- 1Department of Orthopaedics, Honghui Hospital, Xi’an Jiao Tong University, Xi’an, China
- 2Department of Orthopaedics, The Fifth Affiliated Hospital of Sun Yat-Sen University, Zhuhai, Guangdong, China
Introduction: The causal association between non-alcoholic fatty liver disease (NAFLD) and osteoporosis remains controversial in previous epidemiological studies. We employed a bidirectional two-sample Mendelian analysis to explore the causal relationship between NAFLD and osteoporosis.
Method: The NAFLD instrumental variables (IVs) were obtained from a large Genome-wide association study (GWAS) meta-analysis dataset of European descent. Two-sample Mendelian randomization (MR) analyses were used to estimate the causal effect of NAFLD on osteoporosis, fracture, and fall. Reverse Mendelian randomization analysis was conducted to estimate the causal effect of osteoporosis on NAFLD. The inverse-variance weighted (IVW) method was the primary analysis in this analysis. We used the MR-Egger method to determine horizontal pleiotropic. The heterogeneity effect of IVs was detected by MR-Egger and IVW analyses.
Results: Five SNPs (rs2980854, rs429358, rs1040196, rs738409, and rs5764430) were chosen as IVs for NAFLD. In forward MR analysis, the IVW-random effect indicated the causal effect of NAFLD on osteoporosis (OR= 1.0021, 95% CI: 1.0006-1.0037, P= 0.007) but not on fracture (OR= 1.0016, 95% CI: 0.998-1.0053, P= 0.389) and fall (OR= 0.9912, 95% CI: 0.9412-1.0440, P= 0.740). Furthermore, the reverse Mendelian randomization did not support a causal effect of osteoporosis on NAFLD (OR= 1.0002, 95% CI: 0.9997-1.0007, P= 0.231). No horizontal pleiotropic was detected in all MR analyses.
Conclusions: The results of this study indicate a causal association between NAFLD and osteoporosis. NAFLD patients have a higher risk of osteoporosis but not fracture and falling risk. In addition, our results do not support a causal effect of osteoporosis on NAFLD.
Introduction
Osteoporosis (OP) is a bone disorder featured by low bone mass density (BMD) and impaired microarchitecture, leading to a higher fragility fracture risk for the old (1). In a meta-analysis, the global prevalence of osteoporosis in people aged 50-59, 60-69, and 70-79 was 11.4%, 24.8, and 37.6%, respectively (2). In 2013, twenty-two million females and 5.5 million males in European Union (EU) countries suffered from osteoporosis, leading to 3.5 million fragility fractures annually (3). Osteoporosis is a multifactorial disease involving clinical, nutritional, behavioral, medical, and genetic factors in their occurrence (4). Imbalance in bone formation and resorption due to various reasons, such as reduced estrogen levels in postmenopausal women or advanced age, will lead to osteoporosis (5). Previously, gender, age, family osteoporosis history, alcohol consumption, smoking, hormone use, some diseases, and others were considered possible risk factors for osteoporosis (6, 7).
Non-alcoholic fatty liver disease (NAFLD) has become the most common chronic liver disease, threatening about 25% population worldwide (8). With the epidemic of the western diet, sedentary lifestyle, and obesity, the prevalence of NAFLD in the general population has been increasing in recent years (9). NAFLD spectrum includes non-alcoholic fatty liver (NAFL), non-alcoholic steatohepatitis (NASH), and NASH-related end-stage liver disease, such as cirrhosis. Insulin resistance, type 2 diabetes (T2D), hyperlipidemia, hypertension, and metabolic syndrome may play an essential role in the development of NAFLD (10). In previous studies, a large number of studies have demonstrated the possible role of NAFLD in the development of osteoporosis. Studies showed that the prevalence of osteoporosis in NAFLD patients is higher than in patients without NAFLD (11). NAFLD may affect osteoporosis or osteoporotic fractures in several ways, including the changes in bone metabolic transforming factors, vitamin D levels, the chronic inflammatory state of the liver, degree of hepatic fibrosis, and disturbances in lipid metabolism, and others. However, previous observational studies investigating the association between NAFLD and osteoporosis have consistently yielded inconsistent results. Some studies have shown that NAFLD is associated with lower BMD or higher fracture risk in adolescents or adults (12–14), while others have shown no significant correlation or even opposite results (15–17). These observational studies have obvious limitations like unmeasured or imprecisely measured confounders such as gender, age, menstrual status, and others, which inevitably lead to some biases.
Mendelian randomization (MR) is a novel genetic variation method to assess the causal relationship between exposure and outcome (18). It could avoid confounding factors and infer causality since the alleles of exposure genetic variants are randomly assigned (19). Thus, we employed an MR analysis to explore the causal effect of NAFLD on osteoporosis, fracture, and falling risk. A reverse MR analysis was also used to explore the causal effect of osteoporosis on NAFLD.
Materials and methods
Study design
The MR analyses conform to three assumptions: 1. Selected SNPs should be strongly correlated with exposure. 2. Selected SNPs are not related to the outcome through confounders. 3. Selected SNPs are supposed to affect outcomes via exposure, but not the direct association (Figure 1). All information in this study was obtained from public databases or existing publications, which did not need additional ethical approval.
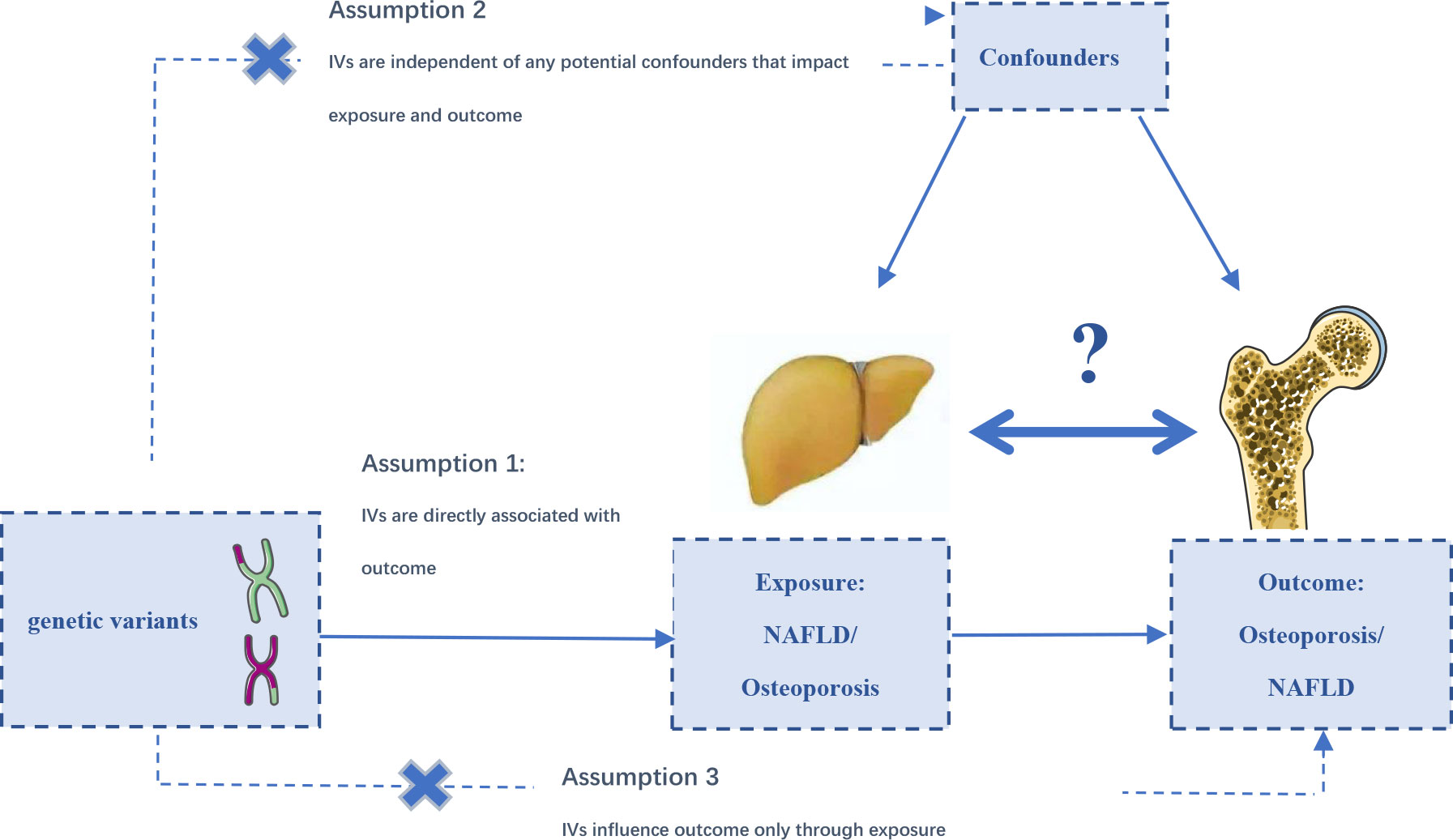
Figure 1 The designs and assumptions for the bidirectional Mendelian randomization study of the causal association of NAFLD and osteoporosis, fracture, and falling risk.
Data sources for NAFLD
The sources of NAFLD were obtained from large GWASs by Ghodsian et al. (20). The GWASs meta-analysis included summary statistics from the FinnGen cohorts eMERGE, the latest GWAS conducted by the Estonian Biobank (4,119 cases and 190,120 controls), and a new NAFLD GWAS from the UK Biobank (2,558 cases and 395,241 controls), with a total of 8,434 NAFLD cases and 770,180 controls. In this GWAS meta-analysis, the associations were conducted by adjusting for gender, age, and ten main ancestry-based principal components and genotyping (20). The electronic health record documented NAFLD with participants of European ancestry with hepatic steatosis, NASH, or liver fibrosis (LF). Finally, five SNPs (rs2980854, rs429358, rs10401969, rs738409, rs5764430) closely associated with NAFLD (p< 5×10-08) without linkage were chosen as alternative instruments for NAFLD (R2<0.001, window size =10,000 kb).
Data sources for osteoporosis, fractures, and fall
Summary-level genetic data on osteoporosis were collected from the UK Biobank (UK Biobank: 20002#1309), including 7,547 osteoporosis samples and 455,386 control samples. The genetic associations with fracture risk were revealed in a large GWAS meta-analysis combining 23andMe cohorts and the UK Biobank, with a total of 1.2 million subjects (21). The fracture diagnosis was defined as a break in bone continuity at any site in the past five years, except for fractures at hands, feet, skull, or pathological fractures due to malignancy, infection, and others (21). GWAS data for falling susceptibility were collected from a UK Biobank study, involving 89,076 fall cases and 362,103 controls (22). A “fall” is defined when the subject gives an affirmative answer to the following question: “Have you fallen in the last year?
Statistical analysis
The bidirectional MR approach was conducted to estimate the causality between NAFLD and osteoporosis, fracture, and fall. Relevant SNPs were selected when genome-wide significance was less than p< 5×10-8. SNPs were excluded when detecting linkage disequilibrium (LD) (R2 > 0.001 or clump distance< 10,000 kb). Five methods were employed to examine the causal association, including inverse-variance weighted (IVW), MR-Egger, weighted mode, weighted median, and simple model, among which IVW was the primary method (23). Random-effects IVW was performed to assess the genetic predictions between them. The MR-Egger method was used to test its horizontal pleiotropic. Outlier SNPs were detected by MR-PRESSO packages and then deleted. Cochran’s Q statistic was applied to examine the heterogeneity of individual SNPs in IVW and MR-Egger tests. We additionally performed sensitivity analysis by removing single SNP one by one. To evaluate the weak instrument bias, we calculated the F statistics using the formula , where N is the sample size, K is the number of IVs, and R2 is the proportion of the variability of the exposure explained by IVs. All data were analyzed by package “TwoSampleMR” of the R language.
Results
Genetical prediction of NAFLD on osteoporosis
Five SNPs (rs2980854 in/near TRIB1, rs429358 in/near APOE, rs10401969 in/near SUGP1, rs738409 in/near PNPLA3, rs5764430 in/near SAMM50) were chosen as IVs for NAFLD (Supplementary Table 1). The SNP was larger than the empirical threshold 10 of the F statistics, indicating a weak bias of the IVs. The causal association of NAFLD variants with osteoporosis was shown in Table 1 and Figure 2. The IVW results found a positive effect of NAFLD on osteoporosis (OR= 1.0021, 95% CI: 1.0006-1.0037, P= 0.007). The consistent result was also found in MR Egger (OR= 1.0041, 95% CI: 1.0003-1.0087, P= 0.012), weighted median (OR= 1.0022, 95% CI: 1.0004-1.0040, P= 0.015), and weighted mode (OR= 1.0023, 95% CI: 1.0002-1.0045, P= 0.024). The Cochran’s Q statistic of MR-Egger (P= 0.972) and IVW methods (P= 0.831) indicated no significant heterogeneity between IVs. No horizontal pleiotropy was detected in MR–Egger intercept (P= 0.346). In the leave-one-out sensitive analyses, we found rs738409 strongly drove the overall effect of NAFLD on osteoporosis (Figure 2C). Furthermore, the MR-PRESSO test did not identify any potential SNP outliers.
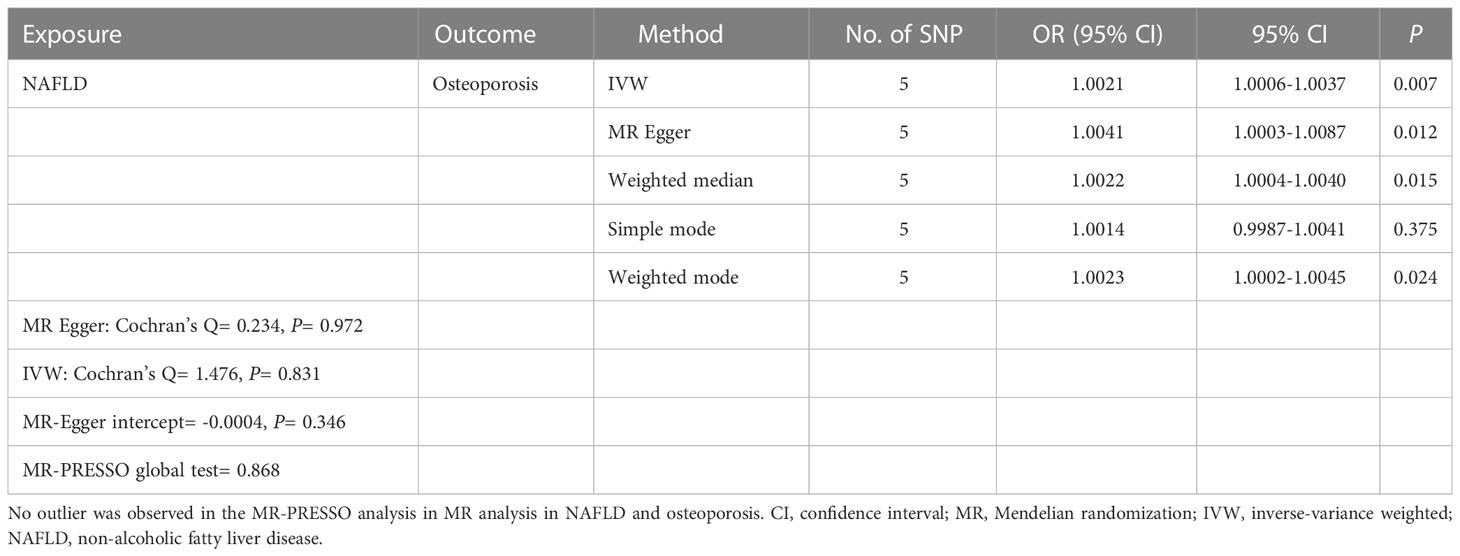
Table 1 Causal effect of NAFLD on osteoporosis using genetic variant randomization in different MR methods.
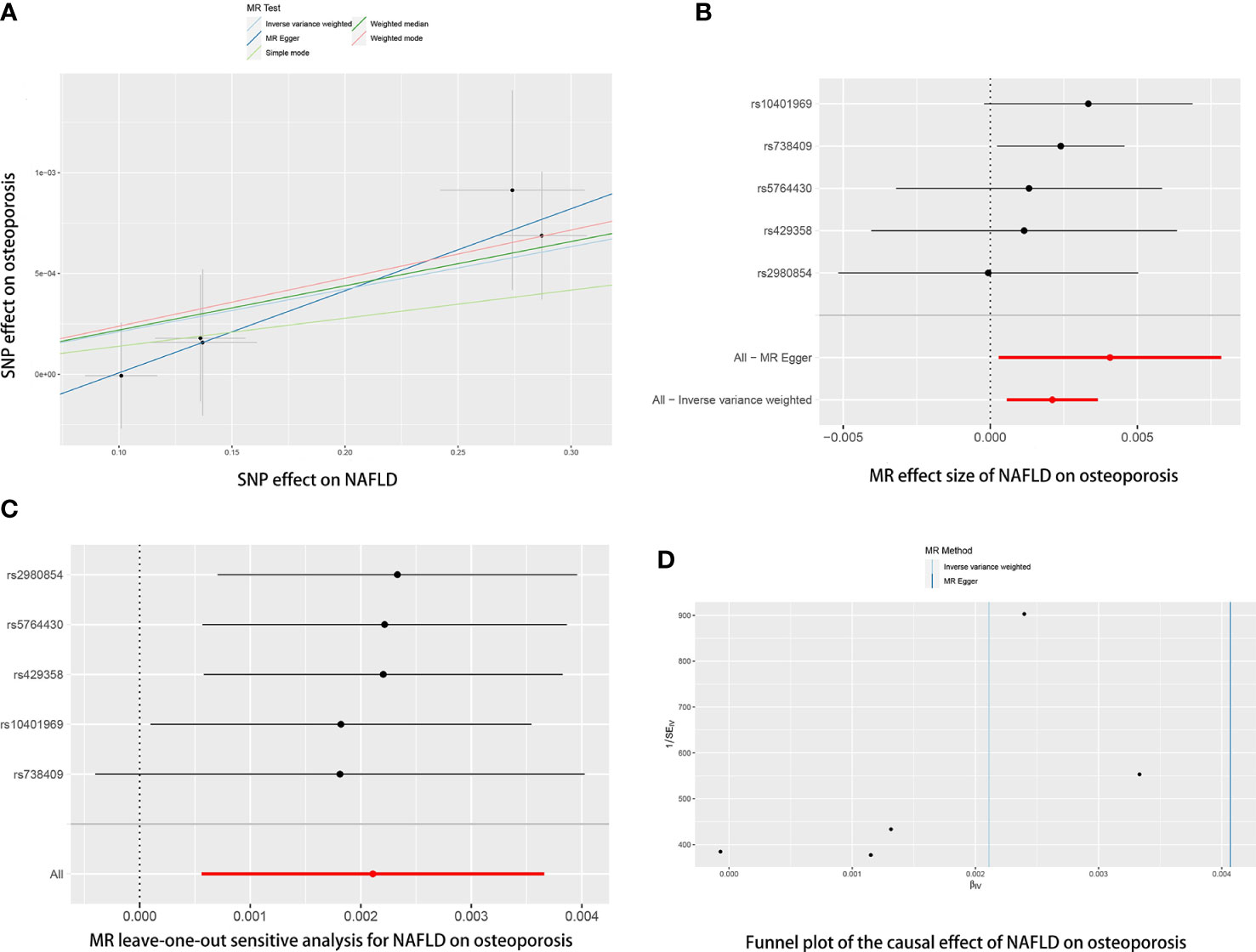
Figure 2 The causal effects of NAFLD on osteoporosis in different MR methods. (A) Scatter plot. The slopes of each line represent the causal association for each method. (B) Forrest plot. (C) Leave-one-out analysis. (D) Funnel plot.
Genetical prediction of NAFLD on fracture risk
The causal association of NAFLD variants with fracture was shown in Table 2 and Figure 3. MR analysis by the IVW method showed that there was no causal effect of NAFLD on fracture (OR= 1.0016, 95% CI: 0.998-1.0053, P= 0.389). The consistent result was also found in MR Egger (OR= 1.0057, 95% CI: 0.9969-1.0147, P= 0.295), weighted median (OR= 1.0012, 95% CI: 0.9970-1.0055, P= 0.581), simple mode (OR= 1.0029, 95% CI: 0.9961-1.0098, P= 0.448) and weighted mode (OR= 1.0013, 95% CI: 0.9963-1.0064, P= 0.632). The Cochran’s Q statistic of MR-Egger (P= 0.396) and IVW methods (P= 0.411) indicated no significant heterogeneity between IVs. No horizontal pleiotropy was detected in MR–Egger intercept (P= 0.391). In addition, the result remains no significant after excluding a single SNP (Figure 3C). MR-PRESSO test did not identify any potential SNP outliers.
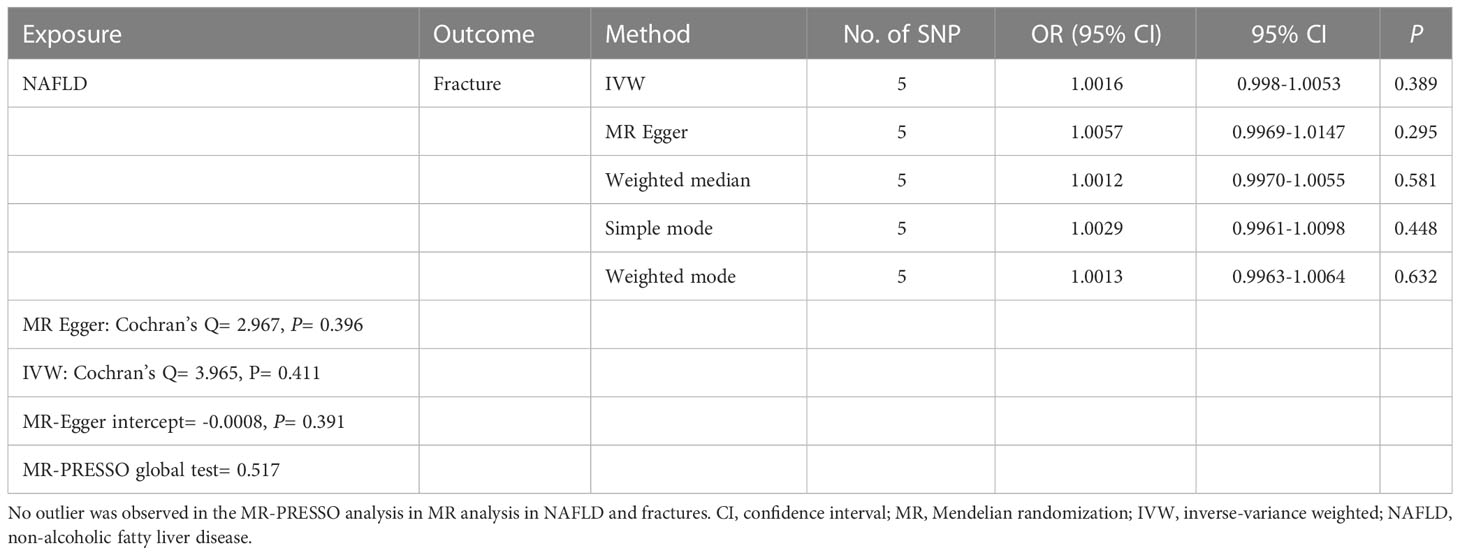
Table 2 Causal effect of NAFLD on fracture risk using genetic variant randomization in different MR methods.
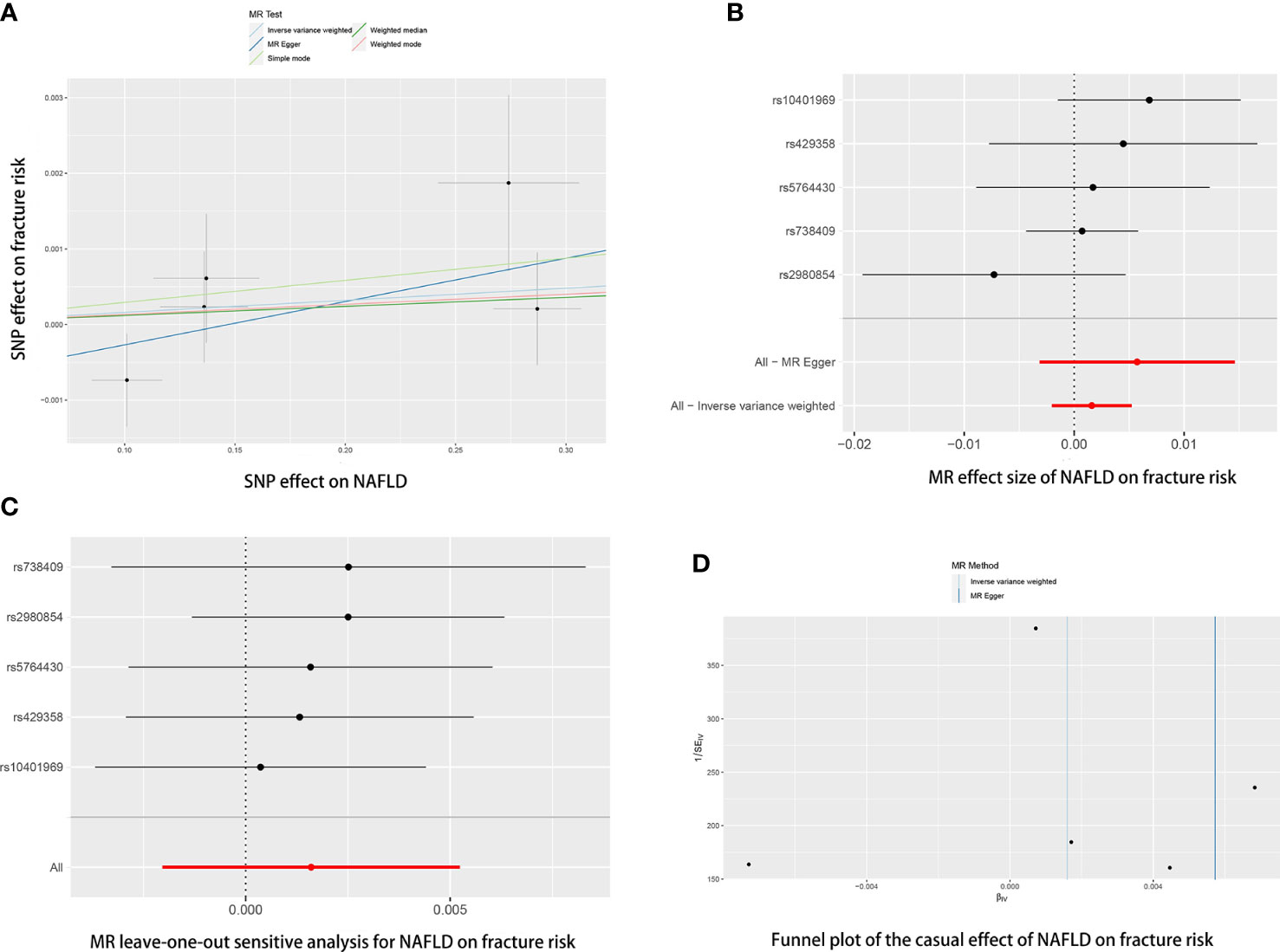
Figure 3 The causal effects of NAFLD on fracture risk in different MR methods. (A) Scatter plot. The slopes of each line represent the causal association for each method. (B) Forrest plot. (C) Leave-one-out analysis. (D) Funnel plot.
Genetical prediction of NAFLD on falling risk
The causal association of NAFLD variants with fracture was shown in Table 3 and Figure 4. MR analysis by the IVW method showed that there was no causal effect of NAFLD on falling risk (OR= 0.9912, 95% CI: 0.9412-1.0440, P= 0.740). The consistent result was also found in MR Egger (OR= 1.0211, 95% CI: 0.8865-1.1763, P= 0.791), weighted median (OR= 0.9987, 95% CI: 0.9681-1.0301, P= 0.932), simple mode (OR= 0.9949, 95% CI: 0.9521-1.0396, P= 0.831) and weighted mode (OR= 0.9964, 95% CI: 0.9613-1.0327, P= 0.852). The Cochran’s Q statistic of MR-Egger (P= 0.002) and IVW methods (P= 0.004) indicated significant heterogeneity between IVs, suggesting the use of the random effect model of IVW. No horizontal pleiotropy was detected in MR–Egger intercept (P= 0.682). The result remains no significant in leave-one-out sensitive analyses (Figure 4C). MR-PRESSO did not identify any potential SNP outliers.
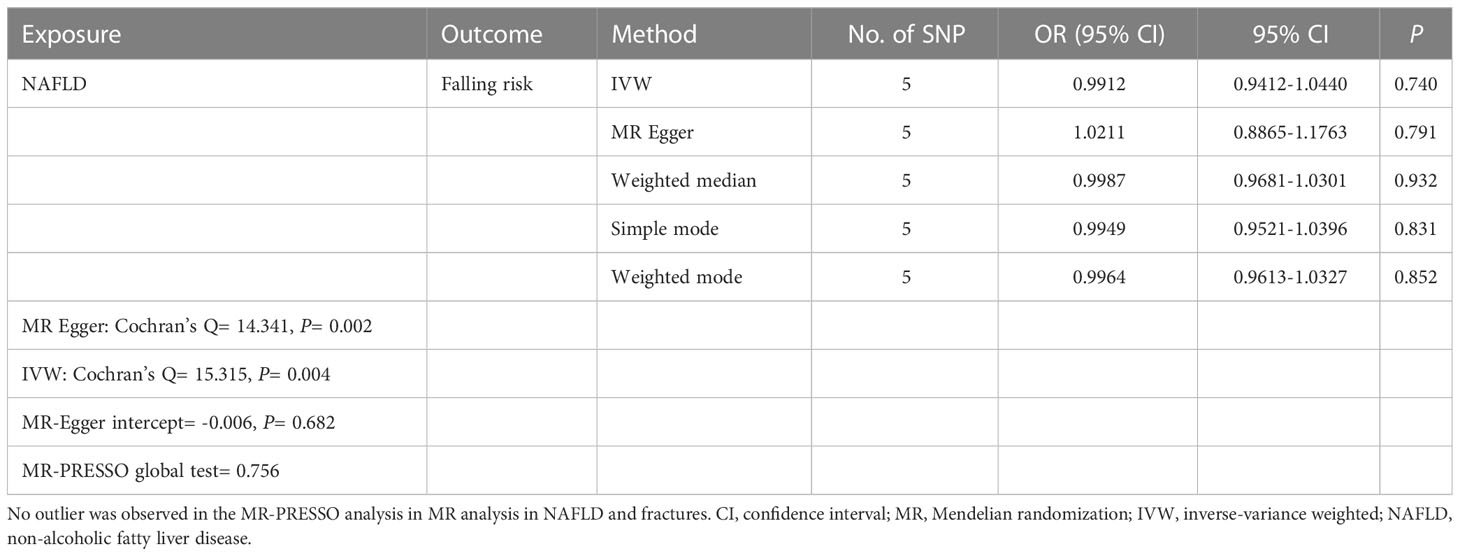
Table 3 Causal effect of NAFLD on falling risk using genetic variant randomization in different MR methods.
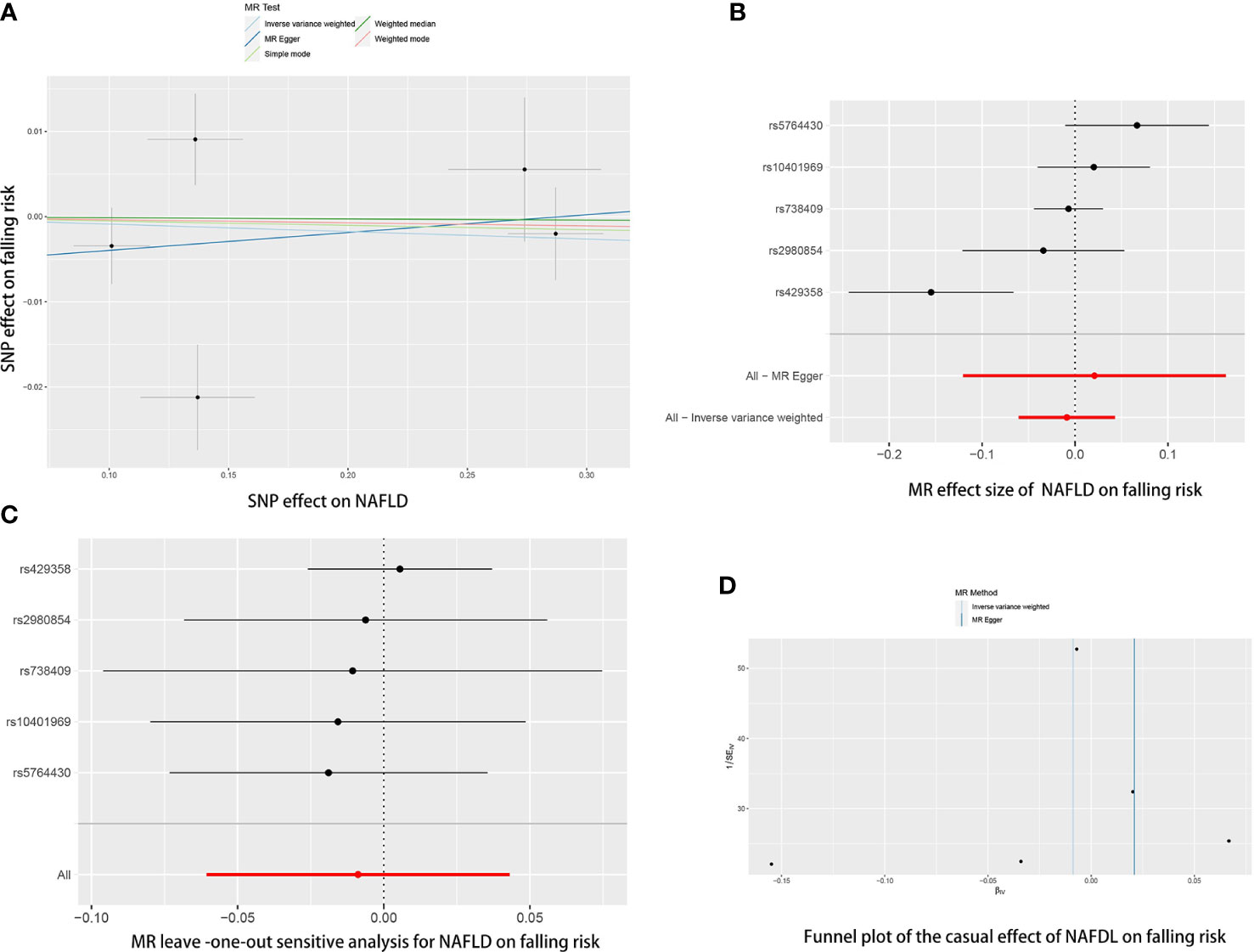
Figure 4 The causal effects of NAFLD on falling risk in different MR methods. (A) Scatter plot. The slopes of each line represent the causal association for each method. (B) Forrest plot. (C) Leave-one-out analysis. (D) Funnel plot.
Reverse Mendelian randomization analysis
Reverse MR analyses were then conducted to assess the causal effect of osteoporosis on NAFLD. Sixteen SNPs without linkage were chosen as the IVs for osteoporosis (Supplementary Table 2). Our study found no causal effect of osteoporosis on NAFLD (OR= 0.9759, 95% CI: 0.9246-1.3000, P= 0.375) (Table 4 and Figure 5). The F-statistic for IVs was 60.2 > 10. The consistent results were also found in other MR methods. The Cochran’s Q statistic of MR-Egger (P= 0.869) and IVW methods (P= 0.847) indicated no significant heterogeneity between IVs. The result remains no significant in leave-one-out sensitive analyses (Figure 5C). No horizontal pleiotropy was detected in MR–Egger intercept (P= 0.296). MR-PRESSO also did not identify any potential SNP outliers.
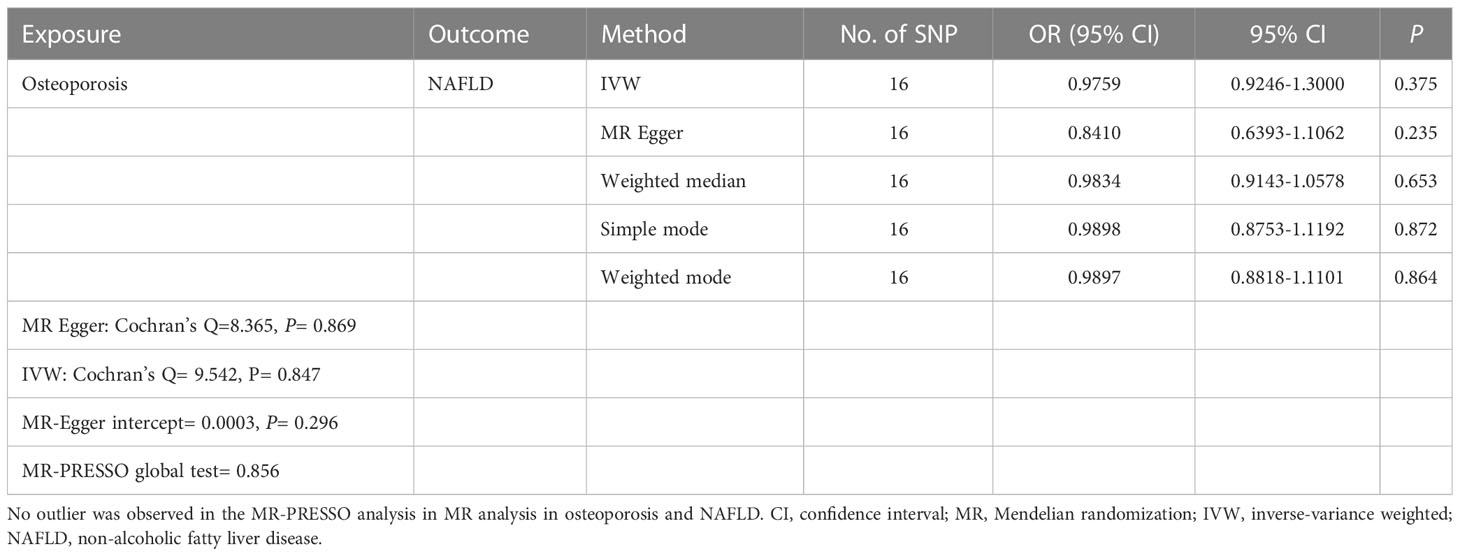
Table 4 Causal effect of osteoporosis on NAFLD using genetic variant randomization in different MR methods.
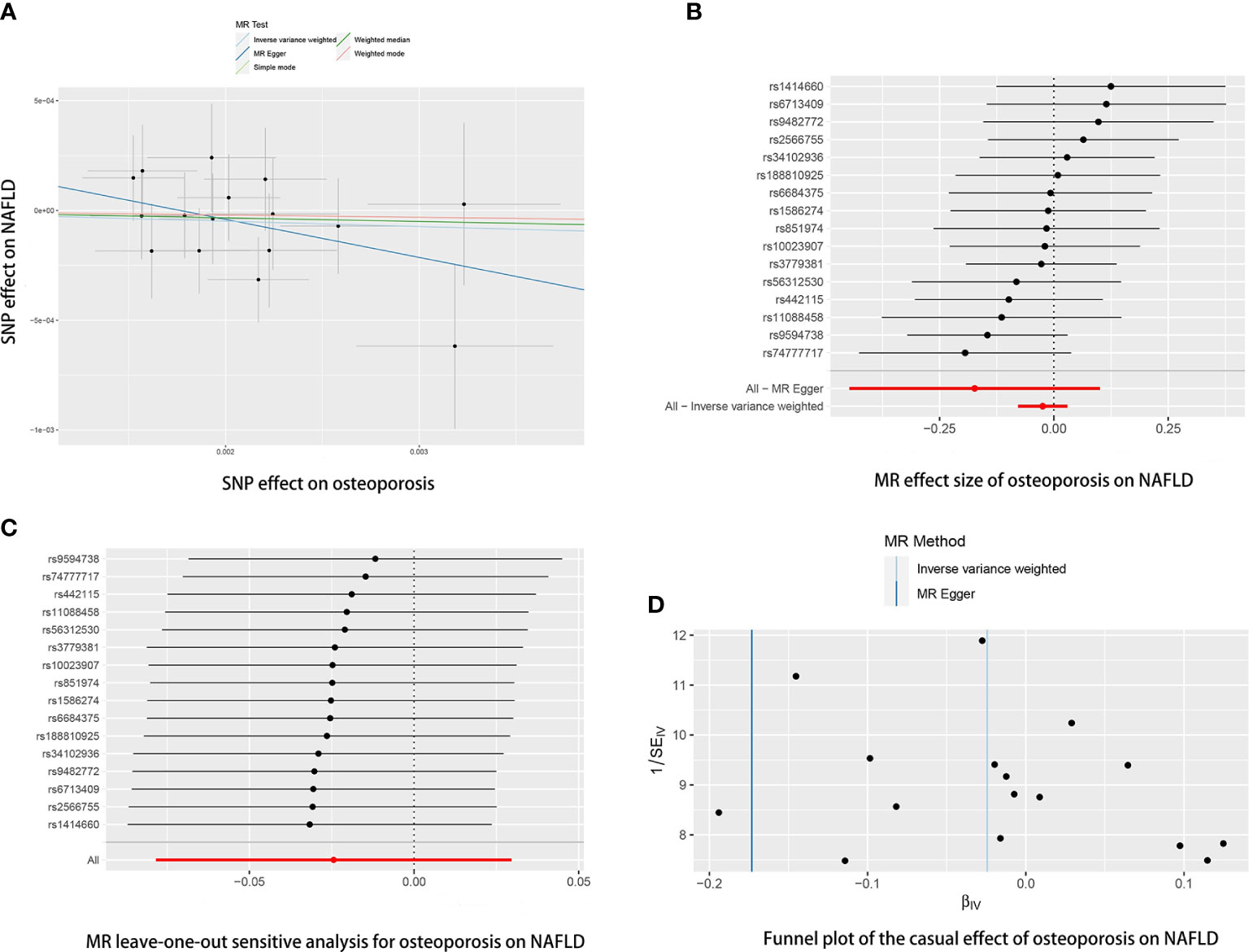
Figure 5 The causal effects of osteoporosis on NAFLD in different MR methods. (A) Scatter plot. The slopes of each line represent the causal association for each method. (B) Forrest plot. (C) Leave-one-out analysis. (D) Funnel plot.
Discussion
Although much work has explored the potential link between NAFLD and osteoporosis, the results are hardly convincing because of the nature of observational studies that cannot overcome the confounding factors. To our knowledge, this is the first MR study to assess the causal association between NAFLD and osteoporosis. Our studies indicated that genetic prediction of NAFLD was associated with a higher risk of osteoporosis. However, our findings did not support a causal association of NAFLD variants with fractures and falling risk. Moreover, the reverse MR analysis showed no genetic prediction of NAFLD on osteoporosis. In this study, five variations (rs2980854, rs429358, rs10401969, rs738409, rs5764430) at the APOE, SUGP1, TRIB1, SAMM50, and PNPLA3 loci linked to NAFLD was chosen for analysis. There are two known susceptibility loci for NAFLD (SAMM50, PNPLA3) and three potentially new candidate genetic regions for a clinical NAFLD diagnosis (APOE, SUGP1, TRIB1) (20).
Previously, several potential mechanisms behind NAFLD in osteoporosis have been widely discussed, including vitamin D deficiency, increased cytokines from the inflamed liver, limited physical activity, and others. In NAFLD, chronic production of pro-inflammatory cytokines like tumor necrosis factor (TNF)-a, interleukin (IL)-1, IL-6, and IL-7. TNF-a may be an important cytokine that mediates bone loss in NAFLD patients. Several studies have reported increased levels of circulating TNF-a in patients with NAFLD (24, 25). Studies showed that increased levels of TNF-α lead to increased osteoclast production and inhibit osteoblast activation (26). In addition, studies have indicated that serum TNF-a level was negatively associated with vitamin D levels (27). Osteocalcin (OCN) is a non-collagen protein expressed by osteoblasts, works as a marker of bone formation, and involves calcium homeostasis. Fernández-Real et al. (28) reported that serum OCN level was negatively associated with the blood markers of liver diseases, such as alanine transaminase (ALT) and aspartate transaminase (AST). In a cohort of 47 subjects, Szalay et al. (29) reported a decreased serum OCN level in NAFLD patients (29). At the same time, OCN may play a role in postmenopausal osteoporosis (30). In a recent study by Xu et al. (31), decreased OCN was associated with bone turn markers and osteopenia, and osteoporosis. Thus, decreased OCN in NAFLD patients could be a potential factor leading to osteoporosis. Osteopontin (OPN) is another multi-functional protein in our body, which is regarded as another factor that may mediate low BMD in NAFLD. Syn et al. (32) demonstrated that OPN was overexpressed in NAFLD patients. They found that the level of OPN is also linked with fibrosis progression in NASH in transgenic mice. On the other hand, higher OPN is also known as a risk of osteoporosis (33, 34). In an animal study, Chiang et al. showed that OPN-deficient mice could resist ovariectomy-induced osteoporosis (33). Another human study by Chang et al. (34) showed the same conclusion. They found a higher serum OPN levels (>14.7 ng/ml) are an essential risk factor for menopausal osteoporosis. Studies have shown that OPN may regulate the function of osteoblasts and osteoclasts by inhibiting the growth of mineral crystals (35). Vitamin D deficiency in NAFLD patients may provide another explanation for osteoporosis tendency. Targher et al. (36) found that NAFLD patients have a lower serum vitamin D level than the control group, and the level of vitamin D decrease was closely related to the histological severity of hepatic steatosis. In a recent study, Ciardullo et al. (37) found an inverse relationship between vitamin D status and NAFLD and fibrosis. The evidence suggests that vitamin D deficiency may play a role in NAFLD occurrence and progression. Furthermore, insufficient physical activity is also an essential factor in developing NAFLD. Physical activity is considered an important treatment to prevent NAFLD and reduce fat accumulation in the liver. At the same time, much clinical evidence has shown that limited physical inactivity is essential to osteoporosis in the elderly. Thus, insufficient physical activity could be an important lifestyle link to NAFLD with osteoporosis.
All in all, much evidence supports the tendency of osteoporosis in NAFLD patients in vivo and in vitro experiments. However, the previous results in clinical observational studies on their association were always controversial. In a retrospective cross-sectional study including 3,739 postmenopausal women, Lee et al. (38) indicated a significant negative association between NAFLD and BMD after adjusting potential confounders. A recent meta-analysis encompassing 7 observational studies showed a similar conclusion (12). In the analysis, Pan et al. showed that NAFLD patients have a higher risk of osteoporosis (OR = 1.33, 95%CI:1.24-1.44) and osteoporotic fractures (OR = 1.57, 95%CI:1.08-2.29) (12). However, after parameter adjustment, the significant association was only found in men but not in women (12). In a recent NHANES study, Xie et al. (39) found a negative association between NAFLD and osteoporosis. However, the association correlation becomes insignificant after adjusting for a large range of covariates, including gender, age, race, poverty income ratio (PIR), body mass index (BMI), and others. In another NAHENS study of 1784 subjects older than 50, Ciardullo et al. (40) shown the same findings. After adjusting for confounders, they found no association between NAFLD and femoral BMD and osteoporosis. These conflicting findings may result from the confounding factor in observational studies. For example, obesity may be an important mediator of the relationship between NAFLD and osteoporosis. In an early study by Li et al. (41), they found a positive association between NAFLD and osteoporosis in multivariable-adjusted models. However, after additionally adjusting visceral adipose tissue and BMI, they found NAFLD was negatively associated with vertebral BMD.
These epidemiological studies are prone to generate biased results because of the residual confounding and reverse causation (42). We first use MR analysis to reveal a causal association between NAFLD and osteoporosis risk. However, although the IVW method showed that NAFLD may increase fracture risk, the results were not statistically significant. Our results do not support that NAFLD increases the risk of fracture. Fracture is influenced by many factors. Our results show that NAFLD only slightly increased osteoporosis risk, so NAFLD may not cause a significantly increased fracture risk. In addition, our results also do not support that NAFLD increases the falling risk. Our study has several advantages. First, MR analysis was designed to reduce confounding and reverse causality using a genetic variation, as SNPs are randomly assigned at conception. Second, we used a large sample of the GWAS meta-analysis dataset (8,434 NAFLD cases and 770,180 controls) to conduct this MR analysis. Large F-statistic values indicated five IVs were closely related to NAFLD. Third, we also performed a reverse MR analysis to explore the causal effect of osteoporosis on NAFLD, which facilitates our better understanding of the interconnection between these two diseases. However, some potential limitations could not be avoided. First, some heterogeneity is found between NAFLD and fracture, which could be led by the choice of some instrumental variables. Second, this MR study reveals the potential causal effect of NAFLD on osteoporosis, but no causal association is observed between NAFLD and fracture and falling risk. The inconsistent result remains elusive and needs further investigation. Third, due to the limitations of GWAS, we did not perform MR analysis by age and sex. Fourth, another thing worth mentioning is that although we found a higher osteoporosis risk in NAFLD patients, this increase was small, and the OR was quite low. So, the conclusion should be interpreted more cautiously. Fifth, these findings should be interpreted cautiously, as the study sample was from a European population. Whether the results can be generalized to other populations will need to be further investigated.
Conclusions
The results of this study indicate a causal association between NAFLD and osteoporosis. NAFLD patients have a higher risk of osteoporosis but not fractures and falling risk. In addition, our results do not support a causal effect of osteoporosis on NAFLD.
Data availability statement
The original contributions presented in the study are included in the article/Supplementary Material. Further inquiries can be directed to the corresponding authors.
Ethics statement
Ethical review and approval was not required for the study on human participants in accordance with the local legislation and institutional requirements. Written informed consent for participation was not required for this study in accordance with the national legislation and the institutional requirements.
Author contributions
Conceptualization: AC, PX, PW. Data curation: ZF, XW, and YZ. Formal analysis, XW and YZ. Investigation: AC, PX, and ZF. Methodology: AC, PX, ZF, JL, SH, and DZ. Project administration: AC, PX, and PW. Software: AC, PX, and ZF. Visualization: HW and YZ. Writing – original draft: AC, PX, ZF, DZ, XW, PW, and YZ. Writing – review & editing, AC, PX, and ZF. All authors contributed to the article and approved the submitted version.
Acknowledgments
We acknowledge the investigators of the original GWAS studies for sharing summary data used in this study.
Conflict of interest
The authors declare that the research was conducted in the absence of any commercial or financial relationships that could be construed as a potential conflict of interest.
Publisher’s note
All claims expressed in this article are solely those of the authors and do not necessarily represent those of their affiliated organizations, or those of the publisher, the editors and the reviewers. Any product that may be evaluated in this article, or claim that may be made by its manufacturer, is not guaranteed or endorsed by the publisher.
Supplementary material
The Supplementary Material for this article can be found online at: https://www.frontiersin.org/articles/10.3389/fendo.2023.1215790/full#supplementary-material
References
1. Styrkarsdottir U, Thorleifsson G, Gudjonsson SA, Sigurdsson A, Center JR, Lee SH, et al. Sequence variants in the PTCH1 gene associate with spine bone mineral density and osteoporotic fractures. Nat Commun (2016) 7:10129. doi: 10.1038/ncomms10129
2. Xiao PL, Cui AY, Hsu CJ, Peng R, Jiang N, Xu XH, et al. Global, regional prevalence, and risk factors of osteoporosis according to the World Health Organization diagnostic criteria: a systematic review and meta-analysis. Osteoporos Int (2022) 33(10):2137–53. doi: 10.1007/s00198-022-06454-3
3. Hernlund E, Svedbom A, Ivergård M, Compston J, Cooper C, Stenmark J, et al. Osteoporosis in the European Union: medical management, epidemiology and economic burden. A report prepared in collaboration with the International Osteoporosis Foundation (IOF) and the European Federation of Pharmaceutical Industry Associations (EFPIA). Arch Osteoporos (2013) 8:136. doi: 10.1007/s11657-013-0136-1
4. Compston JE, McClung MR, Leslie WD. Osteoporosis. Lancet (2019) 393:364–76. doi: 10.1016/S0140-6736(18)32112-3
5. Demontiero O, Vidal C, Duque G. Aging and bone loss: new insights for the clinician. Ther Adv Musculoskelet Dis (2012) 4:61–76. doi: 10.1177/1759720X11430858
7. Gao Y, Shi L. Mindfulness, physical activity and avoidance of secondhand smoke: A study of college students in shanghai. Int J Environ Res Public Health (2015) 12:10106–16. doi: 10.3390/ijerph120810106
8. Fan JG, Kim SU, Wong VW. New trends on obesity and NAFLD in Asia. J Hepatol (2017) 67:862–73. doi: 10.1016/j.jhep.2017.06.003
9. Younossi ZM, Koenig AB, Abdelatif D, Fazel Y, Henry L, Wymer M. Global epidemiology of nonalcoholic fatty liver disease-Meta-analytic assessment of prevalence, incidence, and outcomes. Hepatology (2016) 64:73–84. doi: 10.1002/hep.28431
10. Ye Q, Zou B, Yeo YH, Li J, Huang DQ, Wu Y, et al. Global prevalence, incidence, and outcomes of non-obese or lean non-alcoholic fatty liver disease: a systematic review and meta-analysis. Lancet Gastroenterol Hepatol (2020) 5:739–52. doi: 10.1016/S2468-1253(20)30077-7
11. Yang YJ, Kim DJ. An overview of the molecular mechanisms contributing to musculoskeletal disorders in chronic liver disease: osteoporosis, sarcopenia, and osteoporotic sarcopenia. Int J Mol Sci (2021) 22(5):2604. doi: 10.3390/ijms22052604
12. Pan B, Cai J, Zhao P, Liu J, Fu S, Jing G, et al. Relationship between prevalence and risk of osteoporosis or osteoporotic fracture with non-alcoholic fatty liver disease: A systematic review and meta-analysis. Osteoporos Int (2022) 33:2275–86. doi: 10.1007/s00198-022-06459-y
13. Loosen SH, Roderburg C, Demir M, Qvartskhava N, Keitel V, Kostev K, et al. Non-alcoholic fatty liver disease (NAFLD) is associated with an increased incidence of osteoporosis and bone fractures. Z Gastroenterol (2022) 60:1221–7. doi: 10.1055/a-1482-9236
14. Mantovani A, Dauriz M, Gatti D, Viapiana O, Zoppini G, Lippi G, et al. Systematic review with meta-analysis: non-alcoholic fatty liver disease is associated with a history of osteoporotic fractures but not with low bone mineral density. Aliment Pharmacol Ther (2019) 49:375–88. doi: 10.1111/apt.15087
15. Bhatt SP, Nigam P, Misra A, Guleria R, Qadar Pasha MA. Independent associations of low 25 hydroxy vitamin D and high parathyroid hormonal levels with nonalcoholic fatty liver disease in Asian Indians residing in north India. Atherosclerosis (2013) 230:157–63. doi: 10.1016/j.atherosclerosis.2013.07.006
16. Lee SH, Yun JM, Kim SH, Seo YG, Min H, Chung E, et al. Association between bone mineral density and nonalcoholic fatty liver disease in Korean adults. J Endocrinol Invest (2016) 39:1329–36. doi: 10.1007/s40618-016-0528-3
17. Kaya M, Işık D, Beştaş R, Evliyaoğlu O, Akpolat V, Büyükbayram H, et al. Increased bone mineral density in patients with non-alcoholic steatohepatitis. World J Hepatol (2013) 5:627–34. doi: 10.4254/wjh.v5.i11.627
18. Sekula P, Del Greco MF, Pattaro C, Köttgen A. Mendelian randomization as an approach to assess causality using observational data. J Am Soc Nephrol (2016) 27:3253–65. doi: 10.1681/ASN.2016010098
19. Guo Q, Burgess S, Turman C, Bolla MK, Wang Q, Lush M, et al. Body mass index and breast cancer survival: a Mendelian randomization analysis. Int J Epidemiol (2017) 46:1814–22. doi: 10.1093/ije/dyx131
20. Ghodsian N, Abner E, Emdin CA, Gobeil É, Taba N, Haas ME, et al. Electronic health record-based genome-wide meta-analysis provides insights on the genetic architecture of non-alcoholic fatty liver disease. Cell Rep Med (2021) 2:100437. doi: 10.1016/j.xcrm.2021.100437
21. Morris JA, Kemp JP, Youlten SE, Laurent L, Logan JG, Chai RC, et al. An atlas of genetic influences on osteoporosis in humans and mice. Nat Genet (2019) 51:258–66. doi: 10.1038/s41588-018-0302-x
22. Trajanoska K, Seppala LJ, Medina-Gomez C, Hsu YH, Zhou S, van Schoor NM, et al. Genetic basis of falling risk susceptibility in the UK Biobank Study. Commun Biol (2020) 3:543. doi: 10.1038/s42003-020-01256-x
23. Burgess S, Butterworth A, Thompson SG. Mendelian randomization analysis with multiple genetic variants using summarized data. Genet Epidemiol (2013) 37:658–65. doi: 10.1002/gepi.21758
24. Aigner E, Theurl I, Theurl M, Lederer D, Haufe H, Dietze O, et al. Pathways underlying iron accumulation in human nonalcoholic fatty liver disease. Am J Clin Nutr (2008) 87:1374–83. doi: 10.1093/ajcn/87.5.1374
25. Chu CJ, Lu RH, Wang SS, Chang FY, Wu SL, Lu CL, et al. Risk factors associated with non-alcoholic fatty liver disease in Chinese patients and the role of tumor necrosis factor-alpha. Hepatogastroenterology (2007) 54:2099–102.
26. Zou W, Hakim I, Tschoep K, Endres S, Bar-Shavit Z. Tumor necrosis factor-alpha mediates RANK ligand stimulation of osteoclast differentiation by an autocrine mechanism. J Cell Biochem (2001) 83:70–83. doi: 10.1002/jcb.1202
27. Peterson CA, Heffernan ME. Serum tumor necrosis factor-alpha concentrations are negatively correlated with serum 25(OH)D concentrations in healthy women. J Inflammation (Lond) (2008) 5:10. doi: 10.1186/1476-9255-5-10
28. Fernández-Real JM, Ortega F, Gómez-Ambrosi J, Salvador J, Frühbeck G, Ricart W. Circulating osteocalcin concentrations are associated with parameters of liver fat infiltration and increase in parallel to decreased liver enzymes after weight loss. Osteoporos Int (2010) 21:2101–7. doi: 10.1007/s00198-010-1174-9
29. Szalay F, Lakatos P, Németh J, Abonyi M, Büki B, Tarján G, et al. [Decreased serum osteocalcin level in non-alcoholic and alcoholic chronic liver diseases]. Orv Hetil (1991) 132:1301–5.
30. Pietschmann P, Resch H, Krexner E, Woloszczuk W, Willvonseder R. Decreased serum osteocalcin levels in patients with postmenopausal osteoporosis. Acta Med Austriaca (1991) 18:114–6.
31. Xu Y, Shen L, Liu L, Zhang Z, Hu W. Undercarboxylated osteocalcin and its associations with bone mineral density, bone turnover markers, and prevalence of osteopenia and osteoporosis in chinese population: A cross-sectional study. Front Endocrinol (Lausanne) (2022) 13:843912. doi: 10.3389/fendo.2022.843912
32. Syn WK, Choi SS, Liaskou E, Karaca GF, Agboola KM, Oo YH, et al. Osteopontin is induced by hedgehog pathway activation and promotes fibrosis progression in nonalcoholic steatohepatitis. Hepatology (2011) 53:106–15. doi: 10.1002/hep.23998
33. Chiang TI, Chang IC, Lee HS, Lee H, Huang CH, Cheng YW. Osteopontin regulates anabolic effect in human menopausal osteoporosis with intermittent parathyroid hormone treatment. Osteoporos Int (2011) 22:577–85. doi: 10.1007/s00198-010-1327-x
34. Chang IC, Chiang TI, Yeh KT, Lee H, Cheng YW. Increased serum osteopontin is a risk factor for osteoporosis in menopausal women. Osteoporos Int (2010) 21:1401–9. doi: 10.1007/s00198-009-1107-7
35. Ishijima M, Rittling SR, Yamashita T, Tsuji K, Kurosawa H, Nifuji A, et al. Enhancement of osteoclastic bone resorption and suppression of osteoblastic bone formation in response to reduced mechanical stress do not occur in the absence of osteopontin. J Exp Med (2001) 193:399–404. doi: 10.1084/jem.193.3.399
36. Targher G, Bertolini L, Scala L, Cigolini M, Zenari L, Falezza G, et al. Associations between serum 25-hydroxyvitamin D3 concentrations and liver histology in patients with non-alcoholic fatty liver disease. Nutr Metab Cardiovasc Dis (2007) 17:517–24. doi: 10.1016/j.numecd.2006.04.002
37. Ciardullo S, Muraca E, Cannistraci R, Perra S, Lattuada G, Perseghin G. Low 25 (OH) vitamin D levels are associated with increased prevalence of nonalcoholic fatty liver disease and significant liver fibrosis. Diabetes Metab Res Rev (2023) 39:e3628. doi: 10.1002/dmrr.3628
38. Lee DY, Park JK, Hur KY, Um SH. Association between nonalcoholic fatty liver disease and bone mineral density in postmenopausal women. Climacteric (2018) 21:498–501. doi: 10.1080/13697137.2018.1481380
39. Xie R, Liu M. Relationship between non-alcoholic fatty liver disease and degree of hepatic steatosis and bone mineral density. Front Endocrinol (Lausanne) (2022) 13:857110. doi: 10.3389/fendo.2022.857110
40. Ciardullo S, Muraca E, Zerbini F, Manzoni G, Perseghin G. NAFLD and liver fibrosis are not associated with reduced femoral bone mineral density in the general US population. J Clin Endocrinol Metab (2021) 106:e2856–65. doi: 10.1210/clinem/dgab262
41. Li BT, Simon TG, Wang N, Chung RT, Corey KE, Dichtel LE, et al. Association between liver fat and bone density is confounded by general and visceral adiposity in a community-based cohort. Obes (Silver Spring) (2021) 29:595–600. doi: 10.1002/oby.23100
Keywords: NAFLD, osteoporosis, fracture, Mendelian randomization, genome-wide association study
Citation: Cui A, Xiao P, Fan Z, Lei J, Han S, Zhang D, Wei X, Wang P and Zhuang Y (2023) Causal association of NAFLD with osteoporosis, fracture and falling risk: a bidirectional Mendelian randomization study. Front. Endocrinol. 14:1215790. doi: 10.3389/fendo.2023.1215790
Received: 02 May 2023; Accepted: 19 July 2023;
Published: 09 August 2023.
Edited by:
Michela Rossi, Bambino Gesù Children’s Hospital (IRCCS), ItalyReviewed by:
Atika Dhar, National Institutes of Health (NIH), United StatesStefano Ciardullo, University of Milano Bicocca, Italy
Copyright © 2023 Cui, Xiao, Fan, Lei, Han, Zhang, Wei, Wang and Zhuang. This is an open-access article distributed under the terms of the Creative Commons Attribution License (CC BY). The use, distribution or reproduction in other forums is permitted, provided the original author(s) and the copyright owner(s) are credited and that the original publication in this journal is cited, in accordance with accepted academic practice. No use, distribution or reproduction is permitted which does not comply with these terms.
*Correspondence: Yan Zhuang, zhuangyan2512@163.com; Pengfei Wang, pengfei_wang@163.com; Xing Wei, 92871360@163.com
†These authors have contributed equally to this work
‡ORCID: Peilun Xiao, orcid.org/0000-0002-7256-6279