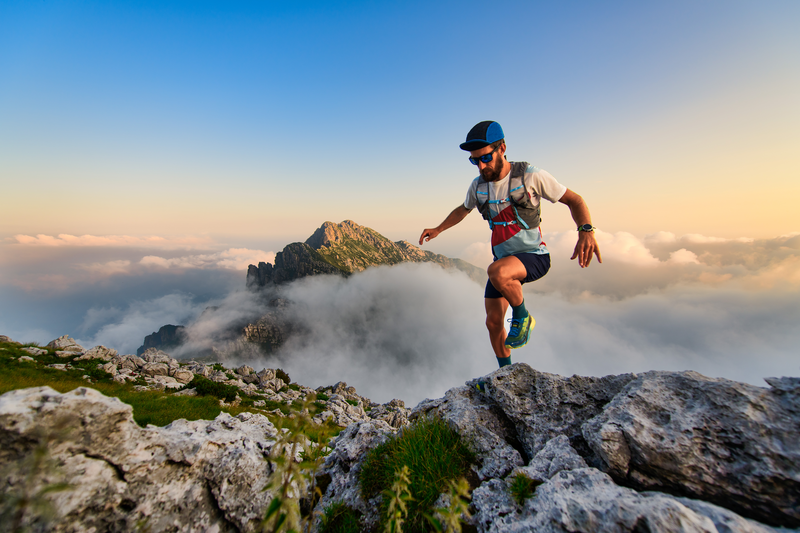
95% of researchers rate our articles as excellent or good
Learn more about the work of our research integrity team to safeguard the quality of each article we publish.
Find out more
REVIEW article
Front. Endocrinol. , 30 January 2024
Sec. Experimental Endocrinology
Volume 15 - 2024 | https://doi.org/10.3389/fendo.2024.1294205
This article is part of the Research Topic Environmental Challenges and Endocrine Dysregulation View all articles
Smog is a form of extreme air pollution which comprises of gases such as ozone, sulfur dioxide, nitrogen and carbon oxides, and solid particles including particulate matter (PM2.5 and PM10). Different types of smog include acidic, photochemical, and Polish. Smog and its constituents are hazardaous to human, animals, and plants. Smog leads to plethora of morbidities such as cancer, endocrine disruption, and respiratory and cardiovascular disorders. Smog components alter the activity of various hormones including thyroid, pituitary, gonads and adrenal hormones by altering regulatory genes, oxidation status and the hypothalamus-pituitary axis. Furthermore, these toxicants are responsible for the development of metabolic disorders, teratogenicity, insulin resistance, infertility, and carcinogenicity of endocrine glands. Avoiding fossil fuel, using renewable sources of energy, and limiting gaseous discharge from industries can be helpful to avoid endocrine disruption and other toxicities of smog. This review focuses on the toxic implications of smog and its constituents on endocrine system, their toxicodynamics and preventive measures to avoid hazardous health effects.
The term “smog” was first devised during the early 20th century to define the low-level pollution covering the city of London. The word “smog” originated from two English words “smoke and fog” (1). Acidic (London smog), photochemical (Los Angeles type), and Polish smog are the three forms of smog. Acidic smog usually ascends from November to January when the atmospheric pressure is low, and air temperature remains a few degrees centigrade beyond zero due to which the concentration of pollutants increases near the ground. A combination of high humidity, low temperature, and pollutants created by the combustion of fossil fuels such as oil, gas, and coal, leads to the formation of smog (2, 3). The core components of acidic smog are oxides of sulfur, nitrogen, and carbon, carbon black, and suspended particulate matter (PM) which are produced by small heating devices when the combustion process is away from ideal conditions (Figure 1) (1).
Photochemical smog is principally a brown haze formed during summer due to intense sunlight and high temperature (>28-30°C) in the subtropical region (4). Biogenic and anthropogenic sources mainly contribute to photochemical smog. Biogenic sources include the production of nitrogen oxides by lightning, bushfires, microbial processes, and the vapors of volatile organic compounds produced from naturally occurring compounds such as terpenes. Nitrogen oxides formed by motor vehicles or power stations through inadequate combustion or burning of fossil fuels and volatile organic compounds are anthropogenic sources of photochemical smog. The primary constituents of photochemical smog are the oxides of nitrogen, carbon monoxide, carbon dioxide, volatile hydrocarbons, and ozone (Figure 1).
Polish smog mostly ensues during frosty season and time of eastern circulation at high pressure and weak winds (5, 6). Polish smog contains suspended PMs such as PM 1 μm, PM 2.5 μm, PM 2.5-10 μm, and various polycyclic aromatic hydrocarbons (PAH) including benzo[a]pyrene as mentioned in Figure 2 and Table 1. These PMs are the most harmful components of smog the chemical composition of which varies significantly (Veras et al., 2010). PM2.5 is composed of sulfates, ammonia, organic compounds, elemental carbon, and metals. PM2.5-10 comprises of crystalline materials such as silicon, iron, calcium, aluminum, and their oxides, large salt particles, and plant debris in the atmosphere (1). The concentration of different components of smog has been summarized in Table 1. The mechanism of formation of different types of smog and their adverse impact on the endocrine and other systems of the human body are described in Figure 2.
The developing countries of South Asia, Southeast Asia, North Africa, Middle East, and South America are mostly affected by air pollution which display low air quality due to widespread industrial activities, motor vehicles, and fossil fuel burning (13). An increase in air pollution has reduced the quality of life, caused air-borne diseases, and reduced the life expectancy (14). In addition, gaeous components and PM2.5 present in smog are associated with endocrine disruption in affected individuals. Endocrine-disrupting chemicals (EDCs) in smog are frequently linked to the reduction in sperm quality, irregular menstrual cycles, and fertility issues. EDCs also interfere with the functions of thyroid and adrenal glands (15) (16). EDCs alter the synthesis and transport of endocrine hormones by affecting the conjugating enzymes or competing for binding to carrier proteins (17). Furthermore, EDCs alter the metabolism of hormones and compete for binding sites by mimicking steroid hormones, especially estrogens and androgens (Darbre 2018). The mechanism of EDCs-induced endocrine disruption is depicted in Figure 3. Therefore, the current review is aimed at identifying the toxic implications and toxicodynamics of smog and its constituents related to the endocrine system. Moreover, this review appraises the available data regarding adverse health implications on humans including the special population groups such as the elderly, young adolescents, and pregnant individuals.
Figure 3 Mechanism of endocrine damage by Endocrine disrupting chemicals (EDC). (A) Mechanism of EDC induced endocrine disruption (B) molecular basis for EDC induced endocrine disruption.
For finding relevant studies regarding the endocrine disruptive effect of smog and its constituents, a wide range of search terms, including but not limited to “ smog”, “smog constituents”, “Acidic smog”, Polish smog” and “Photochemical smog” were searched from different databases such as Scopus, google scholar, and PubMed. For the study of the effects of PM2.5 on various endocrine systems, the search terms such as “PM2.5 and endocrine”, “PM2.5 and pituitary”, “PM2.5 and adrenal”, “PM2.5 and thyroid”, “PM2.5 and estrogen”, “PM2.5 and testosterone” were used to extract data from Pubmed and Scopus. After identifying all records, duplicate records, review articles and animal studies were excluded. Moreover, those articles with missing full text were also excluded from the study. The remaining articles were assessed for the study participants, exposure type, and results. The flow diagram depicting the process for the selection of research studies is shown in Figure 4.
Smog has extensively affected the quality of life by not only instituting numerous health issues but also aggravating the already existing diseases. Gaseous components of smog include ozone, carbon monoxide (CO2), sulfur dioxide, nitrogen oxides, and others.
Several environmental toxicants produce acute and stress-related changes through direct or indirect action (18). Several gases such as ozone and sulfur dioxide alter the metabolism of carbohydrates. Acute ozone exposure causes release of stress hormones through the suppression of hypothalamic-pituitary axis (HPA) (19).
Long-term elevation of stress hormones i.e., leptin and corticosteroids in the circulation results in metabolic diseases and systemic inflammation (20). The HPA axis is involved in acute O3-induced extra-pulmonary effects. In addition to the induction of glucose intolerance, O3 increases the level of leptin and epinephrine (21). Leptin and epinephrine play a pivotal role in the regulation of body temperature and weight (22). Stress hormones target the liver and pancreas to alter glucose and lipid metabolism through the activation of cellular glucocorticoid and adrenergic receptors (23, 24).
The exposure of 0.75 ppm O3 altered the function of parathyroid glands (25). The 48 hours of exposure to 0.75 ppm O3 in the rabbits’ parathyroid glands resulted in hyperplastic parathyroiditis. Inhalation of O3 initiated the autoimmune reaction that resulted in the permanent destruction of the parathyroid glands. At the initial exposure, parathyroid glands were compact and in a cluster arrangement but at a later stage, they were congested and enlarged due to ozone exposure (26). However, evidences of ozone-induced parathyroid toxicity in human are unavailable.
Ozone also induces plasma membrane remolding by stimulating the adrenergic nervous system through the release of catecholamine (20). LH is also responsible for the production of testosterone from the Leydig cell in males.
Ozone exposure reduces progesterone and increases estrogen by post-exposure effect on pituitary gland to alter luteinizing hormone (LH) (31). Exposure to 0.3 ppm O3 causes menstrual cycle disturbance in females by fluctuating LH release. These disturbances affect ovulation, fertilization, maintenance of endometrial lining, and implantation of fertilized ovum. Exposure to O3 at level more than 0.3 ppm caused menstrual disturbances that led to a sterile cycle. In addition, O3 exposure is inversely related to the ovarian reserve. It is found that exposure to O3 is positively associated with low excretion of anti-Muellerian hormone (AMH), an important marker for ovarian reserve (32). However, some studies showed that exposure to O3 was effective in treating female infertility. It can protect from inflammatory problems such as endometritis, and vaginitis, and reduces the chances of ischemia-induced injury in ovaries (33). Another study has shown that O3 therapy might enhance ovarian function by improving oocyte quality and altering the genes involved in the synthesis of steroidal hormones (34). However, ozone, when used as a therapeutic agent, should be generated in controlled concentration from pure oxygen and the intake should be monitored. Despite the compelling therapeutic evidence, future research is necessary to critically explore whether the effects of O3 in the female reproductive system are beneficial or not (35).
The gaseous constituents of smog have significant adverse consequences on pregnancy if a pregnant mother is exposed to outer ambient air pollutants for a long duration due to changes in hormones’ level (36). Preterm birth, preeclampsia, and small-for-gestational age (SGA) are the adverse outcomes of exposure to smog air pollutants (Figure 5). SGA is described as infants with a weight less than 10% at a specified gestational age (37). Previous study showed that the incidence of preterm birth and pre-eclampsia were 4.4 and 2.7% respectively in pregnant women upon long term exposure to smog air, especially ozone. It was further confirmed that a positive association was found between the first trimester, O3, and preterm birth. A positive correlation was also evident between O3 and pre-eclampsia when the concentration of O3 was increased to more than 10 µg/m3 (38).
Air-borne pollutants are highly associated with the risk to diabetes. Acute exposure to O3 changed the metabolism of rats and increased the risk factors associated with metabolic alterations. Additionally, it was found that a high O3 level altered glucose homeostasis by changes in the insulin signaling pathway and liver endoplasmic reticulum stress in rats (39).
Exposure to Sulfur dioxide during pregnancy can cause birth defects and abortion. Short-term exposure a high concentration of SO2 is life-threatening (40). Its exposure to human causes reproductive and developmental effects as mentioned in Figure 6. A study carried out in Finland’s industrial areas revealed that SO2 exposure had resulted in spontaneous abortion (41). Another study in China demonstrated a link between exposure to SO2 during pregnancy and reduced infants’ birth weight (42). Exposure of pregnant rabbits to SO2 resulted in minor skeletal variation and delayed bone hardening (43). In addition, exposure to SO2 caused a significant, dose-related decrease in plasma insulin levels (44).
Figure 6 Effect of smog exposure on the reproductive system in males and females. Exposure to SO2, PM2.5 or NO triggers various cell-damaging processes such as oxidative stress, inflammation, apoptosis, and DNA methylation leading to altered sperm quality and concentration in males while induce abortion and alters hormone level in females. Low birth weight, birth defects and hypertension in mothers are the consequences of their exposure during pregnancy etc.
Sulfur dioxide exhibits reproductive toxicity in male animals. An investigation in the Czech Republic showed that a high-level exposure to SO2 caused sperm abnormalities such as a decreased ability to move (45). In an experimental study in mice, it was found that an exposure to 5 mg/m3 SO2 increased sperm malformation, decreased sperm count, and exhibited aberrant pathological changes in testicles. Additionally, mice exposed to SO2 also increased TUNEL-positive cells, caspase-3 activity, spermatogenic cell counts, hydrogen peroxide (H2O2) and melondialdehyde (MDA) content, and decreased superoxide dismutase (SOD) activity. It was demonstrated that exposure to SO2 altered the expression of steroidogenic-related genes (LHR, StAR, and ABP), lowered serum testosterone levels, and altered the mRNA levels of Bax and Bcl-2 in mice. In conclusion, exposure to SO2 exhibited male reproductive toxicity through induction of apoptosis, lipid dysregulation, and generation of reactive oxygen species (ROS). These outcomes provided a fresh theoretical framework for understanding the interference of SO2 with spermatogenesis and infertility (46).
Gaseous neuromodulators, such as nitrogen monoxide (NO) and carbon monoxide (CO), regulate the hypothalamic release of neuropeptides. The stimulatory and inhibitory effects on the HPA depend upon diverse factors, such as type of stress, intensity of stress, and species. NO regulates the non-adrenergic and non-cholinergic relaxation of smooth muscles in the corpora cavernosa and gastrointestinal tract (47). Exposure to CO inhibits the secretion of corticotropin-releasing hormone (CRH) and oxytocin while raising the secretion of gonadotrophin-releasing hormone (GnRH) and prostaglandin (PGE2) in the rat hypothalamus. Stimulation of the HPA axis increases the body temperature which is termed stress fever (48).
NO2 is one of pivotal factors that contribute to male infertility. Boggia and his coworkers reported that NO2 exposure had led to reduced sperm motility, quality and quantity in exposed males as compared to non-exposed males. Negative effects on sperm quality were noticed even if NO2 concentration was below than recommended allowed limit (49, 50). Investigation on the male genome has shown that NO2 had broken the strands of the sperm DNA ultimately leading to infertility (51).
Outdoor exposure to NO2 is closely associated with lung cancer in females through increased activation of estrogen receptors. A few studies have demonstrated that estrogen receptor activation promoted tumor formation by several genomic and non-genomic pathways (52). Female lung cancer is closely associated with the activation of estrogen receptor pathway and NO2 is implicated to activate this pathway (53).
Various studies have revealed the deleterious effects of exposure to NO2 against pregnancy. Exposure to air pollutants has resulted in increased DNA fragmentation. These toxicants also cause alterations in the placenta’s DNA. Early pregnancy exposure to NOx changed the placental DNA methylation leading to placental immaturity (54, 55). Chronic interaction with NOx is also linked with diabetes mellitus. Leiser et al. reported that exposure to NO2 up to 100 ppb for 7 days had increased the chances of miscarriage by up to 16% (56).
The PMs are comprised of dust, soil, acids, metals, and organic compounds. The PMs are categorized according to their size or diameter i.e., PMs of 2.5-10 µm are considered coarse particles, PMs of less than 2.5 µm are fine particles, and PMs of less than 0.1 µm are ultrafine particles (57). A high concentration of PMs, usually PM2.5 dust, constitutes smog in the winter season. PMs produce systemic effects by influencing metabolic homeostasis. Long-term exposure to PM increases the disease progression (58, 59). Exposure to PM2.5 induces insulin resistance and stimulates the HPA axis which consequently intensifies glucocorticoid production. In addition, PM2.5 increases response of the HPA axis to psycho-social stress especially in adolescent girls who experience high social stress (60).
Thyroid hormones such as thyroxine (T4), and triiodothyronine (T3) are secreted by the thyroid gland under the influence of thyroid stimulating hormone (TSH) secreted by pituitary glands. Industrial chemicals, tobacco smoke, and air pollution impact thyroid hormone production. Thyroid hormones are responsible for fetal growth, metabolism, and neuro-development. Low and high levels of thyroid hormone unfavorably affect child’s growth. PM2.5 exposure may change the thyroid function of a newborn (20, 61, 62). It was found that the exposure to PM2.5 ≥ 16 µg/m3 concentration in air had raised the T4 level to 7.5 percent in the blood. Moreover, PM2.5 increased the conversion of T4 to T3 (20, 63). This might be due to the changes in genes encoding for steroid hormone biosynthesis and glycerolipid metabolism caused by PM2.5 (64).
Exposure to pollutants at the time of birth or during childhood may increase the risk of cancers in children, the most common are leukemias and lymphomas. Ambient PM air pollution is also related to a higher incidence of thyroid cancer (65). Moreover, other studies have revealed that the chronic exposure to air pollution may produce alterations in normal ovarian function e.g., estrogen-like effect or gene mutation, which can lead to ovarian cancer (66). Additionally, transplacental exposure to PM is connected to higher placental mutation rate and such epigenetic alterations may be a reason for placental carcinogenicity (55).
The toxic effect of ambient air pollution may lead to insulin and glucocorticoid resistance by interfering with the signaling pathways involved in inflammation. It was found that increased level of TNF-α caused inhibition of the insulin signaling pathway (67). PM2.5-mediated insulin resistance results in the accumulation of fatty acids in the liver and dysregulates glucose utilization by skeletal muscles through increased expression of C-C Chemokine receptor (CCR-2) and reduced GLUT-4 (68). Another study suggested that the blockage of the glucocorticoid or HPA axis by exposure to smog pollutants may lead to the overproduction of cytokines and inhibition of the glucocorticoid-regulated gene, CYP3A5 (69).
PM2.5 exposure can lead to reproductive damage in males by inducing apoptosis and inflammatory pathways. PM2.5 adversely affects the male reproductive function (Liao et al., 2019). Exposure to PM may result in increased expression of various cytokines and methylation at CpG sites. Exposure to PM2.5 specifically increased the expression of MCP-1, MCP-3, CD40, FGF-2, and other related genes in young adults (70). Moreover, interleukins, Toll-like receptor genes, and genes related to apoptosis are upregulated due to smog exposure. Expression of xenobiotic genes and cytochrome P450 genes is altered by exposure to PM2.5. Genes associated with cancer development such as TGFβ are overexpressed resulting in the activation of the linked signaling pathways (71).
PM2.5 damages ovarian granulosa cells and oocytes by decreasing the levels of AMH and increasing the expression of inflammatory and apoptotic proteins (72). Smog pollutants can delay normal conception and in-vitro fertilization (IVF) (73). The effects of PM2.5 on the female reproductive system include the dysfunction of ovaries and transportation to the embryo or mutation in the embryo’s DNA (74). Data from an infertility clinic showed that exposure to PM2.5 was associated with loss in normal ovarian function resulting in infertility (75).
Congenital hypothyroidism (CH) is the most common endocrine disorder in newborns, affecting 1 in 2000-4000 newborns. Air pollution is also responsible for CH (76). It was found that a exposure to a high concentration of PM is associated with CH which causes delayed physical and mental development and may affect the normal functioning of kidneys, lungs, and heart. The risk of preterm birth, fetal death, low birth weight, congenital imperfections, and macrosomia fetus is increased due to PM2.5 and PM10 exposure. Moreover, the reduction in exposure to PM2.5 was associated with an increased survival rate of newborns (Figure 5) (38). Similarly, an exposure to PM2.5 up to 10 mg/m3 for seven days was accompanied by miscarriage (56). The studies regarding the effect of smog and its constituents on different endocrine systems are listed in Table 2.
Various EDCs present in the environment exert a negative effect on male reproductive health. These factors interfere with the normal hormonal balance especially a reduction in semen production (106). A recent study determined that PM2.5 exposure changed the integrity of the blood-testis barrier by ROS production and caused the loosening of tight junctions (107). The PAHs and heavy metal ions present in the particulate matter exert estrogenic, antiestrogenic, and antiandrogenic activities to disrupt normal hormone functions (59).
During pregnancy and lactation, PM2.5 exposure is linked to metabolic disorders in neonates. Exposure to PM2.5 can elevate the blood pressure in the offspring which is mediated by alterations in the transcriptional activity, DNA methylation, oxidative stress and inflammatory response (108). Studies have shown that maternal exposure to PM may increase the incidence of obesity and other metabolic disorders such as fatty liver disease, diabetes militus, and insulin resistance (109). Methylation of leptin promotor and increased formation of hypothalamic neuropeptide Y in males are responsible for PM-induced obesity. PM exposure causes a reduction in the birth weight of the offspring but in the long run it induces obesity during adulthood (110).
Several studies have demonstrated that the risks of depression, anxiety, and dementia are increased in adolescent girls, elderly individuals, and pregnant females exposed to PM2.5. It was found that the risk of depression in pregnant individuals exposed to PM2.5 during the third trimester was increased due to altered HPA axis (80). Moreover, pregnant individuals exposed to PM2.5 displayed dose-dependent mild thyroid dysfunction which also contributed to depression (84). Estrogen plays a substantial role in cognitive function. Different studies have reported that healthy elderly females were associated with a higher risk of depression and episodic memory decline while those with genetic polymorphism in estrogen receptors showed a higher risk of dementia (85, 87). In addition, adolescent females exhibited a higher response to social stress and showed symptoms of anxiety due to exposure to PM2.5 (60).
PAHs, for instance, benzo[a]pyrene and dimethyl Benz[a]anthracene are the components of Polish smog that are deposited on land and water in dry form. Benzopyrene exists in food, dust, air, water, soil, and smoke. The air contaminated with benzopyrene exerts a profound adverse impact on the health of children and employees of aluminum and coke–oven factories (111). Benzopyrene belongs to the class 1 carcinogen and is a strong mutagenic, genotoxic, teratogenic, anti-fertility and neurotoxic agent. It involves in depurination of DNA, generation of ROS, stimulation of aryl hydrocarbon receptors (AhR), and numerous other epigenetic changes that collectively result in the toxic effects.
TSH secreted by the pituitary gland activates the synthesis of T4 and T3 in the thyroid gland which are essential for regulating development, growth, morphogenesis, basal metabolism, reproduction, and osmoregulatory functions (112). Some studies have suggested that PAHs interrupt the metabolic functions of thyroid hormone (113).
Some PAH like 3-methylcholanthrene, are carcinogenic, and disruptors of thyroid function in animals which alter the structure of the thyroid gland and synthesis of thyroid hormones (114). Small thyroid follicular with cuboidal or columnar epithelial cells have additional secretory activity as compared to large follicles with squamous epithelium. Exposure to benzo[a]anthracene caused thyroid follicles to become large with short epithelium. Furthermore, exposure to benzo[a]anthracene changed the plasma levels of TSH, T4 and T3. In fish, exposure to these EDC caused enlargement of the thyroid gland with an indication of hypothyroidism (113, 115).
Likewise, polyhalogenated aromatic hydrocarbons (PHAH) are considered EDC due to alteration in the thyroid and retinol functions in birds (61, 116). Additionally, these hydrocarbons may interfere with thyroid transport proteins i.e., transthyretin (TTR) and retinol binding protein (RBP). Some PHAHs have structural similarities to T4 and have greater binding affinity to TTR than T4 (117).
Benzopyrene contamination in the atmosphere is a leading threat to health due to hormone receptor binding and activation, post-receptor signaling pathway, and involvement of co-factors (118, 119). Benzopyrene impedes the functions of nuclear hormone receptors i.e., estrogen, androgen, progesterone, thyroid, and retinoid receptors, membrane receptors, non-steroid receptors, and orphan receptors (118, 120).
Testosterone production is stimulated by LH produced by the pituitary gland in response to GnRH (121). Benzo[a]anthracene reduced the serum and intratesticular testosterone level (122). Tian et al. reported that exposure to benzo[a]pyrene had reduced the level of 17β-estradiol and progesterone in ovaries and decreased the expression of metabolizing enzyme, hydroxysteroid dehydrogenase (123).
The production of hormones and exchange of nutrients take place through syncytiotrophoblasts (STs) that are exposed to maternal blood. These STs are formed by the fusion of cytotrophoblasts. Any abnormal change in the formation of STs may cause premature birth or abnormal fetal development. It is previously explored that the trophoblast exposure to formaldehyde was associated with oxidative stress that adversely affected the differentiation and fusion of trophoblasts (124). Cathey et al. studied 659 pregnant women and observed a positive association between PAH and cortisone-releasing hormone, progesterone, and thyroid T3 hormone; however, a negative relation was found with testosterone that consequently affected the physiology of pregnancy (125). Prenatal exposure to PAHs may lead to adverse effects on the fetus, including weight loss and, reduced height and head circumference at birth. PAHs have been found in pregnant women’s blood which provides evidence that they can cross the placenta (126).
Bisphenols, phthalates, and aldehydes are included in the list of EDCs that constitute the smog. These chemicals have the potential to strongly bind to the hormone receptors resulting in the blockade of the hormone function. For instance, bisphenols can bind to the estrogen receptors and reduce the production of estradiol, estrone, testosterone, and androstenedione (127). Some studies have reported a reduced level of progesterone and estradiol in animals exposed to phthalates. In these studies, GnRH was found to be elevated, indicating that these chemicals disrupt the function of the HPA (128).
Exposure to phthalates and other toxicants also affects thyroid function and growth hormone homeostasis (129). This effect might be modulated by thyroid autoantibodies (130). The impact of smog components on the different organs of the endocrine system is described in Figure 7.
There is strong evidence that exposure to formaldehyde is associated with reproductive and developmental toxicities as it can cross the placental barrier. Menstrual irregularities and infertility were observed in females exposed to toxic level of formaldehyde (124). Some studies have demonstrated that a high cortisol level is significantly associated with exposure to smog pollutants. Cortisol plays a significant role in cognition and depression. The response starts in the brain and activates the hypothalamus-pituitary-adrenal axis to produce more cortisol which triggers inflammatory and apoptotic gene expression. This eventually leads to dementia and depression (131).
Several studies have documented the combined effect of smog toxicants on human endocrine system. These endocrine effects include an increase in stress hormones, infertility and insulin resistance.
It is evident from previous studies that a high concentration of NO2 and PM2.5 in the air was strongly linked to increased level of salivary cortisol level, CRH, and adrenocorticotropic hormone (ACTH) in adults. Moreover, the combined exposure to PM2.5 and NO2 was evaluated which showed an increased risk of cancer among women with a higher familial E2 polymorphism exposed only to NO2 only. It was also found that exposure to NO2, O3, and PM2.5 in young male individuals resulted in significant changes in estradiol levels (103). In addition, NO2, CO, SO2, or PM2.5 exposure exhibited a significant change in morning cortisol among children (94). PM10 exposure increased the risk of osteoporosis among women over 40 years of age when they were evaluated for exposure to NO2, CO, SO2, or PM2.5, and PM10. In addition, reduced testosterone, progesterone, and FSH were evident in middle-aged male individuals when exposed to PM2.5, NO2, and SO2 or CO (98).
Various animal experiments have displayed that long-term exposure to ambient air pollutants can result in the reduction of male fertility. It was found that the chronic exposure to PAHs and PM2.5 impaired sperm function and spermatogenesis (132). Exposure to SO2, NO, CO, and CO2 also decreased the sperm quality (132). Even at low concentration, NOx may affect sperm motility and sperm morphology (133). Similarly, SO2 is a proven toxicant for the reproductive organs of mammals (134). Spermatogenesis may consequently improve with a reduction in the level of these toxic oxides (74, 135).
An elevated level of PM, SO2, and O3 may be associated with insulin resistance and metabolic alterations that lead to diabetes mellitus, obesity, and related health risks by triggering oxidative stress, endoplasmic reticulum stress, and activation of c-Jun N-terminal kinase signaling (136). The gaseous components of smog tend to cause more metabolic disorders than PMs (137).
Factors responsible for the formation of smog are globalization, urbanization and heavy transport usage, elevation in temperature, sunny climate, bricks formation, and decline in forestation. There are numerous techniques and procedures to control smog formation. There is a need to convert the toxic volatile compounds emitted from factories into less toxic volatile compounds by changing the operating conditions and recycling the stream to decrease air pollution. Reduction in particle size also helps to mask the hazardous effects of smog. Particle size can be reduced by a gravity chamber, cyclone filtration, bag filters, and precipitate scrubbers (138).
Wet scrubbers should be used for absorption, adsorption, chemical oxidation, and bio-filtrations of gases and vapors discharged from chemical industries. Fossil fuel engines must be replaced with alternative engines. Hydrogen fuel additives are essential to diminish the discharge of pollutants and upsurge the combustion cycle. Photocatalytic treatment must be carried out to reduce particle size and nitrogen oxide pollution (139). Furnaces, condensers, carbon absorbers, scrubbers, and texture channels are additional devices that should be used for air pollution control. The main source of smog is open burning. There should be strict prohibitions and legislation on the open burning of rice stubble, solid waste, and other dangerous materials.
Environmental Protection Agencies (EPA) should issue policies to Air quality index departments to control air pollutants such as PM2.5. EPA should impose rules and regulations for oil refineries to produce Sulphur free oil to decline the level of PM and SO2 gas (140). A single tree fixes approximately 20 kg of CO2 annually. EPA should work with the forest department to intensify the growth of plants as plants are environmentally safe agents to fix carbon and other toxic elements thus declining smog. Large smoke industries should be shut down to reduce the discharge of pollution. The federal government should issue directions to all vehicle manufacturers for the installation of a catalytic converter in motor vehicles to prevent toxic vehicular emissions such as NOx, SOx and COx (141).
This review showed that the gaseous components and PM2.5 present in smog significantly increased the risk of endocrine toxicity through disruption of the HPA axis. These gases and PM can alter the expression of proinflammatory cytokines and metabolizing enzymes to exhibit insulin resistance and metabolic alterations. These chemicals alter the level of sex hormones to predispose infertility in males and females and can culminate in birth defects.
Smog, nowadays, has become a global issue with alarming human health risks. Smog and its constituents are associated with altered functioning of the ovary, testis or pituitary, adrenal, and thyroid glands. Moreover, diabetes mellitus, insulin resistance, infertility, reduced motility and DNA damage in sperms, reduced conception, and DNA methylation are the consequences of altered gene expression due to smog exposure. Preterm birth, preeclampsia, and small for gestational age are the adverse outcomes of exposure to smog air pollutants. Suitable steps should be taken to avoid smog exposure and related health issues. Avoiding wood and coal burning, reducing energy use, using renewable sources of electricity, using public transport, implementing strict limitations on industrial gaseous discharge, and staying inside during times of poor air quality can help in reducing toxic exposure to smog. Federal agencies should enforce environmental protection laws to achieve a smog-free atmosphere or attempts should be made to reduce the exposure duration, amount, and risk of damage to the population.
It is evident from the previous literature that PM2.5 and gaseous constituents result in neurodegeneration as well as psychiatric disorders such as depression and anxiety through altered HPA axis. Learning and memory deficits and psychomotor dysfunction are closely linked to endocrine disruption caused by smog. Therefore, we strongly suggest that further research should be guided to establish a link between PM2.5 and neuroendocrine, CNS toxicity, and hormonal disruption.
AS: Conceptualization, Data curation, Supervision, Writing – original draft, Writing – review & editing. TA: Conceptualization, Data curation, Writing – original draft, Writing – review & editing. MA: Conceptualization, Data curation, Writing – original draft, Writing – review & editing.
The author(s) declare that no financial support was received for the research, authorship, and/or publication of this article.
The authors declare that the research was conducted in the absence of any commercial or financial relationships that could be construed as a potential conflict of interest.
The author(s) declared that they were an editorial board member of Frontiers, at the time of submission. This had no impact on the peer review process and the final decision.
All claims expressed in this article are solely those of the authors and do not necessarily represent those of their affiliated organizations, or those of the publisher, the editors and the reviewers. Any product that may be evaluated in this article, or claim that may be made by its manufacturer, is not guaranteed or endorsed by the publisher.
1. Czerwińska J, Wielgosiński G, Szymańska O. Is the polish smog a new type of smog? Ecol Chem Eng (2019) S 26:465–74. doi: 10.1515/eces-2019-0035
2. Maji KJ, Ye W-F, Arora M, Nagendra SS. Ozone pollution in Chinese cities: Assessment of seasonal variation, health effects and economic burden. Environ pollut (2019) 247:792–801. doi: 10.1016/j.envpol.2019.01.049
3. Mira-Salama D, Grüning C, Jensen N, Cavalli P, Putaud J-P, Larsen B, et al. Source attribution of urban smog episodes caused by coal combustion. Atmospheric Res (2008) 88:294–304. doi: 10.1016/j.atmosres.2007.11.025
4. Muilwijk C, Schrijvers P, Wuerz S, Kenjereš S. Simulations of photochemical smog formation in complex urban areas. Atmospheric Environ (2016) 147:470–84. doi: 10.1016/j.atmosenv.2016.10.022
5. Zawada M, Starostka-Patyk M. Energy efficiency in the context of low-stack emissions reduction on the example of the city of czestochowa. Transportation Res Proc (2016) 16:587–97. doi: 10.1016/j.trpro.2016.11.055
6. Wielgosiński G, Czerwińska J, Namiecińska O, Cichowicz R. Smog episodes in the Lodz agglomeration in the years 2014-17E3S web of conferences EDP Sciences. E3S Web of Conferences (2018) 28:01039. doi: 10.1051/e3sconf/20182801039
7. Henriquez AR, Snow SJ, Jackson TW, House JS, Motsinger-Reif AA, Ward-Caviness CK, et al. Glucose dynamics during ozone exposure measured using radiotelemetry: stress drivers. BioRxiv (2021) 2021:471963. doi: 10.1101/2021.12.09.471963
8. Snow SJ, Broniowska K, Karoly ED, Henriquez AR, Phillips PM, Ledbetter AD, et al. Offspring susceptibility to metabolic alterations due to maternal high-fat diet and the impact of inhaled ozone used as a stressor. Sci Rep (2020) 10:16353. doi: 10.1038/s41598-020-73361-0
9. Bukowska B, Mokra K, Michałowicz J. Benzo [a] pyrene—Environmental occurrence, human exposure, and mechanisms of toxicity. Int J Mol Sci (2022) 23:6348. doi: 10.3390/ijms23116348
10. Li J, Fu Q, Huo J, Wang D, Yang W, Bian Q, et al. Tethered balloon-based black carbon profiles within the lower troposphere of Shanghai in the 2013 East China smog. Atmospheric Environ (2015) 123:327–38. doi: 10.1016/j.atmosenv.2015.08.096
11. Breeze P. Combustion plant emissions: Sulfur dioxide, nitrogen oxides, and acid rain. In: Electricity Generation and the Environment. Amsterdam, The Netherlands: Elsevier (2017).
12. Teil M-J, Moreau-Guigon E, Blanchard M, Alliot F, Gasperi J, Cladière M, et al. Endocrine disrupting compounds in gaseous and particulate outdoor air phases according to environmental factors. Chemosphere. (2016) 146:94–104. doi: 10.1016/j.chemosphere.2015.12.015
13. Mannucci PM, Franchini M. Health effects of ambient air pollution in developing countries. Int J Environ Res Public Health (2017) 14:1048. doi: 10.3390/ijerph14091048
14. Shaddick G, Thomas ML, Mudu P, Ruggeri G, Gumy S. Half the world’s population are exposed to increasing air pollution. Atmospheric Sci (2020) 3:23. doi: 10.1038/s41612-020-0124-2
15. Darbre PD. Overview of air pollution and endocrine disorders. Int J Gen Med (2018) 11:191. doi: 10.2147/IJGM.S102230
16. Rosol TJ, DeLellis RA, Harvey PW, Sutcliffe C. Endocrine SYSTEMHASCHEK and Rousseaux’s Handbook of Toxicologic Pathology Amsterdam (Netherlands): Elsevier. (2013). pp. 2391–492.
17. Guarnotta V, Amodei R, Frasca F, Aversa A, Giordano C. Impact of chemical endocrine disruptors and hormone modulators on the endocrine system. Int J Mol Sci (2022) 23:5710. doi: 10.3390/ijms23105710
18. Masan J, Sramka M, Rabarova D. The possibilities of using the effects of ozone therapy in neurology. Neuroendocrinol Lett (2021) 42:13–21.
19. Kaur J, Jhamaria C. Urban air pollution and human health: a review. Curr World Environ (2021) 16:362. doi: 10.12944/CWE.16.2.04
20. Afzaal M, Bashir N, Rasheed R, Khan WUD, Mazhar I, Iqbal SS. Hormones-Active Substances Environmental Micropollutants. Amsterdam (Netherlands): Elsevier (2022) p. 151–81.
21. Kodavanti UP. Stretching the stress boundary: Linking air pollution health effects to a neurohormonal stress response. Biochim Biophys Acta (BBA)-General Subj (2016) 1860:2880–90. doi: 10.1016/j.bbagen.2016.05.010
22. Perry RJ, Lyu K, Dong J, Li X, Yang Y, Qing H, et al. Leptin mediates postprandial increases in body temperature through hypothalamus–adrenal medulla–adipose tissue crosstalk. J Clin Invest (2020) 130:2001–16. doi: 10.1172/JCI134699
23. Miller DB. Ozone Induces Systemic Metabolic Derangement Through Neuronal Stress Mechanisms. North Carolina (USA): The University of North Carolina at Chapel Hill (2016).
24. Shore SA. The metabolic response to ozone. Front Immunol (2019) 10:2890. doi: 10.3389/fimmu.2019.02890
25. Capen CC. Chemically-Induced Injury of the parathyroid gland: pathophysiology and mechanistic C onsiderations. Endocrine Toxicol CRC Press (1997) 3:13–54.
26. Kovacs K, Bell CD, Juco J, Rotondo F, Anderson J. Parathyroid chief cell adenoma associated with massive chronic parathyroiditis in a woman with hyperparathyroidism. Endocrine Pathol (2007) 18:42–5. doi: 10.1007/s12022-007-0001-0
27. Henriquez A, House J, Miller DB, Snow SJ, Fisher A, Ren H, Kodavanti UP. Adrenal-derived stress hormones modulate ozone-induced lung injury and inflammation. Toxicology and applied pharmacology (2017) 329:249–258.
29. Hu J, Li Y, Zhao T, Liu J, Hu X. M, Liu D, Chang L. An important mechanism of regional O 3 transport for summer smog over the Yangtze River Delta in eastern China. J Ayub Med Coll Abbottabad (2018) 18(22):16239–16251.
30. Teil MJ, Moreau-Guigon E, Blanchard M, Alliot F, Gasperi J, Cladière M, Chevreuil M. Endocrine disrupting compounds in gaseous and particulate outdoor air phases according to environmental factors. Chemosphere (2016) 146:94–104.
31. Fuentes N, Roy A, Mishra V, Cabello N, Silveyra P. Sex-specific microRNA expression networks in an acute mouse model of ozone-induced lung inflammation. Biol sex Dif (2018) 9:1–14. doi: 10.1186/s13293-018-0177-7
32. Martirosyan YO, Silachev DN, Nazarenko TA, Birukova AM, Vishnyakova PA, Sukhikh GT. Stem-cell-derived extracellular vesicles: unlocking new possibilities for treating diminished ovarian reserve and premature ovarian insufficiency. Life (2023) 13:2247. doi: 10.3390/life13122247
33. Merhi Z, Garg B, Moseley-LaRue R, Moseley AR, Smith AH, Zhang J. Ozone therapy: a potential therapeutic adjunct for improving female reproductive health. Med gas Res (2019) 9:101. doi: 10.4103/2045-9912.260652
34. Dias AR, Bitsaktsis C, Emdin D, Bosman L, Smith AH, Merhi Z. Ozone sauna therapy and pulsed electromagnetic field therapy could potentially improve outcome in women with diminished ovarian reserve undergoing assisted reproductive technology. Med gas Res (2023) 13:202. doi: 10.4103/2045-9912.350862
35. Smith NL, Wilson AL, Gandhi J, Vatsia S, Khan SA. Ozone therapy: an overview of pharmacodynamics, current research, and clinical utility. Med gas Res (2017) 7:212. doi: 10.4103/2045-9912.215752
36. Zhai Y, Wang B, Qin L, Luo B, Xie Y, Hu H, et al. Smog and risk of maternal and fetal birth outcomes: A retrospective study in Baoding, China. Open Med (2022) 17:1007–18. doi: 10.1515/med-2022-0489
37. Ramírez V, Bautista RJ, Frausto-González O, Rodríguez-Peña N, Betancourt ET, Bautista CJ. Developmental programming in animal models: critical evidence of current environmental negative changes. Reprod Sci (2022) 30:1–22. doi: 10.1007/s43032-022-00999-8
38. Olsson D, Mogren I, Forsberg B. Air pollution exposure in early pregnancy and adverse pregnancy outcomes: a register-based cohort study. BMJ Open (2013) 3:1–8. doi: 10.1136/bmjopen-2012-001955
39. McGee Hargrove M, Snow SJ, Luebke RW, Wood CE, Krug JD, Krantz QT, et al. Effects of simulated smog atmospheres in rodent models of metabolic and immunologic dysfunction. Environ Sci Technol (2018) 52:3062–70. doi: 10.1021/acs.est.7b06534
40. Samoli E, Aga E, Touloumi G, Nisiotis K, Forsberg B, Lefranc A, et al. Short-term effects of nitrogen dioxide on mortality: an analysis within the APHEA project. Eur Respir J (2006) 27:1129–38. doi: 10.1183/09031936.06.00143905
41. Grippo A, Zhang J, Chu L, Guo Y, Qiao L, Zhang J, et al. Air pollution exposure during pregnancy and spontaneous abortion and stillbirth. Rev Environ Health (2018) 33:247–64. doi: 10.1515/reveh-2017-0033
42. Liu Y, Xu J, Chen D, Sun P, Ma X. The association between air pollution and preterm birth and low birth weight in Guangdong, China. BMC Public Health (2019) 19:1–10. doi: 10.1186/s12889-018-6307-7
43. Murray F, Schwetz B, Crawford A, Henck J, Quast J, Staples R. Embryotoxicity of inhaled sulfur dioxide and carbon monoxide in mice and rabbits. J Environ Sci Health Part C: Environ Health Sci (1979) 13:233–50.
44. Godswill AC, Godspel AC. Physiological effects of plastic wastes on the endocrine system (Bisphenol A, Phthalates, Bisphenol S, PBDEs, TBBPA). Int J Bioinf Comput Biol (2019) 4:11–29.
45. Sram RJ. Impact of air pollution on the health of the population in parts of the Czech Republic. Int J Environ Res Public Health (2020) 17:6454. doi: 10.3390/ijerph17186454
46. Li X, Yi H, Wang H. Sulphur dioxide and arsenic affect male reproduction via interfering with spermatogenesis in mice. Ecotoxicol Environ Saf (2018) 165:164–73. doi: 10.1016/j.ecoenv.2018.08.109
47. Currò D, Preziosi P. Non-adrenergic non-cholinergic relaxation of the rat stomach. Gen Pharmacology: Vasc System (1998) 31:697–703. doi: 10.1016/S0306-3623(98)00096-2
48. Mancuso C, Navarra P, Preziosi P. Roles of nitric oxide, carbon monoxide, and hydrogen sulfide in the regulation of the hypothalamic–pituitary–adrenal axis. J Neurochem (2010) 113:563–75. doi: 10.1111/j.1471-4159.2010.06606.x
49. Boggia B, Carbone U, Farinaro E, Zarrilli S, Lombardi G, Colao A, et al. Effects of working posture and exposure to traffic pollutants on sperm quality. J Endocrinol Invest (2009) 32:430–4. doi: 10.1007/BF03346481
50. Chen Y-A, Chang Y-K, Su Y-R, Chang H-C. Ambient sulfur dioxide could have an impact on testicular volume from a observational study on a population of infertile male. BMC Urol (2020) 20:1–12. doi: 10.1186/s12894-020-00710-6
51. Evenson DP, Wixon R. Environmental toxicants cause sperm DNA fragmentation as detected by the Sperm Chromatin Structure Assay (SCSA®). Toxicol Appl Pharmacol (2005) 207:532–7. doi: 10.1016/j.taap.2005.03.021
52. Niehoff NM, Terry MB, Bookwalter DB, Kaufman JD, O’Brien KM, Sandler DP, et al. Air pollution and breast cancer: an examination of modification by underlying familial breast cancer risk. Cancer Epidemiol Biomarkers Prev (2022) 31:422–9. doi: 10.1158/1055-9965.epi-21-1140
53. Li W, Wang W. Causal effects of exposure to ambient air pollution on cancer risk: Insights from genetic evidence. Sci Total Environ (2023) 912:168843. doi: 10.1016/j.scitotenv.2023.168843
54. Lafuente R, García-Blàquez N, Jacquemin B, Checa MA. Outdoor air pollution and sperm quality. Fertil Steril (2016) 106:880–96. doi: 10.1016/j.fertnstert.2016.08.022
55. Engström K, Mandakh Y, Garmire L, Masoumi Z, Isaxon C, Malmqvist E, et al. Early pregnancy exposure to ambient air pollution among late-onset preeclamptic cases is associated with placental dna hypomethylation of specific genes and slower placental maturation. Toxics (2021) 9:338. doi: 10.3390/toxics9120338
56. Leiser CL, Hanson HA, Sawyer K, Steenblik J, Al-Dulaimi R, Madsen T, et al. Acute effects of air pollutants on spontaneous pregnancy loss: a case-crossover study. Fertil Steril (2019) 111:341–7. doi: 10.1016/j.fertnstert.2018.10.028
57. Anderson JO, Thundiyil JG, Stolbach A. Clearing the air: a review of the effects of particulate matter air pollution on human health. J Med Toxicol (2012) 8:166–75. doi: 10.1007/s13181-011-0203-1
58. Hamanaka RB, Mutlu GM. Particulate matter air pollution: effects on the cardiovascular system. Front Endocrinol (2018) 9:680. doi: 10.3389/fendo.2018.00680
59. Ritz B, Hoffmann B, Peters A. The effects of fine dust, ozone, and nitrogen dioxide on health. Deutsches Ärzteblatt Int (2019) 116:881. doi: 10.3238/arztebl.2019.0881
60. Miller JG, Gillette JS, Kircanski K, LeMoult J, Gotlib IH. Air pollution is associated with elevated HPA-Axis response to stress in anxious adolescent girls. Compr Psychoneuroendocrinol (2020) 4:100015. doi: 10.1016/j.cpnec.2020.100015
61. Gennings C, Carter WH, Carchman RA, DeVito MJ, Simmons JE, Crofton KM. The impact of exposure to a mixture of eighteen polyhalogenated aromatic hydrocarbons on thyroid function: Estimation of an interaction threshold. J Agric Biol Environ Stat (2007) 12:96–111. doi: 10.1198/108571107X176727
62. Porazzi P, Calebiro D, Benato F, Tiso N, Persani L. Thyroid gland development and function in the zebrafish model. Mol Cell Endocrinol (2009) 312:14–23. doi: 10.1016/j.mce.2009.05.011
63. Howe CG, Eckel SP, Habre R, Girguis MS, Gao L, Lurmann FW, et al. Association of prenatal exposure to ambient and traffic-related air pollution with newborn thyroid function: findings from the Children’s Health Study. JAMA network Open (2018) 1:e182172–e182172. doi: 10.1001/jamanetworkopen.2018.2172
64. Shang L, Huang L, Yang W, Qi C, Yang L, Xin J, et al. Maternal exposure to PM2. 5 may increase the risk of congenital hypothyroidism in the offspring: a national database based study in China. BMC Public Health (2019) 19:1–9. doi: 10.1186/s12889-019-7790-1
65. Karzai S, Zhang Z, Sutton W, Prescott J, Segev DL, McAdams-DeMarco M, et al. Ambient particulate matter air pollution is associated with increased risk of papillary thyroid cancer. Surgery (2022) 171:212–9. doi: 10.1016/j.surg.2021.05.002
66. Dehghani S, Moshfeghinia R, Ramezani M, Vali M, Oskoei V, Amiri-Ardekani E, et al. Exposure to air pollution and risk of ovarian cancer: a review. Rev Environ Health (2022) 38(3):439–50. doi: 10.1515/reveh-2021-0129
67. Kwon JY, Seo SG, Yue S, Cheng J-X, Lee KW, Kim K-H. An inhibitory effect of resveratrol in the mitotic clonal expansion and insulin signaling pathway in the early phase of adipogenesis. Nutr Res (2012) 32:607–16. doi: 10.1016/j.nutres.2012.06.014
68. Liu S, Zhou Y, Liu S, Chen X, Zou W, Zhao D, et al. Association between exposure to ambient particulate matter and chronic obstructive pulmonary disease: results from a cross-sectional study in China. Thorax (2016) 72:208910. doi: 10.1136/thoraxjnl-2016-208910
69. Fatmi Z, Rahman A, Kazi A, Kadir MM, Sathiakumar N. Situational analysis of household energy and biomass use and associated health burden of indoor air pollution and mitigation efforts in Pakistan. Int J Environ Res Public Health (2010) 7:2940–52.
70. Sun J, Zhang N, Yan X, Wang M, Wang J. The effect of ambient fine particulate matter (PM 2.5) on respiratory diseases in China: A systematic review and meta-analysis. Stochastic Environ Res Risk Assess (2020) 34:593–610. doi: 10.1007/s00477-020-01786-0
71. Huang Y, Goel S, Duda DG, Fukumura D, Jain RK. Vascular normalization as an emerging strategy to enhance cancer immunotherapy. Cancer Res (2013) 73:2943–8. doi: 10.1158/0008-5472.CAN-12-4354
72. Gai H-F, An J-X, Qian X-Y, Wei Y-J, Williams JP, Gao G-L. Ovarian damages produced by aerosolized fine particulate matter (PM2. 5) pollution in mice: possible protective medications and mechanisms. Chin Med J (2017) 130:1400–10.
73. Hull KL, Harvey S. Growth hormone: a reproductive endocrine-paracrine regulator? Rev Reprod (2000) 5:175–82. doi: 10.1530/ror.0.0050175
74. Hallak J, Veras MM, Saldiva PHN. How environmental and air pollution disrupt spermatogenesis and male reproductive health: a mechanistic approach. In: Bioenvironmental Issues Affecting Men’s Reproductive and Sexual Health. Amsterdam (Netherlands): Elsevier (2018). p. 5–32.
75. Segal TR, Giudice LC. Systematic review of climate change effects on reproductive health. Fertil Steril (2022) 118:215–23. doi: 10.1016/j.fertnstert.2022.06.005
76. Harari-Kremer R, Calderon-Margalit R, Korevaar TI, Nevo D, Broday D, Kloog I, et al. Associations between prenatal exposure to air pollution and congenital hypothyroidism. Am J Epidemiol (2021) 190:2630–8. doi: 10.1093/aje/kwab187
77. Chamot S, Al-Salameh A, Petit P, Bonneterre V, Cancé C, Decocq G, et al. Does prenatal exposure to multiple airborne and tap-water pollutants increase neonatal thyroid-stimulating hormone concentrations? Data Picardy region France. Sci Total Environ (2023) 905:167089. doi: 10.1016/j.scitotenv.2023.167089
78. Cowell W, Kloog I, Just AC, Coull BA, Carroll K, Wright RJ. Ambient PM2. 5 exposure and salivary cortisol output during pregnancy in a multi-ethnic urban sample. Inhalation Toxicol (2023) 35:101–8. doi: 10.1080/08958378.2022.2051647
79. Yao Y, Chen X, Yang M, Han Y, Xue T, Zhang H, et al. Neuroendocrine stress hormones associated with short-term exposure to nitrogen dioxide and fine particulate matter in individuals with and without chronic obstructive pulmonary disease: A panel study in Beijing, China. Environ pollut (2022) 309:119822. doi: 10.1016/j.envpol.2022.119822
80. Ahlers NE, Weiss SJ. Exposure to particulate matter, prenatal depressive symptoms and HPA axis dysregulation. Heliyon (2021) 7:101–8. doi: 10.1016/j.heliyon.2021.e07166
81. Khamirchi R, Moslem A, Agah J, Pozo ÓJ, Miri M, Dadvand P. Maternal exposure to air pollution during pregnancy and cortisol level in cord blood. Sci Total Environ (2020) 713:136622. doi: 10.1016/j.scitotenv.2020.136622
82. Hajat A, Hazlehurst MF, Golden SH, Merkin SS, Seeman T, Szpiro AA, et al. The cross-sectional and longitudinal association between air pollution and salivary cortisol: Evidence from the Multi-Ethnic Study of Atherosclerosis. Environ Int (2019) 131:105062. doi: 10.1016/j.envint.2019.105062
83. Niu Y, Chen R, Xia Y, Cai J, Ying Z, Lin Z, et al. Fine particulate matter constituents and stress hormones in the hypothalamus–pituitary–adrenal axis. Environ Int (2018) 119:186–92. doi: 10.1016/j.envint.2018.06.027
84. Ghassabian A, Pierotti L, Basterrechea M, Chatzi L, Estarlich M, Fernández-Somoano A, et al. Association of exposure to ambient air pollution with thyroid function during pregnancy. JAMA network Open (2019) 2:e1912902–e1912902. doi: 10.1001/jamanetworkopen.2019.12902
85. Fehsel K, Schikowski T, Jänner M, Hüls A, Voussoughi M, Schulte T, et al. Estrogen receptor beta polymorphisms and cognitive performance in women: associations and modifications by genetic and environmental influences. J Neural Transm (2016) 123:1369–79. doi: 10.1007/s00702-016-1620-8
86. Zhao T, Triebner K, Markevych I, Standl M, Altug H, de Hoogh K, et al. Outdoor air pollution and hormone-assessed pubertal development in children: Results from the GINIplus and LISA birth cohorts. Environ Int (2021) 152:106476. doi: 10.1016/j.envint.2021.106476
87. Petkus AJ, Younan D, Wang X, Beavers DP, Espeland MA, Gatz M, et al. Air pollution and the dynamic association between depressive symptoms and memory in oldest-old women. J Am Geriatrics Soc (2021) 69:474–84. doi: 10.1111/jgs.16889
88. White AJ, Gregoire AM, Niehoff NM, Bertrand KA, Palmer JR, Coogan PF, et al. Air pollution and breast cancer risk in the Black Women’s Health Study. Environ Res (2021) 194:110651. doi: 10.1016/j.envres.2020.110651
89. Colicino E, Cowell W, Foppa Pedretti N, Joshi A, Youssef O, Just AC, et al. Maternal steroids during pregnancy and their associations with ambient air pollution and temperature during preconception and early gestational periods. Environ Int (2022) 165:107320. doi: 10.1016/j.envint.2022.107320
90. Younan D, Wang X, Millstein J, Petkus AJ, Beavers DP, Espeland MA, et al. Air quality improvement and cognitive decline in community-dwelling older women in the United States: A longitudinal cohort study. PloS Med (2022) 19:e1003893. doi: 10.1371/journal.pmed.1003893
91. Shin J, Kweon HJ, Kwon KJ, Han S-H. Incidence of osteoporosis and ambient air pollution in South Korea: a population-based retrospective cohort study. BMC Public Health (2021) 21:1794. doi: 10.1186/s12889-021-11866-7
92. Li Y-Z, Huang S-H, Shi S, Chen W-X, Wei Y-F, Zou B-J, et al. Association of long-term particulate matter exposure with all-cause mortality among patients with ovarian cancer: A prospective cohort. Sci Total Environ (2023) 884:163748. doi: 10.1016/j.scitotenv.2023.163748
93. Fruh V, Cheng JJ, Aschengrau A, Mahalingaiah S, Lane KJ. Fine particulate matter and polycystic ovarian morphology. Environ Health (2022) 21:26. doi: 10.1186/s12940-022-00835-1
94. Toledo-Corral CM, Alderete TL, Herting MM, Habre R, Peterson AK, Lurmann F, et al. Ambient air pollutants are associated with morning serum cortisol in overweight and obese Latino youth in Los Angeles. Environ Health (2021) 20:39. doi: 10.1186/s12940-021-00713-2
95. Li L, Feng T, Wu R, Zhang Y, Wang N, Wu M, et al. The role of total antioxidant capacity and malondialdehyde of seminal plasma in the association between air pollution and sperm quality. Environ pollut (2023) 335:122324. doi: 10.1016/j.envpol.2023.122324
96. Shen X, Meng X, Wang C, Chen X, Chen Q, Cai J, et al. Prenatal exposure to fine particulate matter and newborn anogenital distance: a prospective cohort study. Environ Health (2023) 22:16. doi: 10.1186/s12940-023-00969-w
97. Plusquin M, Wang C, Cosemans C, Roels HA, Vangeneugden M, Lapauw B, et al. The association between newborn cord blood steroids and ambient prenatal exposure to air pollution: findings from the ENVIRONAGE birth cohort. Environ Health (2023) 22:63. doi: 10.1186/s12940-023-01010-w
98. Fang L, Ma C, Ma Y, Zhao H, Peng Y, Wang G, et al. Associations of long-term exposure to air pollution and green space with reproductive hormones among women undergoing assisted reproductive technology: A longitudinal study. Sci Total Environ (2023) 905:166941. doi: 10.1016/j.scitotenv.2023.166941
99. Zheng P, Chen Z, Shi J, Xue Y, Bai Y, Kang Y, et al. Association between ambient air pollution and blood sex hormones levels in men. Environ Res (2022) 211:113117. doi: 10.1016/j.envres.2022.113117
100. Belladelli F, Corsini C, Pozzi E, Raffo M, Fallara G, Costa A, et al. Does air pollution impact on semen parameters? Findings from a real-life, cross-sectional study in italian infertile men. World J Mens Health (2023) 41:403–12. doi: 10.5534/wjmh.210240
101. Wei D, Li S, Liu X, Zhang L, Liu P, Fan K, et al. Long-term exposure to particulate matter and residential greenness in relation to androgen and progesterone levels among rural Chinese adults. Environ Int (2021) 153:106483. doi: 10.1016/j.envint.2021.106483
102. Chen Q, Wang F, Yang H, Wang X, Zhang A, Ling X, et al. Exposure to fine particulate matter-bound polycyclic aromatic hydrocarbons, male semen quality, and reproductive hormones: The MARCHS study. Environ pollut (2021) 280:116883. doi: 10.1016/j.envpol.2021.116883
103. Wang F, Chen Q, Zhan Y, Yang H, Zhang A, Ling X, et al. Acute effects of short-term exposure to ambient air pollution on reproductive hormones in young males of the MARHCS study in China. Sci Total Environ (2021) 774:145691. doi: 10.1016/j.scitotenv.2021.145691
104. Radwan M, Jurewicz J, Polańska K, Sobala W, Radwan P, Bochenek M, et al. Exposure to ambient air pollution-does it affect semen quality and the level of reproductive hormones? Ann Hum Biol (2016) 43:50–6. doi: 10.3109/03014460.2015.1013986
105. Wu W, Chen Y, Cheng Y, Tang Q, Pan F, Tang N, et al. Association between ambient particulate matter exposure and semen quality in fertile men. Environ Health (2022) 21:16. doi: 10.1186/s12940-022-00831-5
107. Yang C, Zhang D-M, Song Z-B, Hou Y-Q, Bao Y-L, Sun L-G, et al. Protumoral TSP50 regulates macrophage activities and polarization via production of TNF-α and IL-1β, and activation of the NF-κB signaling pathway. PloS One (2015) 10:e0145095.
108. Li T, Yu Y, Sun Z, Duan J. A comprehensive understanding of ambient particulate matter and its components on the adverse health effects based from epidemiological and laboratory evidence. Particle fibre Toxicol (2022) 19:67. doi: 10.1186/s12989-022-00507-5
109. Liu S, Zhao J, Ye X, Fu M, Zhang K, Wang H, et al. Fine particulate matter and its constituent on ovarian reserve: Identifying susceptible windows of exposure. Sci Total Environ (2023) 904:166744. doi: 10.1016/j.scitotenv.2023.166744
110. Johnson NM, Hoffmann AR, Behlen JC, Lau C, Pendleton D, Harvey N, et al. Air pollution and children’s health—a review of adverse effects associated with prenatal exposure from fine to ultrafine particulate matter. Environ Health Prev Med (2021) 26:1–29. doi: 10.1186/s12199-021-00995-5
111. Puri P, Nandar SK, Kathuria S, Ramesh V. Effects of air pollution on the skin: A review. Indian J Dermatol Venereol Leprol (2017) 83:415. doi: 10.4103/0378-6323.199579
112. Brown SB, Adams BA, Cyr DG, Eales JG. Contaminant effects on the teleost fish thyroid. Environ Toxicol Chemistry: Int J (2004) 23:1680–701. doi: 10.1897/03-242
113. Movahedinia A, Salamat N, Kheradmand P. Effects of the environmental endocrine disrupting compound benzo [a] pyrene on thyroidal status of abu mullet (Liza abu) during short-term exposure. Toxicol Rep (2018) 5:377–82. doi: 10.1016/j.toxrep.2018.02.018
114. Boas M, Feldt-Rasmussen U, Skakkebæk NE, Main KM. Environmental chemicals and thyroid function. Eur J Endocrinol (2006) 154:599–611. doi: 10.1530/eje.1.02128
115. Lauretta R, Sansone A, Sansone M, Romanelli F, Appetecchia M. Endocrine disrupting chemicals: effects on endocrine glands. Front Endocrinol (2019) 10:178. doi: 10.3389/fendo.2019.00178
116. Builee T, Hatherill J. The role of polyhalogenated aromatic hydrocarbons on thyroid hormone disruption and cognitive function: a review. Drug Chem Toxicol (2004) 27:405–24. doi: 10.1081/DCT-200039780
117. Cesh LS, Elliott KH, Quade S, McKinney MA, Maisoneuve F, Garcelon DK, et al. Polyhalogenated aromatic hydrocarbons and metabolites: Relation to circulating thyroid hormone and retinol in nestling bald eagles (Haliaeetus leucocephalus). Environ Toxicol Chem (2010) 29:1301–10. doi: 10.1002/etc.165
118. Diamanti-Kandarakis E, Bourguignon J-P, Giudice LC, Hauser R, Prins GS, Soto AM, et al. Endocrine-disrupting chemicals: an Endocrine Society scientific statement. Endocrine Rev (2009) 30:293–342. doi: 10.1210/er.2009-0002
119. Schug TT, Janesick A, Blumberg B, Heindel JJ. Endocrine disrupting chemicals and disease susceptibility. J Steroid Biochem Mol Biol (2011) 127:204–15. doi: 10.1016/j.jsbmb.2011.08.007
120. Zoeller RT, Brown TR, Doan LL, Gore AC, Skakkebaek N, Soto A, et al. Endocrine-disrupting chemicals and public health protection: a statement of principles from The Endocrine Society. Endocrinology (2012) 153:4097–110.
122. Jowkar F, Saki N, Mokhtarpour A, Saki MR. Comparison of fumaric acid 5% cream versus triamcinolone 0.1% cream in the treatment of hand eczema. Acta Med Iranica (2014) 118(52):528–31.
123. Tian Y-Z, Wu J-H, Shi G-L, Wu J-Y, Zhang Y-F, Zhou L-D, et al. Long-term variation of the levels, compositions and sources of size-resolved particulate matter in a megacity in China. Sci Total Environ (2013) 463:462–8. doi: 10.1016/j.scitotenv.2013.06.055
124. Pidoux G, Gerbaud P, Guibourdenche J, Therond P, Ferreira F, Simasotchi C, et al. Formaldehyde crosses the human placenta and affects human trophoblast differentiation and hormonal functions. PloS One (2015) 10:e0133506. doi: 10.1371/journal.pone.0133506
125. Cathey AL, Watkins DJ, Rosario ZY, Vega CMV, Loch-Caruso R, Alshawabkeh AN, et al. Polycyclic aromatic hydrocarbon exposure results in altered CRH, reproductive, and thyroid hormone concentrations during human pregnancy. Sci Total Environ (2020) 749:141581. doi: 10.1016/j.scitotenv.2020.141581
126. Dehghani S, Fararouei M, Rafiee A, Hoepner L, Oskoei V, Hoseini M. Prenatal exposure to polycyclic aromatic hydrocarbons and effects on neonatal anthropometric indices and thyroid-stimulating hormone in a Middle Eastern population. Chemosphere (2022) 286:131605. doi: 10.1016/j.chemosphere.2021.131605
127. Peretz J, Flaws JA. Bisphenol A down-regulates rate-limiting Cyp11a1 to acutely inhibit steroidogenesis in cultured mouse antral follicles. Toxicol Appl Pharmacol (2013) 271:249–56. doi: 10.1016/j.taap.2013.04.028
128. Ji Y, Wang F, Zhang L, Shan C, Bai Z, Sun Z, et al. A comprehensive assessment of human exposure to phthalates from environmental media and food in Tianjin, China. J Hazard Mater (2014) 279:133–40. doi: 10.1016/j.jhazmat.2014.06.055
129. Huang H-B, Kuo P-H, Su P-H, Sun C-W, Chen WJ, Wang S-L. Prenatal and childhood exposure to phthalate diesters and neurobehavioral development in a 15-year follow-up birth cohort study. Environ Res (2019) 172:569–77. doi: 10.1016/j.envres.2019.02.029
130. Yang M, Chen Z, Cao Z, Mei H, Xiang F, Yu L, et al. Prenatal exposure to phthalates and child growth trajectories in the first 24 months of life. Sci Total Environ (2023) 898:165518. doi: 10.1016/j.scitotenv.2023.165518
131. Thomson EM, Filiatreault A, Williams A, Rider CF, Carlsten C. Exposure to diesel exhaust and plasma cortisol response: A randomized double-blind crossover study. Environ Health Perspect (2021) 129:037701. doi: 10.1289/EHP8923
132. Deng Z, Chen F, Zhang M, Lan L, Qiao Z, Cui Y, et al. Association between air pollution and sperm quality: a systematic review and meta-analysis. Environ pollut (2016) 208:663–9. doi: 10.1016/j.envpol.2015.10.044
133. Zhang G, Jiang F, Chen Q, Yang H, Zhou N, Sun L, et al. Associations of ambient air pollutant exposure with seminal plasma MDA, sperm mtDNA copy number, and mtDNA integrity. Environ Int (2020) 136:105483. doi: 10.1016/j.envint.2020.105483
134. Yang Y, Feng Y, Huang H, Cui L, Li F. PM2. 5 exposure induces reproductive injury through IRE1/JNK/autophagy signaling in male rats. Ecotoxicol Environ Saf (2021) 211:111924. doi: 10.1016/j.ecoenv.2021.111924
135. Pironti C, Ricciardi M, Proto A, Bianco PM, Montano L, Motta O. Endocrine-disrupting compounds: An overview on their occurrence in the aquatic environment and human exposure. Water (2021) 13:1347. doi: 10.3390/w13101347
136. Yang M, Cheng H, Shen C, Liu J, Zhang H, Cao J, et al. Effects of long-term exposure to air pollution on the incidence of type 2 diabetes mellitus: a meta-analysis of cohort studies. Environ Sci pollut Res (2020) 27:798–811. doi: 10.1007/s11356-019-06824-1
137. Gałuszka-Bulaga A, Hajto J, Borczyk M, Gołda S, Piechota M, Korostyński M, et al. Transcriptional response of blood mononuclear cells from patients with inflammatory and autoimmune disorders exposed to “Krakow smog”. Cells (2022) 11:2586. doi: 10.3390/cells11162586
138. Hao J, Wu Y, Wang S. Technologies for air pollution control. Point Sources Pollution: Local Effects their Control-Volume II (2009) 2:134.
139. Usman M, Aamir H, Naz Iqbal H, Arshad H. New techniques for the prevention control of smog and air pollution in Pakistan. Environ pollut Climate Change (2019) 2:2. doi: 10.4172/2573-458X.1000166
140. Maina DM, Njenga LW, Onyari JM, Kyalo BN. Trace element concentrations in some traditional diets consumed in selected parts of eastern province of Kenya. J Environ Prot (2012) 3(7):1–7. doi: 10.4236/jep.2012.37075
Keywords: smog, endocrine disruption, ambient air pollution, ozone, infertility, metabolic diseases
Citation: Saleem A, Awan T and Akhtar MF (2024) A comprehensive review on endocrine toxicity of gaseous components and particulate matter in smog. Front. Endocrinol. 15:1294205. doi: 10.3389/fendo.2024.1294205
Received: 14 September 2023; Accepted: 10 January 2024;
Published: 30 January 2024.
Edited by:
Cuiqing Liu, Zhejiang Chinese Medical University, ChinaReviewed by:
Ben Li, Shanxi Medical University, ChinaCopyright © 2024 Saleem, Awan and Akhtar. This is an open-access article distributed under the terms of the Creative Commons Attribution License (CC BY). The use, distribution or reproduction in other forums is permitted, provided the original author(s) and the copyright owner(s) are credited and that the original publication in this journal is cited, in accordance with accepted academic practice. No use, distribution or reproduction is permitted which does not comply with these terms.
*Correspondence: Muhammad Furqan Akhtar, ZnVycWFuLnBoYXJtYWNpc3RAZ21haWwuY29t
Disclaimer: All claims expressed in this article are solely those of the authors and do not necessarily represent those of their affiliated organizations, or those of the publisher, the editors and the reviewers. Any product that may be evaluated in this article or claim that may be made by its manufacturer is not guaranteed or endorsed by the publisher.
Research integrity at Frontiers
Learn more about the work of our research integrity team to safeguard the quality of each article we publish.