- 1Department of Obstetrics and Gynaecology, The Chinese University of Hong Kong, Hong Kong, Hong Kong SAR, China
- 2Department of Infertility and Reproductive Medicine, Taiwan IVF Group Center, Hsinchu, Taiwan
- 3Department of Fertility and Reproductive Medicine, Ton-Yen General Hospital, Hsinchu, Taiwan
- 4Reproduction and Development Laboratory, Li Ka Shing Institute of Health Sciences, The Chinese University of Hong Kong, Hong Kong, Hong Kong SAR, China
- 5School of Biomedical Sciences, The Chinese University of Hong Kong, Hong Kong, Hong Kong SAR, China
- 6Chinese University of Hong Kong -Sichuan University Joint Laboratory in Reproductive Medicine, The Chinese University of Hong Kong, Hong Kong, Hong Kong SAR, China
Purpose: Investigate whether local angiotensin II (AngII) and its AngII type 1 and 2 receptors (AT1R, AT2R) in the endometrium are different and correlate with microvessel density in women with reproductive failure and pregnancy outcomes.
Methods: Endometrium during the window of implantation from 40 women with recurrent miscarriage (RM) and 40 with recurrent implantation failure (RIF) were compared with 27 fertile women. Peri-implantation endometrium from 54 women prior to euploid embryo transfer were collected and compared in women with successful pregnancy and unsuccessful pregnancy.
Results: Compared with fertile women, expression of AT2R was significantly lower, while AT1R/AT2R expression ratio was significantly higher in the stroma of the RIF group. Endometrium arteriole MVD was significantly lower and negatively correlated with the AT1R/AT2R expression ratio in the stroma of the RIF group. No significant differences and correlations were found in the RM group. Compared with the pregnancy group, expression of AT1R and AT2R were significantly lower in all compartments, but only AT1R/AT2R ratio was significantly higher in the stroma of the non-pregnancy group. Similarly, endometrium arteriole MVD was also significantly lower and negatively correlated with the AT1R/AT2R ratio in the stroma of the non-pregnancy group.
Conclusion: Local renin-angiotensin system is dysregulated in peri-implantation endometrium and associated with abnormal angiogenesis in RIF and poor implantation outcome after embryo transfer.
Introduction
Endometrial receptivity is a major focus in the field of reproductive medicine. Robust angiogenesis in the endometrium is essential for the success of implantation and early pregnancy (1, 2), which is usually achieved by vasodilatation and remodeling of the endometrial arteries (3). Inadequate and excessive vascularity in the endometrium may influence endometrial receptivity thus resulting in implantation failure and miscarriage (4). Numerous studies were conducted to understand the relationship between endometrial blood supply and endometrial receptivity (5–8). Currently, there are two main methods to assess endometrial vascularity, one is the conventional method using immunohistochemical analysis in endometrial biopsy to evaluate microvessel density (MVD), and the other is a relatively new method using 3-dimensional power Doppler ultrasonography (3-DPD) assess the endometrial blood flow strength. However, the histological morphometrics in the endometrium may not necessarily correlate well with the ultrasound findings (9). This could be due to immunohistochemistry assessing the microvasculature, while 3-DPD can only detect macrovasculature (10).
The renin-angiotensin system (RAS) is a classic endocrine system, which has been known for its crucial role in maintaining systematic blood pressure and cardiovascular homeostasis. RAS consists of a cascade of peptides; after being cleaved from angiotensinogen by renin, Angiotensin I (Ang I) is converted by angiotensin-converting enzyme (ACE) to the most bioactive peptide Angiotensin II (Ang II). Ang II has regulatory effects mainly on angiogenesis and vasculogenesis through its two receptors, Ang II type 1 receptor (AT1R) and type 2 receptor (AT2R) (11–14). Both are vasomotor, while AT1R mediates vasoconstriction, AT2R mediates vasodilation (15). The balance between AT1R and AT2R may work in concert to influence the systematic vascular tone, perfusion pressure, and thus blood flow and tissue function (16). In the pathophysiologic state, the balance of AT1R and AT2R is highly controlled (17, 18).
Local RAS has been identified in the reproductive system, indicating its potential biological effects on reproductive functions (19). In women, various components of RAS are expressed in the ovary, uterus, and placenta (20–23). This includes Ang II in particular, which regulates oocyte maturation, ovulation, and corpus luteum under stimulation of gonadotropins and female sex hormones (24–26). Ang II and its receptors are also important in early embryonic development, where RAS is reported to participate in the dialogue between mother and developing embryo in rat and bovine (27, 28). In addition, activation of Ang II and its receptors leads to potent induction of vascular endothelial growth factor (VEGF) (29) and establishment of circulation in the placenta and decidua to maintain pregnancy (30–32). However, systematic investigation of the local RAS system in endometrium and its potential role in endometrium receptivity and implantation in humans is still very limited.
Recurrent miscarriage (RM) and recurrent implantation failure (RIF) are two major hurdles in reproductive medicine, whether they share any common or have different endometrial defects remains controversial (33, 34). Some suggest RM and RIF may share similar etiology (35–37), but cytokine profiling showed different results between the endometrium from women with RM and RIF (38). It was hypothesized that women with unexplained RM could be over-receptive contrary to that of women with RIF, which means the endometrium of RM is less likely to identify the abnormal embryos and prevent them from implantation, hence pregnancy loss after implantation (39, 40). Whether endometrium vasculature is different between RM and RIF and associated with endometrium angiogenesis, in this study our first aim was to investigate the localization and expression of Ang ll and its receptors AT1R and AT2R and correlate with arteriole and capillary in peri-implantation endometrium among women with RM or RIF in natural cycle with fertile women. On the other hand, good-quality embryos are a pre-requisite for successful embryo implantation. Recent technology of preimplantation genetic testing (PGT) allows the detection of aneuploid embryos, and ensures only euploid embryos are transferred and also excludes confounding factors from the embryo. However the IVF outcomes are still not significantly improved and the endometrium factor remained to be evaluated. Therefore, in another cohort of this study we included women in a mock cycle and compared endometrium RAS between women who did and did not conceive prior to PGT selected frozen embryo transfer (FET). Our hypothesis was that derangement of local RAS in peri-implantation endometrium may be associated with abnormal angiogenesis and poor IVF outcome in women with reproductive failure.
Materials and methods
Subjects
In the first cohort study, a total of 107 women, including 27 fertile controls, 40 with unexplained RM, and 40 with unexplained RIF in the natural cycle were recruited and LH surge was identified by daily urine dipstick test from day 9 of the menstrual cycle onwards. Fertile controls were defined as women who had at least one live birth without any major pregnancy complications, such as pregnancy-induced hypertension, within two years. RM was defined as women who had a history of ≥3 consecutive miscarriages before gestational week 20, while RIF was defined as women who failed to achieve a clinical pregnancy after transferring at least 4 morphologically good-quality embryos in a minimum of 3 cycles (41, 42). The subjects were recruited from the Prince of Wales Hospital, The Chinese University of Hong Kong in Hong Kong.
In the second cohort study, a total of 54 women undergoing FET after PGT were recruited. All these women were in a mock hormone replacement treatment (HRT) cycle, and oral estradiol valerate 6 mg daily was administered from the second day of the menstrual cycle. Ultrasound examination was performed to measure endometrial thickness, and vaginal progesterone (utrogestan, 600 mg per day) was administered if the thickness reached more than 8 mm and no peri-ovulatory leading follicle was present. In the following HRT cycle, only one euploid blastocyst confirmed by PGT was transferred. The pregnancy group was defined based on positive serum β-hCG measurement at 9 days after ET, a viable intrauterine fetus in transvaginal ultrasonography at 23 days after ET, and the pregnancy continued till at least 20 weeks gestation. Non-pregnancy group was defined as having negative serum β-hCG (<5 mIU/L) at 9 days and no viable fetus at 23 days after ET. We also excluded those women who conceived but later miscarried. The subjects were recruited from Ton‐Yen General Hospital and Taiwan IVF Group Center in Taiwan.
Inclusion and exclusion criteria
Inclusion criteria included age between 25 and 45 years old and women with regular menstrual periods (25–35 days) and normal BMI. Exclusion criteria included women with endometriosis, hydrosalpinx, structural uterine abnormalities, steroid hormone treatment within 3 months of recruitment, known causes of RM and RIF, including antiphospholipid syndrome, thrombophilia, abnormal thyroid function tests, and any parental chromosomal abnormalities.
Endometrial biopsy
In the first cohort study, the endometrial biopsies were precisely timed at LH surge (LH+7 day) in a natural menstrual cycle. In the second cohort study, endometrial biopsies were precisely timed at 5 days after progesterone treatment (P+5 day) in a mock HRT cycle. All endometrial samples were collected by using a Pipelle sampler (Prodimed, France). Then all the collected samples were rinsed and cleaned with sterile PBS, and then put into 10% neutral‐buffered formalin for overnight fixation before being embedded into paraffin wax for immunohistochemistry staining. The histological endometrium dating was confirmed by standard criteria of Noyes et al. (43) under hematoxylin and eosin staining showing a mid-secretory stage with distended glands and stromal edema.
Immunohistochemistry
After embedding in paraffin wax, endometrial tissues were cut in 3.5 μm sections consecutively and mounted onto 3-aminopropyl-triethoxysilane (Sigma-Aldrich, St. Louis) coated slides. The serial sections were dewaxed, stained, and examined under the standard protocol and conditions to minimize the variations. The sections were dewaxed in xylene, rehydrated through descending alcohols to phosphate-buffered saline (PBS) (pH 7.6), and quenched in 3% hydrogen peroxide in methanol for 20 min. After washing, the antigen was retrieved in sodium citrate buffer (pH 6.0) in a microwave oven. The buffer was pre-heated in the microwave oven until boiling, after that the slides were cooled down for at least 20 min. The slides were washed in PBS and incubated in blocking solution (5% goat serum albumin in PBS) for 1 h at room temperature, and incubated overnight at 4°C with primary antibody, either rabbit anti-human Ang II polyclonal antibody (PA5-33339, Thermo Fisher, US) at 1:400 dilution, rabbit anti-human AT1R polyclonal antibody (AAR-011, Alomone Labs, Israel) at 1:1500 dilution, rabbit anti-human AT2R antibody (ab19134, AbCam, UK) at 1:150 dilution, or rabbit polyclonal anti-human vWF antibody (A0082, DAKO, Japan) at 1:1000 dilution. The sections incubated with blocking solution with no primary antibody were used as the negative control. After incubation, slides were washed in Tween‐20 in PBS and incubated with secondary antibody (goat anti-rabbit) for 1 h. The immunoreactive binding was visualized by incubation with peroxidase substrate DAB (3.3-diaminobenzidene tetrahydrochloride (DAB, Dako). The slides were then washed in distilled water and counterstained with 20% hematoxylin solution for 10 min and dehydrated through alcohols, cleared in xylene, and mounted in DPX medium (Sigma-Aldrich, St. Louis).
Image analysis
All immunostaining results were under a standard microscopic examination protocol in a blinded manner. The first field was selected from the upper left of each section including luminal epithelium (LE) and glandular epithelium (GE) of the endometrium under the magnification at ×400. The subsequent field was then obtained by moving to another field just next to the last field. This was repeated until 5 fields with LE and GE, and also stroma (ST) of the endometrium in every field were captured. Localization of each protein in each compartment of the endometrium was examined and their expression levels were quantified by H score using inForm 2.4 Software (Perkin‐Elmer/Caliper Life Science, US). The intensity of the immunohistochemical signals in each compartment was classified into 0, 1+, 2+, and 3+, and then the percentages of each positively stained cell were computed as absent (0%), weak (30%), moderate (50%), or strong (70%). The average of the measurements from at least 5 sections were calculated for comparison. Inter- and intra-observer variability were 3.42% and 5.61%.
Micro-vessel density
The endometrial sections were scanned to identify the hot spots of microvessels in the ST of the endometrium, representing the areas of high vascularization. AT2R, but not AT1R, mostly stained positive in arteriole endothelium with a clear cuff of one to two layers of smooth muscle. While vWF is mainly expressed in capillary endothelium with thin-walled vessels consisting of a single layer of endothelial cells without any smooth muscle cells (44). Total numbers of arteriole and capillary micro-vessels in the stroma were separately counted in 5 highly vascularized areas in order to avoid selection bias and minimize the variation and then calculated as average endometrium arteriole and capillary MVDs per square millimeter, vessels/mm2 (45, 46). The results were correlated with the non-microvessel AT2R-expressed stroma cells.
Statistics
Data were analyzed using SPSS, version 26.0 (SPSS, Inc). The data distribution was checked by the Shapiro-Wilk test. Quantitative data were expressed as mean ± standard error of the mean (SEM) if normal distribution, and median and inter-quartile range if skewed distribution. AT1R/AT2R expression ratio was calculated based on the expression levels in each compartment quantified by H score. Differences between groups were assessed by independent t-test, One-way ANOVA, or non-parametric Kruskal-WaIIis test where appropriate. Correlation was performed by non-parametric Spearman’s rho and Kendall’s tau- b statistics. The sample size was calculated based on our pilot study with a power of 0.8 and type 2 error <0.05.
Results
Demographic characteristics
Detailed demographic characteristics of the women who participated in each cohort are summarized in Table 1. Age and BMI were not significantly different amongst groups in each cohort, but age was significantly higher in the second cohort with a mock HRT cycle. In the first cohort with the natural cycle, the numbers of previous pregnancies and live births were significantly lower and the number of failed cycles was significantly higher in the RIF group, while the numbers of previous miscarriages were significantly higher in the RM group. In the second cohort with mock HRT cycle, numbers of previous pregnancies, live births, previous miscarriages, and failed cycles were not statistically significant. No significant difference was found between women in a natural cycle and in an HRT cycle.
Comparison among fertile controls, RM, and RIF
There was no significant difference in Ang II expression in either LE, GE, or ST among fertile controls, RM, and RIF groups (Figure 1A). When compared with fertile controls, expression of AT1R was significantly lower in LE (38.8% lower) and GE (51.9% lower) only, and expression of AT2R was significantly lower in aII compartments of the endometrium, including LE (37.5% lower), GE (44.1% decreased), and ST (46.7% lower) in RIF groups (Figure 1B). While there was no significant difference in the expression of AT1R and AT2R in any compartment between women with RM and the control group. AT1R/AT2R ratio was significantly higher only in the stroma of the RIF group (96.3% higher) when compared with fertile control and RM groups, but there was no significant difference in Ang II, AT1R, AT2R expressions, and AT1R/AT2R expression ratio in stroma between women with RM and control group (Figure 1B).
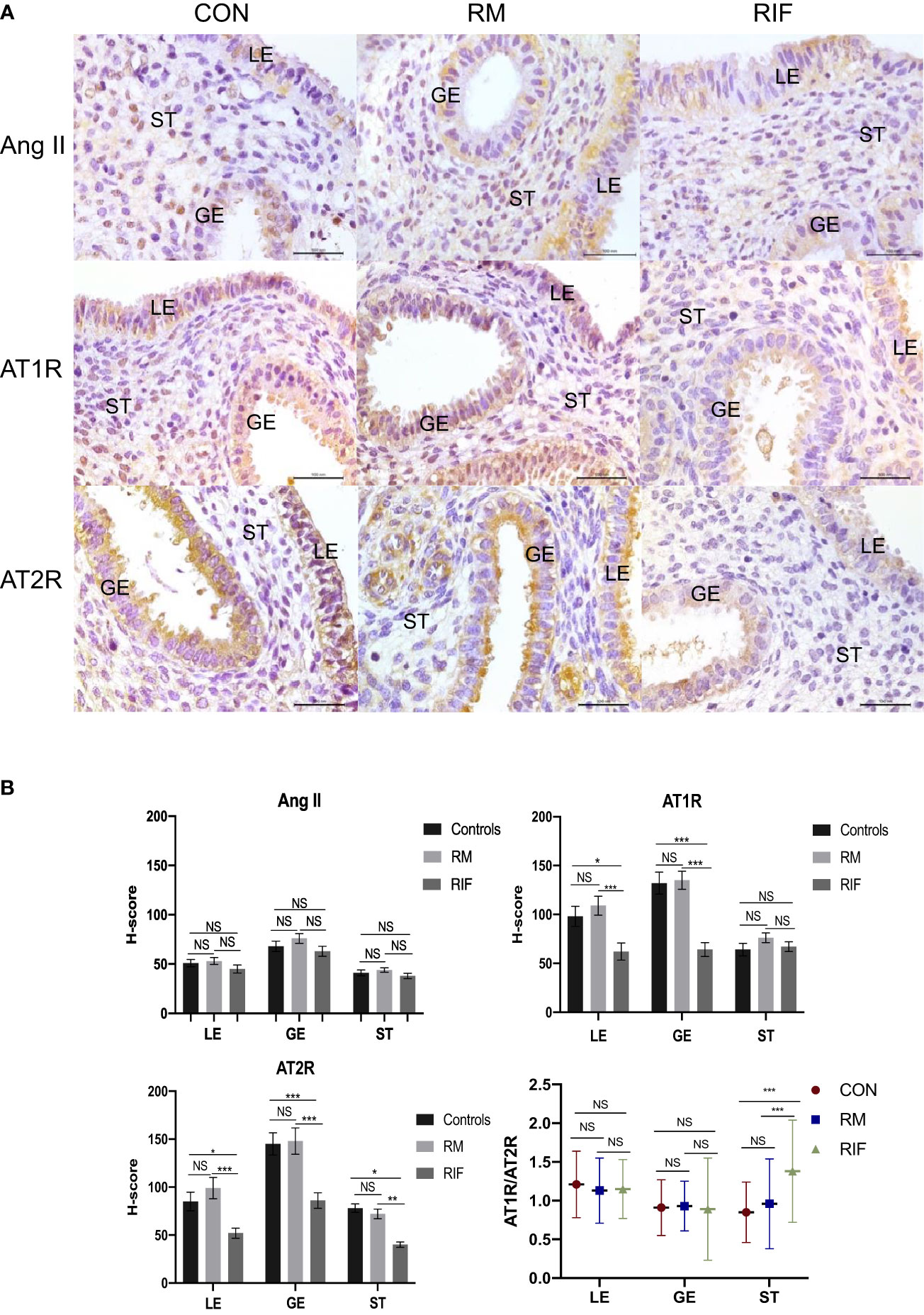
Figure 1 Ang II, AT1R, and AT2R expression in endometrium between fertile controls, RM and RIF. (A) Representative images of immunohistochemical staining of Ang II, AT1R, and AT2R among groups. LE, luminal epithelium; GE, glandular epithelium; ST, stroma cells. Scale bar=100 μm. (B) Corresponding quantitative analysis. Data are presented as mean ± SEM for AngII, AT1R, and AT2R and median (range) for stromal AT1R/AT2R expression ratios in all compartments of endometrium. *p < 0.05, ***p<0.001, NS, not significant.
The endometrium arteriole MVD in the stroma of women with RIF (25.0% decreased) was found to be significantly lower than that in the fertile controls and RM groups, but there was no significant difference between RM and fertile control group (Figures 2A, B). The endometrium capillary MVD was not significantly different among fertile controls, RM, and RIF. In addition, endometrium arteriole MVD (r= -0.419, p=0007), but not capillary MVD (r= -0.157, p=0.3334), in the stroma was significantly and negatively correlated with stromal AT1R/AT2R ratio in the endometrium (Figure 2C).
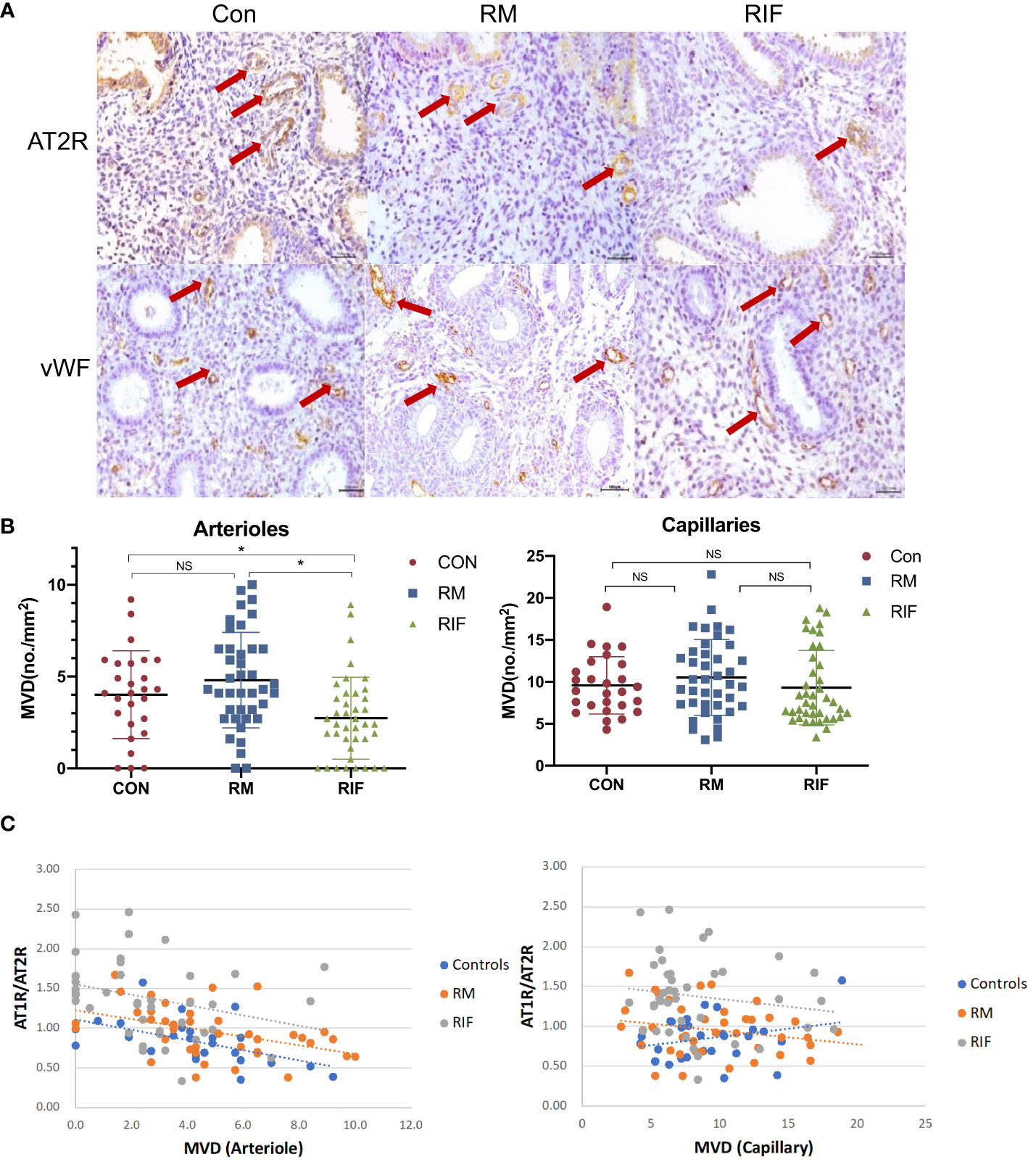
Figure 2 Arteriole and capillary density in endometrium between fertile controls, RM, and RIF. (A) Representative images of arterioles stained by AT2R and capillary stained by vWF (arrows) among groups. Scale bar = 100 μm. (B) Corresponding quantitative analysis. Data are presented as mean ± SEM. *p<0.05; NS, not significant. (C) Correlation analysis of stromal AT1R/AT2R expression ratio with arteriole density in control (r=-0.559, p=0.002), RM (r=-0.437, p=0.001) and RIF (r=-0.419, p=0.007), and its correlation with capillary density in control (r=0.256, p=0.197), RM (r=-0.227, p=0.158), and RIF (r=-0.157, p=0.334).
Comparisons between pregnant and non-pregnant women
There were no significant differences in hormonal profiles, endometrium thickness, and embryo quality (Table 1). Expression of both AT1R and AT2R was found significantly lower in all endometrium compartments (AT1R: LE, 41.2 lower; GE, 51.7% lower; ST, 36.6% lower. AT2R: LE, 49.0% lower; GE, 43.3% lower; ST,.54.5% lower) than the non-pregnant group when compared that with the pregnant group (Figures 3A, B), and AT1R/AT2R ratio was significantly higher in ST (39.4% higher), but not LE and GE, in the non-pregnancy group than in the pregnancy group (Figure 3C). Arteriole MVD was significantly lower in the non-pregnancy group and negatively correlated with stromal AT1R/AT2R ratio (r= -0.577, p=0.001).
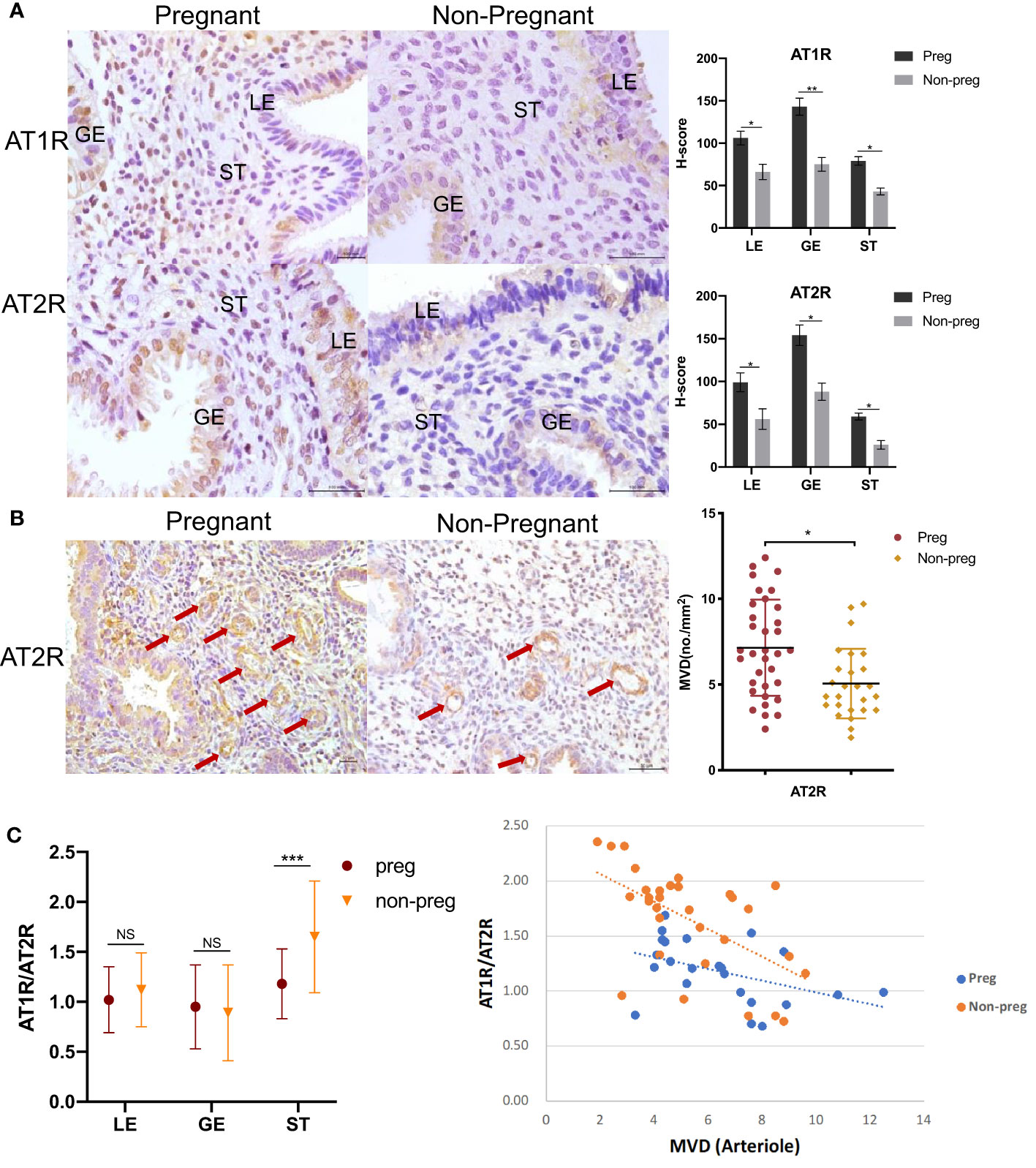
Figure 3 AT1R and AT2R expression in endometrium between women who did (Pregnant) and did not conceive (Non-pregnant) after euploid embryos transfer. (A) Representative images of immunohistochemical staining of AT1R and AT2R are shown on the left. LE=luminal epithelium; GE=glandular epithelium; ST=stroma cells. Scale bar=100 μm. Corresponding quantitative results between groups are shown on the right. Data are presented as mean ± SEM. (B) Representative images of arterioles stained by AT2R (arrows) are shown on the left. Scale bar=50 μm. Corresponding quantitative analysis between groups is shown on the right. Data are presented as mean ± SEM. (C) Comparison of the expression AT1R/AT2R ratio in all compartments of endometrium between the pregnant and non-pregnant groups is shown on the left. Data are presented as median (range). Correlation between stromal AT1R/AT2R ratio with arteriole density in the pregnant group (r=-0.438, p=0.036) and the non-pregnant group (r=-0.577, p=0.001) is shown on the right. *p<0.05, **p<0.01, ***p<0.001, NS, not significant.
Discussion
This is the first study to compare Ang II and its receptors in the endometrium of fertile control, RM, and RIF during the window of implantation and correlate with the pregnancy outcome after euploid embryo transfer. Our results showed that the expressions of AT1R and AT2R were significantly lower, with AT1R/AT2R ratio significantly higher in RIF and non-pregnancy groups. Additionally, arteriole MVD was significantly lower and negatively correlated with the stromal AT1R/AT2R ratio.
Being known as a cyclical tissue, the endometrium undergoes significant physiological changes of angiogenesis (47). Sufficient angiogenesis has been proven to be a beneficial factor to endometrial receptivity (48, 49), while endometrial vascularity was significantly reduced in women with unexplained subfertility during the mid-late secretory phase regardless of estradiol or progesterone concentrations and endometrial morphometry (50). In our study, differential expression of AT1R and AT2R in peri-implantation endometrium was found between RIF and control groups and between pregnancy and non-pregnancy groups. In addition, two receptors showed similar expression differences in endometrium between RM and RIF, but not between RM and control. It suggests a potential role of local Ang II receptors prior to, not after, embryo implantation. Despite AT1R and AT2R having opposite angiogenic regulatory functions in vessels, with AT1R playing a key role in vasoconstriction and oxidative stress (51, 52), whilst AT2R typically plays an opposing and protective role in such responses (53, 54). The counter-balancing vasomotor responses of AT1R and AT2R have been described (55). From our results, there is a positive correlation between the expressions of two receptors in the endometrium, indicating a synergistic regulation of Ang II receptors in the peri-implantation endometrium. The expressions of both AT1R and AT2R in RIF and non-pregnancy groups were significantly lower but the ratio of AT1R/AT2R was significantly higher compared to fertile women. The degree of the decreased expression of AT2R was more severe than that in AT1R, indicating potentially more vasoconstriction and blood flow restriction. Moreover, the implantation site is surrounded by large-diameter vessels, and the vessels closest to the embryo have more and wider average diameters compared to those further away from the implantation site. The blood flow through these dilated vessels near the implantation site was found to be sluggish, which rarely happens in vessels distant to the embryo (56). Therefore, an appropriate dynamic equilibrium between vasoconstrictors and vasodilators may be a prerequisite for vascular supply in the endometrium during the embryo implantation period, and an imbalance between the two receptors may result in implantation failure.
As from our results, while there is no significant difference in capillary MVD in endometrium between different subgroups of women, whereas there was significantly lower arterial MVD in RIF and non-pregnant women compared to controls. In addition, we found that the expression of stromal AT1R/AT2R and MVD was negatively correlated, which provided us with a deeper understanding of the regulation of angiogenesis in local endometrium. The relatively lower expression of AT1R compared with AT2R in stroma may cause vasodilation and dilated arterials may represent rich blood supply, which is necessary for endometrial receptivity. And the rich blood supply could also be a result of abundant MVD. While a significant negative correlation was observed between stromal AT1R/AT2R and endometrial arterial MVD, we still do not have sufficient evidence to tell the cause and effect between AT1R/AT2R and MVD in the endometrium stroma. The vasodilatation response is reported to be endothelium-dependent as well as NO-mediated (57). The complex regulatory networks mastering RAS include positive regulators, namely Wnt/β-catenin signaling (58, 59), (pro)renin receptor (60, 61), and PGE2/PGE2 receptor EP4 subtype (62); and negative regulators, such as vitamin D receptor, Klotho (63, 64), and a bunch of nuclear receptors including liver X receptor (LXR) (65), peroxisomeproliferator–activated receptors (66), and vitamin D receptor (VDR) (67). Therefore, endothelium cell function and its relationship with the NO signaling pathway in the endometrium angiogenesis and dynamic balance of the regulatory meditators on RAS activity should be further studied.
Conventional angiogenic markers for endometrial angiogenesis mainly focused on the VEGF family and angiopoietins (68, 69). Nevertheless, none of these angiogenic markers could reflect the vasoconstriction and vasodilation status of the vessels. By using AT2R as a surrogate marker of arterioles, not only it can determine the MVD, it may also indicate the arterial vascular tension but further studies are necessary. Small arterioles are responsible for the peripheral resistance in the vasculature, they can regulate blood flow by dilating or constricting the vascular smooth muscle (VSM) tone in response to stimuli (70). Thus by comparing AT1R/AT2R expression ratio, we could have a better understanding of the vascular tone in endometrium between different groups of women. Another strength of this study is that we include a cohort to compare the endometrium between pregnant and non-pregnant women after euploid embryos were transferred, given that embryo factors also contributed greatly to implantation failure (71, 72). Therefore, this cohort is an ideal model to explore implantation failure caused by endometrium factors exclusively without confounding factors from the embryo.
AII the endometrium in this study was collected precisely on the putative day of embryo implantation. Given that RAS was shown to undergo cyclic changes in the endometrium during the menstrual cycle (73, 74), collecting samples at precise timing is critical to our results. Besides, objective quantification through computer-assisted scoring techniques was used in this study to decrease misinterpretation of the results and increase standardization of RAS staining evaluation. However, there were several limitations in our study. First, immunohistochemistry is only semiquantitative, but it allows us to compare the distribution of angiogenetic markers in different endometrial compartments. Differential higher expression of Ang II and AT1R in epithelial cells than stromal cells, and abundant micro-vessels in stroma identified by AT2R expression in endothelium would not be possible using other techniques, such as quantitative real-time polymerase chain reaction or Western blot analysis in the bulk endometrium unless single-cell sequencing. The correlation between RAS expression and hormonal level is lacking as the sampling time of blood and endometrial biopsy was not the same. Moreover, in-depth study is needed to explore their molecular regulation and paracrine function of epithelium with stroma. Larger sample size studies are also needed to confirm whether AT1R and AT2R in endometrium could be used as predictive parameters for implantation outcomes.
In conclusion, expressions of AT1R and AT2R in peri-implantation endometrium were significantly lower and associated with lower arteriole MVD in women with RIF and poor IVF outcomes. The insufficient angiogenesis with the dysregulated RAS in endometrium from women with RIF may be involved in the cross-talk and synchronization between the endometrium and the embryo, consequently leading to poor implantation outcomes.
Data availability statement
The raw data supporting the conclusions of this article will be made available by the authors, without undue reservation.
Ethics statement
All procedures followed were in accordance with the ethical standards of the Responsible Committee on Human Experimentation (institutional and national) and with the Helsinki Declaration of 1964 and its later amendments. Informed consent was obtained from all the women who participated in the study. This study was approved by the Joint Chinese University of Hong Kong New Territories East Cluster Clinical Research Ethics Committee (CREC Ref. No. 2014.575) and Taiwan Mackay Memorial Hospital Institutional Review Board Approval of Clinical trial (IRB No. 18MMHIS070e).
Author contributions
RQ wrote the manuscript, RQ and YZ conducted the study and RQ, and CW analyzed the data, W-JY provided the clinical samples, TZ and JC offered clinical advice, all authors read and approved the manuscript.
Funding
The study was supported by General Research Fund from Research Grant Council: 14122021 and The Hong Kong Obstetrical and Gynaecological Trust Fund in 2020.
Acknowledgments
We acknowledge Prof. TC Li for their advice and support in this study. The work is derived from an original thesis RQ. Endometrial Renin-angiotensin System and Angiogenesis during Peri-implantation Period in Reproductive Failure and Pregnancy Outcomes. Ph.D. Chinese University of Hong Kong 2021.
Conflict of interest
The authors declare that the research was conducted in the absence of any commercial or financial relationships that could be construed as a potential conflict of interest.
Publisher’s note
All claims expressed in this article are solely those of the authors and do not necessarily represent those of their affiliated organizations, or those of the publisher, the editors and the reviewers. Any product that may be evaluated in this article, or claim that may be made by its manufacturer, is not guaranteed or endorsed by the publisher.
References
1. Semenza GL. Vasculogenesis, angiogenesis, and arteriogenesis: Mechanisms of blood vessel formation and remodeling. J Cell Biochem (2007) 102(4):840–7. doi: 10.1002/jcb.21523
2. Nardo LG. Vascular endothelial growth factor expression in the endometrium during the menstrual cycle, implantation window and early pregnancy. Curr Opin Obstet Gynecol (2005) 17(4):419–23. doi: 10.1097/01.gco.0000175362.12470.e0
3. Ni Y, May V, Braas K, Osol G. Pregnancy augments uteroplacental vascular endothelial growth factor gene expression and vasodilator effects. Am J Physiol-Heart Circul Physiol (1997) 273(2):H938–44. doi: 10.1152/ajpheart.1997.273.2.H938
4. Torry DS, Leavenworth J, Chang M, Maheshwari V, Groesch K, Ball ER, et al. Angiogenesis in implantation. J Assist Reprod Genet (2007) 24(7):303–15. doi: 10.1007/s10815-007-9152-7
5. Schild RL, Knobloch C, Dorn C, Fimmers R, van der Ven H, Hansmann M. Endometrial receptivity in an in vitro fertilization program as assessed by spiral artery blood flow, endometrial thickness, endometrial volume, and uterine artery blood flow. Fert Steril (2001) 75(2):361–6. doi: 10.1016/S0015-0282(00)01695-2
6. Friedler S, Schenker J, Herman A, Lewin A. The role of ultrasonography in the evaluation of endometrial receptivity following assisted reproductive treatments: a critical review. Hum Reprod Update (1996) 2(4):323–35. doi: 10.1093/humupd/2.4.323
7. Jinno M, Ozaki T, Iwashita M, Nakamura Y, Kudo A, HIrano H. Measurement of endometrial tissue blood flow: a novel way to assess uterine receptivity for implantation. Fert Steril (2001) 76(6):1168–74. doi: 10.1016/S0015-0282(01)02897-7
8. Achache H, Revel A. Endometrial receptivity markers, the journey to successful embryo implantation. Hum Reprod Update (2006) 12(6):731–46. doi: 10.1093/humupd/dml004
9. Chen X, Saravelos SH, Liu Y, Huang J, Wang CC, Li TC. Correlation between three-dimensional power Doppler and morphometric measurement of endometrial vascularity at the time of embryo implantation in women with unexplained recurrent miscarriage. J Mol Hist (2017) 48(3):235–42. doi: 10.1007/s10735-017-9722-7
10. Du J, Li FH, Fang H, Xia JG, Zhu CX. Correlation of real-time gray scale contrast-enhanced ultrasonography with microvessel density and vascular endothelial growth factor expression for assessment of angiogenesis in breast lesions. J Ultrasound Med (2008) 27(6):821–31. doi: 10.7863/jum.2008.27.6.821
11. Yoshimura Y, Karube M, Aoki H, Oda T, Koyama N, Nagai A, et al. Angiotensin II induces ovulation and oocyte maturation in rabbit ovaries via the AT2 receptor subtype. Endocrinology (1996) 137(4):1204–11. doi: 10.1210/endo.137.4.8625890
12. Speth RC, Daubert DL, Grove KL. Angiotensin II: a reproductive hormone too?1100 years of Renin.1. Regulat Peptides (1999) 79(1):25–40. doi: 10.1016/S0167-0115(98)00141-4
13. Khakoo AY, Sidman RL, Pasqualini R, Arap W. Does the renin-angiotensin system participate in regulation of human vasculogenesis and angiogenesis? Cancer Res (2008) 68(22):9112–5. doi: 10.1158/0008-5472.CAN-08-0851
14. Sasaki K, Murohara T, Ikeda H, Sugaya T, Shimada T, Shintani S, et al. Evidence for the importance of angiotensin II type 1 receptor in ischemia-induced angiogenesis. J Clin Invest (2002) 109(5):603–11. doi: 10.1172/JCI0213055
15. Zheng J, Bird IM, Chen D-B, Magness RR. Angiotensin II regulation of ovine fetoplacental artery endothelial functions: interactions with nitric oxide. J Physiol (2005) 565(1):59–69. doi: 10.1113/jphysiol.2004.082420
16. Lautt WW. Resistance or conductance for expression of arterial vascular tone. Microvas Res (1989) 37(2):230–6. doi: 10.1016/0026-2862(89)90040-X
17. Widdop RE, Vinh A, Henrion D, Jones ES. Vascular angiotensin at2 receptors in hypertension and ageing. Clin Expt Pharmacol Physiol (2008) 35(4):386–90. doi: 10.1111/j.1440-1681.2008.04883.x
18. Foulquier S, Dupuis F, Perrin-Sarrado C, Maguin Gaté K, Merhi-Soussi F, Liminana P, et al. High salt intake abolishes AT2-mediated vasodilation of pial arterioles in rats. J Hyperten (2011) 29(7):1392–9. doi: 10.1097/HJH.0b013e328347050e
19. Qi R, Li TC, Chen X. The role of the renin–angiotensin system in regulating endometrial neovascularization during the peri-implantation period: literature review and preliminary data. Ther Adv Endocrinol (2020) 11:2042018820920560. doi: 10.1177/2042018820920560
20. Reis FM, Bouissou DR, Pereira VM, Camargos AF, dos Reis AM, Santos RA. Angiotensin-(1-7), its receptor Mas, and the angiotensin-converting enzyme type 2 are expressed in the human ovary. Fertil Steril (2011) 95(1):176–81. doi: 10.1016/j.fertnstert.2010.06.060
21. Palumbo A, Ávila J, Naftolin F. The ovarian renin-angiotensin system (OVRAS): A major factor in ovarian function and disease. Reprod Sci (2016) 23(12):1644–55. doi: 10.1177/1933719116672588
22. Hagemann A, Nielsen AH, Poulsen K. The uteroplacental renin-angiotensin system: a review. Exp Clin Endocrinol Diabetes (1994) 102(3):252–61. doi: 10.1055/s-0029-1211289
23. Cooper AC, Robinson G, Vinson GP, Cheung WT, Broughton Pipkin F. The localization and expression of the renin–angiotensin system in the human placenta throughout pregnancy. Placenta (1999) 20(5):467–74. doi: 10.1053/plac.1999.0404
24. Kuo TC, Endo K, Dharmarajan AM, Miyazaki T, Atlas SJ, Wallach EE. Direct effect of angiotensin II on in-vitro perfused rabbit ovary. Reproduction (1991) 92(2):469–74. doi: 10.1530/jrf.0.0920469
25. Acosta TJ, Berisha B, Ozawa T, Sato K, Schams D, Miyamoto A. Evidence for a local endothelin-angiotensin-atrial natriuretic peptide systemin bovine mature follicles in vitro: effects on steroid hormones and prostaglandin secretion. Biol Reprod (1999) 61(6):1419–25. doi: 10.1095/biolreprod61.6.1419
26. Lightman A, Tarlatzis BC, Rzasa PJ, Culler MD, Caride VJ, Negro-Vilar AF, et al. The ovarian renin-angiotensin system: Renin-like activity and angiotensin II/III immunoreactivity in gonadotropin-stimulated and unstimulated human follicular fluid. Am J Obstet Gynecol (1987) 156(4):808–16. doi: 10.1016/0002-9378(87)90336-X
27. Tebbs C, Pratten MK, Pipkin FB. Angiotensin II is a growth factor in the peri-implantation rat embryo. J Anat (1999) 195(1):75–86. doi: 10.1046/j.1469-7580.1999.19510075.x
28. Pijacka W, Hunter MG, Pipkin FB, Luck MR. Expression of renin–angiotensin system components in the early bovine embryo. Endocrine Connections (2012) 1(1):22–30. doi: 10.1530/EC-12-0013
29. Herr D, Rodewald M, Fraser HM, Hack G, Konrad R, Kreienberg R, et al. Regulation of endothelial proliferation by the renin–angiotensin system in human umbilical vein endothelial cells. Reproduction (2008) 136(1):125–30. doi: 10.1530/REP-07-0374
30. Pringle KG, Tadros MA, Callister RJ, Lumbers ER. The expression and localization of the human placental prorenin/renin-angiotensin system throughout pregnancy: Roles in trophoblast invasion and angiogenesis? Placenta (2011) 32(12):956–62. doi: 10.1016/j.placenta.2011.09.020
31. Delforce SJ, Wang Y, Van-Aalst ME, Corbisier de Meaultsart C, Morris BJ, Broughton-Pipkin F, et al. Effect of oxygen on the expression of renin–angiotensin system components in a human trophoblast cell line. Placenta (2016) 37:1–6. doi: 10.1016/j.placenta.2015.11.011
32. Wang Y, Lumbers ER, Sykes SD, Pringle KG. Regulation of the renin—Angiotensin system pathways in the human decidua. Reprod Sci (2015) 22(7):865–72. doi: 10.1177/1933719114565029
33. Galgani M, Insabato L, Calì G, Della Gatta AN, Mirra P, Papaccio F, et al. Regulatory T cells, inflammation, and endoplasmic reticulum stress in women with defective endometrial receptivity. Fertil Steril (2015) 103(6):1579–1586.e1. doi: 10.1016/j.fertnstert.2015.03.014
34. Russell P, Anderson L, Lieberman D, Tremellen K, Yilmaz H, Cheerala B, et al. The distribution of immune cells and macrophages in the endometrium of women with recurrent reproductive failure: I: Techniques. J Reprod Immunol (2011) 91(1):90–102. doi: 10.1016/j.jri.2011.03.013
35. Liu S, Wei H, Li Y, Huang C, Lian R, Xu J, et al. Downregulation of ILT4+ dendritic cells in recurrent miscarriage and recurrent implantation failure. Am J Reprod Immunol (2018) 80(4):e12998. doi: 10.1111/aji.12998
36. Diaz-Nuñez M, Rabanal A, Expósito A, Ferrando M, Quintana F, Soria JM, et al. Recurrent miscarriage and implantation failure of unknown cause studied by a panel of thrombophilia conditions: increased frequency of FXIII val34Leu polymorphism. J Reprod Infertil (2019) 20(2):76–82.
37. Yang Y, Chen X, Saravelos SH, Liu Y, Huang J, Zhang J, et al. HOXA-10 and E-cadherin expression in the endometrium of women with recurrent implantation failure and recurrent miscarriage. Fertil Steril (2017) 107(1):136–143.e2. doi: 10.1016/j.fertnstert.2016.09.016
38. Laird S, Tuckerman E, Li T-C. Cytokine expression in the endometrium of women with implantation failure and recurrent miscarriage. Reprod BioMed Online (2006) 13(1):13–23. doi: 10.1016/S1472-6483(10)62011-1
39. Quenby S, Vince G, Farquharson R, Aplin J. Recurrent miscarriage: a defect in nature’s quality control? Hum Reprod (2002) 17(8):1959–63. doi: 10.1093/humrep/17.8.1959
40. Teklenburg G, Salker M, Heijnen C, Macklon NS, Brosens JJ. The molecular basis of recurrent pregnancy loss: impaired natural embryo selection. Mol Hum Reprod (2010) 16(12):886–95. doi: 10.1093/molehr/gaq079
41. Rai R, Regan L. Recurrent miscarriage. Lancet (2006) 368(9535):601–11. doi: 10.1016/S0140-6736(06)69204-0
42. Coughlan C, Ledger W, Wang Q, Liu F, Demirol A, Gurgan T, et al. Recurrent implantation failure: definition and management. Reprod BioMed Online (2014) 28(1):14–38. doi: 10.1016/j.rbmo.2013.08.011
43. Noyes RW, Hertig AT, Rock J. Dating the endometrial biopsy. Fertil Steril (1950) 1(1):3–25. doi: 10.1016/S0015-0282(16)30062-0
44. Mints M, Blomgren B, Falconer C, Palmblad J. Expression of the vascular endothelial growth factor (VEGF) family in human endometrial blood vessels. Scand J Clin Lab Invest (2002) 62(3):167–75. doi: 10.1080/003655102317475425
45. Borre M, Offersen BV, Nerstrøm B, Overgaard J. Microvessel density predicts survival in prostate cancer patients subjected to watchful waiting. Br J Cancer (1998) 78(7):940–4. doi: 10.1038/bjc.1998.605
46. Kini AR, Kay NE, Peterson LC. Increased bone marrow angiogenesis in B cell chronic lymphocytic leukemia. Leukemia (2000) 14(8):1414–8. doi: 10.1038/sj.leu.2401825
47. Smith SK. Regulation of angiogenesis in the endometrium. Trends Endocrinol Metabol (2001) 12(4):147–51. doi: 10.1016/S1043-2760(01)00379-4
48. Lebovitz O, Orvieto R. Treating patients with “thin” endometrium – an ongoing challenge. Gynecol Endocrinol (2014) 30(6):409–14. doi: 10.3109/09513590.2014.906571
49. Yang J-H, Chen C-D, Chou C-H, Wen W-F, Tsao P-N, Lee H, et al. Intentional endometrial injury increases embryo implantation potentials through enhanced endometrial angiogenesis†. Biol Reprod (2019) 100(2):381–9. doi: 10.1093/biolre/ioy205
50. Raine-Fenning NJ, Campbell BK, Kendall NR, Clewes JS, Johnson IR. Endometrial and subendometrial perfusion are impaired in women with unexplained subfertility. Hum Reprod (2004) 19(11):2605–14. doi: 10.1093/humrep/deh459
51. Zhou Y, Chen Y, Dirksen Wessel P, Morris M, Periasamy M. AT1b receptor predominantly mediates contractions in major mouse blood vessels. Circul Res (2003) 93(11):1089–94. doi: 10.1161/01.RES.0000101912.01071.FF
52. Zhang H, Schmeisser A, Garlichs CD, Plötze K, Damme U, Mügge A, et al. Angiotensin II-induced superoxide anion generation in human vascular endothelial cells: role of membrane-bound NADH-/NADPH-oxidases. Cardiovasc Res (1999) 44(1):215–22. doi: 10.1016/s0008-6363(99)00183-2
53. Widdop RE, Jones ES, Hannan RE, Gaspari TA. Angiotensin AT2 receptors: cardiovascular hope or hype? Br J Pharmacol (2003) 140(5):809–24. doi: 10.1038/sj.bjp.0705448
54. Matrougui K, Loufrani L, Heymes C, Lévy BI, Henrion D. Activation of AT2 receptors by endogenous angiotensin II is involved in flow-induced dilation in rat resistance arteries. Hypertension (1999) 34(4):659–65. doi: 10.1161/01.HYP.34.4.659
55. Cosentino F, Savoia C, Paolis PD, Francia P, Russo A, Maffei A, et al. Angiotensin II type 2 receptors contribute to vascular responses in spontaneously hypertensive rats treated with angiotensin II type 1 receptor antagonists*. Am J Hyperten (2005) 18(4):493–9. doi: 10.1016/j.amjhyper.2004.11.007
56. Tawia SA, Rogers P. In vivo microscopy of the subepithelial capillary plexus of the endometrium of rats during embryo implantation. Reproduction (1992) 96(2):673–80. doi: 10.1530/jrf.0.0960673
57. Thijssen DHJ, Black MA, Pyke KE, Padilla J, Atkinson G, Harris RA, et al. Assessment of flow-mediated dilation in humans: a methodological and physiological guideline. Am J Physiol-Heart Circul Physiol (2010) 300(1):H2–12. doi: 10.1152/ajpheart.00471.2010
58. Zhou L, Liu Y. Wnt/β-catenin signaling and renin-angiotensin system in chronic kidney disease. Curr Opin Nephrol Hypertens (2016) 25(2):100–6. doi: 10.1097/MNH.0000000000000205
59. Zhou L, Li Y, Hao S, Zhou D, Tan RJ, Nie J, et al. Multiple genes of the renin-angiotensin system are novel targets of wnt/β-catenin signaling. JASN (2015) 26(1):107–20. doi: 10.1681/ASN.2014010085
60. Burcklé C, Bader M. Prorenin and its ancient receptor. Hypertension (2006) 48(4):549–51. doi: 10.1161/01.HYP.0000241132.48495.df
61. Cruciat C-M, Ohkawara B, Acebron SP, Karaulanov E, Reinhard C, Ingelfinger D, et al. Requirement of prorenin receptor and vacuolar H+-ATPase–mediated acidification for wnt signaling. Science (2010) 327(5964):459–63. doi: 10.1126/science.1179802
62. Pöschke A, Kern N, Maruyama T, Pavenstädt H, Narumiya S, Jensen BL, et al. The PGE2-EP4 receptor is necessary for stimulation of the renin-angiotensin-aldosterone system in response to low dietary salt intake in vivo. Am J Physiol-Renal Physiol (2012) 303(10):F1435–42. doi: 10.1152/ajprenal.00512.2011
63. Hu MC, Kuro-o M, Moe OW. Klotho and chronic kidney disease. Phosphate and vitamin D in chronic. Kidney Dis (2013) 180:47–63. doi: 10.1053/j.ajkd.2019.01.029
64. Kuro-o M, Matsumura Y, Aizawa H, Kawaguchi H, Suga T, Utsugi T, et al. Mutation of the mouse klotho gene leads to a syndrome resembling ageing. Nature (1997) 390(6655):45–51. doi: 10.1038/36285
65. Lu X, Wang F, Xu C, Soodvilai S, Peng K, Su J, et al. Soluble (pro)renin receptor via β-catenin enhances urine concentration capability as a target of liver X receptor. PNAS (2016) 113(13):E1898–906. doi: 10.1073/pnas.1602397113
66. Zhang H, Zhang A, Kohan DE, Nelson RD, Gonzalez FJ, Yang T. Collecting duct-specific deletion of peroxisome proliferator-activated receptor γ blocks thiazolidinedione-induced fluid retention. PNAS (2005) 102(26):9406–11. doi: 10.1073/pnas.0501744102
67. Li YC, Kong J, Wei M, Chen Z-F, Liu SQ, Cao L-P. 1,25-Dihydroxyvitamin D3 is a negative endocrine regulator of the renin-angiotensin system. J Clin Invest (2002) 110(2):229–38. doi: 10.1172/JCI0215219
68. Smith S. Angiogenesis, vascular endothelial growth factor and the endometrium. Hum Reprod Update (1998) 4(5):509–19. doi: 10.1093/humupd/4.5.509
69. Di Carlo C, Bonifacio M, Tommaselli GA, Bifulco G, Guerra G, Nappi C. Metalloproteinases, vascular endothelial growth factor, and angiopoietin 1 and 2 in eutopic and ectopic endometrium. Fertil Steril (2009) 91(6):2315–23. doi: 10.1016/j.fertnstert.2008.03.079
70. Carlson BE, Secomb TW. A theoretical model for the myogenic response based on the length–tension characteristics of vascular smooth muscle. Microcirculation (2005) 12(4):327–38. doi: 10.1080/10739680590934745
71. Patrizio P, Bianchi V, Lalioti MD, Gerasimova T, Sakkas D. High rate of biological loss in assisted reproduction: it is in the seed, not in the soil. Reprod BioMed Online (2007) 14(1):92–5. doi: 10.1016/S1472-6483(10)60769-9
72. Simon A, Laufer N. Repeated implantation failure: clinical approach. Fertil Steril (2012) 97(5):1039–43. doi: 10.1016/j.fertnstert.2012.03.010
73. Janowsky DS, Berens SC, Davis JM. Correlations between mood, weight, and electrolytes during the menstrual cycle: A renin-angiotensin-aldosterone hypothesis of premenstrual tension. Psychoso Med (1973) 35(2):143–54. doi: 10.1097/00006842-197303000-00007
Keywords: endometrium, receptivity, renin angiotensin system, angiogenesis, implantation
Citation: Qi R, Zhang T, Zhang Y, Chung JPW, Yang WJ and Wang CC (2023) Association of angiotensin II and receptors in peri-implantation endometrium with microvessel density and pregnancy outcomes of women with recurrent implantation failure after embryo transfer. Front. Endocrinol. 14:1206326. doi: 10.3389/fendo.2023.1206326
Received: 15 April 2023; Accepted: 31 July 2023;
Published: 29 August 2023.
Edited by:
Zhiqin Bu, Zhengzhou University, ChinaCopyright © 2023 Qi, Zhang, Zhang, Chung, Yang and Wang. This is an open-access article distributed under the terms of the Creative Commons Attribution License (CC BY). The use, distribution or reproduction in other forums is permitted, provided the original author(s) and the copyright owner(s) are credited and that the original publication in this journal is cited, in accordance with accepted academic practice. No use, distribution or reproduction is permitted which does not comply with these terms.
*Correspondence: Wen-Jui Yang, molinda11@yahoo.com.tw; Chi Chiu Wang, ccwang@cuhk.edu.hk
†ORCID: Ruofan Qi, orcid.org/0000-0002-4593-0530
Tao Zhang, orcid.org/0000-0002-8644-8468
Jacqueline Pui Wah Chung, orcid.org/0000-0002-9742-578X
Chi Chiu Wang, orcid.org/0000-0002-3928-7278