- 1Center for Reproductive Medicine, The First Affiliated Hospital of Zhengzhou University, Zhengzhou, China
- 2Henan Key Laboratory of Reproduction and Genetics, The First Affiliated Hospital of Zhengzhou University, Zhengzhou, China
- 3Henan Provincial Obstetrical and Gynecological Diseases (Reproductive Medicine) Clinical Research Center, The First Affiliated Hospital of Zhengzhou University, Zhengzhou, China
- 4Henan Engineering Laboratory of Preimplantation Genetic Diagnosis and Screening, The First Affiliated Hospital of Zhengzhou University, Zhengzhou, China
Background: Male sperm DNA fragmentation (SDF) may be associated with assisted reproductive technology (ART) outcomes, but the impact of SDF on the occurrence of aneuploid-related miscarriage remains controversial.
Methods: Genome-wide single-nucleotide polymorphism-based chromosomal microarray analysis was performed on 495 miscarried chorionic villus samples undergone IVF/ICSI treatment from the Reproductive Medicine Center of the First Affiliated Hospital of Zhengzhou University. SDF was assessed using sperm chromatin structure assay. Patients were divided into four groups according to embryo transfer cycle type and maternal age, and the correlation between SDF and chromosome aberration was analyzed. A receiver operating characteristic (ROC) curve was utilized to find the optimal threshold.
Results: Total chromosomal aneuploidy rate was 54.95%, and trisomy was the most common abnormality (71.32%). The chromosomally abnormal group had higher SDF than the normal group (11.42% [6.82%, 16.54%] vs. 12.95% [9.61%, 20.58%], P = 0.032). After grouping, elevated SDF was significantly correlated with an increasing chromosome aneuploidy rate only in women of advanced age who underwent fresh embryo transfer (adjusted odds ratio:1.14 [1.00–1.29], adjusted-P = 0.045). The receiver operating characteristic curve showed that SDF can predict the occurrence of chromosomal abnormality of miscarried conceptus in this group ((area under the curve = 0.76 [0.60–0.91], P = 0.005), and 8.5% was the optimum threshold. When SDF was ≥ 8.5%, the risk of such patients increased by 5.76 times (adjusted odds ratio: 6.76 [1.20–37.99], adjusted-P = 0.030).
Conclusion: For women of advanced maternal age undergoing fresh embryo transfer, older oocytes fertilized using sperm with high SDF in IVF/ICSI treatment might increase the risk of chromosomal abnormality in miscarried conceptus.
Introduction
The incidence of spontaneous abortion after assisted reproductive technology (ART) pregnancy is about 15%–20%, with approximately 80% occurring within the first 12 weeks (1). Embryonic chromosomal abnormalities, observed in nearly 50%–60% of miscarried tissues, are the most common cause of early miscarriage (2). Advanced maternal age and metabolic disorders are independent risk factors of chromosomal aneuploidy (CA) in embryos (3, 4), however, recent studies discovered male factors may also be associated with embryonic aneuploidy (5).
Male factors account for 40%–50% of infertility cases (6), some of which are well-defined, such as sperm malformation, low semen volume, and low sperm motility (7, 8). However, a substantial proportion of the causes remain unknown, and genetic factors are thought to be the most likely candidates (9). Genomic imprinting studies of miscarried chorionic villus samples (CVS) have shown that various paternally expressed genes play significant roles in fetal growth (10), and the integrity of sperm, especially genome integrity, is of great importance for fertilization, embryo formation and development.
Sperm DNA is more concentrated than that of somatic cells to better resist physical and chemical denaturation; however, heat, radiation, oxidative stress and abnormal apoptosis may damage DNA and lead to sperm fragmentation (11). Human spermatozoa do not have DNA repair activity (DRA) which depends on transcripts stored during oocyte maturation in the process of fertilization (12). However, the repair capacity of oocytes largely depends on their quality and the degree of sperm DNA fragmentation, and the fraction above the repair threshold may be transferred to the embryo and have genetic significance for chromosomal aneuploidy, especially in advanced women (≥ 35 years old) (13–15). Accumulating evidence indicates that high sperm DNA damage directly affects male infertility, which is closely related to poor embryo quality, low pregnancy rate and high miscarriage rate (16–19). A high sperm DNA fragmentation (SDF) index seems to reflect high chromosome abnormalities to a certain extent, thus increasing the risk of aneuploid embryos (5, 20, 21). Although the SDF test has shown potential in predicting male fertility, dissenting voices remain (22). To date, evidence of relationship between SDF level, embryonic euploidy and morphological grading is lacking. Studies on the association between SDF and embryonic chromosomal status are limited. Previous studies have mostly concentrated on the effect of SDF on clinical and laboratory outcomes after ART, but few studies have focused on the association of SDF with aneuploidy in aborted conceptus. Whether male SDF is associated with aneuploidy in miscarriage products and whether SDF can be considered as an evaluation of male infertility and aneuploidy-related miscarriage remains a focus of clinicians. Furthermore, unlike previous studies on fresh cycles, a recent study has indicated that SDF is only associated with fertilization rate but not with laboratory and clinical outcomes in frozen embryo transfer (FET) cycles (23). With the development and prevalence of freeze-all strategy, some advantages of FET cannot be ignored, so comprehensive understanding whether SDF exerts a different impact on embryo aneuploidy in fresh and frozen cycles is also an interesting topic of clinicians’ concern.
Chromosomal microarray analysis (CMA) based on genome-wide single-nucleotide polymorphism (SNP) arrays provides higher resolution and detection of copy number variation, and a higher detection rate of abnormal chromosomes in CVS (24, 25). The aim of our study was to investigate the role of paternal SDF, evaluated using the sperm chromatin structure assay (SCSA), in chromosomal aberration-related early spontaneous miscarriage after SNP array analysis for CVS in early miscarried patients who underwent in vitro fertilization (IVF)/intracytoplasmic sperm injection (ICSI) treatment in our center.
Materials and methods
Study cohort and inclusion criteria
This study retrospectively included couples treated at our Reproductive Medicine Center from January 2015 to December 2020. All men underwent SDF testing and all women had spontaneous abortion within 12 weeks of gestation after IVF/ICSI treatment and received dilation and curettage therapy. Miscarried CVS were genetically analyzed at the Center for Preimplantation Genetic Diagnosis. Patients with chromosomal abnormalities, recurrent pregnancy loss, recurrent implantation failure, sperm freezing or donation, egg freezing or donation, immunological disorders, endocrine diseases, infectious disease, congenital or chronic diseases, varicocele and medication in men after SDF testing were excluded. Data were obtained from the Clinical Reproductive Medicine Management System/Electronic Medical Record Cohort Database (CCRM/EMRCD) in the Reproductive Medicine Center of the First Affiliated Hospital of Zhengzhou University and Henan Key Laboratory of Reproduction and Genetics. The specific inclusion and exclusion criteria were shown in Figure 1; 495 couples were included in this cohort analysis. All clinical and laboratory information of the couples were reviewed to evaluate the relevance between SDF level and CVS chromosomal abnormality in patients with early miscarriage. This study was approved by the Ethics Committee of the First Affiliated Hospital of Zhengzhou University (No.2023-KY-0519-002), and written informed consent was obtained from all patients at the first consultation.
ART protocols
Both IVF and ICSI are applied for ovarian stimulation with gonadotropin-releasing hormone (GnRH) agonists according to the body mass index (BMI) and ovarian function of the patients to stimulate follicle growth and prevent premature LH surges and gonadotropins. Human chorionic gonadotropin (hCG) was administered depending on serum FSH, LH, E2 and P levels when the diameter of the largest follicle was > 20 mm and more than 2/3 of the follicles were > 16 mm. Egg retrieval was performed after 36–38 h under ultrasound guidance. The protocols of the different embryo transfer (ET) strategies were based on those stated in our published article (26) and serum β-hCG level was monitored on days 14 and 18. Clinical pregnancy was defined as the presence of intrauterine gestational blastocyst buds and primitive cardiac tube beats at 35 days after transplantation. Early spontaneous miscarriage was defined as the absence of the intrauterine fetal heartbeat on ultrasound within 12 weeks of confirmed clinical pregnancy.
SNP array analysis
Genetic analysis of CVS in all early spontaneous miscarried patients were performed at the Preimplantation Genetic Diagnosis Center. CVS samples were cleaned and thoroughly separated from villus tissues and decidua by technicians follow the standard procedures to avoid contamination of genetic tissue (27). Samples were stored at -80°C and total villus DNA was extracted with All Prep DNA Mini Kit (Qiagen, Germany). SNP arrayed using Human CytoSNP-12v.21 Array (Illumina, San Diego, California, USA) and the data were analyzed using Genome-Studio (Illumina 2011) and Karyo-Studio V1.4 (Illumina 2011). Candidate pathogenic CNVs were mapped and identified based on the Database of Genomic Variation (DGV) (http://dgv.tcag.ca/dgv/app/faq). At least two independent technicians analyzed the data with rigorous criteria.
SDF
Semen, obtain by masturbation after 3–7 days of abstinence from all male partners, was collected in dry sterile containers and placed in a 37°C thermostat. All examinations were completed within 1 h of sperm collection. Semen analysis was performed according to the World Health Organization standard protocol (28); sperm concentration and viability were assessed according to the instructions of the Makler counting chamber (Sefi Medical Instruments, Israel).
SCSA was utilized to evaluate SDF. All assays were performed strictly according to the instruction of the fragmentation assay kit (Cellpro Biotech, Zhejiang, China), and fluorescence was measured with a BD FACS Canto II flow cytometer (Becton Dickinson, USA). Upon excitation in the flow cytometer, intact DNA emitted green fluorescence, whereas denatured DNA emitted red fluorescence. Then SDF was expressed as the ratio of the intensity of red to the total fluorescence.
Statistical analysis
SPSS 26.0 (IBM Corp., Armonk, NY, USA) was utilized for statistical analyses. Normality of distribution was assessed using Q-Q plots and Kolmogorov-Smirnov test. Mean ± standard deviation and 95% confidence intervals (CI) were utilized to represent continuous variables, and Student’s t-test or one-way ANOVA was applied for comparison between groups. Categorical variables were expressed as frequencies and percentages and Chi-square test, Fisher’s exact test and Bonferroni-adjusted test were applied for comparison. Univariate and multivariable Logistic regression analysis was constructed to identify risk factors for early abortion associated with chromosomal abnormalities. Odds ratio (OR) and adjusted odds ratio (a-OR) with 95% confidence intervals (CI) were calculated. The receiver operating characteristic (ROC) curve was constructed to observe predictive values of risk factors and determine the optimal threshold. All tests were two-side and statistical significance was set at P < 0.05.
Results
Study cohort characteristics
Demographic characteristics of the study couples, sperm parameters and baseline clinical laboratory results were presented in Table 1. Maternal age (33.33 ± 5.34 vs. 30.73 ± 3.83, P < 0.001) and paternal age (33.86 ± 6.44 vs. 31.20 ± 4.24, P < 0.001) in the chromosome aberration group were significantly higher than that in the normal group. Except for the number of antral follicle (14.21 ± 6.60 vs. 16.04 ± 5.82, P = 0.001) and AMH (3.93 ± 3.14 vs. 4.53 ± 3.42, P = 0.043), none of the maternal-related parameters such as BMI, infertility year, E2 and progesterone levels on trigger day showed a marked difference between two groups. For male semen examination, chromosomal aberration-related abortion group showed a higher SDF index (11.42% [6.82%, 16.54%] vs. 12.95% [9.61%, 20.58%], P = 0.032), whereas ejaculatory abstinence, progressive sperm motility, seminal volume and concentration were similar between two groups. Furthermore, compared with the normal group, the abnormal group had higher secondary infertility rate (60.00% vs. 50.45%, P = 0.034), fresh cycle rate (55.88% vs. 39.01%, P < 0.001), cleavage-stage D3 ET rate (58.09% vs. 41.26%, P < 0.001), and double-embryo transfer rate (52.94% vs. 43.50%, P = 0.037).
SNP array analysis results on miscarried chorionic villi
The types and frequencies of abnormal chromosomal karyotypes in miscarried conceptus were shown in Figure 2. SNP array analysis revealed chromosomal aberrations in 54.95% (272/495) of miscarried CVS. The most frequent was trisomy, accounting for 71.32% (194/272) of the total aberrations, of which 84.02% (163/194) were single-chromosome trisomy, 9.28% (18/194) were polysomic trisomy, and 6.70% (13/194) were trisomy accompanied by microduplications and microdeletions. Monosomy and triploidy accounted for 2.94% (8/272) and 4.78% (13/272) of all abnormalities. The proportions of chromosome karyotype 69,XXX and 69,XXY in triploid aberration was 40% (4/10) and 60% (6/10), respectively, and chromosomal structural abnormalities and mosaicism accounted for 11.40% (31/272) and 11.76% (32/272), respectively.
Correlations between variables and CA risk in miscarried conceptus
Univariate and multivariate logistic regression analyses were applied to evaluate the effect of variables on the CA rate (Table 2). After adjusting maternal age, paternal age, infertility type, ET days, fertilization method, number of transferred embryos, embryo quality, cycle type, AMH, number of antral follicle, ejaculatory seminal volume, seminal concentration and progressive sperm motility, there was a significant relationship between SDF and CA rate of miscarried conceptus (a-OR: 1.03 [1.00–1.05], a-P = 0.036). Advanced maternal age (≥ 35 years old) was obviously associated with the elevated CA rate (a-OR: 2.97 [1.68–5.25], a-P < 0.001). When 30–39 years was regarded as the reference of paternal age, male age of ≥ 40 years also significantly related to increased CA rate in the adjusted model (a-OR: 2.83 [1.18–6.79], a-P = 0.020). Moreover, patients undergoing frozen cycle showed a lower odds of chromosome aberrations-related miscarriage (a-OR: 0.57 [0.35–0.86], a-P = 0.008).
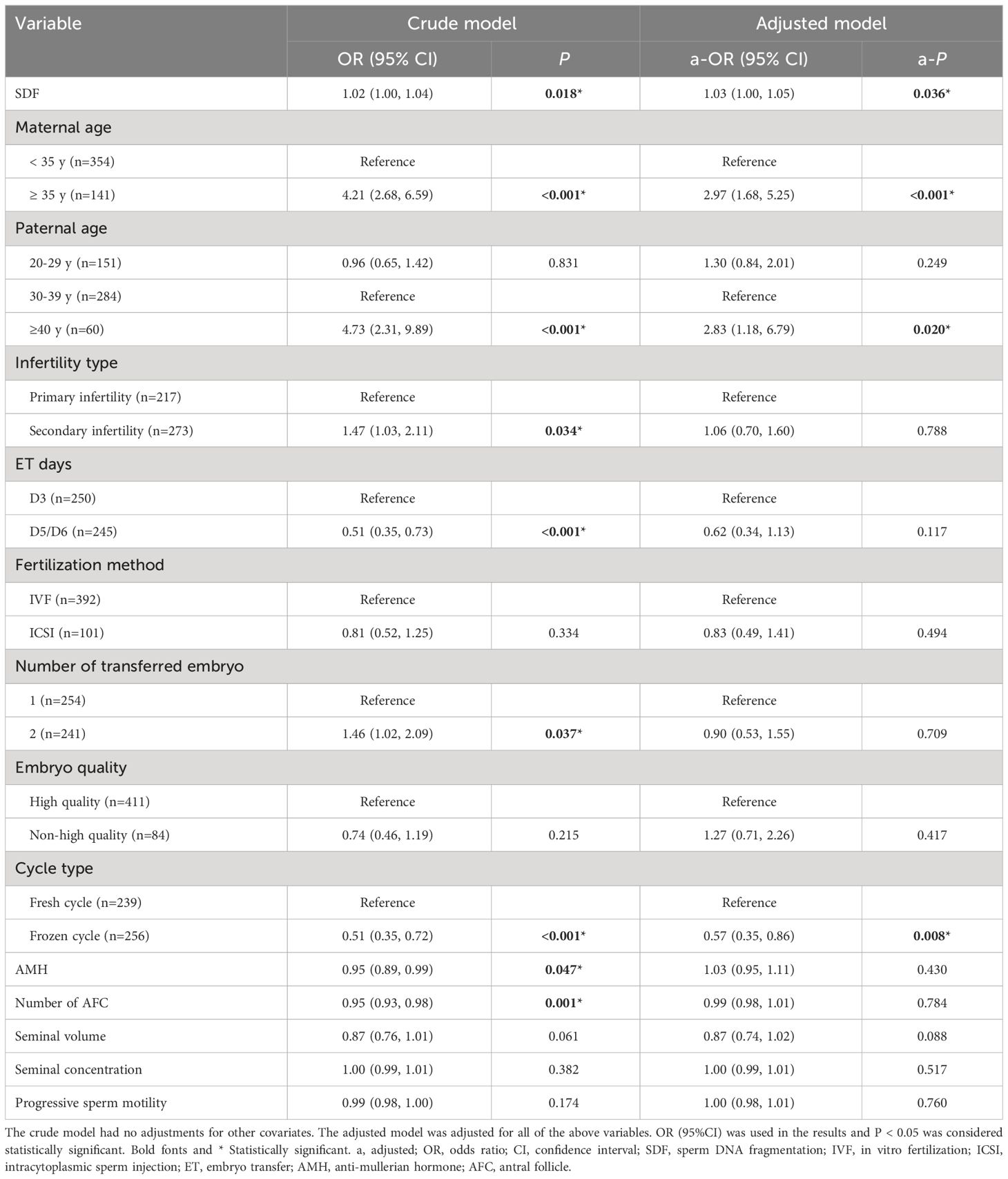
Table 2 Multivariable Logistic analyses of factors related to chromosomal aneuploidy in miscarried conceptus.
Correlation between SDF level and CA rate in miscarried conceptus for different cycle types and maternal age groups
The study population was divided into four groups according to ET type and maternal age. After adjusting the confounding variables, elevated SDF level was significantly correlated with the increase of aneuploidy rate in miscarried conceptus only in the advanced maternal age group who underwent fresh ET treatment (a-OR: 1.14 [1.00–1.29], a-P = 0.045, Table 3). The ROC curve showed that 8.5% was the most appropriate threshold for SDF related to aneuploidy rate in this group (AUC = 0.76 [0.60–0.91], P = 0.005, Figure 3A). After grouping SDF according to 8.5% and adjusting the previously mentioned variables, couples with paternal SDF ≥ 8.5% were 6.76 times more likely to have embryo aneuploid-related miscarriage than those with SDF < 8.5% (a-OR: 6.76 [1.20–37.99], a-P = 0.030, Figure 3B).
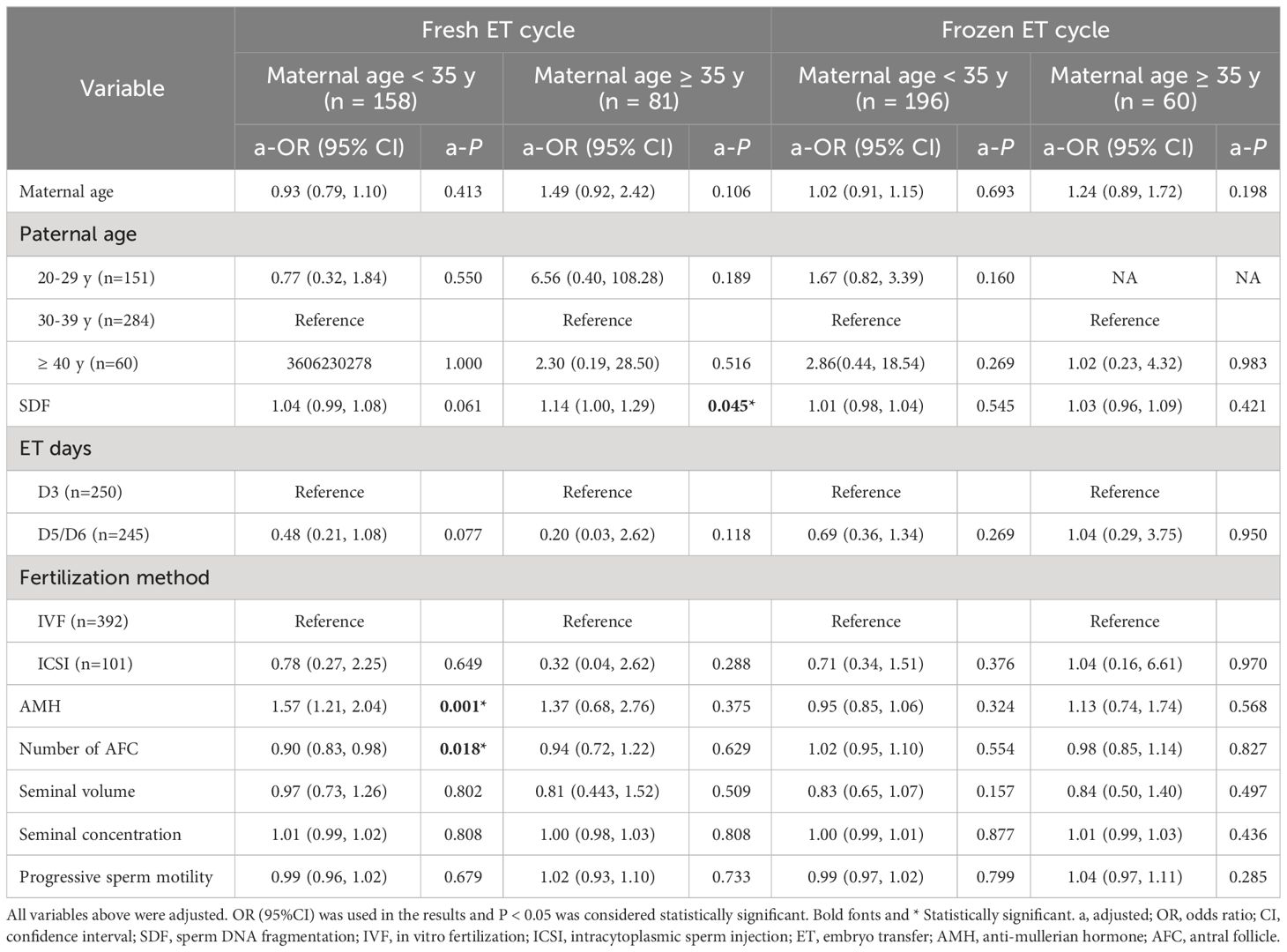
Table 3 Multivariable Logistic analyses of factors related to chromosomal aneuploidy in miscarried conceptus in different groups.
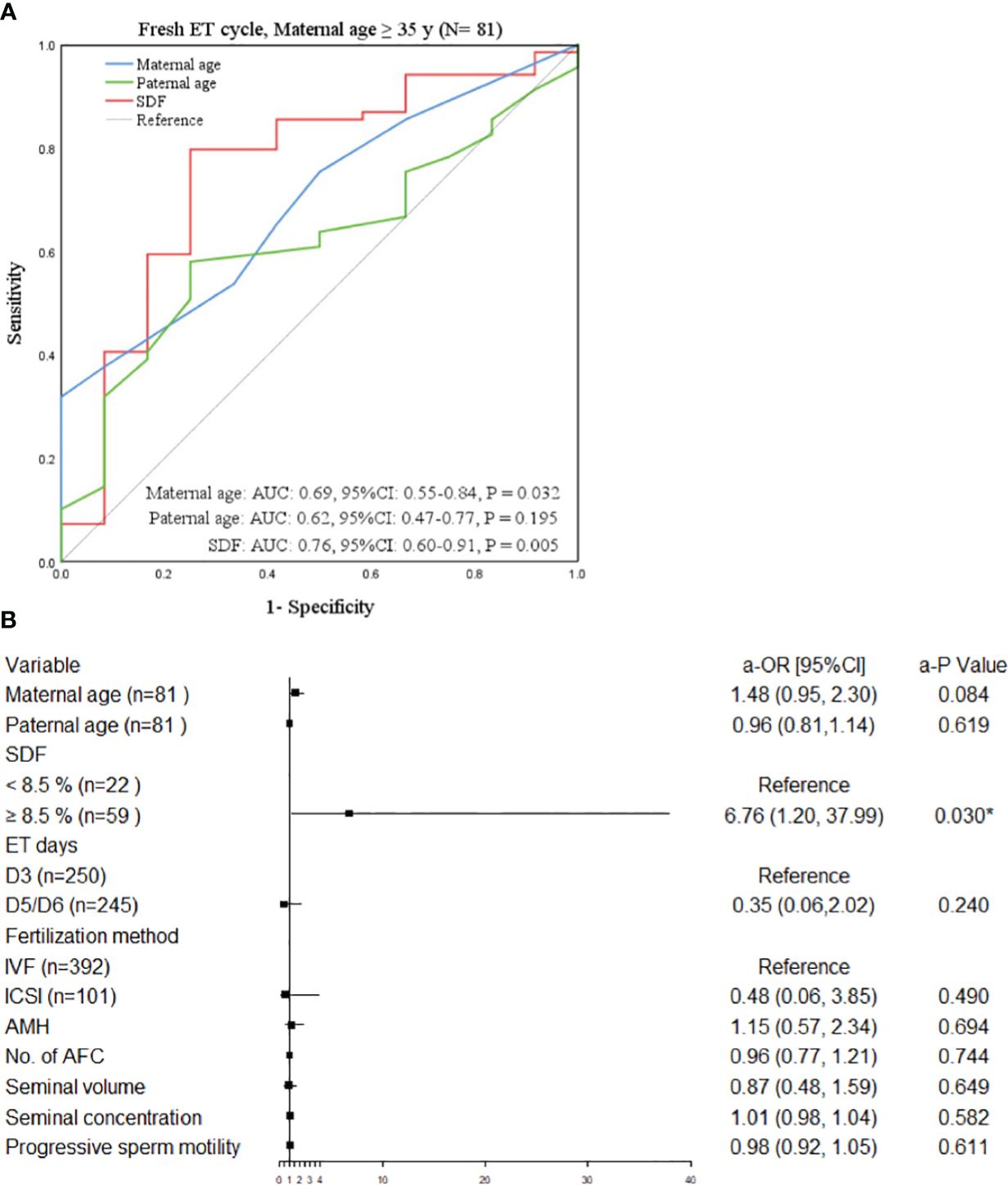
Figure 3 Factors related to chromosomal abnormalities in maternal age ≥35 years undergoing fresh ET. (A) The ROC curve of the subgroup of advanced women aged ≥35 years undergone fresh ET cycle, P < 0.05 was considered statistically significant. (B) The forest plot of the multivariate Logistic regression analysis results of the subgroup of elderly women aged ≥35 years treated with ET. All variables above were adjusted. OR (95%CI) was used in the results and P < 0.05 was considered statistically significant. * Statistically significant. OR, odds ratio; CI, confidence interval; SDF, sperm DNA fragmentation; IVF, in vitro fertilization; ICSI, intracytoplasmic sperm injection; ET, embryo transfer; AMH, anti-mullerian hormone; No., number; AFC, antral follicle.
Correlation between elevated SDF and CA rate in miscarried conceptus
In women aged ≥ 35 years undergoing fresh ET cycle, the aneuploidy rate of chromosomes with SDF ≥ 8.5% was significantly higher than that with SDF < 8.5% (93.32% vs. 63.64%, P < 0.050, Figure 4A). And only for women aged ≥ 35 years undergoing fresh ET cycle, the CA rate of miscarried conceptus increased significantly with the increase of SDF in the adjusted model, achieving the maximum growth rate at SDF = 8.5% (green line: a-OR: 1.13 [1.01–1.27], a-P = 0.043), while this trend was not showed in the other three groups (red line: a-OR: 1.04 [0.99–1.08], a-P = 0.068; orange line: a-OR: 1.01 [0.98–1.04], a-P = 0.691; blue line: a-OR: 1.02 [0.97–1.09], a-P = 0.387, Figure 4B).
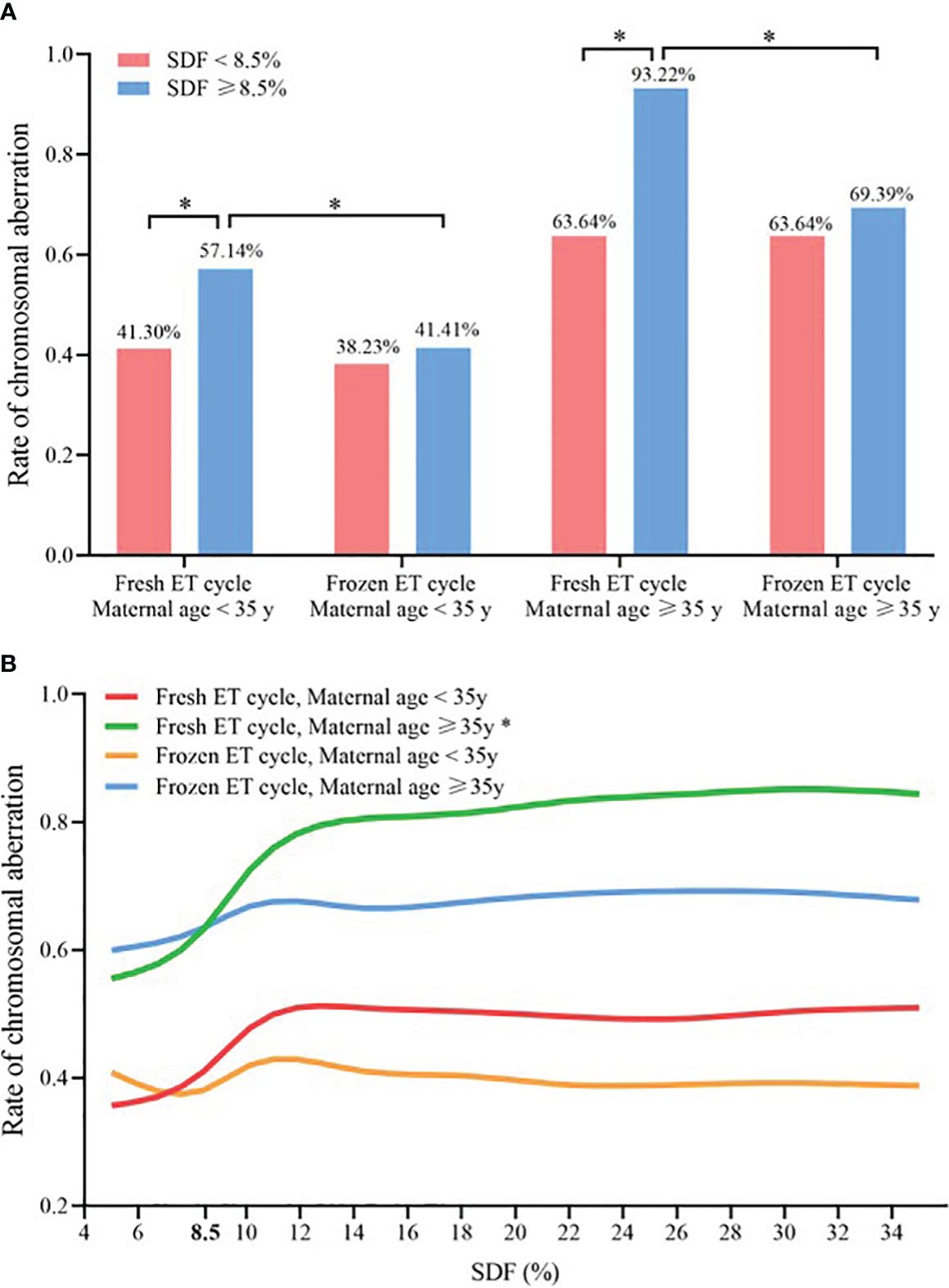
Figure 4 Comparison of chromosomal abnormalities in different ET strategies and maternal age groups (A) Chromosomal aneuploidy rates at SDF < 8.5% and ≥8.5% in the four subgroups, * Statistically significant. (B) Association between SDF and chromosomal aneuploidy rate of embryos in four subgroups, and maternal age, paternal age, ET days, fertilization method, AMH, number of antral follicle, seminal volume, seminal concentration and progressive sperm motility were adjusted. * Statistically significant, P < 0.05. SDF, sperm DNA fragmentation; ET, embryo transfer.
Discussion
Previous studies on paternal SDF and embryo quality and chromosomal status are few and inconsistent. In this retrospective cohort study, we found a CVS aneuploidy rate of 54.95%, with trisomy being the most common, followed by abnormal chromosome structure. We also investigated the association between SDF and the CA rate of miscarried chorionic villi in patients after IVF/ICSI and demonstrated that elevated SDF, maternal age, paternal age and fresh/frozen ET cycle significantly correlated with the CA rate in miscarried conceptus, and for women of advanced age (≥ 35 years) who underwent fresh ET cycle, the use of sperm with paternal SDF ≥ 8.5% dramatically increased the risk of aneuploidy in miscarried products.
The mechanisms by which sperm affect the chromosomal integrity of embryos and the occurrence of miscarriage have not been clarified. Normal fertilization might not be impeded by high levels of sperm DNA fragmentation (29), however, it might jeopardize zygote development, embryo implantation and embryo healthy through genetic and epigenetic components of damaged chromatin (30, 31). Gawecka et al. (32) found that sperm DNA damage might lead to slowed paternal DNA replication or degradation after the S phase, ultimately delaying or stalling embryonic development. Two animal studies also indicated that sperm with damaged DNA might induce the fragmentation and random distribution of paternal chromosome in two-cell–stage embryos and lead to direct unequal cleavage divisions, segregation errors and the formation of haploid and uniparental cells, and specifical sperm fragment contributed to the chaotic aneuploidy of embryos (33, 34). This suggested that the late paternal effect caused by sperm DNA damage may be a key factor affecting embryonic genome integrity, leading to early abortion after activation of the male parent gene at the eight-cell stage (35). Our results showed that elevated SDF still contributed to increased aneuploidy rate in miscarried conceptus after adjusting for confounding factors that might affect embryonic euploidy. Although the potential mechanism is unclear, our results are supported by above-mentioned studies. This study provided a new perspective for the diagnosis and treatment of the association between high male SDF and miscarriage, although further studies are needed to explore the mechanism.
Advanced female age and fresh ET cycle have been proven to be closely related to high embryo chromosomal aneuploidy rate and low livebirth rate (36, 37). Li et al. (26) had found CA rate of miscarried conceptus in women ≥ 35 years old and fresh ET increased by 1.75 times and 0.78 times, respectively. These results are consistent with those of our study. We speculate that this probably owing to the occurrence of aneuploidy caused by epigenetic abnormalities (38), cell cycle checkpoint and meiotic recombination abnormalities (39), mitochondrial energy metabolism disorders (40), and telomere shortening (41) in oocytes during female aging. Moreover, low implantation rate and high miscarriage rate caused by fresh ET had been considered to be related to intimal molecular biology and histological abnormalities caused by overexposure to drugs such as ovulation induction (42). FET can prevent this damage, effectively screen and reject the implantation of low-quality abnormal embryos (43), and reduce the abortion rate of aneuploid embryos.
Interestingly, although we found a positive correlation between SDF and aneuploidy of miscarried CVS in the whole population, when we divided the population into four subgroups according to the maternal age (< 35 or ≥ 35 years old) and ET strategies (fresh ET or FET), only women of advanced age who underwent fresh ET cycle showed a facilitative effect of elevated SDF on aneuploid-related miscarriage. At the same time, the ROC curve showed that 8.5% was an appropriate threshold to predict the risk of aneuploid-related miscarriage in this subgroup, and when paternal SDF ≥ 8.5%, the risk of aneuploid-related miscarriage was 6.76 times higher than that of paternal SDF of < 8.5%. This result is consistent with some previous studies analyzing SDF and laboratory and clinical outcomes, but the threshold is obviously lower. Setti et al. (13) performed a retrospective study of 540 couples treated with ICSI and found that injection of sperm from samples with SDF ≥ 30% in women ≥ 40 years significantly decreased D3 good-quality embryo rate, blastocyst formation rate, implantation rate and clinical pregnancy rate, while increased miscarriage rate. Another study of young RIF patients under ICSI-PGS showed a higher CA rate in SDF > 20% group, especially in the trisomy rate of chromosomes 16 and 20 (5). Horta et al. (29) fertilized young and old mouse oocytes to mouse sperm with different degrees of SDF (≤ 10%, 11-30% and > 30% produced by different doses of radiation), and found the effect of high sperm DNA damage on embryonic development was related to the decrease of DNA repair ability of aged oocytes. There has been no international consensus on the threshold of the effect of SDF on male fertility, although most studies suggest that SDF ≥ 20% or SDF ≥ 30% were more likely to lead to male infertility and adverse pregnancy outcomes (44), our results showed that for women of advanced age (≥ 35 years), the threshold of SDF promoting aneuploid-related miscarriage might be lower than previously thought, which supported the hypothesis that the DNA repair ability of oocytes decreases with female senescence (13, 45).
Numerous studies have confirmed that oocytes had active DNA repair capability, in contrast to sperm. All of DNA repair pathways, including base excision repair, nucleotide excision repair, post replication repair, mismatch repair, double strand break repair and DNA damage response have already been proved to be maternal sources during fertilization and before embryonic genome activation (29, 46, 47). We did not observe a contribution of SDF to aneuploid-related miscarriage in young maternal groups, which suggested that oocytes could effectively repair patrilineal DNA damage. However, female oocytes are produced during the fetal period (48), and female senescence re oocyte senescence, and at this time, the transcripts and proteins stored in oocytes are reduced (49, 50). Compared with young oocytes, the DRA of aging oocytes is greatly decreased, resulting in loss of ability to cope with SDF, and then has a serious negative impact on embryonic development. Zygotes fertilized by DNA-damaged sperm have the potential to develop continuously under the DNA repairment of oocytes, whereas the lesion of DNA repair mechanism caused by oocyte senescence leads to sperm genetic material damage above a low threshold (e.g. 8.5%) that cannot be effectively repaired, resulting in early embryonic development defects, such as the formation of aneuploid embryos (51). Additionally, because of the application of fresh ET cycle, the endometrium function is relatively poor, with a low ability of screening and rejection of abnormal embryos (26, 43). Even though these aneuploid embryos escape the screening of endometrium and successfully implanted, they still failed to develop normally in the later stage, eventually leading to miscarriage.
We also found that males aged ≥40 years had an increased CA risk of CVS compared with those aged 30–39 years. This probably due to the accumulating cellular damage and exposure to environmental toxins in parallel with increasing age, leading to epigenetics changes, DNA methylation defects, increased reactive oxygen species damage, shorter telomere length, and damage of mitotic and meiotic mismatch repair mechanisms (52, 53), ultimately damaging the integrity of embryonic chromosomes and increasing the risk of the formation rate of aneuploidy and mosaic embryos (54, 55). Nevertheless, this phenomenon disappeared after grouping the population. We speculate that this may be due to a statistical deviation caused by a decrease in the proportion of men aged ≥40 years in each subgroup. Besides, the contribution of advanced paternal age may not be as obvious as that of advanced maternal age, which may mask the impact of paternal age. Future work should expand the sample size to determine the contribution of greater paternal age to this risk.
To our knowledge, this is the first study to show the influence of paternal SDF on the rate of chromosomal aberrations in miscarried CVS. We used strict inclusion and exclusion criteria to reduce confounding risk factors (56), while adjusting the variant model for correlation analysis to increase statistical rigor. However, our study also has several limitations. First, retrospective design data obtained from a single center with a small sample size. Since there remains no prospective multicenter study of the effects of SDF on embryonic aneuploidy, we look forward to future high-quality studies with relevant content to provide clinicians with more and more reliable evidence. Second, even though the data collection was reviewed by medical staff, errors in the data collection and follow-up process could not be avoided. Third, patients may be subject to inclusion bias due to missing data on environment, lifestyle, occupational characteristics, psychological status, and family factors. Nevertheless, the findings of this study are still valuable. For couples with advanced maternal age and high paternal SDF index, preimplantation genetic screening and FET may be worth considering to avoid transfer of aneuploid embryos. Further research is required to determine the risks of developing such a clinical strategy.
Conclusion
In conclusion, elevated SDF are significantly associated with an elevated CA rate in miscarried conceptus. In a population of women ≥ 35 years of age undergoing fresh ET cycle, fertilization of older oocytes using sperm with high levels of SDF significantly increases the risk of miscarriage of aneuploid fetuses, especially the risk of single chromosomal trisomy. For women of advanced age treated with ART, sperm with high SDF levels should be used with vigilance during ART to avoid compromising pregnancy outcomes.
Data availability statement
The original contributions presented in the study are included in the article/supplementary material. Further inquiries can be directed to the corresponding author.
Author contributions
WF: Writing – review & editing, Data curation, Writing – original draft. QC: Writing – original draft, Writing – review & editing. ZB: Writing – review & editing. HS: Writing – review & editing. QY: Writing – review & editing. LH: Writing – review & editing, Funding acquisition.
Funding
The author(s) declare financial support was received for the research, authorship, and/or publication of this article. This work was supported by the National Natural Science Foundation of China (Grant No. 82171658), Henan Province Medical Science and Technique R&D Program (Grant No. SBGJ202102094) and The Strategic Collaborative Research Program of the Ferring Institute of Reproductive Medicine, Ferring Pharmaceuticals and Chinese Academy of Sciences (Grant No. FIRMD200501).
Acknowledgments
The authors are grateful to all the participants who supported the collection of these data and for their dedication to this study; and all the patients for their selfless participation.
Conflict of interest
The authors declare that the research was conducted in the absence of any commercial or financial relationships that could be construed as a potential conflict of interest.
Publisher’s note
All claims expressed in this article are solely those of the authors and do not necessarily represent those of their affiliated organizations, or those of the publisher, the editors and the reviewers. Any product that may be evaluated in this article, or claim that may be made by its manufacturer, is not guaranteed or endorsed by the publisher.
References
1. Gu C, Li K, Li R, Li L, Li X, Dai X, et al. Chromosomal aneuploidy associated with clinical characteristics of pregnancy loss. Front Genet. (2021) 12:667697. doi: 10.3389/fgene.2021.667697
2. Quenby S, Gallos ID, Dhillon-Smith RK, Podesek M, Stephenson MD, Fisher J, et al. Miscarriage matters: the epidemiological, physical, psychological, and economic costs of early pregnancy loss. Lancet. (2021) 397:1658–67. doi: 10.1016/S0140-6736(21)00682-6
3. Ozawa N, Ogawa K, Sasaki A, Mitsui M, Wada S, Sago H. Maternal age, history of miscarriage, and embryonic/fetal size are associated with cytogenetic results of spontaneous early miscarriages. J assisted Reprod Genet. (2019) 36:749–57. doi: 10.1007/s10815-019-01415-y
4. Li Y, Wang L, Xu J, Niu W, Shi H, Hu L, et al. Higher chromosomal aberration rate in miscarried conceptus from polycystic ovary syndrome women undergoing assisted reproductive treatment. Fertil Steril. (2019) 111:936–43 e2. doi: 10.1016/j.fertnstert.2019.01.026
5. Asgari F, Gavahi A, Karimi M, Vatannejad A, Amjadi F, Aflatoonian R, et al. Risk of embryo aneuploidy is affected by the increase in sperm DNA damage in recurrent implantation failure patients under ICSI-CGH array cycles. Hum Fertil. (2022) 25:872–80. doi: 10.1080/14647273.2021.1920054
6. Capelouto SM, Nagy ZP, Shapiro DB, Archer SR, Ellis DP, Smith AK, et al. Impact of male partner characteristics and semen parameters on in vitro fertilization and obstetric outcomes in a frozen oocyte donor model. Fertil Steril. (2018) 110:859–69. doi: 10.1016/j.fertnstert.2018.06.003
7. Wang Z, Liu X, Xu J, Yang Q, Niu W, Dai S, et al. Paternal age, body mass index, and semen volume are associated with chromosomal aberrations-related miscarriages in couples that underwent treatment by assisted reproductive technology. Aging. (2020) 12:8459–72. doi: 10.18632/aging.103151
8. Nguyen BT, Chang EJ, Bendikson KA. Advanced paternal age and the risk of spontaneous abortion: an analysis of the combined 2011-2013 and 2013-2015 National Survey of Family Growth. Am J obstetrics gynecology. (2019) 221:476.e1–.e7. doi: 10.1016/j.ajog.2019.05.028
9. Borini A, Tarozzi N, Bizzaro D, Bonu MA, Fava L, Flamigni C, et al. Sperm DNA fragmentation: paternal effect on early post-implantation embryo development in ART. Hum Reprod. (2006) 21:2876–81. doi: 10.1093/humrep/del251
10. Moore GE, Ishida M, Demetriou C, Al-Olabi L, Leon LJ, Thomas AC, et al. The role and interaction of imprinted genes in human fetal growth. Philos Trans R Soc Lond B Biol Sci. (2015) 370:20140074. doi: 10.1098/rstb.2014.0074
11. Agarwal A, Majzoub A, Baskaran S, Panner Selvam MK, Cho CL, Henkel R, et al. Sperm DNA fragmentation: A new guideline for clinicians. World J Mens Health. (2020) 38:412–71. doi: 10.5534/wjmh.200128
12. Menezo Y, Dale B, Cohen M. DNA damage and repair in human oocytes and embryos: a review. Zygote. (2010) 18:357–65. doi: 10.1017/S0967199410000286
13. Setti AS, Braga D, Provenza RR, Iaconelli A Jr., Borges E Jr. Oocyte ability to repair sperm DNA fragmentation: the impact of maternal age on intracytoplasmic sperm injection outcomes. Fertil Steril. (2021) 116:123–9. doi: 10.1016/j.fertnstert.2020.10.045
14. Titus S, Stobezki R, Oktay K. Impaired DNA repair as a mechanism for oocyte aging: is it epigenetically determined? Semin Reprod Med. (2015) 33:384–8. doi: 10.1055/s-0035-1567824
15. Winship AL, Stringer JM, Liew SH, Hutt KJ. The importance of DNA repair for maintaining oocyte quality in response to anti-cancer treatments, environmental toxins and maternal ageing. Hum Reprod Update. (2018) 24:119–34. doi: 10.1093/humupd/dmy002
16. McQueen DB, Zhang J, Robins JC. Sperm DNA fragmentation and recurrent pregnancy loss: a systematic review and meta-analysis. Fertil Steril. (2019) 112:54–60.e3. doi: 10.1016/j.fertnstert.2019.03.003
17. Borges E Jr., Zanetti BF, Setti AS, Braga D, Provenza RR, Iaconelli A Jr. Sperm DNA fragmentation is correlated with poor embryo development, lower implantation rate, and higher miscarriage rate in reproductive cycles of non-male factor infertility. Fertil Steril. (2019) 112:483–90. doi: 10.1016/j.fertnstert.2019.04.029
18. Deng C, Li T, Xie Y, Guo Y, Yang QY, Liang X, et al. Sperm DNA fragmentation index influences assisted reproductive technology outcome: A systematic review and meta-analysis combined with a retrospective cohort study. Andrologia. (2019) 51:e13263. doi: 10.1111/and.13263
19. Zhu C, Chen F, Zhang S, She H, Ju Y, Wen X, et al. Influence of sperm DNA fragmentation on the clinical outcome of in vitro fertilization-embryo transfer (IVF-ET). Front Endocrinol. (2022) 13:945242. doi: 10.3389/fendo.2022.945242
20. Enciso M, Alfarawati S, Wells D. Increased numbers of DNA-damaged spermatozoa in samples presenting an elevated rate of numerical chromosome abnormalities. Hum Reprod. (2013) 28:1707–15. doi: 10.1093/humrep/det077
21. Garcia-Ferreyra J, Luna D, Villegas L, Romero R, Zavala P, Hilario R, et al. High aneuploidy rates observed in embryos derived from donated oocytes are related to male aging and high percentages of sperm DNA fragmentation. Clin Med Insights Reprod Health. (2015) 9:21–7. doi: 10.4137/CMRH.S32769
22. Lin MH, Kuo-Kuang, Lee R, Li SH, Lu CH, Sun FJ, Hwu YM. Sperm chromatin structure assay parameters are not related to fertilization rates, embryo quality, and pregnancy rates in in vitro fertilization and intracytoplasmic sperm injection, but might be related to spontaneous abortion rates. Fertil Steril. (2008) 90:352–9. doi: 10.1016/j.fertnstert.2007.06.018
23. Wang Q, Wang X, Yu M, Sun H, Wang D, Zhong S, et al. Random sperm DNA fragmentation index is not associated with clinical outcomes in day-3 frozen embryo transfer. Asian J Androl. (2022) 24:109–15. doi: 10.4103/aja.aja_17_21
24. Pauta M, Grande M, Rodriguez-Revenga L, Kolomietz E, Borrell A. Added value of chromosomal microarray analysis over karyotyping in early pregnancy loss: systematic review and meta-analysis. Ultrasound Obstet Gynecol. (2018) 51:453–62. doi: 10.1002/uog.18929
25. Dhillon RK, Hillman SC, Morris RK, McMullan D, Williams D, Coomarasamy A, et al. Additional information from chromosomal microarray analysis (CMA) over conventional karyotyping when diagnosing chromosomal abnormalities in miscarriage: a systematic review and meta-analysis. BJOG. (2014) 121:11–21. doi: 10.1111/1471-0528.12382
26. Li J, Zhang F, Sun B, Dai S, Yang Q, Hu L, et al. Lower chromosomal abnormality frequencies in miscarried conceptuses from frozen blastocyst transfers in ART. Hum Reprod. (2021) 36:1146–56. doi: 10.1093/humrep/deaa352
27. Lathi RB, Milki AA. Tissue sampling technique affects accuracy of karyotype from missed abortions. J assisted Reprod Genet. (2002) 19:536–8. doi: 10.1023/a:1020916003666
28. World Health Organization. WHO Laboratory Manual for the Examination and Processing of Human Semen. 5th ed. Geneva, Switzerland: World Health Organization (2010).
29. Horta F, Catt S, Ramachandran P, Vollenhoven B, Temple-Smith P. Female ageing affects the DNA repair capacity of oocytes in IVF using a controlled model of sperm DNA damage in mice. Hum Reprod. (2020) 35:529–44. doi: 10.1093/humrep/dez308
30. Esteves SC, Santi D, Simoni M. An update on clinical and surgical interventions to reduce sperm DNA fragmentation in infertile men. Andrology. (2020) 8:53–81. doi: 10.1111/andr.12724
31. Esteves SC, Roque M, Bedoschi G, Haahr T, Humaidan P. Intracytoplasmic sperm injection for male infertility and consequences for offspring. Nat Rev Urol. (2018) 15:535–62. doi: 10.1038/s41585-018-0051-8
32. Gawecka JE, Marh J, Ortega M, Yamauchi Y, Ward MA, Ward WS. Mouse zygotes respond to severe sperm DNA damage by delaying paternal DNA replication and embryonic development. PloS One. (2013) 8:e56385. doi: 10.1371/journal.pone.0056385
33. Middelkamp S, van Tol HTA, Spierings DCJ, Boymans S, Guryev V, Roelen BAJ, et al. Sperm DNA damage causes genomic instability in early embryonic development. Sci Adv. (2020) 6:eaaz7602. doi: 10.1126/sciadv.aaz7602
34. Daughtry BL, Rosenkrantz JL, Lazar NH, Fei SS, Redmayne N, Torkenczy KA, et al. Single-cell sequencing of primate preimplantation embryos reveals chromosome elimination via cellular fragmentation and blastomere exclusion. Genome Res. (2019) 29:367–82. doi: 10.1101/gr.239830.118
35. Tesarik J, Greco E, Mendoza C. Late, but not early, paternal effect on human embryo development is related to sperm DNA fragmentation. Hum Reprod. (2004) 19:611–5. doi: 10.1093/humrep/deh127
36. Larsen EC, Christiansen OB, Kolte AM, Macklon N. New insights into mechanisms behind miscarriage. BMC Med. (2013) 11:154. doi: 10.1186/1741-7015-11-154
37. Wei D, Liu JY, Sun Y, Shi Y, Zhang B, Liu JQ, et al. Frozen versus fresh single blastocyst transfer in ovulatory women: a multicentre, randomised controlled trial. Lancet. (2019) 393:1310–8. doi: 10.1016/S0140-6736(18)32843-5
38. Hua L, Chen W, Meng Y, Qin M, Yan Z, Yang R, et al. The combination of DNA methylome and transcriptome revealed the intergenerational inheritance on the influence of advanced maternal age. Clin Trans Med. (2022) 12:e990. doi: 10.1002/ctm2.990
39. Kolano A, Brunet S, Silk AD, Cleveland DW, Verlhac MH. Error-prone mammalian female meiosis from silencing the spindle assembly checkpoint without normal interkinetochore tension. Proc Natl Acad Sci United States America. (2012) 109:E1858–67. doi: 10.1073/pnas.1204686109
40. Van Blerkom J. Mitochondrial function in the human oocyte and embryo and their role in developmental competence. Mitochondrion. (2011) 11:797–813. doi: 10.1016/j.mito.2010.09.012
41. Kalmbach KH, Fontes, Antunes DM, Dracxler RC, Knier TW, Seth-Smith ML, Wang F, et al. Telomeres and human reproduction. Fertil Steril. (2013) 99:23–9. doi: 10.1016/j.fertnstert.2012.11.039
42. Ozgur K, Berkkanoglu M, Bulut H, Humaidan P, Coetzee K. Perinatal outcomes after fresh versus vitrified-warmed blastocyst transfer: retrospective analysis. Fertil Steril. (2015) 104:899–907.e3. doi: 10.1016/j.fertnstert.2015.06.031
43. Teklenburg G, Salker M, Heijnen C, Macklon NS, Brosens JJ. The molecular basis of recurrent pregnancy loss: impaired natural embryo selection. Mol Hum Reprod. (2010) 16:886–95. doi: 10.1093/molehr/gaq079
44. Venkatesh S, Singh A, Shamsi MB, Thilagavathi J, Kumar R, Mitra DK, et al. Clinical significance of sperm DNA damage threshold value in the assessment of male infertility. Reprod Sci. (2011) 18:1005–13. doi: 10.1177/1933719111401662
45. Sakkas D, Alvarez JG. Sperm DNA fragmentation: mechanisms of origin, impact on reproductive outcome, and analysis. Fertil Steril. (2010) 93:1027–36. doi: 10.1016/j.fertnstert.2009.10.046
46. Mayer A, Baran V, Sakakibara Y, Brzakova A, Ferencova I, Motlik J, et al. DNA damage response during mouse oocyte maturation. Cell Cycle. (2016) 15:546–58. doi: 10.1080/15384101.2015.1128592
47. Gonzalez-Marin C, Gosalvez J, Roy R. Types, causes, detection and repair of DNA fragmentation in animal and human sperm cells. Int J Mol Sci. (2012) 13:14026–52. doi: 10.3390/ijms131114026
48. Spiller CM, Bowles J. Sex determination in mammalian germ cells. Asian J andrology. (2015) 17:427–32. doi: 10.4103/1008-682X.150037
49. Govindaraj V, Keralapura, Basavaraju R, Rao AJ. Changes in the expression of DNA double strand break repair genes in primordial follicles from immature and aged rats. Reprod biomedicine Online. (2015) 30:303–10. doi: 10.1016/j.rbmo.2014.11.010
50. Menezo Y Jr., Russo G, Tosti E, El, Mouatassim S, Benkhalifa M. Expression profile of genes coding for DNA repair in human oocytes using pangenomic microarrays, with a special focus on ROS linked decays. J assisted Reprod Genet. (2007) 24:513–20. doi: 10.1007/s10815-007-9167-0
51. Nagaoka SI, Hassold TJ, Hunt PA. Human aneuploidy: mechanisms and new insights into an age-old problem. Nat Rev Genet. (2012) 13:493–504. doi: 10.1038/nrg3245
52. Albani E, Castellano S, Gurrieri B, Arruzzolo L, Negri L, Borroni EM, et al. Male age: negative impact on sperm DNA fragmentation. Aging. (2019) 11:2749–61. doi: 10.18632/aging.101946
53. Yang Q, Zhao F, Dai S, Zhang N, Zhao W, Bai R, et al. Sperm telomere length is positively associated with the quality of early embryonic development. Hum Reprod. (2015) 30:1876–81. doi: 10.1093/humrep/dev144
54. Pino V, Sanz A, Valdés N, Crosby J, Mackenna A. The effects of aging on semen parameters and sperm DNA fragmentation. JBRA assisted Reprod. (2020) 24:82–6. doi: 10.5935/1518-0557.20190058
55. Yatsenko AN, Turek PJ. Reproductive genetics and the aging male. J assisted Reprod Genet. (2018) 35:933–41. doi: 10.1007/s10815-018-1148-y
Keywords: sperm DNA fragmentation, chromosomal abnormality, miscarriage, chromosomal microarray analysis, assisted reproductive technology
Citation: Fu W, Cui Q, Bu Z, Shi H, Yang Q and Hu L (2024) Elevated sperm DNA fragmentation is correlated with an increased chromosomal aneuploidy rate of miscarried conceptus in women of advanced age undergoing fresh embryo transfer cycle. Front. Endocrinol. 15:1289763. doi: 10.3389/fendo.2024.1289763
Received: 06 September 2023; Accepted: 20 February 2024;
Published: 08 April 2024.
Edited by:
Lara Tamburrino, University of Florence, ItalyReviewed by:
Marianna Santonastaso, Università degli Studi della Campania “Luigi Vanvitelli”, ItalyMosammat Begum, Infertility Care and Research Center (ICRC) Ltd, Bangladesh
Copyright © 2024 Fu, Cui, Bu, Shi, Yang and Hu. This is an open-access article distributed under the terms of the Creative Commons Attribution License (CC BY). The use, distribution or reproduction in other forums is permitted, provided the original author(s) and the copyright owner(s) are credited and that the original publication in this journal is cited, in accordance with accepted academic practice. No use, distribution or reproduction is permitted which does not comply with these terms.
*Correspondence: Linli Hu, aHVsaW5saTE5OTlAMTYzLmNvbQ==
†These authors share first authorship