- 1Department of Biotechnology, Smt. S.S. Patel Nootan Science and Commerce College, Sankalchand Patel University, Visnagar, Gujarat, India
- 2Department of Electrical Engineering, National Taipei University of Technology, Taipei, Taiwan
- 3Department of Life Sciences, Hemchandracharya North Gujarat University, Gujarat, India
- 4Department of Veterinary Clinical Sciences, College of Veterinary Medicine, Iowa State University, Ames, IA, United States
Oxidative stress, resulting from dysregulation in the secretion of adrenal hormones, represents a major concern in human health. The present review comprehensively examines various categories of endocrine dysregulation within the adrenal glands, encompassing glucocorticoids, mineralocorticoids, and androgens. Additionally, a comprehensive account of adrenal hormone disorders, including adrenal insufficiency, Cushing’s syndrome, and adrenal tumors, is presented, with particular emphasis on their intricate association with oxidative stress. The review also delves into an examination of various nutritional antioxidants, namely vitamin C, vitamin E, carotenoids, selenium, zinc, polyphenols, coenzyme Q10, and probiotics, and elucidates their role in mitigating the adverse effects of oxidative stress arising from imbalances in adrenal hormone levels. In conclusion, harnessing the power of nutritional antioxidants has the potential to help with oxidative stress caused by an imbalance in adrenal hormones. This could lead to new research and therapeutic interventions.
1 Introduction
Adrenal hormone imbalance or dysfunction refers to a condition characterized by aberrant production or regulation of hormones such as cortisol, aldosterone, and dehydroepiandrosterone (DHEA) inside the body. The presence of this imbalance can significantly impact various physiological processes, resulting in a diverse array of health complications (1). Oxidative stress, which occurs when the body’s antioxidant defence systems cannot neutralize reactive oxygen species (ROS), is one of the main causes of these negative effects (abnormal production or regulation of hormones such as cortisol, aldosterone, and DHEA) (2). A study with human cells found that too much glucocorticoid causes too much ROS to be made, which disturbs the balance of metabolic processes and changes the way the vascular endothelium looks and works (3).
Endogenous antioxidant systems control ROS, chemically reactive molecules produced by cellular metabolism (4). However, when there is an imbalance in the adrenal hormones, this delicate balance is disturbed, which results in increased ROS generation and reduced antioxidant defences (5). The aforementioned imbalance may arise due to factors such as chronic anxiety, hormone dysregulation, environmental pollutants, and suboptimal dietary selections (6). Through increased mitochondrial respiration and oxidative phosphorylation, glucocorticoids directly cause oxidative stress in neurons. The incubation of cortical neurons with acute corticosterone resulted in a dose- and time-dependent increase in mitochondrial oxidation, membrane potential, and calcium-holding capacity (7).
Oxidative stress caused by an imbalance in the adrenal hormones has many effects. Oxidative stress can damage lipids, proteins, and DNA, which can cause cellular dysfunction and tissue damage (5). Furthermore, it can turn on inflammatory pathways and mess up the complex signaling networks needed to keep physiology in balance (8). Consequently, adrenal hormone-related diseases like adrenal insufficiency and Cushing’s syndrome often show signs of oxidative stress, like fatigue, immune dysfunction, cognitive impairment, and accelerated aging (9).
A crucial part of physiological balance is the complicated relationship between antioxidants in the diet and oxidative stress-induced adrenal hormone imbalance (10). The finely tuned regulation of adrenal hormones can be disrupted by oxidative stress, which is caused by an imbalance between reactive oxygen species and the body’s antioxidant defense mechanisms (2). Antioxidants serve an important role in preventing oxidative damage by neutralizing free radicals and protecting the delicate equilibrium of the adrenal glands. Adrenaline hormones such as cortisol and adrenaline, which are essential for stress response and overall hormonal harmony, may be dysregulated when this balance is disrupted. Nutritional antioxidants are bioactive substances found in different foods that can eliminate ROS and boost the body’s own antioxidant defences. Some of these molecules are vitamins (like C and E), minerals (like selenium and zinc), phytochemicals (like polyphenols and carotenoids), and other parts of food (11).
The primary objective of this review is to elucidate the mechanisms by which adrenal hormone imbalance induces oxidative stress and investigate the potential contributions of nutritional antioxidants in mitigating such imbalances. Understanding the intricate interplay between adrenal hormone imbalance, oxidative stress, and nutritional antioxidants can give novel insights regarding therapeutic modalities for disorders associated with adrenal hormones, thereby enhancing holistic well-being.
2 Overview of the adrenal gland
The adrenal glands, which are located atop each kidney, are important components of the endocrine system, playing a key role in maintaining homeostasis and responding to stress. Each adrenal gland is divided into two sections: the outer adrenal cortex and the inner adrenal medulla (12). The adrenal cortex is further subdivided into three zones, each of which is responsible for the production of a distinct hormone. Mineralocorticoids, primarily aldosterone, are produced by the outermost zona glomerulosa and regulate electrolyte balance and blood pressure. The zona fasciculata produces glucocorticoids, particularly cortisol, which are involved in glucose metabolism, anti-inflammatory responses, and stress management. Androgens are produced by the innermost zona reticularis, which aids in the development of secondary sexual characteristics (13) (Figure 1).
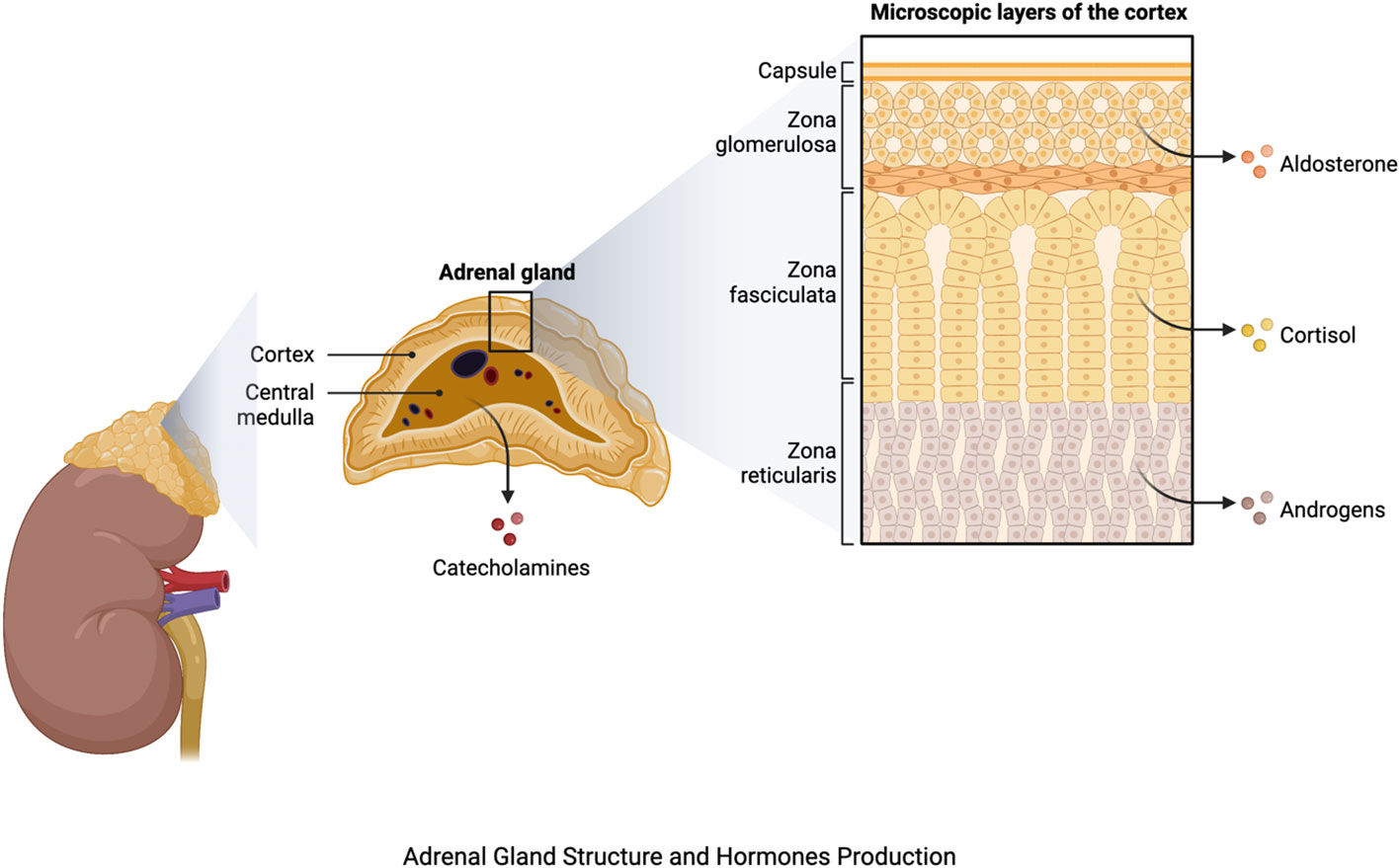
Figure 1 Adrenal gland structure and hormone production. The figure was produced with BioRender (Biorender.com; accessed on 29th July 2023).
The adrenal medulla, an extension of the sympathetic nervous system, on the other hand, produces catecholamines such as epinephrine (adrenaline) and norepinephrine (14) (Figure 1), which have widespread impacts on the cardiovascular system, metabolism, and other body systems. They cause the bloodstream to release glucose and fatty acids, priming the body for increased activity. Furthermore, these hormones increase bronchiole dilation, resulting in enhanced oxygen uptake (15). The hormones produced by the adrenal glands are many and diverse, regulating a wide range of physiological functions. Aldosterone influences blood pressure and electrolyte balance through regulating sodium and potassium levels (16). Cortisol has an impact on metabolism, immunological function, and the body’s reaction to stress. Androgens play a role in the development of secondary sexual characteristics in men (17).
Furthermore, the adrenal glands produce dehydroepiandrosterone (DHEA) and its sulfate (DHEA-S), which are precursors of sex hormones that influence sexual development and reproductive function. The interplay of these hormones is complex, regulating a wide range of physiological processes and contributing to the body’s ability to adapt to both short-term and long-term stressors (18).
3 General overview of adrenal hormone disorders
Adrenal hormone disorders, also called adrenal gland disorders, are characterized by dysfunction or imbalance in the hormones produced by the adrenal glands. The adrenal glands are located on top of the kidneys and are responsible for producing hormones that regulate numerous physiological processes. Common adrenal hormone disorders include adrenal insufficiency, Cushing’s syndrome, and adrenal tumors (19).
3.1 Adrenal insufficiency
When the adrenal glands do not produce enough cortisol and, occasionally, aldosterone, it is known as adrenal insufficiency (20). Adrenal insufficiency is a common disorder with multiple causes that can be categorized as primary (adrenal), secondary (pituitary), and tertiary (hypothalamus) forms (21). Primary adrenal insufficiency, often called Addison’s disease, is mostly caused by autoimmune adrenal gland damage, but infections and genetic abnormalities can also contribute. Primary adrenal insufficiency is characterized by fatigue, frailty, weight loss, low blood pressure, salt cravings, and skin hyperpigmentation (22). When the pituitary gland is unable to produce enough adrenocorticotropic hormone, which stimulates the adrenal glands to synthesize cortisol, secondary adrenal insufficiency develops (23). Tertiary adrenal insufficiency caused by exogenous steroid medication is common but difficult to diagnose due to its non-specific symptoms (21).
3.2 Cushing’s syndrome
Long-term cortisol exposure causes Cushing’s syndrome. Exogenous Cushing’s syndrome is caused by corticosteroid use, while endogenous is caused by adrenal gland excessive production of cortisol (24). The etiology of endogenous Cushing’s syndrome encompasses various factors, including the presence of adrenal tumors (adenomas or carcinomas), pituitary tumors (Cushing’s disease), or tumors that generate ACTH elsewhere in the body (25). Cushing’s syndrome is linked to severe morbidities and a higher mortality rate. Cardiovascular disease is the leading cause of systemic complications and the leading cause of mortality. The prognosis of the disease is primarily influenced by the diagnostic and therapeutic difficulties that continue to be a significant obstacle (26). Weight gain (especially in the trunk and face), muscle weakness, thinning skin, easily bruising, elevated blood pressure, glucose intolerance, and mood swings are all typical signs of Cushing’s syndrome (27).
3.3 Adrenal tumors
Tumors of the adrenal glands can be benign (noncancerous) or malignant (cancerous). Adrenal adenomas are the most prevalent adrenal tumor type and are typically nonfunctional, meaning they do not produce excessive hormones (28). Specific hormones, such as cortisol (which causes Cushing’s disease) or aldosterone (which causes primary aldosteronism), can be overproduced as a result of functional adrenal tumors (29). Rare but aggressive malignant tumors known as adrenal carcinomas can produce too much hormone and invade adjacent tissues (30). Clinical assessment, hormone level measurements (such as cortisol, aldosterone, and adrenal androgens ACTH), imaging tests (such as CT scan and MRI) to look for abnormalities in the adrenal glands, and occasionally specialized tests like the dexamethasone suppression test or adrenal vein sampling are used to diagnose adrenal hormone disorders (31). The exact illness and its underlying cause will determine the available treatments. They could include radiation therapy, surgery to remove adrenal tumors, hormone replacement therapy (such as cortisol or aldosterone replacement), or drugs to control symptoms or hormone production (28).
3.4 Adrenal steroidogenesis
The pathophysiology of lethal adrenal disorders is heavily influenced by oxidative stress, and mutations in antioxidant defense genes can have a considerable impact on adrenal steroidogenesis. Because of their high metabolic activity, the adrenal glands, which are required for the production of steroid hormones that regulate different physiological processes, are vulnerable to oxidative damage (32). Excessive oxidative stress can disrupt adrenal steroidogenesis by disrupting key enzymes involved in hormone synthesis, resulting in cortisol and aldosterone production dysregulation. Mutations in antioxidant defense genes, which are important for reducing oxidative damage, worsen this sensitivity. Such genetic variants weaken cellular defense mechanisms against ROS, raising oxidative stress levels in the adrenal glands. This complex interplay between oxidative stress and genetic factors might lead to the emergence and progression of life-threatening adrenal disorders (33).
4 Oxidative stresses
Oxidative stress occurs when prooxidant molecules like ROS and reactive nitrogen species (RNS) get produced in excess when antioxidant systems are not working efficiently (34, 35). The mitochondrial respiratory chain produces superoxide anion, hydroxyl radical, and hydrogen peroxide during aerobic metabolism (36). RNS includes peroxynitrite-nitrosoperoxycarbonate is produced when peroxynitrite and carbon dioxide react with nitric oxide (NO) (37). Under normal physiological settings, the body makes ROS as a byproduct of metabolism and other cellular processes. Though ROS are important for cell signaling, immune function, and defence against pathogens (38), oxidative stress can be produced by either excessive ROS generation or insufficient antioxidant defence mechanisms (2). Environmental pollutants, exposure to ionizing radiation, certain drugs, chronic inflammation, and lifestyle choices such as excessive alcohol use, a poor diet, and smoking can all contribute to oxidative stress (39). In addition, elevated oxidative stress has been associated with several diseases and conditions, including diabetes, neurological disorders, cardiovascular diseases, and cancer (40).
When ROS levels exceed the antioxidant defences of the body, they can damage lipids, proteins, and DNA. This is also known as oxidative damage, and it can interfere with normal cellular function and contribute to the development of numerous diseases and aging processes (41). This is also linked to Alzheimer’s, Parkinson’s, cardiovascular diseases, and cancer (42). ROS play a major role in disease development, including cancer, neurodegenerative diseases, cardiovascular diseases, diabetes, and inflammatory diseases. The production of ROS is increased in obesity due to the metabolic burden imposed by excessive macronutrient intake and the availability of substrates (43). Metabolic perturbations in the adipose tissue of individuals with obesity arise as a consequence of mitochondrial dysfunction and endoplasmic reticulum stress within the cellular milieu (44). The accumulation of ROS leads to cellular impairment and subsequently contributes to the pathogenesis of inflammatory and cardiovascular disorders (45). The communication of pro-inflammatory cytokines by mitochondrial ROS serves to reinforce the relationship between OS and inflammation (46).
The human body employs a sophisticated array of antioxidants to combat oxidative stress. These include enzymatic antioxidants such as superoxide dismutase (SOD), catalase, glutathione peroxidase, and non-enzymatic antioxidants, including glutathione (GSH). Antioxidants have the ability to counteract ROS and mitigate cellular damage caused by oxidative stress (47).
The management of oxidative stress is crucial for the maintenance of overall health. A balanced, nutritious diet high in antioxidants, regular exercise, avoiding exposure to environmental toxins, and reducing lifestyle factors known to reduce oxidative stress, whereas smoking and binge drinking, are all effectively increase oxidative stress. Additionally, it’s crucial to note that some antioxidant supplements have been investigated for their possible advantages in lowering oxidative stress, while the data for their efficiency is conflicting and should be reviewed with a healthcare provider (39, 48).
5 Adrenal hormonal imbalance-associated oxidative stress
Adrenal hormonal imbalance-related oxidative stress is caused by dysregulation in the finely tuned endocrine system, specifically the adrenal glands (49). The adrenal glands are vital for maintaining physiological homeostasis by secreting hormones such as cortisol and adrenaline. When this equilibrium is upset, either by chronic stress or pathological situations, it can result in the overproduction or underproduction of these hormones, which contributes to oxidative stress (50).
Excess cortisol release, which is frequently associated with chronic stress, activates the glucocorticoid receptor, boosting the creation of reactive oxygen species (ROS) within cells. These ROS molecules, which include superoxide and hydrogen peroxide, cause oxidative damage to cells by destroying lipids, proteins, and DNA (51). Furthermore, disturbed hormonal balance changes antioxidant defense mechanisms, worsening oxidative stress (52).
The mitogen-activated protein kinase (MAPK) and nuclear factor-κB (NF-κB) pathways have both been associated in adrenal hormonal imbalance-associated oxidative stress. When activated by stress-induced hormonal imbalances, these pathways promote the production of pro-inflammatory cytokines and genes associated with oxidative stress, increasing the overall oxidative burden on cells (53). Furthermore, the hypothalamic-pituitary-adrenal (HPA) axis, a key component in stress response, plays an important role in adrenal hormonal imbalance. Abnormal HPA axis signaling can cause persistent cortisol increase, causing oxidative stress via many mechanisms, including mitochondrial dysfunction and endoplasmic reticulum stress (54). The adrenal glands generate hormones such as glucocorticoids, mineralocorticoids, and androgens, (Figure 1) which play significant roles in regulating various physiological processes (55).
The Table 1 provides a brief overview of the impact of hormonal imbalances on oxidative stress, with a focus on glucocorticoids, mineralocorticoids, and androgens. In the case of glucocorticoids, both excess (Cushing’s syndrome) and insufficiency (Addison’s disease) contribute to oxidative stress through a variety of mechanisms, such as decreased antioxidant defenses, increased reactive oxygen species (ROS) generation, and impaired mitochondrial function, which ultimately results in chronic inflammation. Mineralocorticoids, whether in excess (Hyperaldosteronism) or in deficiency (Hypoaldosteronism), are linked to oxidative stress, mainly via activation of the renin-angiotensin-aldosterone system (RAAS) and disruption of cellular homeostasis due to sodium and potassium imbalances. Androgens, whether in excess (Hyperandrogenism) or in deficiency (Hypoandrogenism), cause oxidative stress by affecting immunological responses, fostering chronic inflammation, causing mitochondrial dysfunction, and causing oxidative damage in reproductive organs, thereby affecting fertility and reproductive health (Table 1).
5.1 Glucocorticoids hormone imbalance -associated oxidative stress
In the brain, lungs, and blood cells, the association between glucocorticoids and oxidative stress has been established. Numerous inflammatory and autoimmune disorders are frequently treated with glucocorticoids. The glucocorticoids’s excess led to myopathy, osteoporosis, diabetes, and hypertension, among other diseases. All of the previously mentioned pathophysiological conditions are linked to oxidative stress. ROS from glucocorticoids can cause many pathological conditions (58). Dexamethasone, a synthetic glucocorticoid, has been documented to elicit the production of ROS either through direct means or as a consequence of endothelial nitric oxide synthase (eNOS) uncoupling, which is attributed to the constrained availability of tetrahydrofolate (59, 60). When glucocorticoids are produced in excess, they cause glucose levels to rise; this leads to glycation, which increases ROS production; this, in turn, reduces catalase (CAT), glutathione peroxidase (GPx), and SOD levels in the hippocampus, impairing cognitive functions (61). By raising mitochondrial respiration and oxidative phosphorylation, glucocorticoids directly cause neuronal OS (62). A cascade of negative effects is associated with the role of adrenal corticosterone in hippocampus oxidative stress. This disease is characterized by increased lipid peroxidation and protein carbonyl (PC) concentrations, as well as a decrease in antioxidant enzyme activity such as GPx, SOD, and CAT (63). Another study found that short-term exogenous cortisol administration did not enhance juvenile brown trout oxidative stress levels but did increase GSH levels, indicating that the increased GSH may have reduced the formation of ROS (64). Therefore, cortisol may prevent rather than cause oxidative stress and may activate antioxidant defences via genomic pathways in addition to influencing other systems that regulate the formation of pro-oxidants like ROS (65).
Familial glucocorticoid deficiency (FGD) arises due to mutations in the ACTH-receptor components (MC2R, MRAP) or the general steroidogenesis protein (StAR). These mutations lead to an inability of the adrenocortical cells to synthesize glucocorticoids in response to ACTH stimulation. Nicotinamide Nucleotide Transhydrogenase (NNT) mutations are responsible for the development of FGD. These mutations were observed to decrease the ability of adrenocortical cells to effectively detoxify ROS. Mutations in NNT result in the manifestation of OS as well as phenotypic and functional abnormalities in mitochondrial activity. These findings provide compelling evidence supporting the important role of NNT in maintaining proper mitochondrial function in cases of adrenocortical insufficiency (66).
5.2 Mineralocorticoids hormone imbalance-associated oxidative stress
According to numerous clinical and investigations in animal models, the most significant physiological mineralocorticoid, aldosterone, causes OS and inflammation in patients with chronic and stable heart failure (67). Aldosterone raises blood pressure, affecting the heart. Mineralocorticoid receptors directly affect cardiac function, electrical conduction, OS, inflammation, and fibrosis, further harming the heart (68). Aldosterone/salt-induced hypertension in rats results in renal damage and an increase in the production of ROS in the renal cortex (69). According to Patni et al. (70) findings, an increase in renal OS causes the induction of apoptosis in the renal tubules. It has been demonstrated that aldosterone stimulates superoxide radical formation in endothelial cells by activating Rec1 (71). The mineralocorticoid receptor (MR) is activated during both physiological and pathological events since tissue damage, OS, and inflammation are frequent components of disease situations. It has been utilized in clinical trials to treat heart and kidney disease connected to hypertension and other chronic diseases by blocking MR signaling with MR antagonists (MRAs), which suppresses fibrosis in these organs as MRAs likely have cardio-protective effects by directly blocking cardiac and vascular MR (72, 73).
To avoid aldosterone-induced oxidative damage, kidney cells were tested for their potential to up-regulate nuclear factor-erythroid-2-related factor 2 (Nrf2) (74). Aldosterone first activated Nrf2 in vitro as an antioxidant response. Although aldosterone-induced oxidative or nitrative stress quickly stimulated antioxidant or detoxifying enzymes such SOD, thioredoxin (TRX), HO-1, or GCSc, this adaptive survival response appeared to be fleeting and overpowered by a chronic increased generation of ROS/RNS. As a result, oxidative DNA damage happened. Additionally, even though Nrf2 activation was seen in vivo, aldosterone-treated rat kidneys showed significant DNA damage, showing that the response was insufficient to shield the animals from these side effects (75).
5.3 Androgens hormone imbalance-associated oxidative stress
Testosterone, the main male steroid hormone, causes spermatogenesis and secondary sexual characteristics. Testosterone is anabolic. Usually, it speeds up metabolism. Increased metabolic rate increases O2 consumption and ROS generation. Thus, testosterone increases OS. However, testosterone’s role in OS is controversial. Multiple studies have demonstrated that testosterone induces OS in the muscle, testis, and human placenta (76); others indicate that testosterone has antioxidant properties in the prostate and nervous tissue (77). Testosterone supplements improve the OS parameters in brain tissues and raise antioxidant enzyme levels to reduce oxidative damage. According to in vitro research, testosterone treatment in newborn rats specifically protects the cerebellar granule cells from OS-induced cell death. By inhibiting OS, testosterone contributes to the protection of neurons (78). Adrenal androgen imbalances, such as dehydroepiandrosterone (DHEA) and androstenedione, have been linked to oxidative stress in the body. Elevated amounts of these androgens, which are commonly found in conditions such as polycystic ovarian syndrome (PCOS) and some adrenal disorders, can upset the delicate balance of cellular redox processes. This imbalance can result in an excess of reactive oxygen species (ROS), which then overwhelms the body’s antioxidant defences, resulting in oxidative stress. This type of oxidative stress is recognized as a key factor in the pathogenesis of a variety of health problems, ranging from metabolic disorders to inflammation-related diseases, emphasizing the importance of understanding and managing adrenal androgen imbalances to mitigate oxidative stress and its associated health consequences (79).
According to, Tam et al. (80) study, androgen deprivation increased ROS anabolism and decreased antioxidant detoxification, which in turn caused OS in the ventral prostate of rats. These researchers discovered that castration caused 4-hydroxynonenal and 8-hydroxy-20-deoxy-guanosine protein adducts in the regressing epithelium, which suggests oxidative damage. Additionally, castration considerably decreased the expression of important antioxidant enzymes (AOEs) (GPx1, thioredoxin, SOD2, and peroxiredoxin 5) and markedly increased the expression of ROS-generating NAD(P)H oxidases. Testosterone supplementation partially repaired oxidative damage in ventral prostate epithelia of castrated rats receiving testosterone replacement therapy had a partial decrease in NAD(P)H oxidase expression but an increase in GPx1, SOD2, peroxiredoxin 5 expression, thioredoxin, CAT, glutathione reductase (GR), c-glutamyl transpeptidase, and glutathione synthetase expression in the regenerating ventral prostate tissue. The augmentation of mitochondrial functionality, alterations in intracellular GSH concentrations, and elevation of c-glutamyl transpeptidase activity collectively facilitated the physiological amplification of androgens, leading to the potentiation of OS in LNCaP human prostate cancer cells that are responsive to androgens (81). Prasad et al. (82) showed that testosterone injection to mice caused the down-regulation of CAT, SOD, GST, and GR in the prostate gland. Androgens lowered the activity of AOEs in the heart of rats (83), while orchidectomy enhanced aortic Cu/ZnSOD (84). Borst et al (85) found that testosterone supplementation significantly increased the revival of cardiac work following ischemia/reperfusion in vitro, while orchidectomy significantly lowered rat left ventricular AOE activities. In contrast, Klapcinska et al. (86) showed that castration of male rats lowered the levels of CAT, SOD, GPx, and GR in the left ventricle of the heart, as well as GSH and protein-thiol groups, and increased lipid peroxidation and nitrotyrosine concentrations. Increases in a- and c-tocopherol tissue concentrations in the left ventricle appeared to be a compensatory reaction to the increased OS brought on by gonadectomy. Although androgen replacement restores a healthy serum testosterone level, it decreases left ventricular tissue antioxidant status. The favorable effect of endurance training on SOD and CAT activities was reversed, and myocardial lipid peroxidation was enhanced in adolescent male Wistar rats administered with a high dosage of testosterone (87).
Dehydroepiandrosterone levels gradually decline with age. It has been suggested that DHEA is an effective ageing index with links to geriatric syndromes. There is evidence that low DHEA levels are linked to the beginning and progression of metabolic syndrome and diabetes mellitus, as well as a decrease in bone mineral density (88, 89). Numerous studies have revealed that markers in the oxidative circulation are higher than normal in PCOS patients and that oxidative stress plays a role in the development of PCOS (90). In PCOS, hyperglycemia-induced oxidative stress may be capable of directly promoting hyperandrogenism. The link between plasma testosterone or androstenedione and ROS production suggests this. In vitro investigations have revealed that the ovarian steroidogenic enzymes responsible for androgen production are stimulated by oxidative stress and inhibited by antioxidants such as statins (91, 92).
6 Role of nutritional antioxidants in alleviating adrenal hormone imbalance
Nutritional antioxidants are substances that can be found in a variety of foods and are essential in defending the body against free radical damage, oxidative stress, and the negative impact of reactive oxygen species (ROS). They function by scavenging or neutralizing these harmful chemicals, reducing cellular damage, and improving general health (71). Different types of nutritional antioxidants are depicted in (Figure 2). It was revealed to scavenge hydroxyl radical (˙OH), superoxide anion radical (O2˙-), hydrogen peroxide (H2O2), hypochlorous acid (HOCl), nitric oxide (NO˙), peroxynitrite (ONOO-), singlet oxygen (1O2) and stimulates antioxidant enzymes (AOEs) SOD, Catalase, GPx, GR by nutritional antioxidants (59, 93) (Figure 3).
6.1 Vitamin C against adrenal hormone imbalance-associated oxidative stress
Water-soluble vitamin C, also referred to as ascorbic acid, is a potent antioxidant. Essential for the preservation of overall well-being, it is imperative due to its involvement in numerous pivotal physiological processes (94). Free radicals are unsteady molecules that can harm cells, resulting in several health issues and hastening aging. Vitamin C functions as an antioxidant that assists in scavenging these free radicals and lowering oxidative stress (95). Vitamin C is required for the production of collagen, a structural protein found in the epidermis, bones, tendons, and blood vessels. Collagen is necessary for the formation of scar tissue, so it plays a crucial role in wound healing. Additionally, vitamin C promotes healthy gums, teeth, and cartilage (96). It is well-known that vitamin C supports immune function. It promotes the production of white blood cells, which are essential for fending off infections and pathogens. It enhances natural killer cells and immune system function (97). Plant-based diets and iron supplements contain non-heme iron, which is better absorbed with vitamin C. Iron deficiency anemia can be prevented by boosting iron absorption with vitamin C (98). Other antioxidants in the body, such as vitamin E, are also renewed by vitamin C. It facilitates the restoration of the antioxidant capacity of vitamin E, which enables it to continue fulfilling its protective function in cell membranes. Citrus fruits like lemons, oranges, and grapefruits, berries like strawberries, kiwi, pineapple, mango, papaya, bell peppers (especially yellow and red), Brussels sprouts, broccoli, and leafy greens like spinach and kale are all excellent sources of vitamin C. Furthermore, achieving this outcome is feasible by administering vitamin C supplements (99).
Excess cortisol production in circumstances such as Cushing’s disease or persistent stress can contribute to increased oxidative stress in the body. Vitamin C can help reduce the oxidative stress consequences caused by adrenal hormone imbalance (100).
Vitamin C is a powerful antioxidant capable of scavenging and neutralizing reactive oxygen species (ROS) produced during oxidative stress. It helps stabilize free radicals by giving electrons, preventing them from causing harm to cellular components (95). Vitamin C is essential for regenerating other antioxidants such as vitamin E, glutathione, and coenzyme Q10. These antioxidants also aid in the reduction of oxidative stress and the maintenance of a healthy cellular environment (47). Vitamin C is required for the manufacture of collagen, a protein that provides structure as well as support to a variety of tissues throughout the body, including the adrenal glands. Vitamin C promotes collagen synthesis, which aids in the integrity and function of the adrenal glands, potentially lowering the risk of hormone imbalances. Chronic oxidative stress caused by adrenal hormone abnormalities may weaken the immune system (101). Vitamin C improves immune function by promoting the growth and activity of immune cells like lymphocytes and phagocytes. This can assist the body in fighting infections and other immune-related problems (97). Vitamin C has been demonstrated to influence the body’s stress response. It aids in the regulation of cortisol production, the principal stress hormone generated by the adrenal glands. Vitamin C may indirectly assist in minimizing oxidative stress associated with chronic stress by maintaining adrenal health and normalizing cortisol levels (100).
Several studies have revealed a link between stress-related behavior and ascorbic acid. Animal studies show that ascorbic acid reduces stress-induced cortisol production. Ascorbic acid modulates the hypothalamic-pituitary-adrenal (HPA) axis (Figure 4) by directly “braking” cortisol secretion (102). Since ascorbic acid is a cofactor for adrenal cortex enzymes involved in glucocorticoid biosynthesis, this vitamin is necessary for its production (103). By acting as a cofactor for 11β-hydroxylase, for instance, the ascorbate somewhat increases the conversion of 11-deoxycortisol to cortisol and keeps the cortisol tone at physiological levels (104). Ascorbic acid appears to play a significant part in the stress response, as evidenced by the high amounts of ascorbic acid found in the adrenal glands and the production of ascorbic acid in response to ACTH (100). This is supported by studies showing that ascorbate release occurs before corticosteroid release in the adrenal gland after systemic administration of ACTH to hypophysectomized rats (105), a finding that suggests ascorbate must first be released by the adrenal gland for steroid synthesis (or release) to begin when there is stress.
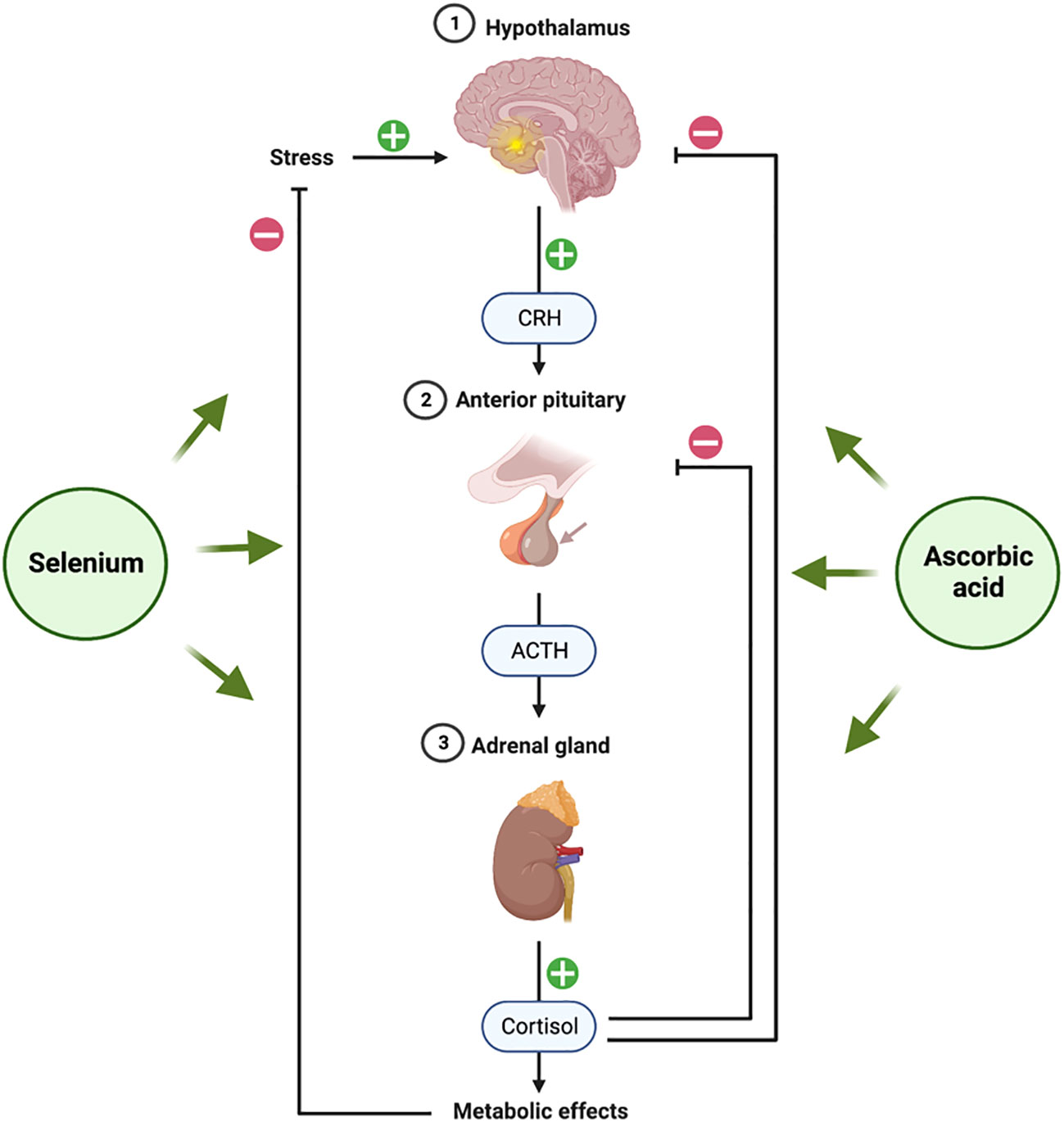
Figure 4 Hypothalamic-Pituitary-Adrenal (HPA) axis and role of selenium and ascorbic acid supplements. The figure was produced with BioRender (Biorender.com; accessed on 29th July 2023).
6.2 Vitamin E against adrenal hormone imbalance-associated oxidative stress
A class of fat-soluble substances with antioxidant capabilities is referred to as vitamin E. It can be found in seeds, leafy green vegetables, nuts, and vegetable oils. There exist two principal variants of vitamin E, namely alpha-tocopherol and gamma-tocopherol. It is worth noting, however, that the term “vitamin E” encompasses a group of eight distinct compounds. These compounds are present in a variety of food sources and are also accessible in the form of dietary supplements (106). Vitamin E antioxidants serve to protect cells against the detrimental effects of free radicals. Free radicals are responsible for inducing cellular damage and contributing to the process of aging. Vitamin E mitigates oxidative stress inside the human body through its ability to counteract the detrimental effects of free radicals (39). Vitamin E is predominantly acknowledged as a lipid-soluble antioxidant, whereby it operates within lipid-rich compartments of cellular structures, such as cell membranes, to mitigate lipid oxidation. Furthermore, it is widely believed that the immune system and gene expression may experience advantageous effects (107).
Adrenal hormone imbalance can increase oxidative stress in the body, and some evidence suggests that vitamin E may protect against it (108). As an antioxidant, Vitamin E neutralizes free radicals and ROS generated during cellular metabolism. Adrenal hormone imbalance can enhance ROS production, causing oxidative damage to cells and tissues. Vitamin E aids in the scavenging of these damaging chemicals, thereby lowering oxidative stress (109). It has been demonstrated that vitamin E affects the body’s hormone levels, especially those of the adrenal glands. Vitamin E may assist in regulating adrenal gland activity and perhaps lessen the production of stress-related chemicals like cortisol by fostering hormonal equilibrium. This may indirectly lessen the oxidative stress brought on by the imbalance of adrenal hormones (110). Chronic adrenal hormone imbalance can cause the body to become inflamed, increasing the risk of oxidative stress. Due to its anti-inflammatory qualities, vitamin E may help lessen inflammation brought on by an imbalance in adrenal hormones. Vitamin E can indirectly lower oxidative stress levels by reducing inflammation (111). Cell membranes are protected from oxidative damage by vitamin E. Unbalanced levels of adrenal hormones can worsen oxidative stress within cells, which can harm biological components. Vitamin E helps maintain appropriate cellular function and lessens oxidative stress-related damage by maintaining the integrity of cell membranes (112).
The consideration of the impact of glucocorticoids on the production of free radicals in the context of stressful situations holds significant importance. Long-term treatment of glucocorticoids is associated with oxidative brain damage in primates, which has been demonstrated (113). According to Al-Sowayan (114), vitamin E therapy reduces exposure to neurotransmitters that cause hypotension by boosting total glutathione, hydrosulfide groups, and selenium levels in the liver and serum. Vitamin E’s chain-breaking antioxidant activities minimize oxidative damage by scavenging free radicals (115).
6.3 Carotenoids against adrenal hormone imbalance-associated oxidative stress
A class of pigments known as carotenoids can be found in many different fruits, plants, and other living things. They have been known for being antioxidants and are important for human nutrition (116). In the human body, carotenoids function as antioxidants to help shield cells from injury from harmful compounds known as free radicals. Oxidative stress is a result of free radicals and is linked to several chronic diseases and the aging process. Carotenoids combat these free radicals, minimizing oxidative damage and boosting general health (117). It is worth noting that a mere fraction of the extensive repertoire of carotenoids, exceeding 600 in number, are habitually ingested by people in general. Beta-carotene, lycopene, lutein, zeaxanthin, and astaxanthin are a few well-known carotenoids. Every carotenoid has different antioxidant capabilities and potential health advantages. Many fruits and vegetables contain large amounts of carotenoids. Beta-carotene is abundant in orange and yellow fruits and vegetables like carrots, sweet potatoes, mangoes, and apricots. Watermelons and tomatoes both contain significant levels of lycopene. Zeaxanthin and lutein are found in leafy green vegetables like kale and spinach. Seafood frequently contains astaxanthin, especially salmon and shrimp (118).
Due to their antioxidant action, carotenoids have been linked to many health advantages. Particularly concentrated in the retina, lutein, and zeaxanthin help prevent age-related macular degeneration (AMD) and cataracts. Beta-carotene and astaxanthin, in particular, can help prevent UV damage to the skin and enhance its appearance (119). Carotenoids reduce inflammation and boost immune cell function, boosting immunity. Certain carotenoids, such as lycopene, have been linked to a reduced risk of heart disease by protecting blood vessels from oxidative injury. Carotenoids are absorbed differently depending on food preparation (cooking, chopping, etc.), the presence of dietary lipids, and individual metabolic differences. Carotenoids are more readily absorbed when consumed with a modest amount of fat. In order to exert their full effects, certain carotenoids, such as beta-carotene, must be converted into vitamin A in the body (120).
Carotenoids have been studied for their possible advantages in lowering oxidative stress and promoting general health because of their well-known antioxidant qualities. Although there is little direct study on the benefits of carotenoids directly on oxidative stress related to oxidative stress linked with adrenal hormone imbalance, their antioxidant and anti-inflammatory characteristics may have beneficial impacts (121).
The body uses carotenoids like beta-carotene, lycopene, and lutein as powerful antioxidants. They aid in scavenging dangerous reactive oxygen species (ROS) and free radicals produced by cellular metabolism. Oxidative stress arises due to increased ROS production, instigated by an aberration in the equilibrium of adrenal hormones. Carotenoids possess the ability to scavenge ROS, thereby mitigating the deleterious effects of oxidative damage (122). Unbalanced levels of adrenal hormones can cause chronic inflammation, which is intimately related to oxidative stress. Anti-inflammatory characteristics found in carotenoids make them useful for controlling the inflammatory response. Carotenoids may indirectly lower oxidative stress levels linked to adrenal hormone imbalance by lowering inflammation (123). Unbalanced adrenal hormones can impact immunological performance and perhaps exacerbate oxidative damage. Through the stimulation of immune cell activity and the modulation of immunological responses, carotenoids have been demonstrated to support immune system function. An immune system that is in good health is better able to tolerate oxidative stress and minimize its effects (124). Although the direct effects of carotenoids on adrenal hormones have not been thoroughly investigated, they may indirectly influence the body’s hormonal balance. Certain hormones, particularly sex hormones, are partly produced and metabolized by carotenoids. Carotenoids may benefit adrenal hormone imbalance by promoting hormonal equilibrium, potentially lowering oxidative stress (125). When combined with other antioxidants like vitamins E and C, carotenoids can offer more antioxidant protection (126).
Depression is significantly influenced by carotenoid-cleaving enzymes, which are involved in the metabolism of carotenoids. It should be noted that the oxidative degradation of carotenoids, facilitated by carotenoid oxygenases, results in the formation of apocarotenoids. Retinal, retinol, retinoic acid, and abscisic acid are examples of apocarotenoids. By hyperactivating the hypothalamic-pituitary-adrenal (HPA) axis, retinoic acid, the active form of vitamin A, has been related to depressed behavior. Retinoic acid can cause suicide in sensitive people (127, 128). According to a study, eating foods high in carotene and vitamin C is linked to less severe depressive symptoms (129). Lower carotenoid levels may also be a result of bad eating habits linked to obesity and overweight, which have been linked to an enhanced risk of depression due to inflammation or HPA axis dysregulation (10).
6.4 Selenium against adrenal hormone imbalance-associated oxidative stress
Selenium is an indispensable trace mineral that serves as a potent antioxidant in the body. It exerts a protective effect on cellular structures by synergistic interactions with other antioxidants, such as vitamin E, thereby mitigating the deleterious impact of free radicals and oxidative stress-induced damage (130). Numerous antioxidant enzymes, such as glutathione peroxidase, which works to scavenge free radicals and lessen oxidative cell damage, require selenium as a cofactor. Free radicals can damage cells and cause chronic diseases like cancer, heart disease, and neurological disorders (131). By scavenging these free radicals, selenium reduces oxidative stress. In order to maintain a strong immune system, selenium is essential. It aids in controlling immunological responses, improves immune cell performance, and encourages the formation of antibodies (132). The synthesis and metabolism of thyroid hormones depend on selenium. It converts inactive thyroid hormone (T4) into active thyroid hormone (T3) to sustain proper thyroid function. Selenium has been investigated for its potential role in lowering the risk of specific cancers, including skin, lung, prostate, and colorectal cancers (133). As an antioxidant, it can aid in preventing DNA damage to cells and stop the development of cancer cells. By lowering oxidative stress, enhancing blood vessel function, and reducing inflammation, selenium may benefit heart health. These outcomes may assist in reducing the risk of cardiovascular conditions, such as heart disease and stroke. The health of the male reproductive system depends on selenium. It contributes to sperm production and aids in preserving the sperm cells’ structural integrity. It has been demonstrated that selenium supplementation enhances sperm motility and lessens sperm DNA damage (134). The recommended daily selenium intake varies depending on various factors, such as age, gender, and specific medical conditions. The recommended daily intake (RDA) for adults is about 55 micrograms per day. It is crucial to remember that consuming too much selenium can be hazardous, so stick to the recommended dosages. Brazil nuts, organ meats like liver and kidney, whole grains, eggs, and poultry are all excellent sources of selenium (135).
Selenium’s antioxidant capabilities and function in promoting the activity of antioxidant enzymes may have implications for lowering oxidative stress, even though there is little direct study on its impact on adrenal hormone imbalance-related oxidative stress (136).
Several antioxidant enzymes, such as thioredoxin reductases and glutathione peroxidases, require selenium in order to function. These enzymes are essential for scavenging ROS and guarding cells against oxidative damage. Increased ROS formation from adrenal hormone imbalance causes oxidative stress. To strengthen the body’s defence against oxidative stress, selenium aids in activating these antioxidant enzymes (137). The formation of glutathione, a potent antioxidant and detoxifying molecule, requires selenium. Glutathione is a key component of cellular antioxidant defence mechanisms and aids in the reduction of oxidative stress. Selenium shortage might hinder the production of glutathione, thereby aggravating the oxidative stress brought on by an imbalance in adrenal hormones. The generation and function of glutathione are supported by adequate selenium levels, promoting antioxidant defence (138). Unbalanced adrenal hormones can impact immunological performance and perhaps exacerbate oxidative damage. Immunomodulatory characteristics of selenium are well recognized, and it supports healthy immune system operation. A healthy immune system can better manage oxidative stress and lessen its harmful effects (139). Thyroid hormone metabolism, which is closely related to the control of adrenal hormones, involves selenium. The thyroid and hormonal equilibrium in the body may be supported by maintaining healthy selenium levels. Selenium may indirectly help to reduce the oxidative stress brought on by the imbalance of adrenal hormones by encouraging hormonal equilibrium (140).
Endocrine components of the “fight or flight” stress response include the hypothalamic-pituitary-adrenal (HPA) axis (Figure 4) (141). Corticotropin-releasing hormone (CRH) from the hypothalamus causes the anterior pituitary to release ACTH in response to stress. The production of corticosteroids, including the glucocorticoid class of stress hormones, is then triggered by ACTH acting on the adrenal gland. Almost every tissue in the body contains the GC receptor (GCR) (142). Because of their ability to reduce inflammation, GCs are frequently given for a wide range of ailments and diseases (143). Selenium appears to have a significant protective effect against the harm and dysfunction brought on by excessive activation of the HPA axis. Our research group has conducted a recent assessment of the progress made in investigating the relationship that has been extensively studied in the brain using rodent models in recent years (144).
A comprehensive investigation on porcine subjects has yielded noteworthy findings about the impact of selenium insufficiency on the antioxidant capacity and subsequent induction of oxidative stress within adrenal tissue. This phenomenon has been observed to occur through the mediation of the toll-like receptor 4 (TLR4)/NF-kB pathway. This observation contributes to the expanding body of evidence regarding the potential involvement of selenium in modulating the physiological functions of the adrenal gland (145). The observed correlation between selenium deficiency and reduced levels of miR-30d-R_1, a microRNA (miRNA) known for its inhibitory effect on TLR4 expression, implies a potential link between the dysregulation of the TLR4/NF-kB pathway and the onset of inflammatory processes (146). It is noteworthy to observe that the overexpression of TLR4 in human adrenocortical cells resulted in a reduction in the production of cortisol and aldosterone (147). Consequently, selenium has the potential to facilitate the functioning of the HPA axis through its ability to induce a mechanism of downregulation of TLR4 miRNA, thereby promoting the synthesis of adrenal steroids. The observed phenomenon of reduced corticosterone secretion due to selenium deficiency can be attributed to the blunting of the adrenal response to ACTH (139). The involvement of selenoproteins in the development of the HPA axis represents a captivating correlation between selenium and the physiological reaction to stress. During the developmental process of neuroendocrine cells, an intriguing observation was made about activating the Selenot gene in the adrenal medulla (148).
6.5 Zinc against adrenal hormone imbalance-associated oxidative stress
The immune system, cell division, and growth are just a few of the basic activities in the body that zinc is crucial for. Despite its relative lack of recognition as an antioxidant, zinc exhibits antioxidant properties and plays a crucial role in bolstering the body’s overarching antioxidant defense mechanisms (149). By scavenging damaging free radicals, zinc functions as an antioxidant to help protect cells from oxidative stress. Free radicals are unstable molecules that can harm cells and speed up the aging process. They also have a role in several disorders. Zinc is an antioxidant that helps to stabilize these free radicals and stop them from doing any harm. In addition to having antioxidant qualities, zinc also helps several enzymes involved in antioxidant defence systems function. It is an essential part of the enzyme SOD, which assists in converting superoxide radicals into less dangerous molecules (150). Additionally, zinc helps in the production of metallothionein, a protein that helps control metal levels and protects against oxidative damage. Enriching zinc consumption is crucial for the body to have a healthy antioxidant system. Oysters, red meat, chicken, beans, nuts, and whole grains are healthy food sources of zinc. Additional zinc supplements are available, which may be advantageous for people with specific health issues or zinc deficiency (151).
Zinc’s antioxidant capabilities and involvement in hormonal balance may have implications for lowering oxidative stress, even though there is little direct study on its effects on adrenal hormone imbalance-related oxidative damage (150).
Superoxide dismutase and catalase are two antioxidant enzymes that utilize zinc as a cofactor. These enzymes aid in reducing oxidative stress and neutralizing reactive oxygen species (ROS). Unbalanced levels of adrenal hormones can increase the generation of ROS, which increases the risk of oxidative damage. The body’s defence against oxidative stress is aided by zinc because of its role in the functioning of antioxidant enzymes (152). The synthesis, secretion, and metabolism of numerous hormones, particularly adrenal hormones, depend heavily on zinc. The proper production and control of these hormones can be hampered by an imbalance in the adrenal hormones. Zinc may indirectly lessen the oxidative stress brought on by an imbalance in adrenal hormones by promoting hormonal equilibrium (153). Unbalanced levels of adrenal hormones might affect how well the immune system works, thereby increasing oxidative stress. Zinc is important for immune system health and influences the growth and functioning of immune cells. A healthy immune system can better manage oxidative stress and lessen its harmful effects (154). Zinc aids in DNA repair processes and promotes healthy cellular operation. DNA can be harmed by oxidative stress, which can also harm other biological components. Zinc’s role in DNA repair promotes cellular health by preserving the integrity of genetic information and minimizing damage brought on by oxidative stress (155). Inflammation, which is strongly related to oxidative stress, can be brought on by a chronic adrenal hormone imbalance. The anti-inflammatory qualities of zinc make it possible to control the inflammatory response. Zinc may indirectly help lower oxidative stress levels linked to an imbalance in adrenal hormones by reducing inflammation (156).
Additionally, research has shown that cortisol impacts micronutrient metabolism, particularly magnesium, zinc, and selenium. Cortisol increases the expression of genes for metallothionein and ZIP-14, which accumulate zinc in the liver and adipose tissue, promoting hypozincemia in obese people (157).
Morais et al. (158) performed a correlation analysis to determine if cortisol affected zinc, magnesium, and selenium homeostasis in study participants. The plasma and erythrocyte zinc levels did not correlate with urine cortisol levels. Cortisol/cortisone ratio and erythrocyte zinc levels also correlated negatively. This study found hypozincemia in obese women due to elevated cortisol levels, which promote the production of metallothionein and ZIP-14 genes.
6.6 Polyphenols against adrenal hormone imbalance-associated oxidative stress
A class of naturally occurring substances called polyphenols can be found in a wide range of plant-based foods, such as fruits, vegetables, whole grains, herbs, and spices. Since they are recognized for having antioxidant capabilities, they can aid in preventing free radical damage to the body’s cells. Polyphenols are dietary antioxidants important for preserving general health and preventing chronic disorders (159).
By scavenging free radicals, polyphenols function as powerful antioxidants. Free radicals, characterized by their inherent instability, possess the capacity to inflict cellular damage and contribute significantly to the pathogenesis of various medical conditions, including but not limited to cancer, cardiovascular illnesses, and neurodegenerative disorders (160). By scavenging free radicals, polyphenols function as powerful antioxidants. Free radicals are unsteady molecules that can harm cells and play a role in the emergence of a number of illnesses, such as cancer, heart disease, and neurological disorders (161). Polyphenol-rich diets may have several positive health effects. Reducing inflammation, preventing cardiovascular disease, promoting brain health, boosting immunological function, and maybe lowering the risk of some cancers are a few of these (160). Inflammatory pathways in the body have been proven to be modulated by polyphenols, which help to lessen chronic inflammation, which is linked to a number of diseases like heart disease, obesity, and some types of cancer. Polyphenols may have biological effects besides their antioxidant action, such as encouraging good gut bacteria, enhancing blood sugar regulation, and promoting healthy aging processes (161).
Fruits, vegetables, tea, coffee, and cocoa are just a few examples of plant-based meals rich in polyphenols, a broad set of substances. Due to their anti-inflammatory and antioxidant capabilities, which may help lower oxidative stress and enhance general health, they have attracted much interest. While there is little direct evidence on how polyphenols affect the oxidative stress brought on by adrenal hormone imbalance, their antioxidant and anti-inflammatory properties may have implications for reducing oxidative stress (162).
Strong antioxidants, polyphenols can trap and deactivate free radicals and reactive oxygen species (ROS) produced by oxidative stress. Unbalanced levels of adrenal hormones can increase the generation of ROS, which increases the risk of oxidative damage. By quenching these detrimental chemicals and shielding cells from oxidative damage, polyphenols can help decrease oxidative stress (160). Inflammation, which is strongly related to oxidative stress, can be brought on by a chronic adrenal hormone imbalance. The inflammatory response can be modulated by polyphenols, which have anti-inflammatory effects. Polyphenols may indirectly help lower oxidative stress levels linked to an imbalance in adrenal hormones by reducing inflammation (163). Polyphenols have been demonstrated to alter the body’s signalling systems and hormone levels. Polyphenols may have indirect impacts on hormone control even though their direct effects on adrenal hormones are not fully understood. Polyphenols may potentially assist in lowering oxidative stress linked to adrenal hormone imbalance by supporting hormonal equilibrium (164). Unbalanced levels of the adrenal hormones can cause mitochondria to malfunction and produce more ROS. It has been demonstrated that polyphenols enhance mitochondrial function and defend them against oxidative damage. Polyphenols support cellular health by protecting mitochondrial health and lowering oxidative stress (163). Superoxide dismutase (SOD) and catalase are two examples of endogenous antioxidant enzymes that can be stimulated by polyphenols. These enzymes are essential for reducing ROS and preserving redox equilibrium. Polyphenols may offer extra defence against oxidative stress related to an imbalance in adrenal hormones by increasing the activity of these antioxidant enzymes (165).
Polyphenols activate the redox-sensitive transcription factor nuclear factor erythroid 2-related factor-2 (Nrf2) (166). Contrarily, research suggests that polyphenols can modify the glucocorticoid receptor’s (GR) activity. In fact, GR and FK506 binding protein 5 (FKBP5) expression can be changed by the polyphenolic flavonoid icariin, which enhances GR stability and lessens GR sensitivity to GC in vivo (167). More research is required in this area since manipulation of the GR regulatory system is currently an intriguing target for the treatment of stress-related illnesses (168).
6.7 Coenzyme Q10 against adrenal hormone imbalance-associated oxidative stress
A naturally occurring substance in the body is coenzyme Q10 (CoQ10). CoQ10 plays a pivotal role in generating adenosine triphosphate (ATP), which serves as the primary energy source for cells. Additionally, CoQ10 performs the role of an antioxidant, assisting in preventing cell deterioration brought on by harmful molecules known as free radicals. CoQ10 acts as an antioxidant to combat free radicals and stop oxidative stress, which can cause cellular damage and be a factor in several health issues (169). In order to stabilize free radicals and lessen their potential for harm, it donates electrons. Small levels of coenzyme Q10 are included in some meals, including meat, fish, and whole grains. However, CoQ10 production by the body tends to decrease with aging, and some diseases or drugs might further lower its levels. CoQ10 supplements are therefore offered to support optimal levels of this substance. CoQ10 has been researched for its possible health advantages in several illnesses, even though it is primarily known for its role in energy production and as an antioxidant (170).
Despite the limited body of research investigating the specific impact of CoQ10 on oxidative stress resulting from imbalances in adrenal hormones, it’s inherent antioxidant properties and involvement in cellular energy metabolism suggest potential efficacy in mitigating oxidative stress (171).
CoQ10 is a powerful antioxidant that protects cells from the damage that free radicals and reactive oxygen species (ROS) cause when they combine with oxygen. Dysregulation of adrenal hormones can lead to increased production of ROS, hence contributing to oxidative stress. CoQ10 helps eliminate these harmful molecules, which lowers oxidative stress and keeps the health of cells (172). CoQ10 is an important part of the electron transport chain, which is a process that helps cells make energy (ATP). A lack of adrenal hormones can change how cells use energy, which can increase oxidative stress. CoQ10 may help restore cellular balance and lower oxidative stress by helping cells make energy more efficiently (173). CoQ10 can make antioxidants like vitamin E, vitamin C, and glutathione, which are important parts of the body’s antioxidant defence system, from scratch. By regenerating and reusing these antioxidants, CoQ10 makes them more effective at fighting oxidative stress caused by an imbalance in adrenal hormones (174). A lack of adrenal hormones that lasts for a long time can cause inflammation, which is linked to oxidative stress. CoQ10 has anti-inflammatory qualities and can help control the way the body reacts to inflammation. CoQ10 may indirectly help reduce oxidative stress by reducing inflammation (51). CoQ10 keeps cell parts, like cell walls and mitochondria, from getting damaged by oxidation. Unbalanced adrenal hormones can cause oxidative stress in cells, which can damage the structures of cells. CoQ10 helps keep cell walls and mitochondria working well, which reduces damage caused by oxidative stress (175).
When it comes to disorders with the pituitary and adrenal glands, there is proof of mitochondrial dysfunction in people with Cushing’s syndrome. This is shown in respiratory chain complex enzyme activity (176) and oxidative stress (measured by total antioxidant capacity and plasma 15-F2t-isoprostane) (177). However, there haven’t been many studies on how CoQ10 affects pituitary and adrenal function in endocrine therapy. Some pituitary/adrenal problems may be associated with low levels of CoQ10 in the blood, according to preliminary investigations (178). Plasma CoQ10 levels were evaluated in six patients with ACTH-dependent adrenal hyperplasia, 19 with secondary solitary hypoadrenalism, and 19 with concurrent hypothyroidism (multiple pituitary deficits). Compared to numerous pituitary deficits and ACTH-dependent adrenal hyperplasia, CoQ10 levels were considerably lower in secondary isolated hypoadrenalism. Patients with acromegaly apparently have lower plasma CoQ10 levels (179).
6.8 Probiotics against adrenal hormone imbalance-associated oxidative stress
Typically, probiotics are not regarded as a direct source of antioxidants. However, some probiotic strains have been demonstrated to have indirect antioxidant benefits or to be able to improve the body’s antioxidant state via a variety of mechanisms (180).
A healthy, diversified gut flora, which is important for the digestion of food components, can be maintained with the use of probiotics. Some helpful bacteria in the stomach can accelerate the breakdown and creation of bioactive molecules with antioxidant capabilities from dietary antioxidants, such as polyphenols found in fruits and vegetables. The availability and potency of antioxidants in the body may increase as a result of this metabolic activity (181). It has been demonstrated that probiotics can indirectly lower oxidative stress by enhancing gut health and lowering inflammation. Probiotics can assist in maintaining gut barrier function, preventing the transfer of risky bacteria and endotoxins into the bloodstream, and reducing systemic inflammation by supporting a healthy gut microbiota. This can then support general antioxidant defence mechanisms and minimize oxidative stress (182). Probiotics can increase the body’s natural synthesis and activity of endogenous antioxidants. For instance, it has been discovered that specific probiotic strains enhance the production of glutathione, a potent antioxidant that aids in neutralizing free radicals and the defence of cells against oxidative damage (180). Probiotics can interact with intestinal mucosal cells and other tissues to enhance their antioxidant defences. They can affect cellular signaling pathways that produce antioxidant enzymes like SOD and catalase, which remove free radicals and minimize oxidative stress (183).
Probiotics, known as beneficial microorganisms that promote a healthy gut microbiota, have exhibited promising potential in modulating the equilibrium of adrenal hormones and mitigating challenges associated with oxidative stress. The interaction between the gut microbiota and the adrenal glands is reciprocal, which means that each can have an impact on the other’s operation. Dysbiosis, a condition characterized by perturbations in the composition and function of the gastrointestinal microbiota, has been implicated in various pathological states, encompassing abnormalities of adrenal hormone levels and elevated oxidative stress (184).
Probiotics support a balanced population of good bacteria in the gut, enhancing overall gut health (Figure 5). Better nutrient absorption, especially of antioxidants and other vital micronutrients necessary for preventing oxidative stress, is made possible by a healthy gut lining (185). Probiotic supplementation has been shown to exert a modulatory effect on the body’s inflammatory and immunological responses. Chronic inflammation can disturb the equilibrium of the adrenal hormones and is linked to increased oxidative stress. Probiotics may indirectly aid adrenal gland function and lessen oxidative stress by lowering inflammation (186). In the gut, some probiotic strains can create antioxidants. For instance, it has been demonstrated that certain Lactobacillus and Bifidobacterium species produce antioxidants like glutathione, which can help mitigate oxidative stress and protect against cell damage (187). Probiotics can convert polyphenols present in fruits and vegetables, for example, into bioactive compounds with antioxidant properties. This transformation engenders heightened accessibility and potency of antioxidants, thereby fostering equilibrium in adrenal hormone levels and mitigating the impact of oxidative stress (180). Probiotics can influence the body’s response to stress and help regulate cortisol levels. By promoting a balanced stress response, probiotics may assist in regulating adrenal hormone production and mitigating the harmful effects of stress-induced oxidative stress (188).
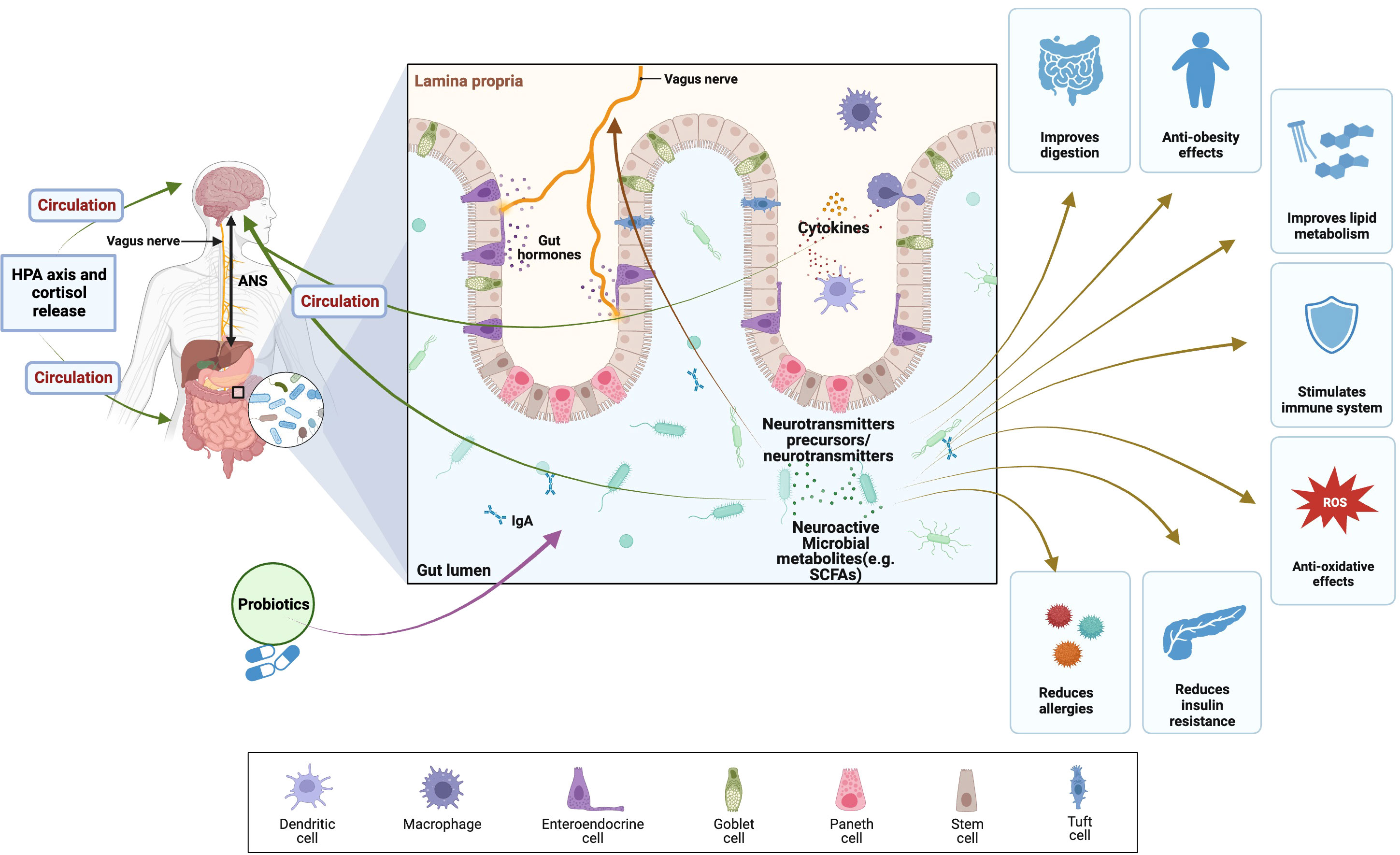
Figure 5 Health benefits of probiotics and their effects on brain, gut, and microbiome (BGM) axis modulating of HPA axis and cortisol release. The BGM axis network of routes that facilitate the exchange of information and signals encompasses neuronal elements (vagus nerve, neurotransmitters, and enteric nervous system), the HPA axis, and stress hormones like cortisol. Furthermore, immune mechanisms, specifically cytokines, contribute to this complex interplay. (SCFAs), Short-chain fatty acids; (ANS), autonomic nervous system; (ROS), reactive oxygen species; (HPA axis), Hypothalamic–pituitary–adrenal axis. The figure was produced with BioRender (Biorender.com; accessed on 30th Oct 2023).
Bidirectional connections within the brain-gut-microbiome (BGM) axis have been demonstrated in several preclinical and clinical research studies (189, 190). The communication between gut microbes and the central nervous system is facilitated through a complex network of interconnected channels, which encompass the nervous, endocrine, and immune signaling mechanisms. The brain possesses the capability to exert influence on the structural and functional organization of the gut microbiota. This influence is primarily mediated by the autonomic nervous system (ANS), which regulates various aspects, including gut permeability, regional gut motility, intestinal transit and secretion. The HPA axis operates under the fundamental mechanism of negative feedback and assumes a pivotal function in eliciting the body’s stress response and governing various physiological processes, encompassing digestion, immune system functionality, and energy equilibrium (191). Stress hormones induce the disruption of tight junctions, consequently leading to increased permeability of the intestinal barrier (192). Dietary probiotic supplementation has been shown to offer potential alleviation of the HPA axis response to acute stress (193). For example, the administration of a probiotic treatment containing L. farciminis in a murine model has been reported to effectively mitigate the stress-induced hyperpermeability, endotoxemia, and, thus, ameliorating the stress response of the HPA axis.
Moreover, recent research findings have indicated that using probiotics and prebiotics, which serve as agents that regulate the composition of the gastrointestinal microbiota, may offer potential advantages in mitigating the manifestations of stress-related infertility (194). The beneficial effects of probiotics on infertility associated with stress have been attributed to various mechanisms, such as regulating the HPA axis, modulation of the immune response, and restoring microbial homeostasis (184). The HPA axis plays a pivotal role in maintaining reproductive health by governing the intricate regulation of the stress response. The HPA axis can be disrupted by stress, resulting in variations in cortisol levels, the primary stress hormone (49). Multiple studies have provided evidence indicating that probiotics possess the ability to modulate the HPA axis and reduce cortisol levels, thereby alleviating the adverse impact of stress on reproductive health (194). Under stressful circumstances, Lactobacillus casei strain Shirota (LcS) may prevent cortisol hypersecretion and physical symptoms, possibly through reducing stress reactivity in the paraventricular nucleus (PVN) and vagal afferent signaling to the brain (195). According to Nasri et al. (196), selenium and probiotic co-administration to women with polycystic ovary syndrome (PCOS) reduced modified Ferriman Gallwey (mF-G) scores and total testosterone.
The role of nutritional antioxidants in alleviating adrenal hormone imbalance is depicted in (Figure 6) (Table 2).
7 Search strategy
This review paper’s search strategy included a thorough study of scientific databases such as PubMed, Scopus, Google Scholar, and Web of Science, utilizing a combination of keywords and controlled vocabulary terms. The most commonly used terms were “oxidative stress,” “oxidative stress,” “nutritional antioxidants,” “reactive oxygen species,” and “adrenal hormone imbalance.” The search was refined using Boolean operators (AND, OR) to ensure relevance to the review’s focus on the potential of nutritional antioxidants against oxidative stress linked with adrenal hormone imbalance. Furthermore, specific terms relating to adrenal gland function, such as “glucocorticoids,” “mineralocorticoids,” “androgens,” and “adrenal hormone disorders” such as “adrenal insufficiency,” “Cushing’s syndrome,” and “adrenal tumors,” were added to collect relevant literature. The search included experimental and clinical investigations, as well as review papers, to provide a full picture of the current level of knowledge on the topic. The inclusion criteria included publications published in the previous decade, and the search approach was iterative, with continual refinement based on the identified literature until a thorough selection of relevant studies was obtained.
8 Conclusions
In conclusion, the effectiveness of nutritional antioxidants in combating oxidative stress caused by adrenal hormone imbalance is undeniable. Antioxidants, such as vitamin C, vitamin E, carotenoids, selenium, zinc, polyphenols, coenzyme Q10, and probiotics, play vital roles in mitigating the negative effects of oxidative stress on adrenal hormone balance. By mitigating dangerous free radicals and reducing oxidative damage, these antioxidants can aid in the restoration and maintenance of adrenal hormone function. In addition, the article discusses adrenal hormone abnormalities such as adrenal insufficiency, Cushing’s syndrome, and adrenal tumors. The findings imply that utilizing the efficacy of dietary antioxidants may offer therapeutic approaches for alleviating oxidative stress associated with adrenal hormone abnormalities, opening up new areas for investigation.
Author contributions
AnP: Data curation, Investigation, Methodology, Writing – original draft, Writing – review & editing. DB: Data curation, Formal Analysis, Resources, Software, Writing – review & editing. VY: Conceptualization, Methodology, Supervision, Validation, Writing – review & editing. K-YL: Resources, Software, Supervision, Validation, Writing – review & editing. AsP: Formal Analysis, Investigation, Validation, Visualization, Writing – original draft, Writing – review & editing. DS: Conceptualization, Formal Analysis, Funding acquisition, Visualization, Writing – original draft, Writing – review & editing.
Funding
The author(s) declare that no financial support was received for the research, authorship, and/or publication of this article.
Conflict of interest
The authors declare that the research was conducted in the absence of any commercial or financial relationships that could be construed as a potential conflict of interest.
Publisher’s note
All claims expressed in this article are solely those of the authors and do not necessarily represent those of their affiliated organizations, or those of the publisher, the editors and the reviewers. Any product that may be evaluated in this article, or claim that may be made by its manufacturer, is not guaranteed or endorsed by the publisher.
References
1. Lotfi CFP, Kremer JL, Dos Santos Passaia B, Cavalcante IP. The human adrenal cortex: growth control and disorders. Clinics (2018) 73(suppl 1):e473s. doi: 10.6061/clinics/2018/e473s
2. Pizzino G, Irrera N, Cucinotta M, Pallio G, Mannino F, Arcoraci V, et al. Oxidative stress: harms and benefits for human health. Oxid Med Cell Longev (2017) 2017:8416763. doi: 10.1155/2017/8416763
3. Bjelaković G, Beninati S, Pavlović D, Kocić G, Jevtović T, Kamenov B, et al. Glucocorticoids and oxidative stress. J Basic Clin PhysiolPharmacol (2007) 18(2):115–27. doi: 10.1515/jbcpp.2007.18.2.115
4. Vona R, Pallotta L, Cappelletti M, Severi C, Matarrese P. The impact of oxidative stress in human pathology: focus on gastrointestinal disorders. Antioxidants (2021) 10(2):201. doi: 10.3390/antiox10020201
5. Roychoudhury S, Chakraborty S, Choudhury AP, Das A, Jha NK, Slama P, et al. Environmental factors-induced oxidative stress: hormonal and molecular pathway disruptions in hypogonadism and erectile dysfunction. Antioxidants (2021) 10(6):837. doi: 10.3390/antiox10060837
6. Madison A, Kiecolt-Glaser JK. Stress, depression, diet, and the gut microbiota: human-bacteria interactions at the core of psychoneuroimmunology and nutrition. Curr Opin Behav Sci (2019) 28:105–10. doi: 10.1016/j.cobeha.2019.01.011
7. Du J, Wang Y, Hunter R, Wei Y, Blumenthal R, Falke C, et al. Dynamic regulation of mitochondrial function by glucocorticoids. Proc Natl Acad Sci U.S.A. (2009) 106(9):3543–8. doi: 10.1073/pnas.0812671106
8. Chen L, Deng H, Cui H, Fang J, Zuo Z, Deng J, et al. Inflammatory responses and inflammation-associated diseases in organs. Oncotarget (2017) 9(6):7204–18. doi: 10.18632/oncotarget.23208
9. Salvador J, Gutierrez G, Llavero M, Gargallo J, Escalada J, López J. Endocrine disorders and psychiatric manifestations. In: Portincasa P, Frühbeck G, Nathoe HM, editors. Endocrinology and Systemic Diseases. Endocrinology. Cham: Springer (2019). p. 311–45. doi: 10.1007/978-3-319-66362-3_12-1
10. Rasmus P, Kozłowska E. Antioxidant and anti-inflammatory effects of carotenoids in mood disorders: an overview. Antioxidants (2023) 12(3):676. doi: 10.3390/antiox12030676
11. Pérez-Torres I, Castrejón-Téllez V, Soto ME, Rubio-Ruiz ME, Manzano-Pech L, Guarner-Lans V. Oxidative stress, plant natural antioxidants, and obesity. Int J Mol Sci (2021) 22(4):1786. doi: 10.3390/ijms22041786
12. Gligorijevic N, Kaljevic M, Radovanovic N, Jovanovic F, Joksimovic B, Singh S, et al. Adrenal abscesses: A systematic review of the literature. J Clin Med (2023) 12(14):4601. doi: 10.3390/jcm12144601
13. Dumontet T, Martinez A. Adrenal androgens, adrenarche, and zona reticularis: A human affair? Mol Cell Endocrinol (2021) 528:111239. doi: 10.1016/j.mce.2021.111239
14. Gordan R, Gwathmey JK, Xie LH. Autonomic and endocrine control of cardiovascular function. World J Cardiol (2015) 7(4):204–14. doi: 10.4330/wjc.v7.i4.204
15. Bennett JM, Reeves G, Billman GE, Sturmberg JP. Inflammation-nature's way to efficiently respond to all types of challenges: implications for understanding and managing "the epidemic" of chronic diseases. Front Med (Lausanne) (2018) 5:316. doi: 10.3389/fmed.2018.00316
16. Tsilosani A, Gao C, Zhang W. Aldosterone-regulated sodium transport and blood pressure. Front Physiol (2022) 13:770375. doi: 10.3389/fphys.2022.770375
17. Stamou MI, Colling C, Dichtel LE. Adrenal aging and its effects on the stress response and immunosenescence. Maturitas (2023) 168:13–9. doi: 10.1016/j.maturitas.2022.10.006
18. Turcu A, Smith JM, Auchus R, Rainey WE. Adrenal androgens and androgen precursors-definition, synthesis, regulation and physiologic actions. Compr Physiol (2014) 4(4):1369–81. doi: 10.1002/cphy.c140006
19. Pignatti E, Flück CE. Adrenal cortex development and related disorders leading to adrenal insufficiency. Mol Cell Endocrinol (2021) 527:111206. doi: 10.1016/j.mce.2021.111206
20. Bouillon R. Acute adrenal insufficiency. Endocrinol Metab Clin (2006) 35(4):767–75. doi: 10.1016/j.ecl.2006.09.004
21. Husebye ES, Pearce SH, Krone NP, Kämpe O. Adrenal insufficiency. Lancet (2021) 397(10274):613–29. doi: 10.1016/S0140-6736(21)00136-7
22. Nieman LK, Chanco Turner ML. Addison's disease. Clin Dermatol (2006) 24(4):276–80. doi: 10.1016/j.clindermatol.2006.04.006
24. Newell-Price J, Bertagna X, Grossman AB, Nieman LK. Cushing's syndrome. Lancet (2006) 367(9522):1605–17. doi: 10.1016/S0140-6736(06)68699-6
25. Lacroix A, Feelders RA, Stratakis CA, Nieman LK. Cushing's syndrome. Lancet (2015) 386(9996):913–27. doi: 10.1016/S0140-6736(14)61375-1
26. Pivonello R, De Martino MC, De Leo M, Lombardi G, Colao A. Cushing's syndrome. Endocrinol Metab Clin North Am (2008) 37(1):135–49. doi: 10.1016/j.ecl.2007.10.010
27. Kirk LF Jr., Hash RB, Katner HP, Jones T. Cushing's disease: clinical manifestations and diagnostic evaluation. Am Fam Physician (2000) 62(5):1119–27.
28. Fassnacht M, Arlt W, Bancos I, Dralle H, Newell-Price J, Sahdev A, et al. Management of adrenal incidentalomas: European Society of Endocrinology Clinical Practice Guideline in collaboration with the European Network for the Study of Adrenal Tumors. Eur J Endocrinol (2016) 175(2):G1–G34. doi: 10.1530/EJE-16-0467
29. Vaduva P, Bonnet F, Bertherat J. Molecular basis of primary aldosteronism and adrenal cushing syndrome. J Endocr Soc (2020) 4(9):bvaa075. doi: 10.1210/jendso/bvaa075
30. Else T, Kim AC, Sabolch A, Raymond VM, Kandathil A, Caoili EM, et al. Adrenocortical carcinoma. Endocr Rev (2014) 35(2):282–326. doi: 10.1210/er.2013-1029
31. Bancos I, Hahner S, Tomlinson J, Arlt W. Diagnosis and management of adrenal insufficiency. Lancet Diabetes Endocrinol (2015) 3(3):216–26. doi: 10.1016/S2213-8587(14)70142-1
32. Corkery-Hayward M, Metherell LA. Adrenal dysfunction in mitochondrial diseases. Int J Mol Sci (2023) 24(2):1126. doi: 10.3390/ijms24021126
33. Prasad R, Kowalczyk JC, Meimaridou E, Storr HL, Metherell LA. Oxidative stress and adrenocortical insufficiency. J Endocrinol (2014) 221(3):R63–73. doi: 10.1530/JOE-13-0346
34. Kochman J, Jakubczyk K. The influence of oxidative stress on thyroid diseases. Antioxidants (2021) 10:1442. doi: 10.3390/antiox10091442
35. Prajapati D, Patani A, Jain T, Patel A, Singh S. ROS responsive silica nanoparticles for controlled and targeted drug delivery. In: Chawla S, Singh S, Husen A, editors. Smart Nanomaterials Targeting Pathological Hypoxia. Smart Nanomaterials Technology. Singapore: Springer (2023). p. 327–46. doi: 10.1007/978-981-99-1718-1_17
36. Macvanin MT, Gluvic Z, Zafirovic S, Gao X, Essack M, Isenovic ER. The protective role of nutritional antioxidants against oxidative stress in thyroid disorders. Front Endocrinol (2023) 13:1092837. doi: 10.3389/fendo.2022.1092837
37. Mangge H, Becker K, Fuchs D, Gostner JM. Antioxidants, inflammation and cardiovascular disease. World J Cardiol (2014) 6:462–77. doi: 10.4330/wjc.v6.i6.462
38. Bardaweel SK, Gul M, Alzweiri M, Ishaqat A, ALSalamat HA, Bashatwah RM. Reactive oxygen species: the dual role in physiological and pathological conditions of the human body. Eurasian J Med (2018) 50(3):193–201. doi: 10.5152/eurasianjmed.2018.17397
39. Sharifi-Rad M, Anil Kumar NV, Zucca P, Varoni EM, Dini L, Panzarini E, et al. Lifestyle, oxidative stress, and antioxidants: back and forth in the pathophysiology of chronic diseases. Front Physiol (2020) 11:694. doi: 10.3389/fphys.2020.00694
40. Singh A, Kukreti R, Saso L, Kukreti S. Oxidative stress: A key modulator in neurodegenerative diseases. Molecules (2019) 24(8):1583. doi: 10.3390/molecules24081583
41. Liu Z, Ren Z, Zhang J, Chuang CC, Kandaswamy E, Zhou T, et al. Role of ROS and nutritional antioxidants in human diseases. Front Physiol (2018) 9:477. doi: 10.3389/fphys.2018.00477
42. Lehrer S, Rheinstein PH. Alzheimer's disease and parkinson's disease may result from reactivation of embryologic pathways silenced at birth. Discov Med (2021) 31(163):89–94.
43. Turrens JF, Boveris A. Generation of superoxide anion by the NADH dehydrogenase of bovine heart mitochondria. Biochem J (1980) 191:421–7. doi: 10.1042/bj1910421
44. Zimmermann MB, Aeberli I. Dietary determinants of subclinical inflammation, dyslipidemia and components of the metabolic syndrome in overweight children: a review. Int J Obes (Lond) (2008) 32 Suppl 6:S11–18. doi: 10.1038/ijo.2008.202
45. Mancini A, Di Segni C. Thyroid hormones, oxidative stress, and inflammation. Mediators Inflammation (2016) 2016:6757154. doi: 10.1155/2016/6757154
46. Siti HN, Kamisah Y, Kamsiah J. The role of oxidative stress, antioxidants and vascular inflammation in cardiovascular disease (a review). VasculPharmacol (2015) 71:40–56. doi: 10.1016/j.vph.2015.03.005
47. Tan BL, Norhaizan ME, Liew WP, Sulaiman Rahman H. Antioxidant and oxidative stress: A mutual interplay in age-related diseases. Front Pharmacol (2018) 9:1162. doi: 10.3389/fphar.2018.01162
48. Patani A, Patel A, Prajapati D, Khare N, Singh S. Various agriculture crop plant-based bioactive compounds and their use in nanomaterial synthesis and applications. In: Husen A, editor. Nanomaterials from Agricultural and Horticultural Products. Smart Nanomaterials Technology. Singapore: Springer (2023). p. 323–241. doi: 10.1007/978-981-99-3435-5_12
49. Hinds JA, Sanchez ER. The role of the hypothalamus–pituitary–adrenal (HPA) axis in test-induced anxiety: assessments, physiological responses, and molecular details. Stresses (2022) 2(1):146–55. doi: 10.3390/stresses2010011
50. Hannibal KE, Bishop MD. Chronic stress, cortisol dysfunction, and pain: a psychoneuroendocrine rationale for stress management in pain rehabilitation. Phys Ther (2014) 94(12):1816–25. doi: 10.2522/ptj.20130597
51. Juszczyk G, Mikulska J, Kasperek K, Pietrzak D, Mrozek W, Herbet M. Chronic stress and oxidative stress as common factors of the pathogenesis of depression and alzheimer's disease: the role of antioxidants in prevention and treatment. Antioxidants (Basel) (2021) 10(9):1439. doi: 10.3390/antiox10091439
52. Di Domenico M, Pinto F, Quagliuolo L, Contaldo M, Settembre G, Romano A, et al. The role of oxidative stress and hormones in controlling obesity. Front Endocrinol (Lausanne) (2019) 10:540. doi: 10.3389/fendo.2019.00540
53. Sahoo DK, Heilmann RM, Paital B, Patel A, Yadav VK, Wong D, et al. Oxidative stress, hormones, and effects of natural antioxidants on intestinal inflammation in inflammatory bowel disease. Front Endocrinol (Lausanne) (2023) 14:1217165. doi: 10.3389/fendo.2023.1217165
54. Herman JP, McKlveen JM, Ghosal S, Kopp B, Wulsin A, Makinson R, et al. Regulation of the hypothalamic-pituitary-adrenocortical stress response. Compr Physiol (2016) 6(2):603–21. doi: 10.1002/cphy.c150015
55. Sheng JA, Bales NJ, Myers SA, Bautista AI, Roueinfar M, Hale TM, et al. The hypothalamic-pituitary-adrenal axis: development, programming actions of hormones, and maternal-fetal interactions. Front Behav Neuro Sci (2021) 14:601939. doi: 10.3389/fnbeh.2020.601939
56. Ames MK, Atkins CE, Pitt B. The renin-angiotensin-aldosterone system and its suppression. J Vet Intern Med (2019) 33(2):363–82. doi: 10.1111/jvim
57. Traish A, Bolanos J, Nair S, Saad F, Morgentaler A. Do androgens modulate the pathophysiological pathways of inflammation? Appraising the contemporary evidence. J Clin Med (2018) 7(12):549. doi: 10.3390/jcm7120549
58. Sahoo DK, Chainy GBN. Hormone-linked redox status and its modulation by antioxidants. VitamHorm (2023) 121:197–246. doi: 10.1016/bs.vh.2022.10.007
59. Chainy GBN, Sahoo DK. Hormones and oxidative stress: an overview. Free Radic Res (2020) 54(1):1–26. doi: 10.1080/10715762.2019.1702656
60. Sugino N, Hirosawa-Takamori M, Zhong L, Telleria CM, Shiota K, Gibori G. Hormonal regulation of copper-zinc superoxide dismutase and manganese superoxide dismutase messenger ribonucleic acid in the rat corpus luteum: induction by prolactin and placental lactogens. Biol Reprod (1998) 59(3):599–605. doi: 10.1095/biolreprod59.3.599
61. Memarzia A, Khazdair MR, Behrouz S, Gholamnezhad Z, Jafarnezhad M, Saadat S, et al. Experimental and clinical reports on anti-inflammatory, antioxidant, and immunomodulatory effects of Curcuma longa and curcumin, an updated and comprehensive review. Biofactors (2021) 47(3):311–50. doi: 10.1002/biof.1716
62. Spiers JG, Chen HJ, Sernia C, Lavidis NA. Activation of the hypothalamic-pituitary-adrenal stress axis induces cellular oxidative stress. Front Neurosci (2015) 8:456. doi: 10.3389/fnins.2014.00456
63. Lee KH, Cha M, Lee BH. Neuroprotective effect of antioxidants in the brain. Int J Mol Sci (2020) 21(19):7152. doi: 10.3390/ijms21197152
64. Birnie-Gauvin K, Peiman KS, Larsen MH, Aarestrup K, Willmore WG, Cooke SJ. Short-term and long-term effects of transient exogenous cortisol manipulation on oxidative stress in juvenile brown trout. J Exp Biol (2017) 220(Pt 9):1693–700. doi: 10.1242/jeb.155465
65. Bisht S, Dada R. Oxidative stress: Major executioner in disease pathology, role in sperm DNA damage and preventive strategies. Front Biosci (Schol Ed) (2017) 9(3):420–47. doi: 10.2741/s495
66. Weinberg-Shukron A, Abu-Libdeh A, Zhadeh F, Carmel L, Kogot-Levin A, Kamal L, et al. Combined mineralocorticoid and glucocorticoid deficiency is caused by a novel founder nicotinamide nucleotide transhydrogenase mutation that alters mitochondrial morphology and increases oxidative stress. J Med Genet (2015) 52(9):636–41. doi: 10.1136/jmedgenet-2015-103078
67. Mocan M, MocanHognogi LD, Anton FP, Chiorescu RM, Goidescu CM, Stoia MA, et al. Biomarkers of inflammation in left ventricular diastolic dysfunction. Dis Markers (2019) 2019:7583690. doi: 10.1155/2019/7583690
68. Lang F. On the pleotropic actions of mineralocorticoids. Nephron Physiol (2014) 128(1-2):1–7. doi: 10.1159/000368263
69. Ayuzawa N, Fujita T. The mineralocorticoid receptor in salt-sensitive hypertension and renal injury. J Am Soc Nephrol (2021) 32(2):279–89. doi: 10.1681/ASN.2020071041
70. Patni H, Mathew JT, Luan L, Franki N, Chander PN, Singhal PC. Aldosterone promotes proximal tubular cell apoptosis: role of oxidative stress. Am J Physiol Renal Physiol (2007) 293(4):F1065–71. doi: 10.1152/ajprenal.00147.2007
71. Martemucci G, Costagliola C, Mariano M, D’andrea L, Napolitano P, D’Alessandro AG. Free radical properties, source and targets, antioxidant consumption and health. Oxygen (2022) 2(2):48–78. doi: 10.3390/oxygen2020006
72. Tesch GH, Young MJ. Mineralocorticoid receptor signaling as a therapeutic target for renal and cardiac fibrosis. Front Pharmacol (2017) 8:313. doi: 10.3389/fphar.2017.00313
73. Prajapati DH, Patel MD, Mehta HH, Patel DA, Dave DP, Patel JR, et al. A review on patient diet management for chronic kidney disease based on clinical trials. IJRESM (2022) 5(11):174–81.
74. Su X, Wang S, Zhang H, Yang G, Bai Y, Liu P, et al. Sulforaphane prevents angiotensin II-induced cardiomyopathy by activation of Nrf2 through epigenetic modification. J Cell Mol Med (2021) 25(9):4408–19. doi: 10.1111/jcmm.16504
75. Brinks R, Wruck CJ, Schmitz J, Schupp N. Nrf2 activation does not protect from aldosterone-induced kidney damage in mice. Antioxidants (2023) 12(3):777. doi: 10.3390/antiox12030777
76. Ramya S, Poornima P, Jananisri A, Geofferina IP, Bavyataa V, Divya M, et al. Role of hormones and the potential impact of multiple stresses on infertility. Stresses (2023) 3(2):454–74. doi: 10.3390/stresses3020033
77. Imran M, Ghorat F, Ul-Haq I, Ur-Rehman H, Aslam F, Heydari M, et al. Lycopene as a natural antioxidant used to prevent human health disorders. Antioxidants (2020) 9(8):706. doi: 10.3390/antiox9080706
78. Yan W, Kang Y, Ji X, Li S, Li Y, Zhang G, et al. Testosterone upregulates the expression of mitochondrial ND1 and ND4 and alleviates the oxidative damage to the nigrostriatal dopaminergic system in orchiectomized rats. Oxid Med Cell Longev (2017) 2017:1202459. doi: 10.1155/2017/1202459
79. Witchel SF, Oberfield SE, Peña AS. Polycystic ovary syndrome: pathophysiology, presentation, and treatment with emphasis on adolescent girls. J Endocr Soc (2019) 3(8):1545–73. doi: 10.1210/js.2019-00078
80. Tam NN, Gao Y, Leung YK, Ho SM. Androgenic regulation of oxidative stress in the rat prostate: involvement of NAD(P)H oxidases and antioxidant defense machinery during prostatic involution and regrowth. Am J Pathol (2003) 163(6):2513–22. doi: 10.1016/S0002-9440(10)63606-1
81. Ripple MO, Henry WF, Rago RP, Wilding G. Prooxidant-antioxidant shift induced by androgen treatment of human prostate carcinoma cells. J Natl Cancer Inst (1997) 89(1):40–8. doi: 10.1093/jnci/89.1.40
82. Prasad S, Kalra N, Singh M, Shukla Y. Protective effects of lupeol and mango extract against androgen induced oxidative stress in Swiss albino mice. Asian J Androl (2008) 10(2):313–8. doi: 10.1111/j.1745-7262.2008.00313.x
83. Argenziano M, Tiscornia G, Moretta R, Casal L, Potilinski C, Amorena C, et al. Arrhythmogenic effect of androgens on the rat heart. J Physiol Sci (2017) 67(1):217–25. doi: 10.1007/s12576-016-0459-y
84. Blanco-Rivero J, Sagredo A, Balfagón G, Ferrer M. Orchidectomy increases expression and activity of Cu/Zn-superoxide dismutase, while decreasing endothelial nitric oxide bioavailability. J Endocrinol (2006) 190(3):771–8. doi: 10.1677/joe.1.06887
85. Borst SE, Quindry JC, Yarrow JF, Conover CF, Powers SK. Testosterone administration induces protection against global myocardial ischemia. HormMetab Res (2010) 42(2):122–9. doi: 10.1055/s-0029-1241843
86. Kłapcińska B, Jagsz S, Sadowska-Krepa E, Górski J, Kempa K, Langfort J. Effects of castration and testosterone replacement on the antioxidant defense system in rat left ventricle. J Physiol Sci (2008) 58(3):173–7. doi: 10.2170/physiolsci.RP002208
87. Szabó R, Börzsei D, Kupai K, Hoffmann A, Gesztelyi R, MagyarinéBerkó A, et al. Spotlight on a new heme oxygenase pathway: testosterone-induced shifts in cardiac oxidant/antioxidant status. Antioxidants (2019) 8(8):288. doi: 10.3390/antiox8080288
88. Samaras N, Samaras D, Frangos E, Forster A, Philippe J. A review of age-related dehydroepiandrosterone decline and its association with well-known geriatric syndromes: is treatment beneficial? Rejuvenation Res (2013) 16(4):285–94. doi: 10.1089/rej.2013.1425
89. Powrie YSL, Smith C. Central intracrine DHEA synthesis in ageing-related neuroinflammation and neurodegeneration: therapeutic potential? J Neuroinflamm (2018) 15:289. doi: 10.1186/s12974-018-1324-0
90. Mohammadi M. Oxidative stress and polycystic ovary syndrome: A brief review. Int J Prev Med (2019) 10:86. doi: 10.4103/ijpvm.IJPVM_576_17
91. Shabbir S, Khurram E, Moorthi VS, Eissa YTH, Kamal MA, Butler AE. The interplay between androgens and the immune response in polycystic ovary syndrome. J Transl Med (2023) 21(1):259. doi: 10.1186/s12967-023-04116-4
92. González F, Sia CL, Shepard MK, Rote NS, Minium J. Hyperglycemia-induced oxidative stress is independent of excess abdominal adiposity in normal-weight women with polycystic ovary syndrome. Hum Reprod (2012) 27(12):3560–8. doi: 10.1093/humrep/des320
93. Hardeland R, Pandi-Perumal SR. Melatonin, a potent agent in antioxidative defense: actions as a natural food constituent, gastrointestinal factor, drug and prodrug. NutrMetab (Lond) (2005) 2:22. doi: 10.1186/1743-7075-2-22
95. Lobo V, Patil A, Phatak A, Chandra N. Free radicals, antioxidants and functional foods: Impact on human health. Pharmacogn Rev (2010) 4(8):118–26. doi: 10.4103/0973-7847.70902
96. Chambial S, Dwivedi S, Shukla KK, John PJ, Sharma P. Vitamin C in disease prevention and cure: an overview. Indian J Clin Biochem (2013) 28(4):314–28. doi: 10.1007/s12291-013-0375-3
97. Carr AC, Maggini S. Vitamin C and immune function. Nutrients (2017) 9(11):1211. doi: 10.3390/nu9111211
98. Piskin E, Cianciosi D, Gulec S, Tomas M, Capanoglu E. Iron absorption: factors, limitations, and improvement methods. ACS Omega (2022) 7(24):20441–56. doi: 10.1021/acsomega.2c01833
99. Jideani AI, Silungwe H, Takalani T, Omolola AO, Udeh HO, Anyasi TA. Antioxidant-rich natural fruit and vegetable products and human health. Int J Food Prop (2021) 24(1):41–67. doi: 10.1080/10942912.2020.1866597
100. Marik PE. Vitamin C: an essential "stress hormone" during sepsis. J Thorac Dis (2020) 12(Suppl 1):S84–8. doi: 10.21037/jtd.2019.12.64
101. Pullar JM, Carr AC, Vissers MCM. The roles of vitamin C in skin health. Nutrients (2017) 9(8):866. doi: 10.3390/nu9080866
102. Brody S. High-dose ascorbic acid increases intercourse frequency and improves mood: a randomized controlled clinical trial. Biol Psychiatry (2002) 52:371–4. doi: 10.1016/S0006-3223(02)01329-X
103. Patak P, Willenberg HS, Bornstein SR. Vitamin C is an important cofactor for both adrenal cortex and adrenal medulla. Endocr Res (2004) 30:871–5. doi: 10.1081/ERC-200044126
104. Jenkins JS. The effect of ascorbic acid on adrenal steroid synthesis in Vitro. Endocrinology (1962) 70:267–71. doi: 10.1210/endo-70-2-267
105. Moritz B, Schmitz AE, Rodrigues ALS, Dafre AL, Cunha MP. The role of vitamin C in stress-related disorders. J NutrBiochem (2020) 85:108459. doi: 10.1016/j.jnutbio.2020.108459
106. Shahidi F, Pinaffi-Langley ACC, Fuentes J, Speisky H, de Camargo AC. Vitamin E as an essential micronutrient for human health: Common, novel, and unexplored dietary sources. Free Radic Biol Med (2021) 176:312–21. doi: 10.1016/j.freeradbiomed.2021.09.025
107. Lee GY, Han SN. The role of vitamin E in immunity. Nutrients (2018) 10(11):1614. doi: 10.3390/nu10111614
108. Ciarcià G, Bianchi S, Tomasello B, Acquaviva R, Malfa GA, Naletova I, et al. Vitamin E and non-communicable diseases: A review. Biomedicines (2022) 10(10):2473. doi: 10.3390/biomedicines10102473
109. Pincemail J, Meziane S. On the potential role of the antioxidant couple vitamin E/selenium taken by the oral route in skin and hair health. Antioxidants (Basel) (2022) 11(11):2270. doi: 10.3390/antiox11112270
110. Sahoo DK, Roy A, Chainy GB. Protective effects of vitamin E and curcumin on L-thyroxine-induced rat testicular oxidative stress. Chem Biol Interact (2008) 176(2-3):121–8. doi: 10.1016/j.cbi.2008.07.009
111. Singh U, Devaraj S, Jialal I. Vitamin E, oxidative stress, and inflammation. Annu Rev Nutr (2005) 25:151–74. doi: 10.1146/annurev.nutr.24.012003.132446
112. Sun Y, Ma A, Li Y, Han X, Wang Q, Liang H. Vitamin E supplementation protects erythrocyte membranes from oxidative stress in healthy Chinese middle-aged and elderly people. Nutr Res (2012) 32(5):328–34. doi: 10.1016/j.nutres.2012.03.012
113. Dhama K, Latheef SK, Dadar M, Samad HA, Munjal A, Khandia R, et al. Biomarkers in stress related diseases/disorders: diagnostic, prognostic, and therapeutic values. Front Mol Biosci (2019) 6:91. doi: 10.3389/fmolb.2019.00091
114. Al-Sowayan NS. Possible modulation of nervous tension-induced oxidative stress by vitamin E. Saudi J Biol Sci (2020) 27(10):2563–6. doi: 10.1016/j.sjbs.2020.05.018
115. Ponnampalam EN, Kiani A, Santhiravel S, Holman BWB, Lauridsen C, Dunshea FR. The importance of dietary antioxidants on oxidative stress, meat and milk production, and their preservative aspects in farm animals: antioxidant action, animal health, and product quality—Invited review. Animals (2022) 12(23):3279. doi: 10.3390/ani12233279
116. Maoka T. Carotenoids as natural functional pigments. J Nat Med (2020) 74(1):1–16. doi: 10.1007/s11418-019-01364-x
117. Swapnil P, Meena M, Singh SK, Dhuldhaj UP, Marwal A. Vital roles of carotenoids in plants and humans to deteriorate stress with its structure, biosynthesis, metabolic engineering and functional aspects. Curr Plant Biol (2021) 26:100203. doi: 10.1016/j.cpb.2021.100203
118. Black HS, Boehm F, Edge R, Truscott TG. The benefits and risks of certain dietary carotenoids that exhibit both anti- and pro-oxidative mechanisms-A comprehensive review. Antioxidants (Basel) (2020) 9(3):264. doi: 10.3390/antiox9030264
119. Mrowicka M, Mrowicki J, Kucharska E, Majsterek I. Lutein and zeaxanthin and their roles in age-related macular degeneration-neurodegenerative disease. Nutrients (2022) 14(4):827. doi: 10.3390/nu14040827
120. Crupi P, Faienza MF, Naeem MY, Corbo F, Clodoveo ML, Muraglia M. Overview of the potential beneficial effects of carotenoids on consumer health and well-being. Antioxidants (Basel) (2023) 12(5):1069. doi: 10.3390/antiox12051069
121. Saini RK, Prasad P, Lokesh V, Shang X, Shin J, Keum YS, et al. Carotenoids: dietary sources, extraction, encapsulation, bioavailability, and health benefits-A review of recent advancements. Antioxidants (Basel) (2022) 11(4):795. doi: 10.3390/antiox11040795
122. Elvira-Torales LI, García-Alonso J, Periago-Castón MJ. Nutritional importance of carotenoids and their effect on liver health: A review. Antioxidants (Basel) (2019) 8(7):229. doi: 10.3390/antiox8070229
123. Kabir MT, Rahman MH, Shah M, Jamiruddin MR, Basak D, Al-Harrasi A, et al. Therapeutic promise of carotenoids as antioxidants and anti-inflammatory agents in neurodegenerative disorders. BioMed Pharmacother (2022) 146:112610. doi: 10.1016/j.biopha.2021.112610
124. Iddir M, Brito A, Dingeo G, Fernandez Del Campo SS, Samouda H, La Frano MR, et al. Strengthening the Immune System and Reducing Inflammation and Oxidative Stress through Diet and Nutrition: Considerations during the COVID-19 Crisis. Nutrients (2020) 12(6):1562. doi: 10.3390/nu12061562
125. Coulter AA, Greenway FL, Zhang D, Ghosh S, Coulter CR, James SL, et al. Naringenin and β-carotene convert human white adipocytes to a beige phenotype and elevate hormone- stimulated lipolysis. Front Endocrinol (Lausanne) (2023) 14:1148954. doi: 10.3389/fendo.2023.1148954
126. Darvin ME, Lademann J, von Hagen J, Lohan SB, Kolmar H, Meinke MC, et al. Carotenoids in human skinIn vivo: antioxidant and photo-protectant role against external and internal stressors. Antioxidants (Basel) (2022) 11(8):1451. doi: 10.3390/antiox11081451
127. Bremner JD, Shearer KD, McCaffery PJ. Retinoic acid and affective disorders: The evidence for an association. J Clin Psychiatry (2012) 73:37–50. doi: 10.4088/JCP.10r05993
128. Ludot M, Mouchabac S, Ferreri F. Inter-relationships between isotretinoin treatment and psychiatric disorders: Depression, bipolar disorder, anxiety, psychosis and suicide risks. World J Psychiatry (2015) 5:222–7. doi: 10.5498/wjp.v5.i2.222
129. Oishi J, Doi H, Kawakami N. Nutrition and depressive symptoms in community-dwelling elderly persons in Japan. Acta Med Okayama (2009) 63:9–17. doi: 10.18926/AMO/31854
130. Xiao J, Khan MZ, Ma Y, Alugongo GM, Ma J, Chen T, et al. The antioxidant properties of selenium and vitamin E; their role in periparturient dairy cattle health regulation. Antioxidants (2021) 10(10):1555. doi: 10.3390/antiox10101555
131. Tinggi U. Selenium: its role as antioxidant in human health. Environ Health Prev Med (2008) 13(2):102–8. doi: 10.1007/s12199-007-0019-4
132. Avery JC, Hoffmann PR. Selenium, selenoproteins, and immunity. Nutrients (2018) 10(9):1203. doi: 10.3390/nu10091203
133. Gorini F, Sabatino L, Pingitore A, Vassalle C. Selenium: an element of life essential for thyroid function. Molecules (2021) 26(23):7084. doi: 10.3390/molecules26237084
134. Shalihat A, Hasanah AN, Mutakin, Lesmana R, Budiman A, Gozali D. The role of selenium in cell survival and its correlation with protective effects against cardiovascular disease: A literature review. BioMed Pharmacother (2021) 134:111125. doi: 10.1016/j.biopha.2020.111125
135. Kipp AP, Strohm D, Brigelius-Flohé R, Schomburg L, Bechthold A, Leschik-Bonnet E, et al. Revised reference values for selenium intake. J Trace Elem Med Biol (2015) 32:195–9. doi: 10.1016/j.jtemb.2015.07.005
136. Sahoo DK, Roy A, Bhanja S, Chainy GB. Hypothyroidism impairs antioxidant defence system and testicular physiology during development and maturation. Gen Comp Endocrinol (2008) 156(1):63–70. doi: 10.1016/j.ygcen.2007.11.007
137. Zoidis E, Seremelis I, Kontopoulos N, Danezis GP. Selenium-dependent antioxidant enzymes: actions and properties of selenoproteins. Antioxidants (Basel) (2018) 7(5):66. doi: 10.3390/antiox7050066
138. Andrade IGA, Suano-Souza FI, Fonseca FLA, Lago CSA, Sarni ROS. Selenium levels and glutathione peroxidase activity in patients with ataxia-telangiectasia: association with oxidative stress and lipid status biomarkers. Orphanet J Rare Dis (2021) 16(1):83. doi: 10.1186/s13023-021-01732-5
139. Toh P, Nicholson JL, Vetter AM, Berry MJ, Torres DJ. Selenium in bodily homeostasis: hypothalamus, hormones, and highways of communication. Int J Mol Sci (2022) 23(23):15445. doi: 10.3390/ijms232315445
140. Sahoo DK. Testicular protection from thyroid hormone mediated oxidative stress. WebmedCentral Reprod (2013) 4(5):WMC004252. doi: 10.9754/journal.wmc.2013.004252
141. McEwen BS. Physiology and neurobiology of stress and adaptation: central role of the brain. Physiol Rev (2007) 87:873–904. doi: 10.1152/physrev.00041.2006
142. Juszczak GR, Stankiewicz AM. Glucocorticoids, genes and brain function. Prog Neuro-Psychopharmacol Biol Psychiatry (2018) 82:136–68. doi: 10.1016/j.pnpbp.2017.11.020
143. Vandewalle J, Luypaert A, De Bosscher K, Libert C. Therapeutic mechanisms of glucocorticoids. Trends Endocrinol Metab (2018) 29:42–54. doi: 10.1016/j.tem.2017.10.010
144. Torres DJ, Alfulaij N, Berry MJ. Stress and the brain: an emerging role for selenium. Front Neurosci (2021) 15:666601. doi: 10.3389/fnins.2021.666601
145. Kaixin Z, Xuedie G, Jing L, Yiming Z, Khoso PA, Zhaoyi L, et al. Selenium-deficient diet induces inflammatory response in the pig adrenal glands by activating TLR4/NF-κB pathway via miR-30d-R. Metallomics (2021) 13:mfab037. doi: 10.1093/mtomcs/mfab037
146. Wang C, Zhang Y, Luo J, Ding H, Liu S, Amer S, et al. Identification of miRNomes reveals ssc-miR-30d-R_1 as a potential therapeutic target for PRRS viral infection. Sci Rep (2016) 6:24854. doi: 10.1038/srep24854
147. Lai F, Zhou G, Mai S, Qin X, Liu W, Zhang Y, et al. Sini decoction improves adrenal function and the short-term outcome of septic rats through downregulation of adrenal toll-like receptor 4 expression. Evid Based ComplementAlternat Med (2018) 2018:5186158. doi: 10.1155/2018/5186158
148. Abid H, Cartier D, Hamieh A, François-Bellan AM, Bucharles C, Pothion H, et al. AMPK activation of PGC-1α/NRF-1-dependent SELENOT gene transcription promotes PACAP-induced neuroendocrine cell differentiation through tolerance to oxidative stress. Mol Neurobiol (2018) 56:4086–101. doi: 10.1007/s12035-018-1352-x
149. Gombart AF, Pierre A, Maggini S. A review of micronutrients and the immune system–working in harmony to reduce the risk of infection. Nutrients (2020) 12(1):236. doi: 10.3390/nu12010236
150. Sahoo DK. Protocols for evaluating antioxidant defence and oxidative stress parameters in rat testis. WebmedCentral Biochem (2013) 4(5):WMC004265. doi: 10.9754/journal.wmc.2013.004265
151. Maywald M, Rink L. Zinc in human health and infectious diseases. Biomolecules (2022) 12(12):1748. doi: 10.3390/biom12121748
152. Lee SR. Critical role of zinc as either an antioxidant or a prooxidant in cellular systems. Oxid Med Cell Longev (2018) 2018:9156285. doi: 10.1155/2018/9156285
153. Baltaci AK, Mogulkoc R, Baltaci SB. Review: The role of zinc in the endocrine system. Pak J Pharm Sci (2019) 32(1):231–9.
154. Wu Q, Gao ZJ, Yu X, Wang P. Dietary regulation in health and disease. Signal Transduct Target Ther (2022) 7(1):252. doi: 10.1038/s41392-022-01104-w
155. Yildiz A, Kaya Y, Tanriverdi O. Effect of the interaction between selenium and zinc on DNA repair in association with cancer prevention. J Cancer Prev (2019) 24(3):146–54. doi: 10.15430/JCP.2019.24.3.146
156. Olechnowicz J, Tinkov A, Skalny A, Suliburska J. Zinc status is associated with inflammation, oxidative stress, lipid, and glucose metabolism. J Physiol Sci (2018) 68(1):19–31. doi: 10.1007/s12576-017-0571-7
157. Morais JBS, Dias TMDS, Cardoso BEP, de Paiva Sousa M, Sousa TGV, Araújo DSC, et al. Adipose tissue dysfunction: impact on metabolic changes? HormMetabRes (2022) 54(12):785–94. doi: 10.1055/a-1922-7052
158. Morais JBS, Severo JS, Beserra JB, de Oiveira ARS, Cruz KJC, de Sousa Melo SR, et al. Association between cortisol, insulin resistance and zinc in obesity: a mini-review. Biol Trace Elem Res (2019) 191(2):323–30. doi: 10.1007/s12011-018-1629-y
159. Michalak M. Plant-derived antioxidants: significance in skin health and the ageing process. Int J Mol Sci (2022) 23(2):585. doi: 10.3390/ijms23020585
160. Rudrapal M, Khairnar SJ, Khan J, Dukhyil AB, Ansari MA, Alomary MN, et al. Dietary polyphenols and their role in oxidative stress-induced human diseases: insights into protective effects, antioxidant potentials and mechanism(s) of action. Front Pharmacol (2022) 13:806470. doi: 10.3389/fphar.2022.806470
161. Bié J, Sepodes B, Fernandes PCB, Ribeiro MHL. Polyphenols in health and disease: gut microbiota, bioaccessibility, and bioavailability. Compounds (2023) 3(1):40–72. doi: 10.3390/compounds3010005
162. Zhang Z, Li X, Sang S, McClements DJ, Chen L, Long J, et al. Polyphenols as plant-based nutraceuticals: health effects, encapsulation, nano-delivery, and application. Foods (2022) 11(15):2189. doi: 10.3390/foods11152189
163. Gessner DK, Ringseis R, Eder K. Potential of plant polyphenols to combat oxidative stress and inflammatory processes in farm animals. J AnimPhysiolAnimNutr (Berl) (2017) 101(4):605–28. doi: 10.1111/jpn.12579
164. Shabbir U, Tyagi A, Elahi F, Aloo SO, Oh DH. The potential role of polyphenols in oxidative stress and inflammation induced by gut microbiota in alzheimer's disease. Antioxidants (Basel) (2021) 10(9):1370. doi: 10.3390/antiox10091370
165. Ighodaro OM, Akinloye OA. First line defence antioxidants-superoxide dismutase (SOD), catalase (CAT) and glutathione peroxidase (GPX): Their fundamental role in the entire antioxidant defence grid. Alexandria J Med (2018) 54(4):287–93. doi: 10.1016/j.ajme.2017.09.001
166. Gopinath K, Sudhandiran G. Naringin modulates oxidative stress and inflammation in 3-nitropropionic acid-induced neurodegeneration through the activation of nuclear factor-erythroid 2-related factor-2 signalling pathway. Neuroscience (2012) 227:134–43. doi: 10.1016/j.neuroscience
167. Wei K, Xu Y, Zhao Z, Wu X, Du Y, Sun J, et al. Icariin alters the expression of glucocorticoid receptor, FKBP5 and SGK1 in rat brains following exposure to chronic mild stress. Int J Mol Med (2016) 38(1):337–44. doi: 10.3892/ijmm.2016.2591
168. Donoso F, Ramírez VT, Golubeva AV, Moloney GM, Stanton C, Dinan TG, et al. Naturally derived polyphenols protect against corticosterone-induced changes in primary cortical neurons. Int J Neuropsychopharmacol (2019) 22(12):765–77. doi: 10.1093/ijnp/pyz052
169. Sifuentes-Franco S, Sánchez-Macías DC, Carrillo-Ibarra S, Rivera-Valdés JJ, Zuñiga LY, Sánchez-López VA. Antioxidant and anti-inflammatory effects of coenzyme Q10 supplementation on infectious diseases. Healthcare (Basel) (2022) 10(3):487. doi: 10.3390/healthcare10030487
170. Barcelos IP, Haas RH. CoQ10 and aging. Biol (Basel) (2019) 8(2):28. doi: 10.3390/biology8020028
171. Saini R. Coenzyme Q10: The essential nutrient. J Pharm Bioallied Sci (2011) 3(3):466–7. doi: 10.4103/0975-7406.84471
172. Silva SVE, Gallia MC, Luz JRDD, Rezende AA, Bongiovanni GA, Araujo-Silva G, et al. Antioxidant effect of coenzyme Q10 in the prevention of oxidative stress in arsenic-treated CHO-K1 cells and possible participation of zinc as a pro-oxidant agent. Nutrients (2022) 14(16):3265. doi: 10.3390/nu14163265
173. Gutierrez-Mariscal FM, Arenas-de Larriva AP, Limia-Perez L, Romero-Cabrera JL, Yubero-Serrano EM, López-Miranda J. Coenzyme Q10 supplementation for the reduction of oxidative stress: clinical implications in the treatment of chronic diseases. Int J Mol Sci (2020) 21(21):7870. doi: 10.3390/ijms21217870
174. Hajiluian G, Heshmati J, Jafari Karegar S, Sepidarkish M, Shokri A, Shidfar F. Diabetes, age, and duration of supplementation subgroup analysis for the effect of coenzyme Q10 on oxidative stress: A systematic review and meta-analysis. Complement Med Res (2021) 28(6):557–70. doi: 10.1159/000515249
175. López-Lluch G. Coenzyme Q homeostasis in aging: Response to non-genetic interventions. Free Radic Biol Med (2021) 164:285–302. doi: 10.1016/j.freeradbiomed
176. Ježková J, Ďurovcová V, Wenchich L, Hansíková H, Zeman J, Hána V, et al. The relationship of mitochondrial dysfunction and the development of insulin resistance in Cushing's syndrome. Diabetes MetabSyndrObes (2019) 12:1459–71. doi: 10.2147/DMSO.S209095
177. Karamouzis I, Berardelli R, D'Angelo V, Fussotto B, Zichi C, Giordano R, et al. Enhanced oxidative stress and platelet activation in patients with Cushing's syndrome. Clin Endocrinol (Oxf) (2015) 82(4):517–24. doi: 10.1111/cen.12524
178. Mancini A, Leone E, Silvestrini A, Festa R, Di Donna V, De Marinis L, et al. Evaluation of antioxidant systems in pituitary-adrenal axis diseases. Pituitary (2010) 13(2):138–45. doi: 10.1007/s11102-009-0213-z
179. Mantle D, Hargreaves IP. Coenzyme Q10 and endocrine disorders: an overview. Antioxidants (2023) 12(2):514. doi: 10.3390/antiox12020514
180. Wang Y, Wu Y, Wang Y, Xu H, Mei X, Yu D, et al. Antioxidant properties of probiotic bacteria. Nutrients (2017) 9(5):521. doi: 10.3390/nu9050521
181. Rodríguez-Daza MC, Pulido-Mateos EC, Lupien-Meilleur J, Guyonnet D, Desjardins Y, Roy D. Polyphenol-mediated gut microbiota modulation: toward prebiotics and further. Front Nutr (2021) 8:689456. doi: 10.3389/fnut.2021.689456
182. Wang X, Zhang P, Zhang X. Probiotics regulate gut microbiota: an effective method to improve immunity. Molecules (2021) 26(19):6076. doi: 10.3390/molecules26196076
183. Feng T, Wang J. Oxidative stress tolerance and antioxidant capacity of lactic acid bacteria as probiotic: a systematic review. Gut Microbes (2020) 12(1):1801944. doi: 10.1080/19490976.2020
184. Sabit H, Kassab A, Alaa D, Mohamed S, Abdel-Ghany S, Mansy M, et al. The effect of probiotic supplementation on the gut–brain axis in psychiatric patients. Curr Issues Mol Biol (2023) 45(5):4080–99. doi: 10.3390/cimb45050260
185. Hemarajata P, Versalovic J. Effects of probiotics on gut microbiota: mechanisms of intestinal immunomodulation and neuromodulation. Therap Adv Gastroenterol (2013) 6(1):39–51. doi: 10.1177/1756283X12459294
186. Plaza-Diaz J, Ruiz-Ojeda FJ, Gil-Campos M, Gil A. Mechanisms of action of probiotics. Adv Nutr (2019) 10(Suppl1):S49–S66. doi: 10.1093/advances/nmy063
187. Biswas S, Banerjee ER. Probiotic treatment of inflammatory bowel disease: Its extent and intensity. World J Immunol (2022) 12(2):15–24. doi: 10.5411/wji.v12.i2.15
188. Anker-Ladefoged C, Langkamp T, Mueller-Alcazar A. The potential impact of selected bacterial strains on the stress response. Healthcare (Basel) (2021) 9(5):494. doi: 10.3390/healthcare9050494
189. Martin CR, Osadchiy V, Kalani A, Mayer EA. The Brain-Gut-Microbiome Axis. Cell Mol Gastroenterol Hepatol (2018) 6(2):133. doi: 10.1016/J.JCMGH.2018.04.003
190. Sahoo DK, Borcherding DC, Chandra L, Jergens AE, Atherly T, Bourgois-Mochel A, et al. Differential Transcriptomic Profiles Following Stimulation with Lipopolysaccharide in Intestinal Organoids from Dogs with Inflammatory Bowel Disease and Intestinal Mast Cell Tumor. Cancers (2022) 14(14):3525. doi: 10.3390/CANCERS14143525
191. Neuman H., Debelius JW, Knight R., Koren O. Microbial endocrinology: the interplay between the microbiota and the endocrine system. FEMS Microbiol Rev (2015) 39(4):509–21. doi: 10.1093/FEMSRE/FUU010
192. Tsigos C, Chrousos GP. Hypothalamic–pituitary–adrenal axis, neuroendocrine factors and stress. J Psychosom Res (2002) 53(4):865–71. doi: 10.1016/S0022-3999(02)00429-4
193. Ait-Belgnaoui A, Durand H, Cartier C, Chaumaz G, Eutamene H, Ferrier L, et al. Prevention of gut leakiness by a probiotic treatment leads to attenuated HPA response to an acute psychological stress in rats. Psychoneuroendocrinology (2012) 37(11):1885–95. doi: 10.1016/J.PSYNEUEN.2012.03.024
194. Rani K, Kaur G, Ali SA. Probiotic-prebiotic therapeutic potential: a new horizon of microbial biotherapy to reduce female reproductive complications. Pharma Nutr (2023) 11:100342. doi: 10.1016/j.phanu.2023.100342
195. Takada M, Nishida K, Kataoka-Kato A, Gondo Y, Ishikawa H, Suda K, et al. Probiotic Lactobacillus casei strain Shirota relieves stress-associated symptoms by modulating the gut-brain interaction in human and animal models. Neurogastroenterol Motil (2016) 28(7):1027–36. doi: 10.1111/nmo.12804
196. Nasri K, Jamilian M, Rahmani E, Bahmani F, Tajabadi-Ebrahimi M, Asemi Z. The effects of synbiotic supplementation on hormonal status, biomarkers of inflammation and oxidative stress in subjects with polycystic ovary syndrome: a randomized, double-blind, placebo-controlled trial. BMC Endocr Disord (2018) 18(1):21. doi: 10.1186/s12902-018-0248-0
197. Aşır F, Nergiz Y, Pala A. Vitamin E protected the mouse adrenal gland against immobilization stress. Pol J Vet Sci (2022) 25(3):447–54. doi: 10.24425/pjvs.2022.142029
198. Nasiadek M, Stragierowicz J, Klimczak M, Kilanowicz A. The role of zinc in selected female reproductive system disorders. Nutrients (2020) 12(8):2464. doi: 10.3390/nu12082464
Keywords: adrenal hormone imbalance, oxidative stress, nutritional antioxidants, reactive oxygen species, HPT axis
Citation: Patani A, Balram D, Yadav VK, Lian K-Y, Patel A and Sahoo DK (2023) Harnessing the power of nutritional antioxidants against adrenal hormone imbalance-associated oxidative stress. Front. Endocrinol. 14:1271521. doi: 10.3389/fendo.2023.1271521
Received: 02 August 2023; Accepted: 17 November 2023;
Published: 30 November 2023.
Edited by:
Ashu Johri, Independent Researcher, New York, NY, United StatesReviewed by:
Shubhadeep Roychoudhury, Assam University, IndiaSonja S. Zafirovic, VINČA Institute of Nuclear Sciences - National Institute of the Republic of Serbia, Serbia
Mirjana Macvanin, University of Belgrade, Serbia
Fangdie Ye, Fudan University, China
Copyright © 2023 Patani, Balram, Yadav, Lian, Patel and Sahoo. This is an open-access article distributed under the terms of the Creative Commons Attribution License (CC BY). The use, distribution or reproduction in other forums is permitted, provided the original author(s) and the copyright owner(s) are credited and that the original publication in this journal is cited, in accordance with accepted academic practice. No use, distribution or reproduction is permitted which does not comply with these terms.
*Correspondence: Virendra Kumar Yadav, yadava94@gmail.com; Kuang-Yow Lian, kylian@mail.ntut.edu.tw; Ashish Patel, uni.ashish@gmail.com; Dipak Kumar Sahoo, dsahoo@iastate.edu