Jardine MJ, et al. Effect of SGLT2 inhibitors on cardiovascular, renal and safety outcomes in patients with type 2 diabetes mellitus and chronic kidney disease: A systematic review and meta-analysis. Diabetes Obes Metab (2019) 21(5):1237–50. doi: 10.1111/dom.13648
36. Lean MEJ, Leslie WS, Barnes AC, Brosnahan N, Thom G, McCombie L, et al. Durability of a primary care-led weight-management intervention for remission of type 2 diabetes: 2-year results of the DiRECT open-label, cluster-randomised trial. Lancet Diabetes Endocrinol (2019) 7(5):344–55. doi: 10.1016/S2213-8587(19)30068-3
37. Mingrone G, Panunzi S, De Gaetano A, Guidone C, Iaconelli A, Capristo E, et al. Metabolic surgery versus conventional medical therapy in patients with type 2 diabetes: 10-year follow-up of an open-label, single-centre, randomised controlled trial. Lancet (2021) 397(10271):293–304. doi: 10.1016/S0140-6736(20)32649-0
38. Grams J, Garvey WT. Weight loss and the prevention and treatment of type 2 diabetes using lifestyle therapy, pharmacotherapy, and bariatric surgery: mechanisms of action. Curr Obes Rep (2015) 4(2):287–302. doi: 10.1007/s13679-015-0155-x
39. Vázquez LA, Romera I, Rubio-de Santos M, Escalada J. Glycaemic control and weight reduction: a narrative review of new therapies for type 2 diabetes. Diabetes Ther (2023) 14(11):1771–84. doi: 10.1007/s13300-023-01467-5
40. Canney AL, Cohen RV, Elliott JA, MA C, Martin WP, Docherty NG, et al. Improvements in diabetic albuminuria and podocyte differentiation following Roux-en-Y gastric bypass surgery. Diabetes Vasc Dis Res (2020) 17(1):1479164119879039. doi: 10.1177/1479164119879039
41. Xiong Y, Zhu W, Xu Q, Ruze R, Yan Z, Li J, et al. Sleeve gastrectomy attenuates diabetic nephropathy by upregulating nephrin expressions in diabetic obese rats. Obes Surg (2020) 30(8):2893–904. doi: 10.1007/s11695-020-04611-3
42. Michos ED, Bakris GL, Rodbard HW, Tuttle KR. Glucagon-like peptide-1 receptor agonists in diabetic kidney disease: A review of their kidney and heart protection. Am J Prev Cardiol (2023) 14:100502. doi: 10.1016/j.ajpc.2023.100502
43. Sabatino A, Regolisti G, Cosola C, Gesualdo L, Fiaccadori E. Intestinal microbiota in type 2 diabetes and chronic kidney disease. Curr Diabetes Rep (2017) 17(3):16. doi: 10.1007/s11892-017-0841-z
44. Vangoitsenhoven R, Mulya A, Mosinski JD, Brethauer SA, Schauer PR, Kirwan JP, et al. Effects of gastric bypass surgery on expression of glucose transporters and fibrotic biomarkers in kidney of diabetic fatty rats. Surg For Obes Relat Dis (2020) 16(9):1242–8. doi: 10.1016/j.soard.2020.04.017
45. Nair M, Martin WP, Zhernovkov V, Elliott JA, Fearon N, Eckhardt H, et al. Characterization of the renal cortical transcriptome following Roux-en-Y gastric bypass surgery in experimental diabetic kidney disease. BMJ Open Diabetes Res Care (2020) 8(1):e001113. doi: 10.1136/bmjdrc-2019-001113
46. Jiang HW, Zhou Y, Zhou PY, Zhang TY, Hu JY, Bai XT. Protective effects of bariatric surgery on kidney functions by inhibiting oxidative stress responses through activating PPARα in rats with diabetes. Front Physiol (2021) 12:662666. doi: 10.3389/fphys.2021.662666
47. Neff KJ, Elliott JA, Corteville C, Abegg K, Boza C, Lutz TA, et al. Effect of Roux-en-Y gastric bypass and diet-induced weight loss on diabetic kidney disease in the Zucker diabetic fatty rat. Surg For Obes Relat Dis (2017) 13(1):21–7. doi: 10.1016/j.soard.2016.08.026
48. Illán-Gómez F, Gonzálvez-Ortega M, Orea-Soler I, Alcaraz-Tafalla MS, Aragón-Alonso A, Pascual-Díaz M, et al. Obesity and inflammation: change in adiponectin, C-reactive protein, tumour necrosis factor-alpha and interleukin-6 after bariatric surgery. Obes Surg (2012) 22(6):950–5. doi: 10.1007/s11695-012-0643-y
49. Fenske WK, Dubb S, Bueter M, Seyfried F, Patel K, Tam FWK, et al. Effect of bariatric surgery-induced weight loss on renal and systemic inflammation and blood pressure: a 12-month prospective study. Surg For Obes Relat Dis (2013) 9(4):559–68. doi: 10.1016/j.soard.2012.03.009
50. You Y-K, Wu W-F, Huang X-R, Li H-D, Ren Y-P, Zeng J-C, et al. Deletion of Smad3 protects against C-reactive protein-induced renal fibrosis and inflammation in obstructive nephropathy. Int J Biol Sci (2021) 17(14):3911–22. doi: 10.7150/ijbs.62929
51. Tesch GH. MCP-1/CCL2: a new diagnostic marker and therapeutic target for progressive renal injury in diabetic nephropathy. Am J Physiol Renal Physiol (2008) 294(4):F697–701. doi: 10.1152/ajprenal.00016.2008
52. Ding H, Zhang Y, Ma X, Zhang Z, Xu Q, Liu C, et al. Bariatric surgery for diabetic comorbidities: A focus on hepatic, cardiac and renal fibrosis. Front Pharmacol (2022) 13:1016635. doi: 10.3389/fphar.2022.1016635
53. Coimbra VOR, Crovesy L, Ribeiro-Alves M, Faller ALK, Mattos F, Rosado EL. Gut microbiota profile in adults undergoing bariatric surgery: a systematic review. Nutrients (2022) 14(23):4979. doi: 10.3390/nu14234979
54. Huang H-H, Lin T-L, Lee W-J, Chen S-C, Lai W-F, Lu C-C, et al. Impact of metabolic surgery on gut microbiota and sera metabolomic patterns among patients with diabetes. Int J Mol Sci (2022) 23(14):7797. doi: 10.3390/ijms23147797
55. Medina DA, Pedreros JP, Turiel D, Quezada N, Pimentel F, Escalona A, et al. Distinct patterns in the gut microbiota after surgical or medical therapy in obese patients. PeerJ (2017) 5:e3443. doi: 10.7717/peerj.3443
56. Rossell J, Brindefalk B, Baena-Fustegueras JA, Peinado-Onsurbe J, Udekwu KI. Diet change affects intestinal microbiota restoration and improves vertical sleeve gastrectomy outcome in diet-induced obese rats. Eur J Nutr (2020) 59(8):3555–64. doi: 10.1007/s00394-020-02190-8
57. Li YJ, Chen X, Kwan TK, Loh YW, Singer J, Liu Y, et al. Dietary Fiber Protects against Diabetic Nephropathy through Short-Chain Fatty Acid-Mediated Activation of G Protein-Coupled Receptors GPR43 and GPR109A. J Am Soc Nephrol (2020) 31(6):1267–81. doi: 10.1681/ASN.2019101029
58. Zhao L, Zhang F, Ding X, Wu G, Lam YY, Wang X, et al. Gut bacteria selectively promoted by dietary fibers alleviate type 2 diabetes. Science (2018) 359(6380):1151–6. doi: 10.1126/science.aao5774
59. Friedman AN, Wahed AS, Wang J, Courcoulas AP, Dakin G, Hinojosa MW, et al. Effect of bariatric surgery on CKD risk. J Am Soc Nephrol (2018) 29(4):1289–300. doi: 10.1681/ASN.2017060707
60. Opazo-Ríos L, Mas S, Marín-Royo G, Mezzano S, Gómez-Guerrero C, Moreno JA, et al. Lipotoxicity and diabetic nephropathy: novel mechanistic insights and therapeutic opportunities. Int J Mol Sci (2020) 21(7):2632. doi: 10.3390/ijms21072632
61. Krebs JD, Elley CR, Parry-Strong A, Lunt H, Drury PL, Bell DA, et al. The Diabetes Excess Weight Loss (DEWL) Trial: A randomised controlled trial of high-protein versus high-carbohydrate diets over 2 years in type 2 diabetes. Diabetologia (2012) 55(4):905–14. doi: 10.1007/s00125-012-2461-0
62. Kim Y, Park CW. Mechanisms of adiponectin action: implication of adiponectin receptor agonism in diabetic kidney disease. Int J Mol Sci (2019) 20(7):1782. doi: 10.3390/ijms20071782
63. Van Craenenbroeck AH, Van Craenenbroeck EM, Van Ackeren K, Vrints CJ, Conraads VM, Verpooten GA, et al. Effect of moderate aerobic exercise training on endothelial function and arterial stiffness in CKD stages 3-4: A randomized controlled trial. Am J Kidney Dis (2015) 66(2):285–96. doi: 10.1053/j.ajkd.2015.03.015
64. Lin Y-C, Lai Y-J, Lin Y-C, Peng C-C, Chen K-C, Chuang M-T, et al. Effect of weight loss on the estimated glomerular filtration rates of obese patients at risk of chronic kidney disease: the RIGOR-TMU study. J Cachexia Sarcopenia Muscle (2019) 10(4):756–66. doi: 10.1002/jcsm.12423
65. Solini A, Seghieri M, Santini E, Giannini L, Biancalana E, Taddei S, et al. Renal resistive index predicts post-bariatric surgery renal outcome in nondiabetic individuals with severe obesity. Obes (Silver Spring Md) (2019) 27(1):68–74. doi: 10.1002/oby.22355
66. Brinkworth GD, Buckley JD, Noakes M, Clifton PM. Renal function following long-term weight loss in individuals with abdominal obesity on a very-low-carbohydrate diet vs high-carbohydrate diet. J Am Diet Assoc (2010) 110(4):633–8. doi: 10.1016/j.jada.2009.12.016
67. Jesudason DR, Pedersen E, Clifton PM. Weight-loss diets in people with type 2 diabetes and renal disease: A randomized controlled trial of the effect of different dietary protein amounts. Am J Clin Nutr (2013) 98(2):494–501. doi: 10.3945/ajcn.113.060889
68. Chagnac A, Weinstein T, Herman M, Hirsh J, Gafter U, Ori Y. The effects of weight loss on renal function in patients with severe obesity. J Am Soc Nephrol (2003) 14(6):1480–6. doi: 10.1097/01.ASN.0000068462.38661.89
69. Rebelos E, Dadson P, Oikonen V, Iida H, Hannukainen JC, Iozzo P, et al. Renal hemodynamics and fatty acid uptake: effects of obesity and weight loss. Am J Physiol Endocrinol Metab (2019) 317(5):E871–8. doi: 10.1152/ajpendo.00135.2019
Keywords: weight loss, obesity, type 2 diabetes, renal function, chronic kidney disease
Citation: Gong X, Zeng X and Fu P (2024) The impact of weight loss on renal function in individuals with obesity and type 2 diabetes: a comprehensive review. Front. Endocrinol. 15:1320627. doi: 10.3389/fendo.2024.1320627
Received: 12 October 2023; Accepted: 15 January 2024;
Published: 31 January 2024.
Edited by:
Renato Branco, Purdue University Indianapolis, United StatesReviewed by:
Yasutaka Takeda, Kanazawa Medical University, JapanXuezhong Gong, Shanghai Municipal Hospital of Traditional Chinese Medicine, China
Copyright © 2024 Gong, Zeng and Fu. This is an open-access article distributed under the terms of the Creative Commons Attribution License (CC BY). The use, distribution or reproduction in other forums is permitted, provided the original author(s) and the copyright owner(s) are credited and that the original publication in this journal is cited, in accordance with accepted academic practice. No use, distribution or reproduction is permitted which does not comply with these terms.
*Correspondence: Ping Fu, fupinghx@scu.edu.cn