- Department of Gynecologic Surgery & Obstetrics, Uniformed Services University of the Health Sciences, Bethesda, MD, United States
From the time of its discovery and isolation in the mammalian hypothalamus, the decapeptide, gonadotropin-releasing hormone (GnRH), has also been found to be expressed in non-hypothalamic tissues and can elicit a diverse array of functions both in the brain and periphery. In cancer, past studies have targeted the gonadotropin-releasing hormone receptors (GnRHR) as a way to treat reproductive cancers due to its anti-tumorigenic effects. On the contrary, its metabolite, GnRH-(1-5), behaves divergently from its parental peptide through putative orphan G-protein coupled receptor (oGPCR), GPR101. In this review, we will focus on the potential roles of GnRH-(1-5) in the periphery with an emphasis on its effects on endometrial cancer progression.
Introduction
The gonadotropin-releasing hormone (GnRH) is a central regulator of mammalian reproductive function through the hypothalamic–pituitary–gonadal (HPG) axis. Since its discovery in the porcine hypothalamus, over 20 different primary structures of GnRH and its receptors have been identified and studied across various species (1–4). The first form of GnRH in vertebrates (pGlu-His-Trp-Ser-Tyr-Gly-Leu-Arg-Pro-Gly-NH2) is designated as GnRH-I, contains highly conserved sequences on NH2 and COOH terminal residues, pGlu-His-Trp-Ser and Pro-Gly respectively (4). While GnRH regulation of the HPG axis have been extensively studied, its role in gonadotrope desensitization or fertility reestablishment in hypogonadal patients has made GnRH analogues and antagonists an appealing target for a wide variety of clinical treatments (5–12). Furthermore, a growing number of studies have determined that GnRHR stimulation by GnRH analogs induces antiproliferative and antimetastatic effects in various types of tumors, therefore, presenting GnRHRs as a good candidate for therapeutic intervention for cancers (4, 13–15).
While GnRH and its pharmacologic analogs impede cancer progression, its metabolite, GnRH-(1–5), elicits an opposing response. Our previous studies have demonstrated that GnRH-(1–5) is a biologically active pentapeptide and its mechanism of action is autonomous from its parental peptide (16). In this review, we will describe the metabolism of GnRH to generate GnRH-(1–5), provide a brief overview on the discovery of its bioactivity, and discuss how the biological responses elicited by GnRH-(1–5) in endometrial cancer cells are mediated by GPR101 to stimulate cell growth and metastatic behavior. Our studies suggests that GnRH-(1–5) is more than a metabolic byproduct and targeting its putative receptors may offer a therapeutic target for halting cancer progression.
GnRH is metabolized to generate bioactive GnRH-(1–5)
The decapeptide, GnRH, is metabolized by zinc metalloendopeptidase EC 3.4.24.15 (EC 3.4.24.15 designation based on the International Union of Biochemistry and Molecular Biology (IUBMB) Enzyme Nomenclature; abbreviated as EP24.15 or THOP1), to generate the pentapeptide GnRH-(1–5) (17). This thimet oligopeptidase belongs to a class of zinc dependent metalloendopeptidases expressed in mammalian tissues; most notably in the brain, pituitary, and testis (18–20). First identified as a 75kDa neuropeptide peptidase in the soluble fraction of rat brain homogenates, it was originally thought to be predominantly localized in the cytoplasm. However, EP24.15 has subsequently been found temporally localized to the extracellular surface of the plasma membrane, as well as synaptosomes and the exofacial leaflet of the lipid raft microenvironment (21–25).
Historically, this peptidase was recognized for its sole function as a classic degrading enzyme terminating its substrate bioactivity by reducing or eliminating the binding potential to its cognate receptor. However, EP24.15 has since been recognized as possessing other abilities to regulate cellular activities. A previous study has demonstrated that within the purified synaptosomal membrane of the rat brain, EP24.15 was able to transform neoendorphin into Leu-enkephalin; an example of transforming an inert precursor into an active neuropeptide (25). EP24.15 can also modulate a peptide to alter its bioactivity in opposition of its original physiological effect, as exhibited by altering angiotensin-I (Ang-I) into fragment angiotensin-(1–7) (Ang-(1–7)) (26–28). Furthermore, our lab has demonstrated that EP24.15 can convert bioactive GnRH-I into another bioactive pentapeptide, GnRH-(1–5), to elicit lordosis behavior in female rats through a receptor independent of its parental receptor, GnRHR-I (16).
The manner of specificity in which GnRH is metabolized suggests that EP24.15 converts rather than simply generating a degraded peptide as an inactive final byproduct. Furthermore, EP24.15 displays preferential cleaving of hydrophobic residues at positions P1, P2 and P30 in larger peptides and P10 in smaller peptides; denoting this fragmentation as a highly distinctive process (29). In vitro, the hydrolysis of GnRH involves a two-step mechanism requiring zinc as a cofactor bound to the active site with the classic HEXXH motif, thiol activation, and phosphorylation of serine residue 644 (Ser644) by protein kinase A (PKA) (18, 30–33).
Kinetic studies in ovine hypothalamic extracts indicated that EP24.15 phosphorylation decreases its affinity for the α-amidated decapeptide GnRH (32, 33). However, upon cleavage of glycine (Gly10) at the carboxyl terminal by prolyl endopeptidase (PE), the intermediate fragment, GnRH-(1–9) becomes a greater substrate for EP24.15 by 10-15-fold (33). This study suggests that basic structural conditions are required for efficient and successful interaction with the binding site and that the amidation of the carboxyl terminal is necessary to avert any nonspecific degradation of GnRH (21). Subsequently in the second step, EP24.15 rapidly cleaves GnRH-(1–9) at the Tyr5-Gly6 bond to generate biologically active product, GnRH-(1–5) (32, 33).
The discovery of GnRH-(1–5) biological activity
The widely accepted paradigm that a cleaved peptide becomes biologically inactive has been disputed by a growing body of literature that suggests otherwise (34). These fragmented peptides appear to regulate a diverse array of functions that are autonomous of its parental peptide (32, 35). In relation to the focus of our review, previous researchers have speculated that GnRH metabolite, GnRH-(1–5), may be a biologically active fragment and warrants further investigations (16, 34, 36–39).
One of the first observations of possible bioactivity were seen in the hypothalamic rat explants where treatment with GnRH-(1–5) resulted in the reversible suppression of the spontaneous pulsatile secretion of GnRH. This suppression was similar in manner to the NMDA competitive antagonist, AP-5 (36). This study suggests that GnRH-(1–5) may act through NMDA receptors to mediate an inhibitory autofeedback of GnRH secretion in various types of cells. Our previous expression study in the immortalized mouse neuron GT1-7 cell line demonstrated that treatment with GnRH-(1–5) stimulated GnRH-I mRNA expression in contrast to GnRH-I treatment, which demonstrated a negative autoregulatory feedback effect on GnRH-I expression (37). Interestingly, the effect of GnRH-(1–5) on LH release may be mediated by kisspeptin neurons (39). In the periphery, our studies with the human Ishikawa cell line, a commonly used model for GnRH-I effects on endometrial cancer studies, demonstrated that GnRH-(1–5) had no effect on the gene expression of the GnRH-I system unlike its parental peptide (38).
In addition to regulating gene expression, GnRH-(1–5) can also regulate lordosis behavior in estradiol-primed ovariectomized female rats (16). The intracerebroventricular (ICV) administration of GnRH-(1–5) elicited lordosis, indicating that the metabolite was just as effective in stimulating sexual behavior as its parental peptide, GnRH-I. This observation was further validated through the use of immunoneutralization studies with antibodies to EP24.15, in which GnRH-I facilitated lordosis was inhibited whereas the GnRH-(1–5)-facilitated lordosis remained unaffected (16). Collectively, these studies indicate that GnRH-(1–5) has different effects from its parental peptide, and its mechanism of action may be mediated through receptors independent of GnRHR (Table 1).
Identification of GPR101 as a putative GnRH-(1–5) receptor
Putative GnRH-(1–5) receptors were discovered by utilizing a high-throughput β-arrestin recruitment assay (43). One of the receptors identified for positive binding was orphan G protein-coupled receptor 101 (GPR101). A previous study noted that the promoter region of the GPR101 gene was hypermethylated in colorectal cancer, therefore, future studies were focused on GPR101 as a possible receptor for GnRH-(1–5) (44). The relationship between GnRH-(1–5) to GPR101 was validated through siRNA mediated downregulation of GPR101 expression studies and the reversal of GnRH-(1–5) effects on EGFR phosphorylation and migration (45). These findings implicate GPR101 as the receptor GnRH-(1–5) acts on to transactivate EGFR phosphorylation in the Ishikawa cell line.
The GPR101 gene is located on chromosome X (Xq26.3) which encodes for a 508-aa, Class A (rhodopsin-like) GPCR protein. It is highly expressed in the fetal pituitary during the somatotrope maturation process as well as the hypothalamus, nucleus accumbens, and other tissues (45–49). While it shares ~30% sequence homology in the transmembrane regions with GPCRs RE2, α-1A-adrenergic receptor, and the serotonin 5HT1A receptor, early studies utilizing the knowledge-restricted hidden Markov model-based algorithm and determination of cAMP levels in overexpressing human GPR101 in human embryonic kidney (HEK293) or rat pituitary GH3 cells indicates that GPR101 may be constitutively coupled to Gαs signaling pathway (46, 50, 51). Other studies utilizing the chinese hamster ovary (CHO-K1) or the Ishikawa cell line report that GPR101 does not constitutively activate or stimulate the cAMP pathway but suggests possible coupling to other G protein mediated pathways such as the Gαi or Gαq/11 pathway (52, 53). These observations suggest that GPR101 and its G-protein selectivity may be tissue dependent and warrants further investigations in future studies. Although studies thus far have focused primarily on GPR101 and its role in endocrine related disorders such as X-linked acrogigantism (X-LAG) (54, 55), new emerging studies indicate other possible functions in mediating the hypothalamic control of energy homeostasis, pro-resolving actions in leukocytes to control inflammation, and stimulating cell proliferation and metastatic behavior in endometrial cancer cells (45, 48, 49, 56, 57).
GnRH-(1–5) effects on cell proliferation in endometrial cancer cells
As stated previously, GnRH-I and its analogs offer treatment to a wide array of conditions including sex hormone-dependent diseases seen in prostate and gynecological cancers (12, 19, 58–63). The activity of GnRH is regulated through GnRHR via distinctive signal transduction pathways in a tissue-dependent manner. Most studies associate the antitumorigenic activity of GnRHR through coupling with the Gαi pathway. This pathway is correlated to the activation of apoptotic signaling cascades, augmentation of phosphotyrosine-phosphatases, promotion of cell cycle arrest, and impeding the MAPK signaling pathway (40–42, 64–66). However, some studies from cells in other tissues have observed GnRH-I coupled to the Gαq subunit (67, 68).
While GnRH analogs are known to have antitumorigenic effects, in our studies, GnRH-(1–5) exerts an opposing effect by promoting cell proliferation and invasion in endometrial cell lines. Our initial study investigated whether a wide range of dosage treatments with GnRH vs GnRH-(1–5) can affect cell proliferation and alter markers for MAPK signaling pathway in the Ishikawa cell line (69). After 24h of treatment, this study demonstrated that GnRH and GnRH-(1–5) had diverging effects on cell proliferation; GnRH decreased cell proliferation whereas GnRH-(1–5) increased cell proliferation. Furthermore, GnRH-(1–5) suppressed caspase-3/7 activity and downregulated ERK-1/2 expression, suggesting for the first time that cell growth and proliferation may be linked to an apoptotic process in endometrial cancer cells.
GnRH-(1-5) signaling pathway
The EGFR signaling pathway is heavily implicated in its role in cancer progression (70, 71). Dysregulation of this signaling pathway in endometrial cancer can lead to transforming tumors into a more aggressive metastatic phenotype; therefore, we performed a study to determine whether GnRH-(1–5) can alter the EGFR signaling pathway in the endometrial cancer Ishikawa cell line (72–75). Our studies suggest that GnRH-(1–5) stimulates epidermal growth factor (EGF) release, increases phosphorylation of EGFR at three tyrosine sites (992, 1045, 1068), and promotes cell migration (Figure 1) (45); however, GnRH and GnRH analogs, (D-Ser6)-GnRH or (D-Trp6)-GnRH had no effect on EGFR phosphorylation and impeded cellular migration compared to untreated cells. Pre-incubation with GnRHR antagonist, Antide, had no effect on GnRH-(1–5) ability to stimulate EGF release and EGFR phosphorylation demonstrating a novel mechanism that’s independent of GnRHR in mediating these effects. Furthermore, studies with G-protein antagonist peptide (GPAnt-2) suggested effects observed with GnRH-(1–5) were G-protein dependent.
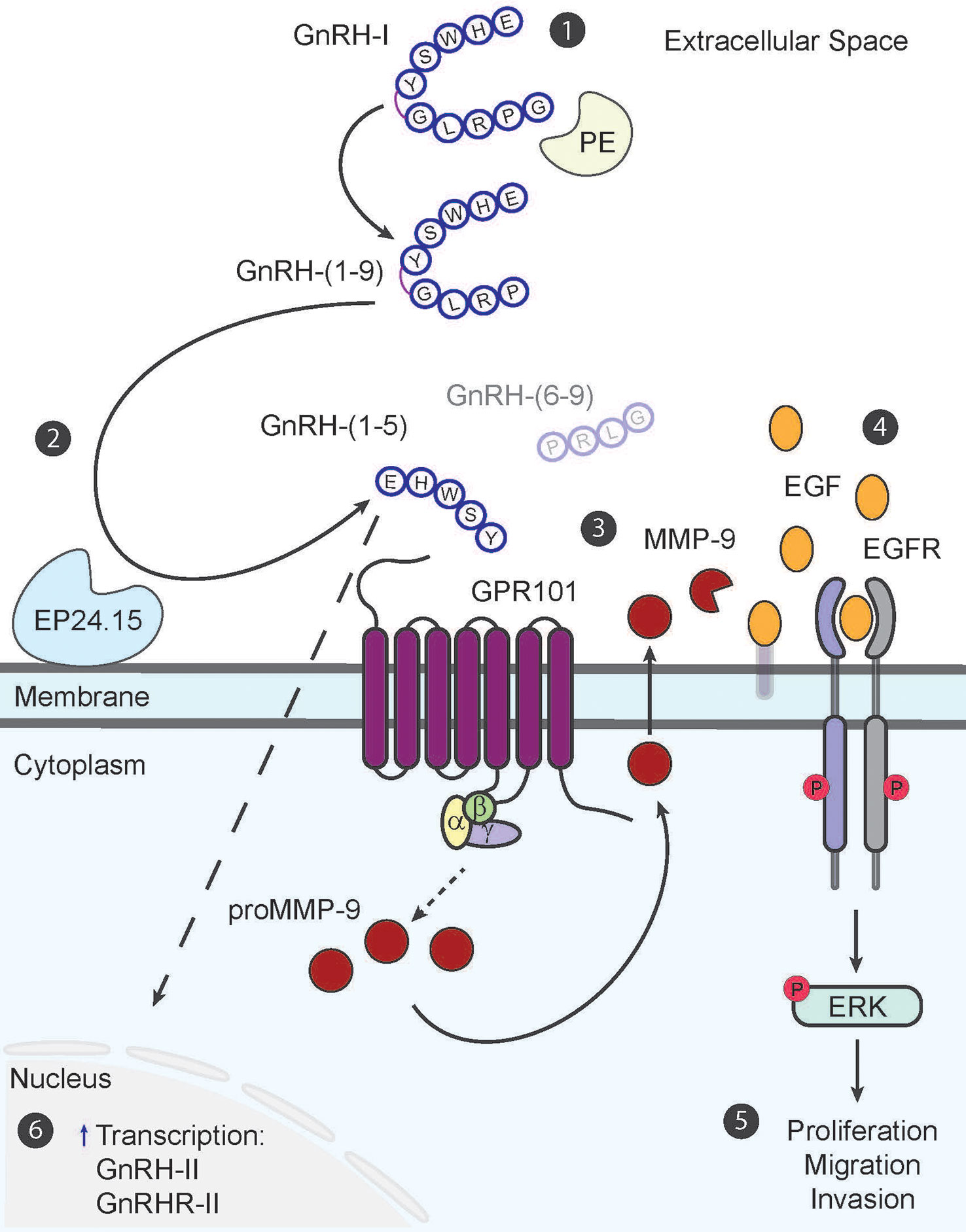
Figure 1 The GnRH-(1–5) paradigm in endometrial cancer cells. The metabolite GnRH-(1–5) is generated in a two-step process from decapeptide, GnRH-I (1). The first step is the removal of Gly10 at the C-terminus by enzyme, prolyl endopeptidase (PE), to generate intermediary fragment, GnRH-(1–9) (2). The second step involves the hydrolyses of GnRH-(1–9) by enzyme, EP24.15, at the covalent bond between Tyr5–Gly6 to produce bioactive pentapeptide fragment, GnRH-(1–5) and tripeptide GnRH-(6–9) (3). GnRH-(1–5) in turn binds to GPR101 to stimulate MMP-9 activity to increase EGF release into the extracellular space in a G-protein dependent manner (4). The release of EGF subsequently increases EGFR phosphorylation at three tyrosine sites (992, 1045, 1068) and initiates signaling cascades (5). Downstream phosphorylation of ERK results in increased cell proliferation, migration, and invasion; all important factors for inciting metastatic potential (6). GnRH-(1–5) can also regulate GnRH-II and GnRHR-II gene expression; however this mechanism still remains elusive.
Our most recent study with GnRH-(1–5) has determined that its effects are dependent on increased matrix metallopeptidase-9 (MMP-9) enzymatic activity in two different endometrial cancer cell models, the Ishikawa and ECC-1 cell lines (57). Upon binding to GPR101, GnRH-(1–5) increases MMP-9 activity to augment EGF release. This in turn increases EGFR phosphorylation to stimulate cellular migration and invasion. These results indicate the important physiological relevance of GnRH-(1–5) effects on mediating MMP-9 activity in increasing the metastatic potential of endometrial cancer cells.
Summary
The novelty of GnRH-(1–5) and its role in the pathophysiology of endometrial cancer adds another layer of complexity to our current understanding of the GnRH paradigm in endometrial cancer. Future studies with GnRH-(1–5) should investigate its effects on other growth factors and related signaling pathways highly implicated in endometrial cancer, such as transforming growth factor beta (TGFβ), vascular endothelial growth factor (VEGF), and platelet-derived growth factor (PDGF) (76–80). The TGFβ pathway is a well-known contributor to the malignant transformation of the precursor lesion endometrial intraepithelial neoplasm (EIN) into invasive carcinoma and rising levels of VEGF are linked to the development of endometrial cancer (76). The presence of PDGF in the microenvironment of carcinomatous endometrium is a hallmark in cancer-associated fibroblasts. PDGF is shown to promote immune cell recruitment through the modulation of the PI3K/Akt and MAPK/ERK pathway to stimulate endometrial cancer cell proliferation, which we have already identified to be a downstream effect of the GnRH-(1–5) signaling pathway (45, 57, 76).
In addition to investigating GnRH-(1–5) effects on other growth factors, exploration of GnRH-(1–5) signaling through another putative receptor, GPR173, should be considered since our studies in the brain have determined that GnRH-(1–5) can act on GPR173 to inhibit neuronal migration (81). Upon stimulation by GnRH-(1–5), GPR173 recruits β-arrestin and phosphatase and tensin homolog (PTEN) as adaptor proteins to inhibit the phosphorylation of signal transducer and activator of transcription 3 (STAT3) leading to decreased migration (43, 81). In women with endometriosis, reduced PNX levels and GPR173 expression may be responsible for HPG axis dysregulation (82).
These new insights may contribute to a better understanding of the pathophysiology of endometrial cancer and provide the basis for a new strategy for diagnosis. Furthermore, in our comparative transcriptome analysis between patient and endometrial cancer cell lines, we identified that one of the top five signaling pathways involved in cancer progression is the neuroprotective role for THOP1 in Alzheimer’s disease; shift from being up- to downregulated as approaching advanced cancer stage III (83). The THOP1 gene encodes for the protein EP24.15, which we have described previously, as the primary enzyme that metabolizes GnRH to GnRH-(1–5). Future studies should address the relationship between increased EP24.15 expression and enzymatic activity to generate GnRH-(1–5) to all its related markers identified to ascertain its role in driving cancer progression since a prior study has implicated augmented EP24.15 activity in prostate cancer (29).
Importantly, continuing to understand the effects of GnRH-(1–5) on endometrial cancer progression will provide future targets for pharmaceutical intervention. Currently, the mainstay treatment for early-stage disease is surgery however depending on the stage of disease and other risk factors, adjuvant radiotherapy and/or chemotherapy is used. Given that primary management for endometrial cancer is surgical intervention, the discovery of pharmaceutical targets will provide the most benefit for patients with comorbidities who are unsuitable for surgery and pre-menopausal or hormone insensitive patients who want to preserve their fertility.
Author contributions
MC-C and AW drafted and edited the manuscript. MC-C generated the Table and Figure. TW edited the drafts and edited the manuscript. All authors contributed to the article and approved the submitted version.
Funding
This work was supported by The National Science Foundation (Grant IOS-1052288) and the National Institutes of Health (R03HD078645).
Conflict of interest
The authors declare that the research was conducted in the absence of any commercial or financial relationships that could be construed as a potential conflict of interest.
Publisher’s note
All claims expressed in this article are solely those of the authors and do not necessarily represent those of their affiliated organizations, or those of the publisher, the editors and the reviewers. Any product that may be evaluated in this article, or claim that may be made by its manufacturer, is not guaranteed or endorsed by the publisher.
References
1. Schally AV, Arimura A, Baba Y, Nair RM, Matsuo H, Redding TW, et al. Isolation and properties of the FSH and LH-releasing hormone. Biochem Biophys Res Commun (1971) 43(2):393–9. doi: 10.1016/0006-291X(71)90766-2
2. Matsuo H, Baba Y, Nair RM, Arimura A, Schally AV. Structure of the porcine LH- and FSH-releasing hormone. i. the proposed amino acid sequence. Biochem Biophys Res Commun (1971) 43(6):1334–9. doi: 10.1016/S0006-291X(71)80019-0
3. Baba Y, Matsuo H, Schally AV. Structure of the porcine LH- and FSH-releasing hormone. II. confirmation of the proposed structure by conventional sequential analyses. Biochem Biophys Res Commun (1971) 44(2):459–63. doi: 10.1016/0006-291x(71)90623-1
4. Millar RP. GnRHs and GnRH receptors. Anim Reprod Sci (2005) 88(1-2):5–28. doi: 10.1016/j.anireprosci.2005.05.032
5. Silverman AJ, Silverman R, Lehman MN, Witkin JW, Millar RP. Localization of a peptide sequence contained in the precursor to gonadotropin releasing hormone (GnRH). Brain Res (1987) 402(2):346–50. doi: 10.1016/0006-8993(87)90042-4
7. Conn PM, Crowley WF Jr.Gonadotropin-releasing hormone and its analogues. N Engl J Med (1991) 324(2):93–103. doi: 10.1056/NEJM199101103240205
8. Barbieri RL. Clinical applications of GnRH and its analogues. Trends Endocrinol Metab (1992) 3(1):30–4. doi: 10.1016/1043-2760(92)90089-J
9. Moghissi KS. Clinical applications of gonadotropin-releasing hormones in reproductive disorders. Endocrinol Metab Clin North Am (1992) 21(1):125–40. doi: 10.1016/S0889-8529(18)30235-4
10. Conn PM, Crowley WF Jr.Gonadotropin-releasing hormone and its analogs. Annu Rev Med (1994) 45:391–405. doi: 10.1146/annurev.med.45.1.391
11. Filicori M. Gonadotrophin-releasing hormone agonists. A guide to Use selection. Drugs (1994) 48(1):41–58. doi: 10.2165/00003495-199448010-00005
12. Emons G, Schally AV. The use of luteinizing hormone releasing hormone agonists and antagonists in gynaecological cancers. Hum Reprod (1994) 9(7):1364–79. doi: 10.1093/oxfordjournals.humrep.a138714
13. Seeburg PH, Mason AJ, Stewart TA, Nikolics K. The mammalian GnRH gene and its pivotal role in reproduction. Recent Prog Horm Res (1987) 43:69–98. doi: 10.1016/B978-0-12-571143-2.50008-3
14. Krsmanovic LZ, Hu L, Leung PK, Feng H, Catt KJ. The hypothalamic GnRH pulse generator: multiple regulatory mechanisms. Trends Endocrinol Metab (2009) 20(8):402–8. doi: 10.1016/j.tem.2009.05.002
15. Krsmanovic LZ, Hu L, Leung PK, Feng H, Catt KJ. Pulsatile GnRH secretion: roles of G protein-coupled receptors, second messengers and ion channels. Mol Cell Endocrinol (2010) 314(2):158–63. doi: 10.1016/j.mce.2009.05.015
16. Wu TJ, Glucksman MJ, Roberts JL, Mani SK. Facilitation of lordosis in rats by a metabolite of luteinizing hormone releasing hormone. Endocrinology. (2006) 147(5):2544–9. doi: 10.1210/en.2005-1646
17. Roberts JL, Mani SK, Woller MJ, Glucksman MJ, Wu TJ. LHRH-(1-5): a bioactive peptide regulating reproduction. Trends Endocrinol Metab (2007) 18(10):386–92. doi: 10.1016/j.tem.2007.09.005
18. Tullai JW, Cummins PM, Pabon A, Roberts JL, Lopingco MC, Shrimpton CN, et al. The neuropeptide processing enzyme EC 3.4.24.15 is modulated by protein kinase a phosphorylation. J Biol Chem (2000) 275(47):36514–22. doi: 10.1074/jbc.M001843200
19. Grundker C, Volker P, Emons G. Antiproliferative signaling of luteinizing hormone-releasing hormone in human endometrial and ovarian cancer cells through G protein alpha(I)-mediated activation of phosphotyrosine phosphatase. Endocrinology. (2001) 142(6):2369–80. doi: 10.1210/endo.142.6.8190
20. Ramakrishnappa N, Rajamahendran R, Lin YM, Leung PC. GnRH in non-hypothalamic reproductive tissues. Anim Reprod Sci (2005) 88(1-2):95–113. doi: 10.1016/j.anireprosci.2005.05.009
21. Orlowski M, Michaud C, Chu TG. A soluble metalloendopeptidase from rat brain. purification of the enzyme and determination of specificity with synthetic and natural peptides. Eur J Biochem (1983) 135(1):81–8. doi: 10.1111/j.1432-1033.1983.tb07620.x
22. Crack PJ, Wu TJ, Cummins PM, Ferro ES, Tullai JW, Glucksman MJ, et al. The association of metalloendopeptidase EC 3.4.24.15 at the extracellular surface of the AtT-20 cell plasma membrane. Brain Res (1999) 835(2):113–24. doi: 10.1016/s0006-8993(99)01494-8
23. Jeske NA, Glucksman MJ, Roberts JL. Metalloendopeptidase EC3.4.24.15 is constitutively released from the exofacial leaflet of lipid rafts in GT1-7 cells. J Neurochem (2004) 90(4):819–28. doi: 10.1111/j.1471-4159.2004.02557.x
24. Oliveira V, Garrido PA, Rodrigues CC, Colquhoun A, Castro LM, Almeida PC, et al. Calcium modulates endopeptidase 24.15 (EC 3.4.24.15) membrane association, secondary structure and substrate specificity. FEBS J (2005) 272(12):2978–92. doi: 10.1111/j.1742-4658.2005.04692.x
25. Acker GR, Molineaux C, Orlowski M. Synaptosomal membrane-bound form of endopeptidase-24.15 generates leu-enkephalin from dynorphin1-8, alpha- and beta-neoendorphin, and met-enkephalin from met-enkephalin-Arg6-Gly7-Leu8. J Neurochem (1987) 48(1):284–92. doi: 10.1111/j.1471-4159.1987.tb13160.x
26. Keidar S, Kaplan M, Gamliel-Lazarovich A. ACE2 of the heart: From angiotensin I to angiotensin (1-7). Cardiovasc Res (2007) 73(3):463–9. doi: 10.1016/j.cardiores.2006.09.006
27. Brosnihan KB, Neves LA, Chappell MC. Does the angiotensin-converting enzyme (ACE)/ACE2 balance contribute to the fate of angiotensin peptides in programmed hypertension? Hypertension (2005) 46(5):1097–9. doi: 10.1161/01.HYP.0000185149.56516.0a
28. Chappell MC, Modrall JG, Diz DI, Ferrario CM. Novel aspects of the renal renin-angiotensin system: angiotensin-(1-7), ACE2 and blood pressure regulation. Contrib Nephrol. (2004) 143:77–89. doi: 10.1159/000078713
29. Swanson TA, Kim SI, Myers M, Pabon A, Philibert KD, Wang M, et al. The role of neuropeptide processing enzymes in endocrine (prostate) cancer: EC 3.4.24.15 (EP24.15). Protein Pept Lett (2004) 11(5):471–8. doi: 10.2174/0929866043406607
30. Dong KW, Yu KL, Roberts JL. Identification of a major up-stream transcription start site for the human progonadotropin-releasing hormone gene used in reproductive tissues and cell lines. Mol Endocrinol (1993) 7(12):1654–66. doi: 10.1210/mend.7.12.8145771
31. Shrimpton CN, Glucksman MJ, Lew RA, Tullai JW, Margulies EH, Roberts JL, et al. Thiol activation of endopeptidase EC 3.4.24.15. a novel mechanism for the regulation of catalytic activity. J Biol Chem (1997) 272(28):17395–9. doi: 10.1074/jbc.272.28.17395
32. Shrimpton CN, Smith AI, Lew RA. Soluble metalloendopeptidases and neuroendocrine signaling. Endocr Rev (2002) 23(5):647–64. doi: 10.1210/er.2001-0032
33. Lew RA, Tetaz TJ, Glucksman MJ, Roberts JL, Smith AI. Evidence for a two-step mechanism of gonadotropin-releasing hormone metabolism by prolyl endopeptidase and metalloendopeptidase EC 3.4.24.15 in ovine hypothalamic extracts. J Biol Chem (1994) 269(17):12626–32.
34. Cleverly K, Wu TJ. Is the metalloendopeptidase EC 3.4.24.15 (EP24.15), the enzyme that cleaves luteinizing hormone-releasing hormone (LHRH), an activating enzyme? Reproduction (2010) 139(2):319–30. doi: 10.1530/REP-09-0117
35. De Camargo AC, Da Fonseca MJ, Caldo H, De Morais Carvalho K. Influence of the carboxyl terminus of luteinizing hormone-releasing hormone and bradykinin on hydrolysis by brain endo-oligopeptidases. J Biol Chem (1982) 257(16):9265–7. doi: 10.1016/S0021-9258(18)34062-6
36. Bourguignon JP, Alvarez Gonzalez ML, Gerard A, Franchimont P. Gonadotropin releasing hormone inhibitory autofeedback by subproducts antagonist at n-methyl-D-aspartate receptors: a model of autocrine regulation of peptide secretion. Endocrinology. (1994) 134(3):1589–92. doi: 10.1210/endo.134.3.8119202
37. Wu TJ, Mani SK, Glucksman MJ, Roberts JL. Stimulation of luteinizing hormone-releasing hormone (LHRH) gene expression in GT1-7 cells by its metabolite, LHRH-(1-5). Endocrinology. (2005) 146(1):280–6. doi: 10.1210/en.2004-0560
38. Baldwin EL, Wegorzewska IN, Flora M, Wu TJ. Regulation of type II luteinizing hormone-releasing hormone (LHRH-II) gene expression by the processed peptide of LHRH-I, LHRH-(1-5) in endometrial cells. Exp Biol Med (Maywood). (2007) 232(1):146–55.
39. Ieda N, Assadullah, Minabe S, Ikegami K, Watanabe Y, Sugimoto Y, et al. GnRH(1-5), a metabolite of gonadotropin-releasing hormone, enhances luteinizing hormone release via activation of kisspeptin neurons in female rats. Endocr J (2020) 67(4):409–18. doi: 10.1507/endocrj.EJ19-0444
40. Kang JL, Wang DY, Wang XX, Yu J. Up-regulation of apoptosis by gonadotrophin-releasing hormone agonist in cultures of endometrial cells from women with symptomatic myomas. Hum Reprod (2010) 25(9):2270–5. doi: 10.1093/humrep/deq178
41. Wu HM, Cheng JC, Wang HS, Huang HY, MacCalman CD, Leung PC. Gonadotropin-releasing hormone type II induces apoptosis of human endometrial cancer cells by activating GADD45alpha. Cancer Res (2009) 69(10):4202–8. doi: 10.1158/0008-5472.CAN-08-4591
42. Grundker C, Emons G. Role of gonadotropin-releasing hormone (GnRH) in ovarian cancer. Reprod Biol Endocrinol (2003) 1:65. doi: 10.1186/1477-7827-1-65
43. Larco DO, Semsarzadeh NN, Cho-Clark M, Mani SK, Wu TJ. Beta-arrestin 2 is a mediator of GnRH-(1-5) signaling in immortalized GnRH neurons. Endocrinology. (2013) 154(12):4726–36. doi: 10.1210/en.2013-1286
44. Kober P, Bujko M, Oledzki J, Tysarowski A, Siedlecki JA. Methyl-CpG binding column-based identification of nine genes hypermethylated in colorectal cancer. Mol Carcinog. (2011) 50(11):846–56. doi: 10.1002/mc.20763
45. Cho-Clark M, Larco DO, Semsarzadeh NN, Vasta F, Mani SK, Wu TJ. GnRH-(1-5) transactivates EGFR in ishikawa human endometrial cells via an orphan G protein-coupled receptor. Mol Endocrinol (2014) 28(1):80–98. doi: 10.1210/me.2013-1203
46. Bates B, Zhang L, Nawoschik S, Kodangattil S, Tseng E, Kopsco D, et al. Characterization of Gpr101 expression and G-protein coupling selectivity. Brain Res (2006) 1087(1):1–14. doi: 10.1016/j.brainres.2006.02.123
47. Trivellin G, Bjelobaba I, Daly AF, Larco DO, Palmeira L, Faucz FR, et al. Characterization of GPR101 transcript structure and expression patterns. J Mol Endocrinol (2016) 57(2):97–111. doi: 10.1530/JME-16-0045
48. Flak MB, Koenis DS, Sobrino A, Smith J, Pistorius K, Palmas F, et al. GPR101 mediates the pro-resolving actions of RvD5n-3 DPA in arthritis and infections. J Clin Invest. (2020) 130(1):359–73. doi: 10.1172/JCI131609
49. Nilaweera KN, Ozanne D, Wilson D, Mercer JG, Morgan PJ, Barrett P. G Protein-coupled receptor 101 mRNA expression in the mouse brain: altered expression in the posterior hypothalamus and amygdala by energetic challenges. J Neuroendocrinol. (2007) 19(1):34–45. doi: 10.1111/j.1365-2826.2006.01502.x
50. Lee DK, Nguyen T, Lynch KR, Cheng R, Vanti WB, Arkhitko O, et al. Discovery and mapping of ten novel G protein-coupled receptor genes. Gene. (2001) 275(1):83–91. doi: 10.1016/S0378-1119(01)00651-5
51. Kakarala KK, Jamil K. Sequence-structure based phylogeny of GPCR class a rhodopsin receptors. Mol Phylogenet Evol (2014) 74:66–96. doi: 10.1016/j.ympev.2014.01.022
52. Martin AL, Steurer MA, Aronstam RS. Constitutive activity among orphan class-a G protein coupled receptors. PloS One (2015) 10(9):e0138463. doi: 10.1371/journal.pone.0138463
53. Abboud D, Daly AF, Dupuis N, Bahri MA, Inoue A, Chevigne A, et al. GPR101 drives growth hormone hypersecretion and gigantism in mice via constitutive activation of g(s) and G(q/11). Nat Commun (2020) 11(1):4752. doi: 10.1038/s41467-020-18500-x
54. Trivellin G, Daly AF, Faucz FR, Yuan B, Rostomyan L, Larco DO, et al. Gigantism and acromegaly due to Xq26 microduplications and GPR101 mutation. N Engl J Med (2014) 371(25):2363–74. doi: 10.1056/NEJMoa1408028
55. Trivellin G, Faucz FR, Daly AF, Beckers A, Stratakis CA. HEREDITARY ENDOCRINE TUMOURS: CURRENT STATE-OF-THE-ART AND RESEARCH OPPORTUNITIES: GPR101, an orphan GPCR with roles in growth and pituitary tumorigenesis. Endocr Relat Cancer (2020) 27(8):T87–97. doi: 10.1530/ERC-20-0025
56. Nilaweera KN, Wilson D, Bell L, Mercer JG, Morgan PJ, Barrett P. G Protein-coupled receptor 101 mRNA expression in supraoptic and paraventricular nuclei in rat hypothalamus is altered by pregnancy and lactation. Brain Res (2008) 1193:76–83. doi: 10.1016/j.brainres.2007.11.048
57. Cho-Clark M, Larco DO, Zahn BR, Mani SK, Wu TJ. GnRH-(1-5) activates matrix metallopeptidase-9 to release epidermal growth factor and promote cellular invasion. Mol Cell Endocrinol (2015) 415:114–25. doi: 10.1016/j.mce.2015.08.010
58. Emons G, Grundker C. The role of gonadotropin-releasing hormone (GnRH) in endometrial cancer. Cells. (2021) 10(2):292. doi: 10.3390/cells10020292
59. Fekete M, Bajusz S, Groot K, Csernus VJ, Schally AV. Comparison of different agonists and antagonists of luteinizing hormone-releasing hormone for receptor-binding ability to rat pituitary and human breast cancer membranes. Endocrinology. (1989) 124(2):946–55. doi: 10.1210/endo-124-2-946
60. Emons G, Schroder B, Ortmann O, Westphalen S, Schulz KD, Schally AV. High affinity binding and direct antiproliferative effects of luteinizing hormone-releasing hormone analogs in human endometrial cancer cell lines. J Clin Endocrinol Metab (1993) 77(6):1458–64. doi: 10.1210/jcem.77.6.8263128
61. Limonta P, Dondi D, Moretti RM, Maggi R, Motta M. Antiproliferative effects of luteinizing hormone-releasing hormone agonists on the human prostatic cancer cell line LNCaP. J Clin Endocrinol Metab (1992) 75(1):207–12. doi: 10.1210/jcem.75.1.1320049
62. Imai A, Horibe S, Takagi H, Fuseya T, Tamaya T. Signal transduction of GnRH receptor in the reproductive tract tumor. Endocr J (1996) 43(3):249–60. doi: 10.1507/endocrj.43.249
63. Schally AV, Comaru-Schally AM, Nagy A, Kovacs M, Szepeshazi K, Plonowski A, et al. Hypothalamic hormones and cancer. Front Neuroendocrinol. (2001) 22(4):248–91. doi: 10.1006/frne.2001.0217
64. Eicke N, Gunthert AR, Emons G, Grundker C. GnRH-II agonist [D-Lys6]GnRH-II inhibits the EGF-induced mitogenic signal transduction in human endometrial and ovarian cancer cells. Int J Oncol (2006) 29(5):1223–9. doi: 10.3892/ijo.29.5.1223
65. Grundker C, Volker P, Schulz KD, Emons G. Luteinizing hormone-releasing hormone agonist triptorelin and antagonist cetrorelix inhibit EGF-induced c-fos expression in human gynecological cancers. Gynecol Oncol (2000) 78(2):194–202. doi: 10.1006/gyno.2000.5863
66. Liebow C, Lee MT, Kamer AR, Schally AV. Regulation of luteinizing hormone-releasing hormone receptor binding by heterologous and autologous receptor-stimulated tyrosine phosphorylation. Proc Natl Acad Sci U S A. (1991) 88(6):2244–8. doi: 10.1073/pnas.88.6.2244
67. Wu HM, Wang HS, Huang HY, Lai CH, Lee CL, Soong YK, et al. Gonadotropin-releasing hormone type II (GnRH-II) agonist regulates the invasiveness of endometrial cancer cells through the GnRH-I receptor and mitogen-activated protein kinase (MAPK)-dependent activation of matrix metalloproteinase (MMP)-2. BMC Cancer. (2013) 13:300. doi: 10.1186/1471-2407-13-300
68. Cheung LW, Leung PC, Wong AS. Gonadotropin-releasing hormone promotes ovarian cancer cell invasiveness through c-jun NH2-terminal kinase-mediated activation of matrix metalloproteinase (MMP)-2 and MMP-9. Cancer Res (2006) 66(22):10902–10. doi: 10.1158/0008-5472.CAN-06-2217
69. Walters K, Chin YP, Wu TJ. A processed metabolite of luteinizing hormone-releasing hormone has proliferative effects in endometrial cells. Am J Obstet Gynecol. (2007) 196(1):33 e1–5. doi: 10.1016/j.ajog.2006.07.054
70. Nicholson RI, Gee JM, Harper ME. EGFR and cancer prognosis. Eur J Cancer. (2001) 37 Suppl 4:S9–15. doi: 10.1016/S0959-8049(01)00231-3
71. Normanno N, De Luca A, Bianco C, Strizzi L, Mancino M, Maiello MR, et al. Epidermal growth factor receptor (EGFR) signaling in cancer. Gene. (2006) 366(1):2–16. doi: 10.1016/j.gene.2005.10.018
72. Dedes KJ, Wetterskog D, Ashworth A, Kaye SB, Reis-Filho JS. Emerging therapeutic targets in endometrial cancer. Nat Rev Clin Oncol (2011) 8(5):261–71. doi: 10.1038/nrclinonc.2010.216
73. Dong P, Kaneuchi M, Konno Y, Watari H, Sudo S, Sakuragi N. Emerging therapeutic biomarkers in endometrial cancer. BioMed Res Int (2013) 2013:130362. doi: 10.1155/2013/130362
74. Reyes HD, Thiel KW, Carlson MJ, Meng X, Yang S, Stephan JM, et al. Comprehensive profiling of EGFR/HER receptors for personalized treatment of gynecologic cancers. Mol Diagn Ther (2014) 18(2):137–51. doi: 10.1007/s40291-013-0070-3
75. Yang WN, Ai ZH, Wang J, Xu YL, Teng YC. Correlation between the overexpression of epidermal growth factor receptor and mesenchymal makers in endometrial carcinoma. J Gynecol Oncol (2014) 25(1):36–42. doi: 10.3802/jgo.2014.25.1.36
76. Makker V, MacKay H, Ray-Coquard I, Levine DA, Westin SN, Aoki D, et al. Endometrial cancer. Nat Rev Dis Primers. (2021) 7(1):88. doi: 10.1038/s41572-021-00324-8
77. Chen HY, Chiang YF, Huang JS, Huang TC, Shih YH, Wang KL, et al. Isoliquiritigenin reverses epithelial-mesenchymal transition through modulation of the TGF-beta/Smad signaling pathway in endometrial cancer. Cancers (Basel) (2021) 13(6):1236. doi: 10.3390/cancers13061236
78. Xiong S, Cheng JC, Klausen C, Zhao J, Leung PC. TGF-beta1 stimulates migration of type II endometrial cancer cells by down-regulating PTEN via activation of SMAD and ERK1/2 signaling pathways. Oncotarget. (2016) 7(38):61262–72. doi: 10.18632/oncotarget.11311
79. Neuzillet C, Tijeras-Raballand A, Cohen R, Cros J, Faivre S, Raymond E, et al. Targeting the TGFbeta pathway for cancer therapy. Pharmacol Ther (2015) 147:22–31. doi: 10.1016/j.pharmthera.2014.11.001
80. Li Q. Tumor-suppressive signaling in the uterus. Proc Natl Acad Sci U S A. (2019) 116(9):3367–9. doi: 10.1073/pnas.1821606116
81. Larco DO, Bauman BM, Cho-Clark M, Mani SK, Wu TJ. GnRH-(1-5) inhibits TGF-beta signaling to regulate the migration of immortalized gonadotropin-releasing hormone neurons. Front Endocrinol (Lausanne). (2018) 9:45. doi: 10.3389/fendo.2018.00045
82. Bauman BM, Yin W, Gore AC, Wu TJ. Regulation of gonadotropin-releasing hormone-(1-5) signaling genes by estradiol is age dependent. Front Endocrinol (Lausanne). (2017) 8:282. doi: 10.3389/fendo.2017.00282
83. Cho-Clark MJ, Sukumar G, Vidal NM, Raiciulescu S, Oyola MG, Olsen C, et al. Comparative transcriptome analysis between patient and endometrial cancer cell lines to determine common signaling pathways and markers linked to cancer progression. Oncotarget. (2021) 12(26):2500–13. doi: 10.18632/oncotarget.28161
Keywords: GnRH-(1-5), endometrial cancer, GnRH, GPR101, EP24.15
Citation: Cho-Clark MJ, Watkins A and Wu TJ (2023) The role of GnRH metabolite, GnRH-(1-5), in endometrial cancer. Front. Endocrinol. 14:1183278. doi: 10.3389/fendo.2023.1183278
Received: 09 March 2023; Accepted: 29 March 2023;
Published: 14 April 2023.
Edited by:
Marco Allán Pérez-Solis, Mexican Social Security Institute (IMSS), MexicoReviewed by:
Maria Andrea Camilletti, National Council for Scientific and Technical Research (CONICET), ArgentinaCopyright © 2023 Cho-Clark, Watkins and Wu. This is an open-access article distributed under the terms of the Creative Commons Attribution License (CC BY). The use, distribution or reproduction in other forums is permitted, provided the original author(s) and the copyright owner(s) are credited and that the original publication in this journal is cited, in accordance with accepted academic practice. No use, distribution or reproduction is permitted which does not comply with these terms.
*Correspondence: T. John Wu, twu@usuhs.edu
†These authors have contributed equally to this work and share first authorship