- Department of Minimally Invasive Gynecology, Beijing Obstetrics and Gynecology Hospital, Capital Medical University, Beijing Maternal and Child Health Care Hospital, Beijing, China
In the past decade, research on ultrasound therapy in obstetrics and gynecology has rapidly developed. Currently, high-intensity ultrasound has been widely used in clinical practice, while low-intensity ultrasound has gradually emerged as a new trend of transitioning from pre-clinical research to clinical applications. Low-intensity pulsed ultrasound (LIPUS), characterized by a non-invasive low-intensity pulse wave stimulation method, employs its non-thermal effects to achieve safe, economical, and convenient therapeutic outcomes. LIPUS converts into biochemical signals within cells through pathways such as cavitation, acoustic flow, and mechanical stimulation, regulating molecular biological mechanisms and exerting various biological effects. The molecular biology mechanisms underlying the application of LIPUS in obstetrics and gynecology mainly include signaling pathways, key gene expression, angiogenesis, inflammation inhibition, and stem cell differentiation. LIPUS plays a positive role in promoting soft tissue regeneration, bone regeneration, nerve regulation, and changes in cell membrane permeability. LIPUS can improve the treatment benefit of premature ovarian failure, pelvic floor dysfunction, nerve damage caused by intrauterine growth restriction, ovariectomized osteoporosis, and incomplete uterine involution through the above biological effects, and it also has application value in the adjuvant treatment of malignant tumors such as ovarian cancer and cervical cancer. This study outlines the biological mechanisms and applications of LIPUS in treating various obstetric and gynecologic diseases, aiming to promote its precise application and provide a theoretical basis for its use in the field.
1 Introduction
Over recent decades, ultrasound has evolved from a diagnostic imaging modality to therapeutic applications, encompassing both high-intensity and low-intensity ultrasound (1). High-intensity focused ultrasound (HIFU) has gained widespread use in obstetrics and gynecology for treating various benign and malignant tumors, particularly uterine fibroids and adenomyosis. HIFU induces coagulation necrosis of target tissues without damaging the surrounding normal tissues in producing instantaneous high thermal effects and mechanical effects (2). In contrast, low-intensity pulsed ultrasound (LIPUS) affects cellular material metabolism processes, accelerates tissue metabolism, improves ischemia and hypoxia states, enhances tissue nutrition, and promotes tissue repair through minimal thermal effects and significant non-thermal effects in the target tissues (3). The non-thermal effects of LIPUS predominantly involve cavitation-induced micro-bubbles and microjets, acoustic flow, and mechanical stimulation that convert into biochemical signals within cells to produce biological effects (4). Tissue absorption of ultrasonic energy is essential for exerting biological effects, with different tissues exhibiting diverse absorption capacities for ultrasonic waves. Highly protein-rich and low-water content tissues absorb ultrasonic energy to a greater extent, with bone and cartilage exhibiting the highest energy absorption, followed by tendon, skin, muscle, nerve, fat, and blood (5).
LIPUS is a type of medium-frequency ultrasound (0.7-3 MHz) that is pulsed in wave mode (100 and 1,000 Hz) and delivered at an intensity (<3 W/cm2) much lower than traditional ultrasound energy (6). Most piezoelectric transducers on the market are made of ceramic materials that can convert input electrical energy into mechanical energy (ultrasound waves). When the ultrasound beam is emitted from the therapeutic ultrasound device’s treatment head, the energy distribution in space within the beam is non-uniform (7). The ultrasound beam closest to the treatment head is called the near field, where the ultrasound energy is higher and varies significantly locally, making it more commonly used in LIPUS therapy applications. The length of the near field is influenced by the transducer radius, speed of sound in the medium, and frequency, which allows for changes in the size and shape of ultrasound transducers to meet the treatment needs of different parts (8). LIPUS is a safe, economical and convenient treatment modality that has been demonstrated to effectively promote healing of surgical incisions, fractures, tendon injuries, and nerve damage (4). The above applications reflect the LIPUS repair of nerves, blood vessels, muscles and other soft tissues and the control of inflammation, which is closely related to many gynecological diseases such as ovarian function decline, pelvic floor dysfunction, and incomplete uterine involution after delivery. In recent years, with the deepening of research on the effects of ultrasound in biology, LIPUS has gradually received widespread attention in the field of obstetrics and gynecology, indicating a broad application prospect (Figure 1). Currently, there is no systematic review summarizing the research progress of LIPUS in the field of obstetrics and gynecology. Thus, this study systematically summarizes the application direction and research advancements of LIPUS in obstetrics and gynecology to promote further LIPUS-related research in these fields.
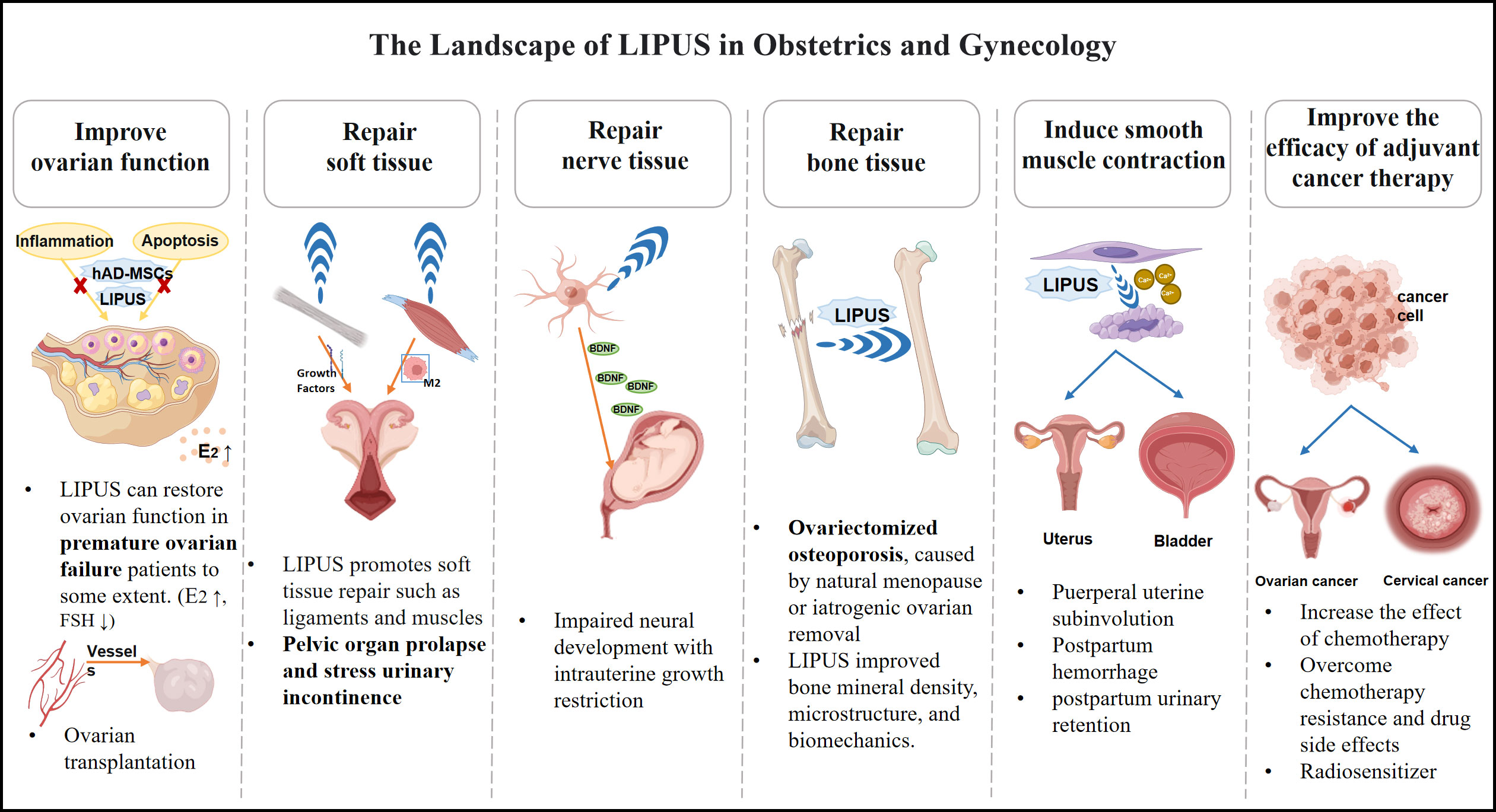
Figure 1 The overview of LIPUS playing a therapeutic role in the field of obstetrics and gynecology.
2 LIPUS improves ovarian function
As a kind of mechanical sound wave, ultrasound penetrates the tissue directly to reach the treatment site, which can be used to improve the pelvic microcirculation, promote tissue nutrition, and accelerate the repair and regeneration of ovarian tissue. As early as in the 1970s, Terhaar et al. (9) found that a small dose of ultrasound could promote the formation of ovarian follicles in mice. However, this finding was not further studied. Until the rapid development of ultrasound therapy in recent years, the impact of ultrasound therapy on ovarian function has come back to the research field.
2.1 LIPUS improves the condition of premature ovarian failure
Premature ovarian failure (POF) is the onset of ovarian failure due to ovarian follicle depletion or iatrogenic injury before the age of 40 years. The main causes include genetic factors, autoimmune diseases and iatrogenic injury, etc., and the pathological characteristics are hypomenorrhea or amenorrhea, increased gonadotropin levels (FSH > 25 IU/L) and decreased estradiol (10). In 2021, a team reported that LIPUS was beneficial to the treatment of cyclophosphamide induced ovarian injury in rats. The results showed that the ultrasound treatment group had fewer atretic follicles, reduced gonadotropin levels, and increased estrogen levels, suggesting that LIPUS could restore ovarian function in POF patients to some extent (11). In the above study, LIPUS had no significant effect on serum anti-Mullerian hormone (AMH) levels and pregnancy rate, but in another model of 4- vinylcyclohexene diepoxide (VCD) -induced ovarian injury in rats, AMH levels were significantly increased after LIPUS treatment (12), this result may be affected by factors such as observation time and sample size. Notably, inflammation and apoptosis play an important role in normal reproduction, and inhibition of inflammatory and apoptotic pathways can alleviate ovarian damage (13). Interestingly, LIPUS reversed the apoptosis and inflammatory state of VCD-induced ovarian injury, resulting in corresponding changes at the cellular structural level, such as the reduction of ovarian cell structural damage, autophagosome occurrence, and endoplasmic reticulum expansion (12).
In addition, mesenchymal stem cell (MSC) transplantation is an effective treatment for POF and has been shown to restore ovarian structure and function in animal models (14). Human amnion-derived mesenchymal stem cells (hAD-MSCs) have been shown to possess the characteristics of MSC (15). The paracrine proteome of hAD-MSCs in the ovarian microenvironment can protect the ovary from iatrogenic injury by reducing apoptosis and promoting angiogenesis, cell proliferation and gene expression (16). LIPUS has been verified to promote the survival and proliferation of hAD-MSCs, and increase the ratio of the anti-apoptotic protein Bcl-2 to pro-apoptotic protein Bax, showing the benefit of the anti-apoptotic effect (17, 18). LIPUS combined with hAD-MSCs has been studied in the treatment of POF. Compared with hAD-MSC transplantation alone, the secretion of stem cell-related factors in the LIPUS pretreatment group increased, which was more conducive to reducing the inflammatory response of ovarian tissue in rats with POF and improving the local microenvironment (18). At the same time, LIPUS can promote the expression of chemokine receptor CXCR 4 in hAD-MSC, further increasing the number of migration and homing of hAD-MSC to the ovary (19). These findings provide evidence that LIPUS combined with stem cell therapy is effective in improving ovarian function in patients with POF.
2.2 LIPUS benefits the outcome of ovarian transplantation
One of the important challenges faced by ovarian transplantation is ischemia-reperfusion injury, which may destroy 30% to 70% of primordial follicles (20). Therefore, the rapid establishment of vascular connections is one of the important factors for successful transplantation and is crucial for follicle survival (21). LIPUS can accelerate angiogenesis and promote tissue repair and enhance regeneration. Previous studies have demonstrated that LIPUS treatment can promote angiogenesis, significantly improve capillary density and myocardial blood flow at the ischemic site, and reduce the degree of myocardial fibrosis in the porcine ischemic cardiomyopathy model (22, 23). In a study of capsule tissues in rabbits, tissue perfusion, hemoglobin content, and vascular density were significantly higher in the LIPUS treatment group, which promoted angiogenesis by stimulating the release of VEGF-α and bFGF, two essential growth factors involved in angiogenesis, from endothelial cells (24). In addition, LIPUS may lead to enhanced angiogenesis by increasing the expression of endothelial nitric oxide synthase, phosphorylated ERK, phosphorylated Akt and phosphorylated YAP protein (23, 25).
From Iran’s biomedical research team, through the animal experiment research LIPUS on ectopic mice ovarian follicle development, angiogenesis and apoptosis after transplantation. The results showed that there was a higher expression of CD31 vascular endothelial growth factor in the LIPUS group, indicating a greater number of blood vessels generated. Ultrasound therapy could effectively accelerate blood vessel formation and reduce ischemic injury (especially within the first 48 hours after transplantation), thus better maintaining the function of transplanted ovaries and reducing ovarian cell apoptosis (20). Therapeutic LIPUS can accelerate and increase angiogenesis, improve inflammation and enhance healing after ovarian tissue transplant stress, and help promote follicular growth in the transplanted ovary. The benefits of LIPUS on ovarian function have been preliminaries confirmed, but most of the current research is still in the stage of animal experiments, so further clinical trails need to make its real applicatiaon in clinical practice.
3 LIPUS promotes the repair of multiple soft tissues
Soft tissue refers to non-mineralized tissues or organs in living organisms and can be divided into two main categories: connective tissue and non-connective tissue. Tendons, skin, fat, and ligaments belong to connective tissues, while blood vessels, nerves, and muscles belong to non-connective tissues (26). Micro-energy therapy can regulate the polarization of macrophages, reduce inflammation and promote tissue repair by reducing the secretion of inflammatory factors such as IL-1β, IL-6, and IL-8 (27, 28). It can also play a key role in the repair of a variety of diseases caused by soft tissue injury by regulating the expression of growth factors VEGF-α, bFGF and TGF-β (24, 29).
Pelvic organ prolapse (POP) is the displacement of pelvic organs caused by weakened pelvic support, which includes muscles, fascia, and ligaments. The main causes of POP are injury due to pregnancy and childbirth, as well as structural atrophy resulting from decreased estrogen levels after menopause. LIPUS treatment is beneficial to the recovery of damaged muscle function, which has been indirectly proved by the results of multiple comprehensive functional tests, and its therapeutic effect has also been directly confirmed by muscle mechanical tests. Both clinical trials and preclinical studies have shown that LIPUS can reduce inflammatory response and accelerate muscle injury recovery, and this beneficial change may be related to increased blood flow, activated mitochondrial biogenesis, and anti-oxidative stress effects (30). Qin et al. (30) found that LIPUS with an intensity of 60 mW/cm2 can promote muscle regeneration significantly by exerting an anti-inflammatory effect through inducing macrophage phenotypic switching from M1 to M2, possibly achieved by upregulating FZD5 expression in the WNT pathway and enhancing nuclear translocation of β-catenin in macrophages. In addition, muscle atrophy is often accompanied by muscle tissue protein metabolism disorders, increased muscle protein breakdown, muscle fiber thinning or even disappearance, and ultrasound therapy can promote protein synthesis in the tissue (31), the key mechanism may be related to promoting protein synthesis and stabilizing alanine, aspartic acid and glutamate metabolism through the MSTN/Akt/mTOR signaling pathway (32). Animal models have also demonstrated that LIPUS treatment can accelerate ligament repair by significantly increasing tissue collagen content (33). Meanwhile, POP not only has the change of pelvic floor support structure, but also includes the damage of pelvic floor nerves and blood vessels. LIPUS stimulation can repair a variety of soft tissue injuries, suggesting that it has a certain therapeutic effect on POP.
Stress urinary incontinence (SUI) is a common concomitant symptom of uterine prolapse, which is one of the manifestations of female pelvic floor dysfunction. At present, there is a lack of effective evidence to confirm the long-term efficacy of drug treatment, and surgery cannot restore the underlying pathological changes of the disease. To demonstrate that ultrasound therapy is a potential treatment for SUI, Yang et al. (34) established a rat model of SUI and treated it with different LIPUS parameters in 2019. The results showed that LIPUS with appropriate parameters could improve the clinical symptoms of SUI. Compared with the SUI non-treatment group, the leakage point pressure and bladder capacity of the 200 mW/cm2 and 300 mW/cm2 groups were restored, and the proliferation and myoblast differentiation of rat skeletal muscle satellite cells were obvious. LIPUS-stimulated regeneration can increase the thickness and integrity of the urethral striated muscle and may play a positive role in relieving the symptoms of SUI.
4 LIPUS promotes the repair of nerve damage
LIPUS is a non-interventional stimulation method characterized by low-intensity pulse waves, which can promote the regeneration of nerve damage. LIPUS can repair peripheral nerve damage and exert neuroprotective effects by affecting nerve tissue engineering seed cells, neurotrophic factors, and nerve conduits (35, 36). In the brain, LIPUS increases the electrical activity of cortical neurons without causing any damage to the brain tissue. Previous studies have demonstrated neuroprotective effects of LIPUS in animal models of traumatic brain injury (37), cerebral ischemia (38), and vascular dementia (39). The mechanisms of neuroprotective effects of LIPUS are multimodal and include enhanced neurotrophic factor release, anti-inflammation, and anti-apoptosis (40).
Survivors of intrauterine growth restriction (IUGR) are at increased risk of impaired neurodevelopment, which may lead to lifelong motor and cognitive impairment, resulting in neurological deficits that currently have no treatment to cure (41, 42). Interventions that increase brain growth factors such as BDNF have therapeutic effects on IUGR-induced brain injury. Prenatal LIPUS treatment may reduce IUGR-induced brain damage by enhancing BDNF/CaMKII/Akt signaling in the offspring brain and increasing BDNF and GLUT 1 expression in the placenta (43). At the same time, maternal LIPUS treatment in IUGR rat model can also increase maternal weight, fetal weight and placental weight, and improve the placental function and fetal growth of IUGR rat model. These data provide a new evidence that LIPUS stimulation can be used as a neuroprotective treatment for IUGR and also has bene fits for IUGR-induced malnrtrition, which is a new technology worthy of attention in perinatal medicine.
5 LIPUS promotes the repair of bone tissue
LIPUS is effective in promoting fracture healing and treating osteoporosis, although its mechanism remains unclear. Studies have shown that LIPUS can significantly improve the mechanical microenvironment of bone trabeculae and osteoblasts through mechanical stimulation, promoting bone tissue repair (44). The combination of LIPUS with cell therapy based on hydrogels has a better promoting effect on osteoblasts and has been approved by the FDA as a method for fracture repair (45). Therefore, it is reasonable to speculate that LIPUS may have therapeutic potential for osteoporosis caused by natural menopause or ovarian function loss due to medical reasons. LIPUS with an intensity of 30 mW/cm2 was sufficient to promote bone defect healing in an ovariectomized rat model of osteoporosis. After 6 weeks of intervention, 150 mW/cm2 LIPUS showed improvement in bone mineral density, microstructure, and biomechanics compared with 30 mW/cm2, indicating that higher intensity LIPUS is more beneficial for osteoporotic bone repair (46). MSTN is a TGF-β family member that acts as a negative regulator of skeletal muscle growth. MSTN deficiency also has a positive effect on bone formation. Experiments have confirmed that LIPUS can effectively reduce the MSTN content in serum and quadriceps muscle of ovariectomized rats, which suggests that LIPUS may improve osteoporosis and promote bone defect healing in ovariectomized rats by inhibiting the MSTN signaling pathway (47). These evidences suggest that LIPUS can benefit women with ovariectomized osteoporosis, but further safe and reliable clinical trials need to be designed.
6 LIPUS induces smooth muscle contraction
Ultrasonic has mechanical effect, thermal effect and physical and chemical effect, biological effect is complex. LIPUS activates calcium (Ca2+) -dependent transcription factors cAMP-responsive element-binding protein and nuclear factor of activated T cells, which significantly increasing Ca2+ influx and increasing intracellular Ca2+ levels (48), which provides a theoretical basis for LIPUS-induced smooth muscle contraction.
Terhaar et al. (9) first found that ultrasound (3 MHz,2 W/cm2) can cause uterine contractions in pregnant mice, and can increase the frequency and amplitude of spontaneous contractions of the uterus. LIPUS induction of uterine smooth muscle contraction may be utilized to promote uterine involution and prevent postpartum hemorrhage. The results of a multicenter, randomized, controlled clinical trial showed that the postpartum women in the LIPUS group were superior to the control group in reducing fundus uteri height, shortening lochia duration, and relieving postpartum pain, with significant differences between the two groups, and no treatment-related adverse reactions and adverse events were observed (49). Therefore, LIPUS is a safe and effective method for treating postpartum uterine involution. Possible reasons why LIPUS can promote postpartum uterine involution include: (1) The mechanical effect of ultrasound can produce mechanical stimulation, and the uterine smooth muscle tissue is very sensitive to a certain frequency of mechanical stimulation and can produce contraction; (2) LIPUS slightly increases the tissue temperature, which may affect the metabolic activity of cells by increasing the enzyme activity; (3) LIPUS changes the permeability of the cell membrane to Ca2+, thereby promoting the increase of intracellular Ca2+ level and initiating the excitation-contraction coupling process of smooth muscle.
LIPUS can also accelerate bladder smooth muscle contraction by opening L-type calcium channels and activating Ca2+ signaling pathways. A retrospective study analyzed the records of 136 patients with postpartum urinary retention (PUR) in two different treatment groups receiving LIPUS and neostigmine, and the results showed that LIPUS had a higher response rate to PUR (80.6% vs. 64.1%, p<0.05), and no early or late adverse events were observed. In addition, The use of nimodipine (Ca2+ inhibitor) demonstrated that ultrasound promoted bladder smooth muscle contraction through activation of Ca2+ signaling pathways and increased troponin expression (50). Therefore, LIPUS is a safe and effective method for the treatment of PUR by inducing the contraction of bladder smooth muscle.
7 LIPUS improves the efficacy of cancer chemotherapy and radiotherapy
Ultrasound is a kind of non-ionizing mechanical wave, which has fewer adverse effects than traditional medical or surgical treatment. On the one hand, the cavitation effect of LIPUS within the tumor causes the rapid generation and rupture of micro-bubbles, generating mechanical shock waves, free radicals, and apoptotic initiators that directly inhibit cancer cell growth (51). The exact reason why LIPUS suppresses cancer cell proliferation may be due to pathways such as promoting cell apoptosis, necrosis, lysis, or disrupting the cell cycle (52, 53). On the other hand, current research focuses more on the combined use of LIPUS and other adjuvant therapies, which can play an important role in cancer treatment by enhancing the ability of anti-tumor drugs. Compared with normal cells, malignant cells are more sensitive to ultrasound irradiation due to their unique cell membrane properties. Therefore, ultrasound can selectively modify the membrane of diseased cells. The microjets produced by cavitation make the cancer cell membrane unstable, thereby increasing the uptake of drugs by cells, enhancing the effect of chemotherapy and targeted therapy, and minimizing the toxicity of drugs to nearby healthy cells (54). LIPUS plays an increasingly clear role in the treatment of cancer, and has also attracted great attention in gynecological cancer. Existing studies mainly focus on ovarian cancer and cervical cancer.
Currently, the main adjuvant treatment for ovarian cancer is chemotherapy, using paclitaxel combined with platinum drugs is the first-line regimen. Traditional cancer chemotherapy has limitations such as drug resistance and drug side effects. The effectiveness of LIPUS combined with platinum to overcome chemoresistance has been demonstrated in platinum-resistant ovarian cancer cells by measuring cell viability, colony formation, and cell cycle analysis (55). The principle may be that LIPUS improves cell membrane permeability and intracellular drug concentration increases the therapeutic effect. Recent studies in a 3D model of cisplatin resistance in ovarian cancer have shown that nanoparticles can further increase the therapeutic effect of ultrasound against resistance (56). The mechanism of action of paclitaxel is mainly to target microtubules, so as to inhibit cell mitosis and play an anti-cancer role. Amaya et al. (57) found that ultrasonic shock wave treatment temporarily disrupted the microtubule cytoskeleton and abolished taxol-induced rigid microtubule bundles. Based on the fact that transient exposure to LIPUS can reduce and/or eliminate the cytotoxicity associated with paclitaxel treatment of ovarian cancer cells, a strategy can be developed to combat the side effects of taxol-based chemotherapy in cancer patients. Local use of LIPUS only at the desired site to eliminate cytotoxicity without affecting the effect of paclitaxel on cancer cell activity is expected to be used to prevent chemotreatment-induced alopecism and peripheral neuropathy and improve the quality of life of patients (58). In addition, the above purpose can also be achieved with the help of molecular materials. Phase-changeable, folate-targeted perfluoropentane nanodroplets loaded with 10- hydroxycamptothecin and superparamagnetic Fe3O4 have been fabricated for multi-modality cancer imaging and targeted therapy. LIPUS-activated nanodroplets can improve the therapeutic effect on cancer cells and relatively reduce the side effects on normal tissues (59).
LIPUS can potentially play a role in radiotherapy and chemotherapy of cervical cancer. Transient mechanical effects mediated by acoustic pores generated by LIPUS enhance membrane permeability and disband the cytoskeleton early after acoustic pores. Cervical cancer HeLa cells were arrested in different cycle stages, and changes in membrane permeability and cytoskeletal arrangement induced by acoustic pore technology were simultaneously analyzed using real-time fluorescence imaging systems. The research showed that S-phase may be considered as the optimal cell cycle for transient acoustic perforation to promote gene/drug delivery therapy (60). The combination of S-phase blocking drugs with LIPUS may increase the efficacy of chemotherapy in treating cervical cancer. In addition, LIPUS can also be used as a sensitizer for radiotherapy. In HeLa cell experiments, radiation at a dose of 2 Gy combined with ultrasound treatment at any intensity (0.5, 1.0, 1.5 W/cm2) resulted in a significant decrease in cell survival compared to incubation for 72 h after radiation alone (61). Therefore, it is reasonable to believe that the combination therapy of LIPUS can enhance the effect of radiotherapy for cervical cancer. However, it needs further verification in subsequent animal experiments and clinical trials.
8 Summary and prospect
LIPUS is a promising non-invasive method that can promote various tissue repair, inhibit inflammatory response, and change cell membrane permeability through mechanical, thermal and physical and chemical effects. LIPUS has valuable applications in reproductive medicine, perinatal medicine, postpartum recovery, gynecological cancer and other directions. Although there is still insufficient understanding of the biological and biodynamic effects of LIPUS in human tissues and their effects on organs and the whole body, the therapeutic results obtained so far in the preclinical stage are very promising. These results may trigger a new research boom in the clinical field in the next few years. The future goals include studying the biological effects and molecular mechanisms of LIPUS on tissues and cells, screening treatment parameters suitable for different tissues, organs, and diseases, clarifying the treatment standards for various diseases, and effectively translating animal and cellular level studies into clinical applications.
Author contributions
XJ conceived the study and edited the manuscript. HD, SW and YC edited and reviewed the manuscript. All authors contributed to the article and approved the submitted version.
Funding
This study was funded by the National Key Research and Development Program of China (2018YFC1004803).
Acknowledgments
We are grateful to Beijing Obstetrics and Gynecology Hospital, Capital Medical University for encouraging and supporting this work.
Conflict of interest
The authors declare that the research was conducted in the absence of any commercial or financial relationships that could be construed as a potential conflict of interest.
Publisher’s note
All claims expressed in this article are solely those of the authors and do not necessarily represent those of their affiliated organizations, or those of the publisher, the editors and the reviewers. Any product that may be evaluated in this article, or claim that may be made by its manufacturer, is not guaranteed or endorsed by the publisher.
References
1. Ning G-Z, Song W-Y, Xu H, Zhu R-S, Wu Q-L, Wu Y, et al. Bone marrow mesenchymal stem cells stimulated with low-intensity pulsed ultrasound: better choice of transplantation treatment for spinal cord injury: treatment for sci by lipus-bmscs transplantation. CNS Neurosci Ther (2019) 25(4):496–508. doi: 10.1111/cns.13071
2. Wang S, Li B-H, Wang J-J, Guo Y-S, Cheng J-M, Ye H, et al. The safety of echo contrast-enhanced ultrasound in high-intensity focused ultrasound ablation for abdominal wall endometriosis: A retrospective study. Quant Imaging Med Surg (2021) 11(5):1751–62. doi: 10.21037/qims-20-622
3. Liufu C, Li Y, Lin Y, Yu J, Du M, Chen Y, et al. Synergistic ultrasonic biophysical effect-responsive nanoparticles for enhanced gene delivery to ovarian cancer stem cells. Drug Delivery (2020) 27(1):1018–33. doi: 10.1080/10717544.2020.1785583
4. Jiang X, Savchenko O, Li Y, Qi S, Yang T, Zhang W, et al. A review of low-intensity pulsed ultrasound for therapeutic applications. IEEE Trans BioMed Eng (2019) 66(10):2704–18. doi: 10.1109/TBME.2018.2889669
5. Watson T. Ultrasound in contemporary physiotherapy practice. Ultrasonics (2008) 48(4):321–9. doi: 10.1016/j.ultras.2008.02.004
6. Xin Z, Lin G, Lei H, Lue TF, Guo Y. Clinical applications of low-intensity pulsed ultrasound and its potential role in urology. Transl Androl Urol (2016) 5(2):255–66. doi: 10.21037/tau.2016.02.04
7. Fung C-H, Cheung W-H, Pounder NM, de Ana FJ, Harrison A, Leung K-S. Investigation of rat bone fracture healing using pulsed 1.5 mhz, 30 mw/cm(2) burst ultrasound–axial distance dependency. Ultrasonics (2014) 54(3):850–9. doi: 10.1016/j.ultras.2013.10.013
8. Harrison A, Lin S, Pounder N, Mikuni-Takagaki Y. Mode & Mechanism of low intensity pulsed ultrasound (Lipus) in fracture repair. Ultrasonics (2016) 70:45–52. doi: 10.1016/j.ultras.2016.03.016
9. Ter Haar G, Dyson M, Talbert D. Ultrasonically induced contractions in mouse uterine smooth muscle in vivo. Ultrasonics (1978) 16(6):275–6. doi: 10.1016/0041-624x(78)90055-0
10. Stuenkel CA, Gompel A. Primary ovarian insufficiency. New Engl J Med (2023) 388(2):154–63. doi: 10.1056/NEJMcp2116488
11. Tang H, Liu Y, Fan Y, Li C. Therapeutic effects of low-intensity pulsed ultrasound on premature ovarian insufficiency. Ultrasound Med Biol (2021) 47(8):2377–87. doi: 10.1016/j.ultrasmedbio.2021.04.022
12. Qin J, Chen J, Xu H, Xia Y, Tang W, Wang W, et al. Low-intensity pulsed ultrasound promotes repair of 4-vinylcyclohexene diepoxide-induced premature ovarian insufficiency in sd rats. J Gerontol A Biol Sci Med Sci (2022) 77(2):221–7. doi: 10.1093/gerona/glab242
13. Elkady MA, Shalaby S, Fathi F, El-Mandouh S. Effects of quercetin and rosuvastatin each alone or in combination on cyclophosphamide-induced premature ovarian failure in female albino mice. Hum Exp Toxicol (2019) 38(11):1283–95. doi: 10.1177/0960327119865588
14. Hoang VT, Nguyen H-P, Nguyen VN, Hoang DM, Nguyen T-ST, Nguyen Thanh L. Adipose-derived mesenchymal stem cell therapy for the management of female sexual dysfunction: literature reviews and study design of a clinical trial. Front Cell Dev Biol (2022) 10:956274. doi: 10.3389/fcell.2022.956274
15. Díaz-Prado S, Muiños-López E, Hermida-Gómez T, Rendal-Vázquez ME, Fuentes-Boquete I, de Toro FJ, et al. Multilineage differentiation potential of cells isolated from the human amniotic membrane. J Cell Biochem (2010) 111(4):846–57. doi: 10.1002/jcb.22769
16. Feng X, Ling L, Zhang W, Liu X, Wang Y, Luo Y, et al. Effects of human amnion-derived mesenchymal stem cell (Had-msc) transplantation in situ on primary ovarian insufficiency in sd rats. Reprod Sci (Thousand Oaks Calif) (2020) 27(7):1502–12. doi: 10.1007/s43032-020-00147-0
17. Ling L, Wei T, He L, Wang Y, Wang Y, Feng X, et al. Low-intensity pulsed ultrasound activates erk1/2 and pi3k-akt signalling pathways and promotes the proliferation of human amnion-derived mesenchymal stem cells. Cell Prolif (2017) 50(6):e12383. doi: 10.1111/cpr.12383
18. Ling L, Feng X, Wei T, Wang Y, Wang Y, Zhang W, et al. Effects of low-intensity pulsed ultrasound (Lipus)-pretreated human amnion-derived mesenchymal stem cell (Had-msc) transplantation on primary ovarian insufficiency in rats. Stem Cell Res Ther (2017) 8(1):283. doi: 10.1186/s13287-017-0739-3
19. Ling L, Hou J, Wang Y, Shu H, Huang Y. Effects of low-intensity pulsed ultrasound on the migration and homing of human amnion-derived mesenchymal stem cells to ovaries in rats with premature ovarian insufficiency. Cell Transplant (2022) 31:9636897221129171. doi: 10.1177/09636897221129171
20. Abtahi NS, Eimani H, Vosough A, Shahverdi A, Fathi R, Hayati N, et al. Effect of therapeutic ultrasound on folliculogenesis, angiogenesis and apoptosis after heterotopic mouse ovarian transplantation. Ultrasound Med Biol (2014) 40(7):1535–44. doi: 10.1016/j.ultrasmedbio.2014.02.006
21. Wang D, Geng M, Gan D, Han G, Gao G, Xing A, et al. Effect of resveratrol on mouse ovarian vitrification and transplantation. Reprod Biol Endocrinol RB&E (2021) 19(1):54. doi: 10.1186/s12958-021-00735-y
22. Hanawa K, Ito K, Aizawa K, Shindo T, Nishimiya K, Hasebe Y, et al. Low-intensity pulsed ultrasound induces angiogenesis and ameliorates left ventricular dysfunction in a porcine model of chronic myocardial ischemia. PloS One (2014) 9(8):e104863. doi: 10.1371/journal.pone.0104863
23. Shindo T, Ito K, Ogata T, Hatanaka K, Kurosawa R, Eguchi K, et al. Low-intensity pulsed ultrasound enhances angiogenesis and ameliorates left ventricular dysfunction in a mouse model of acute myocardial infarction. Arterioscler Thromb Vasc Biol (2016) 36(6):1220–9. doi: 10.1161/ATVBAHA.115.306477
24. Yu M, Bian Y, Wang L, Chen F. Low-intensity pulsed ultrasound enhances angiogenesis in rabbit capsule tissue that acts as a novel vascular bed in vivo. Adv Clin Exp Med (2021) 30(6):581–9. doi: 10.17219/acem/134115
25. Xu X-M, Xu T-M, Wei Y-B, Gao X-X, Sun J-C, Wang Y, et al. Low-intensity pulsed ultrasound treatment accelerates angiogenesis by activating yap/taz in human umbilical vein endothelial cells. Ultrasound Med Biol (2018) 44(12):2655–61. doi: 10.1016/j.ultrasmedbio.2018.07.007
26. Chittajallu SNSH, Richhariya A, Tse KM, Chinthapenta V. A review on damage and rupture modelling for soft tissues. Bioeng (Basel) (2022) 9(1):26. doi: 10.3390/bioengineering9010026
27. Xu Z, Li S, Wan L, Hu J, Lu H, Zhang T. Role of low-intensity pulsed ultrasound in regulating macrophage polarization to accelerate tendon-bone interface repair. J Orthop Res (2022) 41(5):919–29. doi: 10.1002/jor.25454
28. Iacoponi F, Cafarelli A, Fontana F, Pratellesi T, Dumont E, Barravecchia I, et al. Optimal low-intensity pulsed ultrasound stimulation for promoting anti-inflammatory effects in macrophages. APL Bioeng (2023) 7(1):016114. doi: 10.1063/5.0137881
29. İnceoğlu A, Şahin F, Akkaya N, Ök N, Yörükoğlu Ç, Mete G, et al. Effects of low-density pulsed ultrasound treatment on transforming growth factor-beta, collagen level, histology, biomechanics, and function in repaired rat tendons. Turk J Phys Med Rehabil (2021) 67(2):167–74. doi: 10.5606/tftrd.2021.5118
30. Qin H, Luo Z, Sun Y, He Z, Qi B, Chen Y, et al. Low-intensity pulsed ultrasound promotes skeletal muscle regeneration via modulating the inflammatory immune microenvironment. Int J Biol Sci (2023) 19(4):1123–45. doi: 10.7150/ijbs.79685
31. Yang X, Li P, Lei J, Feng Y, Tang L, Guo J. Integrated application of low-intensity pulsed ultrasound in diagnosis and treatment of atrophied skeletal muscle induced in tail-suspended rats. Int J Mol Sci (2022) 23(18):10369. doi: 10.3390/ijms231810369
32. Sun L, An S, Zhang Z, Zhou Y, Yu Y, Ma Z, et al. Molecular and metabolic mechanism of low-intensity pulsed ultrasound improving muscle atrophy in hindlimb unloading rats. Int J Mol Sci (2021) 22(22):12112. doi: 10.3390/ijms222212112
33. Lai WC, Iglesias BC, Mark BJ, Wang D. Low-intensity pulsed ultrasound augments tendon, ligament, and bone-soft tissue healing in preclinical animal models: A systematic review. Arthroscopy (2021) 37(7):2318–33.e3. doi: 10.1016/j.arthro.2021.02.019
34. Yang B, Li M, Lei H, Xu Y, Li H, Gao Z, et al. Low intensity pulsed ultrasound influences the myogenic differentiation of muscle satellite cells in a stress urinary incontinence rat model. Urology (2019) 123:297.e1–.e8. doi: 10.1016/j.urology.2018.09.020
35. Liu X, Zou D, Hu Y, He Y, Lu J. Research progress of low-intensity pulsed ultrasound in the repair of peripheral nerve injury. Tissue Eng Part B Rev (2023). doi: 10.1089/ten.TEB.2022.0194
36. Acheta J, Stephens SBZ, Belin S, Poitelon Y. Therapeutic low-intensity ultrasound for peripheral nerve regeneration - a schwann cell perspective. Front Cell Neurosci (2021) 15:812588. doi: 10.3389/fncel.2021.812588
37. Su W-S, Wu C-H, Chen S-F, Yang F-Y. Transcranial ultrasound stimulation promotes brain-derived neurotrophic factor and reduces apoptosis in a mouse model of traumatic brain injury. Brain Stimul (2017) 10(6):1032–41. doi: 10.1016/j.brs.2017.09.003
38. Chen C-M, Wu C-T, Yang T-H, Liu S-H, Yang F-Y. Preventive effect of low intensity pulsed ultrasound against experimental cerebral ischemia/reperfusion injury via apoptosis reduction and brain-derived neurotrophic factor induction. Sci Rep (2018) 8(1):5568. doi: 10.1038/s41598-018-23929-8
39. Huang S-L, Chang C-W, Lee Y-H, Yang F-Y. Protective effect of low-intensity pulsed ultrasound on memory impairment and brain damage in a rat model of vascular dementia. Radiology (2017) 282(1):113–22. doi: 10.1148/radiol.2016160095
40. Sung C-Y, Chiang P-K, Tsai C-W, Yang F-Y. Low-intensity pulsed ultrasound enhances neurotrophic factors and alleviates neuroinflammation in a rat model of Parkinson's disease. Cereb Cortex (2021) 32(1):176–85. doi: 10.1093/cercor/bhab201
41. Aisa MC, Barbati A, Cappuccini B, De Rosa F, Gerli S, Clerici G, et al. Urinary Nerve Growth Factor in Full-Term, Preterm and Intra Uterine Growth Restriction Neonates: Association with Brain Growth at 30-40 Days of Postnatal Period and with Neuro-Development Outcome at Two Years. A Pilot Study. Neurosci Lett (2021) 741:135459. doi: 10.1016/j.neulet.2020.135459
42. Wixey JA, Chand KK, Pham L, Colditz PB, Bjorkman ST. Therapeutic potential to reduce brain injury in growth restricted newborns. J Physiol (2018) 596(23):5675–86. doi: 10.1113/JP275428
43. Hung T-H, Liu Y-C, Wu C-H, Chen C-C, Chao H, Yang F-Y, et al. Antenatal low-intensity pulsed ultrasound reduces neurobehavioral deficits and brain injury following dexamethasone-induced intrauterine growth restriction. Brain Pathol (Zurich Switzerland) (2021) 31(6):e12968. doi: 10.1111/bpa.12968
44. Tian C, Liu H, Zhao C, Zhang C, Wang W. A numerical study on mechanical effects of low-intensity pulsed ultrasound on trabecular bone and osteoblasts. J Biomech Eng (2023) 145(5):051010. doi: 10.1115/1.4056658
45. Assanah F, Grassie K, Anderson H, Xin X, Rowe D, Khan Y. Ultrasound-derived mechanical stimulation of cell-laden collagen hydrogels for bone repair. J BioMed Mater Res A (2023) 111(8):1200–15. doi: 10.1002/jbm.a.37508
46. Sun S, Tang L, Zhao T, Kang Y, Sun L, Liu C, et al. Longitudinal effects of low-intensity pulsed ultrasound on osteoporosis and osteoporotic bone defect in ovariectomized rats. Ultrasonics (2021) 113:106360. doi: 10.1016/j.ultras.2021.106360
47. Tang L, Kang Y, Sun S, Zhao T, Cao W, Fan X, et al. Inhibition of mstn signal pathway may participate in lipus preventing bone loss in ovariectomized rats. J Bone Miner Metab (2020) 38(1):14–26. doi: 10.1007/s00774-019-01029-5
48. Cheng H-F, Chiu W-T, Lai Y-S, Truong T-T, Lee P-Y, Huang C-C. High-frequency noncontact low-intensity pulsed ultrasound modulates ca2+-dependent transcription factors contributing to cell migration. Ultrasonics (2023) 127:106852. doi: 10.1016/j.ultras.2022.106852
49. Qin Y, Zhao X, Dong X, Liu J, Wang L, Wu X, et al. Low-intensity ultrasound promotes uterine involution after cesarean section: the first multicenter, randomized, controlled clinical trial. Int J Hyperthermia (2022) 39(1):181–9. doi: 10.1080/02656736.2022.2025924
50. Ren Y, Zhu Y, Liu L, Yu T, Dong X. Ultrasound induces contraction of the bladder smooth muscle. Int Urol Nephrol (2016) 48(8):1229–36. doi: 10.1007/s11255-016-1298-8
51. Misík V, Riesz P. Free radical intermediates in sonodynamic therapy. Ann N Y Acad Sci (2000) 899:335–48. doi: 10.1111/j.1749-6632.2000.tb06198.x
52. Katiyar A, Osborn J, DasBanerjee M, Zhang LG, Sarkar K, Sarker KP. Inhibition of human breast cancer cell proliferation by low-intensity ultrasound stimulation. J Ultrasound Med (2020) 39(10):2043–52. doi: 10.1002/jum.15312
53. Sengupta S, Khatua C, Pal A, Bodhak S, Balla VK. Influence of ultrasound and magnetic field treatment time on carcinoma cell inhibition with drug carriers: an in vitro study. Ultrasound Med Biol (2020) 46(10):2752–64. doi: 10.1016/j.ultrasmedbio.2020.06.012
54. Sengupta S, Balla VK. A review on the use of magnetic fields and ultrasound for non-invasive cancer treatment. J Adv Res (2018) 14:97–111. doi: 10.1016/j.jare.2018.06.003
55. Kip B, Aydin O. Overcome of cisplatin drug resistance in ovarian cancer by combination of low-intensity ultrasound and cisplatin. Curr Drug Delivery (2022) 20(9):1380–90. doi: 10.2174/1567201819666220627100857
56. Kip B, Tunc CU, Aydin O. Triple-combination therapy assisted with ultrasound-active gold nanoparticles and ultrasound therapy against 3d cisplatin-resistant ovarian cancer model. Ultrason Sonochem (2022) 82:105903. doi: 10.1016/j.ultsonch.2021.105903
57. Amaya C, Luo S, Baigorri J, Baucells R, Smith ER, Xu X-X. Exposure to low intensity ultrasound removes paclitaxel cytotoxicity in breast and ovarian cancer cells. BMC Cancer (2021) 21(1):981. doi: 10.1186/s12885-021-08722-7
58. Amaya C, Smith ER, Xu X-X. Low intensity ultrasound as an antidote to taxane/paclitaxel-induced cytotoxicity. J Cancer (2022) 13(7):2362–73. doi: 10.7150/jca.71263
59. Liu J, Xu F, Huang J, Xu J, Liu Y, Yao Y, et al. Low-intensity focused ultrasound (Lifu)-activated nanodroplets as a theranostic agent for noninvasive cancer molecular imaging and drug delivery. Biomater Sci (2018) 6(11):2838–49. doi: 10.1039/c8bm00726h
60. Fan P, Zhang Y, Guo X, Cai C, Wang M, Yang D, et al. Cell-cycle-specific cellular responses to sonoporation. Theranostics (2017) 7(19):4894–908. doi: 10.7150/thno.20820
Keywords: LIPUS, tissue repair, biological mechanisms, clinical application, obstetrics and gynecology
Citation: Ji X, Duan H, Wang S and Chang Y (2023) Low-intensity pulsed ultrasound in obstetrics and gynecology: advances in clinical application and research progress. Front. Endocrinol. 14:1233187. doi: 10.3389/fendo.2023.1233187
Received: 01 June 2023; Accepted: 17 July 2023;
Published: 31 July 2023.
Edited by:
Yan Wang, Chongqing Medical University, ChinaReviewed by:
Chengzhi Li, Chongqing Medical University, ChinaYongli Ding, Sichuan Academy of Medical Sciences and Sichuan Provincial People’s Hospital, China
Copyright © 2023 Ji, Duan, Wang and Chang. This is an open-access article distributed under the terms of the Creative Commons Attribution License (CC BY). The use, distribution or reproduction in other forums is permitted, provided the original author(s) and the copyright owner(s) are credited and that the original publication in this journal is cited, in accordance with accepted academic practice. No use, distribution or reproduction is permitted which does not comply with these terms.
*Correspondence: Hua Duan, ZHVhbmh1YUBjY211LmVkdS5jbg==