- 1School of Acupuncture and Tuina, Chengdu University of Traditional Chinese Medicine, Chengdu, China
- 2The Fourth Clinical Medical College, Guangzhou University of Chinese Medicine, Shenzhen, China
- 3School of Clinical Medicine, Chengdu University of Traditional Chinese Medicine, Chengdu, China
- 4Chengdu Integrated TCM & Western Medicine Hospital/Chengdu First People’s Hospital, Chengdu, China
Objective: Investigating the association between inflammatory cytokines and hypothyroidism remains challenging due to limitations in traditional observational studies. In this study, we employed Mendelian randomization (MR) to assess the causal relationship between 41 inflammatory cytokines and hypothyroidism.
Method: Inflammatory cytokines in 30,155 individuals of European ancestry with hypothyroidism and in a GWAS summary containing 8,293 healthy participants were included in the study for bidirectional two-sample MR analysis. We utilized inverse variance weighting (IVW), weighted median (WM), and Mendelian randomization-Egger (MR-Egger) methods. Multiple sensitivity analyses, including MR-Egger intercept test, leave-one-out analysis, funnel plot, scatterplot, and MR-PRESSO, were applied to evaluate assumptions.
Results: We found evidence of a causal effect of IL-7 and macrophage inflammatory protein-1β (MIP-1β) on the risk of hypothyroidism, and a causal effect of hypothyroidism on several cytokines, including granulocyte colony-stimulating factor (G-CSF), IL-13, IL-16, IL-2rα, IL-6, IL-7, IL-9, interferon-γ-inducible protein 10 (IP10), monokine induced by interferon (IFN)-γ (MIG), macrophage inflammatory protein-1β (MIP-1β), stem cell growth factors-β (SCGF-β), stromal cell derived factor-1α (SDF-1α), and tumor necrosis factor-α (TNF-α).
Conclusion: Our study suggests that IL-7 and MIP-1β may play a role in the pathogenesis of hypothyroidism, and that hypothyroidism may induce a systemic inflammatory response involving multiple cytokines. These findings may have implications for the prevention and treatment of hypothyroidism and its complications. However, further experimental studies are needed to validate the causal relationships and the potential of these cytokines as drug targets.
1 Introduction
Hypothyroidism is a common endocrine disorder characterized by high levels of thyroid-stimulating hormone (TSH) and low levels of thyroid hormones, such as triiodothyronine (T3), thyroxine (T4), free triiodothyronine (FT3) and free thyroxine (FT4). The prevalence of hypothyroidism ranges from 0.2% to 5.3% (1, 2). Thyroid dysfunction affects various systems, such as metabolic, cardiovascular, neurological, and immune systems, and increases the risk of mortality (3).
In recent years, evidence has accumulated that inflammation is involved in the progression of hypothyroidism (4, 5). C-reactive protein (CRP), a hepatically synthesized acute-phase reactant, has a response to inflammatory cytokines, including IL-6 (6). Numerous studies have identified a notable positive association between TSH and CRP (7–9). Some cytokines, such as tumor necrosis factor-α (TNF-α) and IL-6, may inhibit the hypothalamic-pituitary-thyroid axis (10, 11). Additionally, it is worth noting that IL-6 levels have the potential to serve as an indicator of the extent of hypothyroidism. This is due to the observed negative correlation between serum IL-6 levels and FT4 levels in individuals diagnosed with autoimmune hypothyroidism (12). The administration of Levothyroxine (LRT) has the potential to decrease the levels of pro-inflammatory cytokines, specifically IL-6 and TNF-α (13). These findings suggest a possible bidirectional relationship between increased inflammatory markers and altered thyroid function; however, the effect of hypothyroidism treatment on systemic inflammation is inconsistent. For example, a study by Díez et al (14)., found no statistically significant decrease in elevated levels of TNF-α and TNF-α receptors following the restoration of thyroid function in individuals with hypothyroidism.
Therefore, the potential causal relationship between inflammatory cytokines and hypothyroidism requires further investigation. Most of the evidence on the relationship between inflammatory cytokines and hypothyroidism comes from observational studies, which are prone to reverse causality, selection bias, and confounding factors. Therefore, more research using innovative methods is needed.
Mendelian randomization (MR) is one such technique that utilizes genetic variation as an instrumental variable to assess causal relationships between exposures and specific outcomes (15).
2 Method
2.1 Data sources
The two datasets included in this MR research were obtained from publicly accessible aggregated genome-wide association study (GWAS) data. The hypothyroidism data were obtained from the MRCIEU GWAS database (https://gwas.mrcieu.ac.uk/), which includes 30,155 cases and 379,986 European pedigree controls. For data on inflammatory factors, we obtained genetic predictors from the Young Finns Cardiovascular Risk (YFS) study, and the Finnish Cardiovascular Risk Study 1997 and 2002 (FINRISK) (16), which included up to 8,293 Finnish participants.
Since all the data were derived from publicly accessible studies, our research did not require patient consent or ethical clearance.
2.2 Study design
Our study design was divided into two main steps: first, we performed a two-sample analysis with 41 inflammatory factors as exposure and hypothyroidism as outcome, respectively. Then, a two-sample analysis was performed with hypothyroidism as the exposure and 41 inflammatory factors as the outcome, respectively.
2.3 Instrumental variable selection
In MR studies, genetic variants frequently serve as instrumental variables (IVs). Three critical assumptions must be met to obtain reliable casual estimates in MR studies: 1) IVs must show a high correlation with the exposure; 2) IVs must not be connected to any possible confounders that could skew the results of the exposure-outcome link; 3) IVs should only affect the result by means of exposure (17, 18). Figure 1 depicts a detailed description.
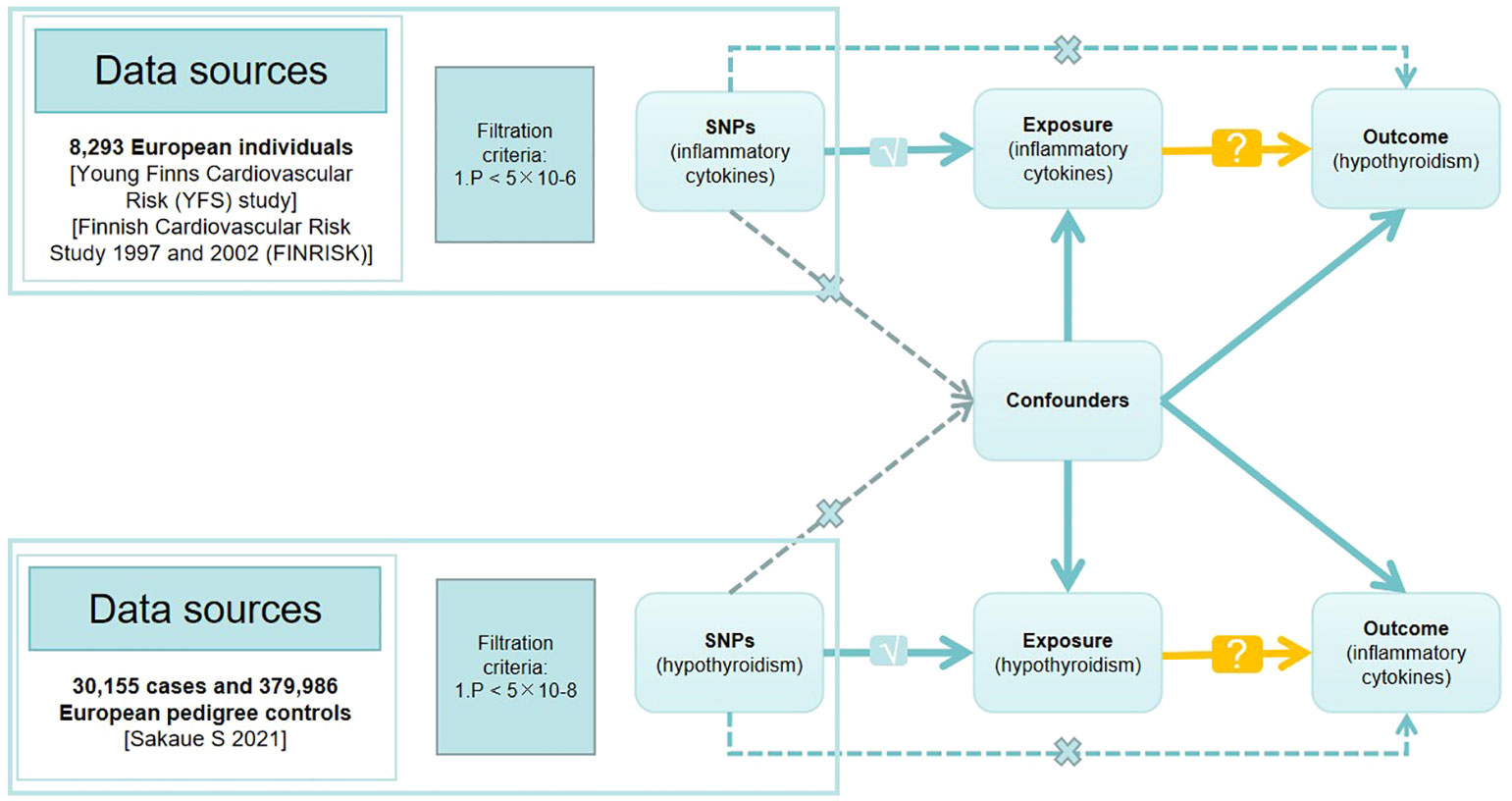
Figure 1 Directed acyclic graph of the Mendelian randomization (MR) framework investigating the causal relationship between exposure and outcome. The ‘×’ means that genetic variants are not associated with confounders or cannot be directly involved in outcome but via the exposure pathway. The ‘√’ means that genetic variants are highly correlated with exposure. SNPs, single-nucleotide polymorphisms.
The genome-wide significance criterion of p<5 × 10-8 was employed to identify SNPs that were significantly linked to both hypothyroidism and inflammatory cytokines. Since only a few SNPs were found, we adopted a higher cutoff (p<5 × 10-6) for inflammatory cytokines as the exposure. After that, we used SNP clustering to eliminate linkage disequilibrium (LD) with a 10,000 kb window size and an R2<0.001 criterion. Subsequently, we checked all the exposure (hypothyroidism) related SNPs in the PhenoScanner database (http://www.phenoscanner.medschl.cam.ac.uk/) to identify any SNPs associated with potential confounders (BMI, smoking, alcohol consumption) and the outcome. We extracted SNP effects from the outcome GWAS dataset and harmonized the impact of the exposure and outcome. We excluded palindromic SNPs with ambiguous results (EAF >0.42). To find and eliminate any outliers, we used the MR-Pleiotropy Residual Sum and Outlier (MR-PRESSO) technique (19). Finally, we assessed instrumental strength using the F-statistic (), following the procedures outlined by Burgess and Thompson (20).
3 Mendelian randomization analyses
For our MR analyses, we employed R (version 4.2.2) packages, namely “TwoSampleMR” (version 0.5.6) and “MR-PRESSO” (version 1.0).
We assessed the association between inflammatory factors and hypothyroidism using three methods: inverse variance weighted (IVW), weighted median (WM), and Mendelian randomization-Egger (MR-Egger) methods. IVW method offers reliable and consistent results under the assumption that all genetic variants used as instruments are legitimate instrumental variables valid IVs (21). Conversely, MR-Egger regression yields reliable estimates, particularly in cases where all the genetic variants under consideration are invalid IVs. The weighted median approach generates consistent appraisals, requiring at least half of the weights to be derived from accurate IVs (22). Our primary result was based on the IVW method, while MR-Egger and WM approaches were used to assess the reliability and stability of the results.
We used the MR-Egger intercept test to detect horizontal pleiotropy, with a P-intercept >0.05 indicating the absence of such pleiotropy. We further employed the IVW method and Egger regression to evaluate heterogeneity, with P<0.05 indicating its presence. Cochran’s Q statistic was used to assess heterogeneity (23). Moreover, we conducted a leave-one-out analysis to investigate whether a single SNP was driving the causal association.
3.1 The effect of inflammatory cytokines on hypothyroidism
When the cutoff value for genome-wide significance was established at 5 × 10-8, only 9 of the 41 available inflammatory cytokines had 3 or more SNPs. Therefore, a higher criterion (P< 5 × 10-6) was employed to guarantee an adequate number of SNPs for further MR analysis. The F-statistic values of the SNPs associated with each inflammatory cytokine were higher than 10, indicating less weak instrumental bias (Supplementary Table 1).
Interleukin-l receptor antagonist (IL-1rα) and M-CSF still failed to be extracted as valid instrumental variables. In Figures 2A and 3A, the primary MR analyses of inflammatory cytokines are displayed. By the IVW method, it was found that the level of IL-7 was associated with increased odds of developing hypothyroidism (IVW-OR: 1.042, 95% CI: 1.004-1.081, p = 0.028). Though the WM technique and MR Egger analysis were unable to identify a statistically significant link, they revealed a similar tendency (WM-OR: 1.045, 95% CI: 0.993-1.100, p = 0.088; MR Egger-OR: 1.039, 95% CI: 0.961-1.123, p = 0.356). The same causal relationship was found between MIP-1β and hypothyroidism (IVW-OR: 1.034, 95% CI: 1.004-1.064, p = 0.027). Similarly, the WM technique and MR Egger analysis were unable to identify a statistically significant link, they revealed a similar tendency (WM-OR: 1.033, 95% CI: 0.995-1.074, p = 0.092; MR Egger-OR: 1.000, 95% CI: 0.943-1.061, p = 0.992). Supplementary Figures 1–3 illustrate the scatter plot, funnel plots, and leave-one-out analyses of IL-7 and MIC-1β in hypothyroidism.
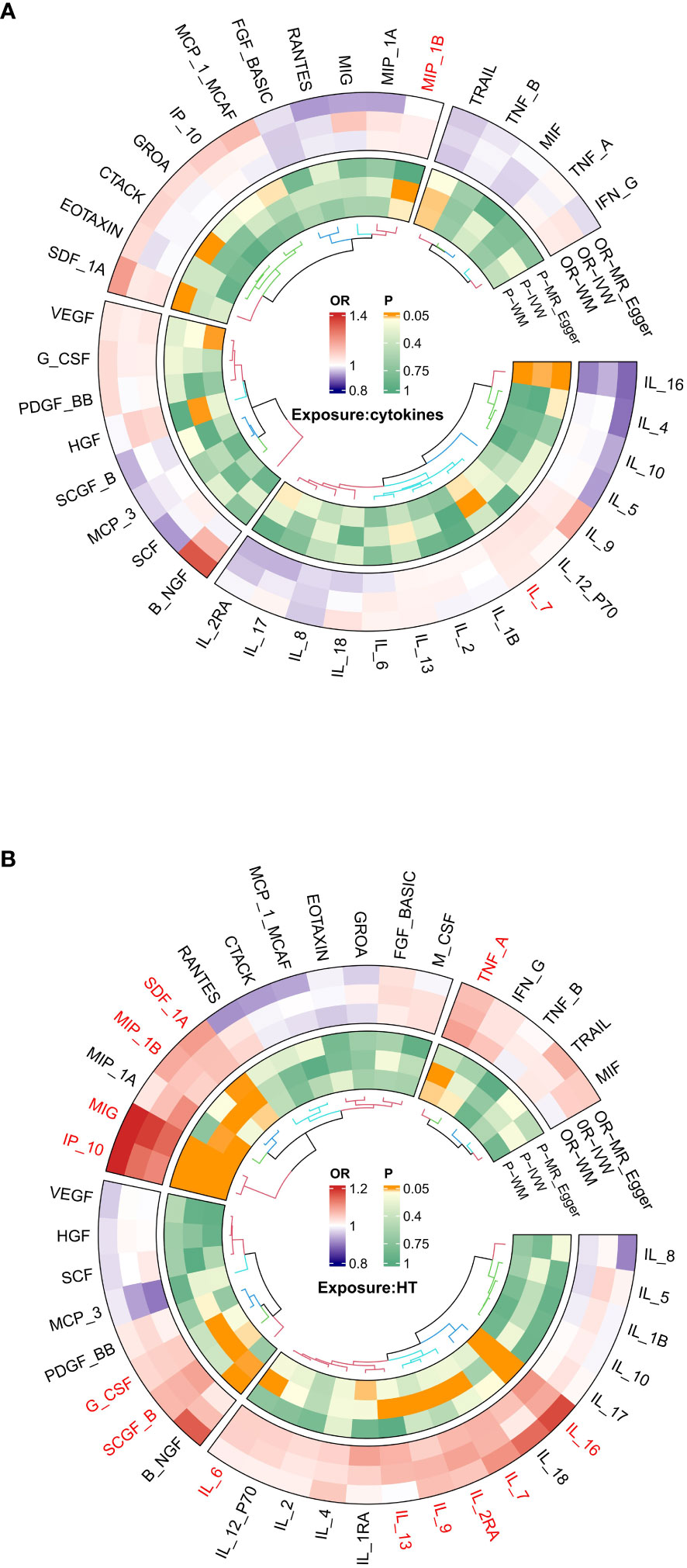
Figure 2 (A) Ring heat map of Mendelian randomization analysis of the causal effect of inflammatory cytokines on hypothyroidism. (B) Ring heat map of Mendelian randomization analysis of the causal effect of hypothyroidism on inflammatory cytokines.
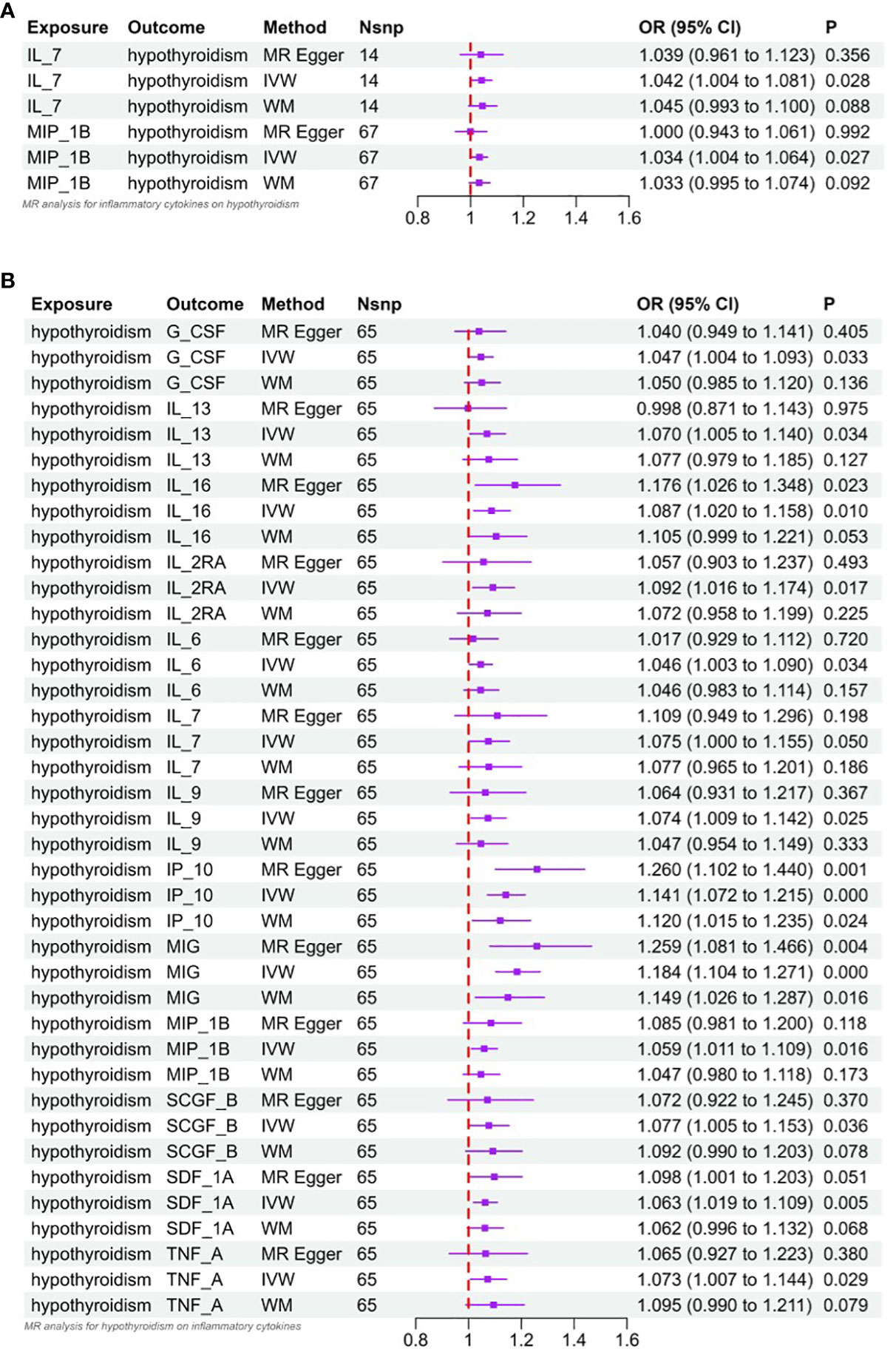
Figure 3 (A) Forest plot of Mendelian randomized analysis of causal effects of inflammatory cytokines on hypothyroidism. (B) Forest plot of Mendelian randomized analysis of causal effects of hypothyroidism on inflammatory cytokines.
According to Cochrane’s Q (Q_pval > 0.05), there was no evidence of heterogeneity in the correlation between IL-7 and MIP-1β, and no outlier SNPs were found using the MR-PRESSO technique. In addition, the MR-Egger intercept did not find evidence of horizontal pleiotropy (P = 0.935; P = 0.218) (Supplementary Table 2). Scatterplots, funnel plots, and leave-one-out analyses of Mendelian randomization analyses of the role of IL-7 and MIP-1β in hypothyroidism are shown in Supplementary Figures 4–6.
3.2 Effect of hypothyroidism on inflammatory cytokines
When TNF-β was used as an outcome, we extracted 61 SNPs as IVs for hypothyroidism. whereas additionally when 40 inflammatory cytokines were used as outcomes, we extracted 65 significant SNPs as IVs for hypothyroidism.
The results of MR analysis regarding the causal relationship between hypothyroidism and inflammatory cytokines are shown in Figures 2B and 3B. The results of the IVW method showed a suggestive correlation between hypothyroidism and elevated levels of colony-stimulating factor (G-CSF), IL-13, IL-16, IL-2rα, IL-6, IL-7, IL-9, IP10, interferon-γ-inducible protein 10 (IP10), monokine induced by interferon (IFN)-γ (MIG), macrophage inflammatory protein-1β (MIP-1β), stem cell growth factors-β (SCGF-β), stromal cell derived factor-1α (SDF-1α), and TNF-α.
In the sensitivity analysis, Cochran’s Q test (Q_pval > 0.05) showed that there was no heterogeneity in the validated IVs used to estimate the effect of hypothyroidism on inflammatory cytokines. In addition, there was no evidence of horizontal pleiotropy (MR-Egger interception P > 0.05) in any of the MR analyses of the effect of hypothyroidism on inflammatory cytokines (Supplementary Table 2). Scatter plots, funnel plots, and leave-one-out analyses of inflammatory cytokines suggestive of relevance are shown in Supplementary Figures 2 and 3.
4 Discussion
This study employed a bidirectional two-sample MR approach to investigate the potential causal association between inflammatory cytokines and hypothyroidism. Our results suggested that IL-7 and MIP-1β might cause hypothyroidism. Conversely, when we treated hypothyroidism as an exposure factor, we detected a causal association between hypothyroidism and 13 inflammatory cytokines (IL-13, IL-16, IL-2rα, IL-6, IL-7, IL-9, G-CSF, SCGF-β, IP-10, MIG, MIP-1β, SDF-1α, and TNF-α).
Several studies have shown that patients with hypothyroidism have increased levels of inflammatory cytokines (14, 24–30). Chronic autoimmune thyroiditis (CAT) is the main cause of hypothyroidism in iodine-sufficient countries (31), and cytokines are involved in autoimmune thyroid disease through various mechanisms. They are produced by inflammatory cells such as lymphocytes and by thyroid follicular cells (TFC) in the thyroid gland. In autoimmune hypothyroidism tissue samples, gene expression of IL-1, IL-2, IL-4, IL-6, IL-8, IL-10, IL-12, IL-13, IL-15, IL-16, IL-18, IFN-γ, TNFα, and some chemokines has been observed (32–35). Gene expression of IL-2, IL-4, IL-6, IL-8, IL-10, IL-13, IL-15, IL-16, IL-17, IFN-γ, and TNFα has also been detected in autoimmune thyroid disease-derived lymphocytes (infiltrating thyroid lymphocyte, ITL). Moreover, TFC can produce cytokines themselves. TFC can express mRNAs for IL-1, IL-6, IL-8, IL-12, IL-13, IL-15, IL-16, IL-17, IL-18, TNFα, MIP-1α, MIP-1β, MCP-1, RANTES, IP-10, and MIG (32, 34–37).
The clinical status of the patient may influence the type of immune response, with Th1-type T cells predominating in hypothyroid patients, whereas euthyroid patients with autoimmune thyroiditis have a mixed Th1/Th2 response (38, 39). The accumulation of Th1 lymphocytes stimulates the overproduction of IFN-γ and TNF-α, which further activate various cells to secrete IFN-γ, creating a positive feedback loop at the site of inflammation and resulting in elevated levels of IFN-γ (39, 40). IP-10 is a chemokine-induced by IFN-γ stimulation. It can be secreted by IFN-γ-activated thyroid cells. Therefore, high levels of IP-10 in peripheral blood indicate a Th1-polarized immune response. Previous studies have shown that patients with autoimmune thyroiditis have increased circulating IP-10 levels (41).
Tayde et al. (41) reported that hypothyroid patients had higher levels of TNF-α, IL-6, and CRP than healthy controls and that levothyroxine treatment reduced but did not normalize these cytokines. Another study also found that levothyroxine treatment modulated the inflammatory profile of hypothyroid patients, decreasing pro-inflammatory cytokines and increasing anti-inflammatory cytokines (13). Antunes et al. (42) showed that TSH activated IL-6, a pro-inflammatory cytokine, in preadipocytes via the cAMP protein kinase A signaling pathway. Whetsell et al. (43) demonstrated that TSH induced TNF-α in myeloid cells. Moreover, several in vitro studies have indicated that TSH can affect cytokine release in different cell types (42–46). Therefore, lower TSH levels may lead to lower levels of several inflammatory factors. Conversely, recombinant human TSH administration can cause a significant increase in blood cytokine levels in patients with differentiated thyroid cancer (47). IFN-γ can inhibit the transcription of the TSH-R gene, which may result in a general suppression of thyroid function in vivo (48).
Thyroid dysfunction is associated with IFNα treatment for malignant tumors or chronic hepatitis (49). A long-term study on the effects of IFNα treatment on thyroid function showed that high levels of thyroid autoantibodies, especially the combination of TPO and TG antibodies, after IFNα treatment were predictive factors for thyroid dysfunction (50). IFNβ treatment for multiple sclerosis may also cause thyroid dysfunction, but this is controversial (51, 52). However, IFN-γ application in humans does not affect thyroid function or thyroid autoantibody production (53). The mechanism of cytokine therapy-induced thyroid dysfunction is unclear. One possibility is that some cytokines have broad immunomodulatory effects that trigger the development of autoimmune thyroid disease (54). Moreover, as mentioned earlier, cytokines can also directly interfere with TFC function and immune responses, which may be a major factor in cytokine therapy-related thyroid dysfunction. Steroid therapy can increase IL-4 and IL-10 levels, suggesting that these anti-inflammatory cytokines may contribute to disease remission (55).
We conducted the first MR study to assess the causal relationship between hypothyroidism and 41 inflammatory cytokines. However, our study had some limitations. First, we used an MR design that excluded known confounders, but unmeasured confounders may still bias the results, which may influence both hypothyroidism and cytokine levels. Second, we focused only on individuals of European ancestry, which may reduce the applicability of our findings to other populations. Therefore, our results should be interpreted with caution for broader populations. In addition, because of the large geographic variation in the incidence of hypothyroidism itself, it is subject to large geographic influences, even for individuals of the same European ancestry. Third, due to limitations in the raw GWAS data, we were unable to stratify the studies by sex. This is a major drawback, as hypothyroidism is more prevalent and severe in women than in men, and sex hormones may modulate the immune response and cytokine production. Therefore, our results may not capture the potential sex-specific effects of hypothyroidism on cytokine levels. Future studies should explore the possible interactions between sex, hypothyroidism, and cytokines, and adjust for sex as a potential confounder or effect modifier. Finally, hypothyroidism has a complex etiology with multiple subtypes, such as autoimmune, iodine deficiency, or drug-induced hypothyroidism, which were not considered in our MR analysis. These subtypes may have different mechanisms and consequences for the inflammatory response and cytokine levels. Therefore, our results may not reflect the heterogeneity and specificity of the relationship between hypothyroidism and cytokines. Future studies should classify hypothyroidism according to its etiology and subtype, and compare the effects of different subtypes on cytokine levels.
5 Conclusion
Our study investigated the potential causal relationship between 41 inflammatory cytokines and hypothyroidism using a bidirectional two-sample MR analysis technique. We report several strong associations, but further experimental validation is needed to assess the potential of these cytokines to be used as drugs to prevent hypothyroidism. Furthermore, the study of causality is advantageous for disease prevention, as well as for promoting the refinement and advancement of treatment. It is necessary to conduct larger epidemiological or metagenomic studies to attain a more comprehensive understanding of the observed correlation between the susceptibility of hypothyroidism in patients with specific inflammatory cytokines.
Data availability statement
The original contributions presented in the study are included in the article/Supplementary Material. Further inquiries can be directed to the corresponding authors.
Ethics statement
Ethical review and approval were not required for the study on human participants in accordance with the local legislation and institutional requirements. Written informed consent from the participant’s legal guardian/next of kin was not required to participate in this study in accordance with the national legislation and institutional requirements.
Author contributions
RL: Conceptualization, Data curation, Formal analysis, Methodology, Writing – original draft, Writing – review & editing. BY: Visualization, Writing – original draft, Writing – review & editing. ZF: Data curation, Visualization, Writing – original draft. XD: Data curation, Visualization, Writing – original draft. XL: Data curation, Visualization, Writing – original draft. YZ: Supervision, Writing – review & editing. DP: Supervision, Writing – review & editing.
Funding
The author(s) declare financial support was received for the research, authorship, and/or publication of this article. This work was supported by Sichuan Provincial Administration of Traditional Chinese Medicine (2021MS090).
Acknowledgments
The authors thank the studies or consortiums referenced and included in the present analysis for providing public datasets.
Conflict of interest
The authors declare that the research was conducted in the absence of any commercial or financial relationships that could be construed as a potential conflict of interest.
Publisher’s note
All claims expressed in this article are solely those of the authors and do not necessarily represent those of their affiliated organizations, or those of the publisher, the editors and the reviewers. Any product that may be evaluated in this article, or claim that may be made by its manufacturer, is not guaranteed or endorsed by the publisher.
Supplementary material
The Supplementary Material for this article can be found online at: https://www.frontiersin.org/articles/10.3389/fendo.2023.1332383/full#supplementary-material
References
1. Garmendia Madariaga A, Santos Palacios S, Guillen-Grima F, Galofre JC. The incidence and prevalence of thyroid dysfunction in Europe: a meta-analysis. J Clin Endocrinol Metab (2014) 99(3):923–31. doi: 10.1210/jc.2013-2409
2. Rosario PW. Subclinical hypothyroidism. New Engl J Med (2017) 377(14):1404. doi: 10.1056/NEJMc1709853
3. Roberts CGP, Ladenson PW. Hypothyroidism. Lancet (London England) (2004) 363(9411):793–803. doi: 10.1016/S0140-6736(04)15696-1
4. Mancini A, Di Segni C, Raimondo S, Olivieri G, Silvestrini A, Meucci E, et al. Thyroid hormones, oxidative stress, and inflammation. Mediators Inflammation (2016) 2016:6757154. doi: 10.1155/2016/6757154
5. Taherinia S, Heidari Z, Salehidoost R, Karimifar M, Arab A, Alshahrani SH, et al. Associations between empirically derived dietary patterns and oxidative stress and inflammation in adults with primary hypothyroidism: a case-control study. BMC Endocr Disord (2023) 23(1):105. doi: 10.1186/s12902-023-01348-9
6. Blake GJ, Ridker PM. Inflammatory bio-markers and cardiovascular risk prediction. J Internal Med (2002) 252(4):283–94. doi: 10.1046/j.1365-2796.2002.01019.x
7. Sharma R, Sharma TK, Kaushik GG, Sharma S, Vardey SK, Sinha M. Subclinical hypothyroidism and its association with cardiovascular risk factors. Clin Lab (2011) 57(9-10):719–24.
8. Gupta G, Sharma P, Kumar P, Itagappa M. Study on subclinical hypothyroidism and its association with various inflammatory markers. J Clin Diagn Res JCDR (2015) 9(11):BC04–6. doi: 10.7860/JCDR/2015/14640.6806
9. Wu J, Tao Y, Gu H, Sui J. Association between cardiovascular risk factors and serum thyrotropin concentration among healthy Chinese subjects and subjects with unsuspected subclinical hypothyroidism. Clin Lab (2016) 62(5):807–14. doi: 10.7754/Clin.Lab.2015.150809
10. Pang XP, Hershman JM, Mirell CJ, Pekary AE. Impairment of hypothalamic-pituitary-thyroid function in rats treated with human recombinant tumor necrosis factor-alpha (cachectin). Endocrinology (1989) 125(1):76–84. doi: 10.1210/endo-125-1-76
11. van Haasteren GA, van der Meer MJ, Hermus AR, Linkels E, Klootwijk W, Kaptein E, et al. Different effects of continuous infusion of interleukin-1 and interleukin-6 on the hypothalamic-hypophysial-thyroid axis. Endocrinology (1994) 135(4):1336–45. doi: 10.1210/endo.135.4.7925094
12. Papanas N, Papazoglou D, Papatheodorou K, Antonoglou C, Kotsiou S, Maltezos E. Thyroxine replacement dose in patients with Hashimoto disease: a potential role for interleukin-6. Cytokine (2006) 35(3-4):166–70. doi: 10.1016/j.cyto.2006.07.017
13. Marchiori RC, Pereira LA, Naujorks AA, Rovaris DL, Meinerz DF, Duarte MM, et al. Improvement of blood inflammatory marker levels in patients with hypothyroidism under levothyroxine treatment. BMC Endocr Disord (2015) 15:32. doi: 10.1186/s12902-015-0032-3
14. Díez JJ, Hernanz A, Medina S, Bayon C, Iglesias P. Serum concentrations of tumour necrosis factor-alpha (TNF-alpha) and soluble TNF-alpha receptor p55 in patients with hypothyroidism and hyperthyroidism before and after normalization of thyroid function. Clin Endocrinol (2002) 57(4):515–21. doi: 10.1046/j.1365-2265.2002.01629.x
15. Davey Smith G, Ebrahim S. What can mendelian randomisation tell us about modifiable behavioural and environmental exposures? BMJ (Clinical Res ed.) (2005) 330(7499):1076–9. doi: 10.1136/bmj.330.7499.1076
16. Ahola-Olli AV, Wurtz P, Havulinna AS, Aalto K, Pitkanen N, Lehtimaki T, et al. Genome-wide association study identifies 27 loci influencing concentrations of circulating cytokines and growth factors. Am J Hum Genet (2017) 100(1):40–50. doi: 10.1016/j.ajhg.2016.11.007
17. Smith GD, Ebrahim S. 'Mendelian randomization': can genetic epidemiology contribute to understanding environmental determinants of disease? Int J Epidemiol (2003) 32(1):1–22. doi: 10.1093/ije/dyg070
18. Davey Smith G, Hemani G. Mendelian randomization: genetic anchors for causal inference in epidemiological studies. Hum Mol Genet (2014) 23(R1):R89–98. doi: 10.1093/hmg/ddu328
19. Verbanck M, Chen CY, Neale B, Do R. Detection of widespread horizontal pleiotropy in causal relationships inferred from Mendelian randomization between complex traits and diseases. Nat Genet (2018) 50(5):693–8. doi: 10.1038/s41588-018-0099-7
20. Burgess S, Thompson SG. Avoiding bias from weak instruments in Mendelian randomization studies. Int J Epidemiol (2011) 40(3):755–64. doi: 10.1093/ije/dyr036
21. Burgess S, Butterworth A, Thompson SG. Mendelian randomization analysis with multiple genetic variants using summarized data. Genet Epidemiol (2013) 37(7):658–65. doi: 10.1002/gepi.21758
22. Bowden J, Del Greco MF, Minelli C, Davey Smith G, Sheehan NA, Thompson JR. Assessing the suitability of summary data for two-sample Mendelian randomization analyses using MR-Egger regression: the role of the I2 statistic. Int J Epidemiol (2016) 45(6):1961–74. doi: 10.1093/ije/dyw220
23. Bowden J, Davey Smith G, Haycock PC, Burgess S. Consistent estimation in Mendelian randomization with some invalid instruments using a weighted median estimator. Genet Epidemiol (2016) 40(4):304–14. doi: 10.1002/gepi.21965
24. Marfella R, Ferraraccio F, Rizzo MR, Portoghese M, Barbieri M, Basilio C, et al. Innate immune activity in plaque of patients with untreated and L-thyroxine-treated subclinical hypothyroidism. J Clin Endocrinol Metab (2011) 96(4):1015–20. doi: 10.1210/jc.2010-1382
25. Zhu C, Gao J, Mei F, Lu L, Zhou D, Qu S. Reduction in thyroid-stimulating hormone correlated with improved inflammation markers in Chinese patients with morbid obesity undergoing laparoscopic sleeve gastrectomy. Obes Surg (2019) 29(12):3954–65. doi: 10.1007/s11695-019-04063-4
26. Tayde PS, Bhagwat NM, Sharma P, Sharma B, Dalwadi PP, Sonawane A, et al. Hypothyroidism and depression: are cytokines the link? Indian J Endocrinol Metab (2017) 21(6):886–92. doi: 10.4103/ijem.IJEM_265_17
27. Türemen EE, Cetinarslan B, Sahin T, Canturk Z, Tarkun I. Endothelial dysfunction and low grade chronic inflammation in subclinical hypothyroidism due to autoimmune thyroiditis. Endocr J (2011) 58(5):349–54. doi: 10.1507/endocrj.K10E-333
28. Gómez-Zamudio JH, Mendoza-Zubieta V, Ferreira-Hermosillo A, Molina-Ayala MA, Valladares-Salgado A, Suarez-Sanchez F, et al. High thyroid-stimulating hormone levels increase proinflammatory and cardiovascular markers in patients with extreme obesity. Arch Med Res (2016) 47(6):476–82. doi: 10.1016/j.arcmed.2016.10.007
29. Dizdarevic-Bostandic A, Burekovic A, Velija-Asimi Z, Godinjak A. Inflammatory markers in patients with hypothyroidism and diabetes mellitus type 1. Med Arch (Sarajevo Bosnia Herzegovina) (2013) 67(3):160–1. doi: 10.5455/medarh.2013.67.160-161
30. Escobar-Morreale HF, Escobar del Rey F, Morreale de Escobar G. Thyroid hormones influence serum leptin concentrations in the rat. Endocrinology (1997) 138(10):4485–8. doi: 10.1210/endo.138.10.5569
31. Weetman AP, McGregor AM. Autoimmune thyroid disease: further developments in our understanding. Endocr Rev (1994) 15(6):788–830. doi: 10.1210/edrv-15-6-788
32. Ajjan RA, Watson PF, Weetman AP. Cytokines and thyroid function. Adv In Neuroimmunol (1996) 6(4):359–86. doi: 10.1016/S0960-5428(97)00027-7
33. Ashhab Y, Dominguez O, Sospedra M, Roura-Mir C, Lucas-Martin A, Pujol-Borrell R. A one-tube polymerase chain reaction protocol demonstrates CC chemokine overexpression in Graves' disease glands. J Clin Endocrinol Metab (1999) 84(8):2873–82. doi: 10.1210/jcem.84.8.5909
34. Takiyama Y, Miyokawa N, Tokusashi Y, Ito K, Kato S, Kimura S, et al. Thyroid-stimulating hormone induces interleukin-18 gene expression in FRTL-5 cells: immunohistochemical detection of interleukin-18 in autoimmune thyroid disease. Thyroid (2002) 12(11):935–43. doi: 10.1089/105072502320908268
35. Ajjan RA, Weetman AP. Cytokines in thyroid autoimmunity. Autoimmunity (2003) 36(6-7):351–9. doi: 10.1080/08916930310001603046
36. García-López MA, Sancho D, Sanchez-Madrid F, Marazuela M. Thyrocytes from autoimmune thyroid disorders produce the chemokines IP-10 and Mig and attract CXCR3+ lymphocytes. J Clin Endocrinol Metab (2001) 86(10):5008–16. doi: 10.1210/jcem.86.10.7953
37. Kemp EH, Metcalfe RA, Smith KA, Woodroofe MN, Watson PF, Weetman AP. Detection and localization of chemokine gene expression in autoimmune thyroid disease. Clin Endocrinol (2003) 59(2):207–13. doi: 10.1046/j.1365-2265.2003.01824.x
38. Mazziotti G, Sorvillo F, Naclerio C, Farzati A, Cioffi M, Perna R, et al. Type-1 response in peripheral CD4+ and CD8+ T cells from patients with Hashimoto's thyroiditis. Eur J Endocrinol (2003) 148(4):383–8. doi: 10.1530/eje.0.1480383
39. Antonelli A, Ferrari SM, Giuggioli D, Ferrannini E, Ferri C, Fallahi P. Chemokine (C-X-C motif) ligand (CXCL)10 in autoimmune diseases. Autoimmun Rev (2014) 13(3):272–80. doi: 10.1016/j.autrev.2013.10.010
40. Liu C, Papewalis C, Domberg J, Scherbaum WA, Schott M. Chemokines and autoimmune thyroid diseases. Hormone Metab Res = Hormon- Und Stoffwechselforschung = Hormones Et Metabolisme (2008) 40(6):361–8. doi: 10.1055/s-2008-1073153
41. Antonelli A, Rotondi M, Fallahi P, Romagnani P, Ferrari SM, Buonamano A, et al. High levels of circulating CXC chemokine ligand 10 are associated with chronic autoimmune thyroiditis and hypothyroidism. J Clin Endocrinol Metab (2004) 89(11):5496–9. doi: 10.1210/jc.2004-0977
42. Antunes TT, Gagnon A, Bell A, Sorisky A. Thyroid-stimulating hormone stimulates interleukin-6 release from 3T3-L1 adipocytes through a cAMP-protein kinase A pathway. Obes Res (2005) 13(12):2066–71. doi: 10.1038/oby.2005.256
43. Whetsell M, Bagriacik EU, Seetharamaiah GS, Prabhakar BS, Klein JR. Neuroendocrine-induced synthesis of bone marrow-derived cytokines with inflammatory immunomodulating properties. Cell Immunol (1999) 192(2):159–66. doi: 10.1006/cimm.1998.1444
44. Antunes TT, Gagnon A, Langille ML, Sorisky A. Thyroid-stimulating hormone induces interleukin-6 release from human adipocytes through activation of the nuclear factor-kappaB pathway. Endocrinology (2008) 149(6):3062–6. doi: 10.1210/en.2007-1588
45. Zhang YJ, Zhao W, Zhu MY, Tang SS, Zhang H. Thyroid-stimulating hormone induces the secretion of tumor necrosis factor-α from 3T3-L1 adipocytes via a protein kinase A-dependent pathway. Exp Clin Endocrinol Diabetes (2013) 121(8):488–93. doi: 10.1055/s-0033-1347266
46. Gagnon A, Langille ML, Chaker S, Antunes TT, Durand J, Sorisky A. TSH signaling pathways that regulate MCP-1 in human differentiated adipocytes. Metabolism: Clin Exp (2014) 63(6):812–21. doi: 10.1016/j.metabol.2014.02.015
47. Dardano A, Ghiadoni L, Plantinga Y, Caraccio N, Bemi A, Duranti E, et al. Recombinant human thyrotropin reduces endothelium-dependent vasodilation in patients monitored for differentiated thyroid carcinoma. J Clin Endocrinol Metab (2006) 91(10):4175–8. doi: 10.1210/jc.2006-0440
48. Nishikawa T, Yamashita S, Namba H, Usa T, Tominaga T, Kimura H, et al. Interferon-gamma inhibition of human thyrotropin receptor gene expression. J Clin Endocrinol Metab (1993) 77(4):1084–9. doi: 10.1210/jcem.77.4.8408457
49. Deutsch M, Dourakis S, Manesis EK, Gioustozi A, Hess G, Horsch A, et al. Thyroid abnormalities in chronic viral hepatitis and their relationship to interferon alfa therapy. Hepatol (Baltimore Md.) (1997) 26(1):206–10. doi: 10.1002/hep.510260127
50. Carella C, Mazziotti G, Morisco F, Manganella G, Rotondi M, Tuccillo C, et al. Long-term outcome of interferon-alpha-induced thyroid autoimmunity and prognostic influence of thyroid autoantibody pattern at the end of treatment. J Clin Endocrinol Metab (2001) 86(5):1925–9. doi: 10.1210/jcem.86.5.7459
51. Monzani F, Caraccio N, Meucci G, Lombardo F, Moscato G, Casolaro A, et al. Effect of 1-year treatment with interferon-beta1b on thyroid function and autoimmunity in patients with multiple sclerosis. Eur J Endocrinol (1999) 141(4):325–31. doi: 10.1530/eje.0.1410325
52. Durelli L, Ferrero B, Oggero A, Verdun E, Ghezzi A, Montanari E, et al. Liver and thyroid function and autoimmunity during interferon-beta 1b treatment for MS. Neurology (2001) 57(8):1363–70. doi: 10.1212/WNL.57.8.1363
53. de Metz J, Romijn JA, Endert E, Corssmit EP, Sauerwein HP. Administration of interferon-gamma in healthy subjects does not modulate thyroid hormone metabolism. Thyroid (2000) 10(1):87–91. doi: 10.1089/thy.2000.10.87
54. Imagawa A, Itoh N, Hanafusa T, Oda Y, Waguri M, Miyagawa J, et al. Autoimmune endocrine disease induced by recombinant interferon-alpha therapy for chronic active type C hepatitis. J Clin Endocrinol Metab (1995) 80(3):922–6. doi: 10.1210/jcem.80.3.7883851
55. Myśliwiec J, Kretowski A, Topolska J, Siewko K, Jakubczyk D, Szelachowska M, et al. Serum Th1 and Th2 profile cytokine level changes in patients with Graves' ophthalmopathy treated with corticosteroids. Hormone Metab Res = Hormon- Und Stoffwechselforschung = Hormones Et Metabolisme (2001) 33(12):739–43. doi: 10.1055/s-2001-19135
Keywords: Mendelian randomization, inflammatory, cytokines, hypothyroidism, causal relationship
Citation: Lai R, Yin B, Feng Z, Deng X, Lv X, Zhong Y and Peng D (2024) The causal relationship between 41 inflammatory cytokines and hypothyroidism: bidirectional two-sample Mendelian randomization study. Front. Endocrinol. 14:1332383. doi: 10.3389/fendo.2023.1332383
Received: 07 November 2023; Accepted: 20 December 2023;
Published: 22 January 2024.
Edited by:
Joseph V. Martin, Rutgers University Camden, United StatesReviewed by:
José Luis Fachi, Washington University in St. Louis, United StatesRaees Tonse, Baptist Hospital of Miami, United States
Copyright © 2024 Lai, Yin, Feng, Deng, Lv, Zhong and Peng. This is an open-access article distributed under the terms of the Creative Commons Attribution License (CC BY). The use, distribution or reproduction in other forums is permitted, provided the original author(s) and the copyright owner(s) are credited and that the original publication in this journal is cited, in accordance with accepted academic practice. No use, distribution or reproduction is permitted which does not comply with these terms.
*Correspondence: Yumei Zhong, emhvbmd5dW1laTEyMDVAMTYzLmNvbQ==; Dezhong Peng, MTU4MjgwNTU2OThAMTYzLmNvbQ==
†These authors have contributed equally to this work and share first authorship