- 1Department of Physiology, Federal University of Health Sciences, Ila Orangun, Nigeria
- 2Reproductive Biology and Toxicology Research Laboratory, Oasis of Grace Hospital, Osogbo, Nigeria
- 3Department of Physiology, Ladoke Akintola University of Technology, Ogbomoso, Nigeria
- 4Department of Physiology, University of Uyo, Uyo, Nigeria
- 5Department of Medical Laboratory Science, Afe Babalola University, Ado-Ekiti, Nigeria
- 6The Brainwill Laboratories and Biomedical Services, Osogbo, Nigeria
- 7Department of Physiology, University of Ilorin, Ilorin, Nigeria
Studies have implicated oxidative stress-sensitive signaling in the pathogenesis of stress-induced male infertility. However, apart from oxidative stress, gonadotropin inhibitory hormone (GnIH) plays a major role. The present study provides a detailed review of the role of GnIH in stress-induced male infertility. Available evidence-based data revealed that GnIH enhances the release of corticosteroids by activating the hypothalamic-pituitary-adrenal axis. GnIH also mediates the inhibition of the conversion of thyroxine (T4) to triiodothyronine (T3) by suppressing the hypothalamic-pituitary-thyroidal axis. In addition, GnIH inhibits gonadotropin-releasing hormone (GnRH), thus suppressing the hypothalamic-pituitary-testicular axis, and by extension testosterone biosynthesis. More so, GnIH inhibits kisspeptin release. These events distort testicular histoarchitecture, impair testicular and adrenal steroidogenesis, lower spermatogenesis, and deteriorate sperm quality and function. In conclusion, GnIH, via multiple mechanisms, plays a key role in stress-induced male infertility. Suppression of GnIH under stressful conditions may thus be a beneficial prophylactic and/or therapeutic strategy.
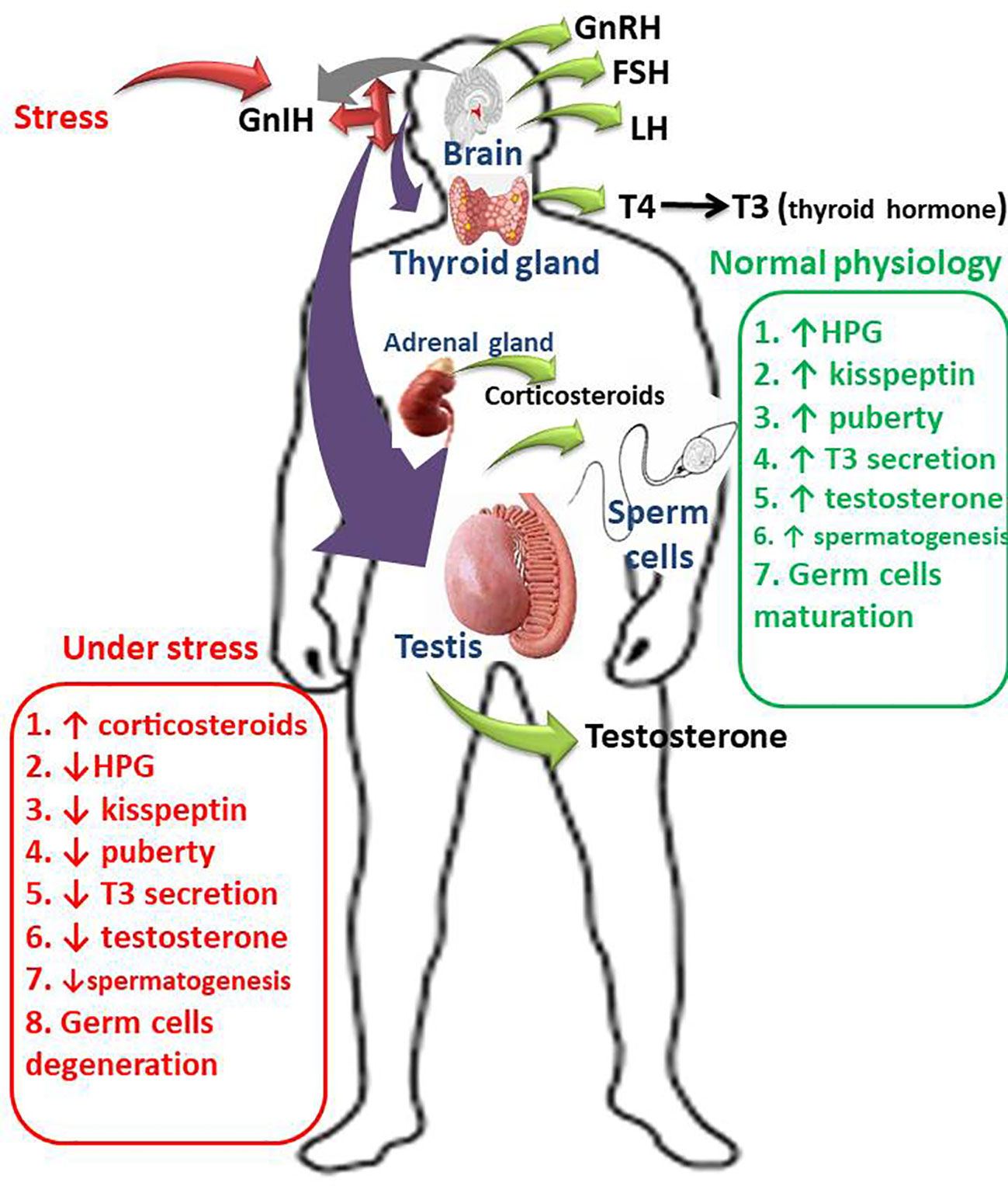
Graphical Abstract Schematic illustration of the impacts of GnIH on male fertility, Gonadotropin inhibitory hormone (GnIH) promotes the release of corticosteroids by activating the hypothalamic-pituitary-adrenal axis. GnIH also inhibits the conversion of thyroxine (T4) to triiodothyronine (T3) by suppressing the hypothalamic-pituitary-thyroidal (HPT) axis. In addition, GnIH inhibits gonadotropin-releasing hormone (GnRH), thus suppressing the hypothalamic-pituitary-gonadal (HPG) axis, therefore suppresses circulating luteinizing hormone (LH), follicle-stimulating hormone (FSH), and testosterone. More so, GnIH inhibits kisspeptin release, thus induces a delay in puberty onset. These series of events is accompanied by GnIH-induced degeneration of germ cells, impairment of spermatogenesis, and deterioration of sperm quality and function.
1 Introduction
Infertility is a global public health issue that impacts an individual’s social, economic, and personal life (1). According to WHO (2), infertility is a reproductive system disease defined by the inability of clinical pregnancy to be achieved after twelve months of regular unprotected sex. Although infertility does not threaten life, it is depicted as a radical life-changing problem because it bears notable psychological trauma and social stigma (3). Infertility is a very distressful state; the stress associated with it causes a drop in sexual self-esteem, a decrease in the frequency of sexual intercourse, and an increase in marital conflict. Infertile individuals often report feeling less of themselves and inadequate (3). Statistics suggest that an estimated 48.5 million couples worldwide are not fertile, accounting for about 15% of all reproductive couples globally (4). About half of all cases of infertility are contributed by male conditions (5). It is thought that about one-third of cases of being unable to give birth are due to male factors, one-third occur as a result of the female, and the remaining third is due to the combination of male and female factors. In approximately 30% of cases, the cause is labeled as idiopathic, and the condition’s origin is never identified (6). Idiopathic infertility may be explained by the role of mental disorders, such as stress, depression, sleep disorders, eating disorders, and addictions (7).
Stress is any change that disrupts homeostasis by inflicting physical, emotional, or psychological strain (8). Under a stressful condition, the organism modifies its behavior and physiological responses to re-establish homeostasis. Psychological stress caused by a mix of achieving personal targets, hassles, meeting demands, and deadlines, and frustrations is a major type of stress affecting individuals globally (9). In the short-term, stress can motivate and sometimes enhance productivity. The physiologic response to acute stress can also assist in maintaining good health, mood, human relation, and quality of life by stimulating the ‘fight and flight’ to maintain homeostasis (10). The response to stressors is important for a sense of well-being, productivity, and socialization. However, acute stress can become sustained in the presence of some negative socioeconomic factors such as financial problems, disease outbreaks (e.g. COVID-19 and Ebola outbreaks), job insecurity, loneliness, or bereavement. Negative socioeconomic factors could frustrate individuals and eventually activate the mechanisms responsible for chronic stress response (9).
Chronic stress may distort the normal metabolic, nervous, and immune responses (11), thus increasing the susceptibility to pathological conditions (12). Although stress has been clinically associated with male infertility, little is known about its possible effect on spermatogenesis and steroidogenesis compared with it inflammatory and oxidative stress mechanism. This narrative review provides compelling shreds of evidence, based on the available data from the literature, on the role of chronic stress on male fertility. It also provides information on the role of gonadotropin inhibiting hormone (GnIH) on male infertility.
2 GnIH
GnIH is a decapeptide hormone that plays a key role in the neural regulation of reproduction (13). It is one of the major hypothalamic neuropeptide hormones responsible for maintaining optimal reproductive functions (14). The novel hormone was discovered in the quail hypothalamo-hypophyseal system in the year 2000 and was named based on its inhibitory effect on the gonadotropic hormones and gonadotropic releasing hormone (GnRH) (15). This opened another research window in reproductive neuroendocrinology challenging the belief that GnRH is the only hypothalamic hormone responsible for regulating reproductive functions. Subsequently, the hormone was found to be present in most vertebrates including humans (16), and it has been established to exert influential activities on sexual behavior and gonadal functions (16).
GnIH is also referred to as RF amide-related peptides (RFRPs) in mammals, while it is known as LPXRF-amide in teleosts because it has LPXRF (X = L or Q) amide motif at the C-termini (14, 17). The two forms of GnIH found in mammals are RFRP-1 and RFRP-3, and have been identified to regulate the hypothalamic-pituitary gonadal (HPG) axis in men (13, 14, 18). GnIH directly inhibits GnRH since its axon is in contact with GnRH neurons (19, 20). Apart from its effect on the hypothalamus, it also inhibits the secretion of follicle stimulating hormone (FSH) and luteinizing hormone (LH) directly through its release into the hypothalamic–hypophyseal portal system (21). Furthermore, the presence of GnIH receptors on the testes of mammals is an indication that it may directly inhibit testicular functions (spermatogenesis and steroidogenesis). In fact, exogenous administration of GnIH has been shown to impair testicular development (22). Hence, GnIH can impair testicular functions at the level of the hypothalamus, pituitary, or testes (Figure 1).
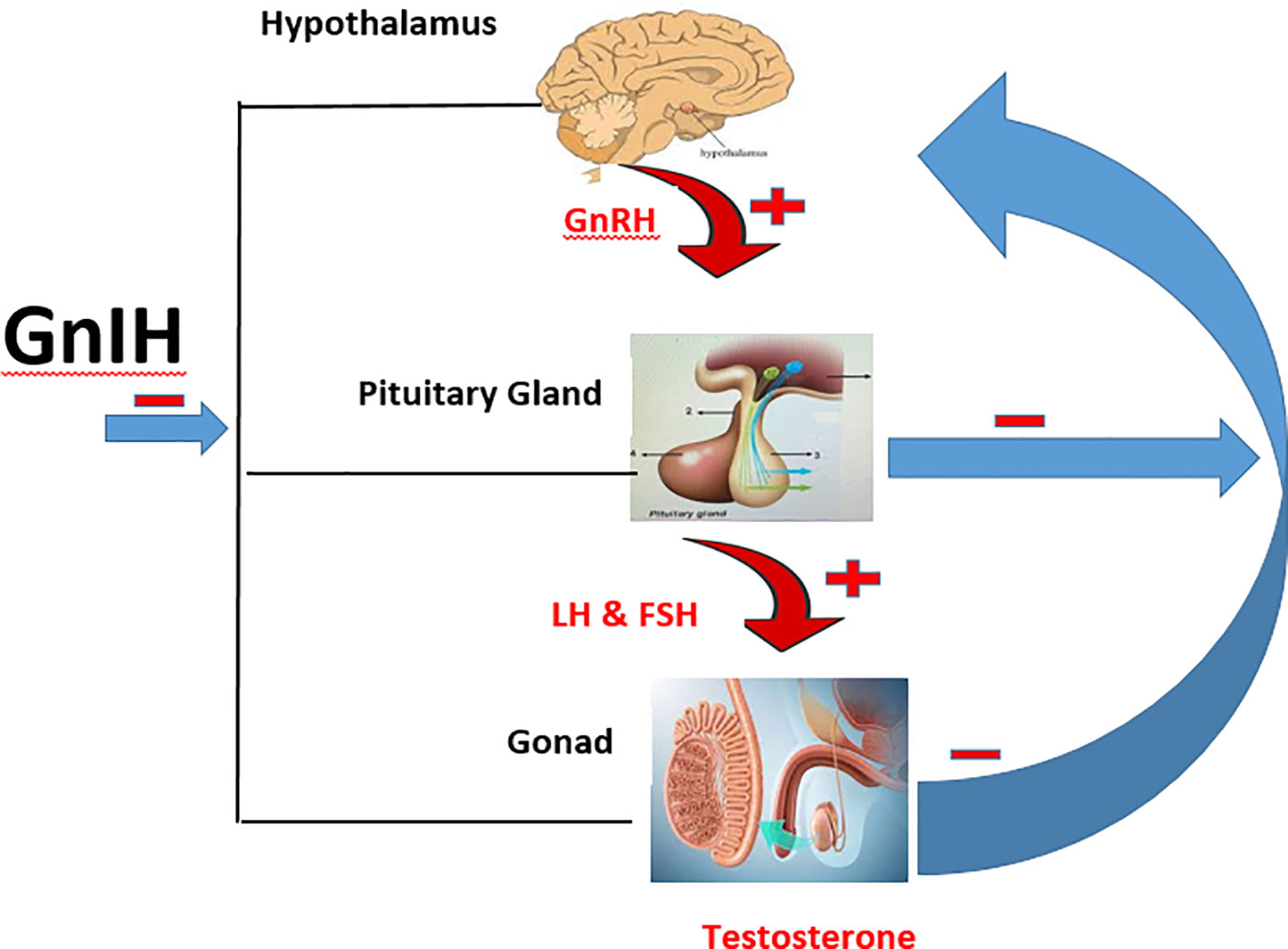
Figure 1 Schematic illustration of the HPG axis and the inhibitory effect of GnIH. ─ is inhibitory effect while ┼ is stimulatory effect.
Neurons responsible for the synthesis of GnIH are present in the mid-ventral continuum from the diagonal band of Broca to the mediobasal hypothalamus (23), while those regulating the secretion of gonadotrophic hormones extend to the median eminence to modulate the synthesis of FSH and LH (13, 24). The released LH and FSH then regulate the synthesis of gonadal hormones which in turn send negative feedback to the hypothalamus and anterior pituitary gland to keep the reproductive axis within the operating limits required for optimal reproductive functions (25). This closed loop is called the hypothalamic-pituitary-gonadal (HPG) axis, and it is known to be solely regulated in the hypothalamus via GnRH (26) until the discovery of GnIH.
2.1 Physiology of stress
Stress is an important phenomenon for survival that requires prompt physiological and behavioral responses for an individual to cope with the different situations in the environment and maintain body homeostasis (27). Physiologically, stress response can be categorized into the fast response mediated by the sympathoneural and sympathoadrenomedullary (SAM) axis and the slow response mediated by the hypothalamic-pituitary-adrenal axis (HPA) (28).
The sympathoneural and SAM are the first line of stress response, and their activation stimulates the release of catecholamine (epinephrine and norepinephrine) from the adrenal gland into the bloodstream. Also, norepinephrine becomes elevated in the brain due to its increased secretion and release from the sympathetic nerves (29). The released epinephrine and norepinephrine stimulate the alpha (α)- and beta (β)- adrenergic receptors found in the central nervous system, smooth muscles, and other organs of the body (30). The released epinephrine and norepinephrine bind to their specific membrane-bound G-protein receptors to activate the intracellular cyclic adenosine monophosphate (cAMP) signaling, which in turn stimulates various cellular responses (31). The activation of these receptors leads to an increase in heart rate, blood pressure, cardiac output, and skeletal muscle blood flow via vasoconstriction of both the smooth and cardiac muscles (32). They also stimulate an increase in blood glucose, lipolysis, oxygen utilization, and thermogenesis, and cause behavioral changes such as enhanced arousal, alertness, and attention (30).
In addition, the activation of the first line of the stress response stimulates the slow response, which is mediated by the activation of the HPA axis to release glucocorticoids (28). Corticotropin-releasing hormone (CRH) is secreted from the paraventricular nucleus (PVN) of the hypothalamus and binds to its receptors (CRH-R1 and CRH-R2). The CRH-R1 is the major receptor for activating the stress-induced secretion and release of adrenocorticotropic hormone (ACTH) (30). The release of CRH into the bloodstream stimulates the release of ACTH from the anterior pituitary gland into the bloodstream, which in turn stimulates the release of glucocorticoid from the adrenal cortex. HPA axis is regulated by the pituitary adenylate cyclase-activating polypeptide (PACAP) that regulates the release of CRH and modulates the HPA axis at different levels (33). PACAP also stimulates the secretion of catecholamines during autonomic response to stress (33).
The interplay between sympathoneural, SAM, and HPA axis in response to stress systematically produces metabolic and behavioral changes that are transient and adaptive (28). However, chronic sympathetic discharge and elevated levels of glucocorticoid during prolonged stress are associated with pathological conditions, such as metabolic disorder and infertility.
2.1.1 Stress and infertility
Chronic stress is a psychological disorder that can lead to various sexual problems such as loss of libido and erectile and ejaculatory dysfunction (34). Besides, infertility itself is stressful because of its attendant complications such as social pressures, low self-esteem, unfulfilled desire, and a financial burden (35). Stress may impair testicular functions, which leads to reduced circulating testosterone, and impaired spermatogenesis and sperm quality (36). The first available report on the effect of stress on human spermatogenesis was obtained from death-sentenced prisoners kept for a long time before execution (37). The study reported impaired spermatogenesis that was so marked that the only cells found in the seminiferous tubules were the Sertoli and spermatogonial cells. In another study, milder stress was reported to significantly reduce circulating testosterone (37).
Various experimental results show a positive correlation between chronic stress and erectile dysfunction. Chronic stress impairs the normal morphology of the penile corpus cavernosum, which in turn impairs penile erection (34, 38). Additionally, chronic stress also `impairs endothelial function (39), which is important for penile erection via the No/cGMP signaling (40).
2.1.2 Stress and GnIH
Chronic stress is positively related to the secretion of GnIH from the hypothalamus (41). In other to confirm the relationship between chronic stress and GnIH, adrenalectomy was performed in male rats, and it was observed that the increase in GnIH secretion under a stressful condition was abolished (42). Also, the study of Son et al. (43) revealed that glucocorticoid receptor (GR) is present in GnIH neurons that are located in the PVN, and treatment with glucocorticoids significantly increases GnIH secretion. GR is also expressed in rHypoE-23, which is a GnIH-expressing neuronal cell line from a rat hypothalamus (43). Stress-induced secretion and release of norepinephrine is responsible for stimulating the release of GnIH (44). Interestingly, the expression alpha-2A adrenergic receptors in GnIH neurons of male quail have been elucidated (44). Thus, it appears that the effect of chronic stress on fertility vis the HPA axis is mediated by the upregulation of the expression of GnIH.
Also, GnIH neurons and those of CRH are in direct contact with the PVN, and their release triggers the activation of the HPA axis (45). In addition, the CRH receptor is present in about 13% of the neurons of GnIH, and its activation upregulates GnIH-R mRNA (42). GnIH and cortisol are up-regulated in the presence of acute and chronic stress mediators (45). In agreement with the earlier findings of Kirby et al. (42), Higuchi et al. (46) demonstrated that GnIH and cortisol levels were markedly elevated during stress. However, like most neuroendocrine responses, sustained stimulation of the CRH receptor by chronic stress may lead to its desensitization, disrupting the HPA axis (47). Thus, it has been speculated that CRH-sensitive GnIH cells might also become desensitized by sustained chronic stress (48), thereby interrupting the GnIH-GnRH neuronal pathway. These pieces of experimental evidence show that CRH and GnIH are positively correlated since CRH can directly stimulate some parts of GnIH neurons, thereby increasing GnIH sensitivity via the upregulation of the GnIH-R.
Furthermore, chronic stress has been revealed to be associated with hypothyroidism by directly inhibiting the activities of the hypothalamic-pituitary-thyroidal (HPT) axis (49). Stress-induced secretion of glucocorticoids has been linked with hypothyroidism by inhibiting the conversion of thyroxine (T4) to triiodothyronine (T3). This is in agreement with the study of Kakucska et al. (50), which showed that the administration of dexamethasone and corticosterone led to a significant decrease in the expression of TRH mRNA within the hypothalamus (51). These observed stress-induced hypothyroidism might be accountable for the surge in GnIH following chronic stress. According to the study of Kiyohara et al. (52), transient hypothyroidism led to GnIH mRNA upregulation and delayed puberty onset in young female rats, these observed hypothyroidism-induced reproductive dysfunction was completely reversed in animals with genetic loss of GnIH. Similarly, Rodrigues et al. (53) reported that the regulatory effect of thyroid hormone on the HPG is via its effect on GnIH secretion. They reported that hypothyroidism is a potent stimulator for the release of GnIH which in turn decreased Kiss1 mRNA expression, and eventually inhibited the release of gonadotropins. This is in tandem with the study of Santos et al. (54) that also reported negative relationship between thyroid hormone and Kiss 1. Additionally, the presence of thyroid hormone receptors and response elements (52) further shows the direct relationship between thyroid hormones and GnIH. In fact, thyroid hormone regulates chromatin modifications of GnIH promoter to either stimulate or inhibit GnIH expression by H3acetylation and H3K9tri-methylation respectively (55). Hence, it is tempting to conclude that another possible mechanism underlying chronic stress-induced elevated GnIH could be due to its inhibitory effect on thyroidal function since hypothyroidism has been linked with elevated GnIH secretion and release.
Another possible mechanism of action responsible for stress-induced increase in GnIH secretion is via a leptin-dependent pathway. Chronic stress has been shown to increase leptin secretion (56), a peptide hormone that is produced and synthesized by the white adipose tissue (57). Leptin is a satiety hormone and has been established to play a role in reproduction by maintaining metabolism in the reproductive axis (58). Different animal studies have described the role of leptin in maintaining the HPG axis. The presence of about 15-20% of GnIH neurons on the long form of the leptin receptor (LepRb), suggests a possible relationship between GnIH and adiposity via leptin and feed intake (45). In fact, a significant decline in GnIH synthesis has been reported in the leptin-deficient ob/ob mice (59). This relationship could be traced to the pro-inflammatory activities of leptin. Increased circulatory leptin is positively related to the production of inflammatory cytokines and resistin (60). Leptin-induced inflammatory response could stimulate the secretion on GnIH, which in turn suppresses the HPG axis activities. This claim is supported by the study of Iwasa et al. (61) that reported the stimulatory effect of lipopolysaccharide on GnIH secretion. Hence, it is plausible that the observed increase in GnIH during chronic stress could be mediated via stress-induced increase in leptin secretion.
2.2 GnIH and HPG axis
The HPG axis is a crucial endocrine pathway that links the hypothalamus, pituitary, and gonads in the body (62). HPG is integral in the establishment and maintenance of normal physiological processes related to reproduction, such as sexual maturation, steroidogenesis, and spermatogenesis. It is responsible for the production of essential reproductive hormones, such as those involved in fertility and sexual maturation. Anatomically, The HPG axis is made up of the hypothalamus, that housed the KNDy and GnRH-producing neurons, the anterior pituitary, where the gonadotropes produced LH and FSH, and the gonads, responsible for sex steroids and gametes production.
The HPG axis is active in the human fetus till one year after birth, after which it goes quiescent till 10 years postnatal life when it becomes active again (63, 64). This is about the time for the onset of puberty. It is occasioned by an increase in GnRH secretion in a pulsatile way, leading to increased gonadotropin secretion as well (63, 64). Reduction in melatonin secretion due to the regression of the pineal gland, together with the increase in leptin and other hormones, contribute favorably to the reactivation of the HPG axis before puberty onset (65). Since melatonin is positively correlated with GnIH (66, 67), it can be speculated that a decline in the secretion of GnIH following melatonin reduction, has a role to play in the reactivation of the HPG axis during the pubertal stage.
The HPG axis is key in the regulation of reproductive functions in vertebrates (64, 65). While the two main hormones at the anterior pituitary level of the HPG axis, luteinizing hormone (LH) and follicle-stimulating hormone (FSH), stimulate gonadal functions (steroidogenesis and spermatogenesis) (68);, the release of these hormones is mainly regulated by neurons at the hypothalamic level that produces GnRH (15, 69). Pulsatile GnRH secretion can be triggered by environmental and tactile cues like food availability, photoperiod, rainfall, and the presence of a mate (70).
GnRH is a decapeptide hormone discovered to stimulate the release of LH and FSH from the pituitary gland of mammals (71). Early findings referred to GnRH as the Luteinizing Hormone-Releasing Hormone (LH-RH), until it was widely referred to as GnRH because of it stimulatory effect on not just LH, but also on FSH. While GnRH stimulates LH and FSH, its stimulatory effects on both secretions are not similar (72). Compared to LH, FSH secretion is more irregular in humans, which could be due to the pulsatility and different stimulatory effects of GnRH (73). This could also be a result of the existence of different gonadotropes subpopulations or different response times to GnRH (74). To support this claim, findings from an ovariectomized sheep administered GnRH antisera, revealed a complete inhibition of LH secretion (LH became undetectable within 24 hours), while FSH release fell slowly and remain detectable (75). Furthermore, the rate of GnRH input has been shown to selectively maintain the transcription of gonadotropin subunit gene. For example, rapid GnRH pulse rates upregulate α and LH-, while the slow pulse frequency increases FSH-β gene transcription (74, 76).
The gonadotroph cells located in the anterior pituitary are responsible for the production of LH and FSH. These cells are made up of large round cell bodies with pronounced Golgi apparatus and endoplasmic reticulum. These cells constitute about 10 to 15% of the functional anterior pituitary cell mass. The LH and FSH produced from these cells are from similar genes which accounted for their similar properties. LH and FSH are glycoproteins consisting of alpha and beta subunit. The alpha subunit is similar while the beta subunit of each hormone is different. The difference in the beta subunit gave each hormone its biological specificity. The alpha subunit of LH and FSH consist of 92 amino acids, while the LH beta sub unit is made up of 120 amino acids and FSH is made up of 118 amino acid (77). Additionally, LH consist of one-two sialic acid residues, which account for its shorter half-life, while FSH is madeup of 5 sialic acid residues, accounting for its longer half-life of 3-4 minutes. LH and FSH are responsible for maintaining gonadal functions. LH is majorly responsible for stimulating the Leydig cells to produce testosterone from the testis, while FSH is responsible for maintaining the Sertoli cells to maintain spermatogenesis. They both also maintain GnRH production via negative feedback mechanism.
Testosterone and its metabolite (dihydrotestosterone) are the androgen in the testis, and are the major male reproductive hormones in mature male mammals. Testosterone is required for maintaining spermatogenesis, and the production of mature sperm is intimately dependent on androgen action within the testis. In fact, the maintenance of optimal sexual and erectile function depends on the effective testosterone secretion (78, 79). Testosterone is also responsible for different biological processes and is important for the development and maintenance of male secondary characteristics. Testosterone is also responsible for maintaining the HPG axis via its negative feedback mechanism to the pituitary gland and hypothalamus.
GnIH is another hormone responsible for maintain the activities of the HPG axis. It is the first hypothalamic neuropeptide found to have an anti-gonadotrophic effect on all vertebrate species by directly inhibiting GnRH via the 2 G-ptotein coupled receptors - GPR147 and GPR74 which have been recognized as GnIH receptors (GnIH-R) of which GPR147 cDNA are found in the brain and pituitary while GPR74 cDNA are conveyed in some tissues. It is important to note that GPR147 is considered the principal GnIH receptors because of its higher binding affinity as compared to GPR74 (55). GnIH also acts by downregulating mRNA levels of luteinizing hormone beta-subunit (LHβ) and inhibiting its release from the anterior pituitary gland (16, 80). GnIH can also inhibit LH synthesis via its stimulatory effect on prolactin secretion (81), which is a potent inhibitor of LH secretion (82). Its action on follicle-stimulating hormone beta-subunit (FSHβ) is not clear since its studies in quail have shown no effect on mRNA levels or FSH release. Whereas, in cockerels and other avian species, LH and FSH were suppressed (83–85). In mammals, it is less evident and controversial, particularly in its correlation with puberty (83).
2.3 GnIH and kisspeptin
Apart from the direct inhibitory effect of GnIH on the HPG axis, it also inhibits the secretion of kisspeptin, which stimulates the release of GnRH from the hypothalamus. Kisspeptin is made up of 52-54 amino acid which cleaves from its precursors and amidation occurs in the C-terminals. While C-terminals 10 amino acids (Kp-10) are similar in mice, rats, cattle, sheep and pigs, in humans tyrosine in the C-terminals is substituted with phenylalanine (86).
Kisspeptin together with its receptor (GPR54/Kiss-1r) are responsible for controlling reproduction and puberty in mammals through their direct stimulatory effect on the GnRH neurons (87, 88). In fact, the gain and loss of function in KISS1/KISS1R genes mutations led to precocious puberty and hypogonadotropic hypogonadism respectively, in human and animal models (89). This is associated with the precocious stimulation or impairment of the HPG axis at the level of the hypothalamus. The presence of Kiss1R on GnRH-secreting neurons membrane further substantiate the direct relationship between kisspeptin and the hypothalamus (90). Aside the direct effect of kisspeptin on the HPG-axis at the hypothalamic level, kisspeptin also modulate the HPG-axis activities at the pituitary and gonadal level. This is supported by the fact that kisspeptin neurons are also intermediate in the sex-steroid mediated feedback mechanisms on reproduction (90). In fact, environmental cues such as environmental toxicants, stress, and diet interferes with HPG-axis activities via Kiss-secreting neurons consisting of kisspeptin/neurokinin B/dynorphin A (KNDy neurons) (89). KNDy neurons are proposed to form the long elusive GnRH/LH pulse generator (91). These neurons are responsible for modulating gonadotropin release and reproductive functions based on peripheral signals (92). In tandem with this claim, KNDy neurons have been shown to maintain reproductive and non-reproductive functions such as negative feedback control of gonadotropin release (93), metabolism (94), stress-induced cues on fertility (95);, and thermoregulation (96).
Although kisspeptin maintains reproductive functions via its modulatory effect on the HPG-axis, it’s effect on the peripheral organs cannot be overlooked. Kisspeptin system has been identified in the testis (97), suggesting its possible autocrine and paracrine intra-testicular communications activities, testosterone synthesis, and sperm production and quality. In fact, kisspeptin, but not GnRH has been identified in the plasma, and the amount was dependent on fertility status (98). Intriguingly, gonadotropin stimulation is not always sufficient in ameliorating the impaired steroidogenesis and spermatogenesis in clinical cases of KISS1R inactivating mutations (89, 99). Additionally, the specific reactivation of the Kiss1R gene in the GnRH secreting neuron of KISS1R−/− knockout mice does not successfully ameliorate the associated impaired spermatogenesis (100). These above pieces of information support our claim that testicular Kiss1R signaling is also important for maintaining steroidogenesis and spermatogenesis.
Based on the above importance of kisspeptin, it is plausible to infer that GnIH inhibit gonadotropin secretion via its inhibitory effect on kisspeptin. Coincidentally, the presence of GnIH-Rs in approximately 9-16% of RP3V kisspeptin neurons in rats (101), and 5- 10% of the anteroventral periventricular nucleus (AVPV) and 25% of ARC Kiss1 neurons in mice (102), further substantiate the direct relationship between GnIH and kisspeptin. Also, GnIH fibers and Kiss 1 neurons are closely located, suggesting that GnIH might be inhibiting reproduction via its direct inhibitory effect on kisspeptin neurons (52). Furthermore, GnIH-R and GPR54 knockout mice displayed a disrupted LH secretion; however, the disruption was prominent in GPR54 knockout mice. In addition, Kiss1 mRNA was observed to be unregulated in GnIH-R knockout mice, while about 33% increase in GnRH neurons was also observed (45, 103, 104). These pieces of information suggest multiple pathways for GnIH inhibitory effect on the HPG axis. Hence, GnIH can inhibit the HPG axis by inhibiting GnRH and/or kisspeptin neuron expression.
2.3.1 GnIH and steroidogenesis
GnIH and its receptor have been reported to be expressed in the hypothalamus and gonads (105). This may infer that GnIH does not only inhibit the HPG axis at the level of the hypothalamus and pituitary gland, but also the level of the gonads (45). Aside from the presence of GnIH receptor in the gonads, GnIH mRNA transcripts have also been found to be synthesized in the testis and localized interstitium (106, 107). This reveals that GnIH may inhibit testosterone production by suppressing the HPG axis or eliciting a direct inhibitory effect on the testis. GnIH treatment has been found to significantly disrupt testicular functions by directly impairing testosterone production from the testis (107).
Testosterone production is a de novo synthesis that involves the transportation of cholesterol from the outer mitochondrial membrane to the inner part, which is a rate-limiting step in the biosynthesis of testosterone. This intra-mitochondrial transport is regulated by the steroidogenic acute regulatory (StAR) protein. In humans, the administration of GnIH down-regulated steroidogenic acute regulatory (StAR) activities, while the administration of GnIH antagonists up-regulated StAR activities (105). Outside steroidogenic enzymes, GnIH has also been shown to impair glucose homeostasis which stimulates the uptake of cholesterol, which is the precursor of steroid hormones (108, 109). Decline cholesterol and StAR activities may mediate GnIH-induced suppression of testosterone synthesis.
2.3.2 GnIH and spermatogenesis
Spermatogenesis is the process of producing sperm cells from spermatogonial cells. This process starts during puberty and continues throughout a man’s life. Spermatogenesis is regulated by a complex interplay of hormones and signaling molecules, including GnIH. Recent findings have revealed that GnIH plays a negative role in spermatogenesis by disrupting the HPG axis (110), which is responsible for the control of gonadal function, including the regulation of spermatogenesis, sperm quality, and sperm function. RFRP-3 is considered to be similar to GnIH in terms of its effect on gonadotropin secretion in mammals and has been observed to exert both autocrine and paracrine action on the gonads where it directly inhibits testicular functions. Bentley et al. (106) reported that GnIH is synthesized in the seminiferous tubules and interstitial cells in birds, while McGuire et al. (111) reported that it inhibited testosterone synthesis in avian testicular cell culture. Bentley et al. (106) also identified the expression of GPR 174 - RFRP-3 receptors - in the epididymis, and vas deferens of birds. Rats (112), sheep (113), mice (105), Syrian hamsters (114), pigs (115), primates and humans (107, 111) can produce RFRP-3 in their gonads. Zhao et al. (114) and Ubuka and Tsutsui (116) also identified RFRP-3 and its receptors in spermatocytes and spermatids through immunohistochemistry and in situ hybridization and recorded an increase in the expression of RFRP-3 and GPR147 in late spermatocytes, signifying RFRP-3’s role in the maturation of sperm. Anjum et al. (117) studied the expression of GnIH in the testis of mice and correlated it with serum testosterone levels from birth to senescence and found that RFRP-3 may cause pubertal activation of senescence in mice testis.
In 118 and his team studied the impact of the GnIH homolog RFRP-3 on the production of sperm and steroids in mice and discovered that treatment with RFRP-3 caused a significant decrease in the levels of circulating steroids, and testicular activity in the mice. It also caused dose-dependent changes in spermatogenesis, such as a decrease in cell proliferation and survival markers, and an increase in markers of cell death in the testes. Both in vivo and in vitro studies showed that RFRP-3 had an inhibitory effect on testosterone production in the testes. RFRP-3 also suppressed the expression of the LHCGR receptor, StAR protein, and enzymes involved in steroid synthesis (CYP11A1 and 3β-hydroxysteroid dehydrogenase) in the testes, leading to dose-dependent suppression of testosterone secretion that is an important factor in spermatogenesis. Testosterone is required for processes that are critical for spermatogenesis including maintaining the BTB, supporting the completion of meiosis, the adhesion of elongated spermatids to Sertoli cells, and the release of sperm (119).
Sperm quality refers to the number, motility, viability, and morphology of sperm cells (78, 120, 121). Factors such as age, lifestyle, ejaculatory abstinence length, and genetic background can affect sperm quality (120). Recent findings have revealed that GnIH may alter sperm quality (122). Marques and Boguszewski (122) demonstrated that GnIH exposure reduces sperm count, motility, and morphology in an animal model. GnIH has also been shown to induce oxidative stress and inflammation, which may contribute to a decline in sperm quality (123).
In addition, GnIH has been reported to affect sperm function (112). GnIH and its regulation of the HPG axis have been associated with several pathologies and disorders related to reduce sperm function (124). GnIH has been found to impair capacitation and acrosome reactions (124). Also, studies have shown that GnIH reduces the fertilizing ability of sperm cells in animals (114, 116).
3 Conclusion and future perspective
In conclusion, stress causes GnIH-induced degeneration of testicular cells and impaired testicular and adrenal steroidogenesis, spermatogenesis, and sperm quality through the activation of the hypothalamic-pituitary-adrenal axis, inhibition of the hypothalamic-pituitary-thyroidal axis, leptin hypersecretion, and suppression of the hypothalamic-pituitary-testicular axis and kisspeptin release. More studies exploring the roles and associated mechanisms of GnIH in male infertility are recommended. Also, suppression of GnIH may likely be a beneficial preventive and therapeutic strategy to avert the negative effects of GnIH, especially during exposure to stress.
Author contributions
AO: Conceptualization, Data curation, Formal analysis, Funding acquisition, Investigation, Methodology, Project administration, Resources, Software, Supervision, Validation, Visualization, Writing – original draft, Writing – review & editing. RA: Data curation, Formal analysis, Investigation, Methodology, Project administration, Resources, Software, Supervision, Validation, Visualization, Writing – review & editing. GB: Conceptualization, Investigation, Methodology, Project administration, Resources, Writing – review & editing. MH: Investigation, Methodology, Project administration, Resources, Writing – review & editing. LO: Data curation, Formal analysis, Funding acquisition, Investigation, Methodology, Project administration, Resources, Software, Supervision, Validation, Visualization, Writing – review & editing.
Funding
The author(s) declare that no financial support was received for the research, authorship, and/or publication of this article.
Conflict of interest
The authors declare that the research was conducted in the absence of any commercial or financial relationships that could be construed as a potential conflict of interest.
Publisher’s note
All claims expressed in this article are solely those of the authors and do not necessarily represent those of their affiliated organizations, or those of the publisher, the editors and the reviewers. Any product that may be evaluated in this article, or claim that may be made by its manufacturer, is not guaranteed or endorsed by the publisher.
Abbreviations
CRH, corticotropin releasing hormone; FSH, follicle-stimulating hormone; GnIH, gonadotropin inhibitory hormone; GnRH, gonadotropin-releasing hormone; HPA, Hypothalamic-pituitary-adrenal axis; HPG, Hypothalamic-pituitary-gonal axis; LH, Luteinizing hormone; RFRP, RF amide-related peptides; T3, triiodothyronine; T4, thyroxine.
References
1. Abebe MS, Afework M, Abaynew Y. Primary and secondary infertility in Africa: systematic review with meta-analysis. Fertility Res Pract (2020) 6(1):20. doi: 10.1186/s40738-020-00090-3
2. World Health Organization (WHO). Sexual and Reproductive health (2020). Available at: https://www.who.int/reproductivehealth/topics/infertility/multiple-definitions/en/.
3. Aiyenigba AO, Weeks AD, Rahman A. Managing psychological trauma of infertility. Afr J Reprod Health (2019) 23(2):76–91.
4. Sengupta P, Borges E, Dutta S, Krajewska-Kulak E. Decline in sperm count in European men during the past 50 years. Hum Exp Toxicol (2018) 37(3):247–55. doi: 10.1177/0960327117703690
5. Kumar N, Singh AK. Trends of male factor infertility, an important cause of infertility: A review of literature. J Hum Reprod Sci (2015) 8(4):191–6. doi: 10.4103/0974-1208.170370
6. Bracke A, Peeters K, Punjabi U, Hoogewijs D, Dewilde S. A search for molecular mechanisms underlying male idiopathic infertility. Reprod Biomed Online (2018) 36(3):327–39. doi: 10.1016/j.rbmo.2017.12.005
7. Szkodziak F, Krzyżanowski J, Szkodziak P. Psychological aspects of infertility. A systematic review. J Int Med Res (2020) 48(6):300060520932403.
8. Chrousos GP. Stress and disorders of the stress system. Nat Rev Endocrinol (2009) 5:374–81. doi: 10.1038/nrendo.2009.106
9. Nargund VH. Effects of psychological stress on male fertility. Nat Rev Urol (2015) 12(7):373–82. doi: 10.1038/nrurol.2015.112
10. McEwen BS. Physiology and neurobiology of stress and adaptation: central role of the brain. Physiol Rev (2007) 87:873–904. doi: 10.1152/physrev.00041.2006
11. Wingfield JC, Sapolaski RM. Reproduction and resistance to stress when and how. J Neuroendocrinol (2003) 15:711–24. doi: 10.1046/j.1365-2826.2003.01033.x
12. Baum A, Posluszny DM. Health psychology: mapping biobehavioral contributions to health and illness. Ann Rev Psychol (1999) 50:137–63. doi: 10.1146/annurev.psych.50.1.137
13. Kriegsfeld LJ, Mei DF, Bentley GE, Ubuka T, Mason AO, Inoue K, et al. Identification and characterization of a gonadotropin-inhibitory system in the brains of mammals. Proc Natl Acad Sci (2006) 103(7):2410–5. doi: 10.1073/pnas.0511003103
14. Tobari Y, Aleksandrova Y, Fukahori Y, Tsutsui K, Meddle SL. Gonadotropin-inhibitory hormone as a regulator of social interactions in vertebrates. Front Neuroendocrinol (2022) 64:100954. doi: 10.1016/j.yfrne.2021.100954
15. Tsutsui K, Saigoh E, Ukena K, Teranishi H, Fujisawa Y, Kikuchi M, et al. A novel avian hypothalamic peptide inhibiting gonadotropin release. Biochem Biophys Res Commun (2000) 275(2):661–7. doi: 10.1006/bbrc.2000.3350
16. Tsutsui K, Ubuka T. Discovery of gonadotropin-inhibitory hormone (GnIH), progress in GnIH research on reproductive physiology and behavior and perspective of GnIH research on neuroendocrine regulation of reproduction. Mol Cell Endocrinol (2020) 514:110914. doi: 10.1016/j.mce.2020.110914
17. Ukena K, Iwakoshi-Ukena E, Osugi T, Tsutsui K. Identification and localization of gonadotropin-inhibitory hormone (GnIH) orthologs in the hypothalamus of the red-eared slider turtle, Trachemys scripta elegans. Gen Comp Endocrinol (2016) 227:69–76. doi: 10.1016/j.ygcen.2015.06.009
18. Xiong X, Hu Y, Pan B, Zhu Y, Fei X, Yang Q, et al. RFRP-3 influences apoptosis and steroidogenesis of yak cumulus cells and compromises oocyte meiotic maturation and subsequent developmental competence. Int J Mol Sci (2023) 24(8):7000. doi: 10.3390/ijms24087000
19. Ubuka T, Son YL, Tobari Y, Narihiro M, Bentley GE, Kriegsfeld LJ, et al. Central and direct regulation of testicular activity by gonadotropin-inhibitory hormone and its receptor. Front Endocrinol (2014) 5. doi: 10.3389/fendo.2014.00008
20. Narwal R, Laxmi RK, Rawat VS, Sehgal N. Molecular cloning and bioinformatic characterization of Gonadotropin Inhibitory Hormone (GnIH) and its receptors in the freshwater murrel, Channa punctatus (Bloch 1793). Fish Physiol Biochem (2023) 49(4):711–36. doi: 10.1007/s10695-023-01211-7
21. Smith JT, Young IR, Veldhuij D, Clark IJ. Gonadotropin-inhibitory hormone (GnIH) secretion into the ovine hypophseal portal system. Endocrinology (2012) 153:3368–75. doi: 10.1210/en.2012-1088
22. Jiang DL, Pan JQ, Li JQ, Zhou XL, Shen X, Xu DN, et al. Effects of gonadotropin-inhibitory hormone on testicular development and reproduction-related gene expression in roosters. Anim Biotechnol (2023), 1–11. doi: 10.1080/10495398.2023.2266645
23. Silverman AJ, Livne I, Witkin JW. The gonadotropin-releasing hormone (GnRH), neuronal systems: immunocytochemistry and in situ hybridization. In: Knobil E, Neill JD, editors. The Physiology of Reproduction, 2nd. New York: Raven (1994). p. 1683–709.
24. Silverman AJ, Witkin JW, Silverman RC, Gibson MJ. Modulation of gonadotropin-releasing hormone neuronal activity as evidenced by uptake of fluorogold from the vasculature. Synapse (New York NY) (1990) 6(2):154–60. doi: 10.1002/syn.890060206
25. Odetayo AF, Adeyemi WJ, Olayaki LA. In vivo exposure to bisphenol F induces oxidative testicular toxicity: role of Erβ and p53/Bcl-2 signaling pathway. Front Reprod Health (2023) 5:1204728. doi: 10.3389/frph.2023.1204728
26. Odetayo AF, Adeyemi WJ, Olayaki LA. Omega-3 fatty acid ameliorates bisphenol F-induced testicular toxicity by modulating Nrf2/NFkB pathway and apoptotic signaling. Front Endocrinol (2023) 14:1256154. doi: 10.3389/fendo.2023.1256154
27. Godoy LD, Rossignoli MT, Delfino-Pereira P, Garcia-Cairasco N, de Lima Umeoka EH. A comprehensive overview on stress neurobiology: basic concepts and clinical implications. Front Behav Neurosci (2018) 12:127. doi: 10.3389/fnbeh.2018.00127
28. González CR, González B. Exploring the stress impact in the paternal germ cells epigenome: can catecholamines induce epigenetic reprogramming? Front Endocrinol (2021) 11:630948. doi: 10.3389/fendo.2020.630948
29. Carter JR, Goldstein DS. Sympathoneural and adrenomedullary responses to mental stress. Compr Physiol (2014), 119–46. doi: 10.1002/cphy.c140030
30. Chu B, Marwaha K, Sanvictores T, Ayers. Physiology, stress reaction, in: StatPearls (2022). Treasure Island (FL: StatPearls Publishing. Available at: https://www.ncbi.nlm.nih.gov/books/NBK541120/ (Accessed Updated 2022 Sep 12).
31. Choi ME, Yoo H, Lee HR, Moon IJ, Lee WJ, Song Y, et al. Carvedilol, an adrenergic blocker, suppresses melanin synthesis by inhibiting the cAMP/CREB signaling pathway in human melanocytes and ex vivo human skin culture. Int J Mol Sci (2020) 21(22):8796. doi: 10.3390/ijms21228796
32. Travers G, Kippelen P, Trangmar SJ, González-Alonso J. Physiological function during exercise and environmental stress in humans-an integrative view of body systems and homeostasis. Cells (2022) 11(3):383. doi: 10.3390/cells11030383
33. King SB, Toufexis DJ, Hammack SE. Pituitary adenylate cyclase activating polypeptide (PACAP), stress, and sex hormones. Stress (Amsterdam Netherlands) (2017) 20(5):465–75. doi: 10.1080/10253890.2017.1336535
34. Demirtaş Şahin T, Yazir Y, Utkan T, Gacar G, Halbutoğulları ZS, Gocmez SS. Depression induced by chronic stress leads to penile cavernosal dysfunction: protective effect of anti-TNF-α treatment. Can J Physiol Pharmacol (2018), 1–10. doi: 10.1139/cjpp-2017-0778
35. Radley J, Morilak D, Viau V, Campeau S. Chronic stress and brain plasticity: Mechanisms underlying adaptive and maladaptive changes and implications for stress-related CNS disorders. Neurosci Biobehav Rev (2015) 58:79–91. doi: 10.1016/j.neubiorev.2015.06.018
36. Bao J, Bedford MT. Epigenetic regulation of the histone-to-protamine transition during spermiogenesis. Reprod (Cambridge England) (2016) 151(5):R55–70. doi: 10.1530/REP-15-0562
37. McGrady AV. Effects of psychological stress on male reproduction: A review. Arch Androl (1984) 13(1):1–7. doi: 10.3109/01485018408987495
38. De Souza DB, Silva D, Cortez CM, Costa WS, Sampaio FJ. Effects of chronic stress on penile corpus cavernosum of rats. J Androl (2012) 33(4):735–759. doi: 10.2164/jandrol.111.014225
39. Neımark BA, Neımark AI, Tishchenko GE. Application of udenafil for the correction of endothelial disorders in patients with stress-induced erectile dysfunction. Urologiia (2013) 4:42–4.
40. Fatai OA, Aribidesi OL. Effect of bisphenol F on sexual performance and quality of offspring in Male Wistar rats. Ecotoxicol Environ Saf (2022) 244:114079. doi: 10.1016/j.ecoenv.2022.11407
41. Tsutsui K, Son YL, Kiyohara M, Miyata I. Discovery of gnIH and its role in hypothyroidism-induced delayed puberty. Endocrinology (2018) 159(1):62–8. doi: 10.1210/en.2017-00300
42. Kirby ED, Geraghty AC, Ubuka T, Bentley GE, Kaufer D. Stress increases putative gonadotropin inhibitory hormone and decreases luteinizing hormone in male rats. Proc Natl Acad Sci United States America (2009) 106(27):11324–9. doi: 10.1073/pnas.0901176106
43. Son YL, Ubuka T, Narihiro M, Fukuda Y, Hasunuma I, Yamamoto K, et al. Molecular basis for the activation of gonadotropin-inhibitory hormone gene transcription by corticosterone. Endocrinology (2014) 155(5):1817–26. doi: 10.1210/en.2013-2076
44. Tobari Y, Son YL, Ubuka T, Hasegawa Y, Tsutsui K. A new pathway mediating social effects on the endocrine system: female presence acting via norepinephrine release stimulates gonadotropin-inhibitory hormone in the paraventricular nucleus and suppresses luteinizing hormone in quail. J Neurosci: Off J Soc Neurosci (2014) 34(29):9803–11. doi: 10.1523/JNEUROSCI.3706-13.2014
45. Bédécarrats GY, Hanlon C, Tsutsui K. Gonadotropin inhibitory hormone and its receptor: potential key to the integration and coordination of metabolic status and reproduction. Front Endocrinol (2022) 12:781543. doi: 10.3389/fendo.2021.781543
46. Higuchi Y, Soga T, Parhar IS. Social defeat stress decreases mRNA for monoamine oxidase A and increases 5-HT turnover in the brain of male nile tilapia (Oreochromis niloticus). Front Pharmacol (2019) 9:1–9. doi: 10.3389/fphar.2018.01549
47. Sánchez MM, Aguado F, Sánchez-Toscano F, Saphier D. Neuroendocrine and immunocytochemical demonstrations of decreased hypothalamo-pituitary-adrenal axis responsivbisphenoleness to restraint stress after long-term social isolation. Endocrinology (1998) 139(2):579–87. doi: 10.1210/endo.139.2.5720
48. Ma X, Lee P, Chisholm DJ, James DE. Control of adipocyte differentiation in different fat depots; implications for pathophysiology or therapy. Front Endocrinol (2015) 6:1. doi: 10.3389/fendo.2015.00001
49. Helmreich DL, Parfitt DB, Lu X-Y, Akil H, Watson SJ. Relation between the hypothalamic-pituitary-thyroid (HPT) axis and the hypothalamic-pituitary-adrenal (HPA) axis during repeated stress. Neuroendocrinology (2005) 81(3):183–92. doi: 10.1159/000087001
50. Kakucska I, Qi Y, Lechan RM. Changes in adrenal status affect hypothalamic thyrotropin-releasing hormone gene expression in parallel with corticotropin-releasing hormone. Endocrinology (1995) 136(7):2795–802. doi: 10.1210/endo.136.7.7789304
51. Bianco AC, Nunes MT, Hell NS, Maciel RM. The role of glucocorticoids in the stress-induced reduction of extrathyroidal 3,5,3’-triiodothyronine generation in rats. Endocrinology (1987) 120(3):1033–8. doi: 10.1210/endo-120-3-1033
52. Kiyohara M, Son YL, Tsutsui K. Involvement of gonadotropin-inhibitory hormone in pubertal disorders induced by thyroid status. Sci Rep (2017) 7(1). doi: 10.1038/s41598-017-01183-8
53. Rodrigues MS, Fallah HP, Zanardini M, Malafaia G, Habibi HR, Nóbrega RH. Interaction between thyroid hormones and gonadotropin inhibitory hormone in ex vivo culture of zebrafish testis: An approach to study multifactorial control of spermatogenesis. Mol Cell Endocrinol (2021) 532:111331. doi: 10.1016/j.mce.2021.111331
54. Santos LC, Dos Anjos Cordeiro JM, da Silva Santana L, Barbosa EM, Santos BR, Mendonça LD, et al. Kisspeptin treatment reverses high prolactin levels and improves gonadal function in hypothyroid male rats. Sci Rep (2023) 13(1):16819. doi: 10.1038/s41598-023-44056-z
55. Son YL, Ubuka T, Tsutsui K. Molecular mechanisms of gonadotropin-inhibitory hormone (GnIH) actions in target cells and regulation of gnIH expression. Front Endocrinol (2019) 10:110. doi: 10.3389/fendo.2019.00110
56. Xiao Y, Liu D, Cline MA, Gilbert ER. Chronic stress, epigenetics, and adipose tissue metabolism in the obese state. Nutr Metab (2020) 17:88. doi: 10.1186/s12986-020-00513-4
57. Enebe JT, Enebe NO, Nwagha TU, Meka IA, Nwankwo ME, Izuka EO, et al. Serum leptin levels and relationship with maternal weight gain at term among obese and non-obese pregnant women in Enugu, Nigeria: a comparative cross-sectional study. J Int Med Res (2023) 51(11). doi: 10.1177/03000605231213265
58. Moschos S, Chan JL, Mantzoros CS. Leptin and reproduction: a review. Fertility Sterility (2002) 77(3):433–44. doi: 10.1016/S0015-0282(01)03010-2
59. Poling MC, Shieh MP, Munaganuru N, Luo E, Kauffman AS. Examination of the influence of leptin and acute metabolic challenge on RFRP-3 neurons of mice in development and adulthood. Neuroendocrinology (2014) 100(4):317–33. doi: 10.1159/000369276
60. Ndisang JF, Rastogi S. Cardiometabolic diseases and related complications: current status and future perspective. BioMed Res Int (2013) 2013:467682. doi: 10.1155/2013/467682
61. Iwasa T, Matsuzaki T, Tungalagsuvd A, Munkhzaya M, Kawami T, Niki H, et al. Hypothalamic Kiss1 and RFRP gene expressions are changed by a high dose of lipopolysaccharide in female rats. Hormones Behav (2014) 66(2):309–16. doi: 10.1016/j.yhbeh.2014.06.007
62. Sengupta P, Dutta S, Karkada IR, Akhigbe RE and Chinni SV. Irisin, energy homeostasis and male reproduction. Front Physiol (2021) 12:746049. doi: 10.3389/fphys.2021.746049
63. Poling MC, Kauffman AS. Regulation and function of RFRP-3 (GnIH) neurons during postnatal development. Frontier Endocrinol (Lausanne) (2015) 6:1–6. doi: 10.3389/fendo.2015.00150
64. Son YL, Ubuka T, Tsutsui K. Regulation of stress response on the hypothalamic-pituitary-gonadal axis via gonadotropin-inhibitory hormone. Frontier Neuroendocrinol (2020) 64:100953. doi: 10.1016/j.yfrne.2021.100953
65. Tsutsui K, Ubuka T. GnIH control of feeding and reproductive behaviors. Frontier Endocrinol (2016) 7:170. doi: 10.3389/fendo.2016.00170
66. Ubuka T, Bentley GE, Ukena K, Wingfield JC, Tsutsui K. Melatonin induces the expression of gonadotropin-inhibitory hormone in the avian brain. Proc Natl Acad Sci United States America (2005) 102(8):3052–7. doi: 10.1073/pnas.0403840102
67. Chowdhury VS, Yamamoto K, Ubuka T, Bentley GE, Hattori A, Tsutsui K. Melatonin stimulates the release of gonadotropin-inhibitory hormone by the avian hypothalamus. Endocrinology (2010) 151(1):271–80. doi: 10.1210/en.2009-0908
68. Adeyemi DH, Odetayo AF, Hamed MA, Akhigbe RE. Impact of COVID 19 on erectile function. Aging Male: Off J Int Soc Study Aging Male (2022) 25(1):202–16. doi: 10.1080/13685538.2022.2104833
69. Kang SS, Zhao E, Braga VF, Xian X, Bose M, Yue Z, et al. Structural organization of gonadotropin-inhibitory hormone neurons and receptor expression in the brain of eusocial naked mole-rats (Heterocephalus glaber). FASEB J (2019) 33(3):4678–89.
70. Lei C, Sonnino S, Yang L, Adams E, Fitak R, McGraw C, et al. Gonadotropin-inhibitory hormone is involved in the regulation of migration and mating activity in Pacific white shrimp. Gen Comp Endocrinol (2019) 276:72–80.
71. Schally AV, Arimura A, Kastin AJ, Matsuo H, Baba Y, Redding TW, et al. Gonadotropin-releasing hormone: one polypeptide regulates secretion of luteinizing and follicle-stimulating hormones. Sci (New York NY) (1971) 173(4001):1036–8. doi: 10.1126/science.173.4001.1036
72. Millar RP, Lu ZL, Pawson AJ, Flanagan CA, Morgan K, Maudsley SR. Gonadotropin-releasing hormone receptors. Endocrine Rev (2004) 25(2):235–75. doi: 10.1210/er.2003-0002
73. Pincus SM, Padmanabhan V, Lemon W, Randolph J, Rees Midgley A. Follicle-stimulating hormone is secreted more irregularly than luteinizing hormone in both humans and sheep. J Clin Invest (1998) 101(6):1318–24. doi: 10.1172/JCI985
74. Marques P, Skorupskaite K, Rozario KS, Anderson RA, George. JT. Physiology of gnRH and gonadotropin secretion, in: Endotext (2022). South Dartmouth (MA: MDText.com, Inc. Available at: https://www.ncbi.nlm.nih.gov/books/NBK279070/ (Accessed Updated 2022 Jan 5).
75. Caraty A, Martin GB, Montgomery G. A new method for studying pituitary responsiveness in vivo using pulses of LH-RH analogue in ewes passively immunized against native LH-RH. Reproduction Nutrition Dev (1984) 24(4):439–48. doi: 10.1051/rnd:19840409
76. Kaiser UB, Jakubowiak A, Steinberger A, Chin WW. Differential effects of gonadotropin-releasing hormone (GnRH) pulse frequency on gonadotropin subunit and GnRH receptor messenger ribonucleic acid levels in vitro. Endocrinology (1997) 138(3). doi: 10.1210/endo.138.3.4968
77. Padmanabhan V, Puttabyatappa M, Cardoso RC. Hypothalamus–pituitary–ovary axis. Encyclopedia Reprod (2018), 121–9. doi: 10.1016/B978-0-12-801238-3.64632-9
78. Akhigbe RE, Hamed MA, Odetayo AF. HAART and anti-Koch’s impair sexual competence, sperm quality and offspring quality when used singly and in combination in male Wistar rats. Andrologia (2021) 53(2):e13951.
79. Akhigbe RE, Hamed MA, Odetayo AF, Akhigbe TM, Oyedokun PA. Zinc improves sexual and erectile function in HAART-treated rats via the upregulation of erectogenic enzymes and maintenance of redox balance. Aging Male: Off J Int Soc Study Aging Male (2023) 26(1):2205517. doi: 10.1080/13685538.2023.2205517
80. Ubuka T, Morgan K, Pawson AJ, Osugi T, Chowdhury VS, Minakata H. Identification of human gnIH homologs, RFRP-1 and RFRP-3, and the cognate receptor, GPR147 in the human hypothalamic pituitary axis. PloS One (2009) 4:1–7. doi: 10.1371/journal.pone.0008400
81. Aquino NSS, Mansano NS, Vieira FAS, Silva KSC, Gusmao DO, Anderson GM, et al. RFamide-related peptide 3 signaling via neuropeptide FF receptor stimulates prolactin secretion in female rats. Endocrinology (2023) 164(8):bqad102. doi: 10.1210/endocr/bqad102
82. Odetayo AF, Olayaki LA. Omega 3 fatty acid improves sexual and erectile function in BPF-treated rats by upregulating NO/cGMP signaling and steroidogenic enzymes activities. Sci Rep (2023) 13:18060. doi: 10.1038/s41598-023-45344-4
83. McGuire NL, Bentley GE. Neuropeptides in the gonads:From evolution to pharmacology. Frontier Pharmacol (2010) 1:114.
84. Ogawa S, Parhar IS. Structural and functional divergence of gonadotropin-inhibitory hormone from jawless fish to mammals. Frontier Endocrinol (Lausanne) (2014) 5:1–17. doi: 10.3389/fendo.2014.00177
85. Atkinson HC, Millar RP, Balthazart J. Neural control of gonadotropin- releasing hormone and gonadotropin-inhibitory hormone, and associated neuronal networks involved in the regulation of testosterone production by the avian gonads. Front Endocrinol (2019) 10(162):1–28.
86. Uenoyama Y, Nagae M, Tsuchida H, Inoue N, Tsukamura H. Role of kndy neurons expressing kisspeptin, neurokinin B, and Dynorphin A as a gnrh pulse generator controlling mammalian reproduction. Front Endocrinol (2021) 12:724632. doi: 10.3389/fendo.2021.724632
87. Irwig MS, Fraley GS, Smith JT, Acohido BV, Popa SM, Cunningham MJ, et al. Kisspeptin activation of gonadotropin releasing hormone neurons and regulation of kiSS-1 mRNA in the male rat. Neuroendocrinology (2004) 80(4):264–72. doi: 10.1159/000083140
88. Han S-K, Gottsch ML, Lee KL, Popa SM, Smith JT, Jakawich SK, et al. Activation of gonadotropin-releasing hormone neurons by kisspeptin as a neuroendocrine switch for the onset of puberty. J Neurosci (2005) 25(49):11349–56. doi: 10.1523/JNEUROSCI.3328-05.2005
89. Meccariello R. The kisspeptin system in male reproduction. Endocrines (2022) 3:168–74. doi: 10.3390/endocrines3020015
90. Pinilla L, Aguilar E, Dieguez C, Millar RP, Tena-Sempere M. Kisspeptins and reproduction: physiological roles and regulatory mechanisms. Physiol Rev (2012) 92(3):1235–316. doi: 10.1152/physrev.00037.2010
91. Moore AM, Coolen LM, Porter DT, Goodman RL, Lehman MN. KNDy cells revisited. Endocrinology (2018) 159(9):3219–34. doi: 10.1210/en.2018-00389
92. Moore AM, Coolen LM, Lehman MN. Kisspeptin/Neurokinin B/Dynorphin (KNDy) cells as integrators of diverse internal and external cues: evidence from viral-based monosynaptic tract-tracing in mice. Sci Rep (2019) 9(1):14768. doi: 10.1038/s41598-019-51201-0
93. Smith JT, Cunningham MJ, Rissman EF, Clifton DK, Steiner RA. Regulation of Kiss1 gene expression in the brain of the female mouse. Endocrinology (2005) 146(9):3686–92. doi: 10.1210/en.2005-0488
94. Nestor CC, Kelly MJ, Rønnekleiv OK. Cross-talk between reproduction and energy homeostasis: central impact of estrogens, leptin and kisspeptin signaling. Hormone Mol Biol Clin Invest (2014) 17(3):109–28. doi: 10.1515/hmbci-2013-0050
95. Grachev P, Li XF, Hu MH, Li SY, Millar RP, Lightman SL, et al. Neurokinin B signaling in the female rat: a novel link between stress and reproduction. Endocrinology (2014) 155(7):2589–601. doi: 10.1210/en.2013-2038
96. Yang JA, Song CI, Hughes JK, Kreisman MJ, Parra RA, Haisenleder DJ, et al. Acute psychosocial stress inhibits LH pulsatility and kiss1 neuronal activation in female mice. Endocrinology (2017) 158(11):3716–23. doi: 10.1210/en.2017-00301
97. Sharma A, Thaventhiran T, Minhas S, Dhillo WS, Jayasena CN. Kisspeptin and testicular function-is it necessary? Int J Mol Sci (2020) 21(8):2958. doi: 10.3390/ijms21082958
98. Ramzan MH, Ramzan M, Ramzan F, Wahab F, Jelani M, Khan MA, et al. Insight into the serum kisspeptin levels in infertile males. Arch Iranian Med (2015) 18(1):12–7.
99. Semple RK, Achermann JC, Ellery J, Farooqi IS, Karet FE, Stanhope RG, et al. Two novel missense mutations in g protein-coupled receptor 54 in a patient with hypogonadotropic hypogonadism. J Clin Endocrinol Metab (2005) 90(3):1849–55. doi: 10.1210/jc.2004-1418
100. León S, Barroso A, Vázquez MJ, García-Galiano D, Manfredi-Lozano M, Ruiz-Pino F, et al. Direct actions of kisspeptins on gnRH neurons permit attainment of fertility but are insufficient to fully preserve gonadotropic axis activity. Sci Rep (2016) 6:19206. doi: 10.1038/srep19206
101. Rizwan MZ, Poling MC, Corr M, Cornes PA, Augustine RA, Quennell JH, et al. RFamide-related peptide-3 receptor gene expression in gnRH and kisspeptin neurons and gnRH-dependent mechanism of action. Endocrinology (2012) 153(8):3770–9. doi: 10.1210/en.2012-1133
102. Poling MC, Quennell JH, Anderson GM, Kauffman AS. Kisspeptin Neurones do not Directly Signal to RFRP-3 Neurones but RFRP-3 may Directly Modulate a Subset of Hypothalamic Kisspeptin Cells in Mice. J Neuroendocrinol (2013) 25(10):876–86. doi: 10.1111/jne.12084
103. Dungan HM, Gottsch ML, Zeng H, Gragerov A, Bergmann JE, Vassilatis DK, et al. The role of kisspeptin GPR54 signaling in the tonic regulation and surge release of gonadotropin-releasing hormone/luteinizing hormone. J Neurosci (2007) 27(44):12088–95. doi: 10.1523/JNEUROSCI.2748-07.2007
104. León S, García-Galiano D, Ruiz-Pino F, Barroso A, Manfredi-Lozano M, Romero-Ruiz A, et al. Physiological roles of gonadotropin-inhibitory hormone signaling in the control of mammalian reproductive axis: studies in the NPFF1 receptor null mouse. Endocrinology (2014) 155(8):2953–65. doi: 10.1210/en.2014-1030
105. Oishi H, Klausen C, Bentley GE, Osugi T, Tsutsui K, Gilks CB, et al. The human gonadotropin-inhibitory hormone ortholog RFamide-related peptide-3 suppresses gonadotropin-induced progesterone production in human granulosa cells. Endocrinology (2012) 153(7):3435–45. doi: 10.1210/en.2012-1066
106. Bentley GE, Ubuka T, McGuire NL, Chowdhury VS, Morita Y, Yano T, et al. Gonadotropininhibitory hormone and its receptor in the avian reproductive system. Gen Comp Endocrinol (2008) 156:34–43. doi: 10.1016/j.ygcen.2007.10.003
107. McGuire NL, Bentley GE. A functional neuropeptide system in vertebrate gonads: gonadotropin-inhibitory hormone and its receptor in testes of field-caught house sparrow (Passer domesticus). Gen Comp Endocrinol (2010) 166:566–72. doi: 10.1016/j.ygcen.2010.01.010
108. Purcell SH, Moley KH. Glucose transporters in gametes and preimplantation embryos. Trends Endocrinol Metab (2009) 20(10):483–9. doi: 10.1016/j.tem.2009.06.006
109. Afolabi OA, Anyogu DC, Hamed MA, Odetayo AF, Adeyemi DH, Akhigbe RE. Glutamine prevents upregulation of NF-kB signaling and caspase 3 activation in ischemia/reperfusion-induced testicular damage: An animal model. Biomed Pharmacother Biomed Pharmacother (2022) 150:113056. doi: 10.1016/j.biopha.2022.113056
110. Mohapatra SS, Mukherjee J, Banerjee D, Das PK, Ghosh PR, Das K. RFamide peptides, the novel regulators of mammalian HPG axis: A review. Vet World (2021) 14(7):1867–73. doi: 10.14202/vetworld.2021.1867-1873
111. McGuire NL, Kangas K, Bentley GE. Effects of melatonin on peripheral reproductive function:Regulation of testicular GnIH and testosterone. Endocrinology (2011) 152(9):3461–70. doi: 10.1210/en.2011-1053
112. Iwasa T, Matsuzaki T, Tungalagsuvd A, Munkhzaya M, Kuwahara A, Yasui T, et al. Effects of LPS injection on the hypothalamic and testicular mRNA expression levels of reproductive factors in male rats. Neurol Endocrinol Lett (2015) 36(3):193–5.
113. Schneider JE, Benton NA, Russo KA, Klingerman CM, Williams WP, Simberlund J, et al. RFamide-related peptide-3 and the trade-off between reproductive and ingestive behavior. Integr Comp Biol (2017) 57(6):1225–39. doi: 10.1093/icb/icx097
114. Zhao S, Zhu E, Yang C, Bentley GE, Tsutsui K, Kriegsfeld LJ. RFamide related peptide and messenger ribonucleic acid expression in mammalian testis:Association with the spermatogenic cycle. Endocrinology (2010) 151(2):617–27. doi: 10.1210/en.2009-0978
115. Zmijewska A, Czelejewska W, Dziekonski M, Gajewska A, Franczak A, Okrasa S. Effect of kisspeptin and RFamide-related peptide-3 on the synthesis and secretion of LH by pituitary cells of pigs during the estrous cycle. Anim Reprod Sci (2020) 214:106275. doi: 10.1016/j.anireprosci.2020.106275
116. Ubuka T, Tsutsui K. Evolution of gonadotropin-inhibitory hormone receptor and its ligand. Gen Comp Endocrinol (2014) 209:148–61. doi: 10.1016/j.ygcen.2014.09.002
117. Anjum S, Krishna A, Sridaran R, Tsutsui K. Localization of gonadotropin-releasing hormone (GnRH), gonadotropin-inhibitory hormone (GnIH), kisspeptin and GnRH receptor and their possible roles in testicular activities from birth to senescence in mice. J Exp Zool A Ecol Genet Physiol (2012) 317(10):630–44. doi: 10.1002/jez.1765
118. Anjum S, Krishna A, Tsutsui K. Inhibitory roles of the mammalian GnIH ortholog RFRP3 in testicular activities in adult mice. J Endocrinol (2014) 223(1):79–91. doi: 10.1530/JOE-14-0333
119. Smith LB, Walker WH. The regulation of spermatogenesis by androgens. Semin Cell Dev Biol (2014) 30:2–13. doi: 10.1016/j.semcdb.2014.02.012
120. Sharpe RM, McKinnell C, Kivlin C, Fisher JS. Proliferation and functional maturation of Sertoli cells, and their relevance to disorders of testis function in adulthood. Reproduction-Cambridge (2003) 125(6):769–84. doi: 10.1530/rep.0.1250769
121. Akhigbe RE, Hamed MA, Odetayo AF, Akhigbe TM, Ajayi AF, Ajibogun F. Omega-3 fatty acid rescues ischaemia/perfusion-induced testicular and sperm damage via modulation of lactate transport and xanthine oxidase/uric acid signaling. Biomed Pharmacother Biomed Pharmacother (2021) 142:111975. doi: 10.1016/j.biopha.2021.111975
122. Marques JVO, Boguszewski CL. Fertility issues in aggressive pituitary tumors. Rev Endocr Metab Disord (2020) 21:225–33. doi: 10.1007/s11154-019-09530-y
123. Sanchez RM, Mattioli M, Valbonetti L, Barboni B, Bernabò N, Capacchietti G. Chapter membrane dynamics of spermatozoa during capacitation: new insight in germ cells signaling. (2018).
Keywords: fertility, reproductive hormones, hypothalamus, pituitary, stress, testosterone
Citation: Odetayo AF, Akhigbe RE, Bassey GE, Hamed MA and Olayaki LA (2024) Impact of stress on male fertility: role of gonadotropin inhibitory hormone. Front. Endocrinol. 14:1329564. doi: 10.3389/fendo.2023.1329564
Received: 29 October 2023; Accepted: 18 December 2023;
Published: 08 January 2024.
Edited by:
Marc Yeste, University of Girona, SpainReviewed by:
Rosaria Meccariello, University of Naples Parthenope, ItalyEbtesam Abdullah Al-Suhaimi, King Abdulaziz and His Companions Foundation for Giftedness and Creativity, Saudi Arabia
Copyright © 2024 Odetayo, Akhigbe, Bassey, Hamed and Olayaki. This is an open-access article distributed under the terms of the Creative Commons Attribution License (CC BY). The use, distribution or reproduction in other forums is permitted, provided the original author(s) and the copyright owner(s) are credited and that the original publication in this journal is cited, in accordance with accepted academic practice. No use, distribution or reproduction is permitted which does not comply with these terms.
*Correspondence: Roland E. Akhigbe, YWtoaWdiZXJvbGFuZEBnbWFpbC5jb20=; Luqman A. Olayaki, b2xheWFraUBnbWFpbC5jb20=