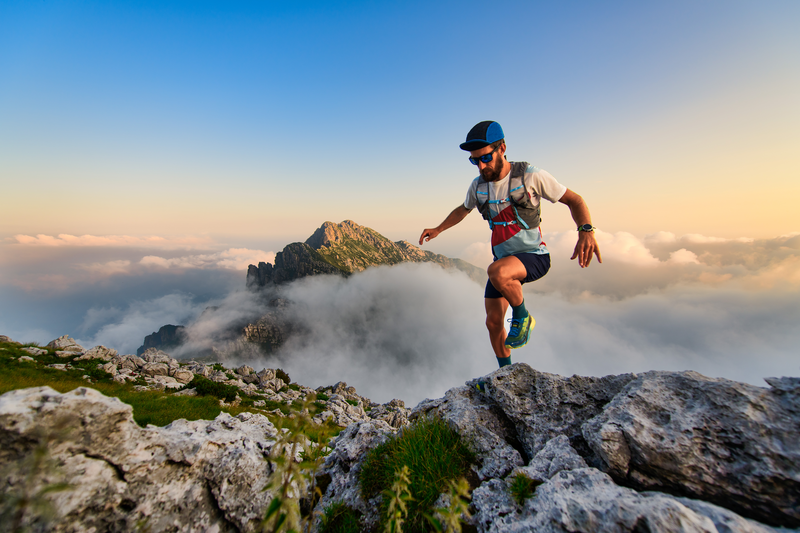
95% of researchers rate our articles as excellent or good
Learn more about the work of our research integrity team to safeguard the quality of each article we publish.
Find out more
ORIGINAL RESEARCH article
Front. Endocrinol. , 16 November 2023
Sec. Bone Research
Volume 14 - 2023 | https://doi.org/10.3389/fendo.2023.1266329
This article is part of the Research Topic Osteodietology View all 14 articles
Objective: The purpose of this study was to investigate the association between serum polyunsaturated fatty acids (PUFAs) and bone mineral density (BMD).
Methods: We performed a cross-sectional study based on data from the National Health and Nutrition Examination Survey (NHANES) 2011-2014. The weighted multiple linear regression model was utilized to determine the association between serum PUFAs and BMD. Further smoothed curve fitting and threshold effect analysis were conducted. Finally, we performed a subgroup analysis.
Results: In total, 1979 participants aged 20-59 years were enrolled. After adjusting for all covariates, we found that serum docosapentaenoic acid (DPA) was positively associated with head BMD (β = 0.0015, 95% Cl: 0.0004, 0.0026, P = 0.008296) and lumbar spine BMD (β = 0.0005, 95% Cl: 0.0000, 0.0010, P = 0.036093), and serum eicosadienoic acid (EDA) was negatively associated with thoracic spine BMD (β = -0.0008, 95% Cl: -0.0016, -0.0000, P = 0.045355). Smoothed curve fitting revealed a nonlinear positive association between serum DPA and lumbar spine BMD. Threshold effect analysis indicated that the threshold of serum DPA was 81.4 µmol/L. Subgroup analysis revealed a positive correlation between serum DPA and head BMD in the subgroup aged 50-59 years (β = 0.0025, 95% Cl: 0.0002, 0.0049, P = 0.035249) and females (β = 0.0026, 95% Cl: 0.0008, 0.0044, P = 0.005005). There was a positive relationship between serum DPA and lumbar spine BMD in females (β = 0.0008, 95% Cl: 0.0001, 0.0015, P = 0.017900) and a negative association between serum EDA and thoracic spine BMD in the subgroup aged 30-39 years (β = -0.0016, 95% Cl: -0.0031, -0.0001, P = 0.041331), males (β = -0.0012, 95% Cl: -0.0023, -0.0001, P = 0.039364) and other races (β = -0.0021, 95% Cl: -0.0037, -0.0006, P = 0.008059).
Conclusion: This study demonstrated a linear positive relationship between serum DPA and head BMD, a nonlinear positive association between serum DPA and lumbar spine BMD, and a linear negative correlation between serum EDA and thoracic spine BMD in US adults.
As a global public health issue, osteoporosis is defined as a degenerative skeletal disorder that manifests as the disruption of bone microstructure and reduced bone mass, resulting in decreased bone strength and higher fracture risk (1, 2). According to a report by the Surgeon General (US), approximately 10 million (M) Americans over the age of 50 years are affected by osteoporosis (3). In Europe, approximately 22M women and 5.5M men have been diagnosed with osteoporosis (4). Most importantly, osteoporosis-related fragility fractures can lead to poor quality of life, severe economic burden, and significantly elevated mortality, especially hip fractures (5, 6). Therefore, the importance of exploring factors associated with osteoporosis should be emphasized.
Both genetic and nongenetic factors strongly correlate with the development of osteoporosis (7). Diet and nutrients, as nongenetic factors, have attracted more attention due to their impact on osteoporosis (8, 9). Polyunsaturated fatty acids (PUFAs) consist of two subtypes, n-3 and n-6, and are essential fatty acids acquired mainly through fish and vegetable oils. After consumption, PUFAs can be transformed into a sequence of long-chain derivatives in the human body. Alpha-linolenic acid (ALA) can be metabolized into eicosapentaenoic acid (EPA) and docosahexaenoic acid (DHA), all of which are crucial components of n-3 PUFAs. Linoleic acid (LA) can be converted into arachidonic acid (AA), both of which are fundamental constituents of n-6 PUFAs (10, 11). The role of n-6 PUFAs and their metabolites including prostaglandins, leukotrienes, and thromboxanes has been linked to various physiological processes such as inflammation generation, and platelet activation, while the n-3 PUFAs have been proven to trigger opposing physiological effects (12, 13). Previous studies have shown that PUFAs are associated with various chronic diseases, including cardiovascular events (14), diabetes (15), depressive disorders (16), osteoarthritis (17, 18), and osteoporosis (19, 20).
Bone mineral density (BMD) scores are widely utilized to evaluate bone mass and diagnose osteoporosis. However, the research evidence for the relationship between dietary PUFAs and BMD remains equivocal. One cohort study found a negative association between dietary PUFA intake and femoral neck BMD in premenopausal women (21). A cross-sectional study demonstrated a positive correlation between dietary intake of PUFAs and total BMD among adults aged 20–59 years (22). While a randomized clinical trial found no correlation between the supplementation with n-3 PUFAs and BMD was observed in kidney transplant recipients (23). These contradictory findings warrant additional investigation. In addition to dietary information, biological samples, such as serum, plasma, or red blood cells (RBCs), can be utilized for PUFA assessment. Currently, only limited research has explored the connection between PUFAs derived from biological samples and BMD (24, 25). Therefore, this research aims to investigate the correlation between serum PUFAs and BMD among adults aged 20-59 years using the National Health and Nutrition Examination Survey (NHANES) 2011-2014.
NHANES is a health project that aims to investigate the health and nutrition status of the US population. The data of 19931 participants from the 2011-2014 cycle was utilized to evaluate the correlation between serum PUFAs and BMD, and to explore differential action of N-3 and N-6 PUFAs on bone. First, we eliminated subjects with ages less than 20 years (n=8602). Second, participants without complete information on serum PUFAs, head BMD, lumbar spine BMD, thoracic spine BMD, trunk BMD, and total BMD were excluded (n=9267). Third, we excluded participants missing information on sample weights (n=39) and covariates (n=44). Finally, we extracted 1979 participants in the study for final analysis. The inclusion and exclusion details of the study participants were shown in Figure 1. The NHANES study received approval from the NCHS Ethics Review Board and all participants provided written informed consent.
Serum specimens were processed, stored, and shipped to the Division of Laboratory Sciences, National Center for Environmental Health, Centers for Disease Control and Prevention, Atlanta, GA for analysis. Fatty acids are detected using electron capture negative-ion mass spectrometry within 34 minutes. Finally, we selected 11 PUFAs for further analysis, including alpha-Linolenic acid (ALA, 18:3n-3), stearidonic acid (SDA, C18:4n-3), eicosapentaenoic acid (EPA, 20:5n-3), docosapentaenoic acid (DPA, 22:5n-3), docosahexaenoic acid (DHA, 22:6n-3), linoleic acid (LA, 18:2n-6), gamma-linolenic acid (GLA, 18:3n-6), eicosadienoic acid (EDA, 20:2n-6), homo-gamma-linolenic acid (HGLA, 20:3n-6), arachidonic acid (AA, 20:4n-6), docosapentaenoic acid (DPA, 22:5n-6).
Dual-energy X-ray absorptiometry (DXA) is a widely used technology for evaluating BMD owing to its rapidity, simplicity, and minimal radiation exposure (26). The DXA examinations based on QDR 4500A fan-beam densitometers (Hologic Inc) were administered by trained and certified radiology technologists and the whole body DXA scans provided the BMD data of the head, lumbar spine, thoracic spine, trunk and total body. Trunk BMD was defined as BMD measurements for trunk bone, including thoracic and lumbar spine, left and right ribs, and pelvis.
Confounding factors potentially associated with BMD were enrolled in this analysis. The demographic data included age, sex, race, and educational level. Body mass index (BMI) was defined as weight(kg) divided by the square of height(m2). Moderate recreational activities were obtained from the questionnaire (in a typical week do you do any moderate-intensity sports, fitness, or recreational activities that cause a small increase in breathing or heart rate such as brisk walking, bicycling, swimming, or volleyball for at least 10 minutes continuously) and we also included smoking data (smoked at least 100 cigarettes in life). Laboratory data included alkaline phosphatase, serum phosphorus, serum calcium, serum bilirubin, uric acid, total cholesterol, triglyceride, glycohemoglobin, and urine albumin creatinine ratio was collected from the fasting blood samples.
The study analysis was performed by using EmpowerStats (http://www.empowerstats.com and R (4.2.3 version) software. Continuous variables were expressed as the mean ± standard deviation and categorical variables were expressed as numbers(n) and percentages (%). The weighted multiple linear regression model was utilized to determine the relationship between serum PUFAs and BMD. No covariates were adjusted in Model 1. Age, gender, and race were adjusted in Model 2. Age, gender, race, educational level, BMI, moderate recreational activities, smoked at least 100 cigarettes in life, alkaline phosphatase, serum phosphorus, serum calcium, serum bilirubin, uric acid, total cholesterol, triglyceride, glycohemoglobin, urine albumin creatinine ratio were adjusted in Model 3. Then, we conducted subgroup analysis by age, gender, and race. P < 0.05 was considered statistically significant. Further, we explored the association between serum PUFAs and BMD by using smoothed curve fitting and a weighted generalized additive model (GAM). The threshold effect of serum DPA on lumbar spine BMD was calculated using two-piece linear regression models.
Of the 1979 study subjects, 1014(52%) were males and 965(48%) were females. Respectively, the average age of males and females was 38.85 ± 11.47 and 39.39 ± 11.69 years old. In terms of educational level, smoked at least 100 cigarettes in life, serum phosphorus, serum calcium, serum bilirubin, uric acid, triglyceride, urine albumin creatinine ratio, GLA, SDA, DPA, DHA, head BMD, thoracic Spine BMD, total BMD, we observed a significantly statistical difference between the two groups. There were no statistical differences in lumbar spine BMD and trunk BMD between the two groups. The weighted, detailed baseline information of the subjects was shown in Table 1.
We performed a weighted multiple linear regression model to investigate the relationship between serum PUFAs and BMD. After all the covariates were adjusted (model 3), we found that serum DPA was positively associated with head BMD (β = 0.0015, 95% Cl: 0.0004,0.0026, P = 0.008296) and lumbar spine BMD (β = 0.0005, 95% Cl: 0.0000,0.0010, P = 0.036093), serum EDA was negatively associated with thoracic spine BMD (β = -0.0008, 95% Cl: -0.0016,-0.0000, P = 0.045355). No relationship was observed between serum PUFAs and trunk BMD and total BMD, result details are shown in Table 2.
Smoothed curve fitting revealed the linear association between serum DPA and head BMD (Figure 2), serum EDA, and thoracic spine BMD (Figure 3). A nonlinear association was found between serum DPA and lumbar BMD (Figure 4). Threshold effect analysis by using a two-piecewise linear regression model indicated the turning point of serum DPA was 81.4umol/L (Table 3). Serum DPA was positively associated with lumbar spine BMD (β = 0.0007, 95% Cl: 0.0001, 0.0012, P = 0.0208) when serum DPA <81.4umol/L. When serum DPA > 81.4umol/L, the relationship was not significant (β = 0.0001, 95% Cl: -0.0008, 0.0010, P = 0.8507).
Figure 2 The association between serum DPA and head BMD. (A) each black point represents a sample. (B) the solid black line represents the smooth curve fit between variables. Dotted line bands represent the 95% confidence interval of the fit. Age, gender, race, educational level, BMI, moderate recreational activities, smoked at least 100 cigarettes in life, alkaline phosphatase, serum phosphorus, serum calcium, serum bilirubin, uric acid, total cholesterol, triglyceride, glycohemoglobin, urine albumin creatinine ratio were adjusted.
Figure 3 The association between serum EDA and thoracic spine BMD. (A) each black point represents a sample. (B) the solid black line represents the smooth curve fit between variables. Dotted line bands represent the 95% confidence interval of the fit. Age, gender, race, educational level, BMI, moderate recreational activities, smoked at least 100 cigarettes in life, alkaline phosphatase, serum phosphorus, serum calcium, serum bilirubin, uric acid, total cholesterol, triglyceride, glycohemoglobin, urine albumin creatinine ratio were adjusted.
Figure 4 The association between serum DPA and lumbar spine BMD. (A) each black point represents a sample. (B) the solid black line represents the smooth curve fit between variables. Dotted line bands represent the 95% confidence interval of the fit. Age, gender, race, educational level, BMI, moderate recreational activities, smoked at least 100 cigarettes in life, alkaline phosphatase, serum phosphorus, serum calcium, serum bilirubin, uric acid, total cholesterol, triglyceride, glycohemoglobin, urine albumin creatinine ratio were adjusted.
Table 3 Threshold effect analysis of serum DPA (umol/L) and lumbar spine BMD(g/cm2) using two-piecewise linear regression model.
Furthermore, we conducted a subgroup analysis by age, gender, and race. In terms of serum DPA and head BMD, when stratified by age, a positive association between serum DPA and head BMD was observed in the subgroup aged 50-59 Years (β = 0.0025, 95% Cl: 0.0002,0.0049, P = 0.035249). When stratified by gender, we found that serum DPA was positively associated with head BMD in females (β = 0.0026, 95% Cl: 0.0008,0.0044, P = 0.005005), the result was shown in Table 4. In terms of serum DPA and lumbar spine BMD, we found a positive relationship in females (β = 0.0008, 95% Cl: 0.0001, 0.0015, P = 0.017900) when stratified by gender, the result was shown in Table 5. In terms of serum EDA and thoracic spine BMD, a negative association was found in the subgroup aged 30-39 Years (β = -0.0016, 95% Cl: -0.0031, -0.0001, P = 0.041331), males (β = -0.0012, 95% Cl: -0.0023, -0.0001, P = 0.039364) and other race (β = -0.0021, 95% Cl: -0.0037, -0.0006, P = 0.008059) when stratified by age, gender and race respectively, the result was shown in Table 6.
For the first time, we utilized the NHANES database to evaluate the association between serum PUFAs and BMD. This study demonstrated a nonlinear positive association between serum DPA and lumbar spine BMD, a linear positive relationship between serum DPA and head BMD, and a linear negative correlation between serum EDA and thoracic spine BMD in US adults. Therefore, we speculated that serum n-3 PUFAs were beneficial for BMD, while n-6 PUFAs had the opposite effect.
Recent years have seen accumulating evidence suggesting potential associations between PUFAs and various human diseases (14–16), including those related to bone health (19, 20). Numerous clinical studies have delved into the relationship between dietary PUFAs and bone health. For instance, a cross-sectional study based on NHANES database demonstrated a positive correlation between total dietary intake of PUFAs and total BMD among adults aged 20–59 years (22). A positive association was also discerned between the consumption of PUFAs intake and both total BMD and lumbar spine BMD (27). However, it should be noted that this association appeared to be limited to the specific demographic of older women without hormone therapy which limited the applicability of this conclusion to other populations. required verification. On the contrary, a longitudinal study demonstrated that an increase in dietary intake of PUFAs and monounsaturated fatty acids (MUFAs) was correlated with decreased femoral neck BMD in women aged 45–55 years of age (21). In addition, total dietary PUFAs intake increased the fracture risk in the older age group >65 years, according to Martínez-Ramírez et al. (28). However, a cohort research demonstrated no association between total dietary PUFAs consumption and hip fracture risk (29). These studies presented varied results regarding the effect of total dietary intake of PUFAs on BMD or fracture risk. The inconsistencies could potentially be attributed to specific factors such as age, gender, and the site of BMD measurement in the study population, which highlighted the need for further and comprehensive investigation. In addition, differences in the impact of dietary PUFAs subgroups on bone health should also be given due consideration.
In fact, the mechanisms by which n-3 PUFAs and n-6 PUFAs function within various body tissues, including bones, have been revealed to differ (12, 30). A pivotal factor is the direction of mesenchymal stem cell (MSC) differentiation, which steers either osteogenesis or adipogenesis. The peroxisome proliferator-activated receptor γ (PPARγ) has a crucial role in driving the differentiation of MSC into adipocytes, subsequently inhibiting osteogenesis (31). Existing studies have shown that n-6 PUFAs impede osteogenesis through the upregulation of PPARγ expression and downregulation of Runx2 expression (32), whereas n-3 PUFAs manifest converse effects (33). N-6 PUFAs are also known to trigger RANKL–RANK signaling, leading to osteoclastogenesis and bone loss (32, 34), a process which is suppressed by n-3 PUFAs (35, 36). Furthermore, n-6 PUFAs are found to elevate pro-inflammatory cytokine levels, promoting bone resorption (37, 38). The outcomes of these experimental findings propose that n-3 PUFAs exert beneficial influences on bone health, standing in contrast to the detrimental effects of n-6 PUFAs. Therefore, further assessment of the effects of different PUFA subclasses on human bone health is required.
A cross-sectional study utilizing the NHANES database showed that dietary supplementation with n-3 PUFAs (EPA, DHA, and SDA) was positively associated with lumbar spine BMD among adults >60 years of age (39). Correspondingly, another cross-sectional study reported a beneficial impact of dietary n-3 PUFAs consumption on lumbar spine BMD in postmenopausal women (40). Beyond its impact on lumbar spine BMD, dietary intake of n-3 PUFAs was also found to enhance hip BMD in the female population aged 19-25 years (41). In addition, dietary n-3 PUFAs intake reduced the levels of biological markers of bone resorption, suggesting that n-3 PUFAs reduced bone loss potentially by inhibiting osteoclast activity (42). While the majority of studies, encompassing the aforementioned ones, endorsed the beneficial effects of dietary n-3 PUFAs on bone health, aligning with prior experimental results, there still existed certain studies that reached inconsistent conclusions. Within the context of a randomized clinical trial, no correlation between n-3 PUFAs supplementation and BMD was observed amongst kidney transplant recipients (23). Furthermore, a comprehensive meta-analysis also suggested that supplementation with dietary n-3 PUFAs had no positive impact on BMD (43), a conclusion that deviated from the results of experimental findings. We proposed that two principal factors contributed to this issue. First, the majority of studies have concentrated on establishing an association between dietary PUFAs consumption and BMD or fracture risk. Nonetheless, data on dietary PUFAs was predominantly obtained from food-frequency questionnaires, which may result in an inaccurate assessment of PUFAs intake. Second, dietary intake of PUFAs did not fully align with bioavailable PUFAs, a factor that could be influenced by the digestion and absorption process. Our hypothesis has garnered support from various studies. For instance, one study highlighted that dietary PUFAs intake was not correlated with serum levels of PUFAs (44). Another study demonstrated that the dietary intake of ALA did not impact its plasma levels, with the connection between dietary consumption and plasma concentration only proving significant for LA, AA, EPA, and DHA (45). Therefore, we advocate the use of biological specimens such as serum, plasma, or red blood cells (RBCs) for a more accurate assessment of PUFAs as opposed to relying solely on dietary information.
However, only a limited number of studies have investigated the association between PUFA levels in biological samples and BMD. A cross-sectional study involving 301 Spanish postmenopausal women demonstrated a positive correlation between the plasma concentration of n-3 PUFAs (inclusive of ALA, EPA, and DHA) and BMD in the spine and neck of the femur (24). A significantly positive correlation between the serum concentration of n-3 PUFAs and femur BMD was observed exclusively within the group having a low n-6:n-3 ratio in another study (25), suggesting that high serum concentrations of n-6 PUFAs potentially impeded the bone health-promoting effects of n-3 PUFAs. This study also showed that the serum concentration of ALA, a class of n-3 PUFAs, was negatively correlated with creatinine-corrected urinary deoxypyridinoline, suggesting that n-3 PUFAs may promote BMD by inhibiting bone resorption. Moreover, PUFA levels from the biological samples were also associated with fracture risk. In a cohort study including 1438 participants, there was a decrease in fracture risk with increased plasma levels of n-3 PUFAs and EPA, while n-6 PUFAs and AA manifested the contrary effects (46). A nested case−control study found that elevated levels of total n-3 PUFAs, ALA, and EPA derived from RBCs, along with a high n-6:n-3 ratio, were associated with a decreased risk of hip fracture (47). These studies indicated that high level of n-3 PUFAs from biological samples were beneficial for promoting BMD or mitigated fracture risk, whereas n-6 PUFAs exerted an opposing effect. Although the conclusions drawn from these studies aligned well with the outcomes of prior experimental results, certain limitations, such as the sample size, the diversity in the types of PUFAs assessed, and the limited number of sites for BMD measurement, needed to be duly acknowledged.
It was worth noting that BMD in various regions had varying clinical significance. Low BMD in the spine might be linked to fragility fractures. Moreover, low head BMD could be connected to hearing impairments (48) and malocclusion in adolescents (49). Therefore, to improve the comprehensiveness and reliability of the study, we included 11 serum PUFAs and 5 sites of BMD to investigate their associations using the NHANES large sample data (n=1979). First, our study revealed a nonlinear positive association between serum DPA and lumbar spine BMD, a relationship that remained consistent solely within the female subgroup but was absent in the male subgroup. Moreover, threshold effect analysis indicated that when serum DPA > 81.4umol/L, the positive relationship was no more significant. Regarding the threshold and nonlinear relationship, previous research proposed that excess dietary intake of n-3 PUFAs beyond the threshold level conferred no additional benefits to bone health (23). In a related animal study, increased consumption of DHA, a type of n-3 PUFA, was shown to benefit BMD, bone mineral content, and peak bone mass. However, no additional benefits were not observed in the group with higher intake of DHA (50). Therefore, we postulated that a threshold might exist for the promotion of lumbar spine BMD by some of the serum N-3 PUFAs, such as DPA. However, this hypothesis warranted further validation through additional clinical studies. Second, our investigation discerned a linear and positive relationship between serum DPA and head BMD, a trend that was consistent within the female subgroup, though not apparent in the male counterpart. A meta-analysis demonstrated that n-3 PUFA supplementation had a better favorable impact on BMD in females (43). An animal study reported that female offspring of mice supplemented with n-3 PUFA had better bone health than male offspring (51). These studies suggested that there was a potential sex differences in the promotion of BMD by n-3 PUFAs, which explained why the positive association of DPA with head BMD and lumbar spine BMD was only significant in the female subgroup in this study. In addition, the positive trend between serum DPA and head BMD was significant in the subgroup aged 50-59 years. BMD decreased with age, potentially highlighting the importance of DPA in promoting BMD among participants aged 50-59 years. Regarding the subtle differences in the effects of serum DPA on head BMD and lumbar spine BMD, we speculated that they are related to the different morphology and activity of osteoclasts, osteogenic capacity of bone marrow stromal cells at the different sites (52–54).Third, our research demonstrated that serum EDA levels had a linear and negative correlation with thoracic spine BMD, which remained consistent within the subgroup aged 30-39 years, males and other race. However, we needed further evidence from large clinical studies to support this subgroup association.
This study had some advantages. First, the research data were extracted from the NHANES database, which ensured the accuracy and representativeness of the data. Second, covariates potentially associated with BMD were adjusted to improve the reliability of this study. However, limitations cannot be ignored. First, it is important to note that this study was a cross-sectional study, which precluded drawing causal inferences regarding serum PUFAs and BMD. Second, this study focused on participants 20-59 years of age, and the conclusions cannot be directly extrapolated to older populations or adolescents. Third, the levels of PUFAs from biological samples were more difficult to obtain compared to food frequency questionnaires and had higher economic costs. Fourth, the relationship between serum PUFAs and BMD was weak. Finally, there is a lack of experimental validation in this study.
In the future, we will conduct further studies focusing on the following aspects. First, we will design clinical randomized controlled trials to investigate the causal association between serum PUFAs and BMD. Second, we aim to expand the study to include elderly individuals who are at high risk for osteoporosis and adolescents. Third, we will investigate the relationship between dietary PUFA intake and serum PUFA levels and ascertain the factors that have an impact on this relationship. Fourth, we will experimentally validate the effects of PUFAs on osteogenesis markers, adipogenesis markers, and inflammatory markers (TNF-α, IL-1β, IL-6, and COX-2).
In conclusion, this study demonstrated a linear positive relationship between serum DPA and head BMD, a nonlinear positive association between serum DPA and lumbar spine BMD, and a linear negative correlation between serum EDA and thoracic spine BMD in US adults aged 20-59 years.
Publicly available datasets were analyzed in this study. This data can be found here: https://www.cdc.gov/nchs/nhanes/index.htm.
The studies involving humans were approved by NCHS Research Ethics Review Board (ERB). The studies were conducted in accordance with the local legislation and institutional requirements. The participants provided their written informed consent to participate in this study.
HL: Formal Analysis, Methodology, Writing – original draft, Writing – review & editing. CX: Formal Analysis, Methodology, Writing – original draft, Writing – review & editing. YL: Methodology, Software, Writing – review & editing. JZ: Methodology, Software, Supervision, Writing – review & editing. YH: Methodology, Software, Writing – review & editing. RZ: Methodology, Software, Writing – review & editing. NZ: Supervision, Writing – review & editing. ZZ: Supervision, Writing – review & editing. XL: Funding acquisition, Supervision, Validation, Writing – review & editing.
The author(s) declare financial support was received for the research, authorship, and/or publication of this article. This work was supported by the Program for Youth Innovation in Future Medicine of Chongqing Medical University (Nos. W0086), Chongqing Technical Innovation and Application Development Special Project (CSTB2021TIAD-KPX0060), and the Chongqing Talent Plan for Innovative Leading talents (CQYC20210303373).
We would like to express gratitude to all NHANES participants and staff.
The authors declare that the research was conducted in the absence of any commercial or financial relationships that could be construed as a potential conflict of interest.
All claims expressed in this article are solely those of the authors and do not necessarily represent those of their affiliated organizations, or those of the publisher, the editors and the reviewers. Any product that may be evaluated in this article, or claim that may be made by its manufacturer, is not guaranteed or endorsed by the publisher.
1. Compston JE, McClung MR, Leslie WD. Osteoporosis. Lancet (2019) 393(10169):364–76. doi: 10.1016/S0140-6736(18)32112-3
2. Consensus development conference: diagnosis, prophylaxis, and treatment of osteoporosis. Am J Med (1993) 94(6):646–50. doi: 10.1016/0002-9343(93)90218-E
3. Office of the Surgeon General (US). Bone health and osteoporosis: A report of the surgeon general. Rockville (MD): office of the surgeon general (US) (2004). Available at: http://www.ncbi.nlm.nih.gov/books/NBK45513/.
4. Hernlund E, Svedbom A, Ivergård M, Compston J, Cooper C, Stenmark J, et al. Osteoporosis in the European Union: medical management, epidemiology and economic burden. A report prepared in collaboration with the International Osteoporosis Foundation (IOF) and the European Federation of Pharmaceutical Industry Associations (EFPIA). Arch Osteoporos (2013) 8(1):136. doi: 10.1007/s11657-013-0136-1
5. Zheng XQ, Xu L, Huang J, Zhang CG, Yuan WQ, Sun CG, et al. Incidence and cost of vertebral fracture in urban China: a 5-year population-based cohort study. Int J Surg (2023) 109(7):1910–8. doi: 10.1097/JS9.0000000000000411
6. Clynes MA, Harvey NC, Curtis EM, Fuggle NR, Dennison EM, Cooper C. The epidemiology of osteoporosis. Br Med Bull (2020) 133(1):105–17. doi: 10.1093/bmb/ldaa005
7. Hendrickx G, Boudin E, Van Hul W. A look behind the scenes: the risk and pathogenesis of primary osteoporosis. Nat Rev Rheumatol (2015) 11(8):462–74. doi: 10.1038/nrrheum.2015.48
8. Guo D, Zhao M, Xu W, He H, Li B, Hou T. Dietary interventions for better management of osteoporosis: An overview. Crit Rev Food Sci Nutr (2023) 63(1):125–44. doi: 10.1080/10408398.2021.1944975
9. Muñoz-Garach A, García-Fontana B, Muñoz-Torres M. Nutrients and dietary patterns related to osteoporosis. Nutrients (2020) 12(7):1986. doi: 10.3390/nu12071986
10. Djuricic I, Calder PC. Beneficial outcomes of omega-6 and omega-3 polyunsaturated fatty acids on human health: an update for 2021. Nutrients (2021) 13(7):2421. doi: 10.3390/nu13072421
11. Kapoor B, Kapoor D, Gautam S, Singh R, Bhardwaj S. Dietary polyunsaturated fatty acids (PUFAs): uses and potential health benefits. Curr Nutr Rep (2021) 10(3):232–42. doi: 10.1007/s13668-021-00363-3
12. Schmitz G, Ecker J. The opposing effects of n-3 and n-6 fatty acids. Prog Lipid Res (2008) 47(2):147–55. doi: 10.1016/j.plipres.2007.12.004
13. Harwood JL. Polyunsaturated fatty acids: conversion to lipid mediators, roles in inflammatory diseases and dietary sources. Int J Mol Sci (2023) 24(10):8838. doi: 10.3390/ijms24108838
14. Marklund M, Wu JHY, Imamura F, Del Gobbo LC, Fretts A, de Goede J, et al. Biomarkers of dietary omega-6 fatty acids and incident cardiovascular disease and mortality. Circulation (2019) 139(21):2422–36. doi: 10.1161/CIRCULATIONAHA.118.038908
15. Forouhi NG, Imamura F, Sharp SJ, Koulman A, Schulze MB, Zheng J, et al. Association of Plasma Phospholipid n-3 and n-6 Polyunsaturated Fatty Acids with Type 2 Diabetes: The EPIC-InterAct Case-Cohort Study. PloS Med (2016) 13(7):e1002094. doi: 10.1371/journal.pmed.1002094
16. Chang JPC, Chang SS, Yang HT, Chen HT, Chien YC, Yang B, et al. Omega-3 polyunsaturated fatty acids in cardiovascular diseases comorbid major depressive disorder - Results from a randomized controlled trial. Brain Behav Immun (2020) 85:14–20. doi: 10.1016/j.bbi.2019.03.012
17. Loef M, Ioan-Facsinay A, Mook-Kanamori DO, Willems van Dijk K, de Mutsert R, Kloppenburg M, et al. The association of plasma fatty acids with hand and knee osteoarthritis: the NEO study. Osteoarthritis Cartilage (2020) 28(2):223–30. doi: 10.1016/j.joca.2019.10.002
18. Stonehouse W, Benassi-Evans B, Bednarz J, Vincent AD, Hall S, Hill CL. Krill oil improved osteoarthritic knee pain in adults with mild to moderate knee osteoarthritis: a 6-month multicenter, randomized, double-blind, placebo-controlled trial. Am J Clin Nutr (2022) 116(3):672–85. doi: 10.1093/ajcn/nqac125
19. Longo AB, Ward WE. PUFAs, bone mineral density, and fragility fracture: findings from human studies. Adv Nutr (2016) 7(2):299–312. doi: 10.3945/an.115.009472
20. El-Sayed E, Ibrahim K. Effect of the types of dietary fats and non-dietary oils on bone metabolism. Crit Rev Food Sci Nutr (2017) 57(4):653–8. doi: 10.1080/10408398.2014.914889
21. Macdonald HM, New SA, Golden MHN, Campbell MK, Reid DM. Nutritional associations with bone loss during the menopausal transition: evidence of a beneficial effect of calcium, alcohol, and fruit and vegetable nutrients and of a detrimental effect of fatty acids. Am J Clin Nutr (2004) 79(1):155–65. doi: 10.1093/ajcn/79.1.155
22. Fang ZB, Wang GX, Cai GZ, Zhang PX, Liu DL, Chu SF, et al. Association between fatty acids intake and bone mineral density in adults aged 20-59: NHANES 2011-2018. Front Nutr (2023) 10:1033195. doi: 10.3389/fnut.2023.1033195
23. Jørgensen HS, Eide IA, Jenssen T, Åsberg A, Bollerslev J, Godang K, et al. Marine n-3 polyunsaturated fatty acids and bone mineral density in kidney transplant recipients: A randomized, placebo-controlled trial. Nutrients (2021) 13(7):2361. doi: 10.3390/nu13072361
24. Roncero-Martín R, Aliaga I, Moran JM, Puerto-Parejo LM, Rey-Sánchez P, de la Luz Canal-Macías M, et al. Plasma fatty acids and quantitative ultrasound, DXA and pQCT derived parameters in postmenopausal spanish women. Nutrients (2021) 13(5):1454. doi: 10.3390/nu13051454
25. Feehan O, Magee PJ, Pourshahidi LK, Armstrong DJ, Slevin MM, Allsopp PJ, et al. Associations of long chain polyunsaturated fatty acids with bone mineral density and bone turnover in postmenopausal women. Eur J Nutr (2023) 62(1):95–104. doi: 10.1007/s00394-022-02933-9
26. Baran DT, Faulkner KG, Genant HK, Miller PD, Pacifici R. Diagnosis and management of osteoporosis: guidelines for the utilization of bone densitometry. Calcif Tissue Int (1997) 61(6):433–40. doi: 10.1007/s002239900362
27. Järvinen R, Tuppurainen M, Erkkilä AT, Penttinen P, Kärkkäinen M, Salovaara K, et al. Associations of dietary polyunsaturated fatty acids with bone mineral density in elderly women. Eur J Clin Nutr (2012) 66(4):496–503. doi: 10.1038/ejcn.2011.188
28. Martínez-Ramírez MJ, Palma S, Martínez-González MA, Delgado-Martínez AD, de la Fuente C, Delgado-Rodríguez M. Dietary fat intake and the risk of osteoporotic fractures in the elderly. Eur J Clin Nutr (2007) 61(9):1114–20. doi: 10.1038/sj.ejcn.1602624
29. Virtanen JK, Mozaffarian D, Willett WC, Feskanich D. Dietary intake of polyunsaturated fatty acids and risk of hip fracture in men and women. Osteoporos Int (2012) 23(11):2615–24. doi: 10.1007/s00198-012-1903-3
30. Saini RK, Keum YS. Omega-3 and omega-6 polyunsaturated fatty acids: Dietary sources, metabolism, and significance - A review. Life Sci (2018) 203:255–67. doi: 10.1016/j.lfs.2018.04.049
31. Takada I, Kouzmenko AP, Kato S. Wnt and PPARgamma signaling in osteoblastogenesis and adipogenesis. Nat Rev Rheumatol (2009) 5(8):442–7. doi: 10.1038/nrrheum.2009.137
32. Casado-Díaz A, Santiago-Mora R, Dorado G, Quesada-Gómez JM. The omega-6 arachidonic fatty acid, but not the omega-3 fatty acids, inhibits osteoblastogenesis and induces adipogenesis of human mesenchymal stem cells: potential implication in osteoporosis. Osteoporos Int (2013) 24(5):1647–61. doi: 10.1007/s00198-012-2138-z
33. Nakanishi A, Tsukamoto I. n-3 polyunsaturated fatty acids stimulate osteoclastogenesis through PPARγ-mediated enhancement of c-Fos expression, and suppress osteoclastogenesis through PPARγ-dependent inhibition of NFkB activation. J Nutr Biochem (2015) 26(11):1317–27. doi: 10.1016/j.jnutbio.2015.06.007
34. Wada T, Nakashima T, Hiroshi N, Penninger JM. RANKL-RANK signaling in osteoclastogenesis and bone disease. Trends Mol Med (2006) 12(1):17–25. doi: 10.1016/j.molmed.2005.11.007
35. Wang Z, Wu J, Li L, Wang K, Wu X, Chen H, et al. Eicosapentaenoic acid supplementation modulates the osteoblast/osteoclast balance in inflammatory environments and protects against estrogen deficiency-induced bone loss in mice. Clin Nutr (2023) 42(9):1715–27. doi: 10.1016/j.clnu.2023.07.022
36. Zhan Q, Tian Y, Han L, Wang K, Wang J, Xue C. The opposite effects of Antarctic krill oil and arachidonic acid-rich oil on bone resorption in ovariectomized mice. Food Funct (2020) 11(8):7048–60. doi: 10.1039/D0FO00884B
37. Abshirini M, Ilesanmi-Oyelere BL, Kruger MC. Potential modulatory mechanisms of action by long-chain polyunsaturated fatty acids on bone cell and chondrocyte metabolism. Prog Lipid Res (2021) 83:101113. doi: 10.1016/j.plipres.2021.101113
38. Rozner R, Vernikov J, Griess-Fishheimer S, Travinsky T, Penn S, Schwartz B, et al. The role of omega-3 polyunsaturated fatty acids from different sources in bone development. Nutrients (2020) 12(11):3494. doi: 10.3390/nu12113494
39. Mangano KM, Kerstetter JE, Kenny AM, Insogna KL, Walsh SJ. An investigation of the association between omega 3 FA and bone mineral density among older adults: results from the National Health and Nutrition Examination Survey years 2005–2008. Osteoporos Int (2014) 25(3):1033–41. doi: 10.1007/s00198-013-2501-8
40. Nawata K, Yamauchi M, Takaoka S, Yamaguchi T, Sugimoto T. Association of n-3 polyunsaturated fatty acid intake with bone mineral density in postmenopausal women. Calcif Tissue Int (2013) 93(2):147–54. doi: 10.1007/s00223-013-9743-5
41. Kuroda T, Ohta H, Onoe Y, Tsugawa N, Shiraki M. Intake of omega-3 fatty acids contributes to bone mineral density at the hip in a younger Japanese female population. Osteoporos Int (2017) 28(10):2887–91. doi: 10.1007/s00198-017-4128-7
42. Griel AE, Kris-Etherton PM, Hilpert KF, Zhao G, West SG, Corwin RL. An increase in dietary n-3 fatty acids decreases a marker of bone resorption in humans. Nutr J (2007) 6:2. doi: 10.1186/1475-2891-6-2
43. Gao J, Xie C, Yang J, Tian C, Zhang M, Lu Z, et al. The effects of n-3 PUFA supplementation on bone metabolism markers and body bone mineral density in adults: A systematic review and meta-analysis of RCTs. Nutrients (2023) 15(12):2806. doi: 10.3390/nu15122806
44. Lelli D, Antonelli Incalzi R, Ferrucci L, Bandinelli S, Pedone C. Association between PUFA intake and serum concentration and mortality in older adults: A cohort study. Clin Nutr (2020) 39(2):510–5. doi: 10.1016/j.clnu.2019.02.030
45. Astorg P, Bertrais S, Laporte F, Arnault N, Estaquio C, Galan P, et al. Plasma n-6 and n-3 polyunsaturated fatty acids as biomarkers of their dietary intakes: a cross-sectional study within a cohort of middle-aged French men and women. Eur J Clin Nutr (2008) 62(10):1155–61. doi: 10.1038/sj.ejcn.1602836
46. Harris TB, Song X, Reinders I, Lang TF, Garcia ME, Siggeirsdottir K, et al. Plasma phospholipid fatty acids and fish-oil consumption in relation to osteoporotic fracture risk in older adults: the Age, Gene/Environment Susceptibility Study. Am J Clin Nutr (2015) 101(5):947–55. doi: 10.3945/ajcn.114.087502
47. Orchard TS, Ing SW, Lu B, Belury MA, Johnson K, Wactawski-Wende J, et al. The association of red blood cell n-3 and n-6 fatty acids with bone mineral density and hip fracture risk in the women’s health initiative. J Bone Miner Res (2013) 28(3):505–15. doi: 10.1002/jbmr.1772
48. Mendy A, Vieira ER, Albatineh AN, Nnadi AK, Lowry D, Gasana J. Low bone mineral density is associated with balance and hearing impairments. Ann Epidemiol (2014) 24(1):58–62. doi: 10.1016/j.annepidem.2013.10.012
49. Konstantynowicz J, Sierpinska T, Kaczmarski M, Piotrowska-Jastrzebska J, Golebiewska M. Dental malocclusion is associated with reduced systemic bone mineral density in adolescents. J Clin Densitom (2007) 10(2):147–52. doi: 10.1016/j.jocd.2007.02.002
50. Farahnak Z, Freundorfer MT, Lavery P, Weiler HA. Dietary docosahexaenoic acid contributes to increased bone mineral accretion and strength in young female Sprague-Dawley rats. Prostaglandins Leukot Essent Fatty Acids (2019) 144:32–9. doi: 10.1016/j.plefa.2019.04.005
51. Vamadeva SG, Patel K, Ravi Mangu S, Ellur G, Sukhdeo SV, Sharan K. Maternal omega-3 LC-PUFA supplementation programs an improved bone mass in the offspring with a more pronounced effect in females than males at adulthood. J Nutr Biochem (2023) 113:109245. doi: 10.1016/j.jnutbio.2022.109245
52. Akintoye SO, Lam T, Shi S, Brahim J, Collins MT, Robey PG. Skeletal site-specific characterization of orofacial and iliac crest human bone marrow stromal cells in same individuals. Bone (2006) 38(6):758–68. doi: 10.1016/j.bone.2005.10.027
53. Goldberg S, Grynpas MD, Glogauer M. Heterogeneity of osteoclast activity and bone turnover in different skeletal sites. Arch Oral Biol (2016) 71:134–43. doi: 10.1016/j.archoralbio.2016.06.026
Keywords: polyunsaturated fatty acids, docosapentaenoic acid (DPA), eicosadienoic acid (EDA), bone mineral density, NHANES
Citation: Liang H, Xiong C, Luo Y, Zhang J, Huang Y, Zhao R, Zhou N, Zhao Z and Luo X (2023) Association between serum polyunsaturated fatty acids and bone mineral density in US adults: NHANES 2011-2014. Front. Endocrinol. 14:1266329. doi: 10.3389/fendo.2023.1266329
Received: 24 July 2023; Accepted: 25 October 2023;
Published: 16 November 2023.
Edited by:
Alberto Falchetti, Italian Auxological Institute (IRCCS), ItalyReviewed by:
Siresha Bathina, Baylor College of Medicine, United StatesCopyright © 2023 Liang, Xiong, Luo, Zhang, Huang, Zhao, Zhou, Zhao and Luo. This is an open-access article distributed under the terms of the Creative Commons Attribution License (CC BY). The use, distribution or reproduction in other forums is permitted, provided the original author(s) and the copyright owner(s) are credited and that the original publication in this journal is cited, in accordance with accepted academic practice. No use, distribution or reproduction is permitted which does not comply with these terms.
*Correspondence: Xiaoji Luo, Y3kyOTgyQDE2My5jb20=
†These authors have contributed equally to this work and share first authorship
Disclaimer: All claims expressed in this article are solely those of the authors and do not necessarily represent those of their affiliated organizations, or those of the publisher, the editors and the reviewers. Any product that may be evaluated in this article or claim that may be made by its manufacturer is not guaranteed or endorsed by the publisher.
Research integrity at Frontiers
Learn more about the work of our research integrity team to safeguard the quality of each article we publish.