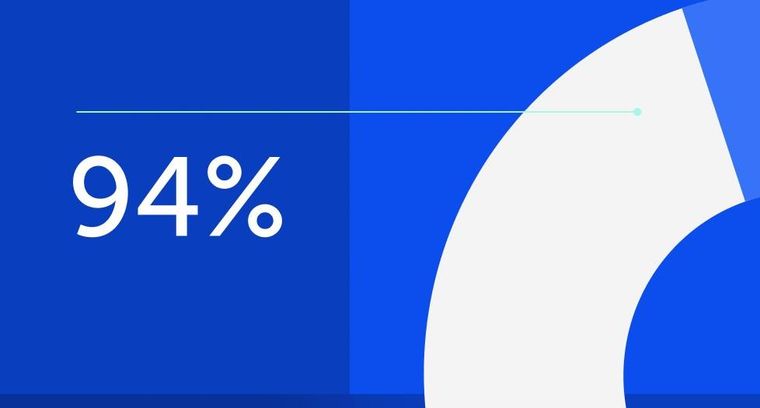
94% of researchers rate our articles as excellent or good
Learn more about the work of our research integrity team to safeguard the quality of each article we publish.
Find out more
ORIGINAL RESEARCH article
Front. Endocrinol., 01 August 2023
Sec. Cellular Endocrinology
Volume 14 - 2023 | https://doi.org/10.3389/fendo.2023.1205977
This article is part of the Research TopicGenetic and Molecular Determinants in Bone Health and DiseasesView all 9 articles
Introduction: Hypophosphatasia (HPP) is a rare genetic disease caused by inactivating variants of the ALPL gene. Few data are available on the clinical presentation in Italy and/or on Italian HPP surveys.
Methods: There were 30 suspected HPP patients recruited from different Italian tertiary cares. Biological samples and related clinical, biochemical, and anamnestic data were collected and the ALPL gene sequenced. Search for large genomic deletions at the ALPL locus (1p36) was done. Phylogenetic conservation and modeling were applied to infer the effect of the variants on the protein structure.
Results: There were 21 ALPL variants and one large genomic deletion found in 20 out of 30 patients. Unexpectedly, NGS-driven differential diagnosis allowed uncovering three hidden additional HPP cases, for a total of 33 HPP subjects. Eight out of 24 coding variants were novel and classified as “pathogenic”, “likely pathogenic”, and “variants of uncertain significance”. Bioinformatic analysis confirmed that all the variants strongly destabilize the homodimer structure. There were 10 cases with low ALP and high VitB6 that resulted negative to genetic testing, whereas two positive cases have an unexpected normal ALP value. No association was evident with other biochemical/clinical parameters.
Discussion: We present the survey of HPP Italian patients with the highest ALPL mutation rate so far reported and confirm the complexity of a prompt recognition of the syndrome, mostly for HPP in adults. Low ALP and high VitB6 values are mandatory for the genetic screening, this latter remaining the gold standard not only to confirm the clinical diagnosis but also to make differential diagnosis, to identify carriers, to avoid likely dangerous therapy in unrecognized cases.
Hypophosphatasia (HPP, MIM #146300) is a rare genetic disorder affecting bone and teeth mineralization with multisystemic manifestations involving the nervous system, musculoskeletal apparatus, and kidneys (1). HPP is due to inactivating variants of the ALPL gene (NM_000478), encoding the TNSALP (tissue non-specific alkaline phosphatase), which determine a decrease up to the total loss of corresponding enzymatic activity (2).
While the primary manifestations of HPP are directly related to a deficient mineralization of bones and teeth (e.g., lack of or retarded ossification, fractures, bone deformities, caries, teeth fragility and loss), a plethora of additional manifestations, e.g., respiratory distress, muscle hypotonia, irritability, seizures, hypercalcemia/hypercalciuria, myalgias, arthralgias, and chondro- and nephrocalcinosis, define a spectrum of different severity depicted through the six phenotypic presentations, from the lethal “in utero” to the mildest form diagnosed in adults (1). Instead, the deficit of the TNSALP enzymatic activity causes the accumulation of three main corresponding substrates: pyrophosphate (PPi) in the extracellular spaces and pyridoxal-5′-phosphate (PLP) and phosphoethanolamine (PEA) in urine (3, 4). PPi is a strong inhibitor of the mineralization process, and its effect is paradigmatic of the primary HPP manifestations. At the neuronal level, in perinatal and infantile forms, HPP manifests with seizures due to the deficit of vitamin B6 (VitB6), which represent the dephosphorylated (i.e., active) PLP form (1). On the contrary, adult HPPs are characterized by mild symptoms such as stress fractures with delayed healing, periodontitis, and/or early loss of deciduous teeth and chondrocalcinosis due to calcium pyrophosphate deposition (1). With regard to PEA, whereas it is well known it accumulates in serum and urine, its specific metabolism is less evident as well as the effect of its accumulation on muscle function (4).
To date, more than 400 different deleterious (missense, nonsense, splicing, frameshift) ALPL variants have been identified, scattered throughout the 1,572-bp coding sequence (5), located at chromosome 1p36.12. Moreover, 14 large genomic deletions involving one or more exons or the whole genomic region encompassing the ALPL locus, have been reported (5), suggesting that large genomic deletions represent a less frequent mutational event of HPP.
HPP is transmitted in both autosomal dominant and autosomal recessive inheritances at the same locus. Variability is marked between and within families, especially in the autosomal dominant form, which is usually attributed to variants with the dominant negative effect (DNE, 6). In addition, the separation of inheritance pattern is not always clear-cut, with families ascertained by an index case with bi-allelic deleterious variants and minimal or mild manifestations in the “carrier” parents, who remained undiagnosed for decades. Inheritance pattern heterogeneity, the still high rate of variants of unknown significance (VUS) in ALPL and the marked variability in clinical expression make HPP a challenge for the health professionals (7).
Here, we present our experience of the last 5 years (March 2017–August 2022) with a cohort of 33 HPP patients recruited in different Italian tertiary clinical cares.
The Division of Medical Genetics, Fondazione IRCCS Casa Sollievo della Sofferenza, includes a regional referral center for hereditary bone metabolism disorders. The laboratory receives requests for genetic analysis from different Italian tertiary care centers. For the current study, cases were recruited from five pediatric (RF, TF, CG, ES, PV), three endocrinology (ASS, AG, RMM/SC), and four bone metabolism (FP/CB/AS, FC, SC/GG, MCe) referral centers for adults, two medical genetic (EG/SM, MC) units. From March 2017 to August 2022, 30 index individuals were enrolled for the study after preliminary biochemistry assessment confirmed the ALP value lower than the minimum range and measured at least twice and the VitB6 level higher than the maximum range (7–18 μg/l). Exclusion criteria were the assumption of drugs affecting bone turnover (i.e., bisphosphonates, denosumab) and/or diseases associated with low ALP activity/Vit B6 value (i.e., hypomagnesaemia, hypozincemia, celiac disease, Wilson disease, hypothyroidism, hypoparathyroidism, assumption of multivitamin supplements).
Three additional patients (#21, #22, and #23) underwent a next-generation sequencing (NGS) multigene panel analysis sequencing for hereditary disorders leading to early-onset osteoporosis/bone fragility and were included in the final survey after the identification of an ALPL variant: (i) subject #21 was a male newborn with fractures in the absence of trauma at 30 days of life with a suspicion of “shaken baby” syndrome; (ii) subject #22 was a boy of 2 years and 10 months, with an initial diagnosis of EDS/bone fragility; (iii) patient #23, was an adult man of 65 years, with persistent osteopenia and fractures. Unexpectedly, subjects (#21) and (#23) showed a normal (repeatedly) ALP value (Table 1).
Totally, 48.4% of the subjects were from South Italy, 27.2% from the Islands (Sardinia and Sicily), and 24.4% from North-Middle Italy. The sex ratio was F/M = 1.72 and median age = 51.8 +/- 19.4 years. Clinical, biochemical, and anamnestic data were collected.
DNA was extracted from blood withdrawal, and the classic Sanger method was performed on 25 (75.7%) out of the 33 patients: DNA was PCR amplified, and amplicons were purified (ExoSAP-IT, Affymetrix, Thermo Fisher) and sequenced (Big Dye Terminator Cycle Sequencing Kit v 1.1, ABI3130XL Sequencer, Thermo Fisher) for the 12 coding exons (Supplementary Material 1 for primers and PCR cycling conditions).
Eight patients (24.3%) were screened through a SureSelect gene panel (Agilent Technologies, USA) designed to capture known genes associated with calcium metabolism disorders and bone fragility, including ANO5, ALPL, B3GALT6, B4GALT7, BMP1, COL1A1, COL1A2, CREB3L1, CRTAP, DKK1, EN1, FAM46A, FKBP10, FKBP14, IFITM5, LRP5, MBTPS2, P3H1, P4HB, PLOD1, PLOD2, PLOD3, PLS3, PPIB, SEC24D, SERPINF1, SERPINH1, SLC39A13, SPARC, SP7, TMEM38B, WNT1, and YY1AP1.
Targeted fragments were sequenced on a MiSeq platform (Illumina, USA) with MiSeq Reagent kit V3 300-cycle flow cells. Data analysis was performed considering the frequency, impact on the encoded protein, conservation, and expression of variants using distinct tools [ANNOVAR (9), dbSNP (10), 1000 Genomes (11), ExAC (12)]. Selected variants were interpreted according to the American College of Medical Genetics and Genomics/Association for Molecular Pathology (ACMGG/AMP) (13). Variants were confirmed by Sanger sequencing and segregation investigation performed on available relatives.
MLPA Kit (P484-ALPL, MRC Holland) was initially applied on negative subjects and then enlarged to the whole cohort, and it was carried out following the manufacturer’s instructions.
The level of conservation of the protein sequence was evaluated for the novel missense variants. From the Ensembl Genome Browser (14), the TNSALP protein orthologous sequences of the following organisms were downloaded: Homo, Gorilla, Pan, Mus, Bos, Xaenopus, Tetraodon, Danio. Then, Clustalw (15) was used to create the multi-alignment.
Atomic coordinates of TNSALP protein were obtained through AlphaFold v.2.0 (16). We retrieved the ALPL protein sequence in FASTA format from the Ensembl Genome Browser (14) and submitted it to ColabFold (17), which could predict the complex folding using the specialized AlphaFold-multimer model.
Then, molecular dynamics (MD) simulation was employed to refine the obtained models. Both the monomer and TNSALP dimer were embedded into a simulation box, extending up to 12 Å, using the tleap module of AmberTools21 (18), and solvated with the TIP3P water model and an appropriate number of Na+ and Cl- counter ions. The Amber ff14SB force field was applied to amino acids. Each model was subjected to energy minimization using steepest descent, followed by conjugate gradient methods. Then, they were gradually heated and equilibrated for approximately 5 ns, by time steps of 1 fs. A 10-Å cutoff was used for non-bonded short-range interactions, whereas long-range electrostatics were treated with the particle-mesh Ewald method. Temperature and pressure were maintained at 300 K and 101.3 kPa, respectively, using the Langevin dynamics and Piston method (19).
Following these preliminary steps, we implemented the Gaussian-accelerated molecular dynamics (GaMD), an enhanced molecular dynamic sampling method that works by providing a harmonic boost potential, which follows a Gaussian distribution, to smooth the system potential energy surface, thus causing the decrease of local energy barriers and accelerating the transitions between low-energy states. The boost potential can be applied in a dual-boost scheme: (i) a dihedral potential boost and (ii) a total potential boost. Maximum, minimum, average, and standard deviation values of the system potential were obtained from an initial ~2 ns conventional simulation with no boost potential, followed by a further GaMD equilibration of ~50 ns in which the boost potential was updated every 1.6 ns, thus reaching the equilibrium. Thus, each system was simulated for 100 ns using a timestep of 2 fs.
Finally, the stability of multiple TNSALP variants, p.(Ser181Leu), p.(Tyr28Asp), p.(Val95Met), p.(His267Pro), p.(His472Arg), p.(Met219Ile), p.(Ala179Thr), p.(Ala33Val), p.(Arg223Gln), (p.Arg391His), p.(Asp109Glu), p.(Gln207Pro), p.(Glu235Gly), p.(Glu298Lys), p.(Glu84Lys), and p.(Glu88Lys), and the double mutant p.(Arg136His)-p.(Phe290Leu), was investigated thermodynamically through the BuildModel and Stability function implemented in the FoldX algorithm (20). It was run with standard parameters. The same workflow was employed to evaluate the stop loss p.(*525Argfs*11) but using ColabFold to model the structure of both the monomer and dimer containing an additional residue fragment.
The original values of ALP were measured for non-uniform ranges; therefore, to make them comparable, the “ALP percentage deviation” = [(lower ALP value (for the corresponding reference interval)) - ALP)/lower ALP value (for the corresponding reference interval) x 100] was calculated. Analysis was performed with R (packages: stats, ggplot2, plyr, dbplyr, dplyr) (21–25).
Apart from early-onset cases or the ones that were resolved through the NGS-differential diagnosis (#21, #22 and #23), the remaining patients were firstly admitted for clinical problems related to bone pain, myalgia, fractures, nephrolithiasis, or early-onset or post-menopausal osteoporosis screening. This biased sample selection influenced the overall clinical spectrum of the cohort; instead, around 48% of patients claimed osteoporosis/low bone mass whereas 27% showed fractures at different sites. However, it is important to highlight that the whole cohort was then enrolled only after the biochemistry showed low ALP and high VitB6 (Table 2).
From a total of 30 index individuals, we identified one or two single-nucleotide variants in 19 and a single-exon deletion (exon 2, Figure 1) in an additional individual, for a total of 20 cases (66.7%). To this cohort, we added three additional cases (#21-23), who received the diagnosis of HPP after the NGS multigene panel testing identified a likely pathogenic ALPL variant. Totally, 21 cases were heterozygous and two resultant compounds were heterozygous, a finding compatible with autosomal recessive inheritance (cases #5 and #10). Of the 25 identified mutated alleles (i.e., 21 heterozygous plus 2 compound heterozygous), we identified 22 different variants (21 single-nucleotide variants and 1, exon 2, deletion). Accordingly, 18 were private whereas 3 (i.e., c.262G>A, c.327C>A, and c.668G>A) recurred in 2 apparently unrelated pedigrees/index individuals. The 21 single occurrences were distributed as follows: 16 were missense, 3 frameshift, with the presumed insertion of a premature stop codon, 1 stop loss with the presumed insertion of 11 additional residues, 1 stop gain. Eight variants out of 21 (38%) were novel since they were not reported in the official database (5) (last release, March 2023). The Franklin online genomic variant interpretation tool (26) was used to determine the pathogenicity level of the eight novel changes, which resulted in one pathogenic, five likely pathogenic, and two VUS, following the ACMGG guidelines (13) (Table 1).
There were 12 out of 23 mutated cases (52%) found to be familial, although for others, a clinical in-depth study or segregation analysis was not available, or yet concluded at the time of this report (Figure 2). Interestingly, 10 (out 30, 33.3%) subjects resulted to be negative, although at least for one case (#29) familiarity for low ALP value and clinical symptoms was reported.
Due to the different assays and normal ranges used in the tertiary cares, we decided to plot the percentage deviation of the ALP value from the minimum value versus the presence/absence of the ALPL mutation. The correlation between the presence of a deleterious ALPL variant and the corresponding ALP serum value was, then, resolved in the boxplot in Figure 3: mutated patients showed an ALP value that was from 11 up to 49% lower than the minimum.
Figure 3 Boxplot showing the correlation between the presence of the ALPL mutation and the percentage deviation of the ALP value to the minimum range.
Other biochemical parameters (Ca, PTH, P, VitD, and Cr) were collected, but no association was found with the presence/absence of an ALPL variant, nor with the ALP value.
Several SNPs were found among positive and negative patients (Supplementary Material 2). p.Arg152His is defined in ClinVar (8) as benign, with a MAF = 1.5%, in gnomAD. However, in our cohort, it was found in three subjects (9%), two of them carrying a pathogenic variant, with an overall frequency ~6 times higher than expected (Supplementary Material 2).
The multi-alignment of the Alpl ortholog clearly showed that the novel missense variants affect residues conserved up to the amphibians, or up to the fishes, strongly supporting that they could have a deleterious effect on the protein function (Figure 4).
Figure 4 Phylogenetic multialignment showing the conservation level of the aminoadic sequence surrounding the novel changes, in different orthologous. Residues mutated are in red and show a high conservation up to Fishes.
FoldX computed the total energy of ALPL dimers, wild-type and mutated, as a proxy of their overall stability and the van der Waals inter-residue clashes, as energy penalization factors. The free energy calculations are reported in Table 3 and Figure 5. The difference in free-energy ΔΔG (ΔGmt – ΔGwt) for the majority of the ALPL amino acid changes was in the range to classify them as destabilizing whether only one or both monomers in the dimeric complex were mutated. In detail, p.(Asp109Glu), p.(His472Arg), p.(Met219Ile), p.(Ser181Leu), p.(Ala179Thr), p.(Arg391His), p.(Glu84Lys), and p.(Tyr28Asp) were observed as destabilizing whereas p.(Gln207Pro), p.(His267Pro), p.(Ala33Val), and p.(Arg223Gln) and the double mutant p.(Arg136His)-p.(Phe290Leu) could be classified as highly destabilizing. Conversely, p.(Glu88Lys) could be classified as stabilizing.
Figure 5 3D structure of ALPL dimer obtained through molecular modeling. Mutations were mapped on the wild-type structure and highlighted in red.
p.(Glu298Lys) exhibited stabilizing effects when only one monomer was mutated; however, this alteration was compensated in the double-mutated dimer; on the contrary, p.(Val95Met) showed a destabilizing effect in the mutant monomer but a stabilizing effect with two mutations. Finally, p.(Glu235Gly) did not exhibit any structural impact on the ALPL protein.
A different approach was applied to the stop loss p.(*525Argfs*11), where the additional residue fragment in the protein structure could have a putative impact on the overall folding. Comparing the stability of both the monomer and the dimer with the wild type, we observed how the mutated protein was highly unstable in its monomeric form (ΔΔG = 555.75 kcal/mol), whereas the dimeric configuration was slightly more stable than the wild-type dimer (ΔΔG = -126.52 kcal/mol). It has to be noted that three mutants (p. (Tyr28Asp), p.(Ala179Thr), and p.(Glu235Gly)) were not part of the mutant collection found in our cohort, but they were previously studied (27, 28) and here included as internal controls.
HPP is a genetic disorder of bone metabolism featured by a variegated clinical presentation. Although the disease is caused by the lack of enzymatic TNSALP activity, symptoms often do not correlate with the severity of the enzymatic loss. The extreme variability of the resulting phenotype, ranging from lethal forms “in utero” to the osteoporotic type in adults, makes the condition hard to be promptly recognized (7, 29). This is mostly true for the milder forms, whose risk is to be confused with postmenopausal/idiopathic osteoporosis and consequently treated with bone antiresorptive drugs (i.e., bisphosphonates) that only succeed in worsening the bone disease (30). It was recently reported that up to 0.5% of osteoporotic patients may have low ALP activity, in which 87% had a deleterious ALPL variant (31). Hence, considering HPP in a wide differential diagnosis of osteoporosis/low bone mass could be relevant for both therapeutic and counseling issues.
In our cohort of suspected 30 HPP cases, we observed a mutation rate of 66.7% (20/30), sensibly higher if compared with previous studies (43%, 47%) (32, 33). Such a difference might lay on heterogeneity of patients’ selection, technical variability, and genetic background. Instead, Tenorio et al. (32) recruited 83 subjects on the basis of their clinical manifestation and low ALP activity, but the PLP value was not considered. Conversely, the cohort of 72 patients reported by Jandl et al. (33) was selected by low ALP activity, higher PLP, and clinical manifestation but consisted of only adult subjects (41.3–68.5 years). In the first case, it can be argued that adding a higher PLP level as an additional inclusion criterion could improve the case selection and the mutation rate. To confirm this assumption, we used, as internal control, a different cohort of subjects with low ALP activity but with normal or (presumably normal) not provided VitB6 value. Screening of the ALPL gene on these subjects was negative (data not shown), indicating that although hypophosphatasemia (with normal VitB6 serum level) is a not infrequent biochemical issue, it would not be related to the TNSALP gene. Concerning the Jandl et al.’s study, it cannot be excluded that the older age of the sample size may have influenced the mutation rate, perhaps due to the overlap with general osteoporosis.
In our survey, 10 patients with low ALP and high VitB6 values, one also having familial hypophosphatasemia, resulted to be negative. With regard to the clinical picture of these patients, whether the phenotype could be softened or worsened by the presence of alleged pathogenic variants lying in genetic regions not covered by the screening (such as deep intronic regions or 5′ and 3′UTR) or compensatory mechanisms or the action of genetic modifiers is far from being elucidated. In this regard, we kindly point out that our NGS panel contained the whole genomic (intronic and regulatory regions) of the ALPL gene, in order to identify deep intronic splicing variants, which, however, had never been found.
Interestingly, patients’ enrollment was driven by biochemistry values, regardless of the clinical spectrum that, as reported in Table 2, was highly variable, ranging from low bone mass/bone pain or just myalgia and actually reflecting the paradigmatic different HPP clinical variability. Thus, our work can confirm the utility of biomarkers in case of blurred clinical presentation, since only the ALP and VitB6 values were confirmed as the most suggestive clues with a strong correlation (up to 65% of cases) with the presence of a genetic lesion (Figure 3). In our previous work, we also suggested the predictive value of PEA (34); however, this metabolite, often along with VitB6, is not included in the routinely biochemistry panel, and, then, no PEA data were available at the time of this report.
Literature data recorded the p.(Gln207Pro) variant once in a severe autosomal recessive perinatal form in compound heterozygosity with p.(Arg71Pro) in a baby girl who died 3 h after birth (2). Patient #21 carried the same 207P variant and was affected by an autosomal dominant perinatal (30 days of life) form featured by severe fractures. However, the mother and grandmother, both carriers of the same variant, had an asymptomatic form with borderline ALPL levels. The p.(Glu88Lys) variant was found once in compound heterozygosity with the p.(Pro37Leu) in an 11-month-old boy affected by nephrocalcinosis and alterations of the fontanelle (35). Both our patients #7 and #23 carried the 88Lys in heterozygosity and #23 had nephrocalcinosis, since he was 10 years old. Whether the 88Lys variant is specifically accountable of the nephrocalcinosis requires further studies. It should be noted that cases #21, #22, and #23 were not initially included in the survey, as ALP values for #21 and #23 were normal. However, the use of NGS in the differential diagnosis helped to clarify, in both, an uncovered HPP form.
Interestingly, 2 subjects, #6 and #9 carrying the same variant, the p.D109E, both came from Sardinia and, although they denied any apparent kinship, a possible ancestor effect cannot be excluded.
About the eight novel amino acid changes, the ALPL database has two different records for residue Glu84 (#18): the first was 84Val found in a family with autosomal dominant odonto-hypophosphatasia also characterized by rickets-like signs (36). The second, 84Asp, was found as a compound heterozygote in a 6-year-old girl with a childhood form. Despite no functional test being done on these variants, the authors suggested that both variants at Glu84 would bear a DNE, since the residue is located at the homodimer interface (36): this assumption was confirmed by our bioinformatic investigation.
No data can be inferred from the literature about the effect of the 267Pro (#15) or 472Arg (#2) mutants. Conversely, with regard to the Phe290 variant, Mornet et al. proved that this residue, together with Glu235, Glu291, and Asp306, coordinates the binding of the calcium atoms (37): it can therefore be deduced that the 290Leu (#10) variant could severely impair the enzymatic activity. Our modeling study confirms that all three mutants destabilize the homodimer structure.
About the stop gain p.(Tyr85*) (#19) and both the frameshift mutations, p.(Ser310Profs*28) (#17) and p.(Arg391Profs*14) (#11), that, presumably, introduce a premature stop codon, the mutated proteins are likely to undergo proteasomal degradation (38), or, if translated, they are totally inactive, due the loss of (more than) 50% of the canonical protein structure. In contrast, p.(*525Argextfs*11) (#13) does not interrupt the coding frame, as it leads to correct translation of the whole protein with presumed 11 additional residues. Although in the absence of functional data, the effect of this supplemental tail could not be considered detrimental for the enzymatic activity; instead, the mutant does not seem to bear the DNE since the phenotype of patient #13 (and of her brother, Figure 2) overlaps with classical osteoporosis with a borderline-low ALP activity. In our computational modeling, the mutant homodimer p.(*525Argextfs*11) appears more stable than the monomer, so we cannot exclude that the destabilized structure is partially mitigated. This is in contrast with data reporting that a similar mutation, p.(Leu520Argfs*86), that adds more than 80 residues at the C-terminal tail was proven to lack GPI binding with a consequent severe reduced enzymatic activity (39).
There were 22 common intronic (nr 14) and exonic (nr 8) variants identified: 17 have a MAF in gnomAD > 0.05, whereas the remaining were rarer (Supplementary Material 3). No correlation was attempted with the presence of fractures, nor with biochemical values, as previously suggested (40, 41), since the distribution of the SNPs in the whole cohort (positive, negative, low, or normal ALP patients) was not different (data not shown).
p.(Arg152His) was found in three patients (out of 33, 9.3%) with a frequency six times higher than that reported in gnomAD (MAF = 1.5%): in two cases (#7 and #19), it was identified together with a pathogenic ALPL variant. Whether 152His could actually play the role of the phenotype modifier or it is in linkage disequilibrium with an actual pathogenic variant located in genetic regions not analyzed would be a hypothesis to shed light on. Moreover, it cannot be excluded that its frequency is higher in the Italian population, although there is no evidence in public databases.
To the best of our knowledge, our genetic study represents the first reporting of a so high mutation rate in an Italian HPP cohort: it follows our previous work on a smaller survey (34) and describes a total of 23 molecularly confirmed HPP cases, 8 novel ALPL variants, 1 large ALPL deletion, and 1 case with familial hypophosphatasemia in which the search for the molecular basis has to date been unsuccessful. The identification of eight novel variants and the detailed clinical description increase the knowledge on the effect of ALPL pathogenic variants associated with this disease and provides a clear landscape about the spread and presentation of this disease in Italy.
We confirm the complexity of a timely recognition of the syndrome, mostly for adult forms, due the high clinical variability In this regard, the suspicious HPP can derive from the biochemical values, low ALP, and high VitB6, and not necessarily from the clinical phenotype that can be extremely variable. The clinical diagnosis must then be confirmed by the genetic analysis that, undoubtedly, represents an essential aid to identify carriers to address to the follow-up and to make the differential diagnosis, avoiding dangerous therapies in unrecognized cases.
The data presented in the study are deposited in the European Variation Archive (EVA) repository at the EMBL-EBI institute, with the following accessions: Project - PRJEB61880 and Analyses - ERZ18242459 (42).
The studies involving human participants were reviewed and approved by Ethics Committee at Fondazione IRCCS Casa Sollievo della Sofferenza (Prot 201/CE, Dec 2017). Written informed consent to participate in this study was provided by the participants’ legal guardian/next of kin.
Conceptualization: LC, ASS, AS, and VG; methodology: LC, DT, BA, and GN; software: TB, FPe, and MM; validation: LC, FPu, and VG; formal analysis: FPu, ASS, and AS; clinical investigation: FPu, ASS, CB, FC, SCo, RF, TF, AG, CG, EG, GG, SM, AP, LP, FPi, RR, ES, PV, SCa, MCe, MCa, and AS; resources: MCa; data curation: LC; writing original draft preparation: AS and VG; writing, review, and editing: MCa, AS, and VG. All authors contributed to the article and approved the submitted version.
This work was partially funded by the Italian Ministry of Health, Ricerca Corrente 2018-2021 and by Alexion, AstraZeneca Rare Disease. The funders were not involved in the study design, analysis, interpretation of data, the writing of this article or the decision to submit it for publication.
The authors gratefully acknowledge the patients and the families participating this study. The authors gratefully acknowledge Giulia Casamassima, Domenica Genovese, and Marianna Scutifero for the insightful support, and Ilaria Olivetti and Antonio Pagliazzo for the invaluable help in the project management and organization and Alexion’s courtesy review.
LC was the recipient of a fellowship by Alexion, AstraZeneca Rare Disease.
The remaining authors declare that the research was conducted in the absence of any commercial or financial relationships that could be construed as a potential conflict of interest.
All claims expressed in this article are solely those of the authors and do not necessarily represent those of their affiliated organizations, or those of the publisher, the editors and the reviewers. Any product that may be evaluated in this article, or claim that may be made by its manufacturer, is not guaranteed or endorsed by the publisher.
The Supplementary Material for this article can be found online at: https://www.frontiersin.org/articles/10.3389/fendo.2023.1205977/full#supplementary-material
2. Henthorn PS, Raducha M, Fedde KN, Lafferty MA, White MP. Different missense mutations at the tissue-nonspecific alkaline phosphatase gene locus in autosomal recessively inherited forms of mild and severe hypophosphatasia. Proc Natl Acad Sci USA (1992) 89:9924–8. doi: 10.1073/pnas.89.20.9924
3. Bianchi ML, Bishop NJ, Guañabens N, Hofmann C, Jakob F, Rouxet C, et al. Hypophosphatasia in adolescents and adults: overview of diagnosis and treatment. Osteoporos. Int (2020) 8:1445–60. doi: 10.1007/s00198-020-05345-9
4. Khan AA, Josse R, Kannu P, Villeneuve J, Paul T, Van Uum S, et al. Hypophosphatasia: Canadian update on diagnosis and management. Osteoporos. Int (2019) 9:1713–22. doi: 10.1007/s00198-019-04921-y
5. ALPL database. Available at: https://alplmutationdatabase.jku.at/table/.
6. Lia-Baldini AS, Brun-Heath I, Carrion C, Simon-Bouy B, Serre JL, Nunes ME, et al. A new mechanism of dominance in hypophosphatasia: the mutated protein can disturb the cell localization of the wild-type protein. Hum Genet (2008) 4:429–32. doi: 10.1007/s00439-008-0480-1
7. Linglart A, Biosse-Duplan M. Hypophosphatasia. Curr Osteoporos Rep (2016) 3:95–105. doi: 10.1007/s11914-016-0309-0
8. ClinVar. Available at: https://www.ncbi.nlm.nih.gov/clinvar/.
9. ANNOVAR. Available at: http://annovar.openbioinformatics.org/en/latest/.
10. dbSNP. Available at: https://www.ncbi.nlm.nih.gov/snp/.
11. 1000 Genomes. Available at: http://www.internationalgenome.org.
12. ExAC. Available at: http://exac.broadinstitute.org.
13. Richards S, Aziz N, Bale S, Bick D, Das S, Gastier-Foster J, et al. Standards and guidelines for the interpretation of sequence variants: a joint consensus recommendation of the American college of medical genetics and genomics and the association for molecular pathology. Genet Med (2015) 5:405–24. doi: 10.1038/gim.2015.30
14. Ensembl. Available at: http://www.ensembl.org/Homo_sapiens/Info/Index.
15. Clustalw. Available at: https://www.genome.jp/tools-bin/clustalw.
16. Jumper J, Evans R, Pritzel A, Green T, Figurnov M, Ronneberger O, et al. Highly accurate protein structure prediction with AlphaFold. Nature (2021) 7873:583–9. doi: 10.1038/s41586-021-03819-2
17. Mirdita M, Ovchinnikov S, Steinegger M. ColabFold - making protein folding accessible to all. bioRxiv (2021). doi: 10.1101/2021.08.15.456425
18. Case DA, Cheatham 3TE, Darden T, Gohlke H, Luo R, Merz jrKM, et al. The amber biomolecular simulation programs. J Comput Chem (2005) 16:1668–88. doi: 10.1002/jcc.20290
19. Biagini T, Chillemi G, Mazzoccoli G, Grottesi A, Fusilli C, Capocefalo D, et al. Molecular dynamics recipes for genome research. Brief. Bioinform (2018) 5:853–62. doi: 10.1093/bib/bbx006
20. Schymkowitz J, Borg J, Stricher F. The FoldX web server: an online force field. Nucleic Acids Res (2005) 33:W382–8. doi: 10.1093/nar/gki387
21. Available at: https://www.r-project.org/.
22. Available at: https://CRAN.R-project.org/package=plyr.
23. Available at: https://CRAN.R-project.org/package=ggplot2.
24. Available at: https://CRAN.R-project.org/package=dbplyr.
25. Available at: https://CRAN.R-project.org/package=dplyr.
26. Franklyn. Available at: https://franklin.genoox.com/clinical-db/home.
27. Weiss MJ, Cole DE, Ray K, Whyte MP, Lafferty MA, Mulivor LA, et al. A missense mutation in the human liver/bone/kidney alkaline phosphatase gene causing a lethal form of hypophosphatasia. Proc Natl Acad Sci USA (1988) 85:7666e9. doi: 10.1073/pnas.85.20.7666
28. Rauch F, Bardai G, Rockman-Greenberg C. ALPL mutations in adults with rheumatologic disorders and low serum alkaline phosphatase activity. J Bone Miner. Metab (2019) 5:893–9. doi: 10.1007/s00774-019-00991-4
29. Offiah AC, Vockley J, Munns CF, Murotsuki J. Differential diagnosis of perinatal hypophosphatasia: radiologic perspectives. Pediatr Radiol (2019) 1:3–22. doi: 10.1007/s00247-018-4239-0
30. Righetti M, Wach J, Desmarchelier R, Coury F. Teriparatide treatment in an adult patient with hypophosphatasia exposed to bisphosphonate and revealed by bilateral atypical fractures. Joint Bone Spine (2018) 3:365–7. doi: 10.1016/j.jbspin.2017.12.001
31. Alonso N, Larraz-Prieto B, Berg K, Lambert Z, Redmond P, Harris SE, et al. Loss-of-Function mutations in the ALPL gene presenting with adult onset osteoporosis and low serum concentrations of total alkaline phosphatase. J Bone Miner. Res (2020) 4:657–61. doi: 10.1002/jbmr.3928
32. Tenorio J, Álvarez I, Riancho-Zarrabeitia L, Martos-Moreno GA, Mandrile G, de la Flor Crespo M, et al. Molecular and clinical analysis of ALPL in a cohort of patients with suspicion of hypophosphatasia. Am J Med Genet A (2017) 3:601–10. doi: 10.1002/ajmg.a.37991
33. Jandl NM, Schmidt T, Rolvien T, Stürznickel J, Chrysostomou K, von Vopelius E, et al. Genotype-phenotype associations in 72 adults with suspected ALPL-associated hypophosphatasia. Calcif. Tissue Int (2021) 3:288–301. doi: 10.1007/s00223-020-00771-7
34. Guarnieri V, Sileri F, Indirli R, Guabello G, Longhi M, Dito G, et al. Clinical, biochemical and genetic findings in adult patients with chronic hypophosphatasemia. J Endocrinol Invest. (2022) 1:125–37. doi: 10.1007/s40618-021-01625-1
35. Mohn A, De Leonibus C, de Giorgis T, Mornet E, Chiarelli F. Hypophosphatasia in a child with widened anterior fontanelle: lessons learned from late diagnosis and incorrect treatment. Acta Paediatr (2011) 7:e43–6. doi: 10.1111/j.1651-2227.2011.02228.x
36. Wei KW, Xuan K, Liu YL, Fang J, Ji K, Wang X, et al. Clinical, pathological and genetic evaluations of Chinese patients with autosomal-dominant hypophosphatasia. Arch Oral. Biol (2010) 12:1017–23. doi: 10.1016/j.archoralbio.2010.08.003
37. Mornet E, Stura E, Lia-Baldini AS, Stigbrand T, Ménez A, Le Du MH. Structural evidence for a functional role of human tissue nonspecific alkaline phosphatase in bone mineralization. J Biol Chem (2001) 33:31171–8. doi: 10.1074/jbc.M102788200
38. Komaru K, Ishida Y, Amaya Y, Goseki-Sone M, Orimo H, Oda K. Novel aggregate formation of a frame-shift mutant protein of tissue-nonspecific alkaline phosphatase is ascribed to three cysteine residues in the c-terminal extension. retarded secretion and proteasomal degradation. FEBS J (2005) 7:1704–17. doi: 10.1111/j.1742-4658.2005.04597.x
39. Orimo H, Hayashi Z, Watanabe A, Hirayama T, Hirayama T, Shimada T. Novel missense and frameshift mutations in the tissue-nonspecific alkaline phosphatase gene in a Japanese patient with hypophosphatasia. Hum Mol Genet (1994) 9:1683–4. doi: 10.1093/hmg/3.9.1683
40. Marini F, Masi L, Giusti F, Cianferotti L, Cioppi F, Marcucci G, et al. ALPL genotypes in patients with atypical femur fractures or other biochemical and clinical signs of hypophosphatasia. J Clin Endocrinol Metab (2021) 5:e2087–94. doi: 10.1210/clinem/dgab914
41. Masi L, Marini F, Franceschelli F, Leoncini G, Cianferotti L, Cioppi L, et al. Polymorphic variants of alkaline phosphatase gene correlate with clinical signs of adult hypophosphatasia? Osteoporos Int (2019) 12:2461–72. doi: 10.1007/s00198-021-05893-8
Keywords: hypophosphatasia, alkaline phosphatase, genetics, dominant negative effect, ALPL, vitamin B6
Citation: Cinque L, Pugliese F, Salcuni AS, Trombetta D, Battista C, Biagini T, Augello B, Nardella G, Conti F, Corbetta S, Fischetto R, Foiadelli T, Gaudio A, Giannini C, Grosso E, Guabello G, Massuras S, Palermo A, Politano L, Pigliaru F, Ruggeri RM, Scarano E, Vicchio P, Cannavò S, Celli M, Petrizzelli F, Mastroianno M, Castori M, Scillitani A and Guarnieri V (2023) Clinical and molecular description of the first Italian cohort of 33 subjects with hypophosphatasia. Front. Endocrinol. 14:1205977. doi: 10.3389/fendo.2023.1205977
Received: 14 April 2023; Accepted: 21 June 2023;
Published: 01 August 2023.
Edited by:
Andrea Del Fattore, Bambino Gesù Children’s Hospital (IRCCS), ItalyReviewed by:
Hao Zhang, Shanghai Jiao Tong University, ChinaCopyright © 2023 Cinque, Pugliese, Salcuni, Trombetta, Battista, Biagini, Augello, Nardella, Conti, Corbetta, Fischetto, Foiadelli, Gaudio, Giannini, Grosso, Guabello, Massuras, Palermo, Politano, Pigliaru, Ruggeri, Scarano, Vicchio, Cannavò, Celli, Petrizzelli, Mastroianno, Castori, Scillitani and Guarnieri. This is an open-access article distributed under the terms of the Creative Commons Attribution License (CC BY). The use, distribution or reproduction in other forums is permitted, provided the original author(s) and the copyright owner(s) are credited and that the original publication in this journal is cited, in accordance with accepted academic practice. No use, distribution or reproduction is permitted which does not comply with these terms.
*Correspondence: Vito Guarnieri, di5ndWFybmllcmlAb3BlcmFwYWRyZXBpby5pdA==
†ORCID: Vito Guarnieri, orcid.org/0000-0002-5500-8558
Disclaimer: All claims expressed in this article are solely those of the authors and do not necessarily represent those of their affiliated organizations, or those of the publisher, the editors and the reviewers. Any product that may be evaluated in this article or claim that may be made by its manufacturer is not guaranteed or endorsed by the publisher.
Research integrity at Frontiers
Learn more about the work of our research integrity team to safeguard the quality of each article we publish.