- 1Guangdong Provincial Key Laboratory of Proteomics, State Key Laboratory of Organ Failure Research, Department of Pathophysiology, School of Basic Medical Sciences, Southern Medical University, Guangzhou, China
- 2Department of Anesthesiology, Nanfang Hospital, Southern Medical University, Guangdong Provincial Key Laboratory of Precision Anaesthesia and Perioperative Organ Protection, Guangzhou, Guangdong, China
- 3School of Traditional Chinese Medicine, Southern Medical University, Guangzhou, Guangdong, China
Background: Many previous studies have revealed a close relationship between lipoprotein metabolism and sepsis, but their causal relationship has, until now, remained unclear. Therefore, we performed a two-sample Mendelian randomization analysis to estimate the causal relationship of lipoprotein-associated phospholipids with the risk of sepsis.
Materials and methods: A two-sample Mendelian randomization (MR) analysis was performed to investigate the causal relationship between lipoprotein-associated phospholipids and sepsis based on large-scale genome-wide association study (GWAS) summary statistics. MR analysis was performed using a variety of methods, including inverse variance weighted as the primary method, MR Egger, weighted median, simple mode, and weighted mode as complementary methods. Further sensitivity analyses were used to test the robustness of the data.
Results: After Bonferroni correction, the results of the MR analysis showed that phospholipids in medium high-density lipoprotein (HDL; ORIVW = 0.82, 95% CI 0.71-0.95, P = 0.0075), large HDL (ORIVW = 0.92, 95% CI 0.85-0.98, P = 0.0148), and very large HDL (ORMR Egger = 0.83, 95% CI 0.72-0.95, P = 0.0134) had suggestive causal relationship associations with sepsis. Sensitivity testing confirmed the accuracy of these findings. There was no clear association between other lipoprotein-associated phospholipids and sepsis risk.
Conclusions: Our MR analysis data suggestively showed a correlation between higher levels of HDL-associated phospholipids and reduced risk of sepsis. Further studies are required to determine the underlying mechanisms behind this relationship.
Introduction
In 2016, the Third International Consensus Definition for Sepsis and Septic Shock (Sepsis-3) defined sepsis as a life-threatening organ dysfunction resulting from dysregulated host responses to infection, and emphasized the primacy of the non-homeostatic host response to infection (1). Sepsis is a life -threatening disease with a high incidence and it remains one of the main causes of death globally. In 2017, an estimated 48.9 million incident cases of sepsis and 11 million sepsis-related deaths were reported worldwide, representing 19.7% of all global deaths (2). Sepsis is therefore significant public health problem with considerable economic consequences.
Based on differences in density, size, lipid and apolipoprotein composition, lipoproteins can be divided into five main subcategories: chylomicrons, very low-density lipoprotein (VLDL), low-density lipoprotein (LDL), intermediate-density lipoprotein (IDL) and high-density lipoprotein (HDL) (3). The lipids found in HDL are mainly surface phospholipids, internal cholesterol esters, and triglycerides. Phospholipids are mainly phosphatidylcholine, lysophosphatidylcholine, and sphingomyelin, each accounting for 32 – 35 mol%, 1.4 – 8.1 mol%, and 5.6 – 6.6 mol% of total lipids in HDL, respectively (4). At present, various activities of HDL have been reported, mainly including anti-inflammatory, anti-oxidative, immunomodulatory, anti-apoptotic, endothelial, and anti-thrombotic functions (5–8). Studies have shown that serum and HDL phospholipids are significantly reduced after a single intravenous dose of endotoxin (9). Phospholipids have also been suggested to play a specific role in HDL’s protective capacity against the pro-inflammatory effects of C-reactive protein (CRP). A study has shown that HDL blocks the upregulation of CRP-induced inflammatory adhesion molecules through its phospholipid components (10). In addition, when recombinant HDL made with HDL apolipoprotein and phosphatidylcholine (PC) instead of saline was administered intravenously 3.5 hours before a single intravenous dose of endotoxin, healthy volunteers showed fewer flu-like symptoms and lower plasma inflammatory cytokines (11). These data suggest that the anti-inflammatory and immunomodulatory effects of HDL are closely related to its phospholipids. Although Mendelian randomization (MR) analysis has shown a causal relationship between high levels of high-density lipoprotein cholesterol and a reduced risk of infectious hospitalizations (12). However, the causal relationship between HDL-related phospholipids and infectious diseases or sepsis is unclear.
One observational study identified a reduction in LDL, HDL, and HDL-associated apolipoproteins in non-survivors of sepsis compared with survivors (13). LDL can reduce sepsis-associated lipopolysaccharide (LPS) damage by binding to LPS (14). In mouse models, the lethal effects of LPS were prevented by removing LDL receptor-induced high endogenous LDL levels (15). Thus, LDL can partially reduce the degree of LPS-induced post-inflammatory acute phase response. Other studies have shown that triglyceride-rich lipoproteins, such as chylomicrons and VLDL, also can inactivate LPS and prevent endotoxin-induced rodent deaths (16–18). IDL is a residual lipoprotein produced by VLDL hydrolysis. In a large study of more than 7,000 participants, endotoxemia was found to be associated with high concentrations of VLDL, IDL, and LDL particles, as well as low concentrations of HDL particles (19). Although the surface of all lipoproteins is composed of phospholipids, mainly PC and sphingomyelins (SM), there are significant differences in phospholipid species for different lipoproteins. Therefore, various lipoproteins and their phospholipids have different functions, and this study aims to explore the causal relationship between lipoprotein- associated phospholipids and sepsis.
Mendelian randomization (MR) refers to a statistical method based on genome-wide association studies (GWAS) that use genetic variation as instrumental variables (IVs) to assess the causality of observed associations between modifiable exposures or risk factors and clinically relevant outcomes (20). MR minimizes traditional confounding and reverses causation because genetic variants are randomly distributed during meiosis and are independent of environment, disease onset, and progression (21). Therefore, MR is not affected by the confounding biases found in traditional observational studies. Based on this knowledge, we applied a two-sample MR analysis to comprehensively investigate the genetic association of lipoprotein-associated phospholipids with sepsis. The results of this study may offer novel strategies for personalized treatments for sepsis.
Materials and methods
MR analysis
MR uses genetic variation as a tool variable to assess non-confounding causal relationships between exposure and outcome and it must satisfy the following three assumptions (22): (1) There is a strong correlation between instrumental variables and exposure factors, (2) there is no connection between instrumental variables and confounding factors, and they are independent of each other, (3) the instrumental variables are associated with outcomes only by exposure, and there is no direct correlation. A flowchart of causal reasoning for lipoprotein-associated phospholipids and sepsis is depicted in Figure 1. In short, lipoprotein-associated phospholipids were classed as the exposure and sepsis was the result. Single nucleotide polymorphisms (SNPs) significantly associated with lipoprotein-associated phospholipids were selected as IVs based on strict inclusion and exclusion criteria. A series of heterogeneity and sensitivity analyses were performed to identify significant associations.
Data sources
This study used publicly available databases. Up to 24,925 individuals were tested in 14 genotype datasets from 10 European studies using additive genetic models. A genome-wide single nucleotide polymorphism (SNP) panel of 39 million genetic markers was tested for univariate associations with the concentrations of lipids and metabolites in 123 humans quantified by high-throughput NMR spectroscopy metabolomics. Individual lipoprotein phospholipids were analyzed using a high-throughput serum Nuclear Magnetic Resonance (NMR) metabolomics platform. The method provided serum measurement information, including lipoprotein subclass distribution and lipoprotein particle concentration, as well as detailed molecular information of serum lipids, including free and esterified cholesterol, sphingomyelin, and fatty acid saturation. (23). Individuals on lipid-lowering medications or pregnant individuals were excluded from the analyses. Genetic statistics for sepsis were derived from the UK Biobank, and we identified 11,643 cases of sepsis, with 474,841 controls of European ancestry. All cases were adjusted for age, sex, chip, and the first 10 principal component analysis.
Selection of IVs
We extracted qualified IVs according to strict selection criteria. First, we selected independent SNPs closely related to various lipoprotein-associated phospholipids and P < 5×10-8 as potential IVs, respectively. Second, the European-based 1,000 Genome Projects reference panel was used to calculate the linkage disequilibrium (LD) and the threshold for clumping was set to R2 < 0.01, while the clumping window size was set to 10,000 kb. Third, SNPs with palindromic and a minor allele frequency (MAF) of less than 0.3 were also eliminated. Finally, IV strength was assessed using the F-statistic to further extract SNPs that were closely related to the exposure. If F > 10, the results should not suffer from weak instrument bias (24).
Statistical analysis
R version 4.3.0 (R Foundation for Statistical Computing, Vienna, Austria) and the Two-Sample MR package were used for statistical analyses (25). P values < 0.05 were to be statistically significant. We performed MR analysis in five different ways: inverse variance weighted (IVW) as the primary method, MR Egger, weighted median, simple mode, and weighted mode as complementary methods (26–28).
We used Cochran’s Q statistic to detect heterogeneity in MR analyses, with P values > 0.05 indicating no heterogeneity (25). If heterogeneity was present, the random-effects model of the IVW method was used. If heterogeneity was absent, then a random-effects analysis was equivalent to a fixed-effect analysis. (29, 30). MR Egger regression was used to examine the effect of horizontal pleiotropy, and P values > 0.05 were indicative of no horizontal pleiotropy (31). Therefore, in the absence of pleiotropy, the IVW analysis method was preferred, and in the presence of pleiotropy, the MR Egger regression method was used (32). MR Egger regression can detect pleiotropy. To test the effect of each SNP on the results, we used leave-one-out analysis to determine whether the estimates were biased or driven by outliers (30). We corrected multiple comparisons using the Bonferroni method and set the statistical significance to P < 0.0042 (0.05/12) based on the number of exposures. If the P value was between 0.0042 and 0.05, we considered suggestive evidence of the potential causal associations (33).
Results
Using the above method, SNPs were screened for this study and details of the selected SNPs are shown in Table S1. Further, the causal effects of each SNP on sepsis are shown in the forest plot (Figure S1).
In the MR analysis, we used a variety of methods to assess the causal relationship between lipoprotein-associated phospholipids and sepsis. The results of the MR analysis suggestively showed that HDL-associated phospholipids are causally related to sepsis (Figure 2). Phospholipids in medium HDL (ORIVW = 0.82, 95% CI 0.71-0.95, P = 0.0075) and phospholipids in large HDL (ORIVW = 0.92, 95% CI 0.85-0.98, P = 0.0148) were negatively associated with sepsis. Due to the pleiotropy of phospholipids in very large HDL, the MR Egger regression method was used (ORMR Egger = 0.83, 95% CI 0.72-0.95, P = 0.0134). However, phospholipids in LDL, IDL, VLDL, and chylomicrons were not causally associated with sepsis (Figure S2; Table S2). Thus, only three HDL-associated phospholipids (phospholipids in medium HDL, phospholipids in large HDL, and phospholipids in very large HDL) suggestively showed significant causal relationship associations with sepsis (Figure 3).
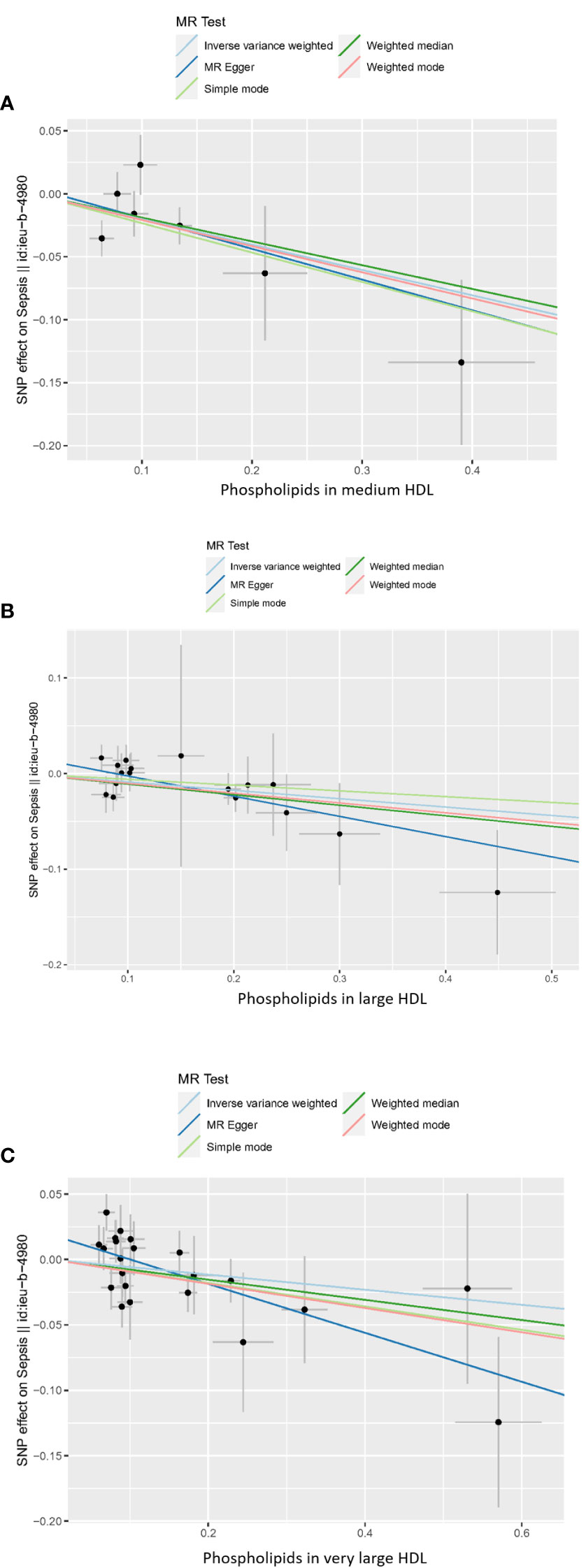
Figure 2 Scatter plot of the causal relationship between HDL-associated phospholipids and sepsis. (A) Phospholipids in medium HDL. (B) Phospholipids in large HDL. (C) Phospholipids in very large HDL. Analyses were conducted using IVW, weighted median, weighted mode, simple mode, and MR Egger methods. The slope of the line indicates the magnitude of the causal relationship. Error bars indicate 95% CI. MR, mendelian randomization; SNP, single nucleotide polymorphism.
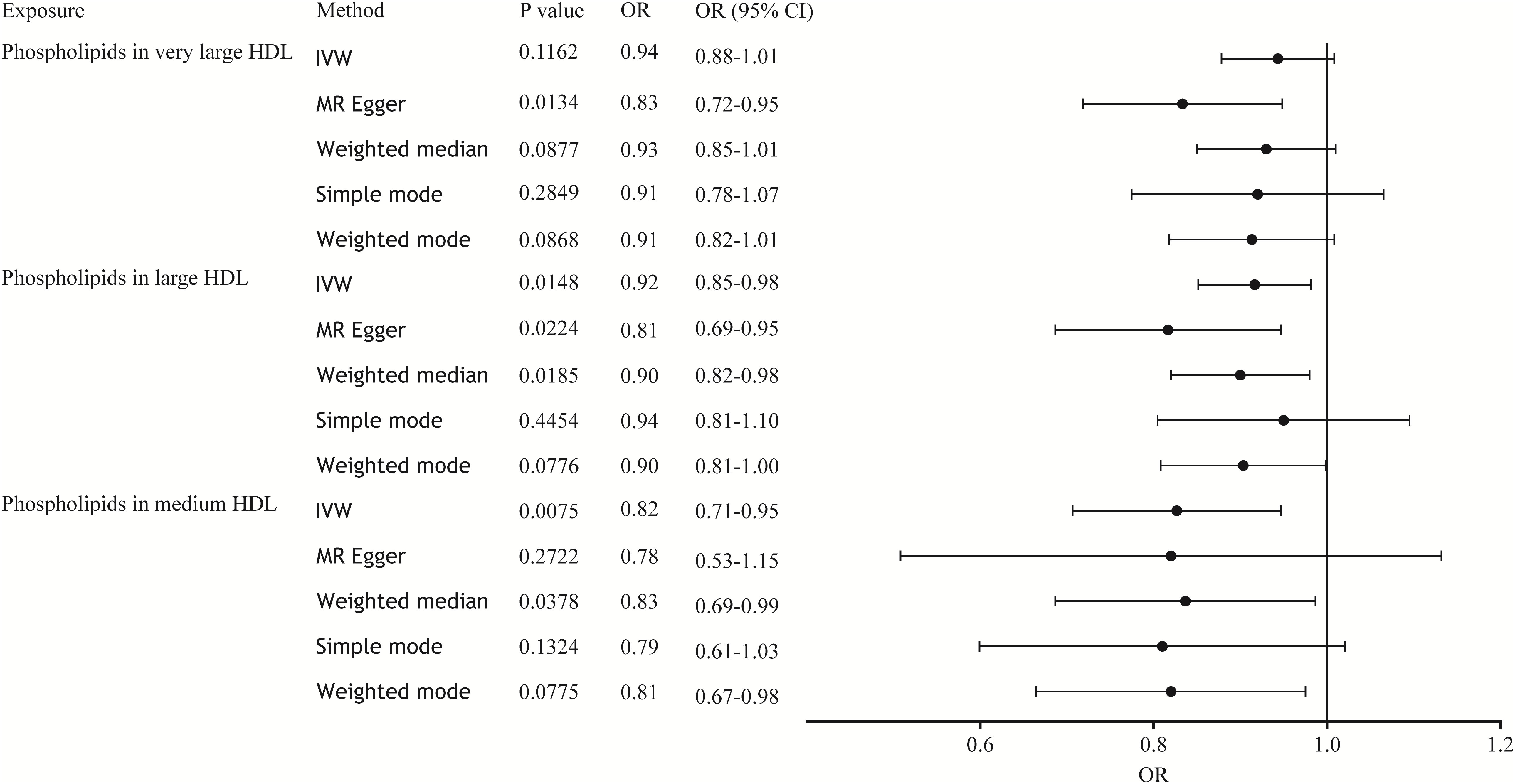
Figure 3 Forest plot of the causal relationship between HDL-associated phospholipids and sepsis. The black dots represent the OR value obtained by each method and the solid line represents the 95% CI. IVW, inverse variance weighted; MR, Mendelian randomization; OR, odds ratio; CI, confidence interval.
In sensitivity analysis, we performed heterogeneity, pleiotropy, and leave-one-out analysis to assess the reliability and robustness of the results (Table 1). Cochran’s Q test showed no heterogeneity between IVs (phospholipids in medium HDL, PIVW = 0.277, PMR Egger = 0.1914; phospholipids in large HDL, PIVW = 0.7786, PMR Egger = 0.8902; phospholipids in very large HDL, PIVW = 0.1084, PMR Egger = 0.2545). The symmetry of the funnel plot also confirmed the absence of heterogeneity, suggesting that causal associations were less likely to be affected by potential bias (Figure S3). Therefore, the random-effects model of the IVW method was used. To reduce bias due to horizontal pleiotropy, we performed MR Egger intercept testing, which showed that overall horizontal pleiotropism was absent in all IVs (phospholipids in medium HDL, P = 0.8224; phospholipids in large HDL, P = 0.1190). When horizontal pleiotropy is present, the MR Egger regression method can be used in MR analysis (phospholipids in very large HDL, P = 0.0435). The leave-one-out analysis (Figure S4) showed no substantial difference in the estimated causal effect when individual SNPs were systematically removed and the MR analysis was repeated. This showed a strong association between exposure and outcomes, thereby validating the reliability of the results of this study.
Discussion
The inference of causality in genetics was usually made by MR analysis. MR analysis was a form of instrumental variable analysis in which genetic marker SNPs were often used as tools to infer the causal effects of exposure variables on outcome variables. SNPs were the basis of genetic polymorphisms and lead to most of the genetic differences between individuals. It was well known that SNPs had a close relationship with disease, and this relationship was the basis for understanding the etiology, medical prevention, and diagnosis of disease. Studies had found that various genetic and environmental factors could lead to abnormalities in blood lipids and lipoproteins, and it could be seen that plasma lipid and lipoprotein concentrations were highly heritable. In addition, in the field of lipid and lipoprotein metabolism, almost 30 different genes encoding key proteins had been implicated, and more than 200 different SNPs had been identified in these genes (34). The genetic variability involved could affect the final plasma lipid levels. The two-sample MR analysis used in this study showed that HDL-associated phospholipids had a suggestive causal relationship with sepsis. There were no clear associations between other lipoprotein-associated phospholipids and sepsis risk, including LDL, IDL, VLDL-associated phospholipids, and phospholipids in chylomicrons.
Sepsis remains the leading cause of morbidity and mortality worldwide, and its pathogenesis is complex and involves multiple interactions between infecting microorganisms and the host. Studies have found a rapid and significant decrease in serum cholesterol, phospholipids, apoB, and apoA-I carried in LDL and HDL during acute phase reactions to endotoxemia and various inflammatory in humans (35, 36). Among them, HDL phospholipids have been shown to selectively decrease, while the number of HDL particles remains unchanged. Phospholipids showed a greater decline of approximately 20%, and a highly statistically significant linear relationship between the percentage reduction in phospholipids and the peak CRP (R2 = 0.97, P = 0.001) (9). Although all lipoproteins bind endotoxins, HDL is the most protective because it is rich in surface phospholipids (37). HDL is the main carrier of phospholipids in lipoproteins and has significant endotoxin neutralization ability. Infusion of HDL prevented the fatal consequences of LPS administration in mice (38) and prevented LPS-induced cytokine production in rabbits (39) and human volunteers (40). Sphingosine 1-phosphate (S1P) is a lipid-signaling molecule and approximately 55% and 35% of plasma S1P is partitioned into HDL and albumin, respectively (41). As an extracellular and intracellular messenger, S1P regulates the pathophysiological processes involved in sepsis progression. In patients with sepsis, serum S1P levels were significantly reduced, leading to sepsis capillary leakage, impaired tissue perfusion, and organ failure, which are all inversely correlated with disease severity (42). Other HDL-associated sphingolipids, such as sphingosinephosphorylcholine and lysosulfatide may also enhance endothelial cell migration, survival, and the cytoprotective effects of HDL (43). It could be seen that the serum HDL-associated sphingolipids level can predict the prognosis of sepsis, which provides ideas for the clinical treatment of sepsis patients.
One study found that phospholipid transfer active protein (PLTP) and endothelial lipase (EL) were significantly higher than in patients with non-sepsis (44). This lipase plays a major role in hydrolyzing the phospholipids in HDL (45). Studies have also shown that increased PLTP activity might promote phospholipid transfer from HDL to tissues (46). Increased transfer of HDL phospholipids to tissues might contribute to the regeneration of damaged cell membranes and lung surfactants, and endotoxins bound to HDL phospholipids might be excreted into the bile (47). Thus, increased PLTP activity during inflammation may be a protective mechanism that can attenuate the LPS response by modulating HDL phospholipids. This study revealed the potential causal role of HDL-associated phospholipids in sepsis, suggesting that therapeutic strategies to increase serum levels of HDL-associated phospholipids might be beneficial in patients with sepsis. However, further studies are required to improve our understanding of the mechanisms by which HDL-associated phospholipids affect various aspects of sepsis pathology.
Our MR analysis showed a correlation between elevated HDL-associated phospholipid levels and a reduced risk of sepsis. HDL was the main carrier of phospholipids in lipoproteins and had a significant endotoxin neutralizing ability. The core functions of HDL were considered to be antioxidant and anti-inflammatory (48). In addition, studies had shown that the antioxidant activity of HDL could be significantly affected by the modulation of HDL surface lipids. The surface phospholipid composition of HDL also influenced the anti-inflammatory, anti-apoptotic and anti-infective effects of HDL (49). In conclusion, not only did the content of HDL-associated phospholipids and their ability to neutralize endotoxins contributed to the prevention of sepsis, but their antioxidant, anti-inflammatory, anti-apoptosis, and anti-infective effects were also beneficial for sepsis. Further research is needed to identify the underlying mechanisms behind this relationship.
This study had several strengths, including resistance to confounding factors in traditional epidemiology. We used a variety of MR analysis methods to obtain more reliable data. This study was more efficient and less costly than RCTs. In addition, instrumental variables with larger sample sizes were selected from recent GWAS studies to ensure adequate statistical power. However, it is important to note the limitations of this study. As our study only included European populations, the data do not apply to extrapolations from other non-European populations. Further research on more diverse populations is required.
Conclusion
Herein, we identified suggestive causal relationship associations between HDL-associated phospholipids levels and the risk of sepsis. These data could aid the development of novel strategies for the diagnosis and treatment of sepsis. However, more research is needed to further explore this question.
Data availability statement
Publicly available datasets were analyzed in this study. This data can be found here: Summary statistics were publicly available in the GWAS catalog (https://www.ebi.ac.uk).
Author contributions
LZ: Data curation, Writing – original draft, Writing – review & editing. HT: Methodology, Writing – review & editing. JC: Investigation, Methodology, Writing – review & editing. YiD: Validation, Writing – review & editing. YZ: Validation, Writing – review & editing. HL: Software, Writing – review & editing. YW: Investigation, Writing – review & editing. YP: Investigation, Writing – review & editing. YoD: Conceptualization, Funding acquisition, Project administration, Writing – original draft, Writing – review & editing.
Funding
The author(s) declare financial support was received for the research, authorship, and/or publication of this article. This study was supported by the National Natural Science Foundation of China (32170693).
Conflict of interest
The authors declare that the research was conducted in the absence of any commercial or financial relationships that could be construed as a potential conflict of interest.
Publisher’s note
All claims expressed in this article are solely those of the authors and do not necessarily represent those of their affiliated organizations, or those of the publisher, the editors and the reviewers. Any product that may be evaluated in this article, or claim that may be made by its manufacturer, is not guaranteed or endorsed by the publisher.
Supplementary material
The Supplementary Material for this article can be found online at: https://www.frontiersin.org/articles/10.3389/fendo.2023.1275132/full#supplementary-material
Abbreviations
MR, Mendelian randomization; GWAS, Genome-wide association study; IVW, Inverse variance weighted; MR-Egger, Mendelian randomization-Egger; OR, Odds ratio; CI, Confidence interval; SNP, Single nucleotide polymorphism; IV, Instrumental variable; HDL, High-density lipoprotein; LDL, Low-density lipoprotein; IDL, Intermediate-density lipoprotein; VLDL, Very low-density lipoprotein; LPS, Lipopolysaccharide; CRP, C-reactive protein; PC, Phosphatidylcholine; SM, Sphingomyelin; MAF, Minor allele frequency; LD, Linkage disequilibrium; S1P, Sphingosine 1-phosphate; PLTP, Phospholipid transfer active protein; EL, Endothelial lipase.
References
1. Singer M, Deutschman CS, Seymour CW, Shankar-Hari M, Annane D, Bauer M, et al. The third international consensus definitions for sepsis and septic shock (Sepsis-3). JAMA (2016) 315:801–10. doi: 10.1001/jama.2016.0287
2. Rudd KE, Johnson SC, Agesa KM, Shackelford KA, Tsoi D, Kievlan DR, et al. Global, regional, and national sepsis incidence and mortality 1990-2017: analysis for the global burden of disease study. Lancet (2020) 395:200–11. doi: 10.1016/S0140-6736(19)32989-7
3. Ding WY, Protty MB, Davies IG, Lip G. Relationship between lipoproteins, thrombosis, and atrial fibrillation. Cardiovasc Res (2022) 118:716–31. doi: 10.1093/cvr/cvab017
4. Qin S. LDL and HDL oxidative modification and atherosclerosis. Adv Exp Med Biol (2020) 1276:157–69. doi: 10.1007/978-981-15-6082-8_10
5. Mackness MI, Durrington PN, Mackness B. How high-density lipoprotein protects against the effects of lipid peroxidation. Curr Opin Lipidol (2000) 11:383–8. doi: 10.1097/00041433-200008000-00007
6. Barter PJ, Nicholls S, Rye KA, Anantharamaiah GM, Navab M, Fogelman AM. Antiinflammatory properties of HDL. Circ Res (2004) 95:764–72. doi: 10.1161/01.RES.0000146094.59640.13
7. Mineo C, Deguchi H, Griffin JH, Shaul PW. Endothelial and antithrombotic actions of HDL. Circ Res (2006) 98:1352–64. doi: 10.1161/01.RES.0000225982.01988.93
8. Marsche G, Saemann MD, Heinemann A, Holzer M. Inflammation alters HDL composition and function: implications for HDL-raising therapies. Pharmacol Ther (2013) 137:341–51. doi: 10.1016/j.pharmthera.2012.12.001
9. Hudgins LC, Parker TS, Levine DM, Gordon BR, Saal SD, Jiang XC, et al. A single intravenous dose of endotoxin rapidly alters serum lipoproteins and lipid transfer proteins in normal volunteers. J Lipid Res (2003) 44:1489–98. doi: 10.1194/jlr.M200440-JLR200
10. Wadham C, Albanese N, Roberts J, Wang L, Bagley CJ, Gamble JR, et al. High-density lipoproteins neutralize C-reactive protein proinflammatory activity. Circulation (2004) 109:2116–22. doi: 10.1161/01.CIR.0000127419.45975.26
11. Pajkrt D, Doran JE, Koster F, Lerch PG, Arnet B, van der Poll T, et al. Antiinflammatory effects of reconstituted high-density lipoprotein during human endotoxemia. J Exp Med (1996) 184:1601–8. doi: 10.1084/jem.184.5.1601
12. Trinder M, Walley KR, Boyd JH, Brunham LR. Causal inference for genetically determined levels of high-density lipoprotein cholesterol and risk of infectious disease. Arterioscler Thromb Vasc Biol (2020) 40:267–78. doi: 10.1161/ATVBAHA.119.313381
13. Barlage S, Gnewuch C, Liebisch G, Wolf Z, Audebert FX, Gluck T, et al. Changes in HDL-associated apolipoproteins relate to mortality in human sepsis and correlate to monocyte and platelet activation. Intensive Care Med (2009) 35:1877–85. doi: 10.1007/s00134-009-1609-y
14. Shor R, Wainstein J, Oz D, Boaz M, Matas Z, Fux A, et al. Low serum LDL cholesterol levels and the risk of fever, sepsis, and Malignancy. Ann Clin Lab Sci (2007) 37:343–8.
15. Netea MG, Demacker PN, Kullberg BJ, Boerman OC, Verschueren I, Stalenhoef AF, et al. Low-density lipoprotein receptor-deficient mice are protected against lethal endotoxemia and severe gram-negative infections. J Clin Invest (1996) 97:1366–72. doi: 10.1172/JCI118556
16. Harris HW, Grunfeld C, Feingold KR, Rapp JH. Human very low density lipoproteins and chylomicrons can protect against endotoxin-induced death in mice. J Clin Invest (1990) 86:696–702. doi: 10.1172/JCI114765
17. Harris HW, Grunfeld C, Feingold KR, Read TE, Kane JP, Jones AL, et al. Chylomicrons alter the fate of endotoxin, decreasing tumor necrosis factor release and preventing death. J Clin Invest (1993) 91:1028–34. doi: 10.1172/JCI116259
18. Read TE, Grunfeld C, Kumwenda ZL, Calhoun MC, Kane JP, Feingold KR, et al. Triglyceride-rich lipoproteins prevent septic death in rats. J Exp Med (1995) 182:267–72. doi: 10.1084/jem.182.1.267
19. Maatta AM, Salminen A, Pietiainen M, Leskela J, Palviainen T, Sattler W, et al. Endotoxemia is associated with an adverse metabolic profile. Innate Immun (2021) 27:3–14. doi: 10.1177/1753425920971702
20. Sekula P, Del GMF, Pattaro C, Kottgen A. Mendelian randomization as an approach to assess causality using observational data. J Am Soc Nephrol (2016) 27:3253–65. doi: 10.1681/ASN.2016010098
21. Lawlor DA, Harbord RM, Sterne JA, Timpson N, Davey SG. Mendelian randomization: using genes as instruments for making causal inferences in epidemiology. Stat Med (2008) 27:1133–63. doi: 10.1002/sim.3034
22. Smith GD, Ebrahim S. 'Mendelian randomization': can genetic epidemiology contribute to understanding environmental determinants of disease? Int J Epidemiol (2003) 32:1–22. doi: 10.1093/ije/dyg070
23. Kettunen J, Demirkan A, Wurtz P, Draisma HH, Haller T, Rawal R, et al. Genome-wide study for circulating metabolites identifies 62 loci and reveals novel systemic effects of LPA. Nat Commun (2016) 7:11122. doi: 10.1038/ncomms11122
24. Pierce BL, Burgess S. Efficient design for Mendelian randomization studies: subsample and 2-sample instrumental variable estimators. Am J Epidemiol (2013) 178:1177–84. doi: 10.1093/aje/kwt084
25. Hemani G, Zheng J, Elsworth B, Wade KH, Haberland V, Baird D, et al. The MR-Base platform supports systematic causal inference across the human phenome. eLife (2018) 7:e34408. doi: 10.7554/eLife.34408
26. Bowden J, Davey SG, Burgess S. Mendelian randomization with invalid instruments: effect estimation and bias detection through Egger regression. Int J Epidemiol (2015) 44:512–25. doi: 10.1093/ije/dyv080
27. Burgess S, Scott RA, Timpson NJ, Davey SG, Thompson SG. Using published data in Mendelian randomization: a blueprint for efficient identification of causal risk factors. Eur J Epidemiol (2015) 30:543–52. doi: 10.1007/s10654-015-0011-z
28. Bowden J, Davey SG, Haycock PC, Burgess S. Consistent estimation in Mendelian randomization with some invalid instruments using a weighted median estimator. Genet Epidemiol (2016) 40:304–14. doi: 10.1002/gepi.21965
29. Burgess S, Thompson SG. Interpreting findings from Mendelian randomization using the MR-Egger method. Eur J Epidemiol (2017) 32:377–89. doi: 10.1007/s10654-017-0255-x
30. Burgess S, Bowden J, Fall T, Ingelsson E, Thompson SG. Sensitivity analyses for robust causal inference from Mendelian randomization analyses with multiple genetic variants. Epidemiology (2017) 28:30–42. doi: 10.1097/EDE.0000000000000559
31. Bowden J, Del GMF, Minelli C, Davey SG, Sheehan NA, Thompson JR. Assessing the suitability of summary data for two-sample Mendelian randomization analyses using MR-Egger regression: the role of the I2 statistic. Int J Epidemiol (2016) 45:1961–74. doi: 10.1093/ije/dyw220
32. Sproviero W, Winchester L, Newby D, Fernandes M, Shi L, Goodday SM, et al. High blood pressure and risk of dementia: A two-sample mendelian randomization study in the UK biobank. Biol Psychiatry (2021) 89:817–24. doi: 10.1016/j.biopsych.2020.12.015
33. Larsson SC, Traylor M, Malik R, Dichgans M, Burgess S, Markus HS. Modifiable pathways in Alzheimer's disease: Mendelian randomization analysis. BMJ (2017) 359:j5375. doi: 10.1136/bmj.j5375
34. Garcia-Rios A, Perez-Martinez P, Delgado-Lista J, Lopez-Miranda J, Perez-Jimenez F. Nutrigenetics of the lipoprotein metabolism. Mol Nutr Food Res (2012) 56:171–83. doi: 10.1002/mnfr.201100513
35. Sammalkorpi K, Valtonen V, Kerttula Y, Nikkila E, Taskinen MR. Changes in serum lipoprotein pattern induced by acute infections. Metabolism (1988) 37:859–65. doi: 10.1016/0026-0495(88)90120-5
36. Barlage S, Frohlich D, Bottcher A, Jauhiainen M, Muller HP, Noetzel F, et al. ApoE-containing high density lipoproteins and phospholipid transfer protein activity increase in patients with a systemic inflammatory response. J Lipid Res (2001) 42:281–90. doi: 10.1016/S0022-2275(20)31690-4
37. Parker TS, Levine DM, Chang JC, Laxer J, Coffin CC, Rubin AL. Reconstituted high-density lipoprotein neutralizes gram-negative bacterial lipopolysaccharides in human whole blood. Infect Immun (1995) 63:253–8. doi: 10.1128/iai.63.1.253-258.1995
38. Levine DM, Parker TS, Donnelly TM, Walsh A, Rubin AL. In vivo protection against endotoxin by plasma high density lipoprotein. Proc Natl Acad Sci USA (1993) 90:12040–4. doi: 10.1073/pnas.90.24.12040
39. Hubsch AP, Powell FS, Lerch PG, Doran JE. A reconstituted, apolipoprotein A-I containing lipoprotein reduces tumor necrosis factor release and attenuates shock in endotoxemic rabbits. Circ Shock (1993) 40:14–23.
40. Pajkrt D, Lerch PG, van der Poll T, Levi M, Illi M, Doran JE, et al. Differential effects of reconstituted high-density lipoprotein on coagulation, fibrinolysis and platelet activation during human endotoxemia. Thromb Haemost (1997) 77:303–7. doi: 10.1055/s-0038-1655958
41. Murata N, Sato K, Kon J, Tomura H, Yanagita M, Kuwabara A, et al. Interaction of sphingosine 1-phosphate with plasma components, including lipoproteins, regulates the lipid receptor-mediated actions. Biochem J (2000) 352 Pt 3:809–15. doi: 10.1042/bj3520809
42. Winkler MS, Nierhaus A, Holzmann M, Mudersbach E, Bauer A, Robbe L, et al. Decreased serum concentrations of sphingosine-1-phosphate in sepsis. Crit Care (2015) 19:372. doi: 10.1186/s13054-015-1089-0
43. Kimura T, Sato K, Malchinkhuu E, Tomura H, Tamama K, Kuwabara A, et al. High-density lipoprotein stimulates endothelial cell migration and survival through sphingosine 1-phosphate and its receptors. Arterioscler Thromb Vasc Biol (2003) 23:1283–8. doi: 10.1161/01.ATV.0000079011.67194.5A
44. Reisinger AC, Schuller M, Sourij H, Stadler JT, Hackl G, Eller P, et al. Impact of sepsis on high-density lipoprotein metabolism. Front Cell Dev Biol (2021) 9:795460. doi: 10.3389/fcell.2021.795460
45. Yasuda T, Ishida T, Rader DJ. Update on the role of endothelial lipase in high-density lipoprotein metabolism, reverse cholesterol transport, and atherosclerosis. Circ J (2010) 74:2263–70. doi: 10.1253/circj.CJ-10-0934
46. Pownall HJ, Hickson-Bick D, Massey JB. Effects of hydrophobicity on turnover of plasma high density lipoproteins labeled with phosphatidylcholine ethers in the rat. J Lipid Res (1991) 32:793–800. doi: 10.1016/S0022-2275(20)42031-0
47. Mimura Y, Sakisaka S, Harada M, Sata M, Tanikawa K. Role of hepatocytes in direct clearance of lipopolysaccharide in rats. Gastroenterology (1995) 109:1969–76. doi: 10.1016/0016-5085(95)90765-3
48. Fazio S, Pamir N. HDL particle size and functional heterogeneity. Circ Res (2016) 119:704–7. doi: 10.1161/CIRCRESAHA.116.309506
Keywords: lipoprotein-associated phospholipids, sepsis, Mendelian randomization, causal relationship, genetics
Citation: Zeng L, Tang H, Chen J, Deng Y, Zhao Y, Lei H, Wan Y, Pan Y and Deng Y (2024) Causal association of lipoprotein-associated phospholipids on the risk of sepsis: a Mendelian randomization study. Front. Endocrinol. 14:1275132. doi: 10.3389/fendo.2023.1275132
Received: 09 August 2023; Accepted: 19 December 2023;
Published: 11 January 2024.
Edited by:
Princy Francis, Mayo Clinic, United StatesReviewed by:
Jesus M. Martin-Campos, Institut de Recerca de l’Hospital de la Santa Creu i Sant Pau, SpainRobert Kiss, McGill University, Canada
Copyright © 2024 Zeng, Tang, Chen, Deng, Zhao, Lei, Wan, Pan and Deng. This is an open-access article distributed under the terms of the Creative Commons Attribution License (CC BY). The use, distribution or reproduction in other forums is permitted, provided the original author(s) and the copyright owner(s) are credited and that the original publication in this journal is cited, in accordance with accepted academic practice. No use, distribution or reproduction is permitted which does not comply with these terms.
*Correspondence: Yongqiang Deng, eW9yazYzMzJAMTYzLmNvbQ==
†These authors have contributed equally to this work