- 1Thyroid Research Group, Division of Infection and Immunity, Cardiff University School of Medicine, Heath Park, Cardiff, United Kingdom
- 2Section of Endocrinology, Ysbyty Ystrad Fawr and Royal Gwent Hospitals, Aneurin Bevan University Health Board, Newport, United Kingdom
Approximately 10%–15% of subjects with hypothyroidism on L-thyroxine (LT4) alone have persistent symptoms affecting their quality of life (QoL). Although the cause is unclear, there is evidence that “tissue T3 lack” may be responsible. If so, combining liothyronine (LT3) with LT4 would be helpful. However, randomized controlled trials (RCT), have not established greater efficacy for the LT3 + LT4 combination in these subjects than for LT4 alone. While the trial design may have been responsible, the use of unphysiological, short-acting LT3 preparations and non-thyroid-specific patient-reported outcome measures (PROMs) may have contributed. We recommend attention to the following aspects of trial design for future RCTs of LT3 + LT4 compared to LT4 alone: (a) Subject selection—(i) measurable symptoms (disadvantages should be recognized); (ii) using a validated thyroid specific PROM such as ThyPRO39 or the Composite scale derived from it; (iii) those taking over 1.2 μg/day or 100 μg/day (for pragmatic reasons) of LT4 defining a population likely without intrinsic thyroid activity who depend on exogenous LT4; (iv) recruiting a preponderance of subjects with autoimmune thyroiditis increasing generalisability; and (v) those with a high symptom load with a greater response to combination therapy e.g. those with the deiodinase 2 polymorphism. (b) The use of physiological LT3 preparations producing pharmacokinetic similarities to T3 profiles in unaffected subjects: two long-acting LT3 preparations are currently available and must be tested in phase 2b/3 RCTs. (c) The superiority of a crossover design in limiting numbers and costs while maintaining statistical power and ensuring that all subjects experienced the investigative medication.
Introduction
Eighteen randomized controlled trials (RCT) of combined liothyronine (LT3) and L-thyroxine (LT4) therapy (LT3 + LT4) have been inconclusive regarding their superiority in improving symptoms and quality of life (QoL) in hypothyroid subjects dissatisfied with LT4 alone (1) (Table 1). While 16 RCTs used short acting LT3 preparations in subjects with primary hypothyroidism (autoimmune, post-radioiodine, post-thyroidectomy), one used natural desiccated thyroid (NDT) and one investigated patients with central hypothyroidism (2, 3). This group of “dissatisfied” hypothyroid subjects on LT4 monotherapy constitutes a significant number estimated to be 10%–15% of all hypothyroid patients in the UK (4–6). Therefore, the unmet needs are significant. Their number will increase because of (a) the rising prevalence of hypothyroidism partly accounted for by lower thresholds for starting LT4 in an aging population (7, 8)—prevalence in the USA increased from 9.5% (2012) to 11.7% (2019), with over 78% receiving LT4 alone (9), and (b) a significant proportion of endocrinologists (51%) who are willing to consider prescribing LT3 for these patients (10).
These patients have many symptoms (fatigue, low energy and mood, memory deficits, “brain fog”) and physiological abnormalities (high BMI, abnormal body composition, low resting energy expenditure [REE]), which significantly affect their QoL. Hypothyroid subjects on long-term LT4 alone have (a) a 15% excess of psychological caseness (5), a 5% increase in BMI, increased cholesterol and a 25%–30% increase in cardiovascular and all-cause mortality (10–13), and (b) unphysiological T4/T3 ratios with higher T4 than in the normal population (12, 14). They have many futile consultations and investigations, leading to dissatisfaction with hypothyroidism management. Some of these investigations pertain to excluding other coexisting causes of their symptoms and should be pursued.
The possible causes of persistent symptoms in these patients have been reviewed elsewhere (15, 16). Of these, there is now evidence from animal experiments, population studies, and human experiments to support the “low tissue T3” hypothesis in some (17, 18). It is plausible that this is caused by exogenous LT4 induced inhibition of deiodinase 2 expression (D2, the main enzyme converting intracellular “prohormone” T4 to “active” T3) with differential effects on D2 activity in hypothalamo-pituitary tissues (D2 largely unaffected by excess T4) and peripheral tissues (D2 expression suppressed) (19). These differences in D2 activity normalize serum TSH (the “gold standard” for LT4 dosing), whereas peripheral tissues remain unreplenished with T3 converted from the same LT4. It would therefore seem logical to combine LT3 with LT4 since “tissue T3 lack” may account for their persistent symptoms and poor QoL.
Designing a RCT in hypothyroid subjects on LT4 with persistent symptoms
The primary objective of a high-quality blinded RCT investigating LT3 + LT4 therapy in hypothyroidism is to provide phase 2b efficacy data showing that LT3 + LT4 is superior to LT4 alone in improving persistent symptoms and QoL while restoring physiological thyroid hormone profiles. The use of patient-reported outcome measures (PROMs) as the primary outcome in RCTs is now internationally validated and accepted (20, 21). Data on pharmacokinetics, safety, and secondary outcomes [weight/BMI, body composition, REE, metabolomic profiles, and bone turnover] should also be sought.
Possible reasons for the inconclusive results of previous RCTs have been discussed in detail elsewhere (22, 23). Here, we highlight the important aspects of designing a future RCT that may provide answers to the disputed question of the efficacy of LT3 + LT4 in this situation:
(1) Selection of trial subjects
(a) Presence of symptoms and threshold for recruitment
The primary outcome of any RCT of LT3 + LT4 in hypothyroidism should be their effect on symptoms and QoL, as recommended by expert bodies (22, 23). Identifiable and measurable symptoms “quantifying” an unsatisfactory response to LT4 alone should be present at recruitment despite TSH being within the reference range (22, 24).
This is achieved by the following:
(i) The use of thyroid-specific PROMs: Most studies hitherto have used generic, non-thyroid-specific PROMs such as SF-36, HADS, BDI, and GHQ. They are not adequately responsive to changes in symptoms and QoL in patients with benign thyroid diseases (25). There is now a consensus that thyroid specific PROMs, ThyPRO and ThyPRO39 (a shorter version) are the best PROMs for use in RCTs (21, 22, 25). An even shorter version, the Composite Scale (utilizing 22 of 39 ThyPRO39 items), shows comparable validity and reliability (26). These thyroid-specific PROMs fulfil two important criteria: content validity (relevant and acceptable to patients and physicians) and responsiveness (detecting subtle changes over time) (21, 27–29).
However, patient preference should also be measured as PROMs alone may not accurately capture subtle differences between treatments (21), as it may be affected by the presence/absence of symptoms at recruitment, and thyroid “activity” during the RCT (e.g., LT3 causing iatrogenic hyperthyroidism). One meta-analysis of crossover trials indicated that 48% preferred combination therapy (30), although another suggested that there was no consistent evidence of improved preference, except through chance (31).
(ii) Symptom threshold for recruitment: A ThyPRO Composite scale score of 32 or TSQ >4 has been shown to represent a score that signifies a worse QoL, which is sufficiently above the mean score in patients with hypothyroidism (32). A potential concern about applying a threshold QoL score in recruitment is that individuals with lower scores may have “normalized” their view of their QoL after many years of LT4 treatment, yet still benefit substantially from a change in therapy.
(iii) Quantifying “minimal important change” after treatment: It is important to define the clinical relevance of a change in PROM score in reference to patient perception of benefit. The term “minimal important change” (MIC) is used to define the “smallest change in score in the construct to be measured that patients perceive as important” (33–35). A change of nine points in the ThyPRO Composite score has been identified as a valid MIC (36).
(b) LT4 dose at recruitment
Hypothyroid subjects who take a minimum of 1.2 μg/kg/day (21, 22) or 100 μg/day (for pragmatic reasons), have minimal or no endogenous thyroid function and/or derive most of their thyroid hormone from exogenous LT4 therapy. These individuals also have the greatest distrurbance of their circulating T3/T4 ratio (14). They may be more likely to derive benefit from thyroid hormone replacement, including LT3 + LT4, than those who have residual function. This study also excluded many subjects with subclinical hypothyroidism.
(c) Cause of hypothyroidism
The only patients without any endogenous thyroid function were those who had undergone total thyroidectomy, e.g., patients with treated thyroid cancer (although such patients on suppressive LT4 therapy should be excluded). However, enrolling only them would considerably reduce the generalizability of the results of an RCT, considerably as most subjects on LT4 have Hashimoto’s thyroiditis. Unrestricted enrolment about the etiology of hypothyroidism and later subgroup analysis is a pragmatic solution.
(d) The Thr92Ala polymorphism
Approximately 12%–14% of the UK population is homozygous for a single nucleotide polymorphism (SNP) in the D2 gene (Thr92Ala in its ubiquitination site) (37). The exact mechanism by which the Thr92Ala SNP affects D2 function is unknown because it affects residues distant to the catalytic site, making diminished catalytic activity unlikely. However, it may cause intracellular organelle dysfunction by abnormal translocation to the Golgi apparatus and disruption of mitochondrial function, apoptosis, and growth factor signalling (18, 38). This polymorphism has been shown to be beneficial in the general population (heterozygosity reduces acute lung injury in sepsis by 35% and reduces mortality from COVID-19 by 50%) (39, 40).
The D2 SNP affected symptom load, thyroid hormone levels, and response to LT3 + LT4 in both animal and human studies. It reduces D2 “activity” in the mouse pituitary and HEK-293 cells (38, 41). Mice homozygous for the SNP showed reduced T3 activity in some brain regions associated with behavioral abnormalities (sleep more and reduced physical activity) (38). Methimazole worsened these abnormalities and improved the response to LT3 + LT4.
As expected, human studies have produced significant but occasionally conflicting results: (i) A UK study showed impaired psychological well-being (GHQ) on LT4 alone in homozygotes and a greater response to LT3 + LT4 compared to those with the wild-type allele (37). (ii) A more recent study showed a preference for LT3 + LT4 in patients with either or both monocarboxylase transporter 10 and Thr92Ala-D2 polymorphisms (42). (iii) A study of thyroidectomized patients with at least one Thr92Ala allele demonstrated lower serum T3 levels and normal TSH concentrations (41). (iv) We observed that in individuals on LT4 alone (n = 573), homozygosity for the Thr92Ala polymorphism was associated with reduced QoL (5). (v) A subsequent study failed to replicate our findings but was potentially underpowered—had fewer subjects on LT4 (n = 364) (43). (vi) We have replicated our findings in the HUNT study in Norway (n = 46,712, n = 1,100 on LT4) (44). The Thr92Ala SNP was present in 13% of the population and was not associated with increased HADS scores in subjects not receiving LT4. HADS was 0.71 points higher (0.39–1.02, p <0.001) in subjects on LT4 overall, and 1.83 points higher (0.93–2.73 p <0.001) in those on LT4 who were homozygous for Thr92Ala compared to individuals not on LT4. Thr92Ala non-homozygous individuals on LT4 were 22% more likely than those not on LT4 to reach the threshold for HADS anxiety, whereas homozygous individuals were 208% more likely.
We believe that initial proof of efficacy should be sought in subjects homozygous for the D2 Thr92Ala SNP, as this group has a 3-fold increased symptom burden. Recruiting subjects with symptoms who have the potential to respond well to combination therapy will reduce the size (and cost) of the study and increase its power. Note, however, that even those non-homozygous for Thr92Ala have a 22% increase in symptoms and may benefit from LT3.
In summary, we justified the selection of subjects with the D2 SNP as follows: (1) Subjects homozygous for Thr92Ala have been shown to have a greater symptom burden on LT4 than non-homozygous individuals in two independent populations. (2) They have been shown to have a greater response to LT4 + LT3 than non-homozygous individuals do. (3) However, we have confirmed that non-homozygous individuals also have an excess of psychological morbidity on LT4 compared to the background population, confirming that all subjects with the SNP on LT4 have the potential to benefit from LT3. (4) Power calculations indicate that studies involving Thr92Ala homozygous subjects allow for a 3-fold smaller study, saving time and money.
(2) Selecting a physiological LT3 preparation
In subjects with preserved endogenous thyroid function, serum T3 fluctuations are narrow (45). LT3 preparations that deliver profiles mimicking normal human T3 profiles make it easier to adjust the dose and may enhance safety. Current short-acting LT3 preparations are non-physiological and need to be administered at least two/three times per day to achieve a semblance of a physiological profile.
(a) Current short acting LT3 preparations
(i) Produce unphysiological “peaks”: One reason why previous RCTs may have been inconclusive was the use of “unphysiological” LT3 preparations (21). Current LT3 preparations, including natural desiccated thyroid (NDT), are short-acting (unpublished data) (Figures 1, 2). They last a few hours in the serum and produce “peaks” with persistent T3 levels above the upper limit of the reference range (ULRR) for a significant length of time, the number of peaks depending on the frequency of administration. Of the 16 RCTs for which information was available, nine used a once/day, four used a twice/day, and one used a three/day LT3 regime (Table 1).
(ii) Difficult to monitor for “dose adjustment”: The unphysiological pharmacokinetic profiles and “peaks” produced (Figures 1, 2) do not lend themselves to monitoring of thyroid hormones for dose adjustment. Figure 1 illustrates the variability of serum free T3 at different times before and after oral short-acting LT3, making recommendations about the timing of blood testing for dose adjustment impossible. The current recommendation for measuring trough free T3 levels does not consider the previously mentioned peaks and time spent outside the reference range (ULRR), which may adversely affect patients both in the short and long term. As we recently showed in hypothyroid subjects given NDT (ERFA and Armour thyroid), there was a significant difference in time spent outside the ULRR despite similar trough-free T3 concentrations (unpublished data) (Figure 2).
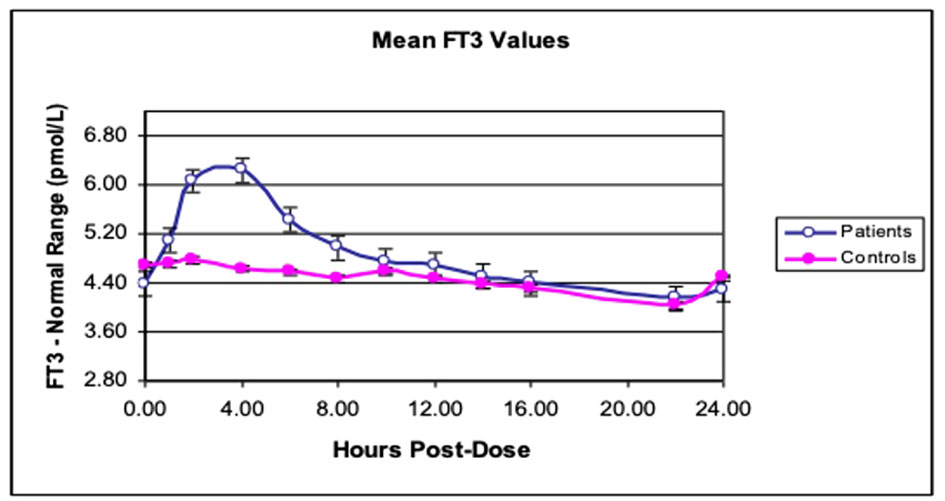
Figure 1 Twenty-four-hour free T3 profiles in hypothyroid subjects. Mean free T3 profiles after giving either LT4 alone (controls) or LT3 + LT4 (patients) in 10 subjects with hypothyroidism. [Reprinted with permission from Saravanan et al. (46)].
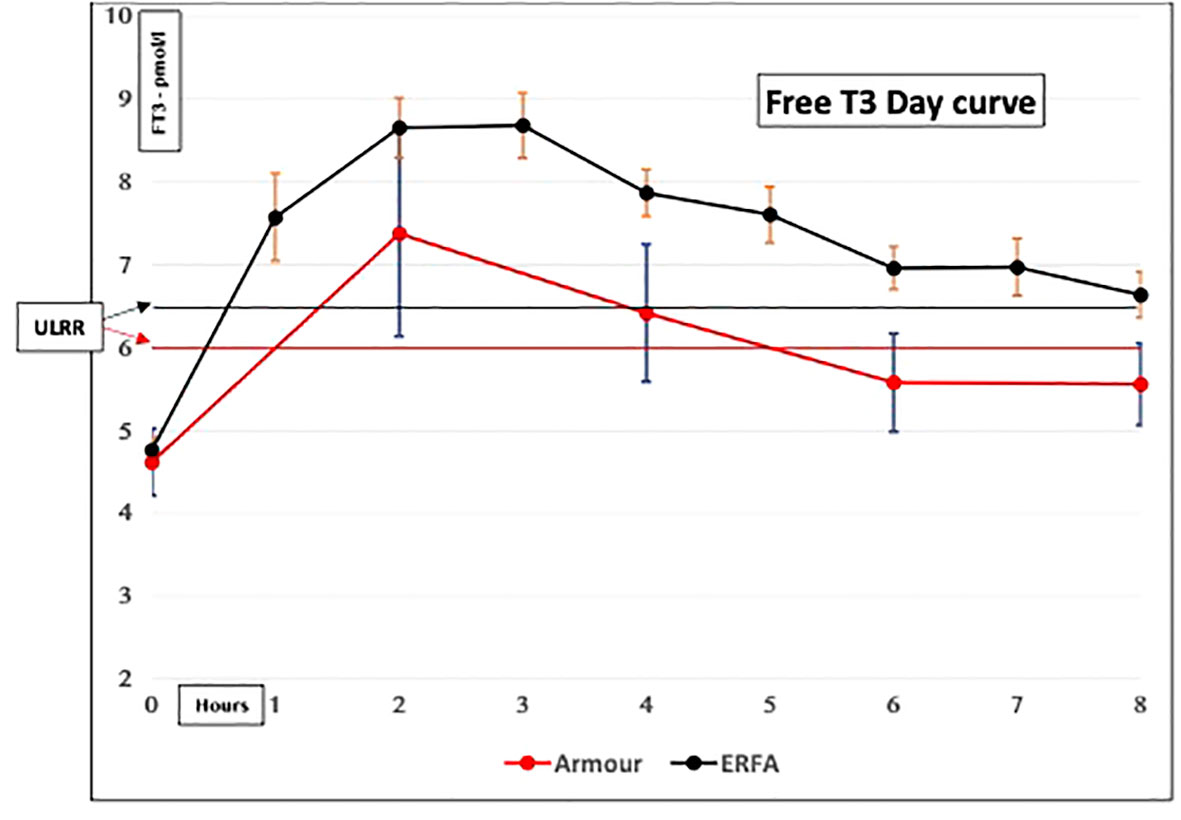
Figure 2 Serum-free T3 concentrations in hypothyroid subjects given natural desiccated thyroid (NDT). Both ERFA and Armour thyroid caused FT3 concentrations to be elevated above the upper limit of the reference range (ULRR) for significant amounts of time (Premawardhana L.D. et al. Oral Presentation, British Thyroid Association, May 2021).
(b) Long acting “physiological” LT3 preparations
There have been calls from Specialist Societies to investigate long acting LT3 in RCTs in symptomatic hypothyroid subjects on LT4 (21, 47). There is a shortage of long-acting preparations with clinical utility (Table 2, known preparations); however, two preparations have undergone preliminary trials.
(i) Poly-zinc-liothyronine (PZL):
PZL is a polymeric compound of zinc and LT3 that adheres to the intestinal mucosa following oral administration (61, 62). This slows down its gut transit time and in essence forms a “depot” for slow release of T3 which is then absorbed into the blood stream (63). A phase 1 trial comparing PZL and LT3 showed a 6-hour plateau in serum T3 Cmax for PZL, which was 30% lower than that for LT3. Furthermore, T3 remained at over half of the Cmax for more than 24 h (52). This preparation may produce more physiologically relevant T3 pharmacokinetics. However, this has not been tested in QoL studies.
(iii) Triiodothyronine sulfate (T3S): Sulfation of the hydroxyl group of T3 generates T3 sulfate (T3S), which is an inactive molecule that is naturally formed during human thyroid hormone metabolism. This targets T3 for destruction by deiodinase 1 (D1). However, if required (e.g., in hypothyroidism), T3S can be desulfated by local sulfatase enzymes (64, 65) or the gut microbiome (66) to regenerate “active” T3.
Sulfation also confers the following qualities to T3S:
(1) Increased solubility targets biliary excretion and reabsorption in enterohepatic circulation. Sulfation and desulfation enzymes are highly expressed in the liver.
(2) T3S is markedly preferred as a substrate over T3 by the D1 deiodinase enzyme expressed in the liver, kidney, and intestine (67).
(3) D1 activity is increased in hyperthyroidism and D2 activity is reduced.
Taken together, these elements create a self-regulating system to stabilize serum T3 levels: if T3 increases, more is sulfated and excreted via the liver and kidney, and increased D1 levels at these sites result in the irreversible destruction of T3S to inactive metabolites. In contrast, in hypothyroidism, when T3 levels are low, D1 activity is low in the liver and intestine, and more T3S excreted in the bile can be reabsorbed and desulfated to restore T3 levels.
When T3S is administered orally, this system generates a favorable pharmacokinetic profile: T3S levels peak and return to baseline in 12 h, but (desulfated) serum T3 levels rise and remain high for over 48 h, likely through enterohepatic recirculation and desulfation. This result is in effect a “slow-release preparation,” as demonstrated in thyroidectomized humans in 2014 (53). Santini et al. also showed in a continuous dosing study over 11 weeks that replacing 25 μg of T4 with 40 μg of T3S in subjects on LT4 monotherapy results in a reduction in serum free T4 without a reduction in free T3. This restores a physiological T4/T3 ratio alongside a normal TSH in 89% (n = 36) of patients as compared to fewer than 45% on LT4 alone (54). A particularly attractive feature of T3S therapy is that, by exploiting natural physiology, it is self-regulating (as described above), with excess levels being rapidly destroyed and/or excreted, generating a very wide therapeutic index and level of safety. Consistent with this, no safety signals were observed in the 11-week study, which was uncontrolled and not designed or powered for clinical endpoints (54).
(3) Appropriate trial design
RCTs provide high-quality evidence, with minimal bias. However, they are difficult to design and execute, expensive, time-consuming, and targeted at specific groups (may therefore be difficult to extrapolate to other groups or to generalize their results).
Several broad principles have been proposed. Such a trial should be randomized (thereby matched for sex, menopausal status, age, etc.), blinded to both participants and investigators (e.g., participants’ thyroid hormone status), and placebo controlled (over-encapsulation or identical preparations). All laboratory assessments should be performed in a single reference laboratory to minimize inter- and intra-assay variations, preferably in a single batch (although this may not be practical). However, safety and thyroid tests for dose adjustment may be performed locally.
A long study duration of 12–24 months is recommended in view of the long half-life of LT4 (approximately 7 days), the time taken to achieve a “steady state” for dose adjustment, and to assess medium-term efficacy and safety for cardiac and bone tissue. However, a long trial may adversely affect enrolment, increase dropout rates, and increase cost.
Of the various trial designs available, a crossover design is attractive to participants, as they are certain to receive the intervention/drug under investigation during one of the trial phases. Furthermore, the ability to perform paired analyses enhances its statistical power, reducing the number of patients needed to be enrolled, and reducing costs. However, “carryover” of effects of one therapy to a different phase of the study, loss, and inability to analyze data if patients drop out are disadvantages. These effects can be mitigated if a parallel-group design is adopted.
Conclusions
The issue of “optimal” treatment for hypothyroidism remains unresolved. LT4 monotherapy satisfies the majority, but a significant minority remain dissatisfied because of persistent symptoms that impair QoL. A trial of LT3 is recommended in them by international specialist societies (30, 47, 68).
Although the theoretical evidence for this recommendation is convincing, evidence for the efficacy of RCTs is limited. However, some limitations of these RCTs should be addressed in future trials: they (i) were underpowered, (ii) used inappropriate outcome measures, (iii) were mainly of short duration, and (iv) used short-acting, unphysiological LT3 preparations. We believe that the availability of long-acting LT3 preparations such as PZL and T3S, overcomes this disadvantage, and their efficacy should be investigated in phase 2b/3 RCTs.
We recommend that in such trials, researchers study subjects with a high symptom burden and propensity to respond better to combination LT3 + LT4 therapy (Box 1). In this regard, subjects with the D2 polymorphism (Thr92Ala) would be ideal, as they fulfilled both criteria. There is a plausible biological role of the D2 polymorphism in determining symptom load and a better response to LT3 + LT4 treatment. This strategy eliminates subjects with the potential for only a minimal, clinically insignificant response to LT3 (e.g., those with subclinical hypothyroidism).
Box 1. Key elements of a trial of combined LT3 + LT4 therapy in hypothyroidism.
Aim: To provide phase 2b/3 efficacy data that combined LT3 + LT4 therapy is superior to LT4 alone
Primary outcome
Symptom improvement using a thyroid specific PROM, e.g., ThyPRO39 or its composite score
Secondary outcomes
1. pharmacokinetic profiles of interventional drug
2. changes to weight/BMI, body composition, REE, metabolomic profiles and bone turnover.
3. safety outcomes
Patient selection
1. presence of symptoms and threshold for recruitment
2. use of thyroid specific PROMs
3. recognizing minimal important change in score
4. higher symptom load and greater response to LT3, e.g., deiodinase 2 snp
LT4 dose at recruitment
Minimum of 1.2 μg/kg/day or 100 μg/day (for pragmatic reasons)
Cause of hypothyroidism
Unrestricted recruitment of subjects with high symptom load and a propensity to respond to treatment
Choice of interventional medication
Long acting LT3 preparations e.g., PZL or T3S
Appropriate Trial design
Randomized placebo-controlled trial using a cross over design
PROM, patient reported outcome measures; snp, single nucleotide polymorphism.
A placebo-controlled, double-blind, crossover RCT would be ideal because of the advantages of such a design—adequately powered with limited numbers, all subjects receiving the active and placebo medications and limiting costs within acceptable limits.
Author contributions
LP: Conceptualization, Writing – original draft, Writing – review & editing. PT: Conceptualization, Writing – review & editing. OO: Conceptualization, Writing – review & editing. MA: Conceptualization, Writing – review & editing. EO: Conceptualization, Writing – review & editing. CD: Writing – original draft, Writing – review & editing, Conceptualization.
Conflict of interest
The authors declare that the research was conducted in the absence of any commercial or financial relationships that could be construed as a potential conflict of interest.
Publisher’s note
All claims expressed in this article are solely those of the authors and do not necessarily represent those of their affiliated organizations, or those of the publisher, the editors and the reviewers. Any product that may be evaluated in this article, or claim that may be made by its manufacturer, is not guaranteed or endorsed by the publisher.
References
1. Millan-Alanis JM, González JG, Flores-Rodríguez A, Singh Ospina N, Maraka S, Moreno-Pena PJ, et al. Benefits and harms of levothyroxine/L-triiodothyronine versus levothyroxine monotherapy for adult patients with hypothyroidism: systematic review and meta-analysis. Thyroid (2021) 11:1613–25. doi: 10.1089/thy.2021.0270
2. Hoang TD, Olsen CH, Mai VQ, Clyde PW, Shakir MKM. Desiccated thyroid extract compared with levothyroxine in the treatment of hypothyroidism: a randomized, double-blind, crossover study. J Clin Endocrinol Metab (2013) 98:1982–90. doi: 10.1210/jc.2012-4107
3. Slawik M, Klawitter B, Meiser E, Schories M, Zwermann O, Borm K, et al. Thyroid hormone replacement for central hypothyroidism: a randomized controlled trial comparing two doses of thyroxine (T4) with a combination of T4 and triiodothyronine. J Clin Endocrinol Metab (2007) 92:4115–22. doi: 10.1210/jc.2007-0297
4. Wekking EM, Appelhof BC, Fliers E, Schene AH, Huyser J, Tijssen JG, et al. Cognitive functioning and wellbeing in euthyroid patients on thyroxine replacement therapy for primary hypothyroidism. Eur J Endocrinol (2005) 153:747–53. doi: 10.1530/eje.1.02025
5. Saravanan P, Chau WF, Roberts N, Vedhara K, Greenwood R, Dayan CM. Psychological well-being in patients on 'adequate' doses of l-thyroxine: results of a large, controlled community-based questionnaire study. Clin Endocrinol (Oxf) (2002) 57:577–85. doi: 10.1046/j.1365-2265.2002.01654.x
6. Panicker V, Evans J, Bjoro T, Asvold BO, Dayan CM, Bjerkeset O. A paradoxical difference in relationship between anxiety, depression and thyroid function in subjects on and not on T4: findings from the HUNT study. Clin Endocrinol (Oxf) (2009) 71:574–80. doi: 10.1111/j.1365-2265.2008.03521.x
7. Taylor PN, Iqbal A, Minassian C, Sayers A, Draman MS, Greenwood R, et al. Falling threshold for treatment of borderline elevated thyrotropin levels-balancing benefits and risks: evidence from a large community-based study. JAMA Intern Med (2014) 174:32–9. doi: 10.1001/jamainternmed.2013.11312
8. Medici BB, Nygaard B, la Cour JL, Grand MK, Siersma V, Nicolaisdottir DR, et al. Changes in prescription routines for treating hypothyroidism between 2001 and 2015: an observational study of 929,684 primary care patients in Copenhagen. Thyroid (2019) 29:910–9. doi: 10.1089/thy.2018.0539
9. Wyne KL, Nair L, Schneiderman CP, Pinsky B, Antunez Flores O, Guo D, et al. Hypothyroidism prevalence in the United States: A retrospective study combining national health and nutrition examination survey and claims data, 2009–2019. J Endocr Soc (2023) 7:1–11. doi: 10.1210/jendso/bvac172
10. Younes YR, Perros P, Hegedüs L, Papini E, Nagy EV, Attanasio R, et al. Use of thyroid hormones in hypothyroid and euthyroid patients: A THESIS questionnaire survey of UK endocrinologists. Clin Endocrinol (Oxf) (2023) 98:238–48. doi: 10.1111/cen.14812
11. Ning Y, Cheng YJ, Liu L, Sara JDS, Cao ZY, Zheng WP, et al. What is the association of hypothyroidism with risks of cardiovascular events and mortality? A meta-analysis of 55 cohort studies involving 1,898,314 participants. BMC Med (2017) 15:21. doi: 10.1186/s12916-017-0777-9
12. Samuels MH, Kolobova I, Smeraglio A, Peters D, Purnell JQ, Schuff KG. Effects of levothyroxine replacement or suppressive therapy on energy expenditure and body composition. Thyroid (2016) 26:347–55. doi: 10.1089/thy.2015.0345
13. McAninch EA, Rajan KB, Miller CH, Bianco AC. Systemic thyroid hormone status during levothyroxine therapy in hypothyroidism: a systematic review and meta-analysis. J Clin Endocrinol Metab (2018) 103:4533–42. doi: 10.1210/jc.2018-01361
14. Gullo D, Latina A, Frasca F, Le Moli R, Pellegriti G, Vigneri R, et al. Levothyroxine monotherapy cannot guarantee euthyroidism in all athyreotic patients. PloS One (2011) 6:e22552. doi: 10.1371/journal.pone.0022552
15. Ettleson MD, Bianco AC. Individualized therapy for hypothyroidism: is T4 enough for everyone? J Clin Endocrinol Metab (2020) 105:e3090–104. doi: 10.1210/clinem/dgaa430
16. Perros P, van der Feltz-Cornelis C, Papini E, Nagy EV, Weetman AP, Hegedus L. The enigma of persistent symptoms in hypothyroid patients treated with levothyroxine: A narrative review. Clin Endocrinol (2023) 98:461–68. doi: 10.1111/cen.14473
17. Escobar-Morreale HF, Obregon MJ, Escobar del Rey F, Morreale de Escobar G. Replacement therapy for hypothyroidism with thyroxine alone does not ensure euthyroidism in all tissues, as studied in thyroidectomized rats. J Clin Invest (1995) 96:2828–38. doi: 10.1172/JCI118353
18. Werneck de Castro JP, Fonseca TL, Ueta CB, McAninch EA, Abdalla S, Wittmann G, et al. Differences in hypothalamic type 2 deiodinase ubiquitination explain localized sensitivity to thyroxine. J Clin Invest (2015) 125:769–81. doi: 10.1172/JCI77588
19. Christoffolete MA, Ribeiro R, Singru P, Fekete C, da Silva WS, Gordon DF, et al. Atypical expression of type 2 iodothyronine deiodinase in thyrotrophs explains the thyroxine-mediated pituitary thyrotropin feedback mechanism. Endocrinol (2006) 147:1735–43. doi: 10.1210/en.2005-1300
20. Watt T. Measuring impact of benign thyroid diseases on quality of life. In: Caplan M, editor. Reference Module in Biomedical Sciences. Amsterdam: Elsevier (2018).
21. Wong CK, Lang BH, Lam CL. A systematic review of quality of thyroid-specific health-related quality-of-life instruments recommends ThyPRO for patients with benign thyroid diseases. J Clin Epidemiol (2016) 78:63–72. doi: 10.1016/j.jclinepi.2016.03.006
22. Jonklaas J, Bianco AC, Cappola AR, Celi FS, Fliers E, Heuer H, et al. Evidence-based use of levothyroxine/liothyronine combinations in treating hypothyroidism: A consensus document. Thyroid (2021) 31:156–82. doi: 10.1089/thy.2020.0720
23. Cappola AR. Design of the optimal trial of combination therapy. Front Endocrinol (Lausanne) (2020) 11:168. doi: 10.3389/fendo.2020.00168
24. Vasileiou M, Gilbert J, Fishburn S, Boelaert K, Guideline Committee. Thyroid disease assessment and management: summary of NICE guidance. Br Med J (2020) 368:m41. doi: 10.1136/bmj.m41
25. Watt T, Cramon PK, Hegedüs L, Bjorner JB, Bonnema SJ, Rasmussen ÅK, et al. The thyroid-related quality of life measure ThyPRO has good responsiveness and ability to detect relevant treatment effects. J Clin Endocrinol Metab (2014) 99:3708–17. doi: 10.1210/jc.2014-1322
26. Watt T, Bjorner JB, Groenvold M, Cramon P, Winther KH, Hegedüs L, et al. Development of a short version of the thyroid-related patient-reported outcome thyPRO. Thyroid (2015) 25:1069–79. doi: 10.1089/thy.2015.0209
27. Watt T, Hegedüs L, Groenvold M, Bjorner JB, Rasmussen AK, Bonnema SJ, et al. Validity and reliability of the novel thyroid-specific quality of life questionnaire, ThyPRO. Eur J Endocrinol (2010) 162:161–7. doi: 10.1530/EJE-09-0521
28. Watt T, Groenvold M, Hegedüs L, Bonnema SJ, Rasmussen ÅK, Feldt-Rasmussen U, et al. Few items in the thyroid-related quality of life instrument ThyPRO exhibited differential item functioning. Qual Life Res (2014) 23:327–38. doi: 10.1007/s11136-013-0462-1
29. Watt T, Groenvold M, Deng N, Gandek B, Feldt-Rasmussen U, Rasmussen ÅK, et al. Confirmatory factor analysis of the thyroid-related quality of life questionnaire ThyPRO. Health Qual Life Outcomes (2014) 12:126. doi: 10.1186/s12955-014-0126-z
30. Wiersinga WM, Duntas L, Fadeyev V, Nygaard B, Vanderpump MP. 2012 ETA guidelines: the use of L-T4 + L-T3 in the treatment of hypothyroidism. Eur Thyroid J (2012) 1:55–71. doi: 10.1159/000339444
31. Akirov A, Fazelzad R, Ezzat S, Thabane L, Sawka AM. A systematic review and meta-analysis of patient preferences for combination thyroid hormone treatment for hypothyroidism. Front Endocrinol (Lausanne) (2019) 24:477. doi: 10.3389/fendo.2019.00477
32. Winther KH, Cramon P, Watt T, Bjorner JB, Ekholm O, Feldt-Rasmussen U, et al. Disease-specific as well as generic quality of life is widely impacted in autoimmune hypothyroidism and improves during the first six months of levothyroxine therapy. PloS One (2016) 11:e0156925. doi: 10.1371/journal.pone.0156925
33. Prinsen CAC, Mokkink LB, Bouter LM, Alonso J, Patrick DL, deVet HCW, et al. COSMIN guideline for systematic reviews of patient reported outcome measures. Qual Life Res (2018) 27:1147–57. doi: 10.1007/s11136-018-1798-3
34. De Vet HCW, Terwee CB, Mokkink LB, Knol DL. Measurement in Medicine. New York: Cambridge University Press (2011).
35. Mokkink LB, Terwee CB, Knoll DL, Stratford PW, Alonso J, Patrick DL, et al. The COSMIN checklist for evaluating the methodological quality of studies on measurement properties: a clarification of its content. BMC Med Res Method (2010) 10:22. doi: 10.1186/1471-2288
36. Nordqvist SF, Boesen VB, Rasmussen AK, Feldt-Rasmussen U, Hegedüs L, Bonnema SJ, et al. Determining minimal important change for the thyroid related quality of life questionnaire ThyPRO. Endo Connect (2021) 10:316–24. doi: 10.1530/EC-21-0026
37. Panicker V, Saravanan P, Vaidya B, Evans J, Hattersley AT, Frayling TM, et al. Common variation in the DIO2 gene predicts baseline psychological wellbeing and response to combination thyroxine plus triiodothyronine therapy in hypothyroid patients. J Clin Endocrinol Metab (2009) 94:1623–9. doi: 10.1210/jc.2008-1301
38. Jo S, Fonseca TL, Bocco BM, Fernandes GW, McAninch EA, Bolin AP, et al. Type 2 deiodinase polymorphism causes ER stress and hypothyroidism in the brain. J Clin Invest (2019) 129:230–45. doi: 10.1172/JCI123176
39. Ma SF, Xie L, Pino-Yanes M, Sammani S, Wade MS, Letsiou E, et al. Type 2 deiodinase and host responses of sepsis and acute lung injury. Am J Respir Cell Mol Biol (2011) 45:1203–11. doi: 10.1165/rcmb.2011-0179OC
40. de Lima Beltrão FE, de Almeida Beltrão DC, Carvalhal G, de Lima Beltrão FE, de Souza Braga Filho J, de Brito Oliveira J, et al. Heterozygote advantage of the type II deiodinase thr92Ala polymorphism on intrahospital mortality of COVID-19. J Clin Endocrinol Metab (2022) 107:e2488–501. doi: 10.1210/clinem/dgac075
41. Castagna MG, Dentice M, Cantara S, Ambrosio R, Maino F, Porcelli T, et al. DIO2 thr92Ala reduces deiodinase-2 activity and serum T3 levels in thyroid deficient patients. J Clin Endocrinol Metab (2017) 102:1623–30. doi: 10.1210/jc.2016-2587
42. Nygaard B, Jensen EW, Kvetny J, Jarløv A, Faber J. Effect of combination therapy with thyroxine (T4) and 3,5,3′-triiodothyronine versus T4 monotherapy in patients with hypothyroidism, a double-blind, randomised cross-over study. Eur J Endocrinol (2009) 161:895–902. doi: 10.1530/EJE-09-0542
43. Wouters HJ, van Loon HC, van der Klauw MM, Elderson MF, Slagter SN, Kobold AM, et al. No effect of the thr92Ala polymorphism of deiodinase-2 on thyroid hormone parameters, health-related quality of life, and cognitive functioning in a large population-based cohort study. Thyroid (2017) 27:147–55. doi: 10.1089/thy.2016.0199
44. Taylor P, Haug E, Heald A, Premawardhana L, Okosieme O, Stedman M, et al. Individuals on levothyroxine have higher HADS anxiety and depression scores than the general population and this is exacerbated by the Thr92Ala substitution in DIO2. Endocr Abstracts (2022) 86:EC1.3. doi: 10.1530/endoabs.86.EC1.3
45. Andersen S, Pedersen KM, Bruun NH, Laurberg P. Narrow individual variations in serum T4 and T3 in normal subjects: a clue to the understanding of subclinical thyroid disease. J Clin Endocrinol Metab (2002) 87:1068–72. doi: 10.1210/jcem.87.3.8165
46. Saravanan P, Siddique H, Simmons DJ, Greenwood R, Dayan CM. Twenty-four hour hormone profiles of TSH, Free T3 and free T4 in hypothyroid patients on combined T3/T4 therapy. Exp Clin Endocrinol Diabetes (2007) 115:261–7. doi: 10.1055/s-2007-973071
47. Jonklaas J, Bianco AC, Bauer AJ, Burman KD, Cappola AR, Celi FS, et al. Guidelines for the treatment of hypothyroidism: prepared by the American Thyroid Association Task Force on thyroid hormone replacement. Thyroid (2014) 4:1670–751. doi: 10.1089/thy.2014.0028
48. Alomari M, Vuddanda PR, Trenfield SJ, DoDoo CC, Velega S, Basit AW, et al. Printing T3 and T4 oral drug combinations as a novel strategy for hypothyroidism. Int J Phar (2018) 549:363–9. doi: 10.1016/j.ijpharm.2018.07.062
49. Bakhteyar H, Cassone C, Kohan HG, Sani SN. Kinetic analysis of drug release from compounded slow-release capsules of liothyronine sodium (T3). Int J Pharm Compd (2017) 21:418–25.
50. Hennemann G, Docter R, Visser TJ, Postema PT, Krenning EP. Thyroxine plus low-dose, slow-release triiodothyronine replacement in hypothyroidism: proof of principle. Thyroid (2004) 14:271–5. doi: 10.1089/105072504323030924
51. Jonklaas J, Burman KD, Wang H, Latham KR. Single-dose T3 administration: kinetics and effects on biochemical and physiological parameters. Ther Drug Monit (2015) 37:110–8. doi: 10.1097/FTD.0000000000000113
52. Dumitrescu AM, Hanlon EC, Arosemena M, Duchon O, Ettleson M, Giurcanu M, et al. Extended absorption of liothyronine from poly-zinc-liothyronine: results from a phase 1, double-blind, randomized, and controlled study in humans. Thyroid (2022) 32(2):196–205. doi: 10.1089/thy.2021.0304
53. Santini. T3 concentrations for 48 hours following T3S. Endocr Pract (2014) 20:680–689. doi: 10.4158/EP13331.OR
54. Santini. Treating hypothyroid patients with L-T4+T3S. Front Endocrinol (2019) 10:826. doi: 10.3389/fendo.2019.00826
55. Bianco AC, Anderson G, Forrest D, Galton VA, Gereben B, Kim BW, et al. American thyroid association guide to investigating thyroid hormone economy and action in rodent and cell models. Thyroid (2014) 24:88–168. doi: 10.1089/thy.2013.0109
56. Sreedharan S, Patel R. (2017). Long-term sustained delivery of liothyronine (L-T3) with subdermal proneuratm implants, in: 87th Annual Meeting of the American Thyroid Association, Vancouver, BC. USA: Thyroid, Mary Ann Liebert Inc
57. Antonica F, Kasprzyk DF, Opitz R, Iacovino M, Liao XH, Dumitrescu AM, et al. Generation of functional thyroid from embryonic stem cells. Nature (2012) 491:66–71. doi: 10.1038/nature11525
58. Kurmann AA, Serra M, Hawkins F, Rankin SA, Mori M, Astapova I, et al. Regeneration of thyroid function by transplantation of differentiated pluripotent stem cells. Cell Stem Cell (2015) 17:527–42. doi: 10.1016/j.stem.2015.09.004
59. Finan B, Clemmensen C, Zhu Z, Stemmer K, Gauthier K, Muller L, et al. Chemical hybridization of glucagon and thyroid hormone optimizes therapeutic impact for metabolic disease. Cell (2016) 167:843–57.e14. doi: 10.1016/j.cell.2016.09.014
60. Mdzinarishvili A, Sutariya V, Talasila PK, Geldenhuys WJ, Sadana P. Engineering triiodothyronine (T3) nanoparticle for use in ischemic brain stroke. Drug Delivery Transl Res (2013) 3:309–17. doi: 10.1007/s13346-012-0117-8
61. Price JD, Piccariello T. Palmer S metal-coordinated pharmaceuticals. Drug Dev Delivery (2014) 14:34–9.
62. Smart JD. The basics and underlying mechanisms of mucoadhesion. Adv Drug Delivery Rev (2005) 57:1556–68. doi: 10.1016/j.addr.2005.07.001
63. Da Conceicao RR, Fernandes GW, Fonseca TL, Bocco B, Bianco AC. Metal coordinated poly-zinc-liothyronine provides stable circulating triiodothyronine levels in hypothyroid rats. Thyroid (2018) 28:1425–33. doi: 10.1089/thy.2018.0205
64. Kung MP, Spaulding SW, Roth JA. Desulfation of 3,5,3-triiodothyronine sulfate by microsomes from human and rat tissues. Endocrinol (1988) 122:1195–200. doi: 10.1210/endo-122-4-1195
65. Santini F, Chopra IJ, Wu SY, Solomon DH, Chua Teco GN. Metabolism of 3,5,3-triiodothyronine sulfate by tissues of the fetal rat: A consideration of the role of desulfation of 3,5,3-triiodothyronine sulfate as a source of T3. Pediatr Res (1992) 31:541–4. doi: 10.1203/00006450-199206000-00001
66. Hazenberg MP, de Herder WW, Visser TJ. Hydrolysis of iodothyronine conjugates by intestinal bacteria. FEMS Microbiol Rev (1988) 4:9–16. doi: 10.1111/j.1574-6968.1988.tb02709.x-i1
67. Foster PA. Steroid sulphatase and its inhibitors: past, present, and future. Molecules (2021) 26:2852. doi: 10.3390/molecules26102852
Keywords: hypothyroidism, combined LT3 and LT4 treatment, deiodinase 2 polymorphism, randomized controlled trial, poor quality of life (QoL)
Citation: Premawardhana LD, Taylor PN, Okosieme OE, Adlan MA, Obuobie EK and Dayan CM (2023) Designing a combined liothyronine (LT3), L- thyroxine (LT4) trial in symptomatic hypothyroid subjects on LT4 - the importance of patient selection, choice of LT3 and trial design. Front. Endocrinol. 14:1282608. doi: 10.3389/fendo.2023.1282608
Received: 24 August 2023; Accepted: 23 October 2023;
Published: 16 November 2023.
Edited by:
Sijie Fang, Shanghai Jiao Tong University, ChinaReviewed by:
Rocky Strollo, Università Telematica San Raffaele, ItalyCopyright © 2023 Premawardhana, Taylor, Okosieme, Adlan, Obuobie and Dayan. This is an open-access article distributed under the terms of the Creative Commons Attribution License (CC BY). The use, distribution or reproduction in other forums is permitted, provided the original author(s) and the copyright owner(s) are credited and that the original publication in this journal is cited, in accordance with accepted academic practice. No use, distribution or reproduction is permitted which does not comply with these terms.
*Correspondence: Lakdasa D. Premawardhana, PremawardhanaLD@cardiff.ac.uk