- 1Department of Obstetrics and Gynecology, Faculty of Medical Sciences, University of Fukui, Fukui, Japan
- 2Department of Nursing, Faculty of Nursing and Welfare Sciences, Fukui Prefectural University, Fukui, Japan
- 3Department of Obstetrics and Gynecology, Ishikawa Prefectural Central Hospital, Ishikawa, Japan
The ovarian microenvironment is critical for follicular development and oocyte maturation. Maternal conditions, including polycystic ovary syndrome (PCOS), endometriosis, and aging, may compromise the ovarian microenvironment, follicular development, and oocyte quality. Chronic low-grade inflammation can induce oxidative stress and tissue fibrosis in the ovary. In PCOS, endometriosis, and aging, pro-inflammatory cytokine levels are often elevated in follicular fluids. In women with obesity and PCOS, hyperandrogenemia and insulin resistance induce ovarian chronic low-grade inflammation, thereby disrupting follicular development by increasing oxidative stress. In endometriosis, ovarian endometrioma-derived iron overload can induce chronic inflammation and oxidative stress, leading to ovarian ferroptosis and fibrosis. In inflammatory aging (inflammaging), senescent cells may secrete senescence-associated secretory phenotype factors, causing chronic inflammation and oxidative stress in the ovary. Therefore, controlling chronic low-grade inflammation and fibrosis in the ovary would present a novel therapeutic strategy for improving the follicular microenvironment and minimizing ovarian dysfunction.
1 Introduction
Acute and chronic low-grade inflammation are two distinct types of inflammatory responses in the body. Acute inflammation is the immediate and transient response to tissue injury, infection, or foreign substances. Although acute inflammation can be associated with severe symptoms, it is a normal and essential process for eliminating harmful stimuli, initiating tissue repair, and restoring homeostasis (1, 2). Acute inflammation typically resolves within a few days or weeks.
In contrast, chronic low-grade inflammation is characterized by long-term, mild, systemic inflammation (3). Unlike acute inflammation, it may not manifest obvious signs or symptoms. Nevertheless, chronic low-grade inflammation disrupts immune function and causes tissue damage. It significantly contributes to the development and progression of various diseases, including cardiovascular diseases, metabolic disorders, neurodegenerative conditions, and certain types of cancer (4).
Although the relationship between inflammation and reproduction is complex, chronic low-grade inflammation can influence many ovarian reproductive processes (5, 6). Therefore, this review highlights recent findings regarding the impact of chronic low-grade inflammation on ovarian function.
2 Overview of chronic low-grade inflammation
Cytokines function as chemical messengers in the immune system, facilitating communication between cells and regulating immune responses. Various immune cells (e.g., macrophages, neutrophils, and lymphocytes) secrete cytokines, which have pro- or anti-inflammatory effects. Notably, pro-inflammatory cytokines are of particular significance in chronic low-grade inflammation. These cytokines, including tumor necrosis factor-alpha (TNFα), interleukin-1 beta (IL-1β), and interleukin-6 (IL-6), are released in response to inflammatory signals (7, 8). Moreover, the dysregulated production of pro-inflammatory cytokines promotes further inflammation and tissue damage via the inhibitor of nuclear factor kappa-B kinase subunit beta/nuclear factor kappa-B (NF-κB) and Janus kinase/signal transducer and activator of transcription signaling pathways.
Chronic low-grade inflammation and reactive oxygen species (ROS) are closely linked. ROS are chemically reactive oxygen-containing molecules, including superoxide (O2-), hydrogen peroxide (H2O2), and hydroxyl radicals (•HO). They play crucial roles in physiological processes, and a delicate balance with the antioxidant defense system maintains cellular homeostasis. However, excessive or uncontrolled ROS production causes oxidative stress in chronic low-grade inflammation (9). ROS can damage cellular components, including lipids, proteins, and DNA, and induce cell death. Consequently, oxidative stress activates several signaling pathways, contributing to further inflammation, tissue damage, and subsequent fibrosis (10, 11).
Fibrosis involves the excessive deposition of extracellular matrix (ECM) components, leading to tissue remodeling and scarring (12). Collagens are the main components of the ECM, and the increased deposition of type I and III collagen is a hallmark of fibrosis (13). Additionally, increased ROS levels and oxidative stress activate transforming growth factor-β1 (TGF-β1), which is a key cytokine involved in the fibrotic process (14). TGF-β1 promotes fibroblast proliferation, fibroblast-to-myofibroblast conversion, and ECM production and deposition (14, 15). Furthermore, fibrosis disrupts tissue architecture and can cause organ dysfunction in the liver, lungs, heart, kidneys, skin, and possibly ovaries (12, 16, 17).
3 Folliculogenesis in the ovarian cortex
The ovarian follicle, comprising an oocyte surrounded by somatic cells (cumulus cells [CCs], granulosa cells [GCs], and theca cells [TCs]), represents the basic functional unit for female reproduction. Folliculogenesis involves the activation of primordial follicles; continual growth through primary, secondary, preantral, and antral follicles; selection and maturation of dominant follicle(s); and ovulation. It is tightly regulated by pituitary gonadotropins (follicle‐stimulating hormone [FSH] and luteinizing hormone [LH]) and intraovarian regulators, including steroids, growth factors, and cytokines (18). More than 99% of follicles do not become dominant, leading to growth arrest and eventual atretic degeneration. Consequently, this results in the loss of follicles and oocytes (19). Oogenesis is a process involving the forming and development of a competent, mature oocyte within ovarian follicles. The surrounding CCs, GCs, and TCs provide essential steroids, growth factors, cytokines, and metabolites for oogenesis (20). Therefore, the follicular microenvironment is critical for oocyte growth, maturation, and the acquisition of developmental competence (21).
The ovarian stroma comprises both the cortex and medulla. The composition and organization of the stromal ECM change dynamically around the follicles (22). In vitro follicle culture experiments have demonstrated that growing follicles are sensitive to the stiffness of the surrounding ECM (16). The biomechanical pressure exerted by the surrounding stroma can influence follicular expansion and development through mechano-transduction pathways. For instance, Hippo signaling disruption and Akt stimulation are known to promote folliculogenesis in rodents and humans (22–24). Early follicles lack direct blood supply and receive various substances through passive diffusion from the surrounding stromal tissue (25). The progression of folliculogenesis beyond the preantral stage requires angiogenesis and vascularity in the ovarian stroma and TC layer to supply nutrients, oxygen, and gonadotropins.
4 Pro-inflammatory cytokines and follicular development
Altered levels of pro-inflammatory cytokines (e.g., TNFα, IL-1β, and IL-6) in the follicular microenvironment can negatively impact ovarian function (26, 27).
TNFα induced apoptosis and inhibited steroidogenesis in rat, bovine, and human GCs, indicating its negative impact on GCs (28, 29). In contrast, the effects of TNFα on TCs functions are inconclusive (30–32). Importantly, TNFα gene deletion in mice showed increased GC proliferation and decreased oocyte apoptosis, resulting in prolonged fertility (33). Therefore, excess TNFα is believed to adversely affect follicular development and cause follicular atresia (26). Moreover, TNFα can induce oxidative stress in porcine oocytes, causing DNA and mitochondrial damage and reducing oocyte quality (34).
IL-1β suppressed FSH and LH receptor (LHR) expression in mice, rat, and porcine GCs (26), as well as estradiol production in rodent and human GCs (26). Conversely, another study found that IL-1β stimulated bovine GC proliferation (35) and suppressed apoptosis in rat follicular cells (36). Notably, IL-1 gene deficiency in mice resulted in an increase rather than a decrease in fertility (37). Therefore, IL-1β negatively impacts follicular development and oocyte maturation (38, 39).
IL-6 inhibited FSH-induced LHR expression in rat and porcine GCs (40, 41) and suppressed FSH-induced steroidogenesis in rat and bovine GCs (42, 43). Although a report indicated that IL-6 enhanced FSH-induced LHR expression in rat GCs (44), excess IL-6 is commonly associated with aging and believed to affect follicular development negatively (45–47).
Chronic low-grade inflammation can cause persistent oxidative stress in the ovary (48). Additionally, high ROS levels and low antioxidant capacity in follicular fluids were associated with poor pregnancy outcomes in human-assisted reproductive technology (ART) (49, 50). Maternal conditions, including polycystic ovary syndrome (PCOS), endometriosis, and aging, may compromise the ovarian microenvironment (47, 48, 51). Therefore, the following sections discuss whether and how chronic low-grade inflammation negatively impacts ovarian function in PCOS, endometriosis, and aging.
5 PCOS and chronic low-grade inflammation
PCOS is the most common cause of ovarian dysfunction, with a prevalence of 8–13% in reproductive-aged women (52). The clinical and pathological hallmarks of PCOS include oligo/anovulatory ovarian dysfunction, polycystic ovarian morphology, and clinical/biochemical hyperandrogenism (53). Follicular development frequently halts at the small antral stage in PCOS, preventing full maturation and ovulation. Hyperandrogenism, insulin resistance, hypothalamic-pituitary-ovarian axis imbalance (LH > FSH), and chronic low-grade inflammation are major contributors to the pathophysiological changes observed in PCOS (54, 55). In ART for PCOS, ovarian stimulation overcomes the follicular growth arrest and allows more oocytes to be retrieved. However, the percentage of high-quality oocytes/embryos was lower in PCOS cases than in non-PCOS cases (56).
Follicular fluid is derived from blood and tissue fluid, and its composition correlates with that of the serum (27). Women with PCOS usually exhibit elevated serum levels of inflammatory markers, including C-reactive protein (CRP), TNFα, and IL-6 (6, 57). They also exhibit higher concentrations of TNF-α and interleukins in follicular fluids (58–60). Furthermore, macrophage and lymphocyte infiltration increases throughout the ovary in these women (61). Systemic and local chronic low-grade inflammation can increase oxidative stress in the ovary and negatively impact folliculogenesis in PCOS (6, 62) (Figure 1A). These phenomena are more pronounced in patients with obesity and PCOS (i.e., obese PCOS) than in normal-weight patients with PCOS (6).
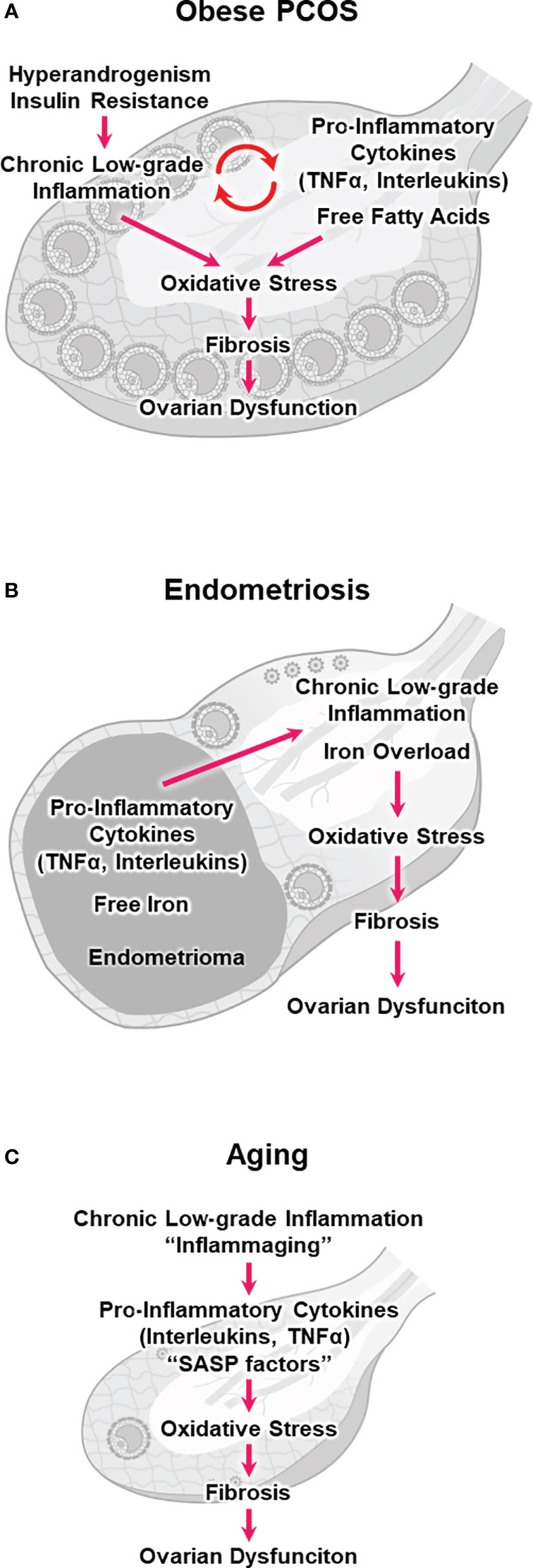
Figure 1 Hypothetical models illustrating chronic low-grade inflammation, oxidative stress, fibrosis, and ovarian dysfunction. (A) obesity and polycystic ovary syndrome (obese PCOS), (B) endometriosis, and (C) aging.
The negative impact of obesity on ovarian function is evident, and ovulatory infertility prevalence in women with obesity is up to three times higher than that in those without obesity (63). Decreased pregnancy and birth rates in women with obesity were overcome by oocyte donation from those without obesity, suggesting that obesity disrupts folliculogenesis and reduces oocyte quality (64). Furthermore, excess adipose tissue produces inflammatory adipokines, including TNFα, IL-6, and free fatty acids (FFAs), which can contribute to cellular lipotoxicity, inflammation, and oxidative stress in the ovary (65). Oocytes from obese mice exhibited decreased germinal vesicle breakdown and polar body extrusion, along with abnormalities in spindle structure, chromosome alignment, and mitochondrial function (65–69). Therefore, these results suggest that obesity-induced oxidative stress negatively impacts both the meiotic and cytoplasmic maturation of oocytes.
Patients with obese PCOS who exhibit hyperandrogenism and insulin resistance are at a higher risk of abnormal folliculogenesis and poor oocyte competence (70). Hyperandrogenemia impairs the hypothalamic-pituitary-ovarian axis, resulting in a sustained increase in the gonadotropin-releasing hormone pulse frequency and the hypersecretion of LH over FSH (71). Hyperinsulinemia stimulates LH activity in TCs and promotes ovarian hyperandrogenism, which prevents follicular maturation and promotes follicular atresia (72). Additionally, insulin resistance can trigger a series of pro-inflammatory events, including hyperglycemia and oxidative stress (73, 74). Androgens promoted the differentiation of pre-adipocytes into mature adipocytes and increased lipolysis, resulting in an elevated release of FFAs (75, 76). Women with PCOS had higher FFA levels in follicular fluid, causing lipotoxicity and endoplasmic reticulum stress in the follicular microenvironment (77). These results suggest that hyperandrogenism and insulin resistance directly or indirectly stimulate chronic low-grade inflammation and increase ovarian oxidative stress (Figure 1A), which consequently compromises follicular maturation and oocyte quality in PCOS without follicle loss (57, 71).
Ovarian dysfunction in women with PCOS may also be correlated with ovarian fibrosis, which is characterized by excessive fibroblast proliferation and ECM deposition in the ovary (65, 78). Follicles in PCOS are surrounded by densely collagenized rigid stroma, which may inhibit follicular development (16). The molecular mechanisms underlying ovarian fibrosis in PCOS remain unclear. However, increased ROS levels and oxidative stress can activate TGF-β1, which is a key cytokine involved in tissue fibrosis (14). TGF-β1 levels were elevated in the serum and ovaries of women with PCOS and rat PCOS models (79–83). Therefore, these results indicate that ROS-induced TGF-β1 signaling may be involved in the pathophysiology of PCOS by stimulating ovarian fibrosis.
6 Endometriosis and chronic low-grade inflammation
Endometriosis is among the most common causes of infertility, with a prevalence of 10–15% in reproductive-aged women (84). It is a disease characterized by the growth of endometrial tissue outside the uterine cavity and estrogen-dependent chronic inflammation, primarily affecting pelvic tissues, including the ovary (i.e., endometrioma) (85). Although the effect of endometrioma on folliculogenesis remains inconclusive, follicular growth was significantly suppressed in the ovarian cortex surrounding the endometrioma (86). In ART, women with endometrioma had fewer retrieved oocytes than those without endometrioma, suggesting that endometrioma reduces follicular response to ovarian stimulation (84, 87, 88).
The endometrioma is surrounded by stroma and a single layer of columnar epithelial cells, but unlike other benign cysts, it is not surrounded by a capsule (17, 22). The toxic components of endometrioma, such as pro-inflammatory cytokines and ROS, can readily diffuse into the ovarian cortex and adjacent follicles (89). Indeed, altered levels of TNFα, interleukins, and ROS have been reported in the follicular fluid adjacent to the endometrioma (90, 91). These pro-inflammatory cytokines and ROS can induce oxidative stress and fibrosis in the ovary, impairing folliculogenesis and oocyte maturation (Figure 1B) (17, 92).
Free iron may also be harmful to the ovary, and the endometrioma fluid contains large amounts of free iron, approximately 10 times higher than the serum level (17). Iron levels were significantly elevated in the follicular fluid adjacent to endometriomas (93). Notably, iron is an essential mineral that plays a crucial role in various physiological processes, such as oxygen transport and energy production. However, ferrous iron (Fe2+) catalyzes the conversion from H2O2 to •HO via the Fenton reaction (Fe2+ + H2O2 → Fe3+ + •HO + OH−). •HO is among the most reactive and toxic ROS. Consequently, unbalanced and excessive iron levels (i.e., iron overload) can induce inflammation, oxidative stress, lipid peroxidation of the cellular membrane, and subsequent ferroptotic cell death (i.e., ferroptosis), a new type of programmed cell death (Figure 1B) (94–97). Although the effect of ferroptosis on ovarian function in endometriosis remains unclear, iron overload in the follicular fluid has been shown to induce GC ferroptosis and oocyte dysmaturation in endometriosis (98).
Ovarian dysfunction in endometriosis may also be correlated with ovarian fibrosis. One of the histologic features associated with endometriomas is fibrosis inside and outside the cyst (99). Indeed, follicular density was lower in the ovarian cortex adjacent to endometriomas, possibly because of the inhibition of angiogenesis, increased follicular atresia, and induction of fibrosis (100–102). Although the molecular mechanisms underlying ovarian fibrosis in endometriosis are yet to be elucidated, it is noteworthy that disruption in iron homeostasis induces organ fibrosis in the liver, heart, and pancreas (45). Endometrioma-derived free iron, pro-inflammatory cytokines, and ROS may cooperate with TGF-β1 signaling to promote stromal fibrosis around endometriomas (99, 103, 104).
Nevertheless, whether endometrioma reduces oocyte quality remains controversial. Many previous studies have shown poor ART outcomes in women with endometriosis (84, 105). Additionally, oocyte donation programs showed that embryos derived from women with endometrioma have lower implantation rates than those from women without endometrioma, suggesting reduced oocyte quality in endometriosis (91, 106). Oocytes from patients with endometrioma exhibited zona pellucida hardening, altered spindle structure, and decreased mitochondrial number (84, 107, 108). However, recent reports have shown that the presence of endometrioma does not affect clinical pregnancy and live birth rates, provided multiple oocytes are successfully retrieved and fertilized in the ART setting (87, 109). Although endometrioma negatively impacts the number of growing follicles and oocytes retrieved, whether the percentage of high-quality oocytes/embryos is lower in endometriosis remains inconclusive (84).
7 Aging and chronic low-grade inflammation
Female fertility begins to decline in the late 20s, and the decline accelerates rapidly beyond the mid-30s (110). Ovarian aging becomes noticeable in women in their late 30s and typically completes around the age of 50, suggesting that ovaries may be more susceptible to aging than other organs (111, 112). Notably, lower live birth rates and higher miscarriage rates in aged women were overcome by oocyte donation from young women, indicating that oocyte quality declines with age (113).
The progressive decline in oocyte quality and quantity is the main cause of age-related decline in female fertility. Multiple oocyte-related factors contribute to age-associated infertility, including chromosome mis-segregation, meiotic recombination errors, DNA damage, telomere shortening, mitochondrial dysfunction, genetic mutations, and protein metabolic dysregulation (111).
Additionally, the inflammatory follicular microenvironment and stromal fibrosis might contribute to ovarian aging (Figure 1C) (46, 47). Physiological aging is associated with chronic low-grade inflammation (114). Cellular senescence is the irreversible cell cycle arrest associated with aging. Senescent cells secrete inflammatory substances known as senescence-associated secretory phenotype (SASP) factors (e.g., IL-6, IL-1β, and IL-8). SASP factors induce chronic inflammation and oxidative stress in surrounding cells (47, 112). Inflammatory aging (i.e., inflammaging) has been implicated in age-related diseases and conditions, such as cardiovascular disease, neurodegenerative disorders, metabolic dysfunction, and possibly ovarian dysfunction (112).
Serum concentrations of IL-6, IL-1β, and TNFα were modestly but definitely increased in aged women (115). Aged women also showed higher IL-6 concentrations and lower antioxidant levels in follicular fluids (27, 116). Aged mice exhibited increased macrophage infiltration and fusion and pro-inflammatory cytokine expression in the ovary (45, 46, 117). Furthermore, the NF-κB pathway, which is a major inflammatory signaling pathway, was also activated in the ovaries of aged mice (118).
Whether and how inflammaging affects physiological ovarian aging remains unknown. Nevertheless, sustained small changes in pro-inflammatory cytokine expression and subsequent long-term accumulation of oxidative stress may deteriorate the follicular microenvironment and cause GC apoptosis and follicular atresia (Figure 1C) (46, 47, 119). Cellular senescence and SASP secretion were significantly increased in the ovaries of aged mice, particularly in TCs (120). Oocyte-somatic cell communication is indispensable for folliculogenesis and oocyte maturation (18, 121, 122). Loss of support from surrounding CCs, GCs, and TCs can result in defects in oocyte chromosomal, genetic, mitochondrial, and cytoplasmic factors, compromising oocyte quality (111, 112).
Furthermore, ovarian ECM deposition and stromal fibrosis were also observed in aged mice and humans (16, 45, 123). Transcriptome analysis of aged women showed that hypoxia stress response-associated genes (e.g., genes downstream of the hypoxia-inducible factor-1 pathway) were overexpressed in CCs, suggesting a hypoxic microenvironment in aging follicles (124). In the aging ovary, stromal changes, such as increased fibrosis and decreased angiogenesis, may induce a stressed environment (e.g., hypoxia), leading to impaired folliculogenesis and reduced oocyte quality (47).
8 Conclusion
Recent research suggests that chronic low-grade inflammation is associated with ovarian dysfunction in women with PCOS, endometriosis, and aging. However, our understanding of the impact of the pro-inflammatory microenvironment and fibrotic ECM remodeling on folliculogenesis and oocyte maturation remains limited. Therefore, explaining the pathologies of ovarian dysfunction in PCOS, endometriosis, and aging by chronic low-grade inflammation is still difficult. It remains unclear whether chronic low-grade inflammation and fibrosis are the cause or consequence of these conditions. Nevertheless, controlling chronic low-grade inflammation and fibrosis in the ovary would represent a novel therapeutic strategy to improve the follicular microenvironment. Animal models have indicated that antioxidants (27, 51), insulin-sensitizing drugs (125), anti-aging drugs (senolytics) (126), immune checkpoint inhibitors (127), and antifibrosis drugs (125) may help minimize follicular depletion and oocyte quality decline. However, further studies are required to determine whether these drugs overcome ovarian dysfunction in PCOS, endometriosis, and aging.
Author contributions
MO: Conceptualization, Writing – original draft. TM: Resources, Visualization, Writing – review & editing. YM: Resources, Visualization, Writing – review & editing. AS: Resources, Visualization, Writing – review & editing. CT: Resources, Visualization, Writing – review & editing. MF: Resources, Visualization, Writing – review & editing. HT: Resources, Visualization, Writing – review & editing. YY: Supervision, Writing – review & editing.
Funding
The author(s) declare financial support was received for the research, authorship, and/or publication of this article. This work was supported by JSPS KAKENHI (18K09224 and 22K09592).
Acknowledgments
We would like to thank Editage (www.editage.com) for English language editing.
Conflict of interest
The authors declare that the research was conducted in the absence of any commercial or financial relationships that could be construed as a potential conflict of interest.
Publisher’s note
All claims expressed in this article are solely those of the authors and do not necessarily represent those of their affiliated organizations, or those of the publisher, the editors and the reviewers. Any product that may be evaluated in this article, or claim that may be made by its manufacturer, is not guaranteed or endorsed by the publisher.
Abbreviations
TNFα, tumor necrosis factor-alpha; IL-1β, interleukin-1 beta; IL-6, interleukin-6; ROS, reactive oxygen species; ECM, extracellular matrix; TGF-β1, transforming growth factor-β1; CCs, cumulus cells; GCs, granulosa cells; TCs, theca cells; FSH, follicle‐stimulating hormone; LH, luteinizing hormone; ART, assisted reproductive technology; PCOS, polycystic ovary syndrome; FFAs, free fatty acids.
References
1. Netea MG, Balkwill F, Chonchol M, Cominelli F, Donath MY, Giamarellos-Bourboulis EJ, et al. A guiding map for inflammation. Nat Immunol (2017) 18:826–31. doi: 10.1038/ni.3790
2. Kotas ME, Medzhitov R. Homeostasis, inflammation, and disease susceptibility. Cell (2015) 160:816–27. doi: 10.1016/j.cell.2015.02.010
3. Straub RH. The brain and immune system prompt energy shortage in chronic inflammation and ageing. Nat Rev Rheumatol (2017) 13:743–51. doi: 10.1038/nrrheum.2017.172
4. Furman D, Campisi J, Verdin E, Carrera-Bastos P, Targ S, Franceschi C, et al. Chronic inflammation in the etiology of disease across the life span. Nat Med (2019) 25:1822–32. doi: 10.1038/s41591-019-0675-0
5. Weiss G, Goldsmith LT, Taylor RN, Bellet D, Taylor HS. Inflammation in reproductive disorders. Reprod Sci (Thousand Oaks Calif) (2009) 16:216–29. doi: 10.1177/1933719108330087
6. Velez LM, Seldin M, Motta AB. Inflammation and reproductive function in women with polycystic ovary syndrome†. Biol Reprod (2021) 104:1205–17. doi: 10.1093/biolre/ioab050
7. Bruunsgaard H, Pedersen M, Pedersen BK. Aging and proinflammatory cytokines. Curr Opin Hematol (2001) 8:131–6. doi: 10.1097/00062752-200105000-00001
8. Chung HY, Cesari M, Anton S, Marzetti E, Giovannini S, Seo AY, et al. Molecular inflammation: underpinnings of aging and age-related diseases. Ageing Res Rev (2009) 8:18–30. doi: 10.1016/j.arr.2008.07.002
9. Juan CA, Pérez de la Lastra JM, Plou FJ, Pérez-Lebeña E. The chemistry of reactive oxygen species (ROS) revisited: outlining their role in biological macromolecules (DNA, lipids and proteins) and induced pathologies. Int J Mol Sci (2021) 22:4642. doi: 10.3390/ijms22094642
10. Mittal M, Siddiqui MR, Tran K, Reddy SP, Malik AB. Reactive oxygen species in inflammation and tissue injury. Antioxidants Redox Signaling (2014) 20:1126–67. doi: 10.1089/ars.2012.5149
11. Schieber M, Chandel NS. ROS function in redox signaling and oxidative stress. Curr biology: CB (2014) 24:R453–462. doi: 10.1016/j.cub.2014.03.034
12. Wynn TA, Ramalingam TR. Mechanisms of fibrosis: therapeutic translation for fibrotic disease. Nat Med (2012) 18:1028–40. doi: 10.1038/nm.2807
13. Herrera J, Forster C, Pengo T, Montero A, Swift J, Schwartz MA, et al. Registration of the extracellular matrix components constituting the fibroblastic focus in idiopathic pulmonary fibrosis. JCI Insight (2019) 4:e125185. doi: 10.1172/jci.insight.125185
14. Richter K, Kietzmann T. Reactive oxygen species and fibrosis: further evidence of a significant liaison. Cell Tissue Res (2016) 365:591–605. doi: 10.1007/s00441-016-2445-3
15. Frangogiannis N. Transforming growth factor-β in tissue fibrosis. J Exp Med (2020) 217:e20190103.
16. Biswas A, Ng BH, Prabhakaran VS, Chan CJ. Squeezing the eggs to grow: The mechanobiology of mammalian folliculogenesis. Front Cell Dev Biol (2022) 10:1038107. doi: 10.3389/fcell.2022.1038107
17. Sanchez AM, Viganò P, Somigliana E, Panina-Bordignon P, Vercellini P, Candiani M. The distinguishing cellular and molecular features of the endometriotic ovarian cyst: from pathophysiology to the potential endometrioma-mediated damage to the ovary. Hum Reprod Update (2014) 20:217–30. doi: 10.1093/humupd/dmt053
18. Orisaka M, Miyazaki Y, Shirafuji A, Tamamura C, Tsuyoshi H, Tsang BK, et al. The role of pituitary gonadotropins and intraovarian regulators in follicle development: A mini-review. Reprod Med Biol (2021) 20:169–75. doi: 10.1002/rmb2.12371
19. Hsueh AJ, Billig H, Tsafriri A. Ovarian follicle atresia: a hormonally controlled apoptotic process. Endocrine Rev (1994) 15:707–24. doi: 10.1210/edrv-15-6-707
20. Arroyo A, Kim B, Yeh J. Luteinizing hormone action in human oocyte maturation and quality: signaling pathways, regulation, and clinical impact. Reprod Sci (Thousand Oaks Calif) (2020) 27:1223–52. doi: 10.1007/s43032-019-00137-x
21. Hennet ML, Combelles CM. The antral follicle: a microenvironment for oocyte differentiation. Int J Dev Biol (2012) 56:819–31. doi: 10.1387/ijdb.120133cc
22. Fiorentino G, Cimadomo D, Innocenti F, Soscia D, Vaiarelli A, Ubaldi FM, et al. Biomechanical forces and signals operating in the ovary during folliculogenesis and their dysregulation: implications for fertility. Hum Reprod Update (2023) 29:1–23. doi: 10.1093/humupd/dmac031
23. Kawamura K, Cheng Y, Suzuki N, Deguchi M, Sato Y, Takae S, et al. Hippo signaling disruption and Akt stimulation of ovarian follicles for infertility treatment. Proc Natl Acad Sci United States America (2013) 110:17474–9. doi: 10.1073/pnas.1312830110
24. Hsueh AJ, Kawamura K, Cheng Y, Fauser BC. Intraovarian control of early folliculogenesis. Endocrine Rev (2015) 36:1–24. doi: 10.1210/er.2014-1020
25. Esencan E, Beroukhim G, Seifer DB. Age-related changes in Folliculogenesis and potential modifiers to improve fertility outcomes - A narrative review. Reprod Biol endocrinology: RB&E (2022) 20:156. doi: 10.1186/s12958-022-01033-x
26. Silva JRV, Lima FEO, Souza ALP, Silva AWB. Interleukin-1β and TNF-α systems in ovarian follicles and their roles during follicular development, oocyte maturation and ovulation. Zygote (Cambridge England) (2020) 28:270–7. doi: 10.1017/S0967199420000222
27. Yang Z, Tang Z, Cao X, Xie Q, Hu C, Zhong Z, et al. Controlling chronic low-grade inflammation to improve follicle development and survival. Am J Reprod Immunol (New York NY: 1989) (2020) 84:e13265. doi: 10.1111/aji.13265
28. Kaipia A, Chun SY, Eisenhauer K, Hsueh AJ. Tumor necrosis factor-alpha and its second messenger, ceramide, stimulate apoptosis in cultured ovarian follicles. Endocrinology (1996) 137:4864–70. doi: 10.1210/endo.137.11.8895358
29. Sasson R, Winder N, Kees S, Amsterdam A. Induction of apoptosis in granulosa cells by TNF alpha and its attenuation by glucocorticoids involve modulation of Bcl-2. Biochem Biophys Res Commun (2002) 294:51–9. doi: 10.1016/S0006-291X(02)00431-X
30. Spaczynski RZ, Arici A, Duleba AJ. Tumor necrosis factor-alpha stimulates proliferation of rat ovarian theca-interstitial cells. Biol Reprod (1999) 61:993–8. doi: 10.1095/biolreprod61.4.993
31. Roby KF, Terranova PF. Effects of tumor necrosis factor-alpha in vitro on steroidogenesis of healthy and atretic follicles of the rat: theca as a target. Endocrinology (1990) 126:2711–8. doi: 10.1210/endo-126-5-2711
32. Spicer LJ. Tumor necrosis factor-alpha (TNF-alpha) inhibits steroidogenesis of bovine ovarian granulosa and thecal cells in vitro. Involvement of TNF-alpha receptors. Endocrine (1998) 8:109–15. doi: 10.1385/ENDO:8:2:109
33. Cui LL, Yang G, Pan J, Zhang C. Tumor necrosis factor α knockout increases fertility of mice. Theriogenology (2011) 75:867–76. doi: 10.1016/j.theriogenology.2010.10.029
34. Ma CH, Yan LY, Qiao J, Sha W, Li L, Chen Y, et al. Effects of tumor necrosis factor-alpha on porcine oocyte meiosis progression, spindle organization, and chromosome alignment. Fertility sterility (2010) 93:920–6. doi: 10.1016/j.fertnstert.2009.01.131
35. Basini G, Baratta M, Bussolati S, Tamanini C. Interleukin-1 beta fragment (163-171) modulates bovine granulosa cell proliferation in vitro: dependence on size of follicle. J Reprod Immunol (1998) 37:139–53. doi: 10.1016/S0165-0378(97)00077-6
36. Chun SY, Eisenhauer KM, Kubo M, Hsueh AJ. Interleukin-1 beta suppresses apoptosis in rat ovarian follicles by increasing nitric oxide production. Endocrinology (1995) 136:3120–7. doi: 10.1210/endo.136.7.7540548
37. Uri-Belapolsky S, Shaish A, Eliyahu E, Grossman H, Levi M, Chuderland D, et al. Interleukin-1 deficiency prolongs ovarian lifespan in mice. Proc Natl Acad Sci United States America (2014) 111:12492–7. doi: 10.1073/pnas.1323955111
38. Popovic M, Sartorius G, Christ-Crain M. Chronic low-grade inflammation in polycystic ovary syndrome: is there a (patho)-physiological role for interleukin-1? Semin immunopathology (2019) 41:447–59. doi: 10.1007/s00281-019-00737-4
39. Kasson BG, Gorospe WC. Effects of interleukins 1, 2 and 3 on follicle-stimulating hormone-induced differentiation of rat granulosa cells. Mol Cell Endocrinol (1989) 62:103–11. doi: 10.1016/0303-7207(89)90118-4
40. Machelon V, Nome F, Salesse R. Comparative IL-6 effects on FSH and hCG-induced functions in porcine granulosa cell cultures. Cell Mol Biol (Noisy-le-Grand France) (1994) 40:373–80.
41. Tamura K, Kawaguchi T, Kogo H. Interleukin-6 inhibits the expression of luteinizing hormone receptor mRNA during the maturation of cultured rat granulosa cells. J Endocrinol (2001) 170:121–7. doi: 10.1677/joe.0.1700121
42. Gorospe WC, Hughes FM Jr., Spangelo BL. Interleukin-6: effects on and production by rat granulosa cells in vitro. Endocrinology (1992) 130:1750–2. doi: 10.1210/endo.130.3.1537322
43. Alpizar E, Spicer LJ. Effects of interleukin-6 on proliferation and follicle-stimulating hormone-induced estradiol production by bovine granulosa cells in vitro: dependence on size of follicle. Biol Reprod (1994) 50:38–43. doi: 10.1095/biolreprod50.1.38
44. Imai F, Kishi H, Nakao K, Nishimura T, Minegishi T. IL-6 up-regulates the expression of rat LH receptors during granulosa cell differentiation. Endocrinology (2014) 155:1436–44. doi: 10.1210/en.2013-1821
45. Briley SM, Jasti S, McCracken JM, Hornick JE, Fegley B, Pritchard MT, et al. Reproductive age-associated fibrosis in the stroma of the mammalian ovary. Reprod (Cambridge England) (2016) 152:245–60. doi: 10.1530/REP-16-0129
46. Lliberos C, Liew SH, Zareie P, La Gruta NL, Mansell A, Hutt K. Evaluation of inflammation and follicle depletion during ovarian ageing in mice. Sci Rep (2021) 11:278. doi: 10.1038/s41598-020-79488-4
47. Babayev E, Duncan FE. Age-associated changes in cumulus cells and follicular fluid: the local oocyte microenvironment as a determinant of gamete quality. Biol Reprod (2022) 106:351–65. doi: 10.1093/biolre/ioab241
48. Dumesic DA, Meldrum DR, Katz-Jaffe MG, Krisher RL, Schoolcraft WB. Oocyte environment: follicular fluid and cumulus cells are critical for oocyte health. Fertility sterility (2015) 103:303–16. doi: 10.1016/j.fertnstert.2014.11.015
49. Bedaiwy MA, Elnashar SA, Goldberg JM, Sharma R, Mascha EJ, Arrigain S, et al. Effect of follicular fluid oxidative stress parameters on intracytoplasmic sperm injection outcome. Gynecological endocrinology: Off J Int Soc Gynecological Endocrinol (2012) 28:51–5. doi: 10.3109/09513590.2011.579652
50. Palini S, Benedetti S, Tagliamonte MC, De Stefani S, Primiterra M, Polli V, et al. Influence of ovarian stimulation for IVF/ICSI on the antioxidant defence system and relationship to outcome. Reprod biomedicine Online (2014) 29:65–71. doi: 10.1016/j.rbmo.2014.03.010
51. Leroy J, Meulders B, Moorkens K, Xhonneux I, Slootmans J, De Keersmaeker L, et al. Maternal metabolic health and fertility: we should not only care about but also for the oocyte! Reproduction fertility Dev (2022) 35:1–18. doi: 10.1071/RD22204
52. Teede H, Misso M, Tassone EC, Dewailly D, Ng EH, Azziz R, et al. Anti-müllerian hormone in PCOS: A review informing international guidelines. Trends Endocrinol metabolism: TEM (2019) 30:467–78. doi: 10.1016/j.tem.2019.04.006
53. Rotterdam ESHRE/ASRM-Sponsored PCOS Consensus Workshop Group. Revised 2003 consensus on diagnostic criteria and long-term health risks related to polycystic ovary syndrome. Fertility sterility (2004) 81:19–25. doi: 10.1016/j.fertnstert.2003.10.004
54. Singh S, Pal N, Shubham S, Sarma DK, Verma V, Marotta F, et al. Polycystic ovary syndrome: etiology, current management, and future therapeutics. J Clin Med (2023) 12:1454. doi: 10.3390/jcm12041454
55. Barrea L, Marzullo P, Muscogiuri G, Di Somma C, Scacchi M, Orio F, et al. Source and amount of carbohydrate in the diet and inflammation in women with polycystic ovary syndrome. Nutr Res Rev (2018) 31:291–301. doi: 10.1017/S0954422418000136
56. Nikbakht R, Mohammadjafari R, Rajabalipour M, Moghadam MT. Evaluation of oocyte quality in Polycystic ovary syndrome patients undergoing ART cycles. Fertility Res Pract (2021) 7:2. doi: 10.1186/s40738-020-00094-z
57. Repaci A, Gambineri A, Pasquali R. The role of low-grade inflammation in the polycystic ovary syndrome. Mol Cell Endocrinol (2011) 335:30–41. doi: 10.1016/j.mce.2010.08.002
58. Amato G, Conte M, Mazziotti G, Lalli E, Vitolo G, Tucker AT, et al. Serum and follicular fluid cytokines in polycystic ovary syndrome during stimulated cycles. Obstetrics gynecology (2003) 101:1177–82. doi: 10.1016/s0029-7844(03)00233-3
59. Gallinelli A, Ciaccio I, Giannella L, Salvatori M, Marsella T, Volpe A. Correlations between concentrations of interleukin-12 and interleukin-13 and lymphocyte subsets in the follicular fluid of women with and without polycystic ovary syndrome. Fertility sterility (2003) 79:1365–72. doi: 10.1016/S0015-0282(03)00344-3
60. Artimani T, Karimi J, Mehdizadeh M, Yavangi M, Khanlarzadeh E, Ghorbani M, et al. Evaluation of pro-oxidant-antioxidant balance (PAB) and its association with inflammatory cytokines in polycystic ovary syndrome (PCOS). Gynecological endocrinology: Off J Int Soc Gynecological Endocrinol (2018) 34:148–52. doi: 10.1080/09513590.2017.1371691
61. Xiong YL, Liang XY, Yang X, Li Y, Wei LN. Low-grade chronic inflammation in the peripheral blood and ovaries of women with polycystic ovarian syndrome. Eur J obstetrics gynecology Reprod Biol (2011) 159:148–50. doi: 10.1016/j.ejogrb.2011.07.012
62. Qiao J, Feng HL. Extra- and intra-ovarian factors in polycystic ovary syndrome: impact on oocyte maturation and embryo developmental competence. Hum Reprod Update (2011) 17:17–33. doi: 10.1093/humupd/dmq032
63. Grodstein F, Goldman MB, Cramer DW. Body mass index and ovulatory infertility. Epidemiol (Cambridge Mass) (1994) 5:247–50. doi: 10.1097/00001648-199403000-00016
64. Luke B, Brown MB, Stern JE, Missmer SA, Fujimoto VY, Leach R. Female obesity adversely affects assisted reproductive technology (ART) pregnancy and live birth rates. Hum Reprod (Oxford England) (2011) 26:245–52. doi: 10.1093/humrep/deq306
65. Snider AP, Wood JR. Obesity induces ovarian inflammation and reduces oocyte quality. Reprod (Cambridge England) (2019) 158:R79–r90. doi: 10.1530/REP-18-0583
66. Hou YJ, Zhu CC, Duan X, Liu HL, Wang Q, Sun SC. Both diet and gene mutation induced obesity affect oocyte quality in mice. Sci Rep (2016) 6:18858. doi: 10.1038/srep18858
67. Wang H, Cheng Q, Li X, Hu F, Han L, Zhang H, et al. Loss of TIGAR induces oxidative stress and meiotic defects in oocytes from obese mice. Mol Cell proteomics: MCP (2018) 17:1354–64. doi: 10.1074/mcp.RA118.000620
68. Luzzo KM, Wang Q, Purcell SH, Chi M, Jimenez PT, Grindler N, et al. High fat diet induced developmental defects in the mouse: oocyte meiotic aneuploidy and fetal growth retardation/brain defects. PloS One (2012) 7:e49217. doi: 10.1371/journal.pone.0049217
69. Zhang L, Han L, Ma R, Hou X, Yu Y, Sun S, et al. Sirt3 prevents maternal obesity-associated oxidative stress and meiotic defects in mouse oocytes. Cell Cycle (Georgetown Tex) (2015) 14:2959–68. doi: 10.1080/15384101.2015.1026517
70. Dumesic DA, Padmanabhan V, Abbott DH. Polycystic ovary syndrome and oocyte developmental competence. Obstetrical gynecological survey (2008) 63:39–48. doi: 10.1097/OGX.0b013e31815e85fc
71. Palomba S, Daolio J, La Sala GB. Oocyte competence in women with polycystic ovary syndrome. Trends Endocrinol metabolism: TEM (2017) 28:186–98. doi: 10.1016/j.tem.2016.11.008
72. Jeanes YM, Reeves S. Metabolic consequences of obesity and insulin resistance in polycystic ovary syndrome: diagnostic and methodological challenges. Nutr Res Rev (2017) 30:97–105. doi: 10.1017/S0954422416000287
73. Ehrmann DA. Polycystic ovary syndrome. New Engl J Med (2005) 352:1223–36. doi: 10.1056/NEJMra041536
74. Yaribeygi H, Farrokhi FR, Butler AE, Sahebkar A. Insulin resistance: Review of the underlying molecular mechanisms. J Cell Physiol (2019) 234:8152–61. doi: 10.1002/jcp.27603
75. Cortón M, Botella-Carretero JI, Benguría A, Villuendas G, Zaballos A, San Millán JL, et al. Differential gene expression profile in omental adipose tissue in women with polycystic ovary syndrome. J Clin Endocrinol Metab (2007) 92:328–37. doi: 10.1210/jc.2006-1665
76. Xu XF, De Pergola G, Björntorp P. Testosterone increases lipolysis and the number of beta-adrenoceptors in male rat adipocytes. Endocrinology (1991) 128:379–82. doi: 10.1210/endo-128-1-379
77. Niu Z, Lin N, Gu R, Sun Y, Feng Y. Associations between insulin resistance, free fatty acids, and oocyte quality in polycystic ovary syndrome during in vitro fertilization. J Clin Endocrinol Metab (2014) 99:E2269–2276. doi: 10.1210/jc.2013-3942
78. Zhou F, Shi LB, Zhang SY. Ovarian fibrosis: A phenomenon of concern. Chin Med J (2017) 130:365–71. doi: 10.4103/0366-6999.198931
79. Tal R, Seifer DB, Shohat-Tal A, Grazi RV, Malter HE. Transforming growth factor-β1 and its receptor soluble endoglin are altered in polycystic ovary syndrome during controlled ovarian stimulation. Fertility sterility (2013) 100:538–43. doi: 10.1016/j.fertnstert.2013.04.022
80. Liu M, Gao J, Zhang Y, Li P, Wang H, Ren X, et al. Serum levels of TSP-1, NF-κB and TGF-β1 in polycystic ovarian syndrome (PCOS) patients in northern China suggest PCOS is associated with chronic inflammation. Clin Endocrinol (2015) 83:913–22. doi: 10.1111/cen.12951
81. Fu X, Shi L, Liu P, Jiao Y, Guo S, Chen Q, et al. Expression and clinical significance of HIF-1α in follicular fluid and granulosa cells in infertile PCOS patients. Reprod Sci (Thousand Oaks Calif) (2023) 30:2263–74. doi: 10.1007/s43032-022-01135-2
82. Zhang X, Zhang C, Shen S, Xia Y, Yi L, Gao Q, et al. Dehydroepiandrosterone induces ovarian and uterine hyperfibrosis in female rats. Hum Reprod (Oxford England) (2013) 28:3074–85. doi: 10.1093/humrep/det341
83. Takahashi N, Harada M, Hirota Y, Nose E, Azhary JM, Koike H, et al. Activation of endoplasmic reticulum stress in granulosa cells from patients with polycystic ovary syndrome contributes to ovarian fibrosis. Sci Rep (2017) 7:10824. doi: 10.1038/s41598-017-11252-7
84. Corachán A, Pellicer N, Pellicer A, Ferrero H. Novel therapeutic targets to improve IVF outcomes in endometriosis patients: a review and future prospects. Hum Reprod Update (2021) 27:923–72. doi: 10.1093/humupd/dmab014
85. Bulun SE, Yilmaz BD, Sison C, Miyazaki K, Bernardi L, Liu S, et al. Endometriosis. Endocrine Rev (2019) 40:1048–79. doi: 10.1210/er.2018-00242
86. Maneschi F, Marasá L, Incandela S, Mazzarese M, Zupi E. Ovarian cortex surrounding benign neoplasms: a histologic study. Am J obstetrics gynecology (1993) 169:388–93. doi: 10.1016/0002-9378(93)90093-X
87. Hamdan M, Omar SZ, Dunselman G, Cheong Y. Influence of endometriosis on assisted reproductive technology outcomes: a systematic review and meta-analysis. Obstetrics gynecology (2015) 125:79–88. doi: 10.1097/AOG.0000000000000592
88. Coccia ME, Rizzello F, Barone S, Pinelli S, Rapalini E, Parri C, et al. Is there a critical endometrioma size associated with reduced ovarian responsiveness in assisted reproduction techniques? Reprod biomedicine Online (2014) 29:259–66. doi: 10.1016/j.rbmo.2014.04.019
89. Bonavina G, Taylor HS. Endometriosis-associated infertility: From pathophysiology to tailored treatment. Front Endocrinol (2022) 13:1020827. doi: 10.3389/fendo.2022.1020827
90. Wu G, Bersinger NA, Mueller MD, von Wolff M. Intrafollicular inflammatory cytokines but not steroid hormone concentrations are increased in naturally matured follicles of women with proven endometriosis. J assisted Reprod Genet (2017) 34:357–64. doi: 10.1007/s10815-016-0865-3
91. Sanchez AM, Vanni VS, Bartiromo L, Papaleo E, Zilberberg E, Candiani M, et al. Is the oocyte quality affected by endometriosis? A review of the literature. J Ovarian Res (2017) 10:43. doi: 10.1186/s13048-017-0341-4
92. Yland J, Carvalho LFP, Beste M, Bailey A, Thomas C, Abrão MS, et al. Endometrioma, the follicular fluid inflammatory network and its association with oocyte and embryo characteristics. Reprod biomedicine Online (2020) 40:399–408. doi: 10.1016/j.rbmo.2019.12.005
93. Sanchez AM, Papaleo E, Corti L, Santambrogio P, Levi S, Viganò P, et al. Iron availability is increased in individual human ovarian follicles in close proximity to an endometrioma compared with distal ones. Hum Reprod (Oxford England) (2014) 29:577–83. doi: 10.1093/humrep/det466
94. Ng SW, Norwitz SG, Taylor HS, Norwitz ER. Endometriosis: the role of iron overload and ferroptosis. Reprod Sci (Thousand Oaks Calif) (2020) 27:1383–90. doi: 10.1007/s43032-020-00164-z
95. Dixon SJ, Lemberg KM, Lamprecht MR, Skouta R, Zaitsev EM, Gleason CE, et al. Ferroptosis: an iron-dependent form of nonapoptotic cell death. Cell (2012) 149:1060–72. doi: 10.1016/j.cell.2012.03.042
96. Doll S, Proneth B, Tyurina YY, Panzilius E, Kobayashi S, Ingold I, et al. ACSL4 dictates ferroptosis sensitivity by shaping cellular lipid composition. Nat Chem Biol (2017) 13:91–8. doi: 10.1038/nchembio.2239
97. Kagan VE, Mao G, Qu F, Angeli JP, Doll S, Croix CS, et al. Oxidized arachidonic and adrenic PEs navigate cells to ferroptosis. Nat Chem Biol (2017) 13:81–90. doi: 10.1038/nchembio.2238
98. Ni Z, Li Y, Song D, Ding J, Mei S, Sun S, et al. Iron-overloaded follicular fluid increases the risk of endometriosis-related infertility by triggering granulosa cell ferroptosis and oocyte dysmaturity. Cell Death Dis (2022) 13:579. doi: 10.1038/s41419-022-05037-8
99. Garcia Garcia JM, Vannuzzi V, Donati C, Bernacchioni C, Bruni P, Petraglia F. Endometriosis: cellular and molecular mechanisms leading to fibrosis. Reprod Sci (Thousand Oaks Calif) (2023) 30:1453–61. doi: 10.1007/s43032-022-01083-x
100. Matsuzaki S, Schubert B. Oxidative stress status in normal ovarian cortex surrounding ovarian endometriosis. Fertility sterility (2010) 93:2431–2. doi: 10.1016/j.fertnstert.2009.08.068
101. Kitajima M, Defrère S, Dolmans MM, Colette S, Squifflet J, Van Langendonckt A, et al. Endometriomas as a possible cause of reduced ovarian reserve in women with endometriosis. Fertility sterility (2011) 96:685–91. doi: 10.1016/j.fertnstert.2011.06.064
102. Kitajima M, Dolmans MM, Donnez O, Masuzaki H, Soares M, Donnez J. Enhanced follicular recruitment and atresia in cortex derived from ovaries with endometriomas. Fertility sterility (2014) 101:1031–7. doi: 10.1016/j.fertnstert.2013.12.049
103. Shi LB, Zhou F, Zhu HY, Huang D, Jin XY, Li C, et al. Transforming growth factor beta1 from endometriomas promotes fibrosis in surrounding ovarian tissues via Smad2/3 signaling. Biol Reprod (2017) 97:873–82. doi: 10.1093/biolre/iox140
104. Xia L, Shen Y, Liu S, Du J. Iron overload triggering ECM-mediated Hippo/YAP pathway in follicle development: a hypothetical model endowed with therapeutic implications. Front Endocrinol (2023) 14:1174817. doi: 10.3389/fendo.2023.1174817
105. Pellicer A, Oliveira N, Ruiz A, Remohí J, Simón C. Exploring the mechanism(s) of endometriosis-related infertility: an analysis of embryo development and implantation in assisted reproduction. Hum Reprod (Oxford England) (1995) 10 Suppl 2:91–7. doi: 10.1093/humrep/10.suppl_2.91
106. Simón C, Gutiérrez A, Vidal A, de los Santos MJ, Tarín JJ, Remohí J, et al. Outcome of patients with endometriosis in assisted reproduction: results from in-vitro fertilization and oocyte donation. Hum Reprod (Oxford England) (1994) 9:725–9. doi: 10.1093/oxfordjournals.humrep.a138578
107. Goud PT, Goud AP, Joshi N, Puscheck E, Diamond MP, Abu-Soud HM. Dynamics of nitric oxide, altered follicular microenvironment, and oocyte quality in women with endometriosis. Fertility sterility (2014) 102:151–159.e155. doi: 10.1016/j.fertnstert.2014.03.053
108. Xu B, Guo N, Zhang XM, Shi W, Tong XH, Iqbal F, et al. Oocyte quality is decreased in women with minimal or mild endometriosis. Sci Rep (2015) 5:10779. doi: 10.1038/srep10779
109. Younis JS. Is oocyte quality impaired in cases with ovarian endometriosis? A second look into the clinical setting. Front Endocrinol (2022) 13:921032. doi: 10.3389/fendo.2022.921032
110. O’Connor KA, Holman DJ, Wood JW. Declining fecundity and ovarian ageing in natural fertility populations. Maturitas (1998) 30:127–36. doi: 10.1016/S0378-5122(98)00068-1
111. Park SU, Walsh L, Berkowitz KM. Mechanisms of ovarian aging. Reprod (Cambridge England) (2021) 162:R19–r33. doi: 10.1530/REP-21-0022
112. Rodichkina V, Kvetnoy I, Polyakova V, Arutjunyan A, Nasyrov R, Ivanov D. Inflammaging of female reproductive system: A molecular landscape. Curr Aging Sci (2021) 14:10–8. doi: 10.2174/1874609813666200929112624
113. Luke B, Brown MB, Wantman E, Lederman A, Gibbons W, Schattman GL, et al. Cumulative birth rates with linked assisted reproductive technology cycles. New Engl J Med (2012) 366:2483–91. doi: 10.1056/NEJMoa1110238
114. Franceschi C, Campisi J. Chronic inflammation (inflammaging) and its potential contribution to age-associated diseases. journals gerontology Ser A Biol Sci Med Sci (2014) 69 Suppl 1:S4–9. doi: 10.1093/gerona/glu057
115. Goldberg EL, Dixit VD. Drivers of age-related inflammation and strategies for healthspan extension. Immunol Rev (2015) 265:63–74. doi: 10.1111/imr.12295
116. Pellicer A, Garrido N, Albert C, Navarro J, Remohí J, Simón C. Cytokines in older patients undergoing in vitro fertilization: the relationship to the response to controlled ovarian hyperstimulation. J assisted Reprod Genet (1999) 16:247–52. doi: 10.1023/A:1020363312252
117. Zhang Z, Schlamp F, Huang L, Clark H, Brayboy L. Inflammaging is associated with shifted macrophage ontogeny and polarization in the aging mouse ovary. Reprod (Cambridge England) (2020) 159:325–37. doi: 10.1530/REP-19-0330
118. Yuan S, Wen J, Cheng J, Shen W, Zhou S, Yan W, et al. Age-associated up-regulation of EGR1 promotes granulosa cell apoptosis during follicle atresia in mice through the NF-κB pathway. Cell Cycle (Georgetown Tex) (2016) 15:2895–905. doi: 10.1080/15384101.2016.1208873
119. Tatone C, Amicarelli F, Carbone MC, Monteleone P, Caserta D, Marci R, et al. Cellular and molecular aspects of ovarian follicle ageing. Hum Reprod Update (2008) 14:131–42. doi: 10.1093/humupd/dmm048
120. Shen L, Chen Y, Cheng J, Yuan S, Zhou S, Yan W, et al. CCL5 secreted by senescent theca-interstitial cells inhibits preantral follicular development via granulosa cellular apoptosis. J Cell Physiol (2019) 234:22554–64. doi: 10.1002/jcp.28819
121. Orisaka M, Jiang JY, Orisaka S, Kotsuji F, Tsang BK. Growth differentiation factor 9 promotes rat preantral follicle growth by up-regulating follicular androgen biosynthesis. Endocrinology (2009) 150:2740–8. doi: 10.1210/en.2008-1536
122. Orisaka M, Tajima K, Tsang BK, Kotsuji F. Oocyte-granulosa-theca cell interactions during preantral follicular development. J Ovarian Res (2009) 2:9. doi: 10.1186/1757-2215-2-9
123. Amargant F, Manuel SL, Tu Q, Parkes WS, Rivas F, Zhou LT, et al. Ovarian stiffness increases with age in the mammalian ovary and depends on collagen and hyaluronan matrices. Aging Cell (2020) 19:e13259. doi: 10.1111/acel.13259
124. Molinari E, Bar H, Pyle AM, Patrizio P. Transcriptome analysis of human cumulus cells reveals hypoxia as the main determinant of follicular senescence. Mol Hum Reprod (2016) 22:866–76. doi: 10.1093/molehr/gaw038
125. Umehara T, Winstanley YE, Andreas E, Morimoto A, Williams EJ, Smith KM, et al. Female reproductive life span is extended by targeted removal of fibrotic collagen from the mouse ovary. Sci Adv (2022) 8:eabn4564. doi: 10.1126/sciadv.abn4564
126. Secomandi L, Borghesan M, Velarde M, Demaria M. The role of cellular senescence in female reproductive aging and the potential for senotherapeutic interventions. Hum Reprod Update (2022) 28:172–89. doi: 10.1093/humupd/dmab038
Keywords: aging, endometriosis, follicular microenvironment, inflammation, ovarian dysfunction, polycystic ovarian syndrome
Citation: Orisaka M, Mizutani T, Miyazaki Y, Shirafuji A, Tamamura C, Fujita M, Tsuyoshi H and Yoshida Y (2023) Chronic low-grade inflammation and ovarian dysfunction in women with polycystic ovarian syndrome, endometriosis, and aging. Front. Endocrinol. 14:1324429. doi: 10.3389/fendo.2023.1324429
Received: 19 October 2023; Accepted: 01 December 2023;
Published: 13 December 2023.
Edited by:
Takashi Minegishi, Gunma University, JapanReviewed by:
Miyuki Harada, The University of Tokyo, JapanTakashi Yazawa, Asahikawa Medical University, Japan
Akira Iwase, Gunma University, Japan
Fardin Amidi, Tehran University of Medical Sciences, Iran
Copyright © 2023 Orisaka, Mizutani, Miyazaki, Shirafuji, Tamamura, Fujita, Tsuyoshi and Yoshida. This is an open-access article distributed under the terms of the Creative Commons Attribution License (CC BY). The use, distribution or reproduction in other forums is permitted, provided the original author(s) and the copyright owner(s) are credited and that the original publication in this journal is cited, in accordance with accepted academic practice. No use, distribution or reproduction is permitted which does not comply with these terms.
*Correspondence: Makoto Orisaka, b3Jpc2FrYUB1LWZ1a3VpLmFjLmpw