- 1Department of Cardiovascular Medicine, The Second Xiangya Hospital, Central South University, Changsha, Hunan, China
- 2Research Institute of Blood Lipid and Atherosclerosis, The Second Xiangya Hospital, Central South University, Changsha, Hunan, China
- 3Department of Neurology, The Second Xiangya Hospital, Central South University, Changsha, Hunan, China
- 4Hunan Key Laboratory of Tumor Models and Individualized Medicine, The Second Xiangya Hospital of Central South University, Changsha, Hunan, China
Background: Since the approval of the proprotein convertase subtilisin/kexin type 9 (PCSK9) monoclonal antibodies for marketing in 2015, PCSK9 inhibitors have attracted significant interest in the field of cardiovascular endocrinology. A large number of clinical trials have confirmed the efficacy and safety of PCSK9 inhibitors in reducing cholesterol and the risk of cardiovascular events. No bibliometric analysis of PCSK9 inhibitors has been performed as of yet. This study aims to analyze the research trends and hotspots of PCSK9 inhibitors through bibliometric analysis.
Methods: We searched the Web of Science Core Collection (WoSCC) database for PCSK9 inhibitor-related publications from 2007 to 2022. Data visualization analysis was performed using CiteSpace software. Microsoft Excel and Graphpad software were used for the drawing of some tables and figures.
Results: A total of 1072 pieces of literature were retrieved between 2007 and 2022. The number of publications concerning PCSK9 inhibitors is growing annually. The top five countries with the most articles published were the United States, England, Canada, Italy, and France. Harvard University, Amgen, Brigham & Women’s Hospital, Harvard Medical School, and Imperial College London are the five institutions with the highest output. The Journal of Clinical Lipidology is the most popular journal in this field. The most frequently cited journal is the New England Journal of Medicine. As for authors, Sabatine MS and Giugliano RP from Brigham & Women’s Hospital have the highest number of published articles. Amgen is the funding agency for most of the research. According to keyword analysis, “low density lipoprotein”, “familial hypercholesterolemia”, “PCSK9 inhibitor”, “PCSK9”, and “efficacy” are the five keywords with the highest frequency of co-occurrence.
Conclusion: The past 15 years have witnessed a rapid and fruitful development of PCSK9 inhibitors. The research trend and focus for PCSK9 inhibitors are from the mechanism of reducing low-density lipoprotein cholesterol to related clinical trials. Developed countries such as the United States have contributed prominently in this area. Coronary artery and inflammation are currently at the forefront of research in the field and are in an explosion period.
1 Introduction
Proprotein convertases (PCs) are responsible for cleaving inactive protein precursors to generate bioactive protein products, which will lead to the activation (in most cases) and inactivation of precursor proteins (1–4). Proprotein convertase subtilisin/kexin type 9 (PCSK9), the last member of the PCs family, was first reported in 2003 (5). Seidah et al. (5), using northern blot analysis in different cell lines, found that PCSK9 is mainly expressed in the liver of humans as well as in the small intestine, liver, and pancreatic islets of rodents. PCSK9 consists of three structural domains: the prodomain, the catalytic subunit, and the C-terminal Cys/His-rich domain (CHRD) (6, 7). PCSK9 maps to the short arm of human chromosome 1p32, implicating it as a possible third gene involved in cholesterol metabolism after low-density lipoprotein receptor (LDLR) and apolipoprotein B (ApoB) (5, 8, 9). A large number of studies have subsequently provided evidence for this hypothesis. In gene regulation, PCSK9 is regulated by dietary cholesterol and sterol regulatory element binding proteins 1a (SREBP1a) or SREBP2 (10). Statins upregulate the transcription levels of PCSK9 and LDLR (11). Abifadel et al. (12) first revealed that PCSK9 mutations lead to autosomal dominant hypercholesterolemia. Subsequent mechanism studies demonstrated that PCSK9 induces liver LDLR degradation through the lysosomal pathway (13, 14). Besides, human circulating PCSK9 levels are directly correlated with serum low-density lipoprotein cholesterol (LDLc) levels (15, 16). Heterozygote loss-of-function mutations in human PCSK9 are associated with reduced LDLc levels and are accompanied by a significant reduction in the incidence of acute cardiovascular and cerebrovascular events (17–20). In animal studies, Pcsk9 knockout mice showed a 40-50% decrease in circulating total cholesterol levels, an 80% decrease in LDLc levels, and an increase in hepatic LDLR protein levels (21, 22). In the atherosclerotic mouse model, Pcsk9 overexpression promotes atherosclerosis, whereas deletion is protective (23, 24). The main research on the PCSK9 mouse model in lipid metabolism are summarized in Table 1.
Currently, the most widely used PCSK9 inhibitors in the clinic are PCSK9 monoclonal antibodies (mAbs) (evolocumab and alirocumab), which inhibit the binding of circulating PCSK9 to the LDLR. Alirocumab monotherapy or in combination with other lipid-lowering drugs reduces LDLc levels by 40 - 70%, although statins upregulate PCSK9 expression through activation of SREBP2 (11, 30–32). FOURIER and ODYSSEY are two large randomized controlled studies aimed at evaluating the role of PCSK9 inhibitors in the secondary prevention of atherosclerotic cardiovascular disease (ASCVD) (33, 34). Evolocumab and alirocumab cumulatively reduced the relative risk of major cardiovascular events by 15% in patients at 3 and 4 years of follow-up, respectively (33, 34). Studies have shown that PCSK9 mAb is well suited for normal and heterozygote familial hypercholesterolemia (HeFH) patients due to its ability to prevent LDLR degradation (35). Another marketed PCSK9 inhibitor is the PCSK9 small interfering RNA (siRNA) (inclisiran), which targets the liver and prevents PCSK9 translation (36). Unlike biweekly or monthly injections of PCSK9 mAb, injections of PCSK9 siRNA twice a year still reduced LDLc levels by 50 - 60% (37). A timeline of significant findings on PCSK9 in lipid metabolism is presented in Figure 1.
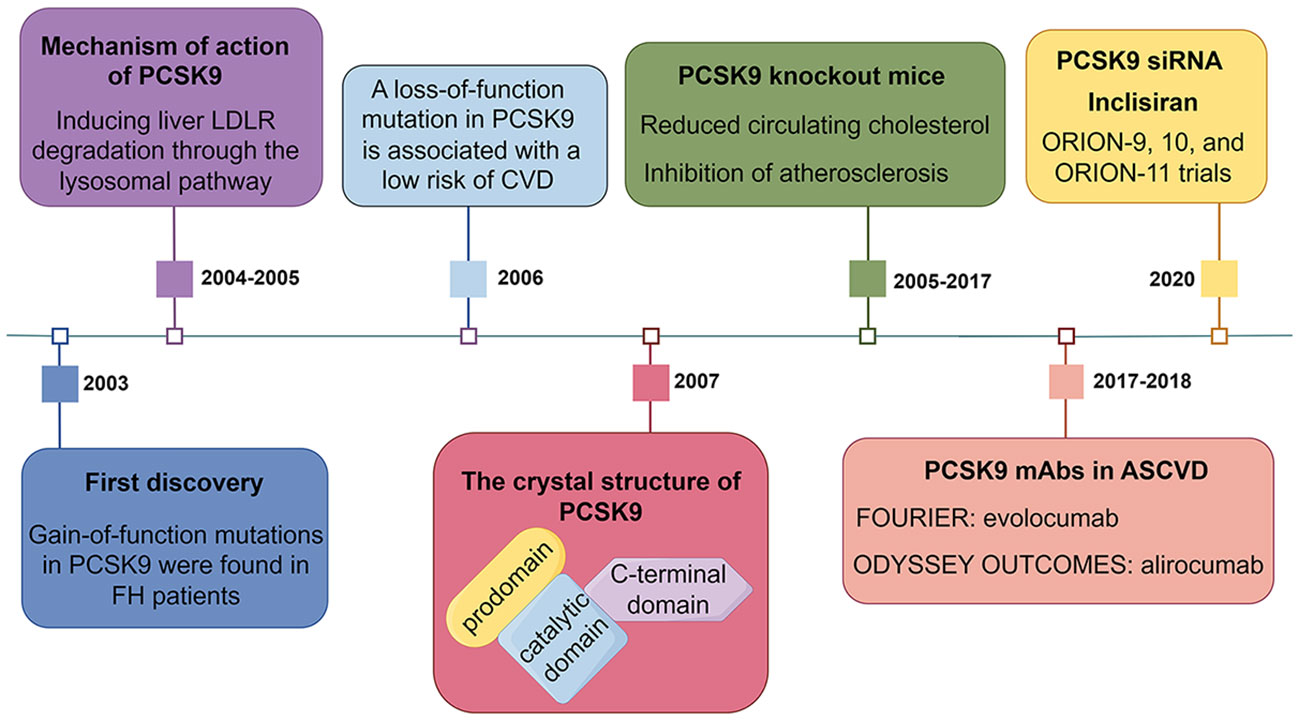
Figure 1 A timeline of significant findings on PCSK9 in lipid metabolism. LDLR, low-density lipoprotein receptor; FH, familial hypercholesterolemia; CVD, cardiovascular disease; mAbs, monoclonal antibodies; ASCVD, atherosclerotic cardiovascular disease; siRNA, small interfering RNA.
Undoubtedly, PCSK9 inhibitors are brilliant stars in the field of lipid metabolism. In addition, the role of PCSK9 in sepsis, vascular inflammation, and tumor immunity is also receiving increasing attention (38). The number of research publications on PCSK9 inhibitors shows an increasing trend year by year. For the field of PCSK9 inhibitors, however, there are no relevant studies with bibliometric analysis. Therefore, we review the related literature on PCSK9 inhibitors and analyze the related data using the bibliometric software CiteSpace, aiming to intuitively present the hotspots and evolution trends of PCSK9 inhibitor-related research and provide new ideas and references for future research.
2 Materials and methods
2.1 Literature search and data collection
We implemented a systematic literature search through the Web of Science Core Collection (WoSCC) database using the following strategy: TS = (“proprotein convertase subtilisin/kexin type 9 inhibitor*” OR “pcsk9 inhibitor*” OR “proprotein convertase subtilisin/kexin type 9 inhibition*” OR “pcsk9 inhibition”) AND LA=(English), from 1999 to December 31, 2022. The results showed that the first article on PCSK9 inhibitors was published in 2007. A total of 2421 records were retrieved. We then excluded 1349 literature studies on review articles, meeting abstracts, editorial material, letters, early access, news items, corrections, proceeding papers, book chapters, data papers, and reprints. The final search yielded 1072 papers. The procedure for searching was presented in Figure 2A.
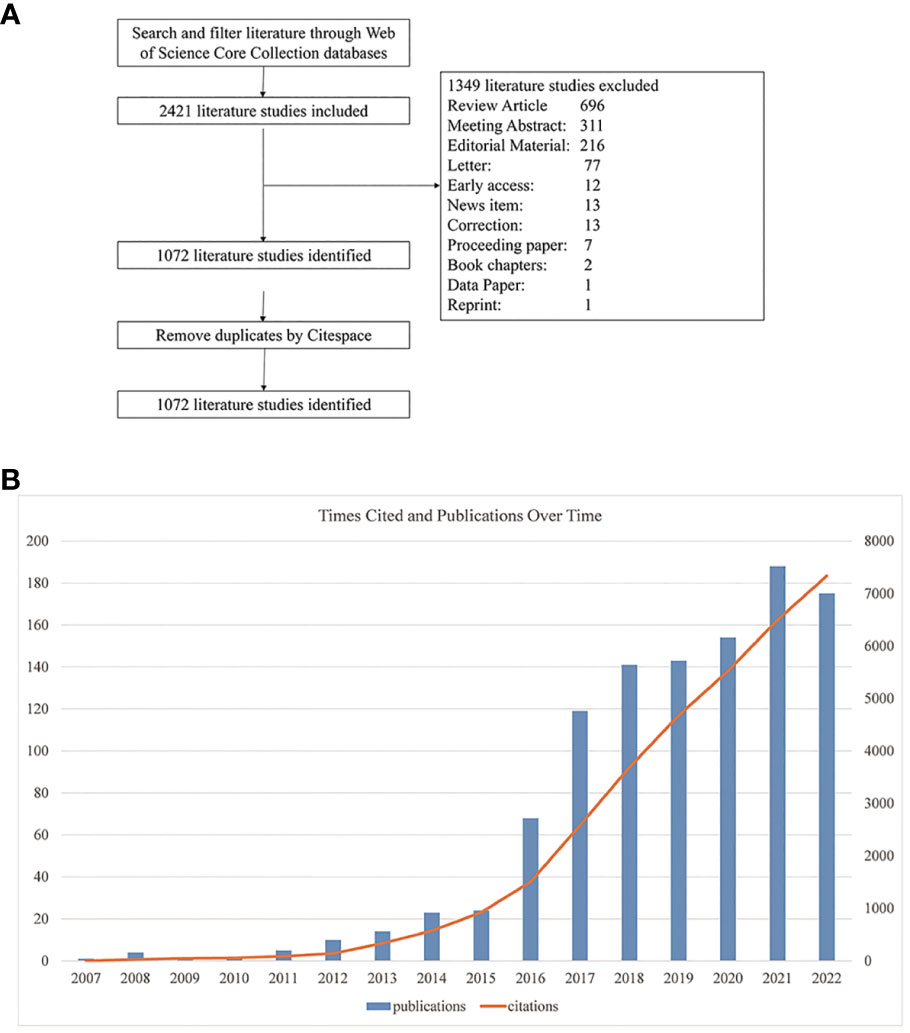
Figure 2 (A) Flowchart of the literature search and selection process. (B) Global trend of annual publications and citations related to PCSK9 inhibitors from 2007 to 2022.
2.2 Data analysis
The retrieved literature data were exported and then imported into CiteSpace software (version 6.2. R2) for further analysis and processing (39). Information extracted from each study included authors, keywords, affiliations, countries and regions, publication years, publication titles, and funding agencies. The visual maps drawn by CiteSpace consist of nodes and connections. Nodes typically denote specific items like authors, nations, or institutions, and their size and color indicate the amount and type of items accordingly. The number of connections between nodes shows how frequently items are co-cited or collaborated upon. The higher a node’s centrality, the more impact it has in the field, as centrality is a measure of importance in a relationship network. We also use Microsoft Excel (version 2019) and Graphpad software (version 9.4.1) for drawings.
3 Results
3.1 The global overview of publication outputs
The number of publications reveals the trend and rate of research development in a certain field. As shown in Figure 2B, the annual volume of literature in this field was less than 30 before 2016; it grew rapidly from 2016 onward, with 175 papers published by 2022. Similar to the number of publications, the citation volume of literature has also grown rapidly year by year. It’s worth noting that the number of articles published in 2022 was lower than in 2021; this could be because of the coronavirus disease 2019 (COVID-19) epidemic, which temporarily shifted the focus of scientific research. As shown in Table 2, the main research areas include cardiac cardiovascular systems (422, 39.37%), pharmacology pharmacy (226, 21.08%), and peripheral vascular disease (132, 12.31%), etc. For journal ranking, most papers related to PCSK9 inhibitors were published in the following journals: Journal of Clinical Lipidology (66, 6.16%), Atherosclerosis (45, 4.2%), Journal of the American College of Cardiology (31, 2.89%), Circulation (25, 2.33%), and Journal of the American Heart Association (25, 2.33%). In terms of funding, Amgen grants supported the most research (200, 18.66%), followed by two major pharmaceuticals, Regeneron (126, 11.75%) and Sanofi (103, 9.61%).
3.2 Analysis of countries and institutions
According to the WoSCC database, among the 89 countries or regions involved in the PCSK9 inhibitors field, the country with the highest volume of publications is the United States (507, 47.29%), followed by England (163, 15.21%), Canada (133, 12.41%), Italy (131, 12.22%), and France (128, 11.94%) (Table 2). Through CiteSpace screening, Figure 3 visualizes the outputs and links across countries. Among the top 10 countries in output ranking (Table 2), France, the United States, and Germany have the highest centrality, denoting their important role in the field of PCSK9 inhibitors. Further, from 2007 through 2022, the publications with the strongest bursts of citations in countries were analyzed. In Figure 4, the blue segment represents the timeline, while the red line represents the citation burst duration. The top 6 countries for the strongest citation bursts (Figure 4A) include the United States of America (USA) (2007–2016), Canada (2008–2013), South Africa (2012–2015), Germany (2013–2014), Scotland (2014–2018), and Thailand (2019–2020). Among these countries, the USA (16.13) showed the highest burst strength and longest duration. According to the WoSCC database, the top 5 institutions for article output in the field of PCSK9 inhibitor research (Table 3) are Harvard University (128, 11.94%), Amgen Inc. (114, 10.63%), Brigham & Women’s Hospital (107, 9.98%), Harvard Medical School (94, 8.77%), and Imperial College London (83, 7.74%). In the collaboration network (Figure 5A) and centrality ranking (Figure 5B) drawn by CiteSpace, the three institutions with the highest centrality are Ben Gurion Univ Negev (0.4), Imperial College London (0.35), and Leiden Univ (0.14), respectively, indicating their important position in the field, although some of them have relatively fewer publications. In addition, we conducted an analysis of citation bursts from various institutions over the past fifteen years (Figure 4B). Among these, the top-ranked institutions for citation bursts include: Univ Witwatersrand (5.79), Univ Amsterdam (5.73), and Harvard Univ (4.74), representing the highest research heat in the early years. In recent years, the Univ Paris, Harvard Med Sch, Icahn Sch Med Mt Sinai, and Inst Cardiovasc Rosario have also shown high enthusiasm for research.
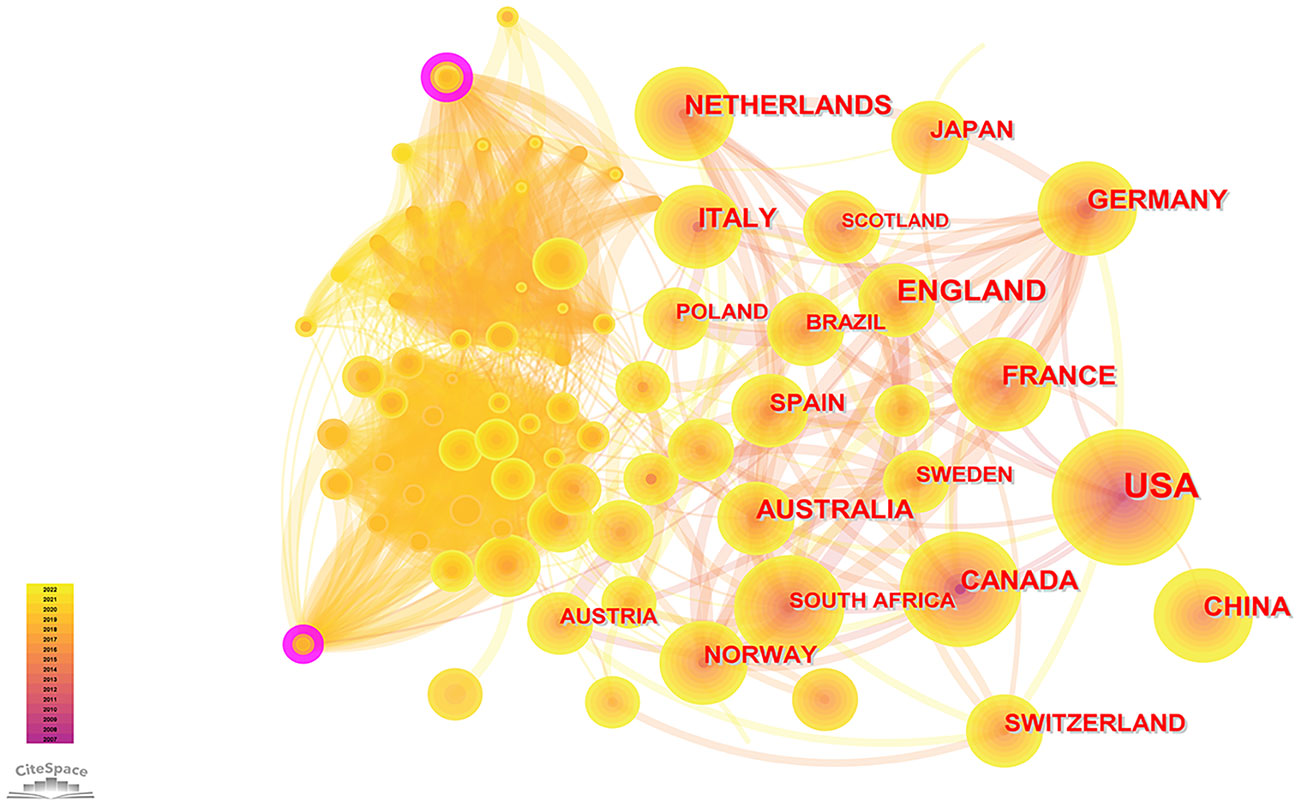
Figure 3 Visual maps of publications over countries studying PCSK9 inhibitors. Each node represents a country, and node size indicates the number of publications. The connection between the nodes represents a co-occurrence relationship. The figure shows the top countries in terms of the number of published articles. The USA ranks first, and the other countries are England, Canada, Italy, and France. The lines between the nodes represent the cooperation between different countries.
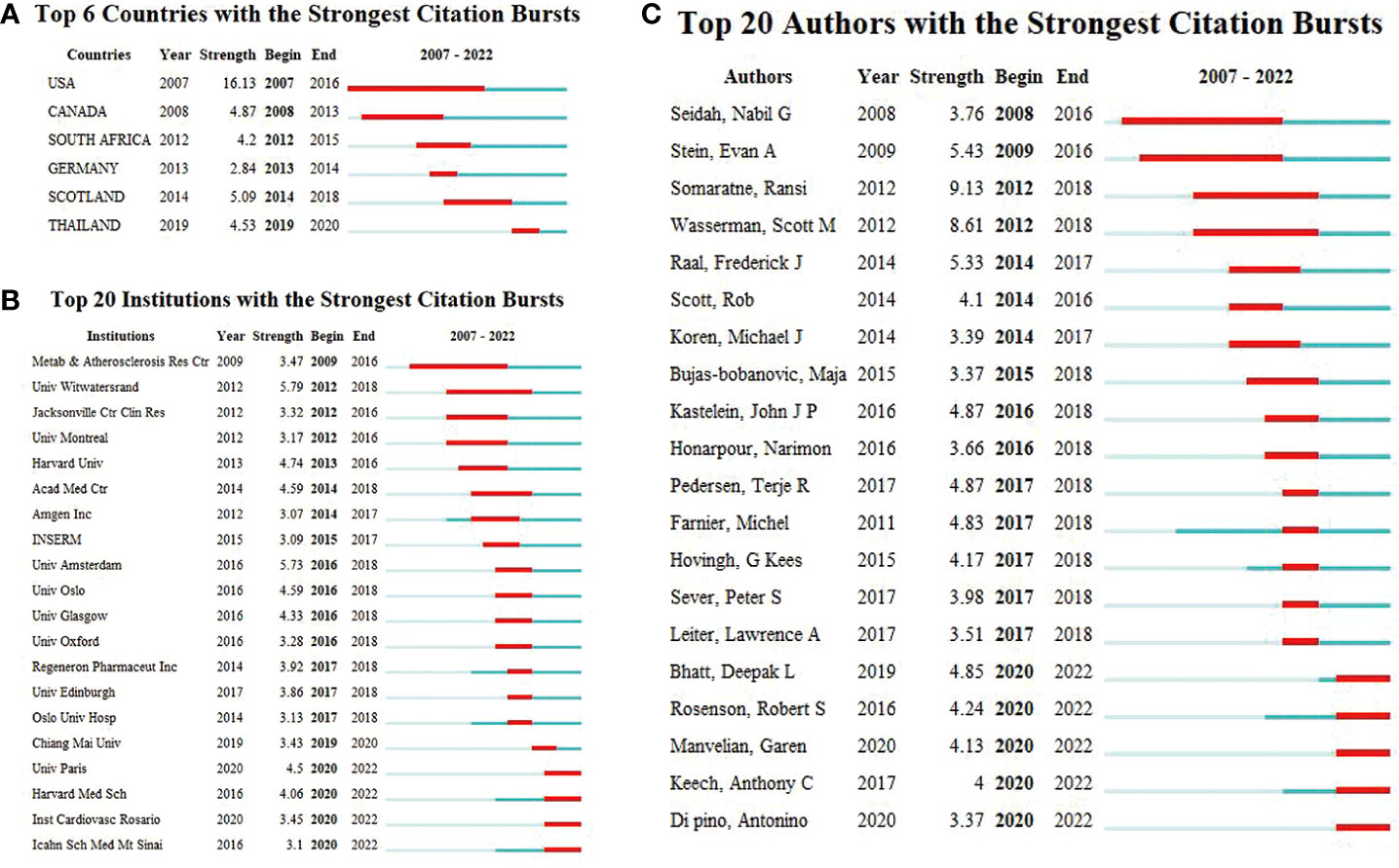
Figure 4 (A) Top six countries. (B) Top 20 institutions, and (C) Top 20 authors with the strongest citation bursts (2007–2022). Burst refers to a sudden increase in the number of citations in a certain period, suggesting an increased research in such a field. The red bands indicate the duration of burst.
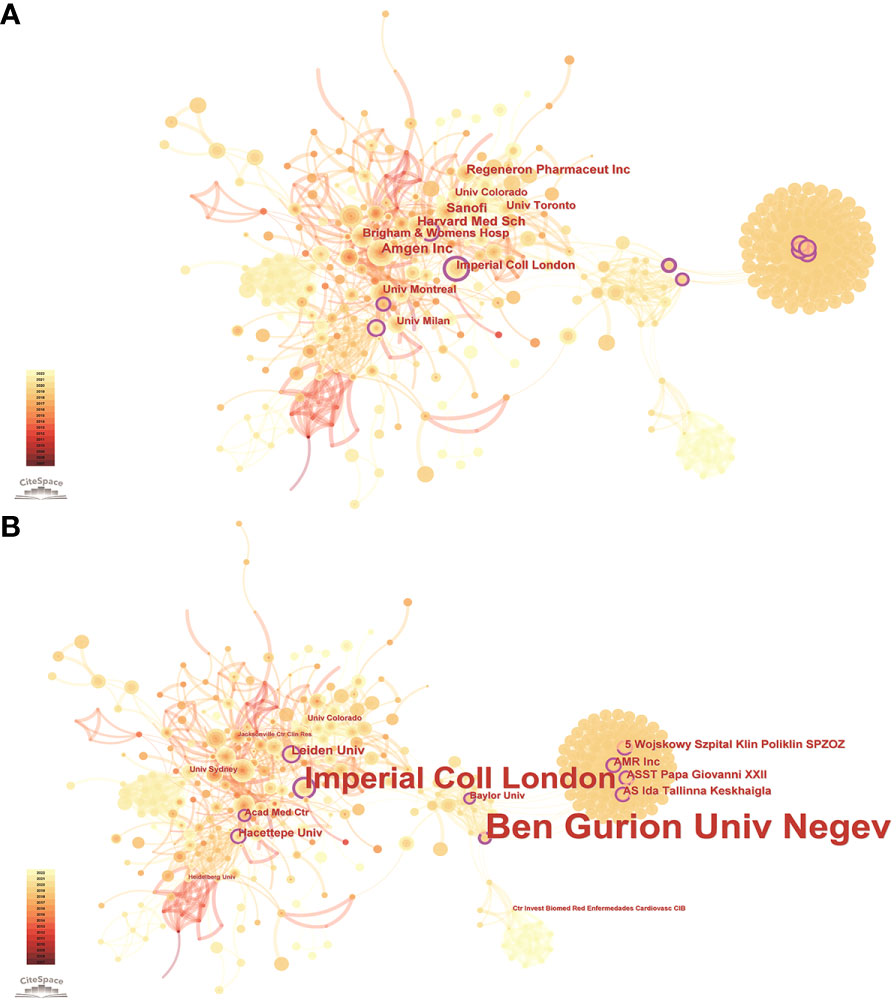
Figure 5 Publications over institutions. The node size represents the number of articles. The larger the node, the greater the number of articles. (A) The highest number of publications is from Amgen Inc, Harvard Med Sch, Sanofi, Regeneron Pharmaceut Inc, Brigham & Womens Hosp, Imperial Coll London, Univ Montreal, Univ Toronto, Univ Milan, Univ Colorado. (B) The highest centrality institutions are Ben Gurion Univ Negev, Imperial Coll London, Leiden Univ, Hacettepe Univ, ASST Papa Giovanni XXII, AMR Inc, Wojskowy Szpital Klin Poliklin SPZOZ, AS Ida Tallinna Keskhaigla, Acad Med Ctr, Baylor Univ. The lines between the nodes represent the cooperation between the different institutions.
3.3 Analysis of authors
According to the WoSCC database, Table 4 enumerates the top 6 authors with the highest number of publications in the field of PCSK9 inhibitors: Sabatine MS (51, 4.76%), Giugliano RP (46, 4.29%), Wasserman SM (38, 3.54%), Mach F (31, 2.89%), Jukema JW (29, 2.71%), and Somaratne R (29, 2.71%). In the ranking of centrality (Table 4), the top-ranked authors included: Wasserman SM (0.07), Cannon CP (0.06), Catapano AL (0.06), Blom DJ (0.06), Banach M (0.05), and Ballantyne CM (0.05). Figure 4C presents a list of the top 20 authors for citation bursts over the past 15 years. The two authors with the highest burst strength are Somaratne R (9.13) and Wasserman SM (8.61), who are much higher than the other authors.
3.4 Highly cited references
Highly cited references indicate that the academic quality and impact of the paper are widely recognized. Highly cited references can reflect hotspots in the field and are the focus of researchers. Table 5 shows the 10 most frequently cited literatures in terms of PCSK9 inhibitors. More than half of these 10 literatures were about clinical trials of PCSK9 mAbs, and almost all were published in the New England Journal of Medicine. The first-, fourth-, seventh-, and eighth-ranked literature examined the effect of evolocumab on LDLc and the risk of cardiovascular events, whereas the second- and third-ranked literature examined the effect of alirocumab on LDLc and the risk of cardiovascular events. The top 30 references, based on the citation bursts with the highest strength, are shown in Figure 6A. The article “Alirocumab and Cardiovascular Outcomes after Acute Coronary Syndrome”, published in 2018, wins the strongest citation burst. This article has a burst intensity of 52.31, and its heat might continue.
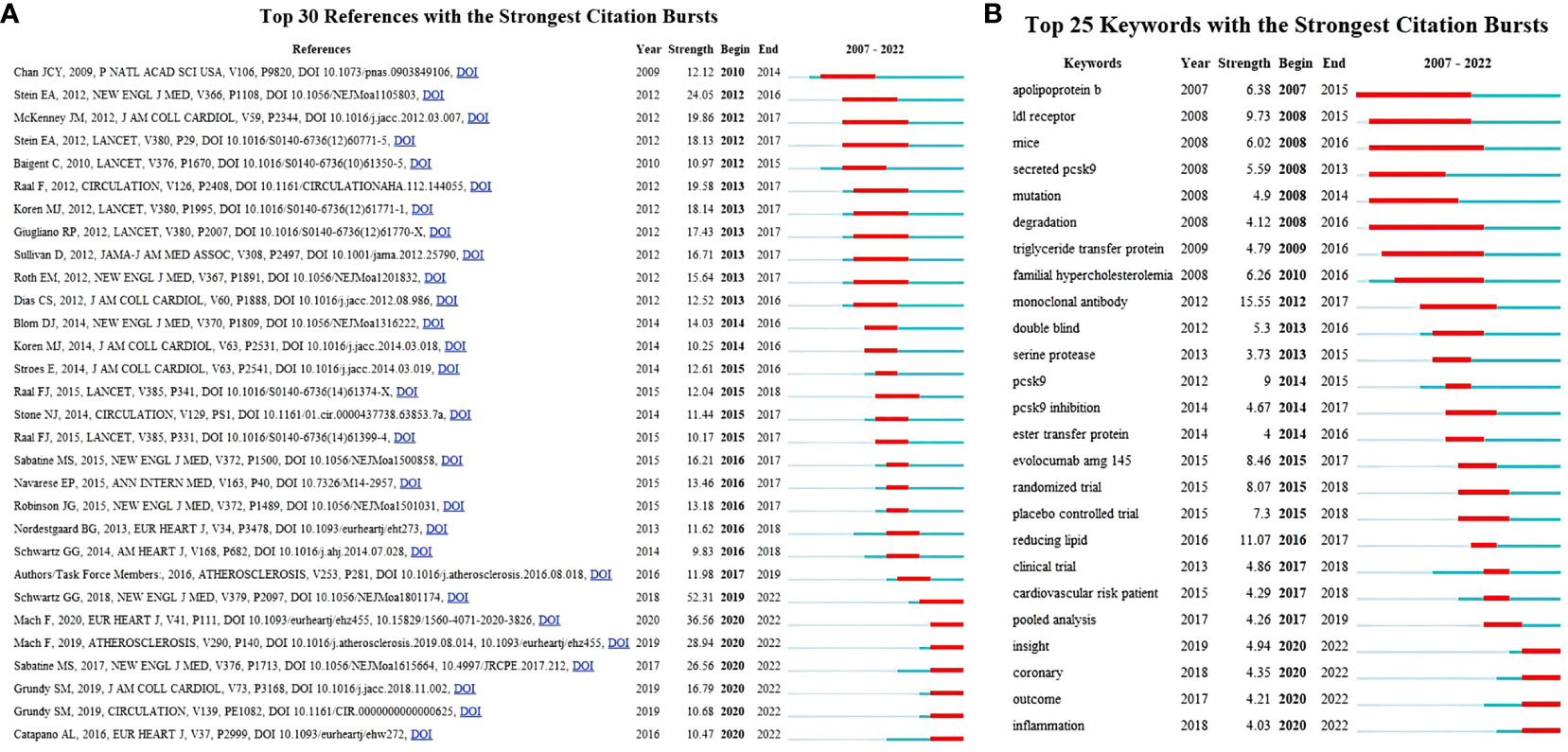
Figure 6 (A) The top 30 references with the strongest citation bursts. The red segment represents the begin and end years of the burst duration. (B) Top 25 keywords with the strongest citation bursts (2007–2022). Burst refers to a sudden increase in the number of citations in a certain period, suggesting an increased research in such a field. The red bands indicate the duration of burst.
3.5 Highly cited journals
The top 10 journals according to the number of citations are shown in Table 6. The New England Journal of Medicine (864) was the most cited journal, followed by Circulation (747), the Journal of the American College of Cardiology (739), the European Heart Journal (674), the Lancet (632), the Atherosclerosis (624), the Jama-Journal of the American Medical Association (565), the Journal of Clinical Lipidology (442), the American Journal of Cardiology (367), and the Journal of Lipid Research (345). The 2021 impact factor for these journals ranged from 3.133 to 202.731, with 60% of journals having an impact factor exceeding 20. Through journal citation reports (JCR) partition analysis, Q1 accounts for 90%. In addition, we also constructed a dual map overlay of journals on PCSK9 inhibitors (Figure 7) to describe the subject distribution of journals. The left part of the figure shows the citing journals, while the right part shows the cited journals. Three main citation pathways are shown in this figure. The two green citation lines indicate that research in Molecular, Biology, Genetics journals and Health, Nursing, Medicine journals is frequently cited by Medicine, Medical, Clinical journals. The orange citation line indicates that research in Molecular, Biology, Genetics journals is frequently cited by Molecular, Biology, Immunology journals.
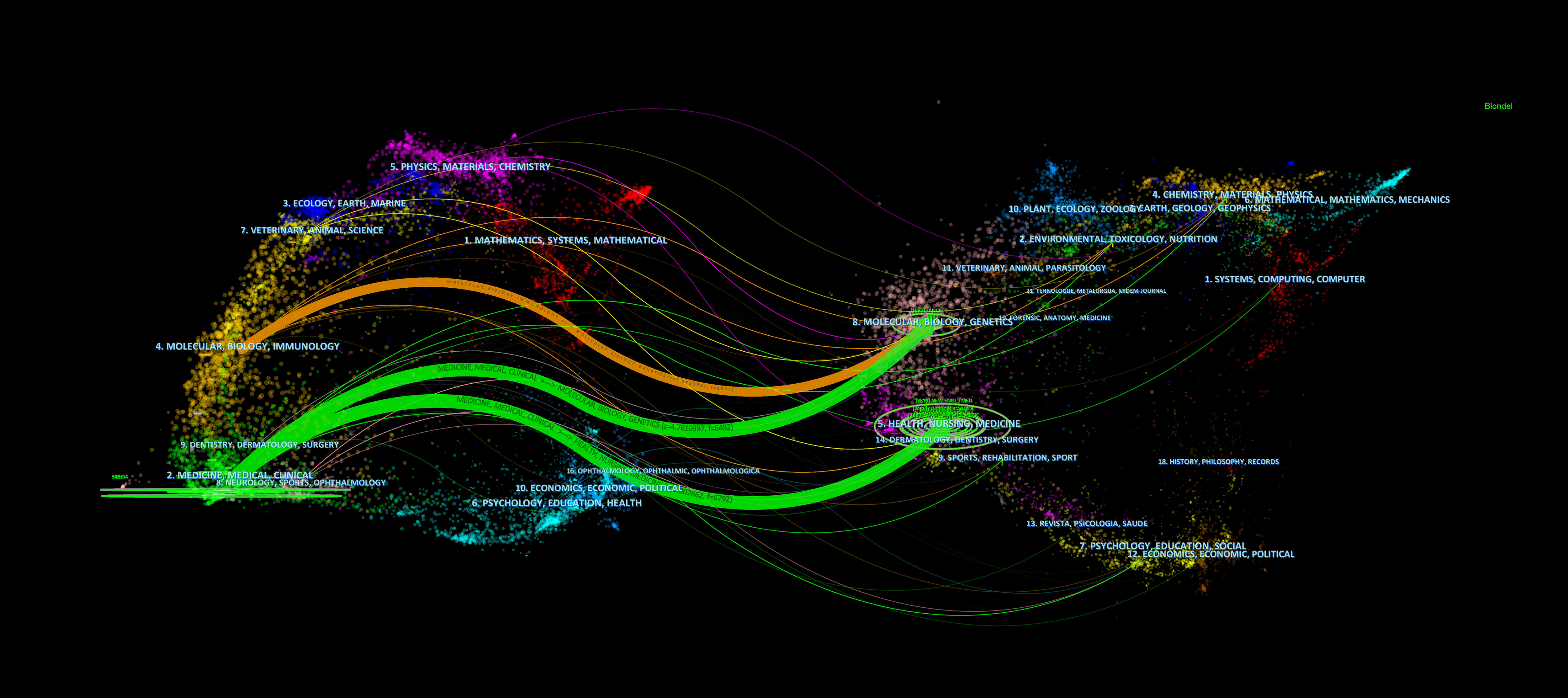
Figure 7 The dual map overlay of journals contributed to publications on the application of PCSK9 inhibitors from 2007 to 2022. All the orange paths showed articles in the research fields of molecular/biology/immunology are more likely to cite articles in the field of molecular, biology, genetics. While the green path showed articles in medicine/medical/clinical fieds are divided into two parts, one is health/nursing/medicine, the other is molecular/biology/genetics.
3.6 Analysis of keywords
The keywords are the core and main points of a paper and can reflect the hotspots and fronts of a certain field. Table 7 and Figure 8A show the co-occurrence analysis of keywords. The keywords with a high frequency of co-occurrence include “low density lipoprotein” (391), “familial hypercholesterolemia” (305), “pcsk9 inhibitor” (265), “pcsk9” (230), “efficacy” (221), “safety” (187), “risk” (164), “cholesterol” (159), “cardiovascular disease” (158), and “monoclonal antibody” (149). The keyword centrality ranking (Table 7) shows that “apolipoprotein b” (0.13), “atorvastatin” (0.11), “expression” (0.11), “cholesterol” (0.1), and “ldl receptor” (0.09) have higher centrality than other keywords, indicating that these keywords are the core of the PCSK9 inhibitors field. We further conducted the keyword cluster timeline using CiteSpace. Keyword clusters refer to groups consisting of keywords with similar research topics. A total of ten clusters are generated as shown in Figure 8B: “familial hypercholesterolaemia”, “hepg2 cell”, “pcsk9-monoclonal antibodies”, “rapid reduction”, “cognitive function”, “pcna guideline”, “secondary prevention”, “reducing low-density lipoprotein cholesterol”, “lowering therapy”, and “drug-target mendelian randomization study”. Circles denoted keywords in the keyword clustering timeline, and the number of occurrences was closely correlated with the circle’s diameter; the purple axis indicated 2007-2022. Meanwhile, we present the top 25 keywords in the field of PCSK9 inhibitors that have had the strongest citation bursts within the past 15 years (Figure 6B). Notably, “monoclonal antibody” (15.55) has the strongest citation bursts, followed by “reducing lipid” (11.07), “ldl receptor” (9.73), “pcsk9” (9), “evolocumab amg 145” (8.46), and “randomized trial” (8.07). As can be seen from Figure 6B, “apolipoprotein b”, “ldl receptor”, “mice”, “secreted pcsk9”, “mutation”, “degradation”, “triglyceride transfer protein”, and “familial hypercholesterolemia” were the earliest keywords in the field of PCSK9 inhibitors and were therefore the subjects of interest to researchers in the early years. Moreover, “insight”, “coronary”, “outcome”, and “inflammation” are at the forefront of the current PCSK9 inhibitor research field and are in a period of explosion.
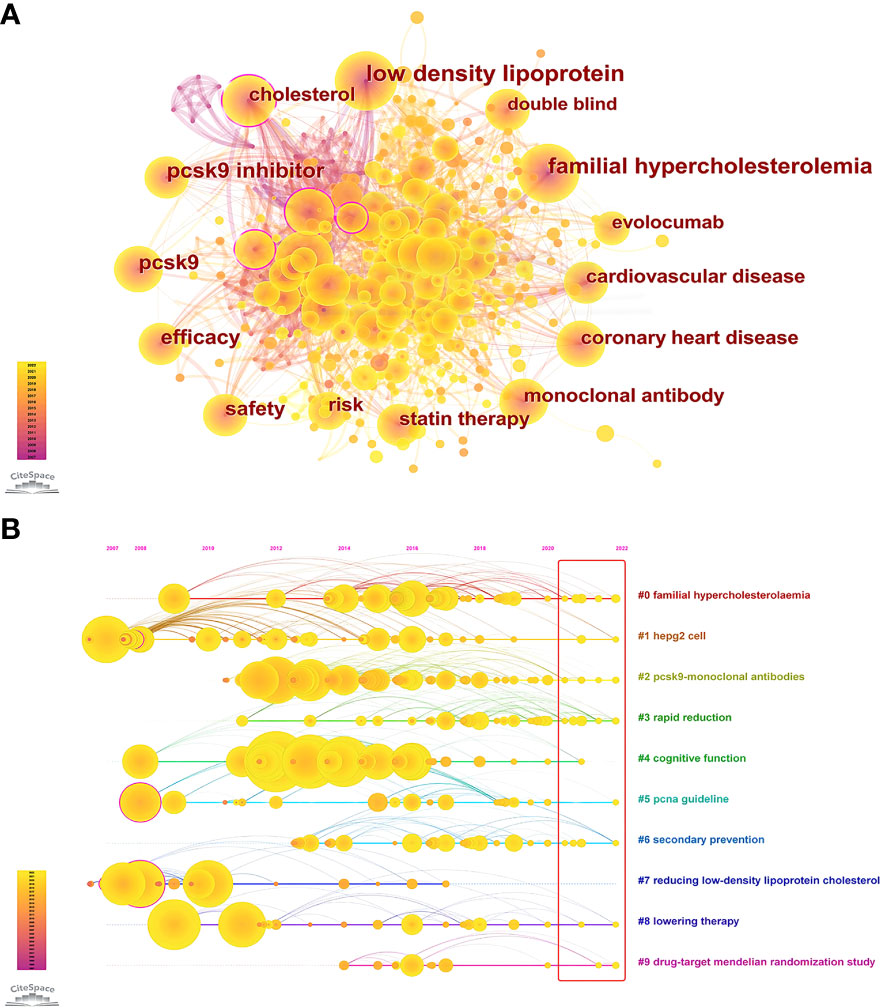
Figure 8 (A) Co-occurrence Analysis of keywords. Each node represents a keyword, and node size indicates the number of occurrences. The connection between the nodes represents a co-occurrence relationship. The top ten co-occurrence keywords are “low density lipoprotein” (391), “familial hypercholesterolemia” (305), “pcsk9 inhibitor” (265), “pcsk9” (230), “efficacy” (221), “safety” (187), “risk” (164), “cholesterol” (159), “cardiovascular disease” (158), and “monoclonal antibody” (149). (B) Circles indicated keywords; the size of the diameter of the circle was closely related to the number of occurrences; those with purple circles stated the existence of significant turning points in scientific knowledge, i.e., higher centrality; the purple axis indicated 2007-2022; yellow circles still in the red rectangle indicated the occurrence of novel related keywords.
4 Discussion
4.1 General tendency of research
In this study, the literature related to PCSK9 inhibitors was analyzed using CiteSpace quantitative analysis software. Publications, nations, organizations, writers, keywords, literature, journals, and other objects were all subjected to a quantitative evaluation. From the number of publications (Figure 2B), very few PCSK9 inhibitor-related articles existed before 2012. The possible reason is that researchers have focused mainly on animal models and the mechanism of the decrease in LDLc caused by PCSK9 deficiency (40–45). Since 2012, with the continuous development of clinical trials of PCSK9 mAbs (46–50), the output of papers in this field has also been increasing year by year. Given the large number of clinical trials of PCSK9 mAbs demonstrating efficacy and safety for lipid lowering (51–53), the US Food and Drug Administration and the European Medicines Agency approved evolocumab and alirocumab, two PCSK9 mAbs, in 2015 for LDLc lowering, particularly in patients with familial hypercholesterolemia (FH). Thus, the number of publications has grown rapidly since 2015. In 2019, with the release of the results of phase 3 trials of inclisiran (54), a PCSK9 siRNA, this field is gaining more attention, even though the paper output is tempered by the outbreak of COVID-19.
As can also be seen in Table 5, multiple heavyweight papers were published in 2015, all of which were published in the New England Journal of Medicine or the Lancet. These articles, including the two most frequently cited ones (Sabatine MS, 2017 and Schwartz GG, 2018), are almost exclusively large clinical trials of PCSK9 mAbs, indicating that clinical trials of PCSK9 inhibitors have received significant attention from researchers and also demonstrating their strong interest and recognition in the clinical application of PCSK9 inhibitors. In addition, two of the top 10 articles were about dyslipidemia management guidelines, demonstrating the important role of guidelines in guiding clinical and scientific research work. As we can see from Table 2, the USA, England, Canada, Italy, and France are the five countries with the largest number of published articles. Notably, the number of publications for American academics was almost the sum of the number of publications from the other four countries. The top five institutions by number of publications include Harvard University, Amgen Inc., Brigham & Women’s Hospital, Harvard Medical School, and Imperial College London, with four institutions located in the United States and one in England. In a similar vein, four of the top five funding organizations are American, and one is French. Consistent with the countries citation bursts intensity (Figure 4A), the United States undoubtedly holds a leading position in the field of PCSK9 inhibitors due to excellent research institutions and sufficient financial support. In the cooperation network of countries (Figure 3), there is close cooperation and communication between developed countries, such as France, the United States, Germany, and so on. It is worth noting that although China is the only Asian country in the top 10 ranking for the number of publications (Table 2), there is little cooperation between China and other countries. And there were not many high-quality studies published in top journals in China. Further analysis of countries bursts (Figure 4A) show that South Africa and Thailand exhibit research enthusiasm in this area, in addition to developed countries such as Europe and the United States. The relatively lagging biomedical industry may be the reason why developing countries such as China have published fewer papers in this field. In addition, the use of PCSK9 inhibitors is particularly constrained in underdeveloped countries by the high expenditures associated with research and development (R&D) and clinical trials. China has abundant biological resources and a huge market demand. With the government’s support, gradually increased R&D input, and enhanced cooperation and communication with other countries, it is believed that China will also achieve high-quality research in this field in the future. Four American authors, one Swiss author, and one Dutch author make up the top-ranked authors for the number of published articles (Table 4). Sabatine MS and Giugliano RP work at Brigham and Women’s Hospital. Wasserman SM and Somaratne R are both employed by Amgen. Mach F works at the University of Geneva. Jukema JW is from Leiden University Medical Center. It is worth mentioning that two professors, Sabatine MS and Giugliano RP, who have been long dedicated to the research of PCSK9 inhibitors in clinical trials since 2012 and have continuously presented high-quality papers to date, have made important contributions to the clinical applications of PCSK9 inhibitors. The top-ranked author for centrality is Wasserman SM from Amgen (Table 4), who has also been involved in a large number of clinical trials of PCSK9 inhibitors, undoubtedly having a profound impact on the development of this field. Overall, our results demonstrate consistency among high-yield countries, institutions, authors, and funding agencies. Developed countries such as the United States and Western European countries have been leading the way in the study of PCSK9 inhibitors. In addition, developing countries such as China should enhance their exchanges and cooperation with other countries to advance this field.
Journal and cited journal ranking analysis helps researchers select the most appropriate journal for their manuscript (55, 56). Table 2 displays the relative number of PCSK9 inhibitor-related publications published in many medical journals. The Journal of Clinical Lipidology (Q1, IF=5.365) leads the pack, followed by Atherosclerosis (Q1, IF=6.847), the Journal of the American College of Cardiology (Q1, IF=27.203), Circulation (Q1, IF=39.918), and the Journal of the American Heart Association (Q2, IF=6.106). This result suggests that high-quality journals favor relevant studies on PCSK9 inhibitors. Of the ranking of cited journals (Table 6), 90% are high impact Q1 international journals. Among these journals, the New England Journal of Medicine and Journal of the American College of Cardiology focus more on the related clinical studies of PCSK9 inhibitors, while the Journal of Lipid Research et al. emphasize the early basic research in the PCSK9 field. This means that the field of PCSK9 inhibitors is currently relatively mature, either in basic or clinical studies. Moreover, the dual journal map overlay (Figure 7) reveals that “molecular, biology, genetics” are frequently cited in “medicine, medical, clinical” demonstrating that current PCSK9 inhibitor research is increasingly concentrated on clinical translation.
4.2 Research focuses and frontiers
Keywords help understand the focus and evolution of specific research areas. Based on the keyword co-occurrence analysis, keyword timeline, and citation bursts, we believe that the research focuses of PCSK9 inhibitors mainly include the following aspects: lipid-lowering mechanisms of PCSK9 inhibitors, PCSK9 mAbs and related clinical trials.
4.2.1 Lipid-lowering mechanisms of PCSK9 inhibitors
Keywords before 2012 included: apolipoprotein B, LDL receptor, mice, secreted pcsk9, degradation, and so on. These keywords appear to correspond to basic research on PCSK9, suggesting that earlier research focused on the mechanisms of lipid lowering by PCSK9 inhibitors. PCSK9 is the third gene related to cholesterol metabolism after ApoB and LDLR; its mechanism of action was first revealed in 2004 by Maxwell and Breslow et al. (13, 14). They found that PCSK9 reduces LDLR protein levels by inducing LDLR degradation in HepG2 cells. Further studies revealed that PCSK9 may induce LDLR degradation through both circulating and intracellular pathways. Through its catalytic subunit, PCSK9 secreted by the liver binds the EGF-A domain of the LDLR and directs it to endosomes/lysosomes for degradation (45, 57, 58). In addition, several lines of evidence support the relatively rapid intracellular pathway of LDLR degradation by PCSK9 through the trans-Golgi to lysosomes (59). For example, autosomal recessive hypercholesterolemia protein (ARH), an adaptor protein, is required for PCSK9-LDLR complex internalization by hepatocytes (60, 61). However, overexpression of Pcsk9 in Arh -/- mice still reduced hepatic LDLR protein levels (62). Blocking the clathrin light chain, which is responsible for intracellular trafficking from the trans-Golgi network to lysosomes, increased LDLR levels within HepG2 cells in a PCSK9 dependent manner without affecting the ability of circulating PCSK9 to enhance LDLR degradation (59). Notably, both intracellular and extracellular LDLR degradation pathways of PCSK9 require the presence of the CHRD, suggesting that this domain is a determinant of LDLR degradation by PCSK9 (63–65). However, the absence of the CHRD domain does not affect the binding of PCSK9 to the LDLR, although it impedes PCSK9-LDLR trafficking to lysosomes (65, 66). On the other hand, mutations in the cytoplasmic domain of LDLR did not affect its degradation by PCSK9 (67). These results indicate that an unknown protein is required for PCSK9 to degrade LDLR. Recent studies have proposed that cyclase-associated protein 1 (CAP1) may be a candidate protein that binds to the M1 and M3 domains of the CHRD and enhances the degradation of PCSK9-LDLR in lysosomes (68).
4.2.2 PCSK9 mAbs and related clinical trials
Keywords from 2012 to 2017 include “monoclonal antibody”, “double blind”, “PCSK9 inhibitor”, “randomized trial”, “evolocumab amg 145”, etc., indicating a shift in researchers’ focus from basic to clinical research, with a focus on PCSK9 monoclonal antibodies and randomized controlled trials (RCTs). Anti-PCSK9 neutralizing antibody (mAb1) was first generated in 2009 by Chan et al., who showed that a single injection of mAb1 resulted in an 80% reduction in circulating LDLc levels in cynomolgus monkeys (41). This exciting discovery lays the foundation for the development of PCSK9 mAbs. Subsequently, since 2012, clinical trials of PCSK9 mAbs have sprouted like mushrooms. In a phase 1 trial, REGN727/SAR236553 (Alirocumab), a PCSK9 mAb developed by Regeneron in collaboration with Sanofi, significantly reduced LDLc levels in the healthy population and in heterozygous hypercholesterolemic patients receiving statin therapy. For the latter, it could be reduced by up to 61% (69). Similar results were observed in a clinical trial of AMG145 (Evolocumab), a PCSK9 mAb developed by Amgen (50). All studies have shown that PCSK9 mAbs (evolocumab or alirocumab) with or without statin therapy significantly reduce LDLc in patients with hypercholesterolemia and heterozygous familial hypercholesterolemia (31, 32, 49, 70). In patients with statin intolerance due to muscle related side effects, the results of the GAUSS study showed that evolocumab similarly reduced LDLc levels (48, 71, 72). Notably, individual responses to the PCSK9 inhibitor evolocumab vary widely among patients with homozygous familial hypercholesterolemia (HoFH). Evolocumab did not lower LDLc in patients with HoFH who completely lacked LDLR (73). Because of the residual LDLR expression and differences seen in most patients with HoFH, the PCSK9 inhibitor evolocumab remains capable of lowering LDLc by 20-30% on statins and ezetimibe basis (73–76). Together, these studies fully demonstrate the lipid-lowering efficacy of PCSK9 mAb. In 2016, GLAGOV, a multicenter randomized controlled study, was the first to evaluate the effect of elovocumab on atherosclerosis (77). Through intravascular ultrasonography (IVUS) imaging measurement, after 78 weeks of treatment with evolocumab on the basis of statins, the percent atheroma volume (PAV) of coronary heart disease patients decreased by 0.95% compared with the baseline (77). In 2017-2018, FOURIER and ODYSSEY OUTCOMES, two large-scale RCTs, respectively, confirmed the lipid-lowering effect and cardiovascular benefits of evolocumab and alirocumab in ASCVD, reducing the relative risk of major cardiovascular endpoint events by 15% (33, 34). The HUYGENS study, published in 2022, assessed the effects of evolocumab on nonculprit vessel plaques in patients with non-ST-segment elevation myocardial infarction based on optical coherence tomography (OCT) (78). The study demonstrated that statin therapy with evolocumab for 50 weeks resulted in an increase in minimum fibrous cap thickness that was approximately 2 times that of placebo, significantly improving plaque vulnerability (78). Similarly, the PACMAN-AMI study evaluated the effectiveness of alirocumab in reversing and stabilizing plaques in patients with acute myocardial infarction (79). The results showed that the reduction in percent atheroma volume and maximum lipid core burden index in the alirocumab group was about twice that of the placebo group (79). The recently released FOURIER-OLE study evaluated the safety and efficacy of long-term use of evolocumab, making it the longest study to date exposed to PCSK9 inhibitors (with a longest follow-up time of up to 8 years) (80). This study further strengthens the position of the PCSK9 mAbs in the secondary prevention of ASCVD patients, advocating for the cardiovascular benefits of early and long-term lipid-lowering therapy. Thus, these clinical trials provide a solid foundation for the evidence-based use of PCSK9 inhibitors in lowering LDLc, reversing atherosclerotic plaques, and reducing cardiovascular events.
4.2.3 PCSK9 inhibitors and inflammation
Based on the keyword bursts, in addition to “coronary” (mentioned above), which is now a hotspot and frontier in the field of PCSK9 inhibitors, inflammation research is likewise at the forefront in this area and is now in an explosive period. In the early years, several observational studies found that plasma PCSK9 concentrations were positively correlated with many inflammatory markers, such as white blood cells, high-sensitivity C-reactive protein (hs-CRP), fibrinogen, etc. (81, 82). Notably, in addition to the liver, small intestine, pancreas, and other organs, PCSK9 is also highly expressed in vascular endothelial cells (EC) and vascular smooth muscle cells (VSMCs) (83). Ding et al. found that PCSK9 in vascular EC and VSMCs is regulated by inflammatory factors such as lectin-like ox-LDL receptor-1 (LOX-1) and tumor necrosis factor-α (TNF-α) (84). As is well known, inflammation is involved in the development and progression of ASCVD. These findings led researchers to focus on the association of PCSK9 with inflammation in atherosclerosis. A clinical study showed that elevated circulating PCSK9 concentrations remained associated with new plaque formation after adjustment for LDLc levels (85). In a basic study, PCSK9 silencing inhibits atherosclerosis without changing plasma cholesterol levels by reducing vascular inflammation and blocking the TLR4/NF-κB signaling pathway (29).In addition, PCSK9 expressed by macrophages promoted atherosclerosis by increasing plaque monocyte infiltration and expression of inflammatory markers independent of circulating cholesterol levels (86). These studies indicate that PCSK9 can directly promote atherosclerotic inflammation, independent of lipid metabolism disorders. Next, the researchers studied the effect of PCSK9 inhibitors on vascular inflammation and atherosclerosis. In animal experiments, Landlinger et al. inhibited circulating PCSK9 by vaccination and found that inhibition of PCSK9 resulted in downregulation of intracellular adhesion molecule-1 (ICAM-1) expression in endothelial cells as well as circulating inflammatory markers (87). In a prospective, observational clinical study, Vlachopoulos et al. assessed arterial inflammation by 18F-fluoro-2-deoxy-D-glucose (FDG) positron emission tomography/computed tomography (PET/CT). They found that 12 months of treatment with evolocumab or alirocumab significantly improved arterial inflammation (88). In addition, several clinical trials have also demonstrated the anti-inflammatory effects of PCSK9 inhibitors (89–91).
COVID-19 is characterized by pathological inflammation and thrombosis (92, 93). High levels of circulating LDLc and lipoprotein (a) [Lp(a)] lead to endothelial dysfunction, accompanied by inflammation and atherosclerosis thrombosis, which are enhanced on the basis of COVID-19, thus aggravating the condition (94–96). Given this, statins have been shown to improve both endothelial dysfunction and prognosis in COVID-19 patients due to their ability to reduce LDLc (97). PCSK9 inhibitors can further reduce the circulating LDLc level when combined with statins and can reduce the serum Lp (a) level by approximately 30% (53), making them a candidate drug for COVID-19. In addition, PCSK9 is directly related to the systemic inflammatory response (98, 99). The anti-inflammatory effects of PCSK9 inhibitors have been widely reported, especially for the interleukin-6 (IL-6)-mediated inflammatory signaling pathway (99–101), which is a driving factor for more severe inflammation in COVID-19 (102). A recent observational study found a significant increase in plasma PCSK9 levels in patients with COVID-19 sepsis compared to those without SARS-CoV-2 infection, suggesting that PCSK9 may be a potential biomarker for COVID-19 (103). PCSK9 inhibitors may inhibit viral infection by reducing viral cholesterol levels, such as dengue virus, according to some previous virus related studies (104, 105). In addition to the improvement of endothelial function related to cholesterol metabolism, PCSK9 inhibitors also benefit from cholesterol-independent mechanisms (104). For example, the PCSK9 inhibitors upregulate the expression of antiviral type 1 interferon in host hepatocytes (106). Therefore, COVID-19 patients with a gain-of-function mutation of PCSK9 or increased circulating PCSK9 levels (like FH) and type 1 interferon immune deficiency may benefit most from PCSK9 inhibitors (104). The results of a recent multicenter clinical trial showed that the use of evolocumab reduces the 30-day mortality rate or intubation requirement in severe COVID-19 patients by 30%, accompanied by a significant reduction in serum IL-6 levels (101, 107). In addition, patients with more severe systemic inflammation experienced a greater decrease in mortality after using PCSK9 inhibitors (101). This study provides compelling evidence that PCSK9 inhibitors directly control inflammation in COVID-19.
4.2.4 PCSK9 inhibitors and tumors
Cholesterol metabolism is crucial to the growth and development of tumors (108). Given that LDLR provides cholesterol to the liver and other tissues, the upregulation of LDLR in tumors is believed to be associated with cancer development (109). Interestingly, the PCSK9 mAbs did not result in a rise in the incidence of cancer despite increasing the levels of LDLR in the liver and other tissues (33). On the contrary, current research has shown that PCSK9 deficiency inhibits tumor growth (110, 111). Recent studies have shown that tumors evade immune responses by expressing PCSK9 to degrade the major histocompatibility type-I receptor (MHC-I) (112). In addition, PCSK9 inhibition enhances the sensitivity of tumors to immune checkpoint therapy (112). Furthermore, Yuan et al. found that PCSK9 damages the anti-tumor activity of CD8+ T cells in the tumor microenvironment by inhibiting LDLR-mediated TCR signals (113). These findings are of great significance for the use of PCSK9 inhibitors in clinical tumor treatment.
The great progress of PCSK9 inhibitors from basic research to clinical conversion over the past decade has largely benefited from their strong reduction in LDLc levels and ASCVD risk without significant side effects, as the keywords “efficacy” and “safety” show in Table 7. However, some aspects of clinical practice still need attention. Hyporesponsiveness of some patients to PCSK9 mAbs therapy, for example, is defined as a decrease in LDLc levels of less than 15% (114, 115). One important reason is improper entry and distribution of antibodies, such as poor adherence to subcutaneous injection and incorrect injection methods (116). In addition, our results indicate that research on PCSK9 inhibitors is limited in developing countries, partly due to the significant economic burden that restricts their clinical use. Therefore, it is crucial to develop other strategies for inhibiting PCSK9 in these situations. Inclisiran, which is still in development and has more persistent effects (54), and even oral peptides (117) may have a chance to solve this problem. Surprisingly, despite the results of three significant Phase III clinical trials of Inclisiran being published in 2020 (54, 118), no Inclisiran-related words were found in our keywords analysis, indicating that research in this field is still in its infancy. The results of studies with cardiovascular outcomes as the endpoint may be highly anticipated. The role of PCSK9 in extrahepatic tissues is largely unknown. Our keywords analysis shows that “inflammation” (Figure 6B) and “drug-target mendelian randomization study” (Figure 8B) are currently the hotspots and frontiers in this field. This suggests that clinicians and researchers should pay attention to the role of PCSK9 inhibitors in non-cardiovascular fields, such as inflammation, tumors, and the nervous system. In fact, recent Mendelian randomization studies have focused on the associations between PCSK9 inhibition and cognitive function (119), COVID-19 (120), and cancer (121). In addition, the latest research suggests a U-shaped correlation between LDLc levels and the risk of all-cause mortality mediated by infection after acute ischemic stroke (122). This study suggests that even though PCSK9 inhibitors have significant cardiovascular system benefits, further study on cerebrovascular accidents is still required. The role of PCSK9 inhibitors in non-cardiovascular fields is anticipated to be the focus of future research.
4.3 Strengths and limitations
The study employs a rigorous approach to comprehend and analyze a substantial amount of data and presents the accumulated scientific findings and subtle evolutionary trends in the area of PCSK9 inhibitors in a visually appealing manner. To our knowledge, this is the first study to analyze the trends in PCSK9 inhibitor research over the past 15 years using the bibliometric tool CiteSpace. Our research aims to lay a solid foundation for future development in this field by assisting clinicians and researchers in quickly obtaining an overview of knowledge in this field and identifying knowledge gaps; identifying potential future research directions and initiating new research ideas; and finally, positioning their expected contributions to the field (123).
However, the present study also has some limitations. First, we only conducted a literature search in the WoSCC database, and relevant studies from other databases may have been missed. Second, only English-language publications were included in our study, so articles published in other languages were missed. These two limitations may lead to biased results.
5 Conclusion
This study presents an analysis of research in the field of PCSK9 inhibitors by CiteSpace. Specifically, we performed a visual analysis of items from different years, countries, institutions, authors, keywords, literature, and so on. In conclusion, the number of publications on PCSK9 inhibitors has risen year by year over the past 15 years. The countries, authors, and institutions with the most published articles in the field of PCSK9 inhibitors are from the United States. Compared with developed countries, developing countries such as China need increased international exchange and cooperation. Studies of PCSK9 inhibitors have focused on the mechanisms of LDLc lowering by PCSK9 inhibitors, PCSK9 mAbs and related clinical trials. Coronary artery and inflammation are currently at the forefront of research in the field and are in an explosion period.
Data availability statement
The original contributions presented in the study are included in the article/supplementary material. Further inquiries can be directed to the corresponding author.
Author contributions
FL conceived the idea. QL wrote the manuscript. QL and PW collected and read the literature. ZT and ZC conducted the data collection and analysis. ZT, FL and ZF read through and corrected the manuscript. All authors contributed to the article and approved the submitted version.
Funding
This research was supported by grants from Hunan Provincial Natural Science Foundation of China [grant number 2021JJ40852]; National Natural Science Foundation of China [grant number 82100495]; Scientific Research Project of Hunan Provincial Health Commission [grant number 202203014009]; Scientific Research Launch Project for new employees of the Second Xiangya Hospital of Central South University; China Postdoctoral Science Foundation; Key Research and Development Program of Hunan Province of China [grant number 2021SK2004].
Acknowledgments
We would like to express our gratitude to all those who helped us during the writing of this manuscript. Figure 1 was drawn by Figraw. We appreciate Figraw’s drawing service. Thanks to all the peer reviewers for their opinions and suggestions.
Conflict of interest
The authors declare that the research was conducted in the absence of any commercial or financial relationships that could be construed as a potential conflict of interest.
Publisher’s note
All claims expressed in this article are solely those of the authors and do not necessarily represent those of their affiliated organizations, or those of the publisher, the editors and the reviewers. Any product that may be evaluated in this article, or claim that may be made by its manufacturer, is not guaranteed or endorsed by the publisher.
References
1. Seidah NG, Sadr MS, Chretien M, Mbikay M. The multifaceted proprotein convertases: Their unique, redundant, complementary, and opposite functions. J Biol Chem (2013) 288:21473–81. doi: 10.1074/jbc.R113.481549
2. Seidah NG, Prat A. The biology and therapeutic targeting of the proprotein convertases. Nat Rev Drug Discovery (2012) 11:367–83. doi: 10.1038/nrd3699
3. Seidah NG, Chretien M. Proprotein and prohormone convertases: A family of subtilases generating diverse bioactive polypeptides. Brain Res (1999) 848:45–62. doi: 10.1016/s0006-8993(99)01909-5
4. Lazure C, Seidah NG, Pelaprat D, Chretien M. Proteases and posttranslational processing of prohormones: A review. Can J Biochem Cell Biol (1983) 61:501–15. doi: 10.1139/o83-066
5. Seidah NG, Benjannet S, Wickham L, Marcinkiewicz J, Jasmin SB, Stifani S, et al. The secretory proprotein convertase neural apoptosis-regulated convertase1 (NARC-1): Liver regeneration and neuronal differentiation. Proc Natl Acad Sci U.S.A. (2003) 100:928–33. doi: 10.1073/pnas.0335507100
6. Cunningham D, Danley DE, Geoghegan KF, Griffor MC, Hawkins JL, Subashi TA, et al. Structural and biophysical studies of PCSK9 and its mutants linked to familial hypercholesterolemia. Nat Struct Mol Biol (2007) 14:413–9. doi: 10.1038/nsmb1235
7. Piper DE, Jackson S, Liu Q, Romanow WG, Shetterly S, Thibault ST, et al. The crystal structure of PCSK9: A regulator of plasma LDL-cholesterol. Structure (2007) 15:545–52. doi: 10.1016/j.str.2007.04.004
8. Varret M, Rabes JP, Saint-Jore B, Cenarro A, Marinoni JC, Civeira F, et al. A third major locus for autosomal dominant hypercholesterolemia maps to1p34.1-p32. Am J Hum Genet (1999) 64:1378–87. doi: 10.1086/302370
9. Hunt SC, Hopkins PN, Bulka K, McDermott MT, Thorne TL, Wardell BB, et al. Genetic localization to chromosome1p32 of the third locus for familial hypercholesterolemia in a Utah kindred. Arterioscler Thromb Vasc Biol (2000) 20:1089–93. doi: 10.1161/01.atv.20.4.1089
10. Maxwell KN, Soccio RE, Duncan EM, Sehayek E, Breslow JL. Novel putative SREBP and LXR target genes identified by microarray analysis in liver of cholesterol-fed mice. J Lipid Res (2003) 44:2109–19. doi: 10.1194/jlr.M300203-JLR200
11. Dubuc G, Chamberland A, Wassef H, Davignon J, Seidah NG, Bernier L, et al. Statins upregulate PCSK9, the gene encoding the proprotein convertase neural apoptosis-regulated convertase-1 implicated in familial hypercholesterolemia. Arterioscler Thromb Vasc Biol (2004) 24:1454–9. doi: 10.1161/01.ATV.0000134621.14315.43
12. Abifadel M, Varret M, Rabes JP, Allard D, Ouguerram K, Devillers M, et al. Mutations in PCSK9 cause autosomal dominant hypercholesterolemia. Nat Genet (2003) 34:154–6. doi: 10.1038/ng1161
13. Maxwell KN, Fisher EA, Breslow JL. Overexpression of PCSK9 accelerates the degradation of the LDLR in a post-endoplasmic reticulum compartment. Proc Natl Acad Sci U.S.A. (2005) 102:2069–74. doi: 10.1073/pnas.0409736102
14. Maxwell KN, Breslow JL. Adenoviral-mediated expression of Pcsk9 in mice results in a low-density lipoprotein receptor knockout phenotype. Proc Natl Acad Sci U.S.A. (2004) 101:7100–5. doi: 10.1073/pnas.0402133101
15. Alborn WE, Cao G, Careskey HE, Qian YW, Subramaniam DR, Davies J, et al. Serum proprotein convertase subtilisin kexin type9 is correlated directly with serum LDL cholesterol. Clin Chem (2007) 53:1814–9. doi: 10.1373/clinchem.2007.091280
16. Lambert G, Ancellin N, Charlton F, Comas D, Pilot J, Keech A, et al. Plasma PCSK9 concentrations correlate with LDL and total cholesterol in diabetic patients and are decreased by fenofibrate treatment. Clin Chem (2008) 54:1038–45. doi: 10.1373/clinchem.2007.099747
17. Cohen J, Pertsemlidis A, Kotowski IK, Graham R, Garcia CK, Hobbs HH. Low LDL cholesterol in individuals of African descent resulting from frequent nonsense mutations in PCSK9. Nat Genet (2005) 37:161–5. doi: 10.1038/ng1509
18. Cohen JC, Boerwinkle E, Mosley TJ, Hobbs HH. Sequence variations in PCSK9, low LDL, and protection against coronary heart disease. N Engl J Med (2006) 354:1264–72. doi: 10.1056/NEJMoa054013
19. Oyama K, Giugliano RP, Tang M, Bonaca MP, Saver JL, Murphy SA, et al. Effect of evolocumab on acute arterial events across all vascular territories: Results from the FOURIER trial. Eur Heart J (2021) 42:4821–9. doi: 10.1093/eurheartj/ehab604
20. Giugliano RP, Pedersen TR, Saver JL, Sever PS, Keech AC, Bohula EA, et al. Stroke prevention with the PCSK9 (Proprotein convertase Subtilisin-Kexin type9) inhibitor evolocumab added to statin in High-Risk patients with stable atherosclerosis. Stroke (2020) 51:1546–54. doi: 10.1161/STROKEAHA.119.027759
21. Rashid S, Curtis DE, Garuti R, Anderson NN, Bashmakov Y, Ho YK, et al. Decreased plasma cholesterol and hypersensitivity to statins in mice lacking Pcsk9. Proc Natl Acad Sci U.S.A. (2005) 102:5374–9. doi: 10.1073/pnas.0501652102
22. Zaid A, Roubtsova A, Essalmani R, Marcinkiewicz J, Chamberland A, Hamelin J, et al. Proprotein convertase subtilisin/kexin type9 (PCSK9): Hepatocyte-specific low-density lipoprotein receptor degradation and critical role in mouse liver regeneration. Hepatology (2008) 48:646–54. doi: 10.1002/hep.22354
23. Denis M, Marcinkiewicz J, Zaid A, Gauthier D, Poirier S, Lazure C, et al. Gene inactivation of proprotein convertase subtilisin/kexin type9 reduces atherosclerosis in mice. Circulation (2012) 125:894–901. doi: 10.1161/CIRCULATIONAHA.111.057406
24. Herbert B, Patel D, Waddington SN, Eden ER, McAleenan A, Sun XM, et al. Increased secretion of lipoproteins in transgenic mice expressing human D374Y PCSK9 under physiological genetic control. Arterioscler Thromb Vasc Biol (2010) 30:1333–9. doi: 10.1161/ATVBAHA.110.204040
25. Rousselet E, Marcinkiewicz J, Kriz J, Zhou A, Hatten ME, Prat A, et al. PCSK9 reduces the protein levels of the LDL receptor in mouse brain during development and after ischemic stroke. J Lipid Res (2011) 52:1383–91. doi: 10.1194/jlr.M014118
26. Roubtsova A, Munkonda MN, Awan Z, Marcinkiewicz J, Chamberland A, Lazure C, et al. Circulating proprotein convertase subtilisin/kexin 9 (PCSK9) regulates VLDLR protein and triglyceride accumulation in visceral adipose tissue. Arterioscler Thromb Vasc Biol (2011) 31:785–91. doi: 10.1161/ATVBAHA.110.220988
27. Demers A, Samami S, Lauzier B, Des Rosiers C, Ngo SE, Ong H, et al. PCSK9 induces CD36 degradation and affects Long-Chain fatty acid uptake and triglyceride metabolism in adipocytes and in mouse liver. Arterioscler Thromb Vasc Biol (2015) 35:2517–25. doi: 10.1161/ATVBAHA.115.306032
28. Roubtsova A, Chamberland A, Marcinkiewicz J, Essalmani R, Fazel A, Bergeron JJ, et al. PCSK9 deficiency unmasks a sex- and tissue-specific subcellular distribution of the LDL and VLDL receptors in mice. J Lipid Res (2015) 56:2133–42. doi: 10.1194/jlr.M061952
29. Tang ZH, Peng J, Ren Z, Yang J, Li TT, Li TH, et al. New role of PCSK9 in atherosclerotic inflammation promotion involving the TLR4/NF-kappaB pathway. Atherosclerosis (2017) 262:113–22. doi: 10.1016/j.atherosclerosis.2017.04.023
30. Roth EM, Taskinen MR, Ginsberg HN, Kastelein JJ, Colhoun HM, Robinson JG, et al. Monotherapy with the PCSK9 inhibitor alirocumab versus ezetimibe in patients with hypercholesterolemia: Results of a24 week, double-blind, randomized Phase3 trial. Int J Cardiol (2014) 176:55–61. doi: 10.1016/j.ijcard.2014.06.049
31. McKenney JM, Koren MJ, Kereiakes DJ, Hanotin C, Ferrand AC, Stein EA. Safety and efficacy of a monoclonal antibody to proprotein convertase subtilisin/kexin type9 serine protease, SAR236553/REGN727, in patients with primary hypercholesterolemia receiving ongoing stable atorvastatin therapy. J Am Coll Cardiol (2012) 59:2344–53. doi: 10.1016/j.jacc.2012.03.007
32. Roth EM, McKenney JM, Hanotin C, Asset G, Stein EA. Atorvastatin with or without an antibody to PCSK9 in primary hypercholesterolemia. N Engl J Med (2012) 367:1891–900. doi: 10.1056/NEJMoa1201832
33. Sabatine MS, Giugliano RP, Keech AC, Honarpour N, Wiviott SD, Murphy SA, et al. Evolocumab and clinical outcomes in patients with cardiovascular disease. N Engl J Med (2017) 376:1713–22. doi: 10.1056/NEJMoa1615664
34. Schwartz GG, Steg PG, Szarek M, Bhatt DL, Bittner VA, Diaz R, et al. Alirocumab and cardiovascular outcomes after acute coronary syndrome. N Engl J Med (2018) 379:2097–107. doi: 10.1056/NEJMoa1801174
35. Kastelein JJ, Hovingh GK, Langslet G, Baccara-Dinet MT, Gipe DA, Chaudhari U, et al. Efficacy and safety of the proprotein convertase subtilisin/kexin type9 monoclonal antibody alirocumab vs placebo in patients with heterozygous familial hypercholesterolemia. J Clin Lipidol (2017) 11:195–203. doi: 10.1016/j.jacl.2016.12.004
36. Fitzgerald K, White S, Borodovsky A, Bettencourt BR, Strahs A, Clausen V, et al. A highly durable RNAi therapeutic inhibitor of PCSK9. N Engl J Med (2017) 376:41–51. doi: 10.1056/NEJMoa1609243
37. Hardy J, Niman S, Pereira E, Lewis T, Reid J, Choksi R, et al. A critical review of the efficacy and safety of inclisiran. Am J Cardiovasc Drugs (2021) 21:629–42. doi: 10.1007/s40256-021-00477-7
38. Seidah NG, Prat A. The multifaceted biology of PCSK9. Endocr Rev (2022) 43:558–82. doi: 10.1210/endrev/bnab035
39. Chen C, Song M. Visualizing a field of research: A methodology of systematic scientometric reviews. PloS One (2019) 14:e223994. doi: 10.1371/journal.pone.0223994
40. Zhao Z, Tuakli-Wosornu Y, Lagace TA, Kinch L, Grishin NV, Horton JD, et al. Molecular characterization of loss-of-function mutations in PCSK9 and identification of a compound heterozygote. Am J Hum Genet (2006) 79:514–23. doi: 10.1086/507488
41. Chan JC, Piper DE, Cao Q, Liu D, King C, Wang W, et al. A proprotein convertase subtilisin/kexin type9 neutralizing antibody reduces serum cholesterol in mice and nonhuman primates. Proc Natl Acad Sci U.S.A. (2009) 106:9820–5. doi: 10.1073/pnas.0903849106
42. Ni YG, Condra JH, Orsatti L, Shen X, Di Marco S, Pandit S, et al. A proprotein convertase subtilisin-like/kexin type9 (PCSK9) C-terminal domain antibody antigen-binding fragment inhibits PCSK9 internalization and restores low density lipoprotein uptake. J Biol Chem (2010) 285:12882–91. doi: 10.1074/jbc.M110.113035
43. Frank-Kamenetsky M, Grefhorst A, Anderson NN, Racie TS, Bramlage B, Akinc A, et al. Therapeutic RNAi targeting PCSK9 acutely lowers plasma cholesterol in rodents and LDL cholesterol in nonhuman primates. Proc Natl Acad Sci U.S.A. (2008) 105:11915–20. doi: 10.1073/pnas.0805434105
44. Duff CJ, Scott MJ, Kirby IT, Hutchinson SE, Martin SL, Hooper NM. Antibody-mediated disruption of the interaction between PCSK9 and the low-density lipoprotein receptor. Biochem J (2009) 419:577–84. doi: 10.1042/BJ20082407
45. Zhang DW, Lagace TA, Garuti R, Zhao Z, McDonald M, Horton JD, et al. Binding of proprotein convertase subtilisin/kexin type9 to epidermal growth factor-like repeat a of low density lipoprotein receptor decreases receptor recycling and increases degradation. J Biol Chem (2007) 282:18602–12. doi: 10.1074/jbc.M702027200
46. Koren MJ, Scott R, Kim JB, Knusel B, Liu T, Lei L, et al. Efficacy, safety, and tolerability of a monoclonal antibody to proprotein convertase subtilisin/kexin type9 as monotherapy in patients with hypercholesterolaemia (MENDEL): A randomised, double-blind, placebo-controlled, phase2 study. Lancet (2012) 380:1995–2006. doi: 10.1016/S0140-6736(12)61771-1
47. Giugliano RP, Desai NR, Kohli P, Rogers WJ, Somaratne R, Huang F, et al. Efficacy, safety, and tolerability of a monoclonal antibody to proprotein convertase subtilisin/kexin type9 in combination with a statin in patients with hypercholesterolaemia (LAPLACE-TIMI57): A randomised, placebo-controlled, dose-ranging, phase2 study. Lancet (2012) 380:2007–17. doi: 10.1016/S0140-6736(12)61770-X
48. Sullivan D, Olsson AG, Scott R, Kim JB, Xue A, Gebski V, et al. Effect of a monoclonal antibody to PCSK9 on low-density lipoprotein cholesterol levels in statin-intolerant patients: The GAUSS randomized trial. JAMA (2012) 308:2497–506. doi: 10.1001/jama.2012.25790
49. Raal F, Scott R, Somaratne R, Bridges I, Li G, Wasserman SM, et al. Low-density lipoprotein cholesterol-lowering effects of AMG145, a monoclonal antibody to proprotein convertase subtilisin/kexin type9 serine protease in patients with heterozygous familial hypercholesterolemia: The Reduction of LDL-C with PCSK9 Inhibition in Heterozygous Familial Hypercholesterolemia Disorder (RUTHERFORD) randomized trial. Circulation (2012) 126:2408–17. doi: 10.1161/CIRCULATIONAHA.112.144055
50. Dias CS, Shaywitz AJ, Wasserman SM, Smith BP, Gao B, Stolman DS, et al. Effects of AMG145 on low-density lipoprotein cholesterol levels: Results from2 randomized, double-blind, placebo-controlled, ascending-dose phase1 studies in healthy volunteers and hypercholesterolemic subjects on statins. J Am Coll Cardiol (2012) 60:1888–98. doi: 10.1016/j.jacc.2012.08.986
51. Robinson JG, Farnier M, Krempf M, Bergeron J, Luc G, Averna M, et al. Efficacy and safety of alirocumab in reducing lipids and cardiovascular events. N Engl J Med (2015) 372:1489–99. doi: 10.1056/NEJMoa1501031
52. Sabatine MS, Giugliano RP, Wiviott SD, Raal FJ, Blom DJ, Robinson J, et al. Efficacy and safety of evolocumab in reducing lipids and cardiovascular events. N Engl J Med (2015) 372:1500–9. doi: 10.1056/NEJMoa1500858
53. Raal FJ, Stein EA, Dufour R, Turner T, Civeira F, Burgess L, et al. PCSK9 inhibition with evolocumab (AMG145) in heterozygous familial hypercholesterolaemia (RUTHERFORD-2): A randomised, double-blind, placebo-controlled trial. Lancet (2015) 385:331–40. doi: 10.1016/S0140-6736(14)61399-4
54. Ray KK, Wright RS, Kallend D, Koenig W, Leiter LA, Raal FJ, et al. Two phase3 trials of inclisiran in patients with elevated LDL cholesterol. N Engl J Med (2020) 382:1507–19. doi: 10.1056/NEJMoa1912387
55. Oelrich B, Peters R, Jung K. A bibliometric evaluation of publications in urological journals among European Union countries between2000-2005. Eur Urol (2007) 52:1238–48. doi: 10.1016/j.eururo.2007.06.050
56. He Z, Dai L, Zuo Y, Chen Y, Wang H, Zeng H. Hotspots and frontiers in pulmonary arterial hypertension research: A bibliometric and visualization analysis from2011 to2020. Bioengineered (2022) 13:14667–80. doi: 10.1080/21655979.2022.2100064
57. Lo SP, Bottomley MJ, Calzetta A, Settembre EC, Cirillo A, Pandit S, et al. Mechanistic implications for LDL receptor degradation from the PCSK9/LDLR structure at neutral pH. EMBO Rep (2011) 12:1300–5. doi: 10.1038/embor.2011.205
58. Lagace TA, Curtis DE, Garuti R, McNutt MC, Park SW, Prather HB, et al. Secreted PCSK9 decreases the number of LDL receptors in hepatocytes and in livers of parabiotic mice. J Clin Invest (2006) 116:2995–3005. doi: 10.1172/JCI29383
59. Poirier S, Mayer G, Poupon V, McPherson PS, Desjardins R, Ly K, et al. Dissection of the endogenous cellular pathways of PCSK9-induced low density lipoprotein receptor degradation: Evidence for an intracellular route. J Biol Chem (2009) 284:28856–64. doi: 10.1074/jbc.M109.037085
60. He G, Gupta S, Yi M, Michaely P, Hobbs HH, Cohen JC. ARH is a modular adaptor protein that interacts with the LDL receptor, clathrin, and AP-2. J Biol Chem (2002) 277:44044–9. doi: 10.1074/jbc.M208539200
61. Garcia CK, Wilund K, Arca M, Zuliani G, Fellin R, Maioli M, et al. Autosomal recessive hypercholesterolemia caused by mutations in a putative LDL receptor adaptor protein. Science (2001) 292:1394–8. doi: 10.1126/science.1060458
62. Park SW, Moon YA, Horton JD. Post-transcriptional regulation of low density lipoprotein receptor protein by proprotein convertase subtilisin/kexin type9a in mouse liver. J Biol Chem (2004) 279:50630–8. doi: 10.1074/jbc.M410077200
63. Zhang DW, Garuti R, Tang WJ, Cohen JC, Hobbs HH. Structural requirements for PCSK9-mediated degradation of the low-density lipoprotein receptor. Proc Natl Acad Sci U.S.A. (2008) 105:13045–50. doi: 10.1073/pnas.0806312105
64. Saavedra YG, Day R, Seidah NG. The M2 module of the Cys-His-rich domain (CHRD) of PCSK9 protein is needed for the extracellular low-density lipoprotein receptor (LDLR) degradation pathway. J Biol Chem (2012) 287:43492–501. doi: 10.1074/jbc.M112.394023
65. Holla OL, Cameron J, Tveten K, Strom TB, Berge KE, Laerdahl JK, et al. Role of the C-terminal domain of PCSK9 in degradation of the LDL receptors. J Lipid Res (2011) 52:1787–94. doi: 10.1194/jlr.M018093
66. Nassoury N, Blasiole DA, Tebon OA, Benjannet S, Hamelin J, Poupon V, et al. The cellular trafficking of the secretory proprotein convertase PCSK9 and its dependence on the LDLR. Traffic (2007) 8:718–32. doi: 10.1111/j.1600-0854.2007.00562.x
67. Strom TB, Holla OL, Tveten K, Cameron J, Berge KE, Leren TP. Disrupted recycling of the low density lipoprotein receptor by PCSK9 is not mediated by residues of the cytoplasmic domain. Mol Genet Metab (2010) 101:76–80. doi: 10.1016/j.ymgme.2010.05.003
68. Jang HD, Lee SE, Yang J, Lee HC, Shin D, Lee H, et al. Cyclase-associated protein1 is a binding partner of proprotein convertase subtilisin/kexin type-9 and is required for the degradation of low-density lipoprotein receptors by proprotein convertase subtilisin/kexin type-9. Eur Heart J (2020) 41:239–52. doi: 10.1093/eurheartj/ehz566
69. Stein EA, Mellis S, Yancopoulos GD, Stahl N, Logan D, Smith WB, et al. Effect of a monoclonal antibody to PCSK9 on LDL cholesterol. N Engl J Med (2012) 366:1108–18. doi: 10.1056/NEJMoa1105803
70. Stein EA, Gipe D, Bergeron J, Gaudet D, Weiss R, Dufour R, et al. Effect of a monoclonal antibody to PCSK9, REGN727/SAR236553, to reduce low-density lipoprotein cholesterol in patients with heterozygous familial hypercholesterolaemia on stable statin dose with or without ezetimibe therapy: A phase2 randomised controlled trial. Lancet (2012) 380:29–36. doi: 10.1016/S0140-6736(12)60771-5
71. Stroes E, Colquhoun D, Sullivan D, Civeira F, Rosenson RS, Watts GF, et al. Anti-PCSK9 antibody effectively lowers cholesterol in patients with statin intolerance: The GAUSS-2 randomized, placebo-controlled phase3 clinical trial of evolocumab. J Am Coll Cardiol (2014) 63:2541–8. doi: 10.1016/j.jacc.2014.03.019
72. Nissen SE, Stroes E, Dent-Acosta RE, Rosenson RS, Lehman SJ, Sattar N, et al. Efficacy and tolerability of evolocumab vs ezetimibe in patients with Muscle-Related statin intolerance: The GAUSS-3 randomized clinical trial. JAMA (2016) 315:1580–90. doi: 10.1001/jama.2016.3608
73. Stein EA, Honarpour N, Wasserman SM, Xu F, Scott R, Raal FJ. Effect of the proprotein convertase subtilisin/kexin9 monoclonal antibody, AMG145, in homozygous familial hypercholesterolemia. Circulation (2013) 128:2113–20. doi: 10.1161/CIRCULATIONAHA.113.004678
74. Thedrez A, Blom DJ, Ramin-Mangata S, Blanchard V, Croyal M, Chemello K, et al. Homozygous familial hypercholesterolemia patients with identical mutations variably express the LDLR (Low-Density lipoprotein receptor): Implications for the efficacy of evolocumab. Arterioscler Thromb Vasc Biol (2018) 38:592–8. doi: 10.1161/ATVBAHA.117.310217
75. Raal FJ, Hovingh GK, Blom D, Santos RD, Harada-Shiba M, Bruckert E, et al. Long-term treatment with evolocumab added to conventional drug therapy, with or without apheresis, in patients with homozygous familial hypercholesterolaemia: An interim subset analysis of the open-label TAUSSIG study. Lancet Diabetes Endocrinol (2017) 5:280–90. doi: 10.1016/S2213-8587(17)30044-X
76. Raal FJ, Honarpour N, Blom DJ, Hovingh GK, Xu F, Scott R, et al. Inhibition of PCSK9 with evolocumab in homozygous familial hypercholesterolaemia (TESLA Part B): A randomised, double-blind, placebo-controlled trial. Lancet (2015) 385:341–50. doi: 10.1016/S0140-6736(14)61374-X
77. Nicholls SJ, Puri R, Anderson T, Ballantyne CM, Cho L, Kastelein JJ, et al. Effect of evolocumab on progression of coronary disease in Statin-Treated patients: The GLAGOV randomized clinical trial. JAMA (2016) 316:2373–84. doi: 10.1001/jama.2016.16951
78. Nicholls SJ, Kataoka Y, Nissen SE, Prati F, Windecker S, Puri R, et al. Effect of evolocumab on coronary plaque phenotype and burden in Statin-Treated patients following myocardial infarction. JACC Cardiovasc Imaging (2022) 15:1308–21. doi: 10.1016/j.jcmg.2022.03.002
79. Raber L, Ueki Y, Otsuka T, Losdat S, Haner JD, Lonborg J, et al. Effect of alirocumab added to High-Intensity statin therapy on coronary atherosclerosis in patients with acute myocardial infarction: The PACMAN-AMI randomized clinical trial. JAMA (2022) 327:1771–81. doi: 10.1001/jama.2022.5218
80. O'Donoghue ML, Giugliano RP, Wiviott SD, Atar D, Keech A, Kuder JF, et al. Long-Term evolocumab in patients with established atherosclerotic cardiovascular disease. Circulation (2022) 146:1109–19. doi: 10.1161/CIRCULATIONAHA.122.061620
81. Zhang Y, Zhu CG, Xu RX, Li S, Guo YL, Sun J, et al. Relation of circulating PCSK9 concentration to fibrinogen in patients with stable coronary artery disease. J Clin Lipidol (2014) 8:494–500. doi: 10.1016/j.jacl.2014.07.001
82. Li S, Zhang Y, Xu RX, Guo YL, Zhu CG, Wu NQ, et al. Proprotein convertase subtilisin-kexin type9 as a biomarker for the severity of coronary artery disease. Ann Med (2015) 47:386–93. doi: 10.3109/07853890.2015.1042908
83. Sun HL, Wu YR, Song FF, Gan J, Huang LY, Zhang L, et al. Role of PCSK9 in the development of mouse periodontitis before and after treatment: A Double-Edged sword. J Infect Dis (2018) 217:667–80. doi: 10.1093/infdis/jix574
84. Ding Z, Liu S, Wang X, Deng X, Fan Y, Shahanawaz J, et al. Cross-talk between LOX-1 and PCSK9 in vascular tissues. Cardiovasc Res (2015) 107:556–67. doi: 10.1093/cvr/cvv178
85. Xie W, Liu J, Wang W, Wang M, Qi Y, Zhao F, et al. Association between plasma PCSK9 levels and10-year progression of carotid atherosclerosis beyond LDL-C: A cohort study. Int J Cardiol (2016) 215:293–8. doi: 10.1016/j.ijcard.2016.04.103
86. Giunzioni I, Tavori H, Covarrubias R, Major AS, Ding L, Zhang Y, et al. Local effects of human PCSK9 on the atherosclerotic lesion. J Pathol (2016) 238:52–62. doi: 10.1002/path.4630
87. Landlinger C, Pouwer MG, Juno C, van der Hoorn J, Pieterman EJ, Jukema JW, et al. The AT04A vaccine against proprotein convertase subtilisin/kexin type9 reduces total cholesterol, vascular inflammation, and atherosclerosis in APOE*3Leiden.CETP mice. Eur Heart J (2017) 38:2499–507. doi: 10.1093/eurheartj/ehx260
88. Vlachopoulos C, Koutagiar I, Skoumas I, Terentes-Printzios D, Zacharis E, Kolovou G, et al. Long-Term administration of proprotein convertase Subtilisin/Kexin type9 inhibitors reduces arterial FDG uptake. JACC Cardiovasc Imaging (2019) 12:2573–4. doi: 10.1016/j.jcmg.2019.09.024
89. Bohula EA, Giugliano RP, Leiter LA, Verma S, Park JG, Sever PS, et al. Inflammatory and cholesterol risk in the FOURIER trial. Circulation (2018) 138:131–40. doi: 10.1161/CIRCULATIONAHA.118.034032
90. Leucker TM, Gerstenblith G, Schar M, Brown TT, Jones SR, Afework Y, et al. Evolocumab, a PCSK9-Monoclonal antibody, rapidly reverses coronary artery endothelial dysfunction in people living with HIV and people with dyslipidemia. J Am Heart Assoc (2020) 9:e16263. doi: 10.1161/JAHA.120.016263
91. Cannon CP, Cariou B, Blom D, McKenney JM, Lorenzato C, Pordy R, et al. Efficacy and safety of alirocumab in high cardiovascular risk patients with inadequately controlled hypercholesterolaemia on maximally tolerated doses of statins: The ODYSSEY COMBO II randomized controlled trial. Eur Heart J (2015) 36:1186–94. doi: 10.1093/eurheartj/ehv028
92. Mehta P, McAuley DF, Brown M, Sanchez E, Tattersall RS, Manson JJ. COVID-19: Consider cytokine storm syndromes and immunosuppression. Lancet (2020) 395:1033–4. doi: 10.1016/S0140-6736(20)30628-0
93. Bikdeli B, Madhavan MV, Jimenez D, Chuich T, Dreyfus I, Driggin E, et al. COVID-19 and thrombotic or thromboembolic disease: Implications for prevention, antithrombotic therapy, and Follow-Up: JACC State-of-the-Art review. J Am Coll Cardiol (2020) 75:2950–73. doi: 10.1016/j.jacc.2020.04.031
94. Sorensen KE, Celermajer DS, Georgakopoulos D, Hatcher G, Betteridge DJ, Deanfield JE. Impairment of endothelium-dependent dilation is an early event in children with familial hypercholesterolemia and is related to the lipoprotein(a) level. J Clin Invest (1994) 93:50–5. doi: 10.1172/JCI116983
95. Vuorio A, Watts GF, Schneider WJ, Tsimikas S, Kovanen PT. Familial hypercholesterolemia and elevated lipoprotein(a): Double heritable risk and new therapeutic opportunities. J Intern Med (2020) 287:2–18. doi: 10.1111/joim.12981
96. Vuorio A, Kovanen PT. Prevention of endothelial dysfunction and thrombotic events in COVID-19 patients with familial hypercholesterolemia. J Clin Lipidol (2020) 14:617–8. doi: 10.1016/j.jacl.2020.06.006
97. Kow CS, Hasan SS. Meta-analysis of effect of statins in patients with COVID-19. Am J Cardiol (2020) 134:153–5. doi: 10.1016/j.amjcard.2020.08.004
98. Momtazi-Borojeni AA, Sabouri-Rad S, Gotto AM, Pirro M, Banach M, Awan Z, et al. PCSK9 and inflammation: A review of experimental and clinical evidence. Eur Heart J Cardiovasc Pharmacother (2019) 5:237–45. doi: 10.1093/ehjcvp/pvz022
99. Walley KR, Thain KR, Russell JA, Reilly MP, Meyer NJ, Ferguson JF, et al. PCSK9 is a critical regulator of the innate immune response and septic shock outcome. Sci Transl Med (2014) 6:143r–258r. doi: 10.1126/scitranslmed.3008782
100. Genga KR, Lo C, Cirstea MS, Leitao FF, Walley KR, Russell JA, et al. Impact of PCSK9 loss-of-function genotype on1-year mortality and recurrent infection in sepsis survivors. Ebiomedicine (2018) 38:257–64. doi: 10.1016/j.ebiom.2018.11.032
101. Navarese EP, Podhajski P, Gurbel PA, Grzelakowska K, Ruscio E, Tantry U, et al. PCSK9 inhibition during the inflammatory stage of SARS-CoV-2 infection. J Am Coll Cardiol (2023) 81:224–34. doi: 10.1016/j.jacc.2022.10.030
102. Sabaka P, Koscalova A, Straka I, Hodosy J, Liptak R, Kmotorkova B, et al. Role of interleukin6 as a predictive factor for a severe course of Covid-19: Retrospective data analysis of patients from a long-term care facility during Covid-19 outbreak. BMC Infect Dis (2021) 21:308. doi: 10.1186/s12879-021-05945-8
103. Mester P, Amend P, Schmid S, Muller M, Buechler C, Pavel V. Plasma proprotein convertase Subtilisin/Kexin type9 (PCSK9) as a possible biomarker for severe COVID-19. Viruses (2023) 15:1511. doi: 10.3390/v15071511
104. Vuorio A, Kovanen PT. PCSK9 inhibitors for COVID-19: An opportunity to enhance the antiviral action of interferon in patients with hypercholesterolaemia. J Intern Med (2021) 289:749–51. doi: 10.1111/joim.13210
105. Gan ES, Tan HC, Le DHT, Huynh TT, Wills B, Seidah NG, et al. Dengue virus induces PCSK9 expression to alter antiviral responses and disease outcomes. J Clin Invest (2020) 130:5223–34. doi: 10.1172/JCI137536
106. Li Z, Liu Q. Proprotein convertase subtilisin/kexin type9 inhibits interferon beta expression through interacting with ATF-2. FEBS Lett (2018) 592:2323–33. doi: 10.1002/1873-3468.13152
107. Goonewardena SN, Rosenson RS. PCSK9: The nexus of lipoprotein metabolism and inflammation in COVID-19. J Am Coll Cardiol (2023) 81:235–6. doi: 10.1016/j.jacc.2022.11.014
108. King RJ, Singh PK, Mehla K. The cholesterol pathway: Impact on immunity and cancer. Trends Immunol (2022) 43:78–92. doi: 10.1016/j.it.2021.11.007
109. Cruz PM, Mo H, McConathy WJ, Sabnis N, Lacko AG. The role of cholesterol metabolism and cholesterol transport in carcinogenesis: A review of scientific findings, relevant to future cancer therapeutics. Front Pharmacol (2013) 4:119. doi: 10.3389/fphar.2013.00119
110. Sun X, Essalmani R, Day R, Khatib AM, Seidah NG, Prat A. Proprotein convertase subtilisin/kexin type9 deficiency reduces melanoma metastasis in liver. Neoplasia (2012) 14:1122–31. doi: 10.1593/neo.121252
111. Nowak C, Arnlov J. A Mendelian randomization study of the effects of blood lipids on breast cancer risk. Nat Commun (2018) 9:3957. doi: 10.1038/s41467-018-06467-9
112. Liu X, Bao X, Hu M, Chang H, Jiao M, Cheng J, et al. Inhibition of PCSK9 potentiates immune checkpoint therapy for cancer. Nature (2020) 588:693–8. doi: 10.1038/s41586-020-2911-7
113. Yuan J, Cai T, Zheng X, Ren Y, Qi J, Lu X, et al. Potentiating CD8(+) T cell antitumor activity by inhibiting PCSK9 to promote LDLR-mediated TCR recycling and signaling. Protein Cell (2021) 12:240–60. doi: 10.1007/s13238-021-00821-2
114. Bays HE, Rosenson RS, Baccara-Dinet MT, Louie MJ, Thompson D, Hovingh GK. Assessment of the1% of Patients with Consistent <15% Reduction in Low-Density Lipoprotein Cholesterol: Pooled Analysis of10 Phase3 ODYSSEY Alirocumab Trials. Cardiovasc Drugs Ther (2018) 32:175–80. doi: 10.1007/s10557-018-6784-z
115. Saeed A, Virani SS, Jones PH, Ballantyne CM, Nambi V. Case reports of proprotein convertase subtilisin/kexin type9 (PCSK9) inhibition nonresponse. J Clin Lipidol (2018) 12:1141–5. doi: 10.1016/j.jacl.2018.05.017
116. Ouyang M, Li C, Hu D, Peng D, Yu B. Mechanisms of unusual response to lipid-lowering therapy: PCSK9 inhibition. Clin Chim Acta (2023) 538:113–23. doi: 10.1016/j.cca.2022.11.018
117. Johns DG, Campeau LC, Banka P, Bautmans A, Bueters T, Bianchi E, et al. Orally bioavailable macrocyclic peptide that inhibits binding of PCSK9 to the low density lipoprotein receptor. Circulation (2023) 148:144–58. doi: 10.1161/CIRCULATIONAHA.122.063372
118. Raal FJ, Kallend D, Ray KK, Turner T, Koenig W, Wright RS, et al. Inclisiran for the treatment of heterozygous familial hypercholesterolemia. N Engl J Med (2020) 382:1520–30. doi: 10.1056/NEJMoa1913805
119. Rosoff DB, Bell AS, Jung J, Wagner J, Mavromatis LA, Lohoff FW. Mendelian randomization study of PCSK9 and HMG-CoA reductase inhibition and cognitive function. J Am Coll Cardiol (2022) 80:653–62. doi: 10.1016/j.jacc.2022.05.041
120. Huang W, Xiao J, Ji J, Chen L. Association of lipid-lowering drugs with COVID-19 outcomes from a Mendelian randomization study. Elife (2021) 10:e73873s. doi: 10.7554/eLife.73873
121. Sun L, Ding H, Jia Y, Shi M, Guo D, Yang P, et al. Associations of genetically proxied inhibition of HMG-CoA reductase, NPC1L1, and PCSK9 with breast cancer and prostate cancer. Breast Cancer Res (2022) 24:12. doi: 10.1186/s13058-022-01508-0
122. Chen ZM, Gu HQ, Mo JL, Yang KX, Jiang YY, Yang X, et al. U-shaped association between low-density lipoprotein cholesterol levels and risk of all-cause mortality mediated by post-stroke infection in acute ischemic stroke. Sci Bull (Beijing) (2023) 68:1327–35. doi: 10.1016/j.scib.2023.05.028
Keywords: PCSK9 inhibitors, cardiovascular endocrinology, bibliometric analysis, data visualization, trend, hotspots
Citation: Luo Q, Tang Z, Wu P, Chen Z, Fang Z and Luo F (2023) A bibliometric analysis of PCSK9 inhibitors from 2007 to 2022. Front. Endocrinol. 14:1218968. doi: 10.3389/fendo.2023.1218968
Received: 08 May 2023; Accepted: 16 November 2023;
Published: 29 November 2023.
Edited by:
Kanwal Rehman, The Women University, PakistanReviewed by:
Nhat Tu Le, Houston Methodist Research Institute, United StatesDa-wei Zhang, University of Alberta, Canada
Copyright © 2023 Luo, Tang, Wu, Chen, Fang and Luo. This is an open-access article distributed under the terms of the Creative Commons Attribution License (CC BY). The use, distribution or reproduction in other forums is permitted, provided the original author(s) and the copyright owner(s) are credited and that the original publication in this journal is cited, in accordance with accepted academic practice. No use, distribution or reproduction is permitted which does not comply with these terms.
*Correspondence: Fei Luo, luofei0058@csu.edu.cn
†These authors have contributed equally to this work and share first authorship