- 1Univ. Grenoble Alpes, INSERM U1055, Pharmacy Department, Grenoble Alpes University Hospital, Laboratory of Fundamental and Applied Bioenergetics, Grenoble, France
- 2Univ. Grenoble Alpes, CEA-Leti, Clinatec, Grenoble, France
- 3Univ. Grenoble Alpes, INSERM U1055, Diabetology and Endocrinology Department, Grenoble Alpes University Hospital, Laboratory of Fundamental and Applied Bioenergetics, Grenoble, France
Introduction: Diabetes is a global health concern characterized by chronic hyperglycemia resulting from insulinopenia and/or insulin resistance. The rising prevalence of diabetes and its associated complications (ulcers, periodontitis, healing of bone defect, neuropathy, retinopathy, cardiopathy and nephropathy) necessitate innovative therapeutic approaches. Photobiomodulation (PBM), involves exposing tissues and cells to low-energy light radiation, leading to biological effects, largely via mitochondrial activation.
Methods: This review evaluates preclinical and clinical studies exploring the potential of PBM in diabetes and its complications, as well all clinical trials, both planned and completed, available on ClinicalTrials database.
Results: This review highlights the variability in PBM parameters across studies, hindering consensus on optimal protocols. Standardization of treatment parameters and rigorous clinical trials are needed to unlock PBM’s full therapeutic potential. 87 clinical trials were identified that investigated PBM in diabetes mellitus (with 5,837 patients planned to be treated with PBM). Clinical trials assessing PBM effects on diabetic neuropathy revealed pain reduction and potential quality of life improvement. Studies focusing on wound healing indicated encouraging results, with PBM enhancing angiogenesis, fibroblast proliferation, and collagen density. PBM’s impact on diabetic retinopathy remains inconclusive however, requiring further investigation. In glycemic control, PBM exhibits positive effects on metabolic parameters, including glucose tolerance and insulin resistance.
Conclusion: Clinical studies have reported PBM-induced reductions in fasting and postprandial glycemia without an increased hypoglycemic risk. This impact of PBM may be related to its effects on the beta cells and islets in the pancreas. Notwithstanding challenges, PBM emerges as a promising adjunctive therapy for managing diabetic neuropathy, wound healing, and glycemic control. Further investigation into its impact on diabetic retinopathy and muscle recovery is warranted.
1 Introduction
Diabetes is characterized by chronic hyperglycemia due to insulinopenia [type 1 diabetes (T1D)] and/or insulin resistance [type 2 diabetes (T2D)]. The International Diabetes Federation reported 537 million of potential cases of diabetes across the world in 2021 with an increment planned for 2045 at 783 million of potential cases (1). As a result, diabetes caused 6.7 million of death in 2021 (1) and led to USD 966 billion health expenditures (1) partially due to numerous complications related to diabetes disease (2) such as macrovascular complication (ischemic cardiomyopathy, stroke and arteriopathy) (2–5) and microvascular complications: 1) retinopathy, diabetes is the first cause of non-traumatic blindness (6), 2) nephropathy, diabetes is the first cause of dialysis, 3) amputation and 4) neuropathy (7, 8) leading to foot ulceration and exposing patient to a risk of lower limb amputation [diabetes is the first cause of non-traumatic amputation (9)]. Finally, patients living with diabetes are also exposed to a risk of periodontitis (10). Altogether, diabetic complications alter quality of life (11, 12). These complications can be prevented through optimal glycemic control and could be managed with some medicines (13, 14). However, despite optimal medical management, prevention of diabetic complications remains a challenge and additional treatment remains mandatory.
Light was used as potential of treatment since the ancient Egypt. The biological reaction to light and its therapeutic applications are not new. For example, the beneficial effect of light on neonatal jaundice, discovered in the 1950s, made phototherapy (with blue light with a wavelength between 420-490 nm) the main modality for its treatment (15). Another example, the effects of light on mood, demonstrated in the 80s, made it possible to propose light therapy as a treatment for seasonal affective disorders, and it has recently been shown to have an effect comparable to antidepressants in episodes major depression (16).
Photobiomodulation therapy (PBM), formerly called “Low level laser therapy”, is a phototherapy based on the exposure of tissues and cells to non-ionizing and very weak light radiation with a wavelength generally ranging from red (between 600-700 nm) to the near infrared (between 700-1400 nm) and resulting in biological effects following its absorption by endogenous chromophores. Historically, PBM was first described by Endre Mester in 1968 who observed faster hair regrowth in rodents exposed to a low-energy laser with a wavelength of 694 nm (17, 18). In the same years, it was also developed by the National Aeronautics and Spatial Administration (NASA) to accelerate the healing and regeneration of muscle cells in astronauts (19). For the past decades, biomedical research relating to PBM has been constantly increasing, indicating a growing interest in its therapeutic potential. Interest in PBM has also been linked to technical developments in illumination technology, with the improvement of LEDs, which are cheaper, safer and give off less heat than lasers (20). Depending on the targeted use, and the illumination device, PBM can be brought along white light, to have the full spectrum of wavelength as a natural light, or along LED to obtain mainly a light targeted around a wavelength, or a laser to deliver only a define wavelength. At the beginning of the 2000s, the use of PBM in aesthetics (hair regrowth, wrinkle reduction) and sports recovery helped to democratize its use. Photobiomodulation is now used to help heal damaged tissues, improve immune response, reduce inflammation, and was recommended to prevent or treat certain side effects of treatments such as chemotherapy and radiotherapy (mucositis and radiodermatitis). In 2010, the first clinical authorization was reached as therapeutics for pain in conditions such as osteoarthritis. Since the 2020s, based on successful preclinical researches, various clinical trials have been initiated to evaluate PBM as a treatment to slow down neurodegenerative diseases, such as Alzheimer’s or Parkinson’s diseases (21, 22). Over the past three years (2020–2022), approximately 850 articles per year have been published and referenced in MEDLINE.
The main mechanism of action involves the mitochondria (23), possessing photo-acceptors sensitive to the lengths used with PBM. Briefly, PBM has been reported to activate non-mitochondrial cellular functions (light/heat-gated ion channels) and restore mitochondrial function (through interfacial water and/or activation of cytochrome C oxidase), resulting in a short-term increase adenosine triphosphate (ATP) energy production in body cells and increased production of NO. This process leads to long-term effects, with the expression of various stimulatory and protective genes. The main biological effects highlighted in preclinical and clinical studies are an anti-inflammatory, analgesic action, an increase in blood circulation, angiogenesis, and a healing/regeneration and tissue proliferation action (24–26). Given these effects, the potential therapeutic applications are numerous. PBM is already used in certain medical disciplines. It is part, for example, of the recommendations for the prevention of mucositis in patients treated by radiotherapy.
The use of PBM in the context of neurodegenerative diseases, and in particular Parkinson’s disease, is currently being studied and is the subject of clinical trials. PBM could represent an innovative therapeutic solution, to slow down the neurodegenerative process. The preclinical results in this direction are very encouraging, and clinical data should be published soon due to ongoing clinical trials. The metabolic syndrome in neurodegenerative diseases, and in particular Parkinson’s disease, are well established (21, 22). The observed effect of PBM on cellular metabolism, inflammatory and scarring processes is a lead that may indicate an interest of PBM in the regulation of phenomena related to metabolic syndromes, such as diabetes.
In the present work, the purpose is to review the clinical studies using PBM and conducted in the field of the treatment of diabetes and diabetic complications.
2 Materials and methods
Identification was made regarding:
- Pre-clinical (animal study) and clinical data available until 23 May 2023
- Clinical trials available on clinicaltrials.gov (27) until 23 May 2023
2.1 Searching strategy and selection criteria of papers
A MEDLINE research was conducted via PubMed using the search terms: [(Photobiomodulation) or (Low-level laser therapy) or (Near-infrared therapy)] AND (diabetes or diabetic or T1D or T2D) AND (1900/01/01:2023/05/23[edat]). In addition, references from cited papers were investigated. For each paper, the following parameters were recorded: 1st author, year of publication, model used (including the number of patients in clinical trials), wavelength, light source (LED or laser), mode of administration (continuous or pulsed), PBM parameters (power density in mW/cm², time per exposure, energy density in J/cm², frequency, sites), and the study’s conclusion. The data were analyzed both collectively and individually, considering different diabetes conditions such as retinopathy, ulcers, and periodontitis. In the case of clinical trial papers, it was specified whether they were randomized clinical trials (RCTs), pre-post interventional trials (Pre-Post ITV) or observational studies.
2.2 Searching strategy for clinical trials and classification
The screening of the ClinicalTrials database (27) was conducted using three terms: photobiomodulation, low-level laser therapy and near-infrared therapy. Only trials related to diabetes were considered eligible, and studies employing methods other than PBM were excluded (Figure 1). For each included trial, the following parameters were recorded: starting date, country of the sponsor, expected number of enrolled patients, and enrolment status (not yet recruiting, recruiting, enrolling by invitation, active, suspended, terminated, completed, withdrawn, or unknown). Regarding clinical trials published in the Medline database, the following parameters were recorded: country of the sponsor and number of enrolled patients. All these trials were classified as completed.
3 Results
Following Medline research, 124 articles met the search criteria. 170 different exposure times were studied and varied widely, with 35% of the studies (60/168) having exposure times (for one session) of less than a minute and 35% (59/168) exceeding 5 min (Figure 2). Laser was the predominant light source investigated in 80% of the studies (Figure 2), and continuous exposure was the primary mode of administration (84% of studies, Figure 2). The power density values ranged from 1 mW/cm² to 8.32 W/cm², whereas the energy density ranged from 0.03 to 420 J/cm². These studies investigated various wavelengths ranging from 425 to 1064 nm, with 82% of studies between 600 and 900 nm, and six studies exploring multiple wavelengths applying simultaneously (Figure 2). It should be noted that these parameters were not fully described or available in 37 studies (29%).
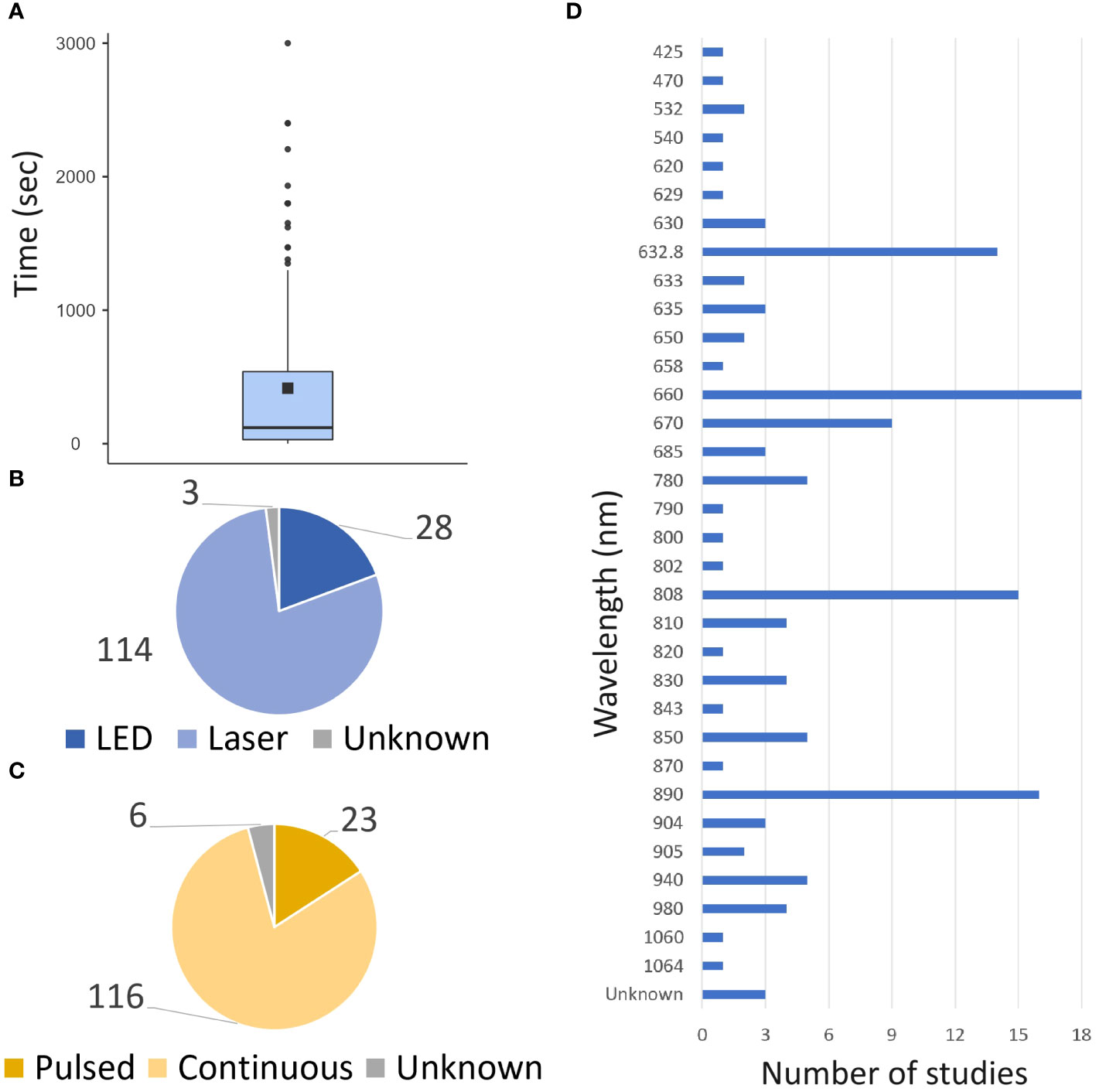
Figure 2 Global PBM parameters. The data from both preclinical and clinical studies are presented. (A) Repartition of time exposure to PBM. (B) Repartition of source of light uses. (C) repartition of the mode of illumination uses. (D) Repartition of wavelength uses.
3.1 Clinical trials in humans
42 trials were identified in ClinicalTrial database (27), and 56 articles were found in Medline. After removing duplicate trials, 87 were conducted or planned (Figure 2). These trials included 5,837 patients (see Table 1 for details). The top three countries planning to conduct trials on PBM and diabetes were the United States (n = 19), Brazil (n = 18), and India (n = 10, Figure 3). 50% of publications were by completely independent teams (n = 21 articles). Regarding diabetic neuropathies, two teams each published three studies: Burke et al. (28–30), and Arun G et al. (31–33). Regarding diabetes chronic periodontitis, three teams each published 2-3 studies: Chava et al. (34, 35), Haaki et al. (36, 37), and Obradovic et al. (38–40). Regarding performance and functionality during or after exercise in patient with diabetes Ferraresi et al. (41–43). published three studies. Finally, two teams have published on neuropathic pain and diabetic ulcers: Arisawa et al. (44, 45), and Schindl et al. (46–48).
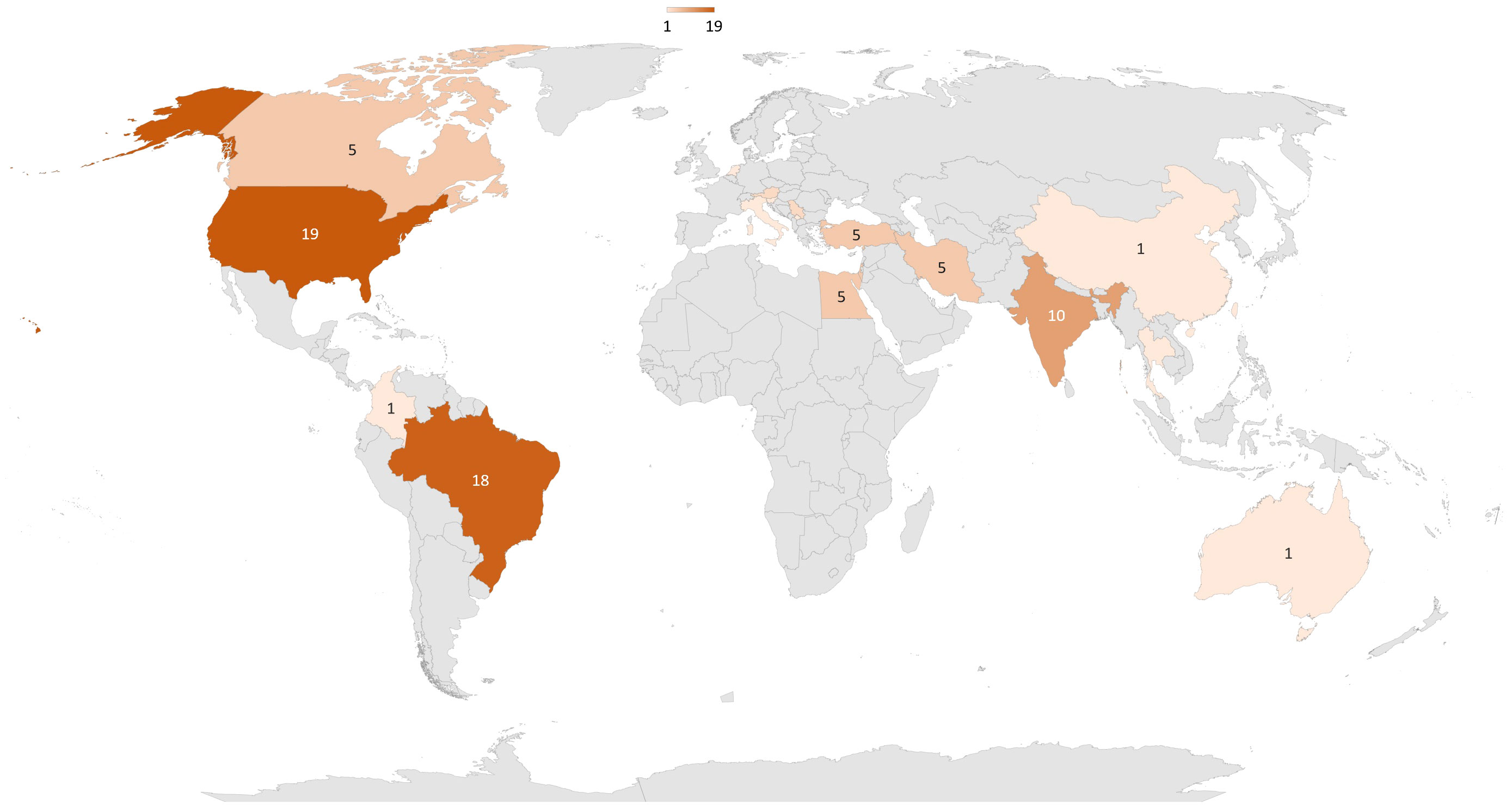
Figure 3 Repartition around the world of clinical trials about PBM and diabetes.87 trials have been conducted or are planned, 43 in America, 26 in Asia, 12 in Europe, 5 in Africa, and 1 in Oceania.
3.2 Impact of PBM on diabetic neuropathy
A total of 23 studies were conducted, including 5 on preclinical models- (49–53) and 18 in humans (28–33, 46, 54–63) (Table 2).
3.2.1 Pain investigation
Among 14 studies investigating pain, 11 reported a decrease in pain after PBM, with 4 studies conducted on preclinical models and 7 on clinical subjects. In clinical trials, pain was evaluated using a visual analogic scale (VAS), either alone or in combination with other pain assessment scores with a follow-up period ranging from 4 to 90 days. For pre-post interventional trials (n = 3), the VAS scores decreased by an average of 2.3 to 5.2 points after PBM. In RCTs (n = 4), the VAS scores decreased more in the PBM group than in the control group (0 to 3 points vs. 3 to 5 points after 10 days) (44, 54), and the VAS scores at the end of the follow-up period were lower in the PBM group than in the control group (6.9-7.9 vs. 5.9-5.3) (33, 56).
3.2.2 QoL investigation
Four studies investigated the effect of PBM on QoL. Two studies reported a positive impact of PBM on QoL associated with a decrease in pain (44, 54). Conversely, 2 studies did not report an impact of PBM on QoL but in these 2 studies PBM did not induced a decrease in pain (58, 59). The first study (58) used the highest power (350W), and the second study (59) used the longest exposure time (40 min) and application frequency (once a day for 90 days).
3.3 Impact of PBM on diabetic retinopathy
A total of 6 studies were conducted, including 4 studies on preclinical models (64–67) and 2 studies in humans (68, 69) (Table 3). Preclinical studies consistently reported a positive effect of PBM on retinal structure associated with a decrease in oxidative stress. In clinical studies focusing on macular oedema, findings were divergent regarding improvement of visual acuity between the two identified studies (68, 69). However, no side effects were reported in either study.
3.4 Impact of PBM on glucose metabolism in T2D
A total of 9 studies were conducted, with 8 studies conducted on preclinical models (70–78) and one study in humans (79) (Table 4). The preclinical studies consistently reported positive effects of PBM on metabolic parameters with an improvement in glucose tolerance [with a glucose tolerance test area under the curve (GTT AUC) decreasing by 12–28%], a decrease in insulin resistance [with a 22% decrease in homeostatic model assessment of insulin resistance (HOMA-IR (70)) and a 10% decrease in insulin tolerance test (ITT) AUC (72)] and a decrease in fasting glycemia. PBM also showed positive effects on lipid profiles with a reduction in free fatty acid, triglycerides and cholesterol levels. In the clinical study (79), PBM applied on eight muscles in patients with T2D resulted in a decrease in glycemia (fasting and 1h post-prandial glucose) observed 30 min to 12 h after PBM treatment. This decrease was evidenced by a reduction in the GTT AUC by 37% and a reduction of 1h postprandial glucose by 16%. Importantly, no additive effect of hypoglycemic treatment was observed and no hypoglycemia were reported.
3.5 Impact of PBM on exercises and muscles in T2D
A total of 7 studies were conducted, including 3 studies on preclinical models (41, 80, 81) and 4 studies in humans (42, 43, 82, 83) (Table 5). In preclinical models, PBM has been demonstrated to have a positive impact on biochemical parameters, such as a decrease in oxidative stress and an increase in antioxidant activity. However, in clinical studies focusing on muscular performance and functionality during or after exercise, PBM has failed to show any improvement. No significant effects on the muscular performance were observed.
3.6 Impact of PBM on healing process
3.6.1 Wound healing
A total of 44 studies were conducted to evaluate impact of PBM on wound healing, including 31 studies on preclinical models (84–114) and 13 studies on humans (45, 47, 48, 115–124) (Table 6). In preclinical models, PBM had a predominantly positive effect on wound healing in 94% of studies. PBM improved various aspects of wound healing, including collagen density, fibroblast proliferation, angiogenesis, granulation tissue formation, and epithelialization. These effects were often accompanied by a decrease inflammatory marker. Only one study, which poorly described PBM parameters, did not report a positive effect of PBM (104). Most studies utilized wavelengths in the red to near-infrared spectrum, whereas studies investigating green wavelengths did not report positive effects of PBM (84, 94). In clinical studies, the majority (92%) reported a positive effect of PBM on chronic ulcers healing. Among the 12 RCTs, there was an increase in wound closure ranging from 15% to 47.3% in the control group compared to 37% to 90.8% in the PBM group. Consequently, the PBM groups had smaller wound areas compared to the control groups, with measurements of 2.39 cm² vs. 8.43 cm² (120), indicating a decrease in wound area of approximately 3.2 cm² vs. 10.4 cm² (122). The only study that did not report a positive effect of PBM utilized the shortest exposure time (less than one second) (121). Two studies specified that PBM did not have any reported side effects (115, 117) while other studies did not explicitly mention it.
3.6.2 Healing of bone defect
A total of 16 studies were conducted to evaluate impact of PBM on healing of bone defect, with 15 studies conducted on preclinical models (125–139) and one study in humans (140) (Table 7). Among these studies, only one preclinical study did not report a positive impact of PBM on bone repair. This study used the highest power among all studies, with a dosage of 369.4J/cm². In addition to the effect of PBM on bone repair, several studies reported an increase in bone vascularization and a decrease in inflammation.
3.6.3 Chronic periodontitis
A total of 16 studies were conducted to evaluate the impact of PBM on chronic periodontitis in humans (34–40, 141–149) (Table 8).
▪ Effect on healing process
The effects of PBM were evaluated using the following measures:
1) gingival index represents inflammation of the gingival tissue (150),
2) the plaque index, which represents the presence of supragingival plaque on all four tooth surfaces (151).
Among the clinical studies, 11 were RCTs, 3 were RCTs specifically focused on pockets treated with PBM, and two were Pre-Post ITV. The results were heterogeneous, with a decrease in the plaque index observed in 50% of the studies, a decrease in the gingival index in 80% of the studies, a reduction in bleeding in 66% of the studies, a decrease in probing depth in 50% of the studies, and improvements in clinical attachment levels in 29% of the studies.
▪ Effect on bacterial population
Two studies investigated the effect of PBM on reducing the bacterial population at periodontitis sites, but the results were contradictory.
3.7 Others utilization described in diabetes mellitus
3.7.1 Erectile function
In a preclinical study, Yang et al. (152) reported a positive impact of PBM on erectile function two weeks after PBM exposure. This suggests a potential therapeutic effect of PBM on improving erectile function (Table 9).
3.7.2 Ischemia reperfusion injury
Asghari et al. (153) conducted a preclinical study and demonstrated a protective effect of PBM against ischemia/reperfusion injury in the diabetic kidney. They observed a decrease in tubular epithelial necrosis, polymorphonuclear cells in the outer medulla, cellular oedema, tubular dilatation, hyaline casts, and medullary congestion. These findings indicate the potential of PBM in mitigating kidney injury associated with ischemia/reperfusion (Table 9).
3.7.3 Facial nerve palsy
Aghemohamdi et al. (154) demonstrated the positive impact of PBM in patients with T2D who experienced facial nerve palsy. After 12 sessions of PBM, 60% of the patients showed recovery on electromyogram without any reported side effects. However, the investigation of QoL outcomes were not investigated in this study (Table 9).
4 Discussion
This review shows a clear interest in the use of PBM in diabetes, both at preclinical (70 studies) and clinical level [56 studies out of 88 clinical trials identified by clinicaltrials.gov (27)]. However, the therapeutic effect of PBM is variable, with inconsistent illumination parameters that are not standardized across studies.
Regarding clinical trials, PBM has generated interest across various fields, with 2,573 clinical trials identified on clinicaltrials.gov (27). Although 42 clinical trials related to PBM in diabetes were found on clinicaltrials.gov (27), an additional 46 studies were identified through the Medline bibliographic search. Since PBM is not considered as a drug, the reporting of trials in the global clinical trials database is not consistent. Moreover, in some cases, trials may be reported directly to national registries, as seen in many studies conducted in Brazil. Another important point to note is that among the 67 completed trials, only 56 were published, indicating a significant publication bias (16%), which is likely underestimated. Moreover, quality of clinical trials must be upgraded, as there is heterogeneity in the number of patients included, in the presence of a control group and in the parameters used. Furthermore, in terms of clinical publications, few teams have conducted more than one study, amplifying the heterogeneity of the PBM parameters used.
Currently, there is no consensus on the optimal PBM parameters to achieve biological or clinical effects. In terms of light sources, some studies have reported superior effects with coherent laser light (155, 156). However, a recent review found no difference in efficacy between LEDs and laser sources, with LEDs being more cost-effective. In the context of diabetes and wound healing, two studies compared lasers and LEDs. Al-Watban et al. (107) reported a better efficacy of a 633 nm laser compared to LEDs with polychromatic light for ulcer healing, may be due to dilution of the effect as the irradiance was comparable for both. Vitoriano et al. (116) reported a greater reduction in ulcer size with an 830 nm laser compared to 850 nm LEDs (with comparable irradiance). Despite these two studies favoring lasers, numerous studies in the field of diabetes have reported positive effects of LED-based PBM. However, the choice between laser and LED was a technologic choice and could be led by the accuracy of wavelength search, the availability of the device and energetic consumption. It is important to note that the principle of PBM is based on the Arndt-Schultz law (20), which describes a biphasic response. A dose that is too low will produce no effect while a dose that is too high can be toxic and induce mitochondrial permeabilization and apoptosis through activation of caspases (157). This biphasic response to PBM was reflected in two studies on ulcer healing in diabetes. Hedge et al. (101) tested a 632.8 nm laser with different irradiances ranging from 1 to 5 J/cm². While irradiances of 1 and 5 J/cm² resulted in poorer and slower wound healing, an irradiance of 3 J/cm² appeared to be optimal for improving and accelerating wound healing. Maiya et al. (109) tested a 632.8 nm laser with different irradiances ranging from 3 to 9 J/cm². Irradiances between 3 and 7 J/cm² had a positive effect on healing, including increased epithelialization, tissue granulation, and accelerated wound healing; whereas irradiances of 8 and 9 J/cm² hindered the healing process. To date, there is no consensus on the power or optimal irradiance to be applied, and the wavelength applied is another parameter of interest that lacks consensus and may depend on the target tissue. Red and near-infrared light correspond to the absorption wavelengths of cytochrome c oxidase in the mitochondria (23). Green light, on the other hand, is rarely used and not very effective in inducing biological changes as it was not in the specter of absorption of cytochrome c oxidase. Two studies compared blue, green, and red wavelengths in diabetes. Dungel et al. (84) reported that blue light (470 nm) had no effect on wound healing, whereas green (540 nm) and red (629 nm) light accelerated wound healing. Fekrazad et al. (94) reported no effect of blue (425 nm) and green (532 nm) light, whereas red light (630 nm) promoted wound healing. Another study (93) reported that a 632.8 nm laser is more effective than an 830 nm laser for wound healing. Given these findings, it is logical that the most commonly used wavelengths (80 studies) fall within the red and near-infrared range (600–810 nm). In any case, numerous articles have shown that the effect of PBM depends on various parameters: wavelength, fluence (J/cm²), total energy received (J), pulsed or continuous emission mode … Moreover, the absorption characteristics of the tissue, as well as the delivery mode, and the frequency of use of the PBM (number of applications, treatment schedule etc.) add complexity. It is now necessary to standardize PBM parameters, and to precise them into papers; in order to be reproducible and identify effective application methods.
The first experiment to investigate the effect of PBM on healing showed promising results (17, 18). Extensive research has been conducted in this field, with several preclinical and clinical reviews reporting positive effects of PBM on wound healing (158), healing of bone defect (159), and periodontitis (160). In a specific population of patients with diabetes, the results regarding these healing processes were encouraging. Numerous preclinical studies have focused on ulcer and wound healing, demonstrating the beneficial effects of PBM. These effects include improved angiogenesis and associated trophic factors, increased fibroblasts, reduced inflammation, increased collagen quantity, and even a reduction in colony-forming units (CFU). Clinically, these results were supported by a significant reduction in wound area (by a factor of 3.5 to 8.2) and increased wound closure (ranging from 22% to 47.9%), which may be associated with reduced pain. Wound healing issues in patients with diabetes significantly impact their QoL (161). However, only one study (121) has examined the impact of PBM on the QoL of patients with diabetes and ulcers and did not demonstrate any beneficial effects of PBM on QoL. Overall, the data on wound healing are encouraging. The ideal parameters may involve repeated exposure over several weeks, at multiple sites as close as possible to the lesion, with a fluence between 1 and 10 J/cm², continuous illumination using LED or laser, and a wavelength ranging from 660 to 830 nm. In wound healing, PBM appeared as a sage approach to enhance healing process in addition to wound standard of care.
Regarding healing of bone defect, 94% of preclinical studies showed positive results (improved vascularization, increased osteoblasts and osteocytes, reduced inflammation, increased bone volumes, and enhanced bone density). Clinically, only one study (140) has been conducted, demonstrating improved bone repair, density, and structure after dental implant insertion with one session of PBM before and after implantation. However, conclusions cannot be drawn from a single clinical study, but the promising results from preclinical and clinical studies should motivate further clinical trials to determine the optimal parameters for PBM.
To date, only clinical studies have investigated the effects of PBM on periodontitis, yielding heterogeneous results due to variations in the applied parameters. The exposure periods ranged from a single session to several days or even weeks. Among studies that examined the gingival index, plaque index, and clinical attachment level, 75% reported improvements in at least one of these parameters. Four studies reported negative results: one had the highest fluence (144), one had the highest exposure frequency [once a day for 8 weeks (35)], one had poorly described illumination parameters and a wavelength beyond the infrared range (142), and one had four PBM exposure sites (148), whereas most studies reported one to two exposure sites. Finally, the ideal parameters could involve a single exposure or exposure over 2 to 3 days, on one to two sites in direct contact with gingival tissue or intra-pocket, with a fluence of 1 to a few J/cm², continuous or pulsed laser illumination, and a wavelength range of 650 to 1064 nm. These data have been supported by previous results on in vitro model (162) reporting positive response of fibroblasts to the PBM in diabetic hypoxic wounded models. Even if three teams published several studies, the lack of rigorous methodology and the heterogeneity of PBM parameters, did not allowed to identify leader in this field. Moreover, due to the wide variability in illumination parameters and obtained results, definitive conclusions regarding the therapeutic effects of PBM on periodontitis cannot be formally drawn. Further clinical trials are required to establish clearer conclusions and defined optimal PBM parameters to use PBM as an added therapy for diabetes chronic periodontitis management.
Regarding neuropathy, five preclinical studies reported a positive effect of PBM on pain, leading to a decrease in cytokines and improvement in mitochondrial parameters. Clinically, in 77% of the studies, a 2-5 points reduction on the VAS was observed for pain. Out of the studies that considered the impact of PBM on QoL (24%), two studies reported no effect (58, 59) (similarly, no effect on pain was observed), while two studies reported a 4-point improvement in Norfolk Quality of Life-Diabetic Neuropathy (54) and SF-36 scores on all these parameters (44). No adverse effects of PBM were reported in any of the studies. Two teams have been identified in this field. Unfortunately, studies of Burka et al. (28–30). lack methodological rigor and a description of the PBM parameters used. On the other hand, Arun G et al. (31–33), succeeded in demonstrating in their three studies (with the same PBM parameters used) an improvement of vibration perception threshold, decrease of pain, improvement of microcirculation and biological parameters. These benefits were observable as early as 10 days after daily PBM with 2 lasers (632.8 nm and 660 nm + 880 nm) over 9 min (3.1 J/cm² of fluence) on the plantar and dorsal surfaces of the feet. These parameters should therefore serve as a basis for future clinical trials aimed at defining whether MBP will be used instead of or in addition to current pharmacological treatments.
For diabetic retinopathy, only a few studies have been conducted in this field. Four preclinical studies reported promising results on the effect of PBM, showing histological improvements in the retina. However, clinically, only two studies reported a reduction in central macular thickness, with (69) or without (68) an improvement in vision. Therefore, it is not possible to conclude whether PBM must be used. Clinical trials must be conducted to demonstrate PBM safety in use and its efficacy as a complementary or alternative therapy to current therapeutic options.
PBM is also gaining popularity among its potential benefits for post-physical activity recovery, this fact was still a source of debate (163, 164). In the context of T2D, a limited number of studies (n = 7) have been conducted. The results of 3 preclinical studies reported positive effects of PBM on oxidative stress, antioxidant activity, and muscular parameters. However, clinically (n = 4), no study reported improvements in performance or muscle functionality. Only one study (42) reported a benefit in terms of lactate concentration and cardiac parameters. Based on these findings, it can be concluded that the current parameters used for PBM do not provide benefits for post-exercise muscle recovery in patients with T2D.
Lastly, since diabetes is a metabolic disease characterized by an imbalance in glycemic control, PBM has also been investigated in this field. Preclinical results have shown promising results, with 83% of the studies that examined glucose tolerance possibly due to a direct impact of PBM on islet insulin secretion capability and insulin resistance reporting an improvement in these parameters. The only negative study (71) applied the highest power (3.57 W/cm²) for the shortest period (8 seconds). Preclinical studies have also demonstrated beneficial effects of PBM on lipid profiles, including reduced ectopic fat in muscle, triglycerides, and free fatty acids. Additionally, a small-scale clinical study (79) (n = 10) reported beneficial effects of PBM, including a 37% reduction in post-meal AUC for glucose and approximately 16% faster postprandial glucose decay. No adverse effects were reported with PBM, and there was no increased risk of hypoglycemia when PBM was combined with hypoglycemic treatments. Overall, these findings support the potential of PBM in improving glycemic control in patients with type 2 diabetes. Further clinical trials with larger sample sizes are warranted to determine the optimal parameters for PBM as an additional therapy in the therapeutic arsenal, helping to improve patients’ glycemic control.
Moreover, the effect of PBM on glucose intolerance could be lead to the action of PBM on beta cells and islets.
Liebman et al. (165) reported improvement of insulin secretion of beta cells and glucagon of alpha cells associated with a rise of calcium activity. Irani et al. (166) demonstrated that PBM could improve insulin secretion of rat pancreatic islets with poor insulin secretion. Huang et al. (167) investigated the effects of PBM on pig islets, which are being explored as a potential source of islets for xenotransplantation. However, they did not observe any significant positive or negative effects on glucose-stimulated insulin secretion. Further research is needed to explore the potential of PBM to enhance islet function for transplantation purposes, even if Asghari et al. (153) reported protector effect of PBM on ischemia-reperfusion injury in diabetic kidney of rats.
5 Conclusion
Overall, this review highlights the growing interest in PBM as a potential therapeutic approach for various aspects of diabetes. This study emphasizes the potential of PBM as a valuable approach for managing wound healing issues and neuropathic pain in diabetic patients in both preclinical and clinical studies. The potential benefits of PBM in healing of bone defect and glycemic control show promise. In retinopathy, the small number of studies make it impossible to draw any conclusion. In periodontitis, more extensive clinical trials are warranted to establish the optimal parameters and protocols for PBM. Likewise, the current evidence does not support the use of PBM for muscle recovery after physical exercise.
Author contributions
QP: Writing – original draft, Resources, Methodology, Investigation, Formal analysis, Data curation, Conceptualization. CM: Writing – review & editing, Validation, Supervision, Funding acquisition. SL: Writing – review & editing, Validation, Supervision, Funding acquisition, Conceptualization.
Funding
The author(s) declare financial support was received for the research, authorship, and/or publication of this article. This work was funded by the “Région Auvergne-Rhône-Alpes”, the Edmond J.Safra Foundation, the “Fond de Dotation-Clinatec” and its sponsors, CEA, UGA, CHUGA.
Acknowledgments
We thank J. Mitrofanis for his proofreading of English.
Conflict of interest
The authors declare that the research was conducted in the absence of any commercial or financial relationships that could be construed as a potential conflict of interest.
Publisher’s note
All claims expressed in this article are solely those of the authors and do not necessarily represent those of their affiliated organizations, or those of the publisher, the editors and the reviewers. Any product that may be evaluated in this article, or claim that may be made by its manufacturer, is not guaranteed or endorsed by the publisher.
References
1. IDF - diabetes atlas (2021). Available at: https://diabetesatlas.org/idfawp/resource-files/2021/07/IDF_Atlas_10th_Edition_2021.
2. Marcovecchio ML, Lucantoni M, Chiarelli F. Role of chronic and acute hyperglycemia in the development of diabetes complications. Diabetes Technol Ther. (2011) 13:389−94. doi: 10.1089/dia.2010.0146
3. Yamazaki D, Hitomi H, Nishiyama A. Hypertension with diabetes mellitus complications. Hypertens Res Off J Jpn Soc Hypertens. (2018) 41:147−56. doi: 10.1038/s41440-017-0008-y
4. Henning RJ. Type-2 diabetes mellitus and cardiovascular disease. Future Cardiol. (2018) 14:491−509. doi: 10.2217/fca-2018-0045
5. Palau V, Riera M, Soler MJ. The reno-cardiovascular connection in the patient with Diabetes mellitus: What’s new? Endocrinol Diabetes Nutr. (2017) 64:237−40. doi: 10.1016/j.endien.2017.03.011
6. Sabanayagam C, Banu R, Chee ML, Lee R, Wang YX, Tan G, et al. Incidence and progression of diabetic retinopathy: a systematic review. Lancet Diabetes Endocrinol. (2019) 7:140−9. doi: 10.1016/S2213-8587(18)30128-1
7. Zakin E, Abrams R, Simpson DM. Diabetic neuropathy. Semin Neurol. (2019) 39:560−9. doi: 10.1055/s-0039-1688978
8. Zhang P, Lu J, Jing Y, Tang S, Zhu D, Bi Y. Global epidemiology of diabetic foot ulceration: a systematic review and meta-analysis. Ann Med. (2017) 49:106−16. doi: 10.1080/07853890.2016.1231932
9. Barnes JA, Eid MA, Creager MA, Goodney PP. Epidemiology and risk of amputation in patients with diabetes mellitus and peripheral artery disease. Arterioscler Thromb Vasc Biol. (2020) 40:1808−17. doi: 10.1161/ATVBAHA.120.314595
10. Preshaw PM, Alba AL, Herrera D, Jepsen S, Konstantinidis A, Makrilakis K, et al. Periodontitis and diabetes: a two-way relationship. Diabetologia. (2012) 55:21−31. doi: 10.1007/s00125-011-2342-y
11. Navarro-Flores E, Cauli O. Quality of life in individuals with diabetic foot syndrome. Endocr Metab Immune Disord Drug Targets. (2020) 20:1365−72. doi: 10.2174/1871530320666200128154036
12. Simpson TC, Clarkson JE, Worthington HV, MacDonald L, Weldon JC, Needleman I, et al. Treatment of periodontitis for glycemic control in people with diabetes mellitus. Cochrane Database Syst Rev. (2022) 4:CD004714. doi: 10.1002/14651858.CD004714.pub4
13. Joseph JJ, Deedwania P, Acharya T, Aguilar D, Bhatt DL, Chyun DA, et al. Comprehensive management of cardiovascular risk factors for adults with type 2 diabetes: A scientific statement from the american heart association. Circulation. (2022) 145:e722−59. doi: 10.1161/CIR.0000000000001040
14. Teo E, Hassan N, Tam W, Koh S. Effectiveness of continuous glucose monitoring in maintaining glycemic control among people with type 1 diabetes mellitus: a systematic review of randomized controlled trials and meta-analysis. Diabetologia. (2022) 65:604−19. doi: 10.1007/s00125-021-05648-4
15. Cortey A, Renesme L, Raignoux J, Bedu A, Casper C, Tourneux P, et al. Management of jaundice in the newborn≥35 GW: From screening to follow-up after discharge. Guidelines for clinical practice. Arch Pediatr Organe Off Soc Francaise Pediatr. (2017) 24:192−203. doi: 10.1016/j.arcped.2016.11.011
16. Geoffroy PA, Schroder CM, Reynaud E, Bourgin P. Efficacy of light therapy versus antidepressant drugs, and of the combination versus monotherapy, in major depressive episodes: A systematic review and meta-analysis. Sleep Med Rev déc. (2019) 48:101213. doi: 10.1016/j.smrv.2019.101213
17. Mester E, Szende B, Spiry T, Scher A. Stimulation of wound healing by laser rays. Acta Chir Acad Sci Hung. (1972) 13:315−24.
18. Mester E, Szende B, Gärtner P. The effect of laser beams on the growth of hair in mice. Radiobiol Radiother (Berl). (1968) 9:621−6.
19. Cotler HB. A NASA discovery has current applications in orthopedics. Curr Orthop Pract. (2015) 26:72. doi: 10.1097/BCO.0000000000000196
20. Heiskanen V, Hamblin MR. Photobiomodulation: lasers vs. light emitting diodes? Photochem Photobiol Sci Off J Eur Photochem Assoc Eur Soc Photobiol. (2018) 17:1003−17. doi: 10.1039/c8pp00176f
21. Glass GE. Photobiomodulation: A review of the molecular evidence for low level light therapy. J Plast Reconstr Aesthetic Surg JPRAS. (2021) 74:1050−60. doi: 10.1016/j.bjps.2020.12.059
22. Desmet KD, Paz DA, Corry JJ, Eells JT, Wong-Riley MTT, Henry MM, et al. Clinical and experimental applications of NIR-LED photobiomodulation. Photomed Laser Surg. (2006) 24:121−8. doi: 10.1089/pho.2006.24.121
23. Pastore D, Greco M, Passarella S. Specific helium-neon laser sensitivity of the purified cytochrome c oxidase. Int J Radiat Biol. (2000) 76:863−70. doi: 10.1080/09553000050029020
24. Hamblin MR. Mechanisms and applications of the anti-inflammatory effects of photobiomodulation. AIMS Biophys. (2017) 4:337−61. doi: 10.3934/biophy.2017.3.337
25. Dompe C, Moncrieff L, Matys J, Grzech-Leśniak K, Kocherova I, Bryja A, et al. Photobiomodulation-underlying mechanism and clinical applications. J Clin Med. (2020) 9:1724. doi: 10.3390/jcm9061724
26. Zein R, Selting W, Hamblin MR. Review of light parameters and photobiomodulation efficacy: dive into complexity. J BioMed Opt. (2018) 23:1−17. doi: 10.1117/1.JBO.23.12.120901
27. Home - clinicalTrials.gov (2023). Available at: https://clinicaltrials.gov/.
28. Harkless LB, DeLellis S, Carnegie DH, Burke TJ. Improved foot sensitivity and pain reduction in patients with peripheral neuropathy after treatment with monochromatic infrared photo energy–MIRE. J Diabetes Complications. (2006) 20:81−7. doi: 10.1016/j.jdiacomp.2005.06.002
29. DeLellis SL, Carnegie DH, Burke TJ. Improved sensitivity in patients with peripheral neuropathy: effects of monochromatic infrared photo energy. J Am Podiatr Med Assoc. (2005) 95:143−7. doi: 10.7547/0950143
30. Kochman AB, Carnegie DH, Burke TJ. Symptomatic reversal of peripheral neuropathy in patients with diabetes. J Am Podiatr Med Assoc. (2002) 92:125−30. doi: 10.7547/87507315-92-3-125
31. Anjo M, Ummer VS, Maiya AG, Hande M, B VS. Effect of photobiomodulation on serum neuron specific enolase (NSE) among patients with diabetic peripheral neuropathy - A pilot study. Diabetes Metab Syndr. (2020) 14:1061−3. doi: 10.1016/j.dsx.2020.06.065
32. Anju M, Chacko L, Chettupalli Y, Maiya AG, Saleena Ummer V. Effect of Low Level Laser Therapy on serum vitamin D and magnesium levels in patients with diabetic peripheral neuropathy - A pilot study. Diabetes Metab Syndr. (2019) 13:1087−91. doi: 10.1016/j.dsx.2019.01.022
33. Yamany AA, Sayed HM. Effect of low level laser therapy on neurovascular function of diabetic peripheral neuropathy. J Adv Res. (2012) 3:21−8. doi: 10.1016/j.jare.2011.02.009
34. Kamatham SA, Chava VK. Comparison of salivary calprotectin levels in periodontitis associated with diabetes mellitus after low-level laser therapy as an adjunct to scaling and root planning: A randomized clinical trial. J Indian Soc Periodontol. (2022) 26:143−50. doi: 10.4103/jisp.jisp_149_21
35. Pulivarthi P, Chava VK, Gunupati S. Salivary tumor necrosis factor-alpha levels in periodontitis associated with diabetes mellitus after low level laser therapy as an adjunct to scaling and root planning: A randomized clinical trial. J Indian Soc Periodontol. (2022) 26:236−44. doi: 10.4103/jisp.jisp_150_21
36. Kocak E, Sağlam M, Arslan U, Kayis SA, Kebapcilar L, Loos BG, et al. Effect of diode laser application as an adjunct to nonsurgical periodontal therapy on the reduction of red complex microorganisms in type 2 diabetics with chronic periodontitis. Lasers Med Sci. (2020) 35:1403−10. doi: 10.1007/s10103-020-02997-1
37. Koçak E, Sağlam M, Kayış SA, Dündar N, Kebapçılar L, Loos BG, et al. Nonsurgical periodontal therapy with/without diode laser modulates metabolic control of type 2 diabetics with periodontitis: a randomized clinical trial. Lasers Med Sci. (2016) 31:343−53. doi: 10.1007/s10103-016-1868-0
38. Obradović R, Kesić L, Mihailović D, Antić S, Jovanović G, Petrović A, et al. A histological evaluation of a low-level laser therapy as an adjunct to periodontal therapy in patients with diabetes mellitus. Lasers Med Sci. (2013) 28:19−24. doi: 10.1007/s10103-012-1058-7
39. Obradović R, Kesić L, Mihailović D, Jovanović G, Antić S, Brkić Z. Low-level lasers as an adjunct in periodontal therapy in patients with diabetes mellitus. Diabetes Technol Ther. (2012) 14:799−803. doi: 10.1089/dia.2012.0027
40. Obradović R, Kesić L, Jovanović G, Petrović D, Goran R, Mihailović D. Low power laser efficacy in the therapy of inflamed gingive in diabetics with parodontopathy. Vojnosanit Pregl. (2011) 68:684−9. doi: 10.2298/VSP1108684O
41. de Oliveira AR, da Silva FS, Bortolin RH, Marques DE da S, Ramos GV, Marqueti RC, et al. Effect of photobiomodulation and exercise on early remodeling of the Achilles tendon in streptozotocin-induced diabetic rats. PloS One. (2019) 14:e0211643. doi: 10.1371/journal.pone.0211643
42. Linares SN, Beltrame T, Galdino GAM, Frade MCM, Milan-Mattos JC, Gois MO, et al. Dose response effect of photobiomodulation on hemodynamic responses and glucose levels in men with type 2 diabetes: A randomized, crossover, double-blind, sham-controlled trial. Photonics. juill. (2022) 9:481. doi: 10.3390/photonics9070481
43. Milan-Mattos JC, de Oliveira Francisco C, Ferroli-Fabrício AM, Minatel V, Marcondes ACA, Porta A, et al. Acute effect of photobiomodulation using light-emitting diodes (LEDs) on baroreflex sensitivity during and after constant loading exercise in patients with type 2 diabetes mellitus. Lasers Med Sci. (2020) 35:329−36. doi: 10.1007/s10103-019-02815-3
44. da Silva Leal MV, Lima MO, Nicolau RA, de Carvallho TMT, Abreu JA de C, Pessoa DR, et al. Effect of modified laser transcutaneous irradiation on pain and quality of life in patients with diabetic neuropathy. Photobiomodulation Photomed Laser Surg. (2020) 38:138−44. doi: 10.1089/photob.2019.4714
45. de Alencar Fonseca Santos J, Campelo MBD, de Oliveira RA, Nicolau RA, Rezende VEA, Arisawa EÂL. Effects of low-power light therapy on the tissue repair process of chronic wounds in diabetic feet. Photomed Laser Surg. (2018) 36:298−304. doi: 10.1089/pho.2018.4455
46. Schindl A, Heinze G, Schindl M, Pernerstorfer-Schön H, Schindl L. Systemic effects of low-intensity laser irradiation on skin microcirculation in patients with diabetic microangiopathy. Microvasc Res. (2002) 64:240−6. doi: 10.1006/mvre.2002.2429
47. Schindl M, Kerschan K, Schindl A, Schön H, Heinzl H, Schindl L. Induction of complete wound healing in recalcitrant ulcers by low-intensity laser irradiation depends on ulcer cause and size. Photodermatol Photoimmunol Photomed. (1999) 15:18−21. doi: 10.1111/j.1600-0781.1999.tb00047.x
48. Schindl A, Schindl M, Schön H, Knobler R, Havelec L, Schindl L. Low-intensity laser irradiation improves skin circulation in patients with diabetic microangiopathy. Diabetes Care. (1998) 21:580−4. doi: 10.2337/diacare.21.4.580
49. Vieira WF, Malange KF, de Magalhães SF, Lemes JBP, Dos Santos GG, Nishijima CM, et al. Anti-hyperalgesic effects of photobiomodulation therapy (904 nm) on streptozotocin-induced diabetic neuropathy imply MAPK pathway and calcium dynamics modulation. Sci Rep. (2022) 12:16730. doi: 10.1038/s41598-022-19947-2
50. Rocha IRC, Perez-Reyes E, Chacur M. Effect of photobiomodulation on mitochondrial dynamics in peripheral nervous system in streptozotocin-induced type 1 diabetes in rats. Photochem Photobiol Sci Off J Eur Photochem Assoc Eur Soc Photobiol. (2021) 20:293−301. doi: 10.1007/s43630-021-00018-w
51. Vieira WF, de Magalhães SF, Farias FH, de Thomaz AA, Parada CA. Raman spectroscopy of dorsal root ganglia from streptozotocin-induced diabetic neuropathic rats submitted to photobiomodulation therapy. J Biophotonics. nov. (2019) 12:e201900135. doi: 10.1002/jbio.201900135
52. Abdel-Wahhab KG, Daoud EM, El Gendy A, Mourad HH, Mannaa FA, Saber MM. Efficiencies of low-level laser therapy (LLLT) and gabapentin in the management of peripheral neuropathy: diabetic neuropathy. Appl Biochem Biotechnol. (2018) 186:161−73. doi: 10.1007/s12010-018-2729-z
53. da Silva Oliveira VR, Cury DP, Yamashita LB, Esteca MV, Watanabe IS, Bergmann YF, et al. Photobiomodulation induces antinociception, recovers structural aspects and regulates mitochondrial homeostasis in peripheral nerve of diabetic mice. J Biophotonics. (2018) 11:e201800110. doi: 10.1002/jbio.201800110
54. Rastogi A, Uppula P, Saikia U, Bhansali A. Effect of monochromatic infrared energy on quality of life and intraepidermal nerve fiber density in painful diabetic neuropathy: A randomized, sham control study. Neurol India. (2021) 69:1331−7. doi: 10.4103/0028-3886.329614
55. Cg SK, Maiya AG, Hande HM, Vidyasagar S, Rao K, Rajagopal KV. Efficacy of low level laser therapy on painful diabetic peripheral neuropathy. Laser Ther. (2015) 24:195−200. doi: 10.5978/islsm.15-OR-12
56. Bashiri H. Evaluation of low level laser therapy in reducing diabetic polyneuropathy related pain and sensorimotor disorders. Acta Med Iran. (2013) 51:543−7.
57. Khamseh ME, Kazemikho N, Aghili R, Forough B, Lajevardi M, Hashem Dabaghian F, et al. Diabetic distal symmetric polyneuropathy: effect of low-intensity laser therapy. Lasers Med Sci. (2011) 26:831−5. doi: 10.1007/s10103-011-0977-z
58. Swislocki A, Orth M, Bales M, Weisshaupt J, West C, Edrington J, et al. A randomized clinical trial of the effectiveness of photon stimulation on pain, sensation, and quality of life in patients with diabetic peripheral neuropathy. J Pain Symptom Manage. (2010) 39:88−99. doi: 10.1016/j.jpainsymman.2009.05.021
59. Lavery LA, Murdoch DP, Williams J, Lavery DC. Does anodyne light therapy improve peripheral neuropathy in diabetes? A double-blind, sham-controlled, randomized trial to evaluate monochromatic infrared photoenergy. Diabetes Care. (2008) 31:316−21. doi: 10.2337/dc07-1794
60. Arnall DA, Nelson AG, López L, Sanz N, Iversen L, Sanz I, et al. The restorative effects of pulsed infrared light therapy on significant loss of peripheral protective sensation in patients with long-term type 1 and type 2 diabetes mellitus. Acta Diabetol. (2006) 43:26−33. doi: 10.1007/s00592-006-0207-5
61. Clifft JK, Kasser RJ, Newton TS, Bush AJ. The effect of monochromatic infrared energy on sensation in patients with diabetic peripheral neuropathy: a double-blind, placebo-controlled study. Diabetes Care. (2005) 28:2896−900. doi: 10.2337/diacare.28.12.2896
62. Leonard DR, Farooqi MH, Myers S. Restoration of sensation, reduced pain, and improved balance in subjects with diabetic peripheral neuropathy: a double-blind, randomized, placebo-controlled study with monochromatic near-infrared treatment. Diabetes Care. (2004) 27:168−72. doi: 10.2337/diacare.27.1.168
63. Zinman LH, Ngo M, Ng ET, Nwe KT, Gogov S, Bril V. Low-intensity laser therapy for painful symptoms of diabetic sensorimotor polyneuropathy: a controlled trial. Diabetes Care. (2004) 27:921−4. doi: 10.2337/diacare.27.4.921
64. Ahmed SA, Ghoneim DF, Morsy ME, Hassan AA, Mahmoud ARH. Low-level laser therapy with 670 nm alleviates diabetic retinopathy in an experimental model. J Curr Ophthalmol. (2021) 33:143−51. doi: 10.4103/JOCO.JOCO_29_20
65. Cheng Y, Du Y, Liu H, Tang J, Veenstra A, Kern TS. Photobiomodulation inhibits long-term structural and functional lesions of diabetic retinopathy. Diabetes. (2018) 67:291−8. doi: 10.2337/db17-0803
66. Saliba A, Du Y, Liu H, Patel S, Roberts R, Berkowitz BA, et al. Photobiomodulation mitigates diabetes-induced retinopathy by direct and indirect mechanisms: evidence from intervention studies in pigmented mice. PloS One. (2015) 10:e0139003. doi: 10.1371/journal.pone.0139003
67. Tang J, Du Y, Lee CA, Talahalli R, Eells JT, Kern TS. Low-intensity far-red light inhibits early lesions that contribute to diabetic retinopathy: in vivo and in vitro. Invest Ophthalmol Vis Sci. (2013) 54:3681−90. doi: 10.1167/iovs.12-11018
68. Shen W, Teo KYC, Wood JPM, Vaze A, Chidlow G, Ao J, et al. Preclinical and clinical studies of photobiomodulation therapy for macular oedema. Diabetologia. (2020) 63:1900−15. doi: 10.1007/s00125-020-05189-2
69. Eells JT, Gopalakrishnan S, Connor TB, Stepien K, Carroll J, Williams V, et al. 670 nm photobiomodulation as a therapy for diabetic macular edema: A pilot study. Invest Ophthalmol Vis Sci. (2017) 58:932.
70. Min SH, Kwon J, Do EJ, Kim SH, Kim ES, Jeong JY, et al. Duodenal dual-wavelength photobiomodulation improves hyperglycemia and hepatic parameters with alteration of gut microbiome in type 2 diabetes animal model. Cells. (2022) 11:3490. doi: 10.3390/cells11213490
71. Bonifacio M, Benfato ID, de Almeida Cruz M, de Sales DC, Pandolfo IL, Quintana HT, et al. Effects of photobiomodulation on glucose homeostasis and morphometric parameters in pancreatic islets of diabetic mice. Lasers Med Sci. (2022) 37:1799−809. doi: 10.1007/s10103-021-03434-7
72. Gong L, Zou Z, Liu L, Guo S, Xing D. Photobiomodulation therapy ameliorates hyperglycemia and insulin resistance by activating cytochrome c oxidase-mediated protein kinase B in muscle. Aging. (2021) 13:10015−33. doi: 10.18632/aging.v13i7
73. Gong L, Zou Z, Huang L, Guo S, Xing D. Photobiomodulation therapy decreases free fatty acid generation and release in adipocytes to ameliorate insulin resistance in type 2 diabetes. Cell Signal. (2020) 67:109491. doi: 10.1016/j.cellsig.2019.109491
74. Guo S, Gong L, Shen Q, Xing D. Photobiomodulation reduces hepatic lipogenesis and enhances insulin sensitivity through activation of CaMKKβ/AMPK signaling pathway. J Photochem Photobiol B. (2020) 213:112075. doi: 10.1016/j.jphotobiol.2020.112075
75. Hsu YH, Chen YC, Chen YW, Chiu TH, Kuo YT, Chen CH. Far-infrared radiation prevents decline in β-cell mass and function in diabetic mice via the mitochondria-mediated Sirtuin1 pathway. Metabolism. (2020) 104:154143. doi: 10.1016/j.metabol.2020.154143
76. Silva G, Ferraresi C, de Almeida RT, Motta ML, Paixão T, Ottone VO, et al. Insulin resistance is improved in high-fat fed mice by photobiomodulation therapy at 630 nm. J Biophotonics. mars. (2020) 13:e201960140. doi: 10.1002/jbio.201960140
77. Silva G, Ferraresi C, de Almeida RT, Motta ML, Paixão T, Ottone VO, et al. Infrared photobiomodulation (PBM) therapy improves glucose metabolism and intracellular insulin pathway in adipose tissue of high-fat fed mice. Lasers Med Sci. (2018) 33:559−71. doi: 10.1007/s10103-017-2408-2
78. Yoshimura TM, Sabino CP, Ribeiro MS. Photobiomodulation reduces abdominal adipose tissue inflammatory infiltrate of diet-induced obese and hyperglycemic mice. J Biophotonics. (2016) 9:1255−62. doi: 10.1002/jbio.201600088
79. Scontri CMCB, de Castro Magalhães F, Damiani APM, Hamblin MR, Zamunér AR, Ferraresi C. Dose and time-response effect of photobiomodulation therapy on glycemic control in type 2 diabetic patients combined or not with hypoglycemic medicine: a randomized, crossover, double-blind, sham controlled trial. J Biophotonics. (2023) 16:e202300083. doi: 10.1002/jbio.202300083
80. da Silva Tonetto L, da Silva CCF, Gonzatti N, Guex CG, Hartmann DD, Boschi ES, et al. Effects of photobiomodulation on oxidative stress in rats with type 2 diabetes mellitus. Lasers Med Sci. (2023) 38:90. doi: 10.1007/s10103-023-03745-x
81. Frigero M, Dos Santos SA, Serra AJ, Dos Santos Monteiro MaChado C, Portes LA, Tucci PJF, et al. Effect of photobiomodulation therapy on oxidative stress markers of gastrocnemius muscle of diabetic rats subjected to high-intensity exercise. Lasers Med Sci. (2018) 33:1781−90. doi: 10.1007/s10103-018-2540-7
82. Gobbi A, de Carvalho G, Sapalo AT, de Jesus Guirro RR. Acute application of photobiomodulation does not bring important gains for the muscular performance and functionality of diabetic individuals. Lasers Med Sci. (2021) 36:995−1002. doi: 10.1007/s10103-020-03135-7
83. Francisco C de O, Beltrame T, Hughson RL, Milan-Mattos JC, Ferroli-Fabricio AM, Galvão Benze B, et al. Effects of light-emitting diode therapy (LEDT) on cardiopulmonary and hemodynamic adjustments during aerobic exercise and glucose levels in patients with diabetes mellitus: A randomized, crossover, double-blind and placebo-controlled clinical trial. Complement Ther Med. (2019) 42:178−83. doi: 10.1016/j.ctim.2018.11.015
84. Dungel P, Sutalo S, Slezak C, Keibl C, Schädl B, Schnidar H, et al. Wavelength-dependent effects of photobiomodulation for wound care in diabetic wounds. Int J Mol Sci. (2023) 24:5895. doi: 10.3390/ijms24065895
85. Ebrahimpour-Malekshah R, Amini A, Mostafavinia A, Ahmadi H, Zare F, Safaju S, et al. The stereological, immunohistological, and gene expression studies in an infected ischemic wound in diabetic rats treated by human adipose-derived stem cells and photobiomodulation. Arch Dermatol Res. (2023) 1717–34. doi: 10.1007/s00403-023-02563-z
86. Mehrvar S, Mostaghimi S, Foomani FH, Abroe B, Eells JT, Gopalakrishnan S, et al. 670 nm photobiomodulation improves the mitochondrial redox state of diabetic wounds. Quant Imaging Med Surg. (2021) 11:107−18. doi: 10.21037/qims
87. Ahmadi H, Amini A, Fadaei Fathabady F, Mostafavinia A, Zare F, Ebrahimpour-Malekshah R, et al. Transplantation of photobiomodulation-preconditioned diabetic stem cells accelerates ischemic wound healing in diabetic rats. Stem Cell Res Ther. (2020) 11:494. doi: 10.1186/s13287-020-01967-2
88. Bagheri M, Mostafavinia A, Abdollahifar MA, Amini A, Ghoreishi SK, Chien S, et al. Combined effects of metformin and photobiomodulation improve the proliferation phase of wound healing in type 2 diabetic rats. BioMed Pharmacother Biomedecine Pharmacother. (2020) 123:109776. doi: 10.1016/j.biopha.2019.109776
89. Kouhkheil R, Fridoni M, Abdollhifar MA, Amini A, Bayat S, Ghoreishi SK, et al. Impact of photobiomodulation and condition medium on mast cell counts, degranulation, and wound strength in infected skin wound healing of diabetic rats. Photobiomodulation Photomed Laser Surg. (2019) 37:706−14. doi: 10.1089/photob.2019.4691
90. Fekrazad R, Sarrafzadeh A, Kalhori KAM, Khan I, Arany PR, Giubellino A. Improved wound remodeling correlates with modulated TGF-beta expression in skin diabetic wounds following combined red and infrared photobiomodulation treatments. Photochem Photobiol. (2018) 94:775−9. doi: 10.1111/php.12914
91. Asghari M, Kanonisabet A, Safakhah M, Azimzadeh Z, Mostafavinia A, Taheri S, et al. The effect of combined photobiomodulation and metformin on open skin wound healing in a non-genetic model of type II diabetes. J Photochem Photobiol B. (2017) 169:63−9. doi: 10.1016/j.jphotobiol.2017.03.002
92. Leite G de PMF, das Neves LMS, Silva CA, Guirro RR de J, de Souza TR, de Souza AK, et al. Photobiomodulation laser and pulsed electrical field increase the viability of the musculocutaneous flap in diabetic rats. Lasers Med Sci. (2017) 32:641−8. doi: 10.1007/s10103-017-2160-7
93. Fahimipour F, Houshmand B, Alemi P, Asnaashari M, Tafti MA, Akhoundikharanagh F, et al. The effect of He-Ne and Ga-Al-As lasers on the healing of oral mucosa in diabetic mice. J Photochem Photobiol B. (2016) 159:149−54. doi: 10.1016/j.jphotobiol.2016.03.020
94. Fekrazad R, Mirmoezzi A, Kalhori KA, Arany P. The effect of red, green and blue lasers on healing of oral wounds in diabetic rats. J Photochem Photobiol B. (2015) 148:242−5. doi: 10.1016/j.jphotobiol.2015.04.018
95. Dancáková L, Vasilenko T, Kováč I, Jakubčová K, Hollý M, Revajová V, et al. Low-level laser therapy with 810 nm wavelength improves skin wound healing in rats with streptozotocin-induced diabetes. Photomed Laser Surg. (2014) 32:198−204. doi: 10.1089/pho.2013.3586
96. Aparecida Da Silva A, Leal-Junior ECP, Alves ACA, Rambo CS, Dos Santos SA, Vieira RP, et al. Wound-healing effects of low-level laser therapy in diabetic rats involve the modulation of MMP-2 and MMP-9 and the redistribution of collagen types I and III. J Cosmet Laser Ther Off Publ Eur Soc Laser Dermatol. (2013) 15:210−6. doi: 10.3109/14764172.2012.761345
97. Fathabadie FF, Bayat M, Amini A, Bayat M, Rezaie F. Effects of pulsed infra-red low level-laser irradiation on mast cells number and degranulation in open skin wound healing of healthy and streptozotocin-induced diabetic rats. J Cosmet Laser Ther Off Publ Eur Soc Laser Dermatol. (2013) 15:294−304. doi: 10.3109/14764172.2013.764435
98. Firat ET, Dağ A, Günay A, Kaya B, Karadede Mİ, Kanay BE, et al. The effects of low-level laser therapy on palatal mucoperiosteal wound healing and oxidative stress status in experimental diabetic rats. Photomed Laser Surg. (2013) 31:315−21. doi: 10.1089/pho.2012.3406
99. Dadpay M, Sharifian Z, Bayat M, Bayat M, Dabbagh A. Effects of pulsed infra-red low level-laser irradiation on open skin wound healing of healthy and streptozotocin-induced diabetic rats by biomechanical evaluation. J Photochem Photobiol B. (2012) 111:1−8. doi: 10.1016/j.jphotobiol.2012.03.001
100. Park JJ, Kang KL. Effect of 980-nm GaAlAs diode laser irradiation on healing of extraction sockets in streptozotocin-induced diabetic rats: a pilot study. Lasers Med Sci. (2012) 27:223−30. doi: 10.1007/s10103-011-0944-8
101. Hegde VN, Prabhu V, Rao SBS, Chandra S, Kumar P, Satyamoorthy K, et al. Effect of laser dose and treatment schedule on excision wound healing in diabetic mice. Photochem Photobiol. (2011) 87:1433−41. doi: 10.1111/j.1751-1097.2011.00991.x
102. Peplow PV, Chung TY, Ryan B, Baxter GD. Laser photobiostimulation of wound healing: reciprocity of irradiance and exposure time on energy density for splinted wounds in diabetic mice. Lasers Surg Med. (2011) 43:843−50. doi: 10.1002/lsm.21094
103. Carvalho PTCde, Silva IS, Reis FA, Perreira DM, Aydos RD. Influence of ingaalp laser (660nm) on the healing of skin wounds in diabetic rats. Acta Cir Bras. (2010) 25:71−9. doi: 10.1590/S0102-86502010000100016
104. Akyol U, Güngörmüş M. The effect of low-level laser therapy on healing of skin incisions made using a diode laser in diabetic rats. Photomed Laser Surg. (2010) 28:51−5. doi: 10.1089/pho.2008.2425
105. Chung TY, Peplow PV, Baxter GD. Laser photobiostimulation of wound healing: defining a dose response for splinted wounds in diabetic mice. Lasers Surg Med. (2010) 42:656−64. doi: 10.1002/lsm.20981
106. Santos NRS, dos Santos JN, dos Reis JA, Oliveira PC, de Sousa APC, de Carvalho CM, et al. Influence of the use of laser phototherapy (lambda660 or 790 nm) on the survival of cutaneous flaps on diabetic rats. Photomed Laser Surg. (2010) 28:483−8. doi: 10.1089/pho.2009.2500
107. Al-Watban FAH. Laser therapy converts diabetic wound healing to normal healing. Photomed Laser Surg. (2009) 27:127−35. doi: 10.1089/pho.2008.2406
108. Güngörmüş M, Akyol UK. Effect of biostimulation on wound healing in diabetic rats. Photomed Laser Surg. (2009) 27:607−10. doi: 10.1089/pho.2008.2349
109. Maiya AG, Kumar P, Nayak S. Photo-stimulatory effect of low energy helium-neon laser irradiation on excisional diabetic wound healing dynamics in Wistar rats. Indian J Dermatol. (2009) 54:323−9. doi: 10.4103/0019-5154.57606
110. Carvalho PdeTCde, Mazzer N, dos Reis FA, Belchior ACG, Silva IS. Analysis of the influence of low-power HeNe laser on the healing of skin wounds in diabetic and non-diabetic rats. Acta Cir Bras. (2006) 21:177−83. doi: 10.1590/S0102-86502006000300010
111. Rabelo SB, Villaverde AB, Nicolau R, Salgado MC, Melo MDS, Pacheco MTT. Comparison between wound healing in induced diabetic and nondiabetic rats after low-level laser therapy. Photomed Laser Surg. (2006) 24:474−9. doi: 10.1089/pho.2006.24.474
112. Maiya GA, Kumar P, Rao L. Effect of low intensity helium-neon (He-Ne) laser irradiation on diabetic wound healing dynamics. Photomed Laser Surg. (2005) 23:187−90. doi: 10.1089/pho.2005.23.187
113. Byrnes KR, Barna L, Chenault VM, Waynant RW, Ilev IK, Longo L, et al. Photobiomodulation improves cutaneous wound healing in an animal model of type II diabetes. Photomed Laser Surg. (2004) 22:281−90. doi: 10.1089/1549541041797977
114. Reddy GK, Stehno-Bittel L, Enwemeka CS. Laser photostimulation accelerates wound healing in diabetic rats. Wound Repair Regener Off Publ Wound Heal Soc Eur Tissue Repair Soc. (2001) 9:248−55. doi: 10.1046/j.1524-475x.2001.00248.x
115. Haze A, Gavish L, Elishoov O, Shorka D, Tsohar T, Gellman YN, et al. Treatment of diabetic foot ulcers in a frail population with severe co-morbidities using at-home photobiomodulation laser therapy: a double-blind, randomized, sham-controlled pilot clinical study. Lasers Med Sci. (2022) 37:919−28. doi: 10.1007/s10103-021-03335-9
116. Vitoriano NAM, Mont’Alverne DGB, Martins MIS, Silva PS, Martins CA, Teixeira HD, et al. Comparative study on laser and LED influence on tissue repair and improvement of neuropathic symptoms during the treatment of diabetic ulcers. Lasers Med Sci. (2019) 34:1365−71. doi: 10.1007/s10103-019-02724-5
117. Frangež I, Nizič-Kos T, Frangež HB. Phototherapy with LED shows promising results in healing chronic wounds in diabetes mellitus patients: A prospective randomized double-blind study. Photomed Laser Surg. (2018) 36:377−82. doi: 10.1089/pho.2017.4382
118. Ruh AC, Frigo L, Cavalcanti MFXB, Svidnicki P, Vicari VN, Lopes-Martins RAB, et al. Laser photobiomodulation in pressure ulcer healing of human diabetic patients: gene expression analysis of inflammatory biochemical markers. Lasers Med Sci. (2018) 33:165−71. doi: 10.1007/s10103-017-2384-6
119. Mathur RK, Sahu K, Saraf S, Patheja P, Khan F, Gupta PK. Low-level laser therapy as an adjunct to conventional therapy in the treatment of diabetic foot ulcers. Lasers Med Sci. (2017) 32:275−82. doi: 10.1007/s10103-016-2109-2
120. Carvalho AFMde, Feitosa MCP, Coelho NPM de F, Rebêlo VCN, Castro JGde, Sousa PRGde, et al. Low-level laser therapy and Calendula officinalis in repairing diabetic foot ulcers. Rev Esc Enferm U P. (2016) 50:628−34. doi: 10.1590/S0080-623420160000500013
121. Sandoval Ortíz MC, Herrera Villabona E, Camargo Lemos DM, Castellanos R. Effects of low level laser therapy and high voltage stimulation on diabetic wound healing. Rev Univ Ind Santander Salud. (2014) 46:107−17.
122. Kajagar BM, Godhi AS, Pandit A, Khatri S. Efficacy of low level laser therapy on wound healing in patients with chronic diabetic foot ulcers-a randomized control trial. Indian J Surg. (2012) 74:359−63. doi: 10.1007/s12262-011-0393-4
123. Kaviani A, Djavid GE, Ataie-Fashtami L, Fateh M, Ghodsi M, Salami M, et al. A randomized clinical trial on the effect of low-level laser therapy on chronic diabetic foot wound healing: a preliminary report. Photomed Laser Surg. (2011) 29:109−14. doi: 10.1089/pho.2009.2680
124. Minatel DG, Frade MAC, França SC, Enwemeka CS. Phototherapy promotes healing of chronic diabetic leg ulcers that failed to respond to other therapies. Lasers Surg Med. (2009) 41:433−41. doi: 10.1002/lsm.20789
125. Dalirsani Z, Ghazi N, Delavarian Z, Pakfetrat A, Esmaily H, Davaji M, et al. Effects of diode low-level laser therapy on healing of tooth extraction sockets: a histopathological study in diabetic rats. Lasers Med Sci. (2021) 36:1527−34. doi: 10.1007/s10103-021-03270-9
126. Lee JH, Kong SC, Chen CH, Lin YC, Lee KT, Wang YH. The effects of photobiomodulation on bone defect repairing in a diabetic rat model. Int J Mol Sci. (2021) 22:11026. doi: 10.3390/ijms222011026
127. Diker N, Aytac D, Helvacioglu F, Dagdelen C, Oguz Y. Evaluation of the effects of low-level laser therapy on diabetic bone healing. J Craniofac Surg. (2019) 30:1994−8. doi: 10.1097/SCS.0000000000005654
128. Gomes MF, Goulart M da GV, Giannasi LC, Hiraoka CM, Melo G de FS, Zangaro RA, et al. Effects of the photobiomodulation using different energy densities on the periodontal tissues under orthodontic force in rats with type 2 diabetes mellitus. Braz Oral Res. (2018) 32:e61. doi: 10.1590/1807-3107bor-2018.vol32.0061
129. Mostafavinia A, Masteri Farahani R, Abdollahifar MA, Ghatrehsamani M, Ghoreishi SK, Hajihossainlou B, et al. Evaluation of the effects of photobiomodulation on partial osteotomy in streptozotocin-induced diabetes in rats. Photomed Laser Surg. (2018) 36:406−14. doi: 10.1089/pho.2018.4438
130. Mostafavinia A, Razavi S, Abdollahifar M, Amini A, Ghorishi SK, Rezaei F, et al. Evaluation of the effects of photobiomodulation on bone healing in healthy and streptozotocin-induced diabetes in rats. Photomed Laser Surg. (2017) 35:537−45. doi: 10.1089/pho.2016.4224
131. Yildirimturk S, Sirin Y, Soluk Tekkesin M, Gurler G, Firat D. The effects of low-level laser therapy on the healing of bone defects in streptozotocin-induced diabetic rats: A histological and morphometric evaluation. J Cosmet Laser Ther Off Publ Eur Soc Laser Dermatol. (2017) 19:397−403. doi: 10.1080/14764172.2017.1341048
132. Patrocínio-Silva TL, Souza AMFde, Goulart RL, Pegorari CF, Oliveira JR, Fernandes KR, et al. Low-level laser therapy associated to a resistance training protocol on bone tissue in diabetic rats. Arch Endocrinol Metab. Arch Endocrinol Metab. (2016) 60:457−64. doi: 10.1590/2359-3997000000190
133. Magri AMP, Fernandes KR, Assis L, Mendes NA, da Silva Santos ALY, de Oliveira Dantas E, et al. Photobiomodulation and bone healing in diabetic rats: evaluation of bone response using a tibial defect experimental model. Lasers Med Sci. (2015) 30:1949−57. doi: 10.1007/s10103-015-1789-3
134. Nascimento MFdo, Almeida BMde, Cunha JLS, Valois RBV, Pinheiro JC, Ribeiro MAG, et al. Improvement of bone repair in diabetic rats subjected to ƛ780 nm low-level laser therapy. Acta Cir Bras. (2015) 30:660−7. doi: 10.1590/S0102-865020150100000002
135. Patrocínio-Silva TL, de Souza AMF, Goulart RL, Pegorari CF, Oliveira JR, Fernandes K, et al. The effects of low-level laser irradiation on bone tissue in diabetic rats. Lasers Med Sci. (2014) 29:1357−64. doi: 10.1007/s10103-013-1418-y
136. Akyol UK, Güngörmüş M. Effect of biostimulation on healing of bone defects in diabetic rats. Photomed Laser Surg. (2010), 28411−6. doi: 10.1089/pho.2008.2478
137. Abdi S, Bayat M, Javadieh F, Mohsenifar Z, Rezaie F, Bayat M. The effects of helium-neon light therapy on healing of partial osteotomy of the tibia in streptozotocin induced diabetic rats. Photomed Laser Surg. (2009) 27:907−12. doi: 10.1089/pho.2008.2421
138. Bayat M, Abdi S, Javadieh F, Mohsenifar Z, Rashid MR. The effects of low-level laser therapy on bone in diabetic and nondiabetic rats. Photomed Laser Surg. (2009) 27:703−8. doi: 10.1089/pho.2008.2351
139. Javadieh F, Bayat M, Abdi S, Mohsenifar Z, Razi S. The effects of infrared low-level laser therapy on healing of partial osteotomy of tibia in streptozotocin-induced diabetic rats. Photomed Laser Surg. (2009) 27:641−6. doi: 10.1089/pho.2008.2370
140. Attia MS, Elewa GM, Abdelgawad N, Ismail RM, Hassan Eid M, Ghoneim MM. The influence of low-level laser therapy on CBCT radiographic and biochemical profiles of type II controlled diabetic patients after dental implant insertion: A randomized case-control study. Cureus. mars. (2023) 15:e36559. doi: 10.7759/cureus.36559
141. Mrasori S, Popovska M, Rusevska B, Shkreta M, Selani A, Bunjaku V. Effects of low level laser therapy (LLLT) on serum values of interleukin 6 (IL-6) in patients with periodontitis and type 2 diabetes mellitus (T2DM). Acta Inform Med AIM J Soc Med Inform Bosnia Herzeg Cas Drustva Za Med Inform BiH. (2021) 29:59−64. doi: 10.5455/aim.
142. Soi S, Bains VK, Srivastava R, Madan R. Comparative evaluation of improvement in periodontal and glycemic health status of type 2 diabetes mellitus patients after scaling and root planing with or without adjunctive use of diode laser. Lasers Med Sci. (2021) 36:1307−15. doi: 10.1007/s10103-021-03261-w
143. Özberk SS, Gündoğar H, Özkaya M, Taner İL, Erciyas K. The effect of photobiomodulation therapy on nonsurgical periodontal treatment in patients with type 2 diabetes mellitus: a randomized controlled, single-blind, split-mouth clinical trial. Lasers Med Sci. (2020) 35:497−504. doi: 10.1007/s10103-019-02897-z
144. Castro Dos Santos N, Andere NMRB, Miguel MMV, Dos Santos LM, Santamaria M, Mathias IF, et al. Photobiomodulation for the treatment of periodontal pockets in patients with type 2 diabetes: 1-year results of a randomized clinical trial. Lasers Med Sci. (2019) 34:1897−904. doi: 10.1007/s10103-019-02799-0
145. Chandra S, Shashikumar P. Diode laser - A novel therapeutic approach in the treatment of chronic periodontitis in type 2 diabetes mellitus patients: A prospective randomized controlled clinical trial. J Lasers Med Sci. (2019) 10:56−63. doi: 10.15171/jlms.2019.09
146. Dengizek Eltas S, Gursel M, Eltas A, Alptekin NO, Ataoglu T. Evaluation of long-term effects of diode laser application in periodontal treatment of poorly controlled type 2 diabetic patients with chronic periodontitis. Int J Dent Hyg. (2019) 17:292−9. doi: 10.1111/idh.12384
147. Li F, Xu HS. Effects of low level laser combined with basic periodontal therapy on cytokines and LPS, leptin in gingival crevicular fluid of diabetes mellitus complicated with chronic periodontitis patients. Shanghai Kou Qiang Yi Xue Shanghai J Stomatol. (2018) 27:637−40.
148. Demirturk-Gocgun O, Baser U, Aykol-Sahin G, Dinccag N, Issever H, Yalcin F. Role of low-level laser therapy as an adjunct to initial periodontal treatment in type 2 diabetic patients: A split-mouth, randomized, controlled clinical trial. Photomed Laser Surg. (2017) 35:111−5. doi: 10.1089/pho.2016.4117
149. Javed F, Al Amri MD, Al-Kheraif AA, Qadri T, Ahmed A, Ghanem A, et al. Efficacy of non-surgical periodontal therapy with adjunct Nd : YAG laser therapy in the treatment of periodontal inflammation among patients with and without type 2 diabetes mellitus: A short-term pilot study. J Photochem Photobiol B. (2015) 149:230−4. doi: 10.1016/j.jphotobiol.2015.06.013
150. Loe H, Silness J. Periodontal disease in pregnancy. i. prevalence and severity. Acta Odontol Scand. (1963) 21:533−51.
151. Silness J, Loe H. Periodontal disease in pregnancy. ii. correlation between oral hygiene and periodontal condtion. Acta Odontol Scand. (1964) 22:121−35.
152. Yang L, Liu G, Jiang D, Lin G, Ren Z, Fan H, et al. Effect of near-infrared laser treatment on improving erectile function in rats with diabetes mellitus. Andrology. (2023) 1472–83. doi: 10.1111/andr.13422
153. Asghari A, Takhtfooladi MA, Hoseinzadeh HA. Effect of photobiomodulation on ischemia/reperfusion-induced renal damage in diabetic rats. Lasers Med Sci. (2016) 31:1943−8. doi: 10.1007/s10103-016-2073-x
154. Aghamohamdi D, Fakhari S, Farhoudi M, Farzin H. The efficacy of low-level laser therapy in the treatment of bell’s palsy in diabetic patients. J Lasers Med Sci. (2020) 11:310−5. doi: 10.34172/jlms.2020.52
155. Hode L. The importance of the coherency. Photomed Laser Surg. (2005) 23:431−4. doi: 10.1089/pho.2005.23.431
156. Zalevsky Z, Belkin M. Coherence and speckle in photomedicine and photobiology. Photomed Laser Surg. (2011) 29:655−6. doi: 10.1089/pho.2010.2939
157. de Freitas LF, Hamblin MR. Proposed mechanisms of photobiomodulation or low-level light therapy. IEEE J Sel Top Quantum Electron Publ IEEE Lasers Electro-Opt Soc. (2016) 22:7000417. doi: 10.1109/JSTQE.2016.2561201
158. Deana NF, Zaror C, Del Sol M, Bagnato VS, Alves N. Wound contraction rate in excised and unexcised burn wounds with laser photobiomodulation: Systematic review and meta-analysis of preclinical studies. Burns J Int Soc Burn Inj. (2023) 49:261−74. doi: 10.1016/j.burns.2022.05.009
159. Lopes C de CA, Limirio JPJO, Zanatta LSA, Simamoto VRN, Dechichi P, Limirio APHJO. Effectiveness of photobiomodulation therapy on human bone healing in dentistry: A systematic review. Photobiomodulation photomed laser surg. Photobiomodul Photomed Laser Surg. (2022) 40:440−53. doi: 10.1089/photob.2021.0092
160. Ebrahimi P, Hadilou M, Naserneysari F, Dolatabadi A, Tarzemany R, Vahed N, et al. Effect of photobiomodulation in secondary intention gingival wound healing-a systematic review and meta-analysis. BMC Oral Health. (2021) 21:258. doi: 10.1186/s12903-021-01611-2
161. Ribu L, Birkeland K, Hanestad BR, Moum T, Rustoen T. A longitudinal study of patients with diabetes and foot ulcers and their health-related quality of life: wound healing and quality-of-life changes. J Diabetes Complications. (2008) 22:400−7. doi: 10.1016/j.jdiacomp.2007.06.006
162. Ayuk SM, Houreld NN, Abrahamse H. Effect of 660 nm visible red light on cell proliferation and viability in diabetic models in vitro under stressed conditions. Lasers Med Sci. (2018) 33:1085−93. doi: 10.1007/s10103-017-2432-2
163. De Marchi T, Ferlito JV, Ferlito MV, Salvador M, Leal-Junior ECP. Can photobiomodulation therapy (PBMT) minimize exercise-induced oxidative stress? A systematic review and meta-analysis. Antioxid Basel Switz. 27 août. (2022) 11:1671. doi: 10.3390/antiox11091671
164. Ferraresi C. Use of photobiomodulation therapy in exercise performance enhancement and postexercise recovery: true or myth? Photobiomodulation Photomed Laser Surg. (2020) 38:705−7. doi: 10.1089/photob.2020.4948
165. Liebman C, Loya S, Lawrence M, Bashoo N, Cho M. Stimulatory responses in α- and β-cells by near-infrared (810 nm) photobiomodulation. J Biophotonics. (2022) 15:e202100257. doi: 10.1002/jbio.202100257
166. Irani S, Mohseni Salehi Monfared SS, Akbari-Kamrani M, Ostad SN, Abdollahi M, Larijani B. Effect of low-level laser irradiation on in vitro function of pancreatic islets. Transplant Proc. (2009) 41:4313−5. doi: 10.1016/j.transproceed.2009.09.065
Keywords: photobiomodulation, diabetes, neuropathy, wound healing, periodontitis, retinopathy, glycemic control
Citation: Perrier Q, Moro C and Lablanche S (2024) Diabetes in spotlight: current knowledge and perspectives of photobiomodulation utilization. Front. Endocrinol. 15:1303638. doi: 10.3389/fendo.2024.1303638
Received: 28 September 2023; Accepted: 26 February 2024;
Published: 19 March 2024.
Edited by:
Maurizio Delvecchio, University of L’Aquila, ItalyReviewed by:
Yuzhen Wang, People’s Liberation Army General Hospital, ChinaMaria Guadalupe Moreno Treviño, University of Monterrey, Mexico
Copyright © 2024 Perrier, Moro and Lablanche. This is an open-access article distributed under the terms of the Creative Commons Attribution License (CC BY). The use, distribution or reproduction in other forums is permitted, provided the original author(s) and the copyright owner(s) are credited and that the original publication in this journal is cited, in accordance with accepted academic practice. No use, distribution or reproduction is permitted which does not comply with these terms.
*Correspondence: Quentin Perrier, cXBlcnJpZXJAY2h1LWdyZW5vYmxlLmZy