- 1Chair of Human Genetics, Institute of Biomedicine and Translational Medicine, University of Tartu, Tartu, Estonia
- 2Andrology Clinic, Tartu University Hospital, Tartu, Estonia
- 3Centre of Pathology, East Tallinn Central Hospital, Tallinn, Estonia
- 4Division of Genetics, Oregon National Primate Research Center, Oregon Health and Science University, Beaverton, OR, United States
- 5Department of Surgery, Institute of Clinical Medicine, University of Tartu, Tartu, Estonia
- 6Department of Pediatric Surgery, Clinic of Surgery, Tartu University Hospital, Tartu, Estonia
- 7Interuniversity Institute of Bioinformatics in Brussels, Université Libre de Bruxelles-Vrije Universiteit Brussel, Brussels, Belgium
- 8Machine Learning Group, Université Libre de Bruxelles, Brussels, Belgium
- 9Department of Biomolecular Medicine, Faculty of Medicine and Health Science, Ghent University, Ghent, Belgium
- 10Hudson Institute of Medical Research and the Department of Obstetrics and Gynecology, Monash University, Clayton, VIC, Australia
- 11Division of Urology, Department of Surgery, Mount Sinai Hospital, University of Toronto, Toronto, ON, Canada
- 12Department of Urology, Weill Cornell Medical College, New York, NY, United States
- 13School of BioSciences, Faculty of Science, The University of Melbourne, Parkville, VIC, Australia
- 14Andrology and IVF Laboratory, Department of Surgery (Urology), University of Utah School of Medicine, Salt Lake City, UT, United States
- 15Artificial Intelligence Laboratory, Vrije Universiteit Brussel, Brussels, Belgium
- 16Center for Embryonic Cell and Gene Therapy, Oregon Health and Science University, Beaverton, OR, United States
RASopathies are syndromes caused by congenital defects in the Ras/mitogen-activated protein kinase (MAPK) pathway genes, with a population prevalence of 1 in 1,000. Patients are typically identified in childhood based on diverse characteristic features, including cryptorchidism (CR) in >50% of affected men. As CR predisposes to spermatogenic failure (SPGF; total sperm count per ejaculate 0–39 million), we hypothesized that men seeking infertility management include cases with undiagnosed RASopathies. Likely pathogenic or pathogenic (LP/P) variants in 22 RASopathy-linked genes were screened in 521 idiopathic SPGF patients (including 155 CR cases) and 323 normozoospermic controls using exome sequencing. All 844 men were recruited to the ESTonian ANDrology (ESTAND) cohort and underwent identical andrological phenotyping. RASopathy-specific variant interpretation guidelines were used for pathogenicity assessment. LP/P variants were identified in PTPN11 (two), SOS1 (three), SOS2 (one), LZTR1 (one), SPRED1 (one), NF1 (one), and MAP2K1 (one). The findings affected six of 155 cases with CR and SPGF, three of 366 men with SPGF only, and one (of 323) normozoospermic subfertile man. The subgroup “CR and SPGF” had over 13-fold enrichment of findings compared to controls (3.9% vs. 0.3%; Fisher’s exact test, p = 5.5 × 10−3). All ESTAND subjects with LP/P variants in the Ras/MAPK pathway genes presented congenital genitourinary anomalies, skeletal and joint conditions, and other RASopathy-linked health concerns. Rare forms of malignancies (schwannomatosis and pancreatic and testicular cancer) were reported on four occasions. The Genetics of Male Infertility Initiative (GEMINI) cohort (1,416 SPGF cases and 317 fertile men) was used to validate the outcome. LP/P variants in PTPN11 (three), LZTR1 (three), and MRAS (one) were identified in six SPGF cases (including 4/31 GEMINI cases with CR) and one normozoospermic man. Undiagnosed RASopathies were detected in total for 17 ESTAND and GEMINI subjects, 15 SPGF patients (10 with CR), and two fertile men. Affected RASopathy genes showed high expression in spermatogenic and testicular somatic cells. In conclusion, congenital defects in the Ras/MAPK pathway genes represent a new congenital etiology of syndromic male infertility. Undiagnosed RASopathies were especially enriched among patients with a history of cryptorchidism. Given the relationship between RASopathies and other conditions, infertile men found to have this molecular diagnosis should be evaluated for known RASopathy-linked health concerns, including specific rare malignancies.
Introduction
RASopathies or RAS/mitogen-activated protein kinase (MAPK) syndromes are a group of phenotypically overlapping congenital syndromes caused by germline disease-causing variants in 22 genes encoding components of the Ras/MAPK pathway (1, 2). Dysfunction of this cellular signaling pathway during fetal development causes a spectrum of syndromes characterized by skeletal, cardiac, gastrointestinal, neurologic, and dermatological conditions and, on some occasions, distinct facial features. Cryptorchidism (CR), testicular maldescent, has been reported in ~60%–80% of male patients with Noonan syndrome (NS) (3, 4), the most prevalent RASopathy. CR due to primary testicular dysgenesis represents an established risk factor for spermatogenic failure (SPGF) in adulthood (5, 6).
There are limited data on reproductive and general health comorbidities in adults carrying germline variants in RASopathy-linked genes, as most studies have been conducted in pediatric cohorts. We recently reported an infertile man with bilateral CR and cryptozoospermia carrying a pathogenic variant in SOS1 and proposed genetic alterations in the Ras/MAPK pathway as a novel etiology implicated in congenital CR and SPGF (7). We hypothesized that SPGF patients seeking infertility management include cases with undiagnosed RASopathies. This study aimed to screen a large cohort of unexplained SPGF cases compared to normozoospermic men for disease-causing variants in the Ras/MAPK pathway genes and to characterize the respective genotype–phenotype links in detail.
Patients and methods
Ethics statement
Research of the ESTonian ANDrology (ESTAND) cohort has been approved by the Ethics Review Committee of Human Research of the University of Tartu, Estonia (permissions no. 74/54 and 118/69 with last amendment 288/M-13; 221/T-6, 286/M-18). All ESTAND study subjects have been recruited at the Andrology Clinic of Tartu University Hospital (AC-TUH), managing >90% of all severe male infertility cases in Estonia (5). Research in the involved Genetics of Male Infertility Initiative (GEMINI) study centers has been approved by IRB_00063950 (IRB of the University of Utah, USA); 16030459 and 0102004794 (IRB of Weill Cornell Medical College, New York, USA); 15-0147-E (IRB of Mount Sinai Hospital, University of Toronto, Toronto, Canada); and human ethics committees of Monash Surgical Day Hospital, Monash Medical Centre and Monash University, Australia.
Written informed consent was obtained from each patient before recruitment. The study was carried out in compliance with the Declaration of Helsinki.
ESTAND cohort study group formation
The study group for whole-exome sequencing (WES) consisted of 844 participants of the ESTAND cohort (PI: M. Punab and M. Laan): 521 patients with idiopathic SPGF and 323 normozoospermic partners of pregnant women as controls (CTRL; Table 1) (5, 9). All men had undergone identical routine andrological workup using the established clinical pipeline and standard protocols at the AC-TUH (Supplementary Methods). SPGF was defined as a total sperm count of 0–39 million per ejaculate (8). Patients were prioritized to WES based on severe unexplained SPGF suggesting a possible genetic cause (e.g., no sperms or extremely low total sperm count per ejaculate, abnormal hormonal parameters, and genitourinary conditions). Men with at least one testicle missing in the scrotum at the recruitment or medical history of CR formed the “SPGF with CR” subgroup (n = 155), whereas the rest were defined as the “SPGF only” subgroup (n = 366). The two patient subgroups did not differ in andrological parameters, except for lower total testis volume (TTV) in CR cases (24.0 vs. 27.5 mL, p = 5.71 × 10−5; Table 1). “SPGF only” patients (median age 35.4 y) were slightly older than “SPGF with CR” (32.4 y) and CTRL men (31.0 y, p < 0.001), and normozoospermic men had lower body mass index (BMI) than patients (25.0 vs. 26.2–26.6, p < 0.05).
Eight of 10 subjects with disease-causing findings in the Ras/MAPK pathway genes consented to a follow-up assessment based on the established clinical recommendations for the phenotyping of RASopathy patients (10, 11) (Supplementary Methods).
Disease-causing variant detection in the WES dataset
WES data were generated in three next-generation sequencing service laboratories: the Institute for Molecular Medicine Finland (FIMM), Helsinki, Finland (n = 447) (7, 12), the McDonnell Genome Institute of Washington University in St. Louis, MO, USA (n = 82) as previously described (13, 14), and the Huntsman Cancer Institute High-Throughput Genomics Core Facility at the University of Utah, Salt Lake City, UT, USA (n = 315) (Supplementary Methods).
VCF files generated by all laboratories were filtered for variant quality using identical parameters (exclusion DP < 10 and GQ < 20) and merged into a single VCF file to be annotated using the Ensembl platform tool VEP v105 (15) (Supplementary Table 1). Likely pathogenic (LP) or pathogenic (P) variants were screened in 22 Ras/MAPK pathway genes, including 20 autosomal dominant (AD) genes, SPRED2 linked to autosomal recessive, and LZTR1 linked to both modes of inheritance (Supplementary Table 2). VEP output files were subjected to three stages of filtering: custom-designed pipeline for automatic exclusion of variants with an unlikely monogenic disease-causing effect (Supplementary Table 3), variant pathogenicity interpretation with the AI-based tool (https://franklin.genoox.com—Franklin by Genoox) to exclude likely benign and benign predictions, and final manual classification of the retained variants using the American College of Medical Genetics and Genomics (ACMG) guidelines for the Ras/MAPK pathway genes (16) (Supplementary Table 5).
All 10 subjects with findings in the Ras/MAPK gene panel underwent extended WES data analysis to assess whether the identified LP/P variant was a monogenic cause explaining the patient’s phenotype. The ORVAL (Oligogenic Resource for Variant AnaLysis) platform (17) and VarCoPP2.0 tool (18) were implemented for statistical modeling and estimating the probability of digenic pathogenicity. Further details of WES data processing and analysis are provided in Supplementary Methods.
Testicular histopathology and immunohistochemical staining
Testis biopsies were collected, and histopathological examinations were carried out for 110 of 521 (21.0%) SPGF cases at the Pathology Centre, Diagnostic Clinic of the East Tallinn Central Hospital, Estonia, or Pathology Department, Tartu University Hospital, Estonia, following the guidelines (19). In the clinical setting, this invasive procedure was undertaken only when justified for clinical decision making in infertility management and consented by the patient.
Immunohistochemistry was applied to analyze PTPN11 protein expression in paraffin-embedded testis biopsy samples from Case 1 with PTPN11 p.N308D (35 y) and a control subject (38 y, obstructive azoospermia, histologically normal active spermatogenesis). The monoclonal rabbit anti-SHP2 (Y478) antibody (Abcam, Cambridge, UK) was diluted 1:10 in Antibody Diluent (Dako, Agilent, Santa Clara, USA) and incubated for 60 minutes at 37°C. The antigen–antibody complex was visualized using the Ventana OptiView DAB IHC Detection and Amplification Kits and Reaction Buffer for washing steps (Roche Diagnostics GmbH, Mannheim, Germany). Details are reported in Supplementary Methods.
Validation cohort for the assessment of RASopathy-linked variants in infertile men
Further assessment for RASopathy-linked variants in SPGF patients was performed in the WES dataset of the GEMINI cohort (PI: D.F. Conrad and K.I. Aston). This cohort has been mainly formed to discover novel monogenic causes of non-obstructive azoospermia (NOA) among idiopathic cases recruited in andrology clinics worldwide (13, 14) (Supplementary Methods). Assessment for LP/P variants in 22 RASopathy-linked genes in the GEMINI cohort was carried out as described above. Variants were screened in 1,416 SPGF patients (1,119 NOA and 297 oligozoospermia cases) and 317 fertile controls (Supplementary Table 6). For 31 SPGF cases, medical history of cryptorchidism had been documented. Variant filtering and interpretation followed an identical pipeline as utilized for the ESTAND data. The final manual classification of the retained variants was carried out according to the ACMG guidelines (16) (Supplementary Table 7).
Experimental confirmation of the predicted LP/P variants
All variants predicted to be disease-causing based on the WES data analysis in the ESTAND and GEMINI cohorts were confirmed by Sanger sequencing (Supplementary Figures 1–3, Supplementary Table 4).
Testicular gene expression dataset
Gene expression levels in distinct testicular cell types in six adult control patients were derived from the human testis single-cell RNA sequencing (scRNA-seq) dataset curated and available at the human infertility single-cell testis atlas (HISTA ver. 2.9.6) (20). As previously described, the gene expression used for this manuscript is the batch-corrected normalized log counts.
Results
Disease-causing variants in RASopathy-linked genes
Nine SPGF patients and one CTRL subject in the ESTAND cohort carried heterozygous LP/P variants in seven of 22 RASopathy-linked genes (Figure 1A, Table 2). All but one variant (SPRED1 p.R325Ter) were missense substitutions. Five of 10 findings were reported in the ClinVar database as LP/P variants linked to NS or Legius syndrome, including two well-established substitutions, PTPN11 p.N308D and p.M504V [Food and Drug Administration (FDA) expert panel: P]. Three variants were novel and two were ultra-rare, not reported in the worldwide population-based Genome Aggregation Database (gnomAD). These were classified as LP according to the guidelines (16) and supported by extended patient phenotyping and family health history.
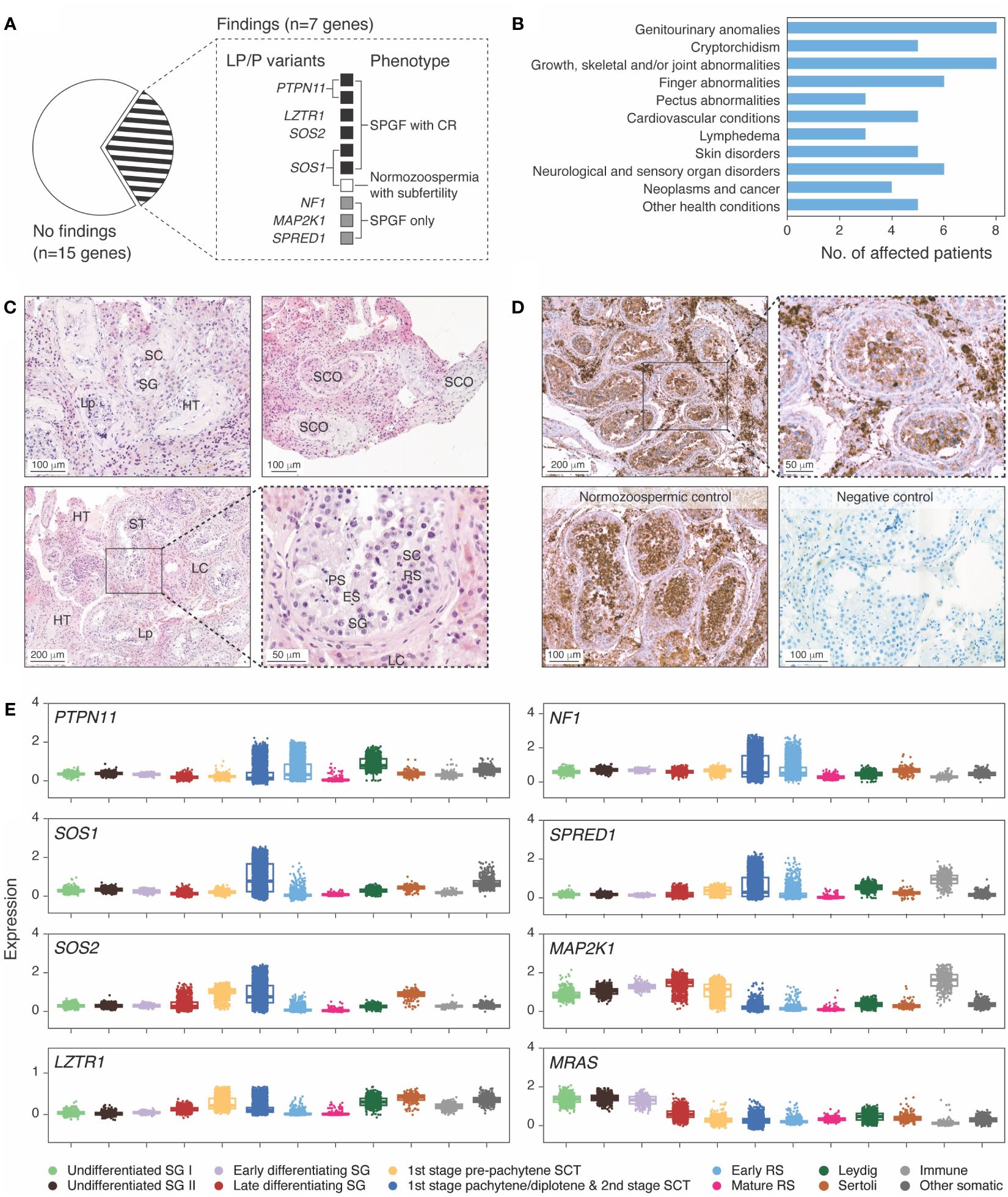
Figure 1 Ras/mitogen-activated protein kinase (MAPK) signaling pathway genes with disease-causing variants in idiopathic male infertility patients. (A) Distribution of identified likely pathogenic (LP) and pathogenic (P) variants across genes and clinical phenotypes. Spermatogenic failure (SPGF) was defined as total sperm count of 0–39 million per ejaculate (8). Cryptorchidism (CR) refers to at least one testicle missing in the scrotum at the recruitment or medical history of testicular maldescent. A subfertile normozoospermic man with SOS1 p.I437T presented low-normal sperm count (50 million per ejaculate) and needed infertility management to achieve pregnancy. (B) Prevalent health conditions in eight (of 10 total) idiopathic SPGF patients carrying LP/P variants in Ras/MAPK pathway genes available for the follow-up clinical assessment. (C) Testicular histology of Case 1 carrying PTPN11 c.922A>G (p.N308D) variant showed mixed testicular atrophy and heterogeneous morphology. Most seminiferous tubules (ST) were narrowed due to thickened lamina propria (Lp), and ~30% were completely or partially hyalinized (HT). ST with Sertoli cells (SC) and spermatogonia (SG) represented <10%, and those with Sertoli cells only (SCO) represented 20%. Maturation arrest in the primary (PS) or secondary spermatocyte stage was observed in ~10%. In the rest of ST, all spermatogenic stages were detected, but the number of round spermatids (RS) and elongated spermatids (ES) was reduced. Severe Leydig cell (LC) hyperplasia was noticeable in the stroma surrounding the tubules. Details are provided in Supplementary Table 8. (D) Immunohistochemical staining of PTPN11 in testicular tissue sections in Case 1 with the PTPN11 p.N308D substitution and in a control case with histologically normal active spermatogenesis (38 y, obstructive azoospermia). PTPN11 protein localized in the cytoplasm of spermatogenic cells, LC and SC. Staining intensity in the control case was the strongest in primary spermatocytes, early round spermatids, and LC, whereas in spermatogonia and SC, only faint PTPN11 positivity was detected. In Case 1 tissue, the strongest staining was detected in LC, consistent with the testicular histology (C). In the negative control performed without the PTPN11 antibody, no staining was detected. Brown color indicates chromogen-labeled antibody, and violet color indicates hematoxylin background staining. (E) Testicular mRNA expression of genes with identified LP/P variants in SPGF patients. Normalized log gene expression levels (Y-axis) in distinct testicular cell types were derived from the human infertility single-cell testis atlas (HISTA ver. 2.9.6) (20). The category “immune cells” includes lymphoid T cells and rare B cells as well as myeloid M1/M2 macrophages. The “other cells” category comprises endothelial and myoid cells. Each dot represents data from one cell. Boxes include 25%–75% of the data points, and the line shows median expression. SCT, spermatocytes; RS, round spermatids.
Differently from other affected RASopathy genes with the established dominant effect of LP/P variants, LZTR1 has been linked to both dominant and recessive forms of NS (24). A previously reported dominant missense substitution LZTR1 p.R283Q (25) was found in an ESTAND patient presenting CR and NOA [Sertoli cell-only syndrome (SCOS)], as well as several characteristics of NS (pectus abnormalities, heart condition, schwannomatosis, and impaired vision and hearing).
RASopathy-linked variants are overrepresented in the “SPGF with CR” subgroup
All 10 ESTAND subjects carrying LP/P variants in RASopathy-linked genes presented congenital genitourinary anomalies (Figure 1B, Table 2). Reduced TTV (<30 mL) was detected in all men except for Case 9 with a SPRED1 truncating variant. The only RASopathy-linked variant identified among the CTRL subjects was SOS1 p.I437T (ClinVar: LP) detected in a man with low-normal total sperm count (50 million per ejaculate), reduced TTV (27 mL), and subfertility requiring supportive management for several years to conceive a child. He had no documented history of cryptorchidism and was unavailable for further clinical assessment. The SOS1 p.I437T variant has been previously reported in familial and sporadic NS cases (26).
The subgroup of “SPGF with CR” showed significant, 13-fold and fivefold enrichment of undiagnosed RASopathy cases (six of 155, 3.9%) compared to men with normozoospermia (one of 323, 0.3%; Fisher’s exact test, p = 5.5 × 10−3) and “SPGF only” cases (three of 366, 0.8%; p = 0.02, Table 3). Cryptorchid SPGF patients carried LP/P variants in classical NS genes PTPN11, SOS1, SOS2, or LZTR1, and all presented extremely low total sperm count (0.0–0.9 × 106 per ejaculate). Testicular histology data were available for Case 1 with bilateral CR and NOA carrying PTPN11 p.N308D. Mixed testicular atrophy, thickened and hyalinized lamina propria of seminiferous tubules, reduced number of mature spermatids, and severe Leydig cell hyperplasia were observed (Figure 1C, Supplementary Table 8). Disease-causing variants in other Ras/MAPK pathway genes (NF1, SPRED1, and MAP2K1) were detected in patients with severe oligozoospermia without CR. These men had achieved fatherhood using their own gametes either at a young age and/or through assisted reproduction.
The spectrum of clinical manifestations in men with RASopathy-linked variants
Eight out of 10 ESTAND carriers of LP/P variants in the Ras/MAPK pathway genes were available for a follow-up assessment (Figure 1B, Table 2). Classical NS-related facial features and the highest number of congenital comorbidities were observed in patients with PTPN11 and SOS2 variants. All eight patients presented genitourinary anomalies and congenital skeletal and joint conditions. Other observed disorders affected skin, cardiac and circulatory, neurological, and sensory organ systems. Importantly, four of eight patients with follow-up data were diagnosed in early adulthood with rare forms of malignancies—schwannomatosis (LZTR1 and MAP2K1), pancreatic cancer (SOS2), and seminoma and germ cell neoplasia in situ (SOS1). Only PTPN11 p.N308D and p.M504V variant carriers presented severe postnatal growth restriction and short stature (<3rd percentile), whereas the rest of the men had reached normal height. The onset of puberty had been normal in all but the patient with PTPN11 p.M504V.
The patient carrying the SOS2 p.E9G variant presented severe developmental delay (DD) with impaired cognitive and intellectual performance (details below). The rest of the men with RASopathy-linked variants had normal intelligence and comprehension, and half of them had received higher education.
Two ESTAND patients with more than one disease-causing variant
Two patients were identified as carriers of additional LP/P variants in genes linked to other congenital conditions as likely co-contributors to their phenotype (Figure 2, Table 2).
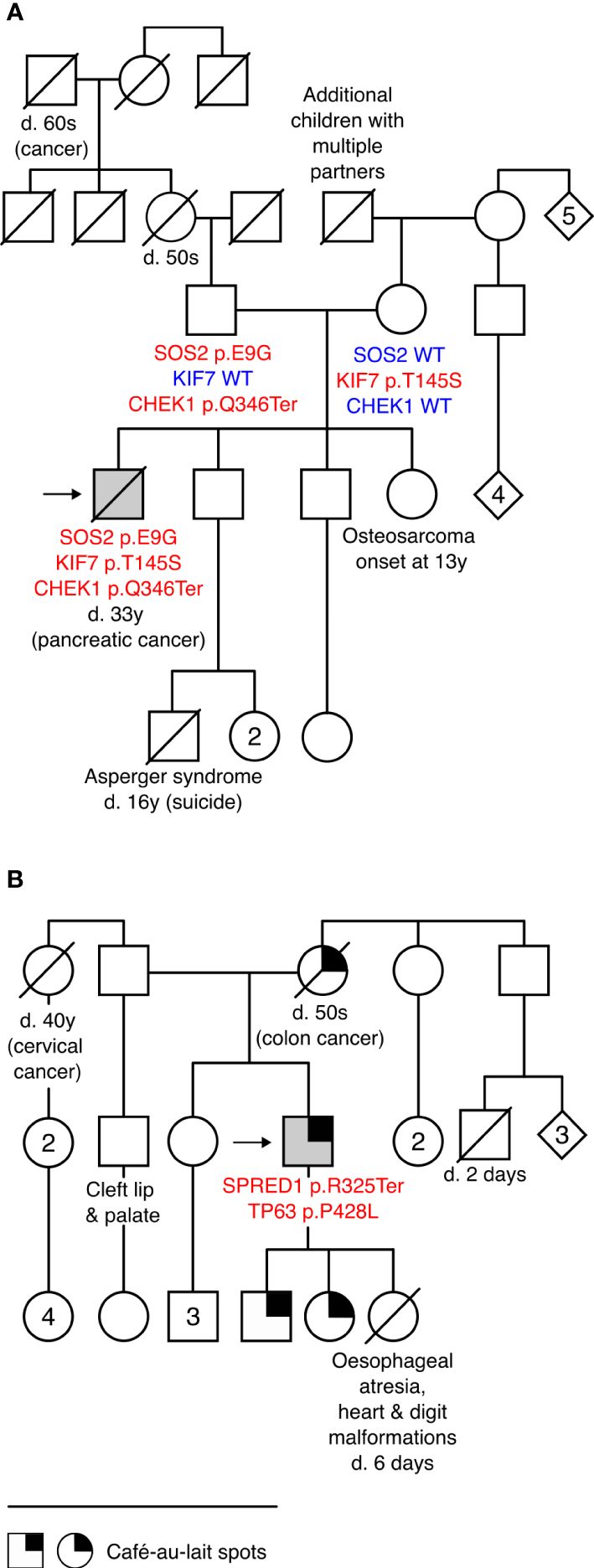
Figure 2 Patients with more than one disease-causing variant and their pedigrees. (A) Pedigree of Case 6 carrying a novel likely pathogenic (LP) variant in Noonan syndrome gene SOS2 c.26A>G (p.E9G) and incidental findings KIF7 c.434A>C (p.Y145S; LP) and CHEK1 c.1036C>T (p.Q346Ter; LP) that are likely co-contributors to the patient’s complex phenotype and comorbidities. (B) Pedigree of Case 9 carrying SPRED1 c.973C>T (p.R325Ter; pathogenic) and an incidental finding TP63 c.1283C>T (p.P428L; LP). Café-au-lait spots, characteristic of Legius syndrome, were reported in three generations. TP63 is linked to several congenital conditions, including hydronephrosis, urethral stenosis (21), and orofacial clefts (22), observed in this family. None of the family members was available for genetic testing. Detailed clinical data on Case 6 and Case 9 are provided in Table 2. d., died; y, years; WT, wild type.
The carrier of the SOS2 p.E9G (Case 6) presented typical facial features of RASopathies, gonadal dysgenesis (CR, low TTV, anejaculation, and hypogonadism), and impaired mobility and cognitive and intellectual performance. He had also been diagnosed with Lennox–Gastaut syndrome, characterized by early-onset epilepsy and pancreatic cancer that are not typically observed in SOS2-linked NS. As SOS2 p.E9G was inherited from a fertile father (andrological data unavailable), the effect on reproductive phenotype likely has incomplete penetrance. Two uncles and a granduncle of the patient’s father were childless (unavailable for genotyping). The patient carried two incidental LP findings: maternally inherited KIF7 p.Y145S and paternally derived CHEK1 p.Q346Ter. Monoallelic variants in KIF7 have been linked to various congenital phenotypes (27) that overlap with the features of Case 6 (cryptorchidism, scoliosis, epilepsy, DD/intellectual disability, characteristic facial features, etc.). Computational modeling of a potential combined effect of SOS2 and KIF7 variants supports digenic pathogenicity with a 0.83 score (99% confidence interval; Supplementary Table 9). As the patient had pancreatic cancer (died at 33 y) and his sister had osteogenic sarcoma at an early age (13 y, unavailable for genotyping), the CHEK1 p.Q346Ter is a likely candidate variant for cancer predisposition (28) in this family.
Case 9 presenting oligozoospermia carried SPRED1 p.R325Ter (primary finding) and TP63 p.P428L (incidental). The patient, his two children, and his mother (who died of colorectal cancer in her 50s) presented extensive café-au-lait macules, a common characteristic of Legius syndrome linked to SPRED1 (29, 30). However, his congenital urethral stenosis, hydronephrosis, and vertebral defects were not fully compatible with the features of this condition. The patient’s first child had developmental malformations causing perinatal mortality at 6 days of age, and his paternal half-brother had been born with a cleft lip and palate. Disease-causing variants in TP63 are a known cause of birth defects with incomplete penetrance, including hydronephrosis, urethral stenosis (21), and orofacial clefts (22). Statistical modeling of the joint effect of SPRED1 p.R325Ter and TP63 p.P428L supported potential digenic pathogenicity with a 0.94 score (99.9% confidence interval, Supplementary Table 9).
Four of seven GEMINI cases with RASopathy-linked variants presented cryptorchidism
The GEMINI cohort was used for validation of undiagnosed RASopathy cases among men with unexplained infertility. Six SPGF subjects and one fertile subject carried LP/P findings in PTPN11 (three), LZTR1 (three), and MRAS (one). Three of these variants have been classified in the ClinVar database as pathogenic, including LZTR1 p.T181RfsTer19 identified in the current study in a normozoospermic man (Figure 3). Except for hormonal parameters, no further health data were available for this subject.
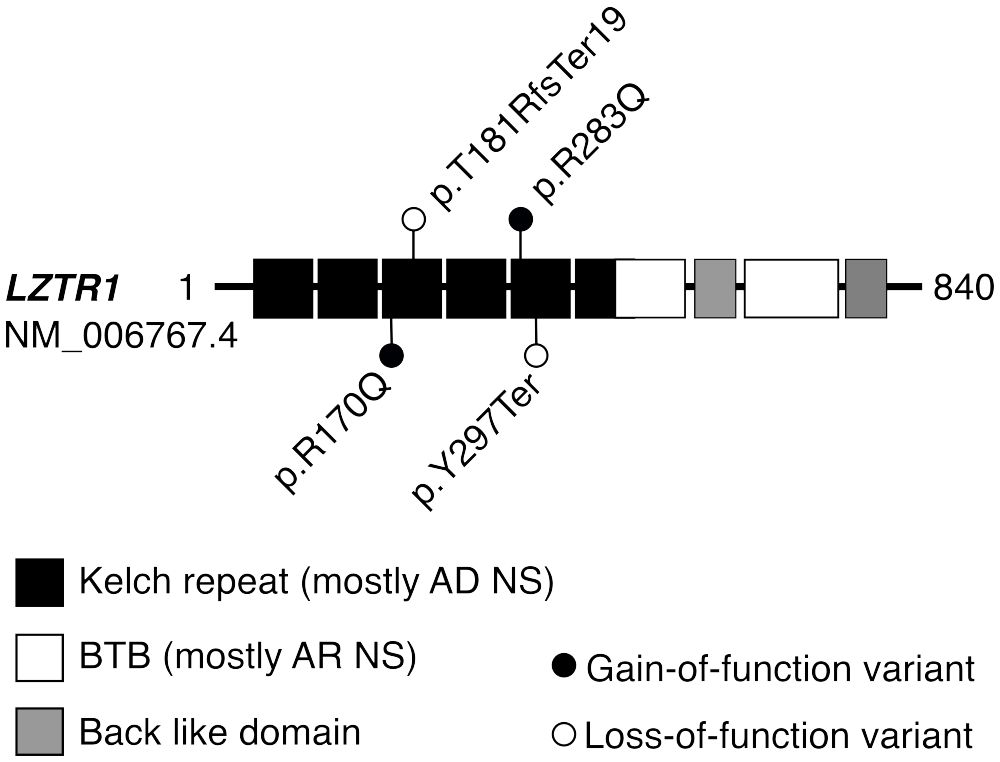
Figure 3 Protein domains of LZTR1 harboring variants linked to dominant or recessive forms of Noonan syndrome. Disease-causing variants in the LZTR1 gene are linked to both autosomal dominant (AD) and recessive (AR) forms of Noonan syndrome (NS), depending on the position of the variant within the encoded protein (24). Heterozygous LP/P variants in the N-terminal Kelch repeat domain are typically linked to AD forms of LZTR1-linked NS, whereas AR forms are mostly caused by biallelic LP/P variants within the C-terminal BTB domain. All identified LP/P findings in the LZTR1 gene were heterozygous, located in the Kelch repeat domain, and previously reported in the ClinVar database as LP/P. Previously, the detected missense substitutions p.R283Q and p.R170Q have been linked with AD form of NS (25) or schwannomatosis (24), respectively. BTB, broad complex, tram track, and bric-a-brac domain.
No statistically significant difference was detected in the prevalence of RASopathy-linked findings in the GEMINI patients (seven of 1,416; 0.5%) compared to fertile men (one of 317; 0.3%). However, four of six SPGF cases with findings had a medical history of CR (Table 4). These cases represent a substantial fraction of the minor group of GEMINI patients with undescended testicles (four of 31, 13%; Supplementary Table 6). This observation was consistent with the ESTAND data showing significant enrichment of RASopathy-linked variants in the patient subgroup “SPGF with CR” (Table 3).
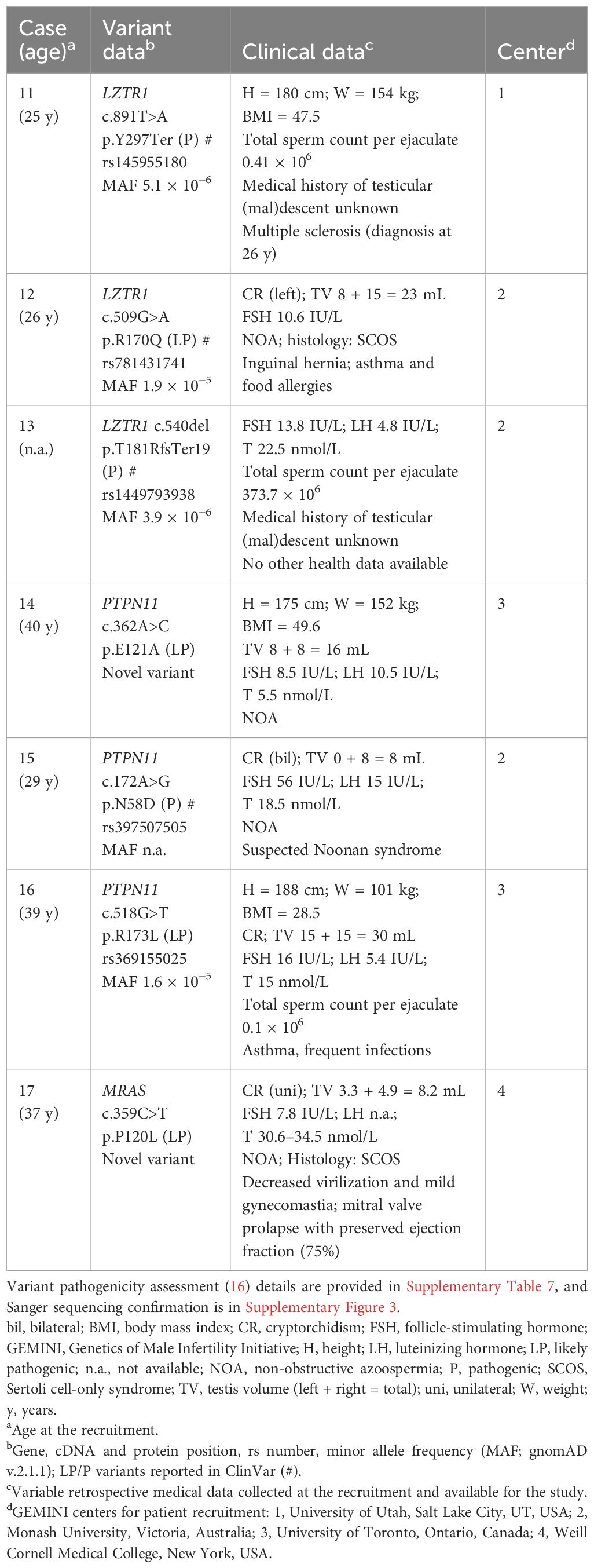
Table 4 RASopathy-linked heterozygous variants identified in men recruited in the USA, Canada, and Australian centers of the GEMINI cohort.
Notably, according to the documented medical records at the recruitment, Noonan syndrome had been previously suspected (but not confirmed by genetic testing) for the GEMINI patient carrying an established NS-linked variant PTPN11 p.N58D. The subject with MRAS p.P120L presented congenital cardiac complications, and the subject with LZTR1 p.Y297Ter presented multiple sclerosis. Both PTPN11 p.R173L and LZTR1 p.R170Q carriers had been diagnosed with asthma.
Testicular expression profile of RASopathy-linked genes
Testicular biopsy for immunohistochemical staining was available for Case 1 carrying the PTPN11 p.N308D variant and a control subject with obstructive azoospermia but histologically active spermatogenesis (Figure 1D). In both subjects, the strongest staining intensity for PTPN11 was detected in the cytoplasm of primary spermatocytes, early round spermatids, and Leydig cells. Consistent with the abnormal testicular phenotype in Case 1 (Figure 1C, Supplementary Table 8), weaker PTPN11 expression was observed in spermatogenic compared to Leydig cells.
All Ras/MAPK pathway genes with LP/P findings showed considerable mRNA expression in both spermatogenic and testicular somatic cells (Figure 1E). Most of these genes had the highest expression in spermatocytes, except for MRAS and MAP2K1 with elevated transcript levels in different stages of spermatogonia. High transcript levels of PTPN11 and SOS2 were also observed in Leydig and Sertoli cells, respectively. MAP2K1 and SPRED1 showed additionally increased expression in testicular immune cells, M1/M2 macrophages, and lymphoid T cells. Consistent with their immunomodulatory role, the respective affected patients presented dermatological conditions.
Discussion
This study showed that men with low sperm count include a considerable number of subjects with undiagnosed RASopathies. In the ESTAND cohort, disease-causing variants in seven Ras/MAPK pathway genes were identified in nine of 521 analyzed SPGF patients (1.7%) and one subfertile normozoospermic man (Figure 1). A significant, ~13-fold enrichment of carriers was identified in the “SPGF with CR” compared to the CTRL subgroup (6/155, 3.9% vs. 1/323, 0.3%; p = 5.5 × 10−3; Tables 2, 3). Of note, the incidence of RASopathies in the general population has been estimated to be 1 in 1,000 (1, 2).
Together with the findings in the GEMINI validation cohort, undiagnosed RASopathies were detected in total for 17 subjects, 15 SPGF patients, and two fertile men (Tables 2, 4). The study data strongly supported the contention that the Ras/MAPK pathway genes represent a novel etiology of syndromic SPGF, frequently accompanied by cryptorchidism (10 of 15 infertility patients with findings).
Consistent with syndromic SPGF, all affected Estonian men presented congenital genitourinary anomalies, skeletal and joint conditions, and other clinical characteristics linked to RASopathies (Figure 1B, Table 2). Importantly, rare forms of malignancies (seminoma, schwannomatosis, and pancreatic cancer) were reported in half of these cases. The observed broad phenotypic expressivity and variable penetrance of identified variants was consistent with previous studies (1–4, 26, 31–34). Recently, it has been suggested that the clinical phenotype of RASopathies may also be modulated by digenic effects (35). This study identified two ESTAND patients with more than one disease-causing variant potentially contributing to the phenotype.
The study detected heterozygous LP/P variants in eight of 22 RASopathy-linked genes. More than one finding was detected in the PTPN11 (five) and SOS1 (three) genes. PTPN11 and SOS1 are the most affected NS genes, accounting for ~50% and ~10%–15% of patients, respectively (31, 32). Surprisingly, three patients carried frequently observed variants of PTPN11-linked NS (Tables 2, 4). They presented concordant andrological phenotypes: CR and NOA. Notably, the ESTAND patients with PTPN11 p.N308D and p.M504V had all major RASopathy-linked clinical conditions (10, 11), and the GEMINI case carrying PTPN11 p.N58D had been suspected with NS during his medical assessment. All findings in the SOS1 gene were missense variants, typical of SOS1-linked NS (26). Both SPGF patients with novel SOS1 variants had CR and cryptozoospermia (Table 2). SOS1 p.Y136H carrier also presented dermatological conditions, frequently observed in NS patients (26). The patient with SOS1 p.Q214H had been diagnosed with testicular cancer. It has been shown that patients with SOS1 heterozygous variants may have a higher risk for solid tumors (26).
Four LP/P variants in the LZTR1 gene were identified in three SPGF subjects and one fertile subject (Figure 3). The cases with previously reported dominant missense substitutions LZTR1 p.R170Q (24) (GEMINI) and p.R283Q (25) (ESTAND) presented concordant andrological phenotypes: unilateral CR, NOA, and SCOS. The former patient was also suffering from schwannomatosis, characteristic of LZTR1-linked NS (34). However, no ultimate conclusions could be drawn for the phenotypic effect of heterozygous loss-of-function (LoF) variants in LZTR1, detected in one cryptozoospermia subject and one normozoospermia subject in the GEMINI cohort. Their data on testicular (mal)descent and general health conditions were unavailable, except for multiple sclerosis diagnosed as the carrier of LZTR1 p.Y297Ter. In the literature, LZTR1 LoF variants have been reported in patients with schwannomatosis, glioblastoma, and autosomal recessive NS (36, 37).
NF1 is one of the largest genes in the human genome with high genetic heterogeneity (38). The ESTAND patient with NF1 finding did not present neurofibromas and skin conditions typical of neurofibromatosis 1. However, he had been diagnosed with several orthopedic, ocular, and nervous system disorders and lymphedema, which are frequently reported in RASopathy patients (39). Rare MRAS-linked RASopathies are typically linked to congenital heart diseases (40). The GEMINI carrier of MRAS p.P120L presented CR and NOA (SCOS) and had been also diagnosed with mitral valve prolapse.
The gathered data suggested that on some occasions, congenital defects in the Ras/MAPK pathway may primarily manifest as a testicular phenotype. Others have also reported pathogenic variants in NS genes SOS1, NRAS, and BRAF in boys with isolated CR without typical characteristics of NS (41). The Ras/MAPK pathway genes with findings are highly expressed in spermatogenic and testicular somatic cells with likely contributions to testis development and function (Figures 1D, E). In men with NS, dysfunction of Sertoli and Leydig cells has been reported, reflected by altered reproductive hormone levels (42, 43).
Referring to the vital role of the Ras/MAPK pathway in mammalian development, homozygous knockout (KO) mouse models of most RASopathy genes have been reported to suffer embryonic or fetal lethality and various placental defects (44). However, heterozygous KO mouse models do not present male infertility. Exceptions are Ptpn11 KO mice for whom azoospermia, spermatogenic arrest, and seminiferous tubule degeneration have been reported (45), matching the testicular histopathology observed in Case 1 (Figure 1C, Supplementary Table 8). The irrelevance of KO mouse models to the RASopathy phenotype is explained by gain-of-function changes present on most occasions (46). Also, in this study, most of the identified variants were missense substitutions. The identified truncating variant SPRED1 p.R325Ter (Case 9) is linked to Legius syndrome, a rare RASopathy caused by inactivating mutations.
De novo variants in the Ras/MAPK pathway genes have been shown to be under positive selection, leading to clonal expansion in the germline of aging men (47). Unfortunately, parental DNA was unavailable in most cases of this study due to the sensitive and personal nature of infertility diagnosis. As CR is the most prevalent male birth defect (48), a fraction of cases likely arises due to de novo mutations in the Ras/MAPK pathway genes during spermatogenesis, representing a novel potential etiology behind sporadic CR cases.
Conclusions
This study revealed that congenital defects in the Ras/MAPK pathway genes represent a novel genetic etiology of SPGF, most likely due to affected testicular development and function. In perspective, special attention must be paid to screening RASopathy-linked variants in unexplained SPGF cases with a medical history of CR to offer optimal and timely management and counseling for reproductive and general health. Given the relationship between RASopathies and other conditions, infertile men found to have this molecular diagnosis should be evaluated for congenital genitourinary anomalies, skeletal and joint conditions, and other RASopathy-linked health concerns, including specific rare malignancies associated with this diagnosis.
Data availability statement
All relevant details of genotype-phenotype data for this study are included in the manuscript and the supplementary files. The presented data are deposited in the NCBI ClinVar database (https://www.ncbi.nlm.nih.gov/clinvar/), accession numbers: SCV004231722 - SCV004231724, SCV004231705 - SCV004231720 and SCV002525862.1.
Ethics statement
The studies involving humans were approved by Ethics Review Committee of Human Research of the University of Tartu, Estonia; IRB of University of Utah, USA; IRB of Weill Cornell Medical College, New York, USA; IRB of Mount Sinai Hospital, University of Toronto, Toronto, Canada; human ethics committees of Monash Surgical Day Hospital, Monash Medical Centre and Monash University, Australia. The studies were conducted in accordance with the local legislation and institutional requirements. The participants provided their written informed consent to participate in this study.
Author contributions
A-GJ: Formal analysis, Investigation, Writing – original draft, Writing – review & editing, Validation, Visualization. KP: Investigation, Methodology, Resources, Writing – review & editing. AD: Data curation, Formal analysis, Methodology, Writing – review & editing. ET: Investigation, Methodology, Resources, Writing – review & editing, Visualization. AV: Formal analysis, Validation, Writing – review & editing. KL: Formal analysis, Validation, Writing – review & editing. EM: Formal analysis, Software, Visualization, Writing – review & editing. STj: Writing – review & editing, Investigation. GB: Writing – review & editing, Formal analysis, Validation. VK: Writing – review & editing, Investigation, Methodology. HC-M: Writing – review & editing, Data curation. AR-E: Writing – review & editing, Investigation, Validation. LP: Writing – review & editing, Formal analysis, Validation. LN: Writing – review & editing, Data curation. OP: Writing – review & editing, Investigation. VV: Writing – review & editing, Investigation. MS: Writing – review & editing, Investigation. NV: Writing – review & editing, Methodology. SP: Writing – review & editing, Methodology. RIM: Writing – review & editing, Investigation, Funding acquisition, Resources. KAJ: Writing – review & editing, Investigation, Resources. PNS: Writing – review & editing, Investigation, Resources. STe: Writing – review & editing, Investigation. PK: Writing – review & editing, Investigation. KV-C: Writing – review & editing, Visualization. MKO'B: Funding acquisition, Resources, Writing – review & editing. KIA: Data curation, Funding acquisition, Writing – review & editing. TL: Funding acquisition, Methodology, Writing – review & editing. DFC: Data curation, Funding acquisition, Writing – review & editing. LK: Formal analysis, Investigation, Methodology, Writing – review & editing. MP: Conceptualization, Data curation, Funding acquisition, Investigation, Methodology, Project administration, Resources, Supervision, Writing – review & editing. ML: Conceptualization, Data curation, Formal analysis, Funding acquisition, Investigation, Methodology, Project administration, Resources, Supervision, Writing – original draft, Writing – review & editing.
Funding
The author(s) declare financial support was received for the research, authorship, and/or publication of this article. This work was supported by grants from the Estonian Research Council (PRG1021 to ML), the National Institutes of Health (R01HD078641 to DC and KA and P50HD096723 to DC), a Research Foundation-Flanders (F.W.O.), the National Health Research Council (APP1120356 to MO’B, RM, and DC). Infrastructure project was associated with ELIXIR Belgium (I002819N to TL) and Innoviris Joint R&D project Genome4Brussels (2020 RDIR 55b to NV and TL). SP was supported by a personal fellowship from Fonds de la Recherche Scientifique—FNRS Postdoctoral Researcher Fellowship (40005602). ML was personally supported by the Baltic-American Freedom Foundation (BAFF) Research Scholar Programme.
Acknowledgments
We would like to thank all study participants engaged in the ESTAND cohort and the clinical team at the Andrology Clinic, Tartu University Hospital for patient phenotyping and recruitment. We acknowledge the personnel of the Chair of Human Genetics at the Institute of Biomedicine and Translational Medicine, University of Tartu, for the contribution to DNA extractions and management of the ESTAND Androgenetics Biobank. Special thanks to Kaia Karja (Centre of Pathology, East Tallinn Central Hospital) for her professional assistance in immunohistochemical staining experiments. We thank Carson Holt, Steve Boyden, and the Utah Center for Genetics Discovery for assistance with the primary processing of the GEMINI WES data. We thank Brendan Houston for his assistance in retrieving data from the GEMINI cohort.
Conflict of interest
The authors declare that the research was conducted in the absence of any commercial or financial relationships that could be construed as a potential conflict of interest.
Publisher’s note
All claims expressed in this article are solely those of the authors and do not necessarily represent those of their affiliated organizations, or those of the publisher, the editors and the reviewers. Any product that may be evaluated in this article, or claim that may be made by its manufacturer, is not guaranteed or endorsed by the publisher.
Supplementary material
The Supplementary Material for this article can be found online at: https://www.frontiersin.org/articles/10.3389/fendo.2024.1312357/full#supplementary-material
References
1. Tartaglia M, Aoki Y, Gelb BD. The molecular genetics of RASopathies: An update on novel disease genes and new disorders. Am J Med Genet C Semin Med Genet. (2022) 190:425–39. doi: 10.1002/ajmg.c.32012
2. Zenker M. Clinical overview on RASopathies. Am J Med Genet C Semin Med Genet. (2022) 190:414–24. doi: 10.1002/ajmg.c.32015
3. Sharland M, Burch M, McKenna WM, Paton MA. A clinical study of Noonan syndrome. Arch Dis Child. (1992) 67:178–83. doi: 10.1136/adc.67.2.178
4. Zenker M, Edouard T, Blair JC, Cappa M. Noonan syndrome: improving recognition and diagnosis. Arch Dis Child. (2022) 107:1073–8. doi: 10.1136/archdischild-2021-322858
5. Punab M, Poolamets O, Paju P, Vihljajev V, Pomm K, Ladva R, et al. Causes of male infertility: a 9-year prospective monocentre study on 1737 patients with reduced total sperm counts. Hum Reprod. (2017) 32:18–31. doi: 10.1093/humrep/dew284
6. Elamo HP, Virtanen HE, Toppari J. Genetics of cryptorchidism and testicular regression. Best Pract Res Clin Endocrinol Metab. (2022) 36:101619. doi: 10.1016/j.beem.2022.101619
7. Laan M, Kasak L, Timinskas K, Grigorova M, Venclovas Č, Renaux A, et al. NR5A1 c.991-1G > C splice-site variant causes familial 46,XY partial gonadal dysgenesis with incomplete penetrance. Clin Endocrinol (Oxf). (2021) 94:656–66. doi: 10.1111/cen.14381
8. World Health Organization. WHO laboratory manual for the examination and processing of human semen, Sixth Edition. Geneva: World Health Organization. (2021).
9. Ehala-Aleksejev K, Punab M. Relationships between total testicular volume, reproductive parameters and surrogate measures of adiposity in men presenting for couple’s infertility. Andrologia. (2017) 50:e12952. doi: 10.1111/and.12952
10. Romano AA, Allanson JE, Dahlgren J, Gelb BD, Hall B, Pierpont ME, et al. Noonan syndrome: clinical features, diagnosis, and management guidelines. Pediatrics. (2010) 126:746–59. doi: 10.1542/peds.2009-3207
12. Kasak L, Rull K, Yang T, Roden DM, Laan M. Recurrent pregnancy loss and concealed long-QT syndrome. J Am Heart Assoc. (2021) 10:e021236. doi: 10.1161/JAHA.121.021236
13. Nagirnaja L, Lopes AM, Charng WL, Miller B, Stakaitis R, Golubickaite I, et al. Diverse monogenic subforms of human spermatogenic failure. Nat Commun. (2022) 13:7953. doi: 10.1038/s41467-022-35661-z
14. Kasak L, Lillepea K, Nagirnaja L, Aston KI, Schlegel PN, Gonçalves J, et al. Actionable secondary findings following exome sequencing of 836 non-obstructive azoospermia cases and their value in patient management. Hum Reprod. (2022) 37:1652–63. doi: 10.1093/humrep/deac100
15. McLaren W, Gil L, Hunt SE, Riat HS, Ritchie GR, Thormann A, et al. The ensembl variant effect predictor. Genome Biol. (2016) 17:122. doi: 10.1186/s13059-016-0974-4
16. Gelb BD, Cavé H, Dillon MW, Gripp KW, Lee JA, Mason-Suares H, et al. ClinGen’s RASopathy Expert Panel consensus methods for variant interpretation. Genet Med. (2018) 20:1334–45. doi: 10.1038/gim.2018.3
17. Renaux A, Papadimitriou S, Versbraegen N, Nachtegael C, Boutry S, Nowé A, et al. ORVAL: a novel platform for the prediction and exploration of disease-causing oligogenic variant combinations. Nucleic Acids Res. (2019) 47:W93–8. doi: 10.1093/nar/gkz437
18. Versbraegen N, Gravel B, Nachtegael C, Renaux A, Verkinderen E, Nowé A, et al. Faster and more accurate pathogenic combination predictions with VarCoPP2.0. BMC Bioinf. (2023) 24:179. doi: 10.1186/s12859-023-05291-3
19. McLachlan RI, Rajpert-De Meyts E, Hoei-Hansen CE, de Kretser DM, Skakkebaek NE. Histological evaluation of the human testis–approaches to optimizing the clinical value of the assessment: mini review. Hum Reprod. (2007) 22:2–16. doi: 10.1093/humrep/del279
20. Mahyari E, Guo J, Lima AC, Lewinsohn DP, Stendahl AM, Vigh-Conrad KA, et al. Comparative single-cell analysis of biopsies clarifies pathogenic mechanisms in Klinefelter syndrome. Am J Hum Genet. (2021) 108:1924–45. doi: 10.1016/j.ajhg.2021.09.001
21. Hyder Z, Beale V, O’Connor R, Clayton-Smith J. Genitourinary malformations: an under-recognized feature of ectrodactyly, ectodermal dysplasia and cleft lip/palate syndrome. Clin Dysmorphol. (2017) 26:78–82. doi: 10.1097/MCD.0000000000000172
22. Rinne T, Bolat E, Meijer R, Scheffer H, van Bokhoven H. Spectrum of p63 mutations in a selected patient cohort affected with ankyloblepharon-ectodermal defects-cleft lip/palate syndrome (AEC). Am J Med Genet A. (2009) 149A:1948–51. doi: 10.1002/ajmg.a.32793
23. Richards S, Aziz N, Bale S, Bick D, Das S, Gastier-Foster J, et al. Standards and guidelines for the interpretation of sequence variants: a joint consensus recommendation of the American College of Medical Genetics and Genomics and the Association for Molecular Pathology. Genet Med. (2015) 17:405–24. doi: 10.1038/gim.2015.30
24. Motta M, Fidan M, Bellacchio E, Pantaleoni F, Schneider-Heieck K, Coppola S, et al. Dominant Noonan syndrome-causing LZTR1 mutations specifically affect the Kelch domain substrate-recognition surface and enhance RAS-MAPK signaling. Hum Mol Genet. (2019) 28:1007–22. doi: 10.1093/hmg/ddy41240
25. Umeki I, Niihori T, Abe T, Kanno SI, Okamoto N, Mizuno S, et al. Delineation of LZTR1 mutation-positive patients with Noonan syndrome and identification of LZTR1 binding to RAF1-PPP1CB complexes. Hum Genet. (2019) 138:21–35. doi: 10.1007/s00439-018-1951-7
26. Lepri F, De Luca A, Stella L, Rossi C, Baldassarre G, Pantaleoni F, et al. SOS1 mutations in noonan syndrome: molecular spectrum, structural insights on pathogenic effects, and genotype–phenotype correlations. Hum Mutat. (2011) 32:760–72. doi: 10.1002/humu.21492
27. Putoux A, Thomas S, Coene KL, Davis EE, Alanay Y, Ogur G, et al. KIF7 mutations cause fetal hydrolethalus and acrocallosal syndromes. Nat Genet. (2011) 43:601–6. doi: 10.1038/ng.826
28. Klomp JE, Lee YS, Goodwin CM, Papke B, Klomp JA, Waters AM, et al. CHK1 protects oncogenic KRAS-expressing cells from DNA damage and is a target for pancreatic cancer treatment. Cell Rep. (2021) 37:110060. doi: 10.1016/j.celrep.2021.110060
29. Brems H, Chmara M, Sahbatou M, Denayer E, Taniguchi K, Kato R, et al. Germline loss-of-function mutations in SPRED1 cause a neurofibromatosis 1-like phenotype. Nat Genet. (2007) 39:1120–6. doi: 10.1038/ng2113
30. Messiaen L, Yao S, Brems H, Callens T, Sathienkijkanchai A, Denayer E, et al. Clinical and mutational spectrum of neurofibromatosis type 1-like syndrome. JAMA. (2009) 302:2111–8. doi: 10.1001/jama.2009.1663
31. Tartaglia M, Kalidas K, Shaw A, Song X, Musat DL, van der Burgt I, et al. PTPN11 mutations in noonan syndrome: molecular spectrum, genotype-phenotype correlation, and phenotypic heterogeneity. Am J Hum Genet. (2002) 70:1555–63. doi: 10.1086/340847
32. Roberts AE, Araki T, Swanson KD, Montgomery KT, Schiripo TA, Joshi VA, et al. Germline gain-of-function mutations in SOS1 cause Noonan syndrome. Nat Genet. (2007) 39:70–4. doi: 10.1038/ng1926
33. Athota JP, Bhat M, Nampoothiri S, Gowrishankar K, Narayanachar SG, Puttamallesh V, et al. Molecular and clinical studies in 107 Noonan syndrome affected individuals with PTPN11 mutations. BMC Med Genet. (2020) 21:50. doi: 10.1186/s12881-020-0986-5
34. Dhamija R, Plotkin S, Gomes A, Babovic-Vuksanovic D. LZTR1- and SMARCB1-related schwannomatosis. In: Adam MP, Feldman J, Mirzaa GM, Pagon RA, Wallace SE, Bean LJH, et al, editors. GeneReviews® [Internet]. University of Washington, Seattle, Seattle (WA) (2018).
35. Ferrari L, Mangano E, Bonati MT, Monterosso I, Capitanio D, Chiappori F, et al. Digenic inheritance of subclinical variants in Noonan Syndrome patients: an alternative pathogenic model? Eur J Hum Genet. (2020) 28:1432–45. doi: 10.1038/s41431-020-0658-0
36. Johnston JJ, van der Smagt JJ, Rosenfeld JA, Pagnamenta AT, Alswaid A, Baker EH, et al. Autosomal recessive Noonan syndrome associated with biallelic LZTR1 variants. Genet Med. (2018) 20:1175–85. doi: 10.1038/gim.2017.249
37. Deiller C, Van-Gils J, Zordan C, Tinat J, Loiseau H, Fabre T, et al. Coexistence of schwannomatosis and glioblastoma in two families. Eur J Med Genet. (2019) 62:103680. doi: 10.1016/j.ejmg.2019.103680
38. Napolitano F, Dell'Aquila M, Terracciano C, Franzese G, Gentile MT, Piluso G, et al. Genotype-phenotype correlations in neurofibromatosis type 1: identification of novel and recurrent NF1 gene variants and correlations with neurocognitive phenotype. Genes (Basel). (2022) 13:1130. doi: 10.3390/genes13071130
39. Rauen KA. The RASopathies. Annu Rev Genomics Hum Genet. (2013) 14:355–69. doi: 10.1146/annurev-genom-091212-153523
40. Pires LVL, Bordim RA, Maciel MBR, Tanaka ACS, Yamamoto GL, Honjo RS, et al. Atypical, severe hypertrophic cardiomyopathy in a newborn presenting Noonan syndrome harboring a recurrent heterozygous MRAS variant. Am J Med Genet A. (2021) 185:3099–103. doi: 10.1002/ajmg.a.62376
41. Rodríguez F, Vallejos C, Ponce D, Unanue N, Hernández MI, Célis S, et al. Study of Ras/MAPK pathway gene variants in Chilean patients with Cryptorchidism. Andrology. (2018) 6:579–84. doi: 10.1111/andr.12501
42. Moniez S, Pienkowski C, Lepage B, Hamdi S, Daudin M, Oliver I, et al. Noonan syndrome males display Sertoli cell-specific primary testicular insufficiency. Eur J Endocrinol. (2018) 179:409–18. doi: 10.1530/EJE-18-0582
43. Ankarberg-Lindgren C, Westphal O, Dahlgren J. Testicular size development and reproductive hormones in boys and adult males with Noonan syndrome: a longitudinal study. Eur J Endocrinol. (2011) 165:137–44. doi: 10.1530/EJE-11-0092
44. Hebron KE, Hernandez ER, Yohe ME. The RASopathies: from pathogenetics to therapeutics. Dis Model Mech. (2022) 15:dmm049107. doi: 10.1242/dmm.049107
45. Li Y, Liu WS, Yi J, Kong SB, Ding JC, Zhao YN, et al. The role of tyrosine phosphatase Shp2 in spermatogonial differentiation and spermatocyte meiosis. Asian J Androl. (2020) 22:79–87. doi: 10.4103/aja.aja_49_19
46. Riller Q, Rieux-Laucat F. RASopathies: From germline mutations to somatic and multigenic diseases. BioMed J. (2021) 44:422–32. doi: 10.1016/j.bj.2021.06.004
47. Maher GJ, Ralph HK, Ding Z, Koelling N, Mlcochova H, Giannoulatou E, et al. Selfish mutations dysregulating RAS-MAPK signaling are pervasive in aged human testes. Genome Res. (2018) 28:1779–90. doi: 10.1101/gr.239186.118
Keywords: cryptorchidism, syndromic male infertility, exome sequencing, RAS/MAPK pathway, molecular diagnosis, cancer, congenital testicular dysgenesis, multidisciplinary management
Citation: Juchnewitsch A-G, Pomm K, Dutta A, Tamp E, Valkna A, Lillepea K, Mahyari E, Tjagur S, Belova G, Kübarsepp V, Castillo-Madeen H, Riera-Escamilla A, Põlluaas L, Nagirnaja L, Poolamets O, Vihljajev V, Sütt M, Versbraegen N, Papadimitriou S, McLachlan RI, Jarvi KA, Schlegel PN, Tennisberg S, Korrovits P, Vigh-Conrad K, O’Bryan MK, Aston KI, Lenaerts T, Conrad DF, Kasak L, Punab M and Laan M (2024) Undiagnosed RASopathies in infertile men. Front. Endocrinol. 15:1312357. doi: 10.3389/fendo.2024.1312357
Received: 10 October 2023; Accepted: 11 March 2024;
Published: 09 April 2024.
Edited by:
Katharina Maria Main, Rigshospitalet, DenmarkCopyright © 2024 Juchnewitsch, Pomm, Dutta, Tamp, Valkna, Lillepea, Mahyari, Tjagur, Belova, Kübarsepp, Castillo-Madeen, Riera-Escamilla, Põlluaas, Nagirnaja, Poolamets, Vihljajev, Sütt, Versbraegen, Papadimitriou, McLachlan, Jarvi, Schlegel, Tennisberg, Korrovits, Vigh-Conrad, O’Bryan, Aston, Lenaerts, Conrad, Kasak, Punab and Laan. This is an open-access article distributed under the terms of the Creative Commons Attribution License (CC BY). The use, distribution or reproduction in other forums is permitted, provided the original author(s) and the copyright owner(s) are credited and that the original publication in this journal is cited, in accordance with accepted academic practice. No use, distribution or reproduction is permitted which does not comply with these terms.
*Correspondence: Maris Laan, bWFyaXMubGFhbkB1dC5lZQ==
†These authors have contributed equally to this work and share last authorship