- Department of Rehabilitation Medicine, West China Hospital, Sichuan University, Chengdu, Sichuan, China
Bone metabolism is the basis for maintaining the normal physiological state of bone, and imbalance of bone metabolism can lead to a series of metabolic bone diseases. As a member of the IL-6 family, IL-11 acts primarily through the classical signaling pathway IL-11/Receptors, IL-11 (IL-11R)/Glycoprotein 130 (gp130). The regulatory role of IL-11 in bone metabolism has been found earlier, but mainly focuses on the effects on osteogenesis and osteoclasis. In recent years, more studies have focused on IL-11’s roles and related mechanisms in different bone metabolism activities. IL-11 regulates osteoblasts, osteoclasts, BM stromal cells, adipose tissue-derived mesenchymal stem cells, and chondrocytes. It’s involved in bone homeostasis, including osteogenesis, osteolysis, bone marrow (BM) hematopoiesis, BM adipogenesis, and bone metastasis. This review exams IL-11’s role in pathology and bone tissue, the cytokines and pathways that regulate IL-11 expression, and the feedback regulations of these pathways.
1 Introduction
Bone is in constant renewal and is a relatively dynamic organ compared with other organs (1). Bone metabolism is the basis for maintaining the normal physiological state of bone, mainly manifested as the coordination of bone resorption and bone formation (2). Imbalance of bone metabolism can lead to a series of metabolic bone diseases (3). The metabolism and remodeling of the bone is around 100% in the first years of life and is reduced to around 18% in adulthood, undergoes a progressive decrease, giving rise to conditions of osteoporosis. Osteoporosis, with the aging of the global population, will represent a global health problem in the future, and in this sense knowing all the mechanisms that contribute to its development is of fundamental importance for implementing preventive strategies. Bone cells interact with immune cells under physiological and pathological conditions (4). Therefore, immune-related cytokines play an important role in regulating bone metabolism. The interleukin family is an important part of pro-inflammatory cytokines. At present, IL-1, IL-4, IL-6, IL-17, IL-18 are the most explored interleukin factors in bone metabolism (5).
The pleotropic cytokine interleukin-11 (IL-11) exists mainly in a tetra helical structure and exerts its effects on hematopoietic cells. Human IL-11 cDNA encodes a 19-kDa protein containing 178 amino acids (6). IL-11, together with IL-27, IL-6, IL-31, leukemia inhibitory factor, oncostatin-M, cardiotrophin-1, cardiotrophin-like cytokine factor 1, and ciliary neurotrophic factor, belongs to the family of IL-6 cytokine that acts through the glycoprotein 130 (gp130) receptor subunit. IL-11 was initially detected in a primate bone marrow (BM) cell line and promotes plasmacytoma cell proliferation (7). It is expressed in several tissues and cells, including fibroblasts, mesenchymal stem cells, epithelial cells, hematopoietic cells, central nervous system neurons, osteoclasts, osteoblasts, adipocytes, synovial cells, chondrocytes, testis, and the gastrointestinal tract (8–12). IL-11 participates in several physiological processes, including bone remodeling, hematopoiesis, carcinogenesis, fertility (13), and nervous system pathologies (14).
IL-11Rα1 as well as IL-11Rα2 receptors showing transcytotic activity are localized in the basolateral and apical membranes of polarized epithelial cells. IL-11Rα1 shows expression in the brain, thymus, lungs, heart, spleen, kidneys, bladder, muscles, large and small intestines, BM, salivary glands, ovaries, uterus, and testes (15), and IL11Rα2 shows expression in the thymus, lymph nodes, and testes (16). After binding to IL-11R, a nonsignaling membrane receptor, IL-11 induces heterodimer formation in the signaling receptor gp130 (17–19) and stimulates the mitogen-activated protein kinase (MAPK) cascade and the Janus kinase/signal sensor and activator of transcription (JAK/STAT) pathway (20, 21). The IL-11/IL-11R complex formation differs from that of other cytokines in terms of thermodynamic and structural mechanisms (22). After binding to IL-11R1, IL-11 is transported through transcytosis and then secreted into the extracellular space (23). IL-11 is also implicated in a trans-signaling pathway as it binds to the soluble form of IL-11R (sIL-11R) and activate cells not expressing IL-11R (24–27). However, sIL-11R may antagonize IL-11 activity based on the amount of gp130 molecules in cells expressing transmembrane IL-11R (28).
As a member of the IL-6 family, the regulatory role of IL-11 in bone metabolism has been found earlier, but mainly focuses on the effects on osteogenesis and osteoclasis. In recent years, more studies have focused on IL-11 and its role and related mechanisms in different bone metabolism activities, and some studies have suggested that IL-11 can be used as a possible target for the treatment of bone diseases. This article will briefly summarize the role of IL-11 in inflammatory diseases, detail the regulatory role and related mechanisms of IL-11 in bone metabolism, and provide new ideas for the studies and treatment of metabolic bone diseases.
2 The regulatory role of IL-11 in inflammation
As a downstream gene of profibrotic factors, particularly TGF-β1 (29), IL-11 upregulation increases fibroblast proliferation and transformation (30), thereby leading to fibrosis and failure of tissues and organs (31–33). IL-11 is produced by lung stromal cells in response to virus infection and other factors. Furthermore, IL-11 upregulation in mouse airway cells triggers lymphocytic inflammation, which is characterized by fibroblast accumulation and increased collagen I and III expression (34). IL-11 modulates airway remodeling in individuals with asthma by promoting airway fibrosis and inhibiting alveolar development (35). IL-11Rα and IL-11 show a high expression in stellate as well as parenchymal cells in the pancreas and liver and induce inflammation, fibrosis, steatosis, and organ failure through secretion in an autocrine or paracrine manner (36–39), his is an important pathogenetic mechanism in alcoholic hepatitis and acute pancreatitis (40). IL-11 blocks gastric acid secretion in the gastrointestinal tract (41), leading to chronic gastroenteritis (42, 43). Hence, IL-11 could serve as a potential therapeutic target for inhibiting the TGF-β1/IL-11/MEK/ERK/mTOR and TGF-β1/Smad3/HIF-1α/IL-11 signaling pathways to prevent senescence-associated organ inflammation and fibrosis (44–48). IL-11Rα knockdown or deletion can also effectively prevent these diseases (30).
IL-11 exerts anti-inflammatory effects. Treatment with IL-11 decreases the expression of pro-inflammatory mediators such as IL-1β, IFN-γ, NF-κB, IL-6, TNF-α, and nitric oxide (49); reduces IL-12 production in macrophages; and increases the expression level of IL-4 and IL-10 (50). The IL-11-mediated regulation of the anti-inflammatory effect restores Th1 and Th2 cell balance as well as alleviates T cell-mediated liver injury (51–53). IL-11 prevents IFN-γ-induced death of hepatocytes by downregulating the IFN-γ/STAT1, JAK-STAT, MAPK, PI3K/AKT, and NF-κB signaling pathways (54). IL-11 reduces acute inflammation (55–57). Moreover, although IL-11 promotes airway fibrosis, it inhibits asthma-like inflammation (58, 59), and its expression is regulated by IL-13 (60, 61).
IL-11 also participates in tumor initiation and progression by regulating epithelial cell turnover (62) (9) and the IL-11/gp130/STAT3 signaling pathway. Neoplastic cell invasion and tumor growth were reduced following the pharmacological inhibition of IL-11/STAT3 in human tumor cell xenografts and mouse gastrointestinal cancer models (63).And IL-11 can alleviate cardiac fibrosis after myocardial infarction (64) and protects hepatocytes in liver ischemia through the activation of the IL-11/gp130/STAT3 signaling pathway (65).
3 Role of IL-11 in the regulation of bone metabolism
3.1 Bone formation
IL-11 regulates osteogenesis through several pathways. IL-11 signaling by membrane-bound receptors is a critical factor that allows craniofacial bones and teeth to develop normally in mice (66). Previous studies also confirmed this finding by demonstrating that mutations in IL-11 receptors induce abnormal bone development in mice (67–69). Fracture hematoma contains a large amount of inflammatory and immunomodulatory mediators. The high levels of anti-inflammatory molecules, including IL-11, in fracture hematoma induce extracellular matrix (ECM) production, angiogenesis, and osteogenesis (70). Quantitative PCR analysis showed the highest IL-11 mRNA expression level (increase by 73-fold, p < 0.0001) at 24 h following an ulnar stress fracture, thus indicating that IL-11 plays a key role in activating tissue remodeling and stress fracture healing (71). IL-11 induces osteoblast differentiation through the MAPK, Wnt, and other signaling pathways (72–74). Differentiated osteoblasts express bone sialic acid protein (BSP), a mineralized tissue-specific protein, which is probably involved in the early stage of bone mineralization (75, 76). IL-11 can promote the transcription of BSP directly by targeting CRE1, CRE2, HOX, and FRE in the proximal promoter of the BSP gene in rats (77). The effects of IL-11 on BSP transcription may be regulated by the transcription factors c-Fos, Dlx5, CREB1, JunD, c-Jun, Msx2, Smad1, and Runx2 (78). Furthermore, IL-11 promotes the transcription of the target genes of bone morphogenetic protein (BMP) through STAT3 (79), protein kinase A, PI3K, and ERK1/2 pathways (77). Recombinant human IL-11 (rhIL-11) induces the differentiation of osteoblasts in mouse mesenchymal progenitor cells, enhances the activity of alkaline phosphatase, and upregulates mRNA expression levels of BSP, osteocalcin, and parathyroid hormone (PTH) receptor (80). Moreover, rhIL-11 alone or together with recombinant human BMP-2 induces osteoblast differentiation into progenitor cells and accelerates bone formation without impairing bone strength (81–83).
As mentioned above, IL-11 can promote the transcription and expression of osteogenic factors through classical signaling pathways, induce osteogenic differentiation of mesenchymal stem cells, and promote normal bone development, bone remodeling and fracture healing. However, the mechanisms and pathways of IL-11 in regulating osteogenesis have not been fully elucidated. The effects of IL-11 on osteogenesis under normal and pathological conditions and related mechanisms need to be further studied.
3.2 Bone resorption
BM stromal cells produce IL-11, which induces osteoclast formation (84, 85) and directly affects osteoclast precursors (86). Because osteoblasts and mature osteoclasts express the mRNA of IL-11Rα, bone-resorbing cells as well as bone-forming cells are IL-11’s potential targets (12). In vitro studies have shown that IL-11 inhibits bone formation (87), triggers osteoclast formation by inducing osteoblast-mediated osteoid degradation and affects the development or survival of osteoclast progenitor cells (88). RANKL promotes osteoclast maturation and differentiation through the RANK/RANKL/OPG pathway, resulting in increased bone resorption (89). Independent of RANKL, IL-11 activates osteoclastogenesis through the JAK1/STAT3 signaling pathway and STAT3-mediated c-Myc expression (90, 91). Also, IL-11 inhibits osteoprotegerin (OPG) production, while the interaction between IL-11 and RANKL upregulates RANKL expression (92–95). Furthermore, IL-11 secretion by bone-derived endothelial cells was found to induce osteolysis in a murine calvarial bone organ culture model (96).
IL-11 is associated with osteolytic bone diseases. In rheumatoid arthritis (RA), a chronic immunological disease with bone destruction and synovitis, an association was observed between IL-11 expression and osteoarthritis (97–102). A previous study revealed that RA patients showed significantly higher serum IL-11 expression level than the control group (103). IL-11 controls bone turnover and is involved in bone loss induced by estrogen deficiency (86). Treatment of ovariectomized mice with IL-11 antagonists significantly increased cancellous bone volume, trabecular width, and osteoblast activity and decreased the number and activity of osteoclasts (104). Postmenopausal women who have osteoporosis show a significant increase in serum IL-11 levels, which were found to be positively correlated with the levels of bone resorption markers (NTX and DPyr). Serum IL-11 levels also accurately predicted bone turnover and the outcomes of antiresorption therapy (105). An in vivo study in estrogen-deficient rats showed that bone mineral density and osteocalcin concentrations were not significantly affected by rhIL-11 (106). Consistent with this finding, Sims et al. demonstrated that estrogen deficiency in mice affected bone homeostasis independent of IL-11 (86). Therefore, further investigations are essential to clarify the mechanisms underlying IL-11’s effects on osteoporosis.
In summary, IL-11 can induce osteoclast formation through Jak/STAT pathway, MAPK/PI3K pathway, phosphorylation of Yes-associated protein 1 (YAP1) and RANKL-independent mechanism, and is associated with rheumatoid arthritis, femoral arthritis, osteoporosis and other bone diseases. However, how IL-11 balances osteogenesis and osteoclastogenesis and its mechanism of action in bone diseases need to be further explored. A diagram of the main mechanism of the role of IL-11 in bone formation and bone resorption is provided in Figure 1.
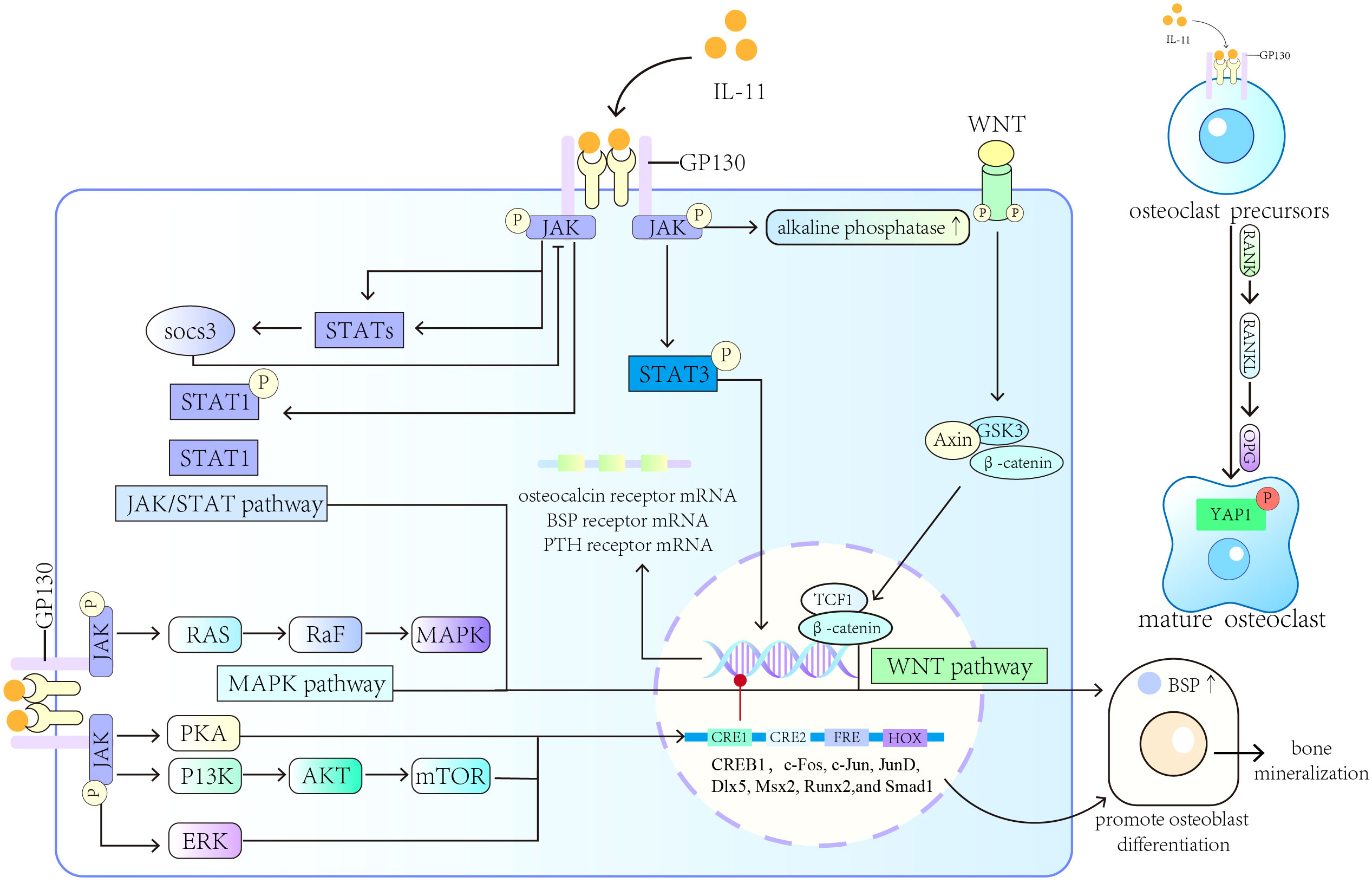
Figure 1 Roles of IL-11 in bone formation and bone resorption. IL-11 induces osteoblast differentiation and promotes the transcription of BSP through the MAPK, Wnt, and other signaling pathways. IL-11 can also induce osteoclast formation and different bone diseases.
3.3 Inhibition of adipogenesis
Both bone marrow adipocytes and osteoblasts are differentiated from bone marrow mesenchymal stem cells, expansion of bone marrow adipose tissue volume results in reduced osteogenic differentiation (107), the pro-adipose transcription factor PPAR promotes RANKL expression (108) and osteoclast formation (109, 110), and mature bone marrow adipocytes release pro-inflammatory cytokines (111); therefore, bone marrow adipose tissue is closely related to bone metabolism and bone remodeling. Established clinical studies have also shown that there is a negative correlation between bone marrow adipose volume and bone mineral density (112), and that estrogen deficiency, glucocorticoid use, and aging can cause expansion of bone marrow adipose tissue volume as the main pathogenesis of bone metabolic diseases such as osteoporosis (113, 114).
Many studies have investigated how IL-11 influences adipogenesis. Kawashima et al. performed DNA sequencing analysis (115) to determine whether adipogenesis inhibitory factor (AGIF) was associated with IL-11; the authors found that AGIF inhibited BM-derived preadipocyte production (116, 117). IL-11 decreased fat accumulation in the BM (79, 118), probably by preventing preadipocytes to differentiate into adipocytes (119). Furthermore, the formation of white adipose tissue and BM adipose tissue was significantly increased in mice following IL-11 knockout (72). Prostaglandin F2α activates STAT1 through an autocrine negative feedback loop mediated by IL-11/gp130 to inhibit adipocyte differentiation and adipogenesis (120, 121). IL-11 activates several signaling pathways in adipose tissue, including p44/42 MAP kinase, STAT1, PI3 kinase, and STAT3; promotes the migration and proliferation of adipose-derived mesenchymal stem cells (ADSCs) and skeletal myogenic cells; and attenuates ADSC apoptosis (122–124). IL-11 also regulates gene transcription and protein synthesis in differentiated adipocytes (125).
In summary, existing studies have shown that IL-11 can reduce bone marrow adipogenesis. However, the role and regulatory mechanism of IL-11 on bone marrow adipogenesis need to be further explored.
3.4 Hematopoiesis
Together with other cytokines, rhIL-11 enhances primitive hematopoietic and lymphopoietic progenitor cell formation in the BM (126). IL-11 promotes erythroid lineage formation and stimulates the proliferation and differentiation of megakaryocytes (127). Furthermore, in young mice, IL-11 increases the count of peripheral platelets based on the administered dose (128, 129). An in vitro study revealed that IL-11 synergizes with the Flt3 ligand, which is critically involved in early hematopoiesis in mouse BM (130), and this combination enhances megakaryocyte and progenitor cell production and stimulates the differentiation and expansion of myeloid lineage (131, 132). The autocrine regulator hepatocyte growth factor from myeloid stromal cells sustains the hematopoietic microenvironment by adhering to the ECM and promotes hematopoiesis by inducing IL-11 production through stromal cells (133–135).
IL-11-mediated effects on hematopoiesis could be clinically beneficial. The cytokine binds to the endothelial IL-11Rα and promotes angiogenesis induction, VEGF (proangiogenic factor) secretion, and fibroblast migration (99). Through the activation of the JAK/STAT3 pathway, IL-11 stimulates the secretion of stem cell factor (SCF), a c-kit ligand, in BM primitive stem cells (136, 137). IL-11 also reduced TGF-β1’s ability to suppress megakaryocyte production. IL-11 together with SCF or IL-3 promoted the recovery of leukocytes, platelets, and erythrocytes in mice subjected to irradiation (138). The effects of chemotherapy, radiotherapy, and BM transplantation on thrombocytopenia are reduced by IL-11 (139–142). Furthermore, IL-11 alleviates the function of the mitochondria by decreasing the production of reactive oxygen species, altering the mitochondrial membrane potential, maintaining mitochondrial number and function, reducing BM cell apoptosis, stimulating the growth of BM damaged by radiation, and minimizing radiation-induced immune response (143). A low-dose IL-11 therapy showed low toxicity and alleviated BM failure (144).
In summary, IL-11 can promote bone marrow hematopoiesis independently or in synergy with other hematopoietic factors, and can also protect mitochondrial function, which has a certain clinical therapeutic effect. However, extensive studies on IL-11 for clinical treatment of bone diseases are not enough. It is necessary to further explore the therapeutic effect, effectiveness and safety of IL-11 in different bone diseases.
3.5 Bone metastases
IL-11 participates in bone resorption induced by malignant osteolysis and tumor growth. IL-11 enhances chondrosarcoma cell migration by activating the NF-κB and PI3K/AKT pathways and increasing IL-11Rα-associated intercellular adhesion molecule-1 expression (145). Bone-derived TGF-β also acetylates KLF5, a transcription factor, in pancreatic cancer-related bone metastasis, and following its acetylation, KLF5 activates CXCR4 to induce osteoclast differentiation, thereby leading to IL-11 secretion (146). IL-11 also influences breast cancer (BC)-related bone metastases, and its expression is upregulated through the Smad2/3 and p38 MAPK pathway activation by the TGF-β/TGF-βR axis (147). Patients with BC and showing a high IL-11 expression are prone to develop bone metastases (148, 149). Smad4 induces IL-11 secretion (150) to enhance the morphological transition of epithelial cells to fibroblast-like cells (151). IL-11 also affects tumor cells present in the bone microenvironment to promote bone resorption and the formation of osteoclasts (152). Runx2/core-binding factor β (CBFβ) inhibits the Wnt signaling pathway by inducing sclerostin secretion and is required to modulate the functions of osteoblasts and osteoclasts in metastatic BC cells. IL-11 acts as a target gene for Runx2/CBFβ in metastatic BC cells and as a target of miR-124 (153) as it mediates miR-124’s inhibitory effect in osteoclasts and BC-related bone metastasis in vivo (154).
IL-11 is an appropriate candidate for antimetastatic therapy (155). IL-11Rα also acts as a therapeutic target for bone metastasis (156, 157). Because IL-11 plays a mediating role in cancer-related bone metastasis, researchers developed a ligand-targeted peptidomimetic drug, namely BMTP-11 (bone metastasis-targeting peptidomimetic-11), which binds to IL-11Rα in the tumor vascular endothelium of castrate-resistant prostate cancer patients (158). Clinical trials demonstrated that BMTP-11 effectively treats human leukemia and lymphoma (159); moreover, as a cell surface target, it prevents the bone metastasis and growth of human prostate cancer and osteosarcoma (160). However, because of few clinical trials, the clinical application of BMTP-11 is limited, and its therapeutic effects on other cancers should be further investigated.
4 Regulators of IL-11
4.1 Bone formation
PTH and glucocorticoids modulate IL-11 expression to regulate the differentiation and apoptosis of osteoblasts (73). Recombinant human PTH (1-34) promotes osteogenesis by increasing Smad1/5 phosphorylation and ΔFosB expression to induce IL-11 gene transcription. Dexamethasone prevents PTH (1-34)-induced IL-11 gene transcription but does not affect ΔFosB expression and Smad1 phosphorylation (161–163). PTH can upregulate IL-11 expression to reverse glucocorticoid-induced osteoblast apoptosis (164, 165). Thyroid-stimulating hormone (TSH) modulates bone homeostasis by increasing the expression of IL-11 through the cAMP pathway (166).Senescence-accelerated osteoporotic SAMP6 mice showed a low IL-11 expression in BM cells in comparison with normal B6 mice of the same age (167). However, IL-11 secretion was increased significantly in human BM-derived mesenchymal stromal cells (168–170), probably through the cAMP/PKA pathway activation (171). Moreover, after BM cells isolated from normal allogeneic mice are introduced into the BM cavity of irradiated SAMP6 mice, the recipient mice showed substantial increment in IL-11 expression, bone mineral density, and trabecular bone density (172). Key transcription factors modulate the osteogenic effects of IL-11. For example, the heterodimer AP-1, i.e., activator protein 1, comprises c-Jun and c-Fos, and its reduced activity or synergy with the zinc finger protein Zac1 decreases IL-11 expression in BM stromal cells (173) and impairs bone formation in elderly people (174).
In summary, hormones, cytokines, and cell aging will affect the expression and secretion of IL-11. TSH and PTH can promote the expression of IL-11, while glucocorticoids, transcription factors (AP-1, ZAC1 protein), cell aging will inhibit the expression of IL-11 (Figure 2).
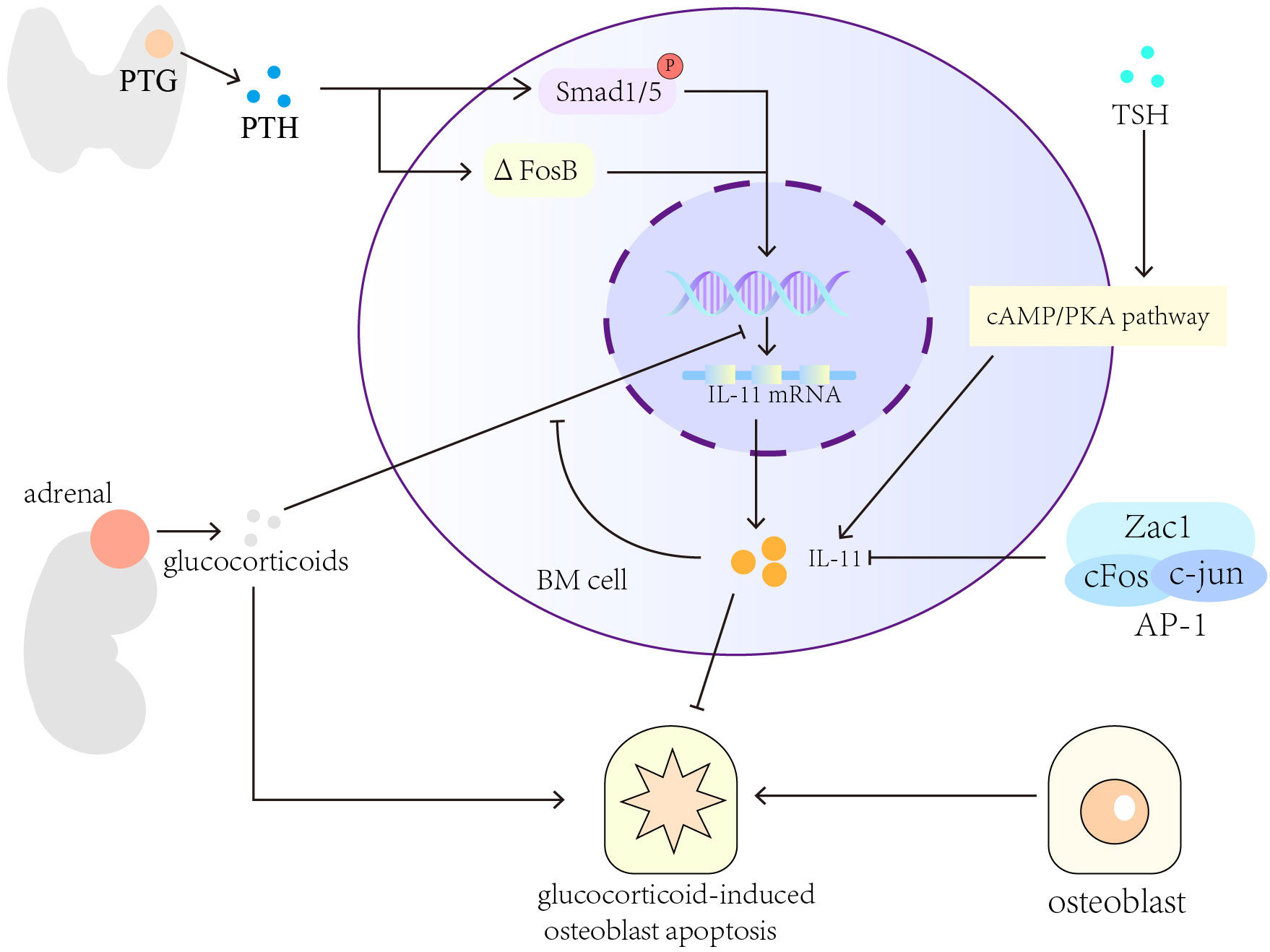
Figure 2 Cytokines and pathways that regulate the expression of IL-11 in bone formation. Hormones, for example, TSH, PTH and glucocorticoids, can regulate the expression and secretion of IL-11. Cytokines and cell aging will also affect the secretion of IL-11.
4.2 Bone resorption
Many cytokines are involved in regulating the role of IL-11 in bone resorption. IL-11 stimulates bone resorption through a mechanism involving PGE2 (175–177). Interferon-alpha downregulates IL-11 expression in human BM stromal cell cultures (178). TGF-β1 upregulates IL-11 gene expression by inducing the interaction between the transcriptional activators Runx2-c-Jun and Runx2-Smad (179). IL-11 participates in the process of bone resorption induced by lipopolysaccharides and enhances the formation of osteoclasts independent of IL-1 and TNF signaling in vivo (180, 181). Heparin enhances the formation of the STAT3-DNA complex and transactivation induced by IL-11 as well as increases RANKL and gp130 activator expression (182). Treatment with heparin for a long term leads to cancellous bone loss in rats (183). Protease-activated receptor-2 expressed in osteoblasts inhibits RANKL expression, reduces RANKL to OPG expression ratio, and prevents IL-11-induced osteoclast differentiation (184). In rheumatoid joints, TNF-α and IL-1α synergistically stimulate IL-11 production through the production of PGE2, and indomethacin inhibits this synergistic effect (185). IL-4 protects against bone resorption by inhibiting IL-11 production in rheumatoid joints (186). Fas (Apo-1/CD95/TNFRSF6) ligand (FasL), a TNF superfamily member, is involved in the pathogenetic mechanisms of autoimmune diseases, including rheumatoid arthritis. In rheumatoid arthritis, FasL affects the expression of IL-11 in fibroblast-like synovial cells (187).
In summary, several factors regulate IL-11’s osteolytic activity, including IL-1, tumor necrosis factor (TNF)-α, PTH, TSH, TGF-β, polyphosphate, and prostaglandin E2 (PGE2) (11, 12, 188–193) (Figure 3).
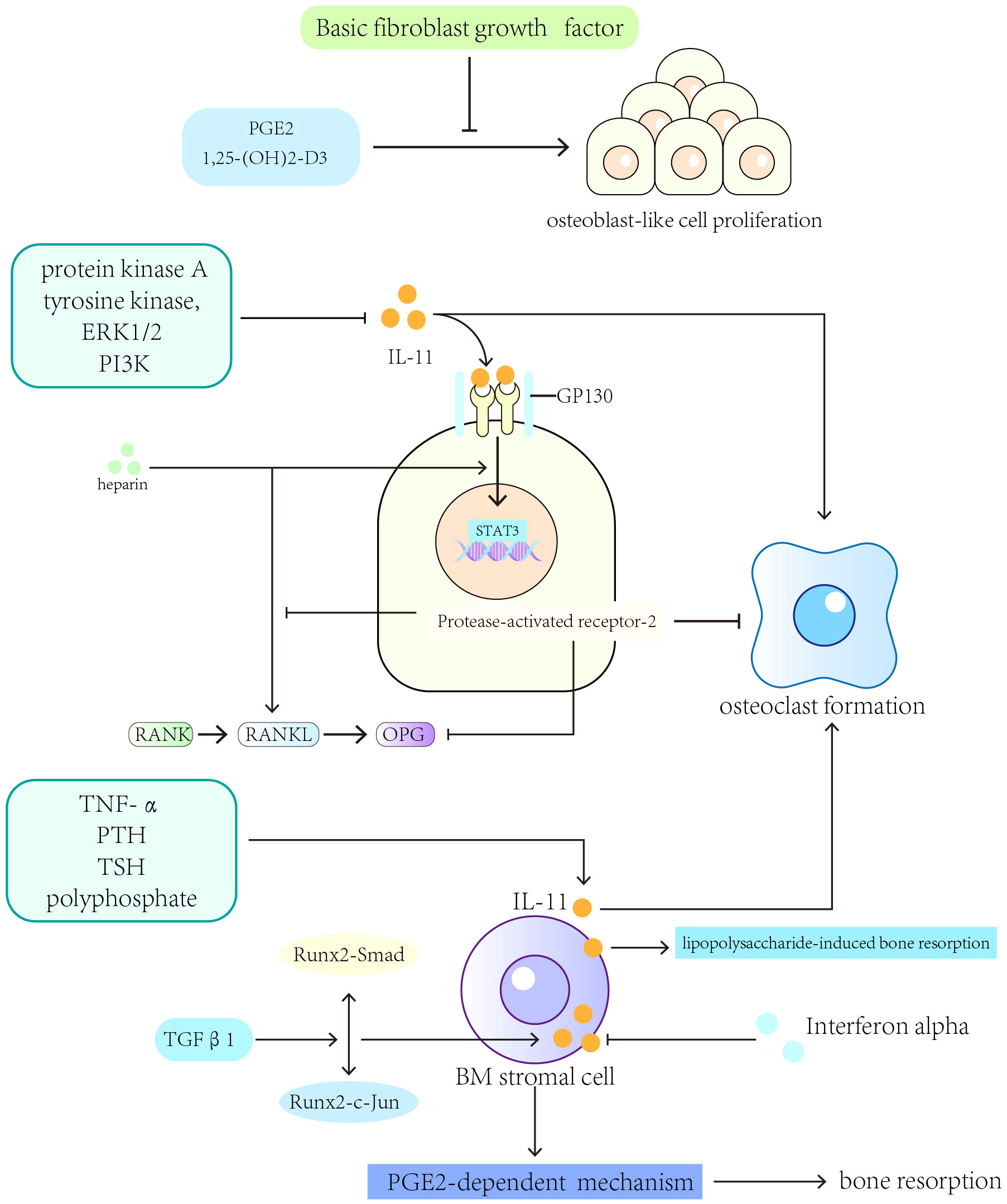
Figure 3 Cytokines and pathways that regulate the expression of IL-11 in bone resorption. IL-1, tumor necrosis factor (TNF)-α, PTH, TSH, TGF-β, polyphosphate, and prostaglandin E2 (PGE2) can regulate IL-11’s osteolytic activity.
5 Limitations and further studies
The present study reviewed studies published in English over the past 21 years on how IL-11 affects bone metabolism and the regulators and signaling pathways that influence its effects. The results were consistent, although the analyzed studies were conducted in different countries.
The present study has some limitations. First, there were inconsistencies in cell type (human vs. mouse), tissue preparation (fresh vs. frozen), cell line origin, and gene expression analysis methods across different studies. Second, no statistical tests were performed.
Because IL-11 plays a variety of roles in bone formation and resorption, future studies should investigate the mechanisms and pathways through which IL-11 regulates bone remodeling and adipose tissue formation in normal conditions, the mechanisms and pathways of regulating bone metabolism in different pathological conditions (such as fracture, different types of osteoporosis, bone tumors, bone metastasis of malignant tumors, etc.), the cytokines and related mechanisms that affect IL-11 expression, the cytokines and related mechanisms regulated by IL-11 expression, the therapeutic potential of IL-11 to treat bone and joint diseases, and its safety and side effects as drug targets. At the same time, further research needs to focus on the relationship and difference between IL-11 and other inflammatory factors in regulating bone remodeling and bone metabolism.
6 Conclusion
IL-11 regulates inflammation and fibrosis, hematopoiesis, adipogenesis, fertility, and cancer development. Bone metabolism is the basis of physiological activities of bone tissue. Imbalance or pathological state of bone metabolism can lead to a series of bone diseases. The role of IL-11 in bone metabolism has been discovered earlier, mainly focusing on the regulation of osteogenesis and osteoclasis. In recent years, more research has focused on exploring the effect of IL-11 on bone metabolism. IL-11 promotes tooth and bone development and bone remodeling after fracture, induces osteogenic gene expression in differentiating osteoblasts, and improves mitochondrial function. The osteogenic effects of IL-11 are regulated by PTH/GC, cellular senescence and mechanical stress. IL-11’s bone resorption effects are facilitated by TNF-α, IL-1, TGF-β, 1,25-(OH)2-D3, PGE2, and other factors. Furthermore, IL-11 promotes bone resorption through the JAK/STAT and MAPK/PI3K pathways, YAP1 phosphorylation, and RANKL/OPG expression regulation. IL-11 is implicated in postmenopausal osteoporosis and RA. Although IL-11 has a dual role in regulating bone formation and bone resorption, the blockade of IL-11 signaling severely affects bone development and bone homeostasis. Recent studies have focused more on the central role of IL-11 in promoting bone formation and inhibiting bone marrow adipogenesis. However, overexpression of IL-11 may be associated with bone resorption-associated bone disease. Therefore, the regulatory role of IL-11 on osteogenesis and osteoclastogenesis needs to be further explored. IL-11 exerts its effects on ADSCs to inhibit white adipose tissue production. IL-11 also supports the formation of primitive hematopoietic and lymphopoietic progenitor cells in the BM; enhances the formation of peripheral erythrocytes, leucocytes, and platelets; and stimulates the differentiation and proliferation of megakaryocytes. The hematopoietic effects of IL-11 can be used clinically to treat bone and joint diseases. This study provides a basis to determine the underlying mechanism through which IL-11 exerts its effects and the signaling pathways participating in bone remodeling and to assess the therapeutic capability of IL-11 to treat rheumatic and osteoarticular diseases.
Author contributions
YH: Conceptualization, Investigation, Writing – original draft. HG: Writing – review & editing. XG: Funding acquisition, Writing – review & editing. JL: Writing – review & editing. CB: Writing – review & editing. CH: Funding acquisition, Resources, Writing – review & editing.
Funding
The author(s) declare financial support was received for the research, authorship, and/or publication of this article. CH was supported by National Natural Science Foundation of China (Grant numbers [82272599]). XG was supported by National Outstanding Youth Science Fund Project of National Natural Science Foundation of China (Grant numbers [82102657]).
Conflict of interest
The authors declare that the research was conducted in the absence of any commercial or financial relationships that could be construed as a potential conflict of interest.
Publisher’s note
All claims expressed in this article are solely those of the authors and do not necessarily represent those of their affiliated organizations, or those of the publisher, the editors and the reviewers. Any product that may be evaluated in this article, or claim that may be made by its manufacturer, is not guaranteed or endorsed by the publisher.
References
1. Datta HK, Ng WF, Walker JA, Tuck SP, Varanasi SS. The cell biology of bone metabolism. J Clin Pathol (2008) 61(5):577–87. doi: 10.1136/Jcp.2007.048868
2. Taipaleenmäki H. Regulation of bone metabolism by micrornas. Curr Osteoporosis Rep (2018) 16(1):1–12. doi: 10.1007/S11914-018-0417-0
3. Khosla S, Hofbauer LC. Osteoporosis treatment: recent developments and ongoing challenges. Lancet Diabetes Endocrinol (2017) 5(11):898–907. doi: 10.1016/S2213-8587(17)30188-2
4. Okamoto K, Nakashima T, Shinohara M, Negishi-Koga T, Komatsu N, Terashima A, et al. Osteoimmunology: the conceptual framework unifying the immune and skeletal systems. Physiol Rev (2017) 97(4):1295–349. doi: 10.1152/Physrev.00036.2016
5. Kapoor M, Martel-Pelletier J, Lajeunesse D, Pelletier J-P, Fahmi H. Role of proinflammatory cytokines in the pathophysiology of osteoarthritis. Nat Rev Rheumatol (2011) 7(1):33–42. doi: 10.1038/Nrrheum.2010.196
6. Trepicchio WL, Dorner AJ. Interleukin-11: A gp130 cytokine. Ann N Y Acad Sci (1998) 856(1):12–21. doi: 10.1111/J.1749-6632.1998.Tb08308.X
7. Paul SR, Bennett F, Calvetti JA, Kelleher K, Wood CR, O’Hara RM, et al. Molecular cloning of A cdna encoding interleukin 11, A stromal cell-derived lymphopoietic and hematopoietic cytokine. Proc Natl Acad Sci USA (1990) 87(19):7512–6. doi: 10.1073/Pnas.87.19.7512
8. Silva WA, Covas DT, Panepucci RA, Proto-Siqueira R, Siufi JLC, Zanette DL, et al. The profile of gene expression of human marrow mesenchymal stem cells. Stem Cells (Dayton Ohio) (2003) 21(6):661–9. doi: 10.1634/Stemcells.21-6-661
9. Airapetov M, Eresko S, Ignatova P, Lebedev A, Bychkov E, Shabanov P. A brief summary regarding the roles of interleukin-11 in neurological diseases. Bioscience Trends (2022) 16(5):367–70. doi: 10.5582/Bst.2022.01331
10. Leng SX, Elias JA. Interleukin-11. Int J Biochem Cell Biol (1997) 29(8):1059–62. doi: 10.1016/S1357-2725(97)00017-4
11. Maier R, Ganu V, Lotz M. Interleukin-11, an inducible cytokine in human articular chondrocytes and synoviocytes, stimulates the production of the tissue inhibitor of metalloproteinases. J Biol Chem (1993) 268(29):21527–32. doi: 10.1016/S0021-9258(20)80573-0
12. Romas E, Udagawa N, Zhou H, Tamura T, Saito M, Taga T, et al. The role of gp130-mediated signals in osteoclast development: regulation of interleukin 11 production by osteoblasts and distribution of its receptor in bone marrow cultures. J Exp Med (1996) 183(6):2581–91. doi: 10.1084/Jem.183.6.2581
13. Santos MD, Yasuike M, Kondo H, Hirono I, Aoki T. Teleostean il11b exhibits complementing function to il11a and expansive involvement in antibacterial and antiviral responses. Mol Immunol (2008) 45(12):3494–501. doi: 10.1016/J.Molimm.2008.02.004
14. Du X, Williams DA. Interleukin-11: review of molecular, cell biology, and clinical use. Blood (1997) 89(11):3897–908. doi: 10.1182/Blood.V89.11.3897
15. Putoczki T, Ernst M. More than A sidekick: the il-6 family cytokine il-11 links inflammation to cancer. J Leukocyte Biol (2010) 88(6):1109–17. doi: 10.1189/Jlb.0410226
16. Robb L, Hilton DJ, Brook-Carter PT, Begley CG. Identification of A second murine interleukin-11 receptor α-chain gene (Il11ra2) with A restricted pattern of expression. Genomics (1997) 40(3):387–94. doi: 10.1006/Geno.1996.4579
17. Sims NA, Walsh NC. Gp130 cytokines and bone remodelling in health and disease. Bmb Rep (2010) 43(8):513–23. doi: 10.5483/Bmbrep.2010.43.8.513
18. Fourcin M, Chevalier S, Lebrun JJ, Kelly P, Pouplard A, Wijdenes J, et al. Involvement of gp130/interleukin-6 receptor transducing component in interleukin-11 receptor. Eur J Immunol (1994) 24(1):277–80. doi: 10.1002/Eji.1830240143
19. Putoczki TL, Ernst M. Il-11 signaling as A therapeutic target for cancer. Immunotherapy (2015) 7(4):441–53. doi: 10.2217/Imt.15.17
20. Garbers C, Scheller J. Interleukin-6 and interleukin-11: same same but different. Biol Chem (2013) 394(9):1145–61. doi: 10.1515/Hsz-2013-0166
21. Magrangeas F, Boisteau O, Denis S, Jacques Y, Minvielle S. Negative cross-talk between interleukin-3 and interleukin-11 is mediated by suppressor of cytokine signalling-3 (Socs-3). Biochem J (2001) 353(Pt 2):223–30. doi: 10.1042/0264-6021:3530223
22. Metcalfe RD, Aizel K, Zlatic CO, Nguyen PM, Morton CJ, Lio DS-S, et al. The structure of the extracellular domains of human interleukin 11α Receptor reveals mechanisms of cytokine engagement. J Biol Chem (2020) 295(24):8285–301. doi: 10.1074/Jbc.Ra119.012351
23. Monhasery N, Moll J, Cuman C, Franke M, Lamertz L, Nitz R, et al. Transcytosis of il-11 and apical redirection of gp130 is mediated by il-11α Receptor. Cell Rep (2016) 16(4):1067–81. doi: 10.1016/J.Celrep.2016.06.062
24. Sammel M, Peters F, Lokau J, Scharfenberg F, Werny L, Linder S, et al. Differences in shedding of the interleukin-11 receptor by the proteases adam9, adam10, adam17, meprin α, meprin β And mt1-mmp. Int J Mol Sci (2019) 20(15):3677. doi: 10.3390/Ijms20153677
25. Lokau J, Nitz R, Agthe M, Monhasery N, Aparicio-Siegmund S, Schumacher N, et al. Proteolytic cleavage governs interleukin-11 trans-signaling. Cell Rep (2016) 14(7):1761–73. doi: 10.1016/J.Celrep.2016.01.053
26. Lokau J, Garbers C. The length of the interleukin-11 receptor stalk determines its capacity for classic signaling. J Biol Chem (2018) 293(17):6398–409. doi: 10.1074/Jbc.Ra118.001879
27. Lokau J, Kespohl B, Kirschke S, Garbers C. The role of proteolysis in interleukin-11 signaling. Biochim Biophys Acta BBA - Mol Cell Res (2022) 1869(1):119135. doi: 10.1016/J.Bbamcr.2021.119135
28. Curtis DJ, Hilton DJ, Roberts B, Murray L, Nicola N, Begley CG. Recombinant soluble interleukin-11 (Il-11) receptor alpha-chain can act as an il-11 antagonist. Blood (1997) 90(11):4403–12. doi: 10.1182/blood.V90.11.4403
29. Adami E, Viswanathan S, Widjaja AA, Ng B, Chothani S, Zhihao N, et al. Il11 is elevated in systemic sclerosis and il11-dependent erk signalling underlies tgfβ-mediated activation of dermal fibroblasts. Rheumatol (Oxford England) (2021) 60(12):5820–6. doi: 10.1093/Rheumatology/Keab168
30. Xiao R, Gu L, Li AM, Gan Y-L, He C-Y, Liao J-X, et al. Il-11 drives the phenotypic transformation of tracheal epithelial cells and fibroblasts to enhance abnormal repair after tracheal injury. Biochim Et Biophys Acta Mol Cell Res (2023) 1870(4):119438. doi: 10.1016/J.Bbamcr.2023.119438
31. Schafer S, Viswanathan S, Widjaja AA, Lim W-W, Moreno-Moral A, DeLaughter DM, et al. Il-11 is A crucial determinant of cardiovascular fibrosis. Nature (2017) 552(7683):110–5. doi: 10.1038/Nature24676
32. Corden B, Adami E, Sweeney M, Schafer S, Cook SA. Il-11 in cardiac and renal fibrosis: late to the party but A central player. Br J Pharmacol (2020) 177(8):1695–708. doi: 10.1111/Bph.15013
33. Jiang W, Jin L, Ju D, Lu Z, Wang C, Guo X, et al. The pancreatic clock is A key determinant of pancreatic fibrosis progression and exocrine dysfunction. Sci Trans Med (2022) 14(664):Eabn3586. doi: 10.1126/Scitranslmed.Abn3586
34. Tang W, Geba GP, Zheng T, Ray P, Homer RJ, Kuhn C, et al. Targeted expression of il-11 in the murine airway causes lymphocytic inflammation, bronchial remodeling, and airways obstruction. J Clin Invest (1996) 98(12):2845–53. doi: 10.1172/Jci119113
35. Minshall E, Chakir J, Laviolette M, Molet S, Zhu Z, Olivenstein R, et al. Il-11 expression is increased in severe asthma: association with epithelial cells and eosinophils. J Allergy Clin Immunol (2000) 105(2 Pt 1):232–8. doi: 10.1016/S0091-6749(00)90070-8
36. Ng B, Viswanathan S, Widjaja AA, Lim W-W, Shekeran SG, Goh JWT, et al. Il11 activates pancreatic stellate cells and causes pancreatic inflammation, fibrosis and atrophy in A mouse model of pancreatitis. Int J Mol Sci (2022) 23(7):3549. doi: 10.3390/Ijms23073549
37. Widjaja AA, Chothani SP, Cook SA. Different roles of interleukin 6 and interleukin 11 in the liver: implications for therapy. Hum Vaccines Immunother (2020) 16(10):2357–62. doi: 10.1080/21645515.2020.1761203
38. Dong J, Viswanathan S, Adami E, Singh BK, Chothani SP, Ng B, et al. Hepatocyte-specific il11 cis-signaling drives lipotoxicity and underlies the transition from nafld to nash. Nat Commun (2021) 12(1):66. doi: 10.1038/S41467-020-20303-Z
39. Widjaja AA, Singh BK, Adami E, Viswanathan S, Dong J, D’Agostino GA, et al. Inhibiting interleukin 11 signaling reduces hepatocyte death and liver fibrosis, inflammation, and steatosis in mouse models of nonalcoholic steatohepatitis. Gastroenterology (2019) 157(3):777–792.E14. doi: 10.1053/J.Gastro.2019.05.002
40. Effenberger M, Widjaja AA, Grabherr F, Schaefer B, Grander C, Mayr L, et al. Interleukin-11 drives human and mouse alcohol-related liver disease. Gut (2023) 72(1):168–79. doi: 10.1136/Gutjnl-2021-326076
41. Howlett M, Chalinor HV, Buzzelli JN, Nguyen N, van Driel IR, Bell KM, et al. Il-11 is A parietal cell cytokine that induces atrophic gastritis. Gut (2012) 61(10):1398–409. doi: 10.1136/Gutjnl-2011-300539
42. Lim WW, Ng B, Widjaja A, Xie C, Su L, Ko N, et al. Transgenic interleukin 11 expression causes cross-tissue fibro-inflammation and an inflammatory bowel phenotype in mice. PloS One (2020) 15(1):E0227505. doi: 10.1371/Journal.Pone.0227505
43. Ernst M, Najdovska M, Grail D, Lundgren-May T, Buchert M, Tye H, et al. Stat3 and stat1 mediate il-11-dependent and inflammation-associated gastric tumorigenesis in gp130 receptor mutant mice. J Clin Invest (2008) 118(5):1727–38. doi: 10.1172/Jci34944
44. Song C, Liu X, Tan W, Guo X, Mao Y, Zhou Q, et al. Fluorofenidone attenuates pulmonary inflammation and fibrosis by inhibiting the il-11/mek/erk signaling pathway. Shock (Augusta Ga.) (2022) 58(2):137–46. doi: 10.1097/Shk.0000000000001960
45. Ng B, Dong J, D’agostino G, Viswanathan S, Widjaja AA, Lim W-W, et al. Interleukin-11 is A therapeutic target in idiopathic pulmonary fibrosis. Sci Trans Med (2019) 11(511):Eaaw1237. doi: 10.1126/Scitranslmed.Aaw1237
46. Widjaja AA, Viswanathan S, Jinrui D, Singh BK, Tan J, Wei Ting JG, et al. Molecular dissection of pro-fibrotic il11 signaling in cardiac and pulmonary fibroblasts. Front In Mol Biosci (2021) 8:740650. doi: 10.3389/Fmolb.2021.740650
47. Chen H, Chen H, Liang J, Gu X, Zhou J, Xie C, et al. Tgf-β1/il-11/mek/erk signaling mediates senescence-associated pulmonary fibrosis in A stress-induced premature senescence model of bmi-1 deficiency. Exp Mol Med (2020) 52(1):130–51. doi: 10.1038/S12276-019-0371-7
48. Yin L, Liu MX, Li W, Wang F-Y, Tang Y-H, Huang C-X. Over-expression of inhibitor of differentiation 2 attenuates post-infarct cardiac fibrosis through inhibition of tgf-β1/smad3/hif-1α/il-11 signaling pathway. Front In Pharmacol (2019) 10:1349. doi: 10.3389/Fphar.2019.01349
49. Trepicchio WL, Bozza M, Pedneault G, Dorner AJ. Recombinant human il-11 attenuates the inflammatory response through down-regulation of proinflammatory cytokine release and nitric oxide production. J Immunol (Baltimore Md.: 1950) (1996) 157(8):3627–34. doi: 10.4049/jimmunol.157.8.3627
50. Bozza M, Bliss JL, Dorner AJ, et al. Interleukin-11 modulates th1/th2 cytokine production from activated cd4+ T cells. J Interferon Cytokine Res (2001) 21(1):21–30. doi: 10.1089/107999001459123
51. Curti A, Ratta M, Corinti S, Girolomoni G, Ricci F, Tazzari P, et al. Interleukin-11 induces th2 polarization of human cd4(+) T cells. Blood (2001) 97(9):2758–63. doi: 10.1182/Blood.V97.9.2758
52. Bozza M, Bliss JL, Maylor R, Erickson J, Donnelly L, Bouchard P, et al. Interleukin-11 reduces T-cell-dependent experimental liver injury in mice. Hepatol (Baltimore Md.) (1999) 30(6):1441–7. doi: 10.1002/Hep.510300616
53. Yao R, Lin Y, Li Q, Zhou X, Pan X, Bao Y, et al. Downregulation of T-bet/gata-3 ratio induced by il-11 treatment is responsible for th1/th2 balance restoration in human immune thrombocytopenic purpura (Itp). J Thromb And Thrombolysis (2014) 38(2):183–9. doi: 10.1007/S11239-013-1036-3
54. Miyawaki A, Iizuka Y, Sugino H, Watanabe Y. Il-11 prevents ifn-Γ-induced hepatocyte death through selective downregulation of ifn-Γ/stat1 signaling and ros scavenging. PloS One (2019) 14(2):E0211123. doi: 10.1371/Journal.Pone.0211123
55. Lai PC, Cook HT, Smith J, Keith JC, Pusey CD, Tam FWK. Interleukin-11 attenuates nephrotoxic nephritis in Wistar Kyoto rats. J Am Soc Nephrology: JASN (2001) 12(11):2310–20. doi: 10.1681/Asn.V12112310
56. Nadler EP, Stanford A, Zhang XR, Schall LC, Alber SM, Watkins SC, et al. Intestinal cytokine gene expression in infants with acute necrotizing enterocolitis: interleukin-11 mrna expression inversely correlates with extent of disease. J Pediatr Surg (2001) 36(8):1122–9. doi: 10.1053/Jpsu.2001.25726
57. Shimizu T, Shiratori K, Sawada T, et al. Recombinant human interleukin-11 decreases severity of acute necrotizing pancreatitis in mice. Pancreas (2000) 21(2):134–40. doi: 10.1097/00006676-200008000-00005
58. Zhu Z, Lee CG, Zheng T, Chupp G, Wang J, Homer RJ, et al. Airway inflammation and remodeling in asthma. Lessons from interleukin 11 and interleukin 13 transgenic mice. Am J Respir Crit Care Med (2001) 164:S67–70. doi: 10.1164/Ajrccm.164.Supplement_2.2106070
59. Zheng T, Zhu Z, Wang J, Homer RJ, Elias JA. Il-11: insights in asthma from overexpression transgenic modeling. J Allergy Clin Immunol (2001) 108(4):489–96. doi: 10.1067/Mai.2001.118510
60. Lee CG, Hartl D, Matsuura H, Dunlop FM, Scotney PD, Fabri LJ, et al. Endogenous il-11 signaling is essential in th2- and il-13–induced inflammation and mucus production. Am J Respir Cell Mol Biol (2008) 39(6):739–46. doi: 10.1165/Rcmb.2008-0053oc
61. Chen Q, Rabach L, Noble P, Zheng T, Lee CG, Homer RJ, et al. Il-11 receptor alpha in the pathogenesis of il-13-induced inflammation and remodeling. J Immunol (Baltimore Md.: 1950) (2005) 174(4):2305–13. doi: 10.4049/Jimmunol.174.4.2305
62. Howlett M, Giraud AS, Lescesen H, Jackson CB, Kalantzis A, Van Driel IR, et al. The interleukin-6 family cytokine interleukin-11 regulates homeostatic epithelial cell turnover and promotes gastric tumor development. Gastroenterology (2009) 136(3):967–977.E3. doi: 10.1053/J.Gastro.2008.12.003
63. Putoczki TL, Thiem S, Loving A, Busuttil RA, Wilson NJ, Ziegler PK, et al. Interleukin-11 is the dominant il-6 family cytokine during gastrointestinal tumorigenesis and can be targeted therapeutically. Cancer Cell (2013) 24(2):257–71. doi: 10.1016/J.Ccr.2013.06.017
64. Obana M, Maeda M, Takeda K, Hayama A, Mohri T, Yamashita T, et al. Therapeutic activation of signal transducer and activator of transcription 3 by interleukin-11 ameliorates cardiac fibrosis after myocardial infarction. Circulation (2010) 121(5):684–91. doi: 10.1161/Circulationaha.109.893677
65. Zhu M, Lu B, Cao Q, Wu Z, Xu Z, Li W, et al. Il-11 attenuates liver ischemia/reperfusion injury (Iri) through stat3 signaling pathway in mice. PloS One (2015) 10(5):E0126296. doi: 10.1371/Journal.Pone.0126296
66. Nieminen P, Morgan NV, Fenwick AL, Parmanen S, Veistinen L, Mikkola ML, et al. Inactivation of il11 signaling causes craniosynostosis, delayed tooth eruption, and supernumerary teeth. Am J Hum Genet (2011) 89(1):67–81. doi: 10.1016/J.Ajhg.2011.05.024
67. Ng B, Widjaja AA, Viswanathan S, Dong J, Chothani SP, Lim S, et al. Similarities and differences between il11 and il11ra1 knockout mice for lung fibro-inflammation, fertility and craniosynostosis. Sci Rep (2021) 11(1):14088. doi: 10.1038/S41598-021-93623-9
68. Kaneyama K, Segami N, Sato J, Nishimura M, Yoshimura H. Interleukin-6 family of cytokines as biochemical markers of osseous changes in the temporomandibular joint disorders. Br J Oral Maxillofac Surg (2004) 42(3):246–50. doi: 10.1016/S0266-4356(03)00258-4
69. Agthe M, Brügge J, Garbers Y, Wandel M, Kespohl B, Arnold P, et al. Mutations in craniosynostosis patients cause defective interleukin-11 receptor maturation and drive craniosynostosis-like disease in mice. Cell Rep (2018) 25(1):10–18.E5. doi: 10.1016/J.Celrep.2018.09.005
70. Pountos I, Walters G, Panteli M, Einhorn TA, Giannoudis PV. Inflammatory profile and osteogenic potential of fracture haematoma in humans. J Clin Med (2019) 9(1):47. doi: 10.3390/Jcm9010047
71. Kidd LJ, Stephens AS, Kuliwaba JS, Fazzalari NL, Wu ACK, Forwood MR. Temporal pattern of gene expression and histology of stress fracture healing. Bone (2010) 46(2):369–78. doi: 10.1016/J.Bone.2009.10.009
72. Dong B, Hiasa M, Higa Y, Ohnishi Y, Endo I, Kondo T, et al. Osteoblast/osteocyte-derived interleukin-11 regulates osteogenesis and systemic adipogenesis. Nat Commun (2022) 13(1):7194. doi: 10.1038/S41467-022-34869-3
73. Endo I. Glucocorticoid and bone. The effect of glucocorticoid and pth in osteoblast apoptosis and differentiation via interleukin 11 expression. Clin Calcium (2014) 24(9):1321–8.
74. Leon ER, Iwasaki K, Komaki M, Kojima T, Ishikawa I. Osteogenic effect of interleukin-11 and synergism with ascorbic acid in human periodontal ligament cells. J Periodontal Res (2007) 42(6):527–35. doi: 10.1111/J.1600-0765.2007.00977.X
75. Bouleftour W, Juignet L, Verdière L, Machuca-Gayet I, Thomas M, Laroche N, et al. Deletion of opn in bsp knockout mice does not correct bone hypomineralization but results in high bone turnover. Bone (2019) 120:411–22. doi: 10.1016/J.Bone.2018.12.001
76. Bouleftour W, Juignet L, Bouet G, Granito RN, Vanden-Bossche A, Laroche N, et al. The role of the sibling, bone sialoprotein in skeletal biology - contribution of mouse experimental genetics. Matrix Biol J Int Soc Matrix Biol (2016) 52-54:60–77. doi: 10.1016/J.Matbio.2015.12.011
77. Matsumura H, Nakayama Y, Takai H, Ogata Y. Effects of interleukin-11 on the expression of human bone sialoprotein gene. J Bone Miner Metab (2015) 33(2):142–53. doi: 10.1007/S00774-014-0576-8
78. Wang S, Sasaki Y, Zhou L, Matsumura H, Araki S, Mezawa M, et al. Transcriptional regulation of bone sialoprotein gene by interleukin-11. Gene (2011) 476(1-2):46–55. doi: 10.1016/J.Gene.2011.01.016
79. Takeuchi Y, Watanabe S, Ishii G, Takeda S, Nakayama K, Fukumoto S, et al. Interleukin-11 as A stimulatory factor for bone formation prevents bone loss with advancing age in mice. J Biol Chem (2002) 277(50):49011–8. doi: 10.1074/Jbc.M207804200
80. Suga K, Saitoh M, Fukushima S, Takahashi K, Nara H, Yasuda S, et al. Interleukin-11 induces osteoblast differentiation and acts synergistically with bone morphogenetic protein-2 in C3h10t1/2 cells. J Interferon Cytokine Res (2001) 21(9):695–707. doi: 10.1089/107999001753124435
81. Liu S-B, Hu P-Z, Hou Y, Li P, Cao W, Tian Q, et al. Recombinant human bone morphogenetic protein-2 promotes the proliferation of mesenchymal stem cells in vivo and in vitro. Chin Med J (2009) 122(7):839–43.
82. Suga K, Saitoh M, Kokubo S, Nozaki K, Fukushima S, Yasuda S, et al. Synergism between interleukin-11 and bone morphogenetic protein-2 in the healing of segmental bone defects in A rabbit model. J Interferon Cytokine Res (2004) 24(6):343–9. doi: 10.1089/107999004323142204
83. Suga K, Saitoh M, Kokubo S, Fukushima S, Kaku S, Yasuda S, et al. Interleukin-11 acts synergistically with bone morphogenetic protein-2 to accelerate bone formation in A rat ectopic model. J Interferon Cytokine Res (2003) 23(4):203–7. doi: 10.1089/107999003765027401
84. Manolagas SC, Jilka RL. Bone marrow, cytokines, and bone remodeling. Emerging insights into the pathophysiology of osteoporosis. New Engl J Med (1995) 332(5):305–11. doi: 10.1056/Nejm199502023320506
85. Giannoni P, Marini C, Cutrona G, Matis S, Capra MC, Puglisi F, et al. Chronic lymphocytic leukemia cells impair osteoblastogenesis and promote osteoclastogenesis: role of tnfα, il-6 and il-11 cytokines. Haematologica (2021) 106(10):2598–612. doi: 10.3324/Haematol.2019.231456
86. Sims NA, Jenkins BJ, Nakamura A, Quinn JMW, Li R, Gillespie MT, et al. Interleukin-11 receptor signaling is required for normal bone remodeling. J Bone Miner Res (2005) 20(7):1093–102. doi: 10.1359/Jbmr.050209
87. Hughes FJ, Howells GL. Interleukin-11 inhibits bone formation in vitro. Calcified Tissue Int (1993) 53(5):362–4. doi: 10.1007/Bf01351844
88. Hill PA, Tumber A, Papaioannou S, Meikle MC. The cellular actions of interleukin-11 on bone resorption in vitro. Endocrinology (1998) 139(4):1564–72. doi: 10.1210/Endo.139.4.5946
89. Mukkamalla SKR, Malipeddi D. Myeloma bone disease: A comprehensive review. Int J Mol Sci (2021) 22(12):6208. doi: 10.3390/Ijms22126208
90. Feng W, Guo J, Li M. Rankl-independent modulation of osteoclastogenesis. J Oral Biosci (2019) 61(1):16–21. doi: 10.1016/J.Job.2019.01.001
91. Kudo O, Sabokbar A, Pocock A, Itonaga I, Fujikawa Y, Athanasou NA. Interleukin-6 and interleukin-11 support human osteoclast formation by A rankl-independent mechanism. Bone (2003) 32(1):1–7. doi: 10.1016/S8756-3282(02)00915-8
92. Suda T, Takahashi N, Udagawa N, Jimi E, Gillespie MT, Martin TJ. Modulation of osteoclast differentiation and function by the new members of the tumor necrosis factor receptor and ligand families. Endocrine Rev (1999) 20(3):345–57. doi: 10.1210/Edrv.20.3.0367
93. Bezerra MC, Carvalho JF, Prokopowitsch AS, Pereira RMR. Rank, rankl and osteoprotegerin in arthritic bone loss. Braz J Med Biol Res Rev Bras Pesqui Medicas E Biol (2005) 38(2):161–70. doi: 10.1590/S0100-879x2005000200004
94. Ahlen J, Andersson S, Mukohyama H, Roth C, Bäckman A, Conaway HH, et al. Characterization of the bone-resorptive effect of interleukin-11 in cultured mouse calvarial bones. Bone (2002) 31(1):242–51. doi: 10.1016/S8756-3282(02)00784-6
95. Rosa-Rañal M, de la Cruz DA, Lorena-Rubio Y, Larrea F. New paradigms in the regulation of bone metabolism. Rev Investig Clin Organo Hosp Enfermedades Nutr (2001) 53(4):362–9.
96. Zhang Y, Fujita N, Oh-Hara T, Morinaga Y, Nakagawa T, Yamada M, et al. Production of interleukin-11 in bone-derived endothelial cells and its role in the formation of osteolytic bone metastasis. Oncogene (1998) 16(6):693–703. doi: 10.1038/Sj.Onc.1201581
97. Hu Y, Li K, Swahn H, Ordoukhanian P, Head SR, Natarajan P, et al. Transcriptomic analyses of joint tissues during osteoarthritis development in A rat model reveal dysregulated mechanotransduction and extracellular matrix pathways. Osteoarthritis Cartilage (2023) 31(2):199–212. doi: 10.1016/J.Joca.2022.10.003
98. Lisignoli G, Piacentini A, Toneguzzi S, Grassi F, Cocchini B, Ferruzzi A, et al. Osteoblasts and stromal cells isolated from femora in rheumatoid arthritis (Ra) and osteoarthritis (Oa) patients express il-11, leukaemia inhibitory factor and oncostatin M. Clin Exp Immunol (2000) 119(2):346–53. doi: 10.1046/J.1365-2249.2000.01114.X
99. Elshabrawy HA, Volin MV, Essani AB, Chen Z, McInnes IB, Van Raemdonck K, et al. Il-11 facilitates A novel connection between ra joint fibroblasts and endothelial cells. Angiogenesis (2018) 21(2):215–28. doi: 10.1007/S10456-017-9589-Y
100. Trontzas P, Kamper EF, Potamianou A, Kyriazis NC, Kritikos H, Stavridis J. Comparative study of serum and synovial fluid interleukin-11 levels in patients with various arthritides. Clin Biochem (1998) 31(8):673–9. doi: 10.1016/S0009-9120(98)00062-9
101. Tuerlings M, Van Hoolwerff M, Houtman E, Suchiman EHED, Lakenberg N Mei H, et al. Rna sequencing reveals interacting key determinants of osteoarthritis acting in subchondral bone and articular cartilage: identification of il11 and Chadl as attractive treatment targets. Arthritis Rheumatol (Hoboken N.J.) (2021) 73(5):789–99. doi: 10.1002/Art.41600
102. Kokebie R, Aggarwal R, Lidder S, Hakimiyan AA, Rueger DC, Block JA, et al. The role of synovial fluid markers of catabolism and anabolism in osteoarthritis, rheumatoid arthritis and asymptomatic organ donors. Arthritis Res Ther (2011) 13(2):R50. doi: 10.1186/Ar3293
103. Zou R, Huang X, Xu P. The study of gp130/the inflammatory factors regulating osteoclast differentiation in rheumatoid arthritis. Biochem Biophysics Rep (2021) 26:100934. doi: 10.1016/J.Bbrep.2021.100934
104. Shaughnessy SG, Walton KJ, Deschamps P, Butcher M, Beaudin SM. Neutralization of interleukin-11 activity decreases osteoclast formation and increases cancellous bone volume in ovariectomized mice. Cytokine (2002) 20(2):78–85. doi: 10.1006/Cyto.2002.1981
105. Shaarawy M, Zaki S, Sheiba M, El-Minawi AM. Circulating levels of osteoclast activating cytokines, interleukin-11 and transforming growth factor-beta2, as valuable biomarkers for the assessment of bone turnover in postmenopausal osteoporosis. Clin Lab (2003) 49(11-12):625–36.
106. Verhaeghe J, Van Herck E, Van Bree R, Bouillon R, Dequeker J, Keith JC. Recombinant human interleukin-11 does not modify biochemical parameters of bone remodeling and bone mineral density in adult ovariectomized rats. J Interferon Cytokine Res (1998) 18(1):49–53. doi: 10.1089/Jir.1998.18.49
107. Scheller EL, Rosen CJ. What’s the matter with mat? Marrow adipose tissue, metabolism, and skeletal health. Ann N Y Acad Sci (2014) 1311(1):14–30. doi: 10.1111/Nyas.12327
108. Takeshita S, Fumoto T, Naoe Y, Ikeda K. Age-related marrow adipogenesis is linked to increased expression of rankl. J Biol Chem (2014) 289(24):16699–710. doi: 10.1074/Jbc.M114.547919
109. Wan Y, Chong LW, Evans RM. Ppar-Γ Regulates osteoclastogenesis in mice. Nat Med (2007) 13(12):1496–503. doi: 10.1038/Nm1672
110. Kelly KA, Tanaka S, Baron R, Gimble JM. Murine bone marrow stromally derived bms2 adipocytes support differentiation and function of osteoclast-like cells in vitro. Endocrinology (1998) 139(4):2092–101. doi: 10.1210/Endo.139.4.5915
111. Muruganandan S, Sinal CJ. The impact of bone marrow adipocytes on osteoblast and osteoclast differentiation. IUBMB Life (2014) 66(3):147–55. doi: 10.1002/Iub.1254
112. Paccou J, Hardouin P, Cotten A, Penel G, Cortet B. The role of bone marrow fat in skeletal health: usefulness and perspectives for clinicians. J Clin Endocrinol Metab (2015) 100(10):3613–21. doi: 10.1210/Jc.2015-2338
113. Li J, Zhang N, Huang X, Xu J, Fernandes JC, Dai K, et al. Dexamethasone shifts bone marrow stromal cells from osteoblasts to adipocytes by C/ebpalpha promoter methylation. Cell Death Dis (2013) 4(10):E832. doi: 10.1038/Cddis.2013.348
114. Shanmugam M, Rajgopal G, Sinal CJ. Bone marrow adipose tissue and skeletal health. Curr Osteoporosis Rep (2018) 16(4):434–42. doi: 10.1007/S11914-018-0451-Y
115. Kawashima I, Ohsumi J, Mita-Honjo K, Shimoda-Takano K, Ishikawa H, Sakakibara S, et al. Molecular cloning of cdna encoding adipogenesis inhibitory factor and identity with interleukin-11. FEBS Lett (1991) 283(2):199–202. doi: 10.1016/0014-5793(91)80587-S
116. Ohsumi J, Miyadai K, Kawashima I, Ishikawa-Ohsumi W, Sakakihars S. A novel inhibitory regulator of adipose conversion in bone marrow. FEBS Lett (1991) 288(1-2):13–6. doi: 10.1016/0014-5793(91)80991-b
117. Kawashima I, Ohsumi J, Miyadai K, Takiguchi Y. Function, molecular structure and gene expression of interleukin-11 (IL-11/AGIF). Nihon Rinsho Japanese J Clin Med (1992) 50(8):1833–9.
118. Kodama Y, Takeuchi Y, Suzawa M, Fukumoto S, Murayama H, Yamato H, et al. Reduced expression of interleukin-11 in bone marrow stromal cells of senescence-accelerated mice (Samp6): relationship to osteopenia with enhanced adipogenesis. J Bone Mineral Res (1998) 13(9):1370–7. doi: 10.1359/Jbmr.1998.13.9.1370
119. Keller DC, Du XX, Srour EF, Hoffman R, Williams DA. Interleukin-11 inhibits adipogenesis and stimulates myelopoiesis in human long-term marrow cultures. Blood (1993) 82(5):1428–35. doi: 10.1182/Blood.V82.5.1428.Bloodjournal8251428
120. Hoang B, Trinh A, Birnbaumer L, Edwards RA. Decreased mapk- and pge2-dependent il-11 production in gialpha2-/- colonic myofibroblasts. Am J Physiol Gastrointestinal Liver Physiol (2007) 292(6):G1511–1519. doi: 10.1152/Ajpgi.00307.2006
121. Annamalai D, Clipstone NA. Prostaglandin F2α Inhibits adipogenesis via an autocrine-mediated interleukin-11/glycoprotein 130/stat1-dependent signaling cascade. J Cell Biochem (2014) 115(7):1308–21. doi: 10.1002/Jcb.24785
122. Idris NM, Ashraf M, Ahmed RPH, Shujia J, Haider KH. Activation of il-11/stat3 pathway in preconditioned human skeletal myoblasts blocks apoptotic cascade under oxidant stress. Regenerative Med (2012) 7(1):47–57. doi: 10.2217/Rme.11.109
123. Yang W, Zhang S, Ou T, Jiang H, Jia D, Qi Z, et al. Interleukin-11 regulates the fate of adipose-derived mesenchymal stem cells via stat3 signalling pathways. Cell Proliferation (2020) 53(5):E12771. doi: 10.1111/Cpr.12771
124. Zhuang Z, Pan X, Zhao K, Gao W, Liu J, Deng T, et al. The effect of interleukin-6 (Il-6), interleukin-11 (Il-11), signal transducer and activator of transcription 3 (Stat3), and akt signaling on adipocyte proliferation in A rat model of polycystic ovary syndrome. Med Sci Monitor: Int Med J Exp Clin Res (2019) 25:7218–27. doi: 10.12659/Msm.916385
125. Tenney R, Stansfield K, Pekala PH. Interleukin 11 signaling in 3t3-L1 adipocytes. J Cell Physiol (2005) 202(1):160–6. doi: 10.1002/Jcp.20100
126. Du XX, Scott D, Yang ZX, Cooper R, Xiao XL, Williams DA. Interleukin-11 stimulates multilineage progenitors, but not stem cells, in murine and human long-term marrow cultures. Blood (1995) 86(1):128–34. doi: 10.1182/blood.V86.1.128.bloodjournal861128
127. Schlerman FJ, Bree AG, Kaviani MD, Nagle SL, Donnelly LH, Mason LE, et al. Thrombopoietic activity of recombinant human interleukin 11 (Rhuil-11) in normal and myelosuppressed nonhuman primates. Stem Cells (Dayton Ohio) (1996) 14(5):517–32. doi: 10.1002/Stem.140517
128. Dams-Kozlowska H, Kwiatkowska-Borowczyk E, Gryska K, Lewandowska A, Marszalek A, Adamczyk S, et al. Effects of designer hyper-interleukin 11 (H11) on hematopoiesis in myelosuppressed mice. PloS One (2016) 11(5):E0154520. doi: 10.1371/Journal.Pone.0154520
129. Goldman SJ. Preclinical biology of interleukin 11: A multifunctional hematopoietic cytokine with potent thrombopoietic activity. Stem Cells (Dayton Ohio) (1995) 13(5):462–71. doi: 10.1002/Stem.5530130503
130. Shurin MR, Esche C, Lotze MT. Flt3: receptor and ligand. Biology and potential clinical application. Cytokine Growth Factor Rev (1998) 9(1):37–48. doi: 10.1016/S1359-6101(97)00035-X
131. Jacobsen SE, Okkenhaug C, Myklebust J, Veiby OP, Lyman SD. The flt3 ligand potently and directly stimulates the growth and expansion of primitive murine bone marrow progenitor cells in vitro: synergistic interactions with interleukin (Il) 11, il-12, and other hematopoietic growth factors. J Exp Med (1995) 181(4):1357–63. doi: 10.1084/Jem.181.4.1357
132. Yonemura Y, Ku H, Lyman SD, Ogawa M. In vitro expansion of hematopoietic progenitors and maintenance of stem cells: comparison between flt3/flk-2 ligand and kit ligand. Blood (1997) 89(6):1915–21. doi: 10.1182/blood.V89.6.1915
133. Yang L, Yang YC. Heparin inhibits the expression of interleukin-11 and granulocyte-macrophage colony-stimulating factor in primate bone marrow stromal fibroblasts through mrna destabilization. Blood (1995) 86(7):2526–33. doi: 10.1182/blood.V86.7.2526.2526
134. Matsuda-Hashii Y, Takai K, Ohta H, Fujisaki H, Tokimasa S, Osugi Y, et al. Hepatocyte growth factor plays roles in the induction and autocrine maintenance of bone marrow stromal cell il-11, sdf-1 alpha, and stem cell factor. Exp Hematol (2004) 32(10):955–61. doi: 10.1016/J.Exphem.2004.06.012
135. Strømme O, Psonka-Antonczyk KM, Stokke BT, Sundan A, Arum C-J, Brede G. Myeloma-derived extracellular vesicles mediate hgf/C-met signaling in osteoblast-like cells. Exp Cell Res (2019) 383(1):111490. doi: 10.1016/J.Yexcr.2019.07.003
136. Weich NS, Wang A, Fitzgerald M, Neben TY, Donaldson D, Giannotti J, et al. Recombinant human interleukin-11 directly promotes megakaryocytopoiesis in vitro. Blood (1997) 90(10):3893–902. doi: 10.1182/Blood.V90.10.3893
137. Mauch P, Lamont C, Neben TY, Quinto C, Goldman SJ, Witsell A. Hematopoietic stem cells in the blood after stem cell factor and interleukin-11 administration: evidence for different mechanisms of mobilization. Blood (1995) 86(12):4674–80. doi: 10.1182/Blood.V86.12.4674.Bloodjournal86124674
138. Jenkins BJ, Roberts AW, Greenhill CJ, NajdovskaM, Lundgren-May T, Robb L, et al. *Pathologic consequences of stat3 hyperactivation by il-6 and il-11 during hematopoiesis and lymphopoiesis. Blood (2007) 109(6):2380–8. doi: 10.1182/Blood-2006-08-040352
139. Maze R, Moritz T, Williams DA. Increased survival and multilineage hematopoietic protection from delayed and severe myelosuppressive effects of A nitrosourea with recombinant interleukin-11. Cancer Res (1994) 54(18):4947–51.
140. Noach EJK, Ausema A, Dillingh JH, Dontje B, Weersing E, Akkerman I, et al. Growth factor treatment prior to low-dose total body irradiation increases donor cell engraftment after bone marrow transplantation in mice. Blood (2002) 100(1):312–7. doi: 10.1182/Blood.V100.1.312
141. Galmiche MC, Vogel CA, Delaloye AB, Schmidt PM, Healy F, Mach JP, et al. Combined effects of interleukin-3 and interleukin-11 on hematopoiesis in irradiated mice. Exp Hematol (1996) 24(11):1298–306.
142. Du XX, Neben T, Goldman S, Williams DA. Effects of recombinant human interleukin-11 on hematopoietic reconstitution in transplant mice: acceleration of recovery of peripheral blood neutrophils and platelets. Blood (1993) 81(1):27–34. doi: 10.1182/blood.V81.1.27.27
143. Huang L, Zhang Z, Lv W, Zhang M, Yang S, Yin L, et al. Interleukin 11 protects bone marrow mitochondria from radiation damage. In: Van Huffel S, Naulaers G, Caicedo A, et al, editors. Oxygen transport to tissue XXXV. New York, Ny: Springer New York (2013). p. 257–64. Available at: http://link.springer.com/10.1007/978-1-4614-7411-1_35. doi: 10.1007/978-1-4614-7411-1_35
144. Tsimberidou AM, Giles FJ, Khouri I, Bueso-Ramos C, Pilat S, Thomas DA, et al. Low-dose interleukin-11 in patients with bone marrow failure: update of the MD. Anderson cancer center experience. Ann Oncol Off J Eur Soc Med Oncol (2005) 16(1):139–45. doi: 10.1093/Annonc/Mdi007
145. Li T-M, Wu C-M, Huang H-C, Chou P-C, Fong Y-C, Tang C-H. Interleukin-11 increases cell motility and up-regulates intercellular adhesion molecule-1 expression in human chondrosarcoma cells. J Cell Biochem (2012) 113(11):3353–62. doi: 10.1002/Jcb.24211
146. Zhang B, Li Y, Wu Q, Xie L, Barwick B, Fu C, et al. *Acetylation of klf5 maintains emt and tumorigenicity to cause chemoresistant bone metastasis in prostate cancer. Nat Commun (2021) 12:1714. doi: 10.1038/S41467-021-21976-W
147. Gupta J, Robbins J, Jilling T, Seth P. Tgfβ-dependent induction of interleukin-11 and interleukin-8 involves smad and P38 mapk pathways in breast tumor models with varied bone metastases potential. Cancer Biol Ther (2011) 11(3):311–6. doi: 10.4161/Cbt.11.3.14096
148. Ren L, Wang X, Dong Z, Liu J, Zhang S. Bone metastasis from breast cancer involves elevated il-11 expression and the gp130/stat3 pathway. Med Oncol (Northwood London England) (2013) 30(3):634. doi: 10.1007/S12032-013-0634-4
149. Ren L, Yu Y, Wang X, Ge J, Wen L. Levels and clinical significances of interleukin 11 in breast tissue and serum of bone metastasis of breast cancer. Zhonghua Yi Xue Za Zhi (2014) 94(34):2656–60. doi: 10.3760/cma.j.issn.0376-2491.2014.34.004
150. Kang Y, He W, Tulley S, Gupta GP, Serganova I, Chen C-R, et al. Breast cancer bone metastasis mediated by the smad tumor suppressor pathway. Proc Natl Acad Sci USA (2005) 102(39):13909–14. doi: 10.1073/Pnas.0506517102
151. Deckers M, Van Dinther M, Buijs J, Que I, Löwik C, van der Pluijm G, et al. The tumor suppressor smad4 is required for transforming growth factor beta-induced epithelial to mesenchymal transition and bone metastasis of breast cancer cells. Cancer Res (2006) 66(4):2202–9. doi: 10.1158/0008-5472.Can-05-3560
152. Sterling JA, Edwards JR, Martin TJ, Mundy GR. Advances in the biology of bone metastasis: how the skeleton affects tumor behavior. Bone (2011) 48(1):6–15. doi: 10.1016/J.Bone.2010.07.015
153. Yu L, Wang S, Lin X, Lu Y, Gu P. MicroRNA-124a inhibits cell proliferation and migration in liver cancer by regulating interleukin-11. Mol Med Rep (2018) 17(3):3972–8. doi: 10.3892/Mmr.2017.8348
154. Cai W-L, Huang W-D, Li B, Chen T-R, Li Z-X, Zhao C-L, et al. *MicroRNA-124 inhibits bone metastasis of breast cancer by repressing interleukin-11. Mol Cancer (2018) 17:9. doi: 10.1186/S12943-017-0746-0
155. Maroni P, Bendinelli P, Ferraretto A, Lombardi G. Interleukin 11 (Il-11): role(S) in breast cancer bone metastases. Biomedicines (2021) 9(6):659. doi: 10.3390/Biomedicines9060659
156. Irawan C, Atmakusumah D, Siregar NC, Tean TB, Kong LW, Kiat OC, et al. Expression of biomarkers cxcr4, il11-ra, tff1, mlf1p in advanced breast cancer patients with bone metastatic: A diagnostic study. Acta Med Indonesiana (2016) 48(4):261–8.
157. Lewis VO, Ozawa MG, Deavers MT, Wang G, Shintani T, Arap W, et al. The interleukin-11 receptor alpha as A candidate ligand-directed target in osteosarcoma: consistent data from cell lines, orthotopic models, and human tumor samples. Cancer Res (2009) 69(5):1995–9. doi: 10.1158/0008-5472.Can-08-4845
158. Pasqualini R, Millikan RE, Christianson DR, Cardó-Vila M, Driessen WHP, Giordano RJ, et al. Targeting the interleukin-11 receptor α In metastatic prostate cancer: A first-in-man study. Cancer (2015) 121(14):2411–21. doi: 10.1002/Cncr.29344
159. Karjalainen K, Jaalouk DE, Bueso-Ramos C, Bover L, Sun Y, Kuniyasu A, et al. Targeting il11 receptor in leukemia and lymphoma: A functional ligand-directed study and hematopathology analysis of patient-derived specimens. Clin Cancer Res (2015) 21(13):3041–51. doi: 10.1158/1078-0432.Ccr-13-3059
160. Lewis VO, Devarajan E, Cardó-Vila M, Thomas DG, Kleinerman ES, Marchiò S, et al. Bmtp-11 is active in preclinical models of human osteosarcoma and A candidate targeted drug for clinical translation. Proc Natl Acad Sci USA (2017) 114(30):8065–70. doi: 10.1073/Pnas.1704173114
161. Haynesworth SE, Baber MA, Caplan AI. Cytokine expression by human marrow-derived mesenchymal progenitor cells in vitro: effects of dexamethasone and il-1 alpha. J Cell Physiol (1996) 166(3):585–92. doi: 10.1002/(Sici)1097-4652(199603)166:3<585::Aid-Jcp13>3.0.Co;2-6
162. Kim CH, Cheng SL, Kim GS. Effects of dexamethasone on proliferation, activity, and cytokine secretion of normal human bone marrow stromal cells: possible mechanisms of glucocorticoid-induced bone loss. J Endocrinol (1999) 162(3):371–9. doi: 10.1677/Joe.0.1620371
163. Kuriwaka-Kido R, Kido S, Miyatani Y, Ito Y, Kondo T, Omatsu T, et al. Parathyroid hormone (1-34) counteracts the suppression of interleukin-11 expression by glucocorticoid in murine osteoblasts: A possible mechanism for stimulating osteoblast differentiation against glucocorticoid excess. Endocrinology (2013) 154(3):1156–67. doi: 10.1210/En.2013-1915
164. Angeli A, Dovio A, Sartori ML, Masera RG, Ceoloni B, Prolo P, et al. Interactions between glucocorticoids and cytokines in the bone microenvironment. Ann N Y Acad Sci (2002) 966:97–107. doi: 10.1111/J.1749-6632.2002.Tb04207.X
165. Dovio A, Sartori ML, Masera RG, Ceoloni B, Reimondo G, Prolo P, et al. Autocrine down-regulation of glucocorticoid receptors by interleukin-11 in human osteoblast-like cell lines. J Endocrinol (2003) 177(1):109–17. doi: 10.1677/Joe.0.1770109
166. Boutin A, Gershengorn MC, Neumann S. β-arrestin 1 in thyrotropin receptor signaling in bone: studies in osteoblast-like cells. Front Endocrinol (2020) 11:312. doi: 10.3389/Fendo.2020.00312
167. Ueda Y, Inaba M, Takada K, Fukui J, Sakaguchi Y, Tsuda M, et al. Induction of senile osteoporosis in normal mice by intra-bone marrow-bone marrow transplantation from osteoporosis-prone mice. Stem Cells (Dayton Ohio) (2007) 25(6):1356–63. doi: 10.1634/Stemcells.2006-0811
168. Potapova IA, Gaudette GR, Brink PR, Robinson RB, Rosen MR, Cohen IS, et al. Mesenchymal stem cells support migration, extracellular matrix invasion, proliferation, and survival of endothelial cells in vitro. Stem Cells (Dayton Ohio) (2007) 25(7):1761–8. doi: 10.1634/Stemcells.2007-0022
169. Giuliani N, Colla S, Morandi F, Rizzoli V. The rank/rank ligand system is involved in interleukin-6 and interleukin-11 up-regulation by human myeloma cells in the bone marrow microenvironment. Haematologica (2004) 89(9):1118–23. doi: 10.3324/%x
170. Fayyad-Kazan H, Faour WH, Badran B, Lagneaux L, Najar M. The immunomodulatory properties of human bone marrow-derived mesenchymal stromal cells are defined according to multiple immunobiological criteria. Inflamm Res (2016) 65(6):501–10. doi: 10.1007/S00011-016-0933-2
171. Siddappa R, Martens A, Doorn J, Leusink A, Olivo C, Licht R, et al. cAMP/PKA pathway activation in human mesenchymal stem cells in vitro results in robust bone formation in vivo. Proc Natl Acad Sci USA (2008) 105(20):7281–6. doi: 10.1073/Pnas.0711190105
172. Ichioka N, Inaba M, Kushida T, Esumi T, Takahara K, Inaba K, et al. Prevention of senile osteoporosis in samp6 mice by intrabone marrow injection of allogeneic bone marrow cells. Stem Cells (Dayton Ohio) (2002) 20(6):542–51. doi: 10.1634/Stemcells.20-6-542
173. Kuo C-L, Liu S-T, Chang Y-L, Wu C-C, Huang S-M. Zac1 regulates il-11 expression in osteoarthritis. Oncotarget (2018) 9(65):32478–95. doi: 10.18632/Oncotarget.25980
174. Tohjima E, Inoue D, Yamamoto N, Kido S, Ito Y, Kato S, et al. Decreased ap-1 activity and interleukin-11 expression by bone marrow stromal cells may be associated with impaired bone formation in aged mice. J Bone Mineral Res (2003) 18(8):1461–70. doi: 10.1359/Jbmr.2003.18.8.1461
175. Sasaki K, Komamura S, Matsuda K. Extracellular stimulation of lung fibroblasts with arachidonic acid increases interleukin 11 expression through P38 and erk signaling. Biol Chem (2023) 404(1):59–69. doi: 10.1515/Hsz-2022-0218
176. Morinaga Y, Fujita N, Ohishi K, Tsuruo T. Stimulation of interleukin-11 production from osteoblast-like cells by transforming growth factor-beta and tumor cell factors. Int J Cancer (1997) 71(3):422–8. doi: 10.1002/(SICI)1097-0215(19970502)71:3<422::AID-IJC20>3.0.CO;2-G
177. Morinaga Y, Fujita N, Ohishi K, Zhang Y, Tsuruo T. Suppression of interleukin-11-mediated bone resorption by cyclooxygenases inhibitors. J Cell Physiol (1998) 175(3):247–54. doi: 10.1002/(SICI)1097-4652(199806)175:3<247::AID-JCP2>3.0.CO;2-O
178. Aman MJ, Bug G, Aulitzky WE, Huber C, Peschel C. Inhibition of interleukin-11 by interferon-alpha in human bone marrow stromal cells. Exp Hematol (1996) 24(8):863–7.
179. Zhang X, Wu H, Dobson JR, Browne G, Hong D, Akech J, et al. Expression of the il-11 gene in metastatic cells is supported by runx2-smad and runx2-cjun complexes induced by tgfβ1. J Cell Biochem (2015) 116(9):2098–108. doi: 10.1002/Jcb.25167
180. Kilroy GE, Foster SJ, Wu X, Ruiz J, Sherwood S, Heifetz A, et al. Cytokine profile of human adipose-derived stem cells: expression of angiogenic, hematopoietic, and pro-inflammatory factors. J Cell Physiol (2007) 212(3):702–9. doi: 10.1002/Jcp.21068
181. Li L, Khansari A, Shapira L, Graves DT, Amar S. Contribution of interleukin-11 and prostaglandin(S) in lipopolysaccharide-induced bone resorption in vivo. Infection Immun (2002) 70(7):3915–22. doi: 10.1128/Iai.70.7.3915-3922.2002
182. Okada Y, Montero A, Zhang X, Sobue T, Lorenzo J, Doetschman T, et al. Impaired osteoclast formation in bone marrow cultures of fgf2 null mice in response to parathyroid hormone. J Biol Chem (2003) 278(23):21258–66. doi: 10.1074/Jbc.M302113200
183. Walton KJ, Duncan JM, Deschamps P, Shaughnessy SG. Heparin acts synergistically with interleukin-11 to induce stat3 activation and in vitro osteoclast formation. Blood (2002) 100(7):2530–6. doi: 10.1182/Blood.V100.7.2530
184. Smith R, Ransjö M, Tatarczuch L, Song S-J, Pagel C, Morrison JR, et al. Activation of protease-activated receptor-2 leads to inhibition of osteoclast differentiation. J Bone Mineral Res (2004) 19(3):507–16. doi: 10.1359/Jbmr.0301248
185. Girasole G, Passeri G, Jilka RL, Manolagas SC. Interleukin-11: A new cytokine critical for osteoclast development. . J Clin Invest (1994) 93(4):1516–24. doi: 10.1172/Jci117130
186. Taki H, Sugiyama E, Kuroda A, Mino T, Kobayashi M. Interleukin-4 inhibits interleukin-11 production by rheumatoid synovial cells. Rheumatol (Oxford England) (2000) 39(7):728–31. doi: 10.1093/Rheumatology/39.7.728
187. Fukuda K, Miura Y, Maeda T, Hayashi S, Matsumoto T, Kuroda R. Expression profiling of genes in rheumatoid fibroblast-like synoviocytes regulated by fas ligand via cdna microarray analysis. Exp Ther Med (2021) 22(3):1000. doi: 10.3892/Etm.2021.10432
188. Souza PPC, Palmqvist P, Lundberg P, Lundgren I, Hänström L, Souza JAC, et al. Interleukin-4 and interleukin-13 inhibit the expression of leukemia inhibitory factor and interleukin-11 in fibroblasts. Mol Immunol (2012) 49(4):601–10. doi: 10.1016/J.Molimm.2011.10.009
189. Elias JA, Tang W, Horowitz MC. Cytokine and hormonal stimulation of human osteosarcoma interleukin-11 production. Endocrinology (1995) 136(2):489–98. doi: 10.1210/Endo.136.2.7835281
190. Boutin A, Neumann S, Gershengorn MC. Multiple transduction pathways mediate thyrotropin receptor signaling in preosteoblast-like cells. Endocrinology (2016) 157(5):2173–81. doi: 10.1210/En.2015-2040
191. Ahmed N, Sammons J, Carson RJ, Khokher MA, Hassan HT. Effect of bone morphogenetic protein-6 on haemopoietic stem cells and cytokine production in normal human bone marrow stroma. Cell Biol Int (2001) 25(5):429–35. doi: 10.1006/Cbir.2000.0662
192. Lui ELH, Ao CKL, Li L, Khong M-L, Tanner JA. Inorganic polyphosphate triggers upregulation of interleukin 11 in human osteoblast-like saos-2 cells. Biochem Biophys Res Commun (2016) 479(4):766–71. doi: 10.1016/J.Bbrc.2016.09.137
Keywords: IL-11, bone, metabolism, immunity, systematic review
Citation: Han Y, Gao H, Gan X, Liu J, Bao C and He C (2024) Roles of IL-11 in the regulation of bone metabolism. Front. Endocrinol. 14:1290130. doi: 10.3389/fendo.2023.1290130
Received: 09 September 2023; Accepted: 29 December 2023;
Published: 30 January 2024.
Edited by:
Cristina Vassalle, Gabriele Monasterio Tuscany Foundation (CNR), ItalyReviewed by:
Jae-Hyuck Shim, University of Massachusetts Medical School, United StatesEmanuele Bizzi, ASST Fatebenefratelli Sacco, Italy
Copyright © 2024 Han, Gao, Gan, Liu, Bao and He. This is an open-access article distributed under the terms of the Creative Commons Attribution License (CC BY). The use, distribution or reproduction in other forums is permitted, provided the original author(s) and the copyright owner(s) are credited and that the original publication in this journal is cited, in accordance with accepted academic practice. No use, distribution or reproduction is permitted which does not comply with these terms.
*Correspondence: Chengqi He, aHhrZmhjcTIwMTVAMTI2LmNvbQ==; Xinling Gan, NDUyMDI3MDcxQHFxLmNvbQ==