- 1Maternal-Fetal Medicine Institute, Department of Obstetrics and Gynaecology, Shenzhen Baoan Women’s and Children’s Hospital, Shenzhen University, Shenzhen, China
- 2Fertility Preservation Research Center, Department of Obstetrics and Gynaecology, The Chinese University of Hong Kong, Hong Kong, Hong Kong SAR, China
Successful pregnancy requires the tolerance of the maternal immune system for the semi-allogeneic embryo, as well as a synchrony between the receptive endometrium and the competent embryo. The annexin family belongs to calcium-regulated phospholipid-binding protein, which functions as a membrane skeleton to stabilize the lipid bilayer and participate in various biological processes in humans. There is an abundance of the annexin family at the maternal–fetal interface, and it exerts a crucial role in embryo implantation and the subsequent development of the placenta. Altered expression of the annexin family and dysfunction of annexin proteins or polymorphisms of the ANXA gene are involved in a range of pregnancy complications. In this review, we summarize the current knowledge of the annexin A protein family at the maternal–fetal interface and its association with female reproductive disorders, suggesting the use of ANXA as the potential therapeutic target in the clinical diagnosis and treatment of pregnancy complications.
1 Introduction
The maternal–fetal interface is a crucial site for the establishment and maintenance of normal pregnancy, where the trophoblast cells, decidual cells, and the immune microenvironment exist and interact with each other. Dysregulation of the functions of the trophoblast and disturbance of the maternal–fetal immune tolerance can lead to reproductive disorders, including infertility, spontaneous miscarriage, preeclampsia, and intrauterine growth restriction.
Annexins, a well-known multigene family, are secreted proteins in the cytoplasm attaching to the phospholipid membrane and highly conservative Ca2+-dependent membrane-binding proteins that participate in a variety of physiological and pathological processes in humans (1–3). The role of the annexin family participating in several human pathologies such as tumorigenesis, obesity, and atherosclerosis has been extensively reviewed in a bunch of excellent papers (4–7). The expression of the annexin family was identified at the maternal–fetal interface, involving both the trophoblasts and decidual cells, but its role in successful pregnancy is not fully clear yet. Herein, we reviewed the current knowledge of the expression and possible functions of the annexin family at the maternal–fetal interface and its relationship with female reproductive diseases.
2 The annexin family
Annexin is an evolutionally conserved Ca2+-regulated phospholipid-binding protein superfamily, which is named for its ability to “annex,” implying that it integrates membranes, and is widely distributed in eukaryote cells (8). To date, more than 1,000 members of the annexin superfamily have been identified and classified into five groups (groups A–E) according to different species, with ANXA12 being a pseudogene. The annexin A group comprises 12 different members (AnxA1–AnxA11, AnxA13) in human organs. Annexins participate in extracellular activities including proinflammatory and profibrotic responses, as well as subcellular functions consisting of membrane repair, cytoskeletal changes, intracellular organelle transportation, and signal transduction. Over the past decades, a great number of studies on annexins have been performed for their involvement in a variety of physiological and pathological processes in humans, among which AnxA1 and AnxA5 are in the process of development as potential therapeutic targets for atherosclerosis, fibrosis, rheumatoid arthritis, and cardiovascular disease currently (9–12).
2.1 Molecular structure of the annexin family
The 12 human annexin genes are dispensed throughout the genome on chromosomes 1, 2, 4, 5, 8, 9, 10, and 15, with the genomic size ranging from 15 kb (ANXA9) to 96 kb (ANXA10). The expression levels of the annexin gene are very wide and present different abundance levels in specific tissues (13).
Structurally, the protein of the annexin A family consists of two domains: the conserved C-terminal and the variable N-terminal (14). All annexins share the conserved C-terminal domain, which is a highly helical and tightly folded protein center consisting of four (or eight in AnxA6) annexin repeat units; thus, a characteristic “type 2” calcium-binding site is formed through hydrophobic interactions and ensures structural stabilization (15). In contrast, unlike the core domain, the N-terminal (also known as the tail domain) is a variable domain containing sites for post-translational modification and is unique in each member, enabling these members to bind to various ligands and other proteins; thus, the members present a diversity of locations and functions (16) (Figure 1A).
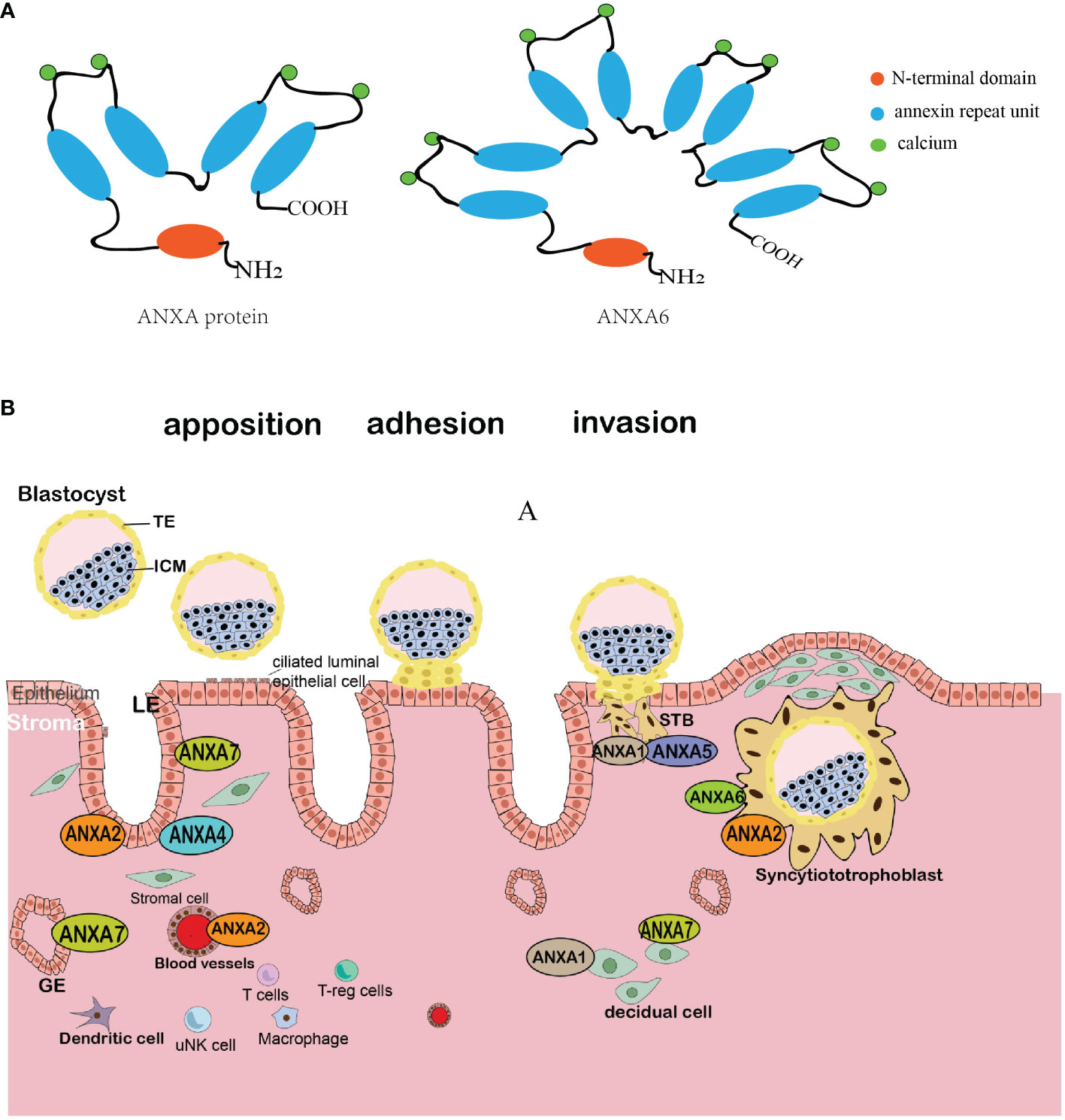
Figure 1 Schematic diagram of the annexin molecular structure and comprehensive schematic illustration of the location of the annexin family at the maternal–fetal interface. (A) The ANXA protein structure comprises two main regions: a highly conserved C-terminal core with four repetitive sequences (or eight in ANXA6), each harboring a calcium-binding motif, and a variable N-terminal with differing amino acid arrangements and lengths across each ANXA variant. (B) The blastocyst implants to the maternal endometrium following the sequential stages of apposition, adhesion, and invasion. Members of the annexin family show different expression patterns in diverse cell types as the blastocyst interacts with the endometrium. TE, trophectoderm; ICM, inner cell mass; LE, luminal epithelium; GE, glandular and luminal endometrial epithelium; STBs, syncytial trophoblasts; uNK, decidual natural killer cell.
In most circumstances, annexins bind reversibly to cell membranes via the featured calcium-binding site. Another common scenario, as mentioned above, the unique N-terminal domain, mediates the interaction between annexins with other specific intracellular protein partners, such as the S100 family cytoplasmic proteins. The S100 protein is a dimeric EF-hand calcium-binding protein and forms a protein–protein interaction with the N-terminal domain of AnxA2 through a highly specific binding site, thus collaborating to regulate multiple cellular functions, leading to the AnxA2/S100A10 complex possessing biological activities (17–19).
2.2 Functions of annexins
Annexins are involved in several cellular membrane-related processes, including cellular structural organization, growth regulation, and vesicle transportation. To date, the relationship between annexins and various cellular processes including vesicle trafficking, membrane repair, cell proliferation and apoptosis, cell migration, anti-inflammation, promotion of angiogenesis, and anticoagulation has been reported in in-vitro gain-of-function or loss-of-function experiments, and these processes are independent of calcium signaling. On a pathologic front, accumulating evidence indicates that annexins exert essential roles in the pathogenesis or progression of numerous human diseases, including various types of cancer, autoimmune disorders, diabetes, and cardiovascular diseases.
The expression patterns of annexins differ significantly in various organs with high expression patterns found in the smooth muscle, thymus, and lung and relatively low expression patterns in the testis, adrenal glands, and brain. Interestingly, the expression of annexins could show a dynamic change along with the cell cycle, which may be associated with the microtubule, vesicle transportation, and Ca2+-regulated exocytosis in tissues undergoing myriads of cellular processes or mechanical changes throughout the body (20). As the annexin family is abundantly expressed at the maternal–fetal interface, and both the endometrium and blastocyst undergo rapid growth and differentiation during pregnancy, it is believed to play a series of roles both in the fetus-derived placenta and maternally derived decidual cells.
3 The annexin A protein family at the maternal–fetal interface
3.1 The establishment of the maternal–fetal interface
The fetus-derived trophoblast cells and maternal decidua constitute the maternal–fetal interface. On the fetal side, when the embryo implants completely into the endometrium, the trophoblast is the epithelial overlying layer of the fetal villi floating in the maternal blood, where the trophoblast proliferates and differentiates into cytotrophoblast cells (CTBs). CTBs further differentiate into the syncytiotrophoblasts (STBs) and the extravillous trophoblasts (EVTs). The syncytial trophoblast serves as the outer layer of the placental villi, which directly contacts with decidual glandular secretions and participates in the exchange of nutrients, gases, and waste products between maternal and fetal blood. Furthermore, the STBs dominate the synthesis and secretion of hormones. On the maternal side, the decidual tissue is stimulated by the decidualization inducer and transformed from the endometrium, consisting of luminal epithelial cells, glandular epithelial cells, endothelial cells, differentiated stromal cells, and a diversity of immune cells. Decidual stromal cells (DSCs) are dominant components of the decidua and secrete a wide range of factors for the regulation of the microenvironment at the maternal–fetal interface during early pregnancy (21). In addition, immune cells accumulate and endometrial arteries become more distorted and elongated during decidualization (22). In humans, the endometrium undergoes pre-decidualization as it transforms into the secretory phase during every menstrual cycle, thus allowing the embryo to attach and penetrate (23).
3.2 The annexin A protein family at the maternal–fetal interface
3.2.1 Annexin A1
Annexin A1 (AnxA1) (also known as lipocortin-1 or p35) is a glucocorticoid-induced protein extensively researched as a potent anti-inflammatory molecule in the periphery (24). The expression of AnxA1 in the placenta is mainly in the cytotrophoblast cell surface and syncytial knots as well as in decidual cells (25, 26). A recent study has reported that AnxA1 inhibits inflammation to maintain the optimal microenvironment for implantation (27, 28). Furthermore, AnxA1 was found to be associated with the dynamic interaction between the uterine epithelium and vascular endothelium, a crucial process for successful and adequate decidualization (29).
3.2.2 Annexin A2
Annexin A2 (AnxA2) is ubiquitously expressed and presents an abundance typically in endothelial cells and monocytes, and the upregulation of AnxA2 expression is observed in tumor cells and regarded as a marker of multiple tumors (30). AnxA2 was also upregulated in human receptive endometrium, especially in the luminal epithelium (LE) (31), which was an essential intracellular protein for embryo attachment (32). Matorras et al. have also reported decreased levels of AnxA2 in human endometrial fluid aspirated during the pregnancy cycle compared with the unconceived cycle (33). Another study further proved the function of AnxA2 in the process of decidualization (34). Furthermore, both estrogen and progesterone at physiological levels increase the overall expression of ANXA2 in human endometrial cells (35). Meanwhile, using proximity ligation technology, researchers have observed the precise in-situ location of AnxA2/S100A10 complexes in the human placenta, which showed high levels of expression of the complexes in the amniotic membrane and vascular endothelium cells; however, the expression level was lower in the brush border region of syncytial and trophoblast cells (36).
3.2.3 Annexin A4
Annexin A4 (AnxA4) is predominantly expressed in epithelial cells and reported to increase in epithelial cell tumor (37). AnxA4 is among the most intensively studied proteins in the human endometrial proteome, which is located in the glandular and luminal epithelium and has an impact on endometrial functions via the regulatory effect of ion and water movement across the membrane (38).
3.2.4 Annexin A5
In humans, annexin A5 (AnxA5) is a protein that is most abundantly expressed in the placenta, and its local anticoagulant function has been well described (39). AnxA5 is highly expressed on the apical surfaces of STBs and exerts a crucial role in maintaining blood flow for placental circulation. It has recently been reported that AnxA5 is important in successive steps of membrane overlap and cohesion in both human cytotrophoblasts and STBs (40). AnxA5 presents a cyclical change in the uterine cycle and is found to be upregulated in the luteal phase endometrium in healthy fertile women (41, 42). Meanwhile, the antiphospholipid antibody could mediate the crystallization destruction of AnxA5 on the phospholipid bilayers and cell membranes including endothelial cells and trophoblast cells, accounting for the pathology in antiphospholipid syndrome (43, 44).
3.2.5 Annexin A6
The dual-core annexin A6 (AnxA6) is predominantly found at the plasma membrane and endosomal compartment (45, 46). As a well-known plasma membrane repair protein, AnxA6 acts as a multifunctional scaffolding protein and interacts with phospholipid membranes and different signaling proteins (15, 47). One earlier study has reported that AnxA6 is expressed in the apical and basal STB membranes and could regulate the Maxi-chloride channel in the human placenta (48).
3.2.6 Annexin A7
Annexin A7 (AnxA7) is the first member of the annexin family proteins to be discovered in humans. ANXA7 is expressed in the endometrial glands, stroma, and luminal epithelium according to the Human Protein Atlas (49). The expression of ANXA7 was found upregulated during the process of decidualization, which indicated its conserved role in regulating endometrial receptivity and embryo implantation (50).
3.2.7 Annexin A8
Annexin A8 (AnxA8), also known as vascular anticoagulant-beta 1 (VAC-beta), is expressed at a smidgen level in the placenta, liver, cornea, and lungs (51, 52). The protein is a minor component in the placenta, accounting for less than 1% of all extracted annexins (53). The role of AnxA8 in regulating the proliferation of endometrial cells was observed in porcine (54). However, whether AnxA8 plays a similar role in human endometrium remains unclear.
4 The annexin family and female reproductive disorders
Disruptions of the components at the maternal–fetal interface could lead to placenta dysfunction, including impairing trophoblast invasion function, hindering angiogenesis in the uterus, affecting the process of decidualization, and compromising maternal–fetal immune tolerance (55, 56). Such disturbances are implicated in a spectrum of pregnancy-related complications. Within the human body exists a regulatory network characterized by both precision and complexity, and many mechanistic experiments are conducted utilizing some specific cell lines in vitro. Many antibodies and molecules existing in the peripheral blood play a unique role at the maternal–fetal interface. For instance, research has shown that antiphospholipid antibodies (aPLs) interacting with β2-glycoprotein I (β2GPI) modulate the expression of Bcl-2 and Bax proteins in primary human trophoblasts (57). Furthermore, the influence of anti-transglutaminase type 2 (anti-TG2) autoantibodies on endometrial angiogenesis has been explored using human endometrial endothelial cells (HEECs), shedding light on potential pathogenic mechanisms underlying placental damage in celiac disease (58). Additionally, recent findings indicate the presence of HLA-DR in STBs and STB-derived extracellular vesicles (STEVs) in a significant number of preeclampsia cases, as opposed to control placentas, suggesting a novel avenue of investigation in placental pathology in preeclampsia (59). Alterations in the expression levels of the annexin A proteins are also proven to interrupt those key processes in the establishment of pregnancy.
4.1 Infertility and recurrent implantation failure
In human-assisted reproductive technology (ART) programs, more than 60% of women treated with in-vitro fertilization (IVF) procedures fail to achieve clinical pregnancy after their first transfer and almost 20% of them suffer from unexplained recurrent implantation failures (RIFs) (60). RIF can be defined as failure to clinical pregnancy in a woman under 40 after the transfer of at least four good-quality embryos in at least three fresh or frozen cycles (61). The protein S100-A10 (S100A10), a binding partner of AnxA2, was identified as a critical factor in endometrial receptivity attainment and was downregulated in the mid-secretory phase of the endometrium of infertile women (62). Recent studies have reported that maternal and paternal M2/ANXA5 haplotype carriages are both risk factors for RIF, which shed light on the pathogenesis of RIF and provided possible forecasts for couples who are at a pertinent risk ahead of the ART programs (63).
4.2 Recurrent pregnancy loss
Recurrent pregnancy loss (RPL) is defined as at least two or three spontaneous miscarriages before the 24th gestational week and impacts approximately 1%–3% of reproductive-age women (64). Anatomical abnormalities, endocrine disorders, genetic factors, and immunological factors are considered responsible for RPL; however, almost half of the patients suffered for unexplained reasons (65–67). The role of AnxA5 in RPL has been extensively investigated since Rand JH et al. found that the level of AnxA5 and its anticoagulant activity are significantly reduced in the plasma of RPL patients (68). Moreover, the variants of ANXA5 in the placenta are found important in the pathology of RPL. The haplotype M1 is defined as the combination of two alleles, namely, c.−448 ANC and c.−422 TNC, and reveals single nucleotide polymorphisms (SNPs) of 1A/C and 27T/C. The M2 haplotype, on the other hand, is identified as having four small alleles corresponding to these four SNPs, rs112782763 (c.−467 GNA), rs28717001 (c.−448 ANC), rs28651243 (c.−422 TNC), and rs113588187 (c.−373 GNA), which contains a combination of SNPs of 19G/A, 1A/C, 27T/C, and 76G/A and can be passed on to the offspring (69, 70). Several independent studies have shown that the M2 haplotype in ANXA5 has been linked to greater overall RPL risk mostly for early miscarriage, ranging between the 10th and 15th gestational weeks (71–73). A meta-analysis of 14 independent retrospective case–control studies also summarized that M2/ANXA5 haplotypes in couple populations have a significantly higher risk for RPL in comparison to the normal haplotype (74). Antiphospholipid syndrome (APS) is characterized as an autoimmune disorder that predominantly manifests in thrombotic events. It is observed that approximately 6% of patients with APS experience complications related to pregnancy (75). Extensive research indicates that antibodies in patients diagnosed with APS impede the crystallization and anticoagulant function of AnxA5. This interference leads to a diminished response to the anticoagulant properties of AnxA5 (43). Furthermore, it has been established through in-vitro studies that the application of anti-annexin V monoclonal antibodies (mAbs) precipitates apoptosis in trophoblast cells and results in a marked decrease in human chorionic gonadotropin (hCG) secretion (76).
4.3 Preeclampsia
Preeclampsia (PE) refers to new-onset hypertension, proteinuria with maternal multi-organic dysfunction, or fetal growth restriction after the 20th gestational week (77). PE is the leading cause of maternal and perinatal mortality, occurring in approximately 5% of pregnancies (78, 79). However, the pathological mechanism of PE remains obscure. It is believed that PE is related to shallow invasion of the trophoblast and poor placental perfusion at the maternal–fetal interface, as well as maternal vascular endothelial injury and vascular endothelial dysfunction accompanied by maternal systematic inflammation (56, 80). A recent study has reported that modulation of AnxA1 in the trophoblast is associated with systemic inflammatory response-related preeclampsia (81). Using the 2D-PAGE technique, Gharesi-Fard Behrouz et al. (82) found that AnxA1, as a placental protein, is increased in PE patients, indicating exacerbated systemic inflammation in PE (83). One earlier study has reported that the AnxA2 protein both in the placenta and peripheral maternal blood was downregulated significantly in patients with PE compared with normal pregnancies, which was linked to microvascular thrombin formation in PE (84). Defects in decidualization are also considered as the maternal factor of preeclampsia (85, 86). Researchers further found that defective expression of endometrial ANXA2 might impair the decidualization of endometrial stromal cells in vitro and in vivo, and inhibition of Anxa2 in mice failed to support embryo invasion in vivo functionally (32, 87). Xu et al. demonstrated that ANXA4 expression is downregulated in human placentas in PE, and ANXA4 overexpression in human trophoblast cells may promote trophoblast invasion via the PI3K/AKT/eNOS pathway (88). Another systematic review, the first to combine proteomic studies of the placental biopsies of PE and polycystic ovary syndrome, found five biomarkers for PE which are common in women with PCOS, among which AnxA4 was downregulated in both groups of patients (89). In addition, the M2 haplotype of ANXA5 was also observed to be more prevalent in the placenta of women with PE compared with the controls, which may significantly increase the risk for PE by impairing the thrombomodulatory function of AnxA5 at the maternal–fetal interface (90, 91).
4.4 Intrauterine growth restriction
Small for gestational age (SGA) is defined as the birth weight of a newborn less than the 10th percentile for the corresponding gestational age (92). SGA fetuses are diagnosed with intrauterine growth retardation (IUGR) if they fail to achieve their genetically determined growth potential at any gestational age. Intrauterine growth restriction (IUGR) affects 10%–15% of all pregnancies worldwide (93). IUGR may result from maternal, placental, or fetal factors (94).
Earlier studies have reported that AnxA5 is present in the amniotic fluid and increased during 15 to 24 weeks of gestation. AF-Anxa5 levels are elevated in patients who develop IUGR, which indicates AF-AnxA5 a potential marker for identifying IUGR (95, 96). Another study further reported that decreased ANXA5 mRNA levels were detected in the placenta from SGA pregnancies in comparison to normal outcomes (97). Moreover, a recent study has demonstrated a significantly higher prevalence of the M2 haplotype in women who have delivered an SGA fetus (98), which coordinated with the extensively reported dysfunction of the ANXA5 haplotype in RPL and pre-eclampsia.
5 Conclusion
The expression of annexins in the maternal–fetal interface suggests their roles in embryo implantation and pregnancy. For the maternal side, the expression levels of AnxA1, AnxA2, AnxA4, and AnxA7 are found in decidual stromal cells and epithelial cells, and AnxA1, AnxA2, AnxA5, and AnxA6 are expressed in the trophoblast, especially in the apical and basal STB membranes (Figure 1B). In addition, there is an altered expression of annexins in women with reproductive disorders, such as recurrent implantation failure, endometriosis, adenomyosis, and recurrent pregnancy loss (Table 1). However, whether the annexin family can be used as clinical markers remains uncertain, and further studies are required.
Author contributions
JH: Writing – original draft. LC: Writing – review & editing. JR: Writing – review & editing. XC: Writing – review & editing.
Funding
This study was supported by Natural Science Funding of China (Grant No. 82201851), Shenzhen Key Medical Discipline Construction Fund (Grant No. SZXK028), and Shenzhen Science and Technology Program (Grant No. JCYJ20210324141403009, RCYX20210609104608036).
Conflict of interest
The authors declare that the research was conducted in the absence of any commercial or financial relationships that could be construed as a potential conflict of interest.
Publisher’s note
All claims expressed in this article are solely those of the authors and do not necessarily represent those of their affiliated organizations, or those of the publisher, the editors and the reviewers. Any product that may be evaluated in this article, or claim that may be made by its manufacturer, is not guaranteed or endorsed by the publisher.
References
1. Yap T, Davis B, Guo L, Normando E, Cordeiro M. Annexins in glaucoma. Int J Mol Sci. (2018) 19(4):1218. doi: 10.3390/ijms19041218
2. Grewal T, Enrich C, Rentero C, Buechler C. Annexins in adipose tissue: novel players in obesity. Int J Mol Sci. (2019) 20(14):3449. doi: 10.3390/ijms20143449
3. Li N, Xi Y, Du H, Zhou H, Xu Z. Annexin A4 is dispensable for hair cell development and function. Front Cell Dev Biol. (2021) 9. doi: 10.3389/fcell.2021.680155
4. Lim HI, Hajjar KA. Annexin A2 in fibrinolysis, inflammation and fibrosis. Int J Mol Sci. (2021) 22:6836. doi: 10.3390/ijms22136836
5. Sousa S, Santos M, Teixeira SC, Ferro EAV, Oliani SM. ANNEXIN A1: roles in placenta, cell survival, and nucleus. Cells. (2022) 11(13):2057. doi: 10.3390/cells11132057
6. Zhao R-R, Mao X-R, Wang X-F, Zheng Y, Wang Y-P, Zhou Y-N. Role of annexin A family in tumorigenesis and chemoresistance of gastric cancer. Neoplasma. (2022) 69(02):251–63. doi: 10.4149/neo_2021_210629N872
7. Zhang H, Zhang Z, Guo T, Chen G, Liu G, Song Q, et al. Annexin A protein family: Focusing on the occurrence, progression and treatment of cancer. Front Cell Dev Biol. (2023) 11. doi: 10.3389/fcell.2023.1141331
8. Gerke V, Moss SE. Annexins: from structure to function. Physiol Rev. (2002) 82:331–71. doi: 10.1152/physrev.00030.2001
9. Abd El-Latif M, Azzam H, Othman M, Warda O, El-Sharawy S, Ghoneim H. Assessment of annexin A5 and annexin A2 levels as biomarkers for pre-eclampsia: A pilot study. Pregnancy Hypertension: Int J Women's Cardiovasc Health. (2017) 8:65–9. doi: 10.1016/j.preghy.2017.03.006
10. de Souza Ferreira LP, da Silva RA, Gil CD, Geisow MJ. Annexin A1, A2, A5, and A6 involvement in human pathologies. Proteins: Structure Function Bioinf. (2023) 91:1191–204. doi: 10.1002/prot.26512
11. Li Y-Z, Wang Y-Y, Huang L, Zhao Y-Y, Chen L-H, Zhang C. Annexin A protein family in atherosclerosis. Clinica Chimica Acta. (2022) 531:406–17. doi: 10.1016/j.cca.2022.05.009
12. Yang YH, Morand E, Leech M. Annexin A1: potential for glucocorticoid sparing in RA. Nat Rev Rheumatol. (2013) 9:595–603. doi: 10.1038/nrrheum.2013.126
13. Lauritzen SP, Boye TL, Nylandsted J. Annexins are instrumental for efficient plasma membrane repair in cancer cells. Semin Cell Dev Biol. (2015) 45:32–8. doi: 10.1016/j.semcdb.2015.10.028
14. Boye TL, Nylandsted J. Annexins in plasma membrane repair. Biol Chem. (2016) 397:961–9. doi: 10.1515/hsz-2016-0171
15. Grewal T, Hoque M, Conway JRW, Reverter M, Wahba M, Beevi SS, et al. Annexin A6—A multifunctional scaffold in cell motility. Cell Adhesion Migration. (2017) 11:288–304. doi: 10.1080/19336918.2016.1268318
16. Wu Q, Wei H, Meng W, Xie X, Zhang Z, Su G. Effect of annexinA group translocated in extracellular vesicles on tumorigenesis. Curr Mol Med. (2021) 21:347–53. doi: 10.2174/1566524020666200825163512
17. Bharadwaj A, Kempster E, Waisman DM. The annexin A2/S100A10 complex: the mutualistic symbiosis of two distinct proteins. Biomolecules. (2021) 11:1849. doi: 10.3390/biom11121849
18. Weisz J, Uversky VN. Zooming into the dark side of human annexin-S100 complexes: dynamic alliance of flexible partners. Int J Mol Sci. (2020) 21(16):5879. doi: 10.3390/ijms21165879
19. Liu Y, Myrvang HK, Dekker LV. Annexin A2 complexes with S100 proteins: structure, function and pharmacological manipulation. Br J Pharmacol. (2015) 172:1664–76. doi: 10.1111/bph.12978
20. Bendix PM, Simonsen AC, Florentsen CD, Häger SC, Mularski A, Zanjani AAH, et al. Interdisciplinary synergy to reveal mechanisms of annexin-mediated plasma membrane shaping and repair. Cells. (2020) 9(4):1029. doi: 10.3390/cells9041029
21. Vinketova K, Mourdjeva M, Oreshkova T. Human decidual stromal cells as a component of the implantation niche and a modulator of maternal immunity. J Pregnancy. (2016) 2016:1–17. doi: 10.1155/2016/8689436
22. Harris LK. IFPA Gabor Than Award lecture: Transformation of the spiral arteries in human pregnancy: Key events in the remodelling timeline. Placenta. (2011) 32:S154–8. doi: 10.1016/j.placenta.2010.11.018
23. Diessler ME, Hernández R, Gomez Castro G, Barbeito CG. Decidual cells and decidualization in the carnivoran endotheliochorial placenta. Front Cell Dev Biol. (2023) 11. doi: 10.3389/fcell.2023.1134874
24. Perucci LO, Sugimoto MA, Gomes KB, Dusse LM, Teixeira MM, Sousa LP. Annexin A1 and specialized proresolving lipid mediators: promoting resolution as a therapeutic strategy in human inflammatory diseases. Expert Opin Ther Targets. (2017) 21:879–96. doi: 10.1080/14728222.2017.1364363
25. Sun M, Liu Y, Gibb W. Distribution of annexin I and II in term human fetal membranes, decidua and placenta. Placenta. (1996) 17:181–4. doi: 10.1016/S0143-4004(96)80012-9
26. Moore KG, Goulet F, Sartorelli AC. Purification of annexin I and annexin II from human placental membranes by high-performance liquid chromatography. Protein Expression Purification. (1992) 3:1–7. doi: 10.1016/1046-5928(92)90049-3
27. D'Acunto CW, Gbelcova H, Festa M, Ruml T. The complex understanding of Annexin A1 phosphorylation. Cell Signalling. (2014) 26:173–8. doi: 10.1016/j.cellsig.2013.09.020
28. Boudhraa Z, Bouchon B, Viallard C, D'Incan M, Degoul F. Annexin A1 localization and its relevance to cancer. Clin Sci. (2016) 130:205–20. doi: 10.1042/CS20150415
29. Hebeda CB, Sandri S, Benis CM, Paula-Silva Md, Loiola RA, Reutelingsperger C, et al. Annexin A1/formyl peptide receptor pathway controls uterine receptivity to the blastocyst. Cells. (2020) 9(5):1188. doi: 10.3390/cells9051188
30. Liu W, Hajjar KA. The annexin A2 system and angiogenesis. Biol Chem. (2016) 397:1005–16. doi: 10.1515/hsz-2016-0166
31. Kristoffersen EK, Matre R. Surface annexin II on placental membranes of the fetomaternal interface. Am J Reprod Immunol. (1996) 36:141–9. doi: 10.1111/j.1600-0897.1996.tb00155.x
32. Garrido-Gómez T, Dominguez F, Quiñonero A, Estella C, Vilella F, Pellicer A, et al. Annexin A2 is critical for embryo adhesiveness to the human endometrium by RhoA activation through F-actin regulation. FASEB J. (2012) 26:3715–27. doi: 10.1096/fj.12-204008
33. Matorras R, Quevedo S, Corral B, Prieto B, Exposito A, Mendoza R, et al. Proteomic pattern of implantative human endometrial fluid in in vitro fertilization cycles. Arch Gynecology Obstetrics. (2018) 297:1577–86. doi: 10.1007/s00404-018-4753-1
34. Graham A, Holbert J, Nothnick WB. miR-181b-5p modulates cell migratory proteins, tissue inhibitor of metalloproteinase 3, and annexin A2 during in vitro decidualization in a human endometrial stromal cell line. Reprod Sci. (2017) 24:1264–74. doi: 10.1177/1933719116682877
35. Wang B, Shao Y. Annexin A2 acts as an adherent molecule under the regulation of steroids during embryo implantation. Mol Hum Reprod. (2020) 26:825–36. doi: 10.1093/molehr/gaaa065
36. Abd El-Aleem SA, Dekker LV. Assessment of the cellular localisation of the annexin A2/S100A10 complex in human placenta. J Mol Histol. (2018) 49:531–43. doi: 10.1007/s10735-018-9791-2
38. Chen JIC, Hannan NJ, Mak Y, Nicholls PK, Zhang J, Rainczuk A, et al. Proteomic characterization of midproliferative and midsecretory human endometrium. J Proteome Res. (2009) 8:2032–44. doi: 10.1021/pr801024g
39. Krikun G, Lockwood C, Wu X, Zhou X, Guller S, Calandri C, et al. The expression of the placental anticoagulant protein, annexin V, by villous trophoblasts: Immunolocalization and in vitro regulation. Placenta. (1994) 15:601–12. doi: 10.1016/S0143-4004(05)80407-2
40. Bouter A, Carmeille R, Gounou C, Bouvet F, Degrelle SA, Evain-Brion D, et al. Review: Annexin-A5 and cell membrane repair. Placenta. (2015) 36:S43–9. doi: 10.1016/j.placenta.2015.01.193
41. Parmar T, Gadkar-Sable S, Savardekar L, Katkam R, Dharma S, Meherji P, et al. Protein profiling of human endometrial tissues in the midsecretory and proliferative phases of the menstrual cycle. Fertility Sterility. (2009) 92:1091–103. doi: 10.1016/j.fertnstert.2008.07.1734
42. Rai P, Kota V, Sundaram CS, Deendayal M, Shivaji S. Proteome of human endometrium: Identification of differentially expressed proteins in proliferative and secretory phase endometrium. Proteomics - Clin Appl. (2010) 4:48–59. doi: 10.1002/prca.200900094
43. Rand JH, Wu XX, Quinn AS, Taatjes DJ. The annexin A5-mediated pathogenic mechanism in the antiphospholipid syndrome: role in pregnancy losses and thrombosis. Lupus. (2010) 19:460–9. doi: 10.1177/0961203310361485
44. Singh NK, Yadav DP, Gupta A, Singh U, Godara M. Role of anti-annexin A5 in pathogenesis of hypercoagulable state in patients with antiphospholipid syndrome. Int J Rheumatic Dis. (2013) 16:325–30. doi: 10.1111/1756-185X.12044
45. Grewal T, Koese M, Rentero C, Enrich C. Annexin A6-regulator of the EGFR/Ras signalling pathway and cholesterol homeostasis. Int J Biochem Cell Biol. (2010) 42:580–4. doi: 10.1016/j.biocel.2009.12.020
46. Cao J, Wan S, Chen S, Yang L. ANXA6: a key molecular player in cancer progression and drug resistance. Discover Oncol. (2023) 14(1):53. doi: 10.1007/s12672-023-00662-x
47. Ngo JM, Williams JK, Lehman IM, Schekman R. Annexin A6 mediates calcium-dependent exosome secretion during plasma membrane repair. eLife. (2023) 12:e86556. doi: 10.7554/eLife.86556
48. Riquelme G, Llanos P, Tischner E, Neil J, Campos B. Annexin 6 modulates the maxi-chloride channel of the apical membrane of syncytiotrophoblast isolated from human placenta. J Biol Chem. (2004) 279:50601–8. doi: 10.1074/jbc.M407859200
49. Uhlén M, Björling E, Agaton C, Szigyarto CA-K, Amini B, Andersen E, et al. A human protein atlas for normal and cancer tissues based on antibody proteomics. Mol Cell Proteomics. (2005) 4:1920–32. doi: 10.1074/mcp.M500279-MCP200
50. Alauddin M, Salker MS, Umbach AT, Rajaxavier J, Okumura T, Singh Y, et al. Annexin A7 regulates endometrial receptivity. Front Cell Dev Biol. (2020) 8. doi: 10.3389/fcell.2020.00770
51. Gerke V. Annexins A2 and A8 in endothelial cell exocytosis and the control of vascular homeostasis. Biol Chem. (2016) 397:995–1003. doi: 10.1515/hsz-2016-0207
52. Poeter M, Brandherm I, Rossaint J, Rosso G, Shahin V, Skryabin BV, et al. Annexin A8 controls leukocyte recruitment to activated endothelial cells via cell surface delivery of CD63. Nat Commun. (2014) 5:3738. doi: 10.1038/ncomms4738
53. Pepinsky RB, Hauptmann R. Detection of VAC-β (annexin-8) in human placenta. FEBS Lett. (1992) 306:85–9. doi: 10.1016/0014-5793(92)80843-6
54. Jiang X, Xue S, Kang T, Liu H, Ren H, Hua R, et al. Annexin A8 (ANXA8) regulates proliferation of porcine endometrial cells via Akt signalling pathway. Reprod Domest Anim. (2019) 54:3–10. doi: 10.1111/rda.13280
55. Brosens I, Puttemans P, Benagiano G. Placental bed research: I. The placental bed: from spiral arteries remodeling to the great obstetrical syndromes. Am J Obstet Gynecol. (2019) 221:437–56. doi: 10.1016/j.ajog.2019.05.044
56. Garrido-Gomez T, Castillo-Marco N, Cordero T, Simon C. Decidualization resistance in the origin of preeclampsia. Am J Obstet Gynecol. (2022) 226:S886–94. doi: 10.1016/j.ajog.2020.09.039
57. di Simone N, Castellani R, Raschi E, Borghi MO, Meroni PL, Caruso A. Anti-beta-2 glycoprotein I antibodies affect Bcl-2 and Bax trophoblast expression without evidence of apoptosis. Ann N Y Acad Sci. (2006) 1069:364–76. doi: 10.1196/annals.1351.034
58. Di Simone N, De Spirito M, Di Nicuolo F, Tersigni C, Castellani R, Silano M, et al. Potential new mechanisms of placental damage in celiac disease: anti-transglutaminase antibodies impair human endometrial angiogenesis. Biol Reprod. (2013) 89:88. doi: 10.1095/biolreprod.113.109637
59. Tersigni C, Redman CW, Dragovic R, Tannetta D, Scambia G, Di Simone N, et al. HLA-DR is aberrantly expressed at feto-maternal interface in pre-eclampsia. J Reprod Immunol. (2018) 129:48–52. doi: 10.1016/j.jri.2018.06.024
60. Coughlan C, Ledger W, Wang Q, Liu F, Demirol A, Gurgan T, et al. Recurrent implantation failure: definition and management. Reprod BioMedicine Online. (2014) 28:14–38. doi: 10.1016/j.rbmo.2013.08.011
61. Cimadomo D, Craciunas L, Vermeulen N, Vomstein K, Toth B. Definition, diagnostic and therapeutic options in recurrent implantation failure: an international survey of clinicians and embryologists. Hum Reprod. (2021) 36:305–17. doi: 10.1093/humrep/deaa317
62. Bissonnette L, Drissennek L, Antoine Y, Tiers L, Hirtz C, Lehmann S, et al. Human S100A10 plays a crucial role in the acquisition of the endometrial receptivity phenotype. Cell Adhesion Migration. (2016) 10:282–98. doi: 10.1080/19336918.2015.1128623
63. Rogenhofer N, Markoff A, Ennerst X, Bogdanova N, Thaler C. Maternal and paternal carriage of the annexin A5 M2 haplotype: a possible risk factor for recurrent implantation failure (RIF). J Assisted Reprod Genet. (2020) 38:235–42. doi: 10.1007/s10815-020-01978-1
64. Giouleka S, Tsakiridis I, Arsenaki E, Kalogiannidis I, Mamopoulos A, Papanikolaou E, et al. Investigation and management of recurrent pregnancy loss: A comprehensive review of guidelines. Obstetrical Gynecological Survey. (2023) 78:287–301. doi: 10.1097/OGX.0000000000001133
65. Melo P, Dhillon-Smith R, Islam MA, Devall A, Coomarasamy A. Genetic causes of sporadic and recurrent miscarriage. Fertility Sterility. (2023) 120(5):940–4. doi: 10.1016/j.fertnstert.2023.08.952
66. Béquet YLBN, Lashley EELO, Goddijn M, van der Hoorn MLP. The role of uterine natural killer cells in recurrent pregnancy loss and possible treatment options. Fertility Sterility. (2023) 120(5):945–7. doi: 10.1016/j.fertnstert.2023.08.949
67. Inversetti A, Perna G, Lalli G, Grande G, Di Simone N. Depression, stress and anxiety among women and men affected by recurrent pregnancy loss (RPL): A systematic review and meta-analysis. Life. (2023) 13(6):1268. doi: 10.3390/life13061268
68. Rand JH, Arslan AA, Wu X-X, Wein R, Mulholland J, Shah M, et al. Reduction of circulating annexin A5 levels and resistance to annexin A5 anticoagulant activity in women with recurrent spontaneous pregnancy losses. Am J Obstetrics Gynecology. (2006) 194:182–8. doi: 10.1016/j.ajog.2005.05.034
69. Bogdanova N, Horst J, Chlystun M, Croucher PJP, Nebel A, Bohring A, et al. A common haplotype of the annexin A5 (ANXA5) gene promoter is associated with recurrent pregnancy loss. Hum Mol Genet. (2007) 16:573–8. doi: 10.1093/hmg/ddm017
70. Hayashi Y, Sasaki H, Suzuki S, Nishiyama T, Kitaori T, Mizutani E, et al. Genotyping analyses for polymorphisms of ANXA5 gene in patients with recurrent pregnancy loss. Fertility Sterility. (2013) 100:1018–24. doi: 10.1016/j.fertnstert.2013.06.020
71. Tüttelmann F, Ivanov P, Dietzel C, Sofroniou A, Tsvyatkovska TM, Komsa-Penkova RS, et al. Further insights into the role of the annexin A5 M2 haplotype as recurrent pregnancy loss factor, assessing timing of miscarriage and partner risk. Fertility Sterility. (2013) 100:1321–5. doi: 10.1016/j.fertnstert.2013.06.046
72. Alijotas-Reig J, Ferrer-Oliveras R, Rodrigo-Anoro MJ, Farran-Codina I, Llurba-Olivé E, Vilardell-Tarres M, et al. Anti-annexin A5 antibodies in women with spontaneous pregnancy loss. Medicina Clínica. (2010) 134:433–8. doi: 10.1016/j.medcli.2009.09.040
73. Miyamura H, Nishizawa H, Ota S, Suzuki M, Inagaki A, Egusa H, et al. Polymorphisms in the annexin A5 gene promoter in Japanese women with recurrent pregnancy loss. Mol Hum Reprod. (2011) 17:447–52. doi: 10.1093/molehr/gar008
74. Ang K-C, Bogdanova N, Markoff A, Ch'ng ES, Tang TH. Association between M2/ANXA5 haplotype and repeated pregnancy loss: a meta-analysis. Fertility Sterility. (2019) 111:971–981.e2. doi: 10.1016/j.fertnstert.2019.01.015
75. Scholz P, Auler M, Brachvogel B, Benzing T, Mallman P, Streichert T, et al. Detection of multiple annexin autoantibodies in a patient with recurrent miscarriages, fulminant stroke and seronegative antiphospholipid syndrome. Biochem Med (Zagreb). (2016) 26:272–8. doi: 10.11613/issn.1846-7482
76. Di Simone N, Castellani R, Caliandro D, Caruso A. Monoclonal anti-annexin V antibody inhibits trophoblast gonadotropin secretion and induces syncytiotrophoblast apoptosis. Biol Reprod. (2001) 65:1766–70. doi: 10.1095/biolreprod65.6.1766
77. Chappell LC, Cluver CA, Kingdom J, Tong S. Pre-eclampsia. Lancet. (2021) 398:341–54. doi: 10.1016/S0140-6736(20)32335-7
78. Say L, Chou D, Gemmill A, Tunçalp Ö, Moller A-B, Daniels J, et al. Global causes of maternal death: a WHO systematic analysis. Lancet Global Health. (2014) 2:e323–33. doi: 10.1016/S2214-109X(14)70227-X
79. Honigberg MC, Truong B, Khan RR, Xiao B, Bhatta L, Vy HMT, et al. Polygenic prediction of preeclampsia and gestational hypertension. Nat Med. (2023) 29(6):1540–9. doi: 10.1038/s41591-023-02374-9
80. Peng M, Yang M, Ding Y, Yu L, Deng Y, Lai W, et al. Mechanism of endogenous digitalis-like factor−induced vascular endothelial cell damage in patients with severe preeclampsia. Int J Mol Med. (2018) 41(2):985–94. doi: 10.3892/ijmm.2017.3316
81. Ruikar K, Aithal M, Shetty P, Dinesh US, Bargale A, Sadashiv R, et al. Placental expression and relative role of anti-inflammatory annexin A1 and animal lectin galectin-3 in the pathogenesis of preeclampsia. Indian J Clin Biochem. (2021) 37:60–8. doi: 10.1007/s12291-020-00952-z
82. Behrouz G-F, Farzaneh G-s, Leila J, Jaleh Z, Eskandar K-S. Presence of auto-antibody against two placental proteins, annexin A1 and vitamin D binding protein, in sera of women with pre-eclampsia. J Reprod Immunol. (2013) 99:10–6. doi: 10.1016/j.jri.2013.04.007
83. Kanellopoulos-Langevin C, Perucci LO, Carneiro FS, Ferreira CN, Sugimoto MA, Soriani FM, et al. Annexin A1 is increased in the plasma of preeclamptic women. PloS One. (2015) 10(9):e0138475. doi: 10.1371/journal.pone.0138475
84. Xin H, Zhang Y, Wang H, Sun S. Alterations of profibrinolytic receptor annexin A2 in pre-eclampsia: a possible role in placental thrombin formation. Thromb Res. (2012) 129:563–7. doi: 10.1016/j.thromres.2011.07.039
85. Garrido-Gomez T, Dominguez F, Quiñonero A, Diaz-Gimeno P, Kapidzic M, Gormley M, et al. Defective decidualization during and after severe preeclampsia reveals a possible maternal contribution to the etiology. Proc Natl Acad Sci. (2017) 114(40):E8468–77. doi: 10.1073/pnas.1706546114
86. Rabaglino MB, Post Uiterweer ED, Jeyabalan A, Hogge WA, Conrad KP. Bioinformatics approach reveals evidence for impaired endometrial maturation before and during early pregnancy in women who developed preeclampsia. Hypertension. (2015) 65:421–9. doi: 10.1161/HYPERTENSIONAHA.114.04481
87. Garrido-Gomez T, Quiñonero A, Dominguez F, Rubert L, Perales A, Hajjar KA, et al. Preeclampsia: a defect in decidualization is associated with deficiency of Annexin A2. Am J Obstetrics Gynecology. (2020) 222:376.e1–376.e17. doi: 10.1016/j.ajog.2019.11.1250
88. Xu Y, Sui L, Qiu B, Yin X, Liu J, Zhang X. ANXA4 promotes trophoblast invasion via the PI3K/Akt/eNOS pathway in preeclampsia. Am J Physiology-Cell Physiol. (2019) 316(4):C481–91. doi: 10.1152/ajpcell.00404.2018
89. Khan GH, Galazis N, Docheva N, Layfield R, Atiomo W. Overlap of proteomics biomarkers between women with pre-eclampsia and PCOS: a systematic review and biomarker database integration. Hum Reprod. (2014) 30:133–48. doi: 10.1093/humrep/deu268
90. Ota S, Miyamura H, Nishizawa H, Inagaki H, Inagaki A, Inuzuka H, et al. Contribution of fetal ANXA5 gene promoter polymorphisms to the onset of pre-eclampsia. Placenta. (2013) 34:1202–10. doi: 10.1016/j.placenta.2013.09.010
91. Margaglione M, Favuzzi G, Sciannamè N, Pisanelli D, Chinni E, Colaizzo D, et al. Haplotype M2 in the annexin A5 (ANXA5) gene and the occurrence of obstetric complications. Thromb Haemostasis. (2017) 102:309–13. doi: 10.1160/TH09-02-0123
92. Sutherland MR, Black MJ. The impact of intrauterine growth restriction and prematurity on nephron endowment. Nat Rev Nephrol. (2023) 19:218–28. doi: 10.1038/s41581-022-00668-8
93. Armengaud JB, Yzydorczyk C, Siddeek B, Peyter AC, Simeoni U. Intrauterine growth restriction: Clinical consequences on health and disease at adulthood. Reprod Toxicol. (2021) 99:168–76. doi: 10.1016/j.reprotox.2020.10.005
94. Zhou H, Zhao C, Wang P, Yang W, Zhu H, Zhang S. Regulators involved in trophoblast syncytialization in the placenta of intrauterine growth restriction. Front Endocrinol. (2023) 14. doi: 10.3389/fendo.2023.1107182
95. Van Eerden P, Wu X-X, Chazotte C, Rand JH. Annexin A5 levels in midtrimester amniotic fluid: Association with intrauterine growth restriction. Am J Obstetrics Gynecology. (2006) 194:1371–6. doi: 10.1016/j.ajog.2005.11.005
96. Dundar O, Yoruk P, Tutuncu L, Muhcu M, Ipcioglu O, Ergur AR, et al. Second trimester amniotic fluid annexin A5 levels and subsequent development of intrauterine growth restriction. Prenatal Diagnosis. (2008) 28:887–91. doi: 10.1002/pd.2076
97. Sifakis S, Soufla G, Koukoura O, Soulitzis N, Koutroulakis D, Maiz N, et al. Decreased Annexin A5 mRNA placental expression in pregnancies complicated by fetal growth restriction. Thromb Res. (2010) 125:326–31. doi: 10.1016/j.thromres.2009.11.033
98. Tiscia G, Colaizzo D, Favuzzi G, Vergura P, Martinelli P, Margaglione M, et al. The M2 haplotype in the ANXA5 gene is an independent risk factor for idiopathic small-for-gestational age newborns. MHR: Basic Sci Reprod Med. (2012) 18:510–3. doi: 10.1093/molehr/gas023
99. Paula R, Oliani AH, Vaz-Oliani DCM, D’Ávila SCGP, Oliani SM, Gil CD. The intricate role of mast cell proteases and the annexin A1-FPR1 system in abdominal wall endometriosis. J Mol Histol. (2014) 46:33–43. doi: 10.1007/s10735-014-9595-y
100. Perucci LO, Vieira ÉLM, Teixeira AL, Gomes KB, Dusse LM, Sousa LP. Decreased plasma concentrations of brain-derived neurotrophic factor in preeclampsia. Clinica Chimica Acta. (2017) 464:142–7. doi: 10.1016/j.cca.2016.11.034
101. Zhou S, Yi T, Liu R, Bian C, Qi X, He X, et al. Proteomics identification of annexin A2 as a key mediator in the metastasis and proangiogenesis of endometrial cells in human adenomyosis. Mol Cell Proteomics. (2012) 11:M112.017988–1-M112.017988-24. doi: 10.1074/mcp.M112.017988
102. Liu F, Liu L, Zheng J. Expression of annexin A2 in adenomyosis and dysmenorrhea. Arch Gynecology Obstetrics. (2019) 300:711–6. doi: 10.1007/s00404-019-05205-w
103. Amjadi F, Mehdizadeh M, Ashrafi M, Nasrabadi D, Taleahmad S, Mirzaei M, et al. Distinct changes in the proteome profile of endometrial tissues in polycystic ovary syndrome compared with healthy fertile women. Reprod BioMedicine Online. (2018) 37:184–200. doi: 10.1016/j.rbmo.2018.04.043
104. Sahar T, Nigam A, Anjum S, Waziri F, Jain SK, Wajid S. Differential expression of Lumican, Mimecan, Annexin A5 and Serotransferrin in ectopic and matched eutopic endometrium in ovarian endometriosis: a case-control study. Gynecological Endocrinol. (2020) 37:56–60. doi: 10.1080/09513590.2020.1824218
105. Gourvas V, Soulitzis N, Konstantinidou A, Dalpa E, Koukoura O, Koutroulakis D, et al. Reduced ANXA5 mRNA and protein expression in pregnancies complicated by preeclampsia. Thromb Res. (2014) 133:495–500. doi: 10.1016/j.thromres.2013.12.027
106. Dasgupta S, Maity P, Banerjee U, Mukhopadhyay P, Saha S, Das B. Clinicopathological study of annexin A5 & apelin in pre-eclamptic placentae with emphasis on foetal outcome. Indian J Med Res. (2021) 154(6):813–22. doi: 10.4103/ijmr.IJMR_433_21
107. Zheng M, Yan J, Jiang L, Dai Z, Liu X. Association between ANXA5 haplotypes and the risk of recurrent pregnancy loss. J Int Med Res. (2022) 50(7). doi: 10.1177/03000605211026809
Keywords: annexin family, calcium and phospholipid binding proteins, maternal-fetal interface, trophoblast, female reproductive disorder
Citation: Hu J, Chen L, Ruan J and Chen X (2024) The role of the annexin A protein family at the maternal–fetal interface. Front. Endocrinol. 15:1314214. doi: 10.3389/fendo.2024.1314214
Received: 10 October 2023; Accepted: 09 February 2024;
Published: 01 March 2024.
Edited by:
Eytan R. Barnea, BioIncept, LLC, United StatesReviewed by:
Nicoletta Di Simone, Humanitas University, ItalyCopyright © 2024 Hu, Chen, Ruan and Chen. This is an open-access article distributed under the terms of the Creative Commons Attribution License (CC BY). The use, distribution or reproduction in other forums is permitted, provided the original author(s) and the copyright owner(s) are credited and that the original publication in this journal is cited, in accordance with accepted academic practice. No use, distribution or reproduction is permitted which does not comply with these terms.
*Correspondence: Xiaoyan Chen, Y2hlbnhpYW95YW5AY3Voay5lZHUuaGs=