- 1Department of Endocrinology, Tongji Hospital, Tongji Medical College, Huazhong University of Science and Technology, Wuhan, Hubei, China
- 2Branch of National Clinical Research Center for Metabolic Diseases, Wuhan, Hubei, China
- 3Department of Clinical Nutrition, Deyang People’s Hospital, Deyang, Sichuan, China
Background: Heart failure with preserved ejection fraction (HFpEF), a major cause of morbidity and mortality in patients with type 2 diabetes mellitus (T2DM), is frequently coexisted with obesity, poor glycemic, blood pressure (BP), and/or lipid control. We aimed to investigate the associations of nonalcoholic fatty liver disease (NAFLD) and its advanced fibrosis with HFpEF according to obesity, glycated hemoglobin A1c (HbA1c), BP, and low-density lipoprotein cholesterol (LDL-C) goal achievement status in T2DM patients.
Methods: A total of 2,418 T2DM patients who were hospitalized were cross-sectionally assessed. Liver fibrosis was evaluated by non-invasive biomarkers. Logistic regression analysis was used to evaluate the independent and combined associations of fibrosis status and diabetic care goal attainments with HFpEF risk.
Results: Simple steatosis was not associated with HFpEF risk compared with patients without steatosis, while advanced liver fibrosis was found to have significantly higher odds for HFpEF risk (odds ratio,1.59; 95% confidence interval, 1.22-2.08). Advanced fibrosis in NAFLD was significantly associated with an increased risk of HFpEF, regardless of obesity status, HbA1c, BP, and LDL-C goal achievement status. P values for the interactions between fibrosis status and HbA1c control status, fibrosis status and BP control status, fibrosis status and LDL-C control status, and fibrosis status and body mass index (BMI) status on HFpEF risk were 0.021, 0.13, 0.001, and 0.23, respectively.
Conclusion: In patients with T2DM, advanced hepatic fibrosis was significantly associated with HFpEF risk, irrespective of obesity status, HbA1c, BP, and LDL-C goal attainment status. Further, HbA1c and LDL-C goal attainment status modified this association.
1 Introduction
Heart failure (HF) is a major cause of morbidity and mortality in type 2 diabetes mellitus (T2DM) (1). T2DM patients initially progress to diastolic dysfunction (HF with preserved ejection fraction, HFpEF) and then to systolic dysfunction (HF with reduced ejection fraction, HFrEF) (2). Similar to HFrEF, HFpEF is associated with worse clinical prognosis and increased risk of hospitalization and mortality (3). HFpEF is more frequent in diabetic patients with obesity, poor glycemic, blood pressure (BP), and/or lipid control. Evidence showed that weight control and management of hyperglycemia, hypertension, and/or dyslipidemia are effective in preventing HF (4). Thus, the contribution of such modifiable risk factors to HFpEF risk is becoming a concern for its prevention.
Nonalcoholic fatty liver disease (NAFLD) has become one of the major liver diseases and a common indication for liver transplantation worldwide (5). The NAFLD epidemic has paralleled that of the diabetes epidemic. Approximately 60-70% of patients with T2DM suffered from NAFLD (6, 7). These two frequently overlapping diseases negatively influence each other’s prognoses. For example, T2DM increases the burden of NAFLD due to an increased risk of progression to non-alcoholic steatohepatitis (NASH), fibrosis, and cirrhosis (8). Vice versa, patients with NAFLD more commonly progress toward diabetic micro- and macro-vascular complications (9). Although one study has reported the associations of NAFLD and the severity of liver fibrosis with HFpEF in patients with T2DM (10), it included limited sample size. In addition, it remains unclear whether NAFLD and its advanced stages are associated with different risks of HFpEF in distinct populations defined by obesity status, glycated hemoglobin A1c (HbA1c), BP, and low-density lipoprotein cholesterol (LDL-C) goal attainment status.
In view of the links between diabetes care goal achievements and HFpEF (11, 12), and amid the ongoing NAFLD and diabetes epidemics, understanding the role of NAFLD and its advanced fibrosis as risk factors for HFpEF in different care goal attainment status is of clinical importance. Thus, we aimed to investigate how, independent of other risk factors, NAFLD and its advanced fibrosis and diabetic care goal achievements interactively influence HFpEF risk in T2DM patients.
2 Methods
2.1 Study design and population
This cross-sectional study included 3,011 T2DM patients hospitalized in the Department of Endocrinology, Tongji Hospital, Tongji Medical College, Huazhong University of Science and Technology (Wuhan, China) between 2018 and 2021. T2DM was diagnosed according to the 2022 American Diabetes Association (ADA) criteria (13). Patients with implantable cardiac devices such as pacemakers, and implantable cardioverter-defibrillators were excluded to avoid HFpEF caused by tachycardiomyopahy. Patients with structural causes of HFpEF such as amyloidosis, hypertrophic obstructive cardiomyopathy, and dilated cardiomyopathy were also excluded. Further, we excluded 18 patients with left ventricular ejection fraction (LVEF) <50%, 154 patients with a positive hepatitis B surface antigen or hepatitis C antibody, 2 with excessive alcohol intake (>30 g/day for men and > 20g/day for women), and 412 with missing data on echocardiography measurement, liver ultrasonography, and/or biochemical measurements. Patients with hereditary causes of liver disease such as Wilson disease, and hereditary hemochromatosis, or taking drugs such as amiodarone, and corticosteroids that may incur fatty liver were also excluded. The remaining available 2,418 patients were included in the present analyses. The flow diagram for the target population in the study was shown in Figure 1.
According to the Private Information Protection Law, information that might identify patients was safeguarded by the Computer Center. This study was approved by the ethics committee of Tongji Hospital. Because we only retrospectively accessed a de-identified database for purposes of analysis, the informed consent requirement was exempted by the institutional review board. The procedures were followed in accordance with the ethical standards of the responsible committee on human experimentation and with the Helsinki Declaration of 1975, as revised in 1983.
2.2 Clinical measurements
Patients’ data including age, sex, height, weight, histories of current and previous illness, and medical treatments were obtained from medical records. Height, weight, waist circumference (WC), and BP were measured following standardized protocols from the World Health Organization (WHO). Patients’ seated BP was measured twice for every 5 min on the right arm after 5 min of rest with a sphygmomanometer. The mean of the two readings was used in data analysis. Body mass index (BMI) was calculated as weight (in kilograms) divided by the square of height (in meters).
Overnight fasting (for at least 8 h) blood samples were collected. All blood specimens were tested immediately after collection. HbA1c was measured using high performance liquid chromatography (D-10™; Bio-Rad Laboratories, Hercules, CA, USA). Fasting plasma glucose (FPG), triglycerides (TG), total cholesterol (TC), high-density lipoprotein cholesterol (HDL-C), LDL-C, alanine aminotransferase (ALT), aspartate aminotransferase (AST), and albumin (ALB) were measured on an autoanalyzer (Cobas C8000, Roche, Mannheim, Germany). Platelet (PLT) was assessed by sheath flow DC assay (XN-9000, Sysmex, Kobe, Japan). Hepatitis viral antigens/antibodies were detected with corresponding Architect reagents (Architect i2000, Abbott Diagnostics, Abbott Park, IL). All the blood measurements followed the same protocol.
2.3 Ultrasonography
Ultrasound tests were performed by certified sonographers using a high-resolution, real-time scanner (model SSD-2000; Aloka Co., Ltd., Tokyo Japan). All individuals were fasted overnight before imaging. Certified radiologists used standard criteria in evaluating the presence or absence of hepatic fat. Liver steatosis was defined based on the following criteria: the brightness of liver parenchyma, presence of liver to kidney contrast, presence of deep beam attenuation, and vascular blurring (14). Left ventricular end diastolic and end systolic volumes and ejection fraction (EF) were measured by 2-dimensional M-mode echocardiography at the apical two chamber and four-chamber views when patients were at rest. Transmitral peak early diastolic velocity (E) and peak late diastolic velocity (A) were measured by pulsed-wave Doppler echocardiography. Early annular diastolic tissue velocity (e’) was measured by pulsed-wave tissue Doppler echocardiography (15).
2.4 Definitions
According to the WHO Asia-Pacific guidelines (16), obesity was defined as BMI ≥ 25 kg/m2
According to the American Diabetes Association (ADA) criteria (17, 18), HbA1c goal achievement was defined as HbA1c level < 7.0%; BP goal achievement as systolic/diastolic BP < 130/80 mmHg; LDL-C goal achievement as LDL-C level < 100 mg/mL.
HFpEF was defined as EF ≥ 50% with either (1) E/A < 0.8, E/e’ < 8, and peak e’ < 10 cm/s, or (2) 0.8 < E/A < 1.5, 8 < E/e’ < 14, and peak e’ < 8 cm/s, or (3) E/A > 1.5, E/e’ >14 and peak e’ < 5 cm/s (19).
The presence of advanced liver fibrosis in patients with NAFLD was defined as the presence of a high probability for advanced fibrosis calculated by NAFLD fibrosis score (NFS) (20), BARD score (21), and fibrotic NASH index (FNI) (22), which have been extensively validated (23). The NFS was calculated as -1.675 + 0.037×age (years) + 0.094×BMI (kg/m2) + 1.13×impaired fasting glucose or diabetes (yes=1, no=0) + 0.99AST/ALT ratio-0.013×platelet count (109/L) - 0.66×albumin (g/dL). Advanced fibrosis was defined as NFS ≥-1.455. The BARD score includes AST/ALT ratio ≥0.8 (2 points), BMI ≥28 kg/m2 (1 point), and the presence of diabetes (1 point). A score of ≥ 2 was associated with advanced fibrosis. The FNI was calculated as e(-10.33+2.54*1nAST[U/L]+3.86*lnHbA1c[%]-1.66*lnHDL[mg/dL])/1+e(-10.33+2.54*1nAST[U/L]+3.86*lnHbA1c[%]-1.66*lnHDL[mg/dL]) (22). Advanced fibrosis was defined as FNI >0.1. The homeostasis model assessment estimate of insulin resistance (HOMA-IR) was calculated using the following formula: HOMA-IR=fasting plasma glucose (mmol/L) ×fasting insulin (mIU/L)/22.5 (24).
2.5 Statistical analysis
All statistical analyses were conducted using the SPSS software (version 20.0 for Windows; SPSS, Chicago, IL, USA). Continuous variables were presented as means and standard deviations (SDs). Categorical variables were presented as percentages. One-way analysis of variance was applied to compare differences in means across groups. Bonferroni correction was applied to adjust P values for multiple comparisons. A Chi-square test was performed to assess differences of proportions across groups. Logistic regression models were used to estimate the associations (odds ratios [ORs], with 95% confidence intervals [CIs]) between the presence of NAFLD or advanced liver fibrosis and the risk of HFpEF. Three models were fitted. Model 1 was adjusted for age and sex. Model 2 was additionally adjusted for the duration of T2DM, antihypertensive drug use, lipid-lowering drug use, antidiabetic agent use, smoking, alcohol drinking, BMI, systolic BP, HbA1c, TG, and LDL-C. Model 3 was further adjusted for HOMA-IR. Further examination of the association of NAFLD and advanced liver fibrosis with HFpEF risk in BMI categories, HbA1c, BP, and LDL-C status were also evaluated. To assess if the associations differed by BMI status, logistic regression analysis was utilized to examine potential interaction effects between NAFLD/advanced liver fibrosis and BMI categories. The process was repeated separately for subgroups stratified by HbA1c, BP, and LDL-C status. Significance was accepted at a two-tailed P <0.05.
3 Results
Of the 2,418 T2DM patients included in the present analyses, the mean age was 52.9 years, and 62.8% were men. Nearly half of the T2DM patients (49.7%) suffered from NAFLD. The prevalence of NAFLD with advanced fibrosis defined by NFS and BARD was 29.5% and 35.2%, respectively. HFpEF was presented in 492 (20.3%) patients.
As shown in Table 1, T2DM patients with simple steatosis were younger, more likely to be male, and had higher BMI, WC, diastolic BP, AST, ALT, HbA1c, and adverse lipids than patients without NAFLD (all P value <0.05). T2DM patients with NFS-defined advanced fibrosis had even worse deteriorated anthropometric and cardiovascular risk profile. Further, they had the lowest septal e’ and E/A ratio, the largest IVSD and LVIDD, and were more likely to suffer from HFpEF (all P value <0.05). Similar findings were observed when BARD was used to define advanced fibrosis (Supplementary Table 1). HOMA-IR showed a positive relationship with NFS (r=0.017), BARD (r=0.018), and FNI (r=0.046).
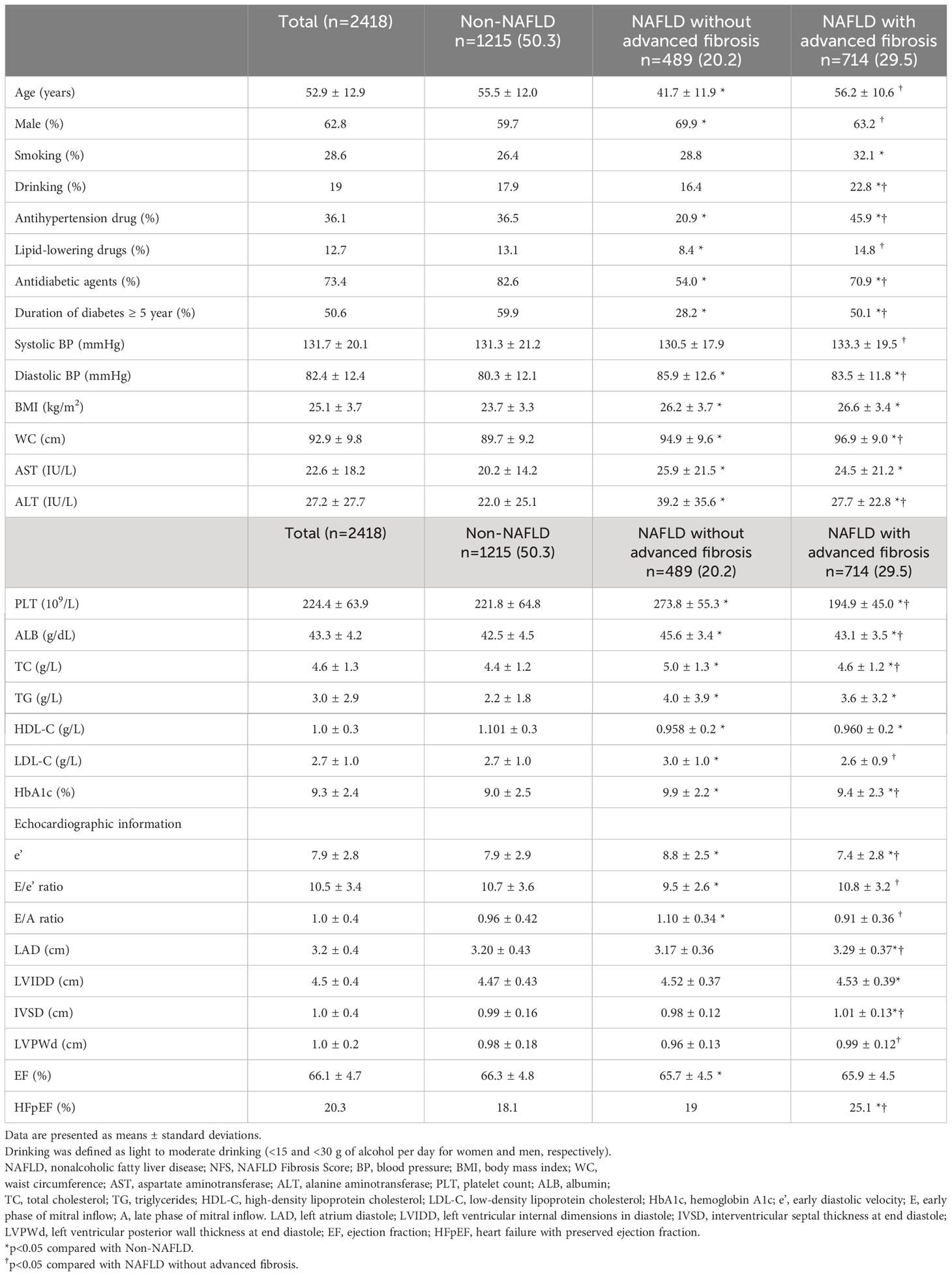
Table 1 Characteristics of the study population according to NAFLD and advanced fibrosis status based on NFS.
The ORs and 95% CIs for HFpEF from NAFLD and its advanced form compared with those without NAFLD were presented in Table 2. NAFLD was associated with an increased risk of HFpEF (model 1). Further adjustment for potential intermediate variables including BMI and HOMA-IR (model 3), NAFLD was still strongly associated with HFpEF risk (OR,1.50; 95% CI, 1.18 to 1.91; P=0.001). However, when NAFLD was stratified by NFS-defined advanced liver fibrosis, simple steatosis was no longer associated with HFpEF risk compared with patients without steatosis, while NFS-defined advanced liver fibrosis was found to have a significantly higher OR for HFpEF after sufficient adjustment (OR,1.59; 95% CI, 1.22 to 2.08; P=0.001) (model 3). When BARD or FNI was used to define advanced fibrosis, similar findings were observed (Table 2).
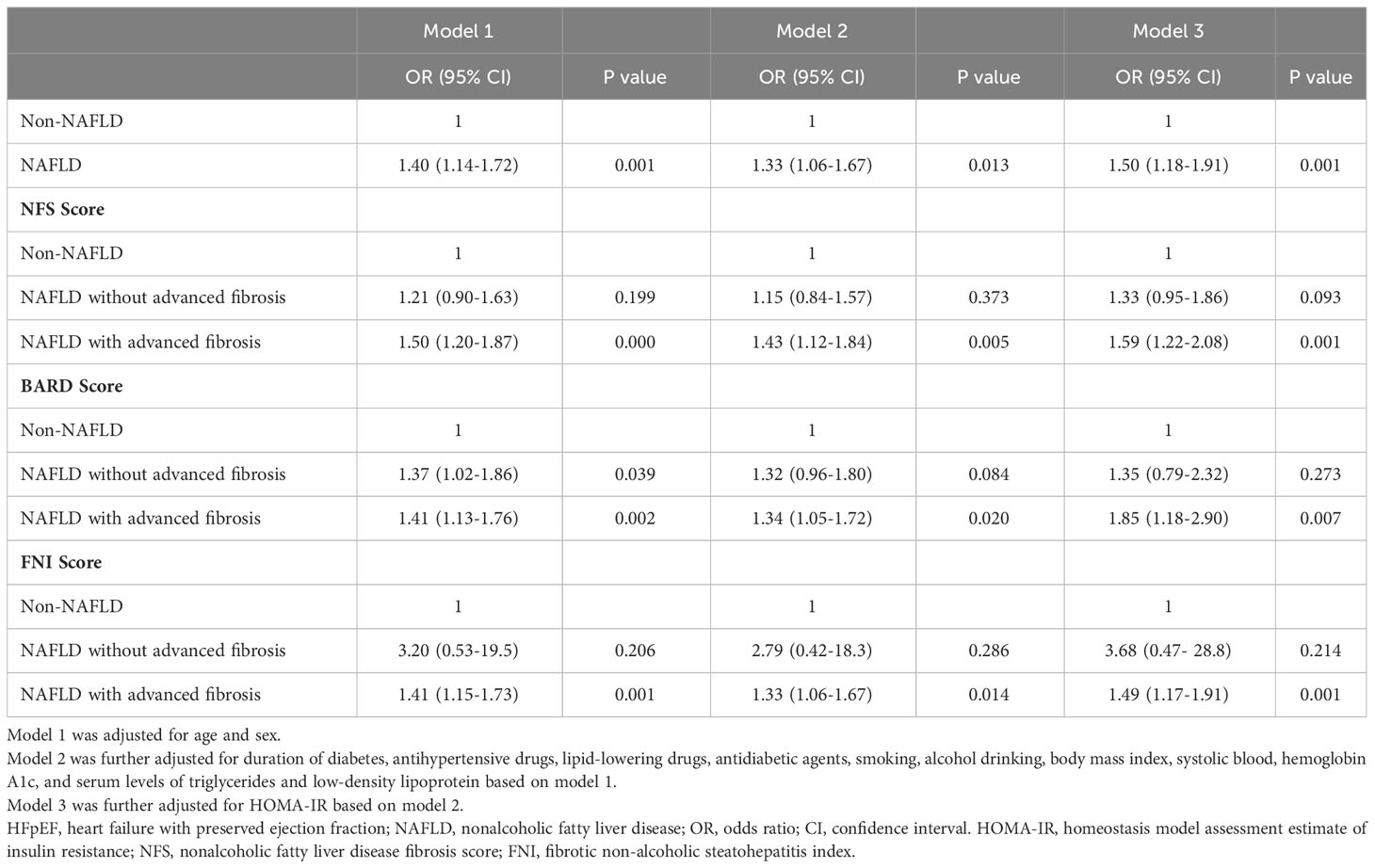
Table 2 Odds ratios (95% confidence intervals, CI) of the risk of HFpEF from NAFLD and its advanced fibrosis.
Since comorbidities, such as chronic kidney disease (CKD) and cardio-cerebrovascular disease, that frequently coexisted with hospitalized diabetic patients were identified as important determinants that influence the short-term and long-term outcomes (25), we stratified our analysis by comorbidity status. The prevalence of CKD and cardio-cerebrovascular disease was 34.7%, and 13.6%, respectively. Advanced liver fibrosis was associated with an increased HFpEF risk, regardless of CKD status (Supplementary Tables 2, 3) and cardio-cerebrovascular disease status (Supplementary Table 4, 5).
We did a sensitivity analysis after excluding smokers. The results were essentially the same (Supplementary Table 6).
Table 3 listed the independent and joint associations of NAFLD status and diabetic care goal attainments with HFpEF risk. After adjusting for potential intermediate variables including HOMA-IR, NFS-defined fibrosis remained a significant predictor of HFpEF, irrespective of HbA1c goal attainment status (OR, 2.65; 95% CI, 1.43 to 4.91; P <0.001 in subjects with fibrosis but optimal HbA1c control, and OR, 1.97; 95% CI, 1.27 to 3.06; P <0.001 in subjects with fibrosis and poor HbA1c control). The higher OR in patients with optimal HbA1c but with liver fibrosis than in patients with poor HbA1c control but without liver fibrosis (OR, 1.78; 95% CI, 1.09 to 2.90; P <0.001) and the significant interactions between fibrosis status and HbA1c control status on the risk of HFpEF (P=0.02) indicated that the association between liver fibrosis and HFpEF was modified by HbA1c goal attainment status.
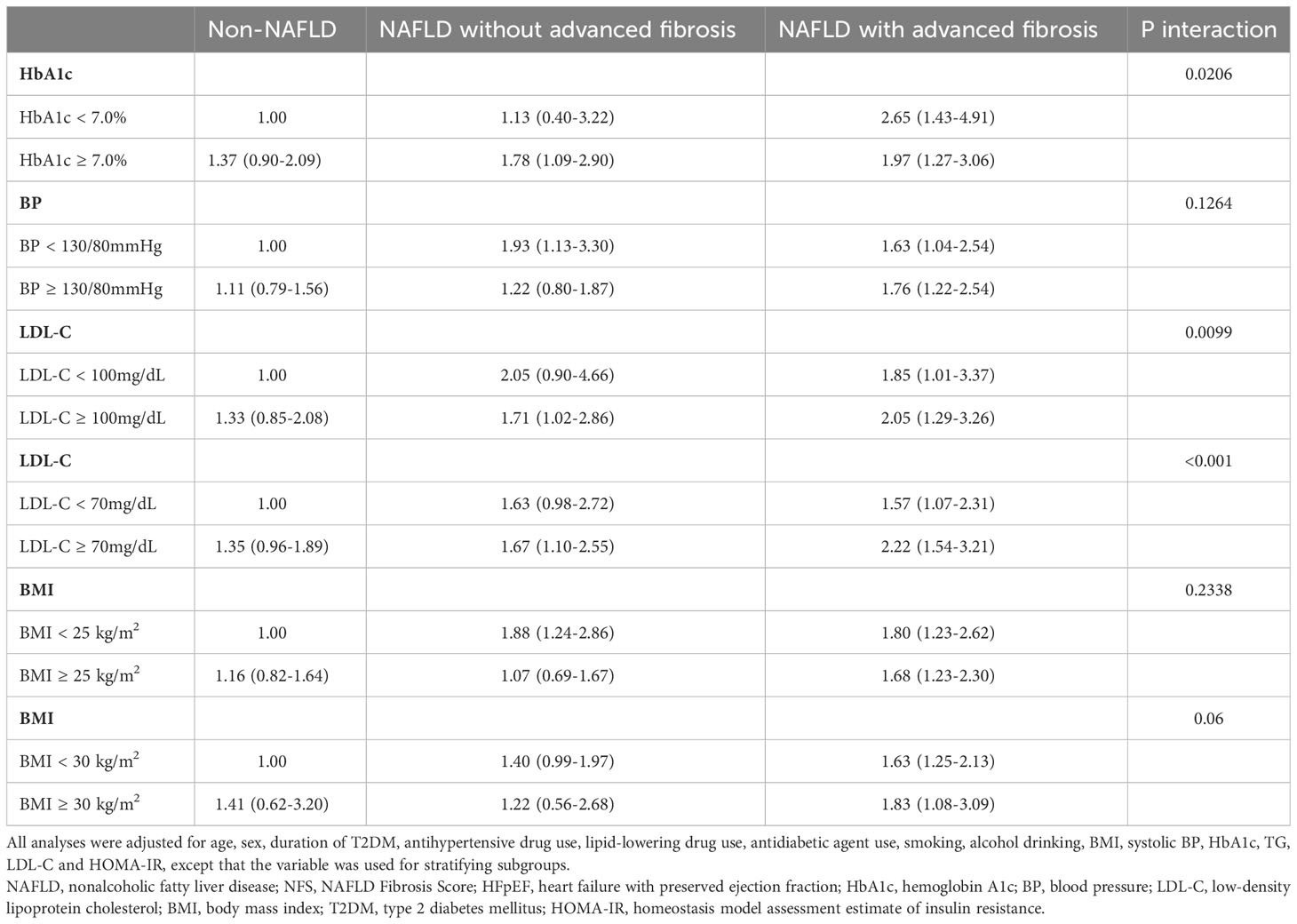
Table 3 Odds ratios (95% confidence intervals) of the independent and joint associations of NAFLD and its advanced fibrosis based on NFS and diabetic care goal attainment status for HFpEF risk.
The relative risks were higher in patients with NAFLD with significant NFS-defined liver fibrosis compared with subjects with NAFLD but without liver fibrosis or those without NAFLD, regardless of BP goal attainment status (OR, 1.63; 95% CI, 1.04 to 2.54; P <0.001 in subjects with fibrosis but optimal BP control, and OR, 1.76; 95% CI, 1.22 to 2.54; P <0.001 in subjects with fibrosis and poor BP control) (Table 3). The interactions between fibrosis status and BP goal attainment status on HFpEF did not reach statistical significance (P=0.13), indicating that the association of fibrosis with HFpEF did not differ by BP goal attainment status.
The relative risks were higher in patients with NAFLD with significant NFS-defined liver fibrosis compared with subjects with NAFLD but without liver fibrosis or those without NAFLD, regardless of LDL-C goal attainment status (OR, 1.85; 95% CI, 1.01 to 3.37; P <0.001 in subjects with fibrosis but optimal LDL-C control, and OR, 2.05; 95% CI, 1.29 to 3.26; P <0.001 in subjects with fibrosis and poor LDL-C control) (Table 3). Simple steatosis also increased the risk of HFpEF in patients with poor LDL-C control (OR, 1.71; 95% CI, 1.02 to 2.86; P <0.001). The P value for NAFLD status × LDL-C goal attainment status interaction was 0.01 (Table 3), indicating that the association between liver fibrosis and HFpEF was modified by LDL-C goal attainment status.
A goal of LDL-C< 2.6 mmol/L (<100 mg/dL) for diabetic patients who were at high risk and a goal of LDL-C<1.8 mmol/L (<70 mg/dL) for diabetic patients who were at very high risk is recommended (26). Since most patients included in our study had at least one cardiovascular risk factors, we repeated the analysis when LDL-C goal achievement was defined as LDL-C<70 mg/dl. The results were essentially the same (Table 3).
NFS-defined fibrosis was associated with an increased risk of HFpEF, irrespective of BMI status (OR, 1.80; 95% CI, 1.23 to 2.62; P <0.001 in subjects with fibrosis but BMI< 25 kg/m2, and OR, 1.68; 95% CI, 1.23 to 2.30; P <0.001 in subjects with fibrosis and BMI ≥25 kg/m2) (Table 3). In addition, subjects with simple steatosis and BMI< 25 kg/m2 (OR, 1.88; 95% CI, 1.24 to 2.86; P <0.001) also had an increased HFpEF risk compared with those without NAFLD and BMI< 25 kg/m2. The interactions between fibrosis status and BMI status on HFpEF did not reach statistical significance (P=0.23), indicating that the associations of fibrosis with HFpEF did not differ by BMI status. When obesity was defined as BMI ≥ 30 kg/m2. The results were essentially the same (Table 3).
The independent and combined associations of NAFLD status and diabetic care goal attainments with HFpEF risk showed similar patternswhen BARD or FNI was used to define advanced fibrosis (Supplementary Tables 7, 8).
4 Discussion
This is, as far as we are aware, the first report to describe the associations of hepatic steatosis and/or the presence of advanced liver fibrosis with HFpEF in T2DM patients according to obesity status and metabolic goal achievement status. We found that in patients with T2DM, advanced fibrosis in NAFLD was significantly associated with an increased risk of HFpEF, regardless of obesity status, HbA1c, BP, and LDL-C goal attainment status. Further, in patients with poor LDL-C control, simple hepatic steatosis and advanced liver fibrosis were associated with an increased HFpEF risk.
The associations of HFpEF with NAFLD have been established in both the general population and T2DM patients (10, 27–30). However, most of these studies did not examine its relationship with liver fibrosis (28, 29), which is the major prognostic factor in NAFLD. The risk of cardiovascular disease as well as liver-related morbidity and mortality increased exponentially during the transition of NAFLD to advanced liver fibrosis and cirrhosis (31). Recently, Lee et al. reported that liver fibrosis, but not steatosis, was independently associated with HFpEF in non-cirrhotic subjects (32). For patients with T2DM, only one study investigated the relationship between NAFLD and its advanced fibrosis with HFpEF (10) and reported that HFpEF was independently associated with liver fibrosis, but not with steatosis. However, no information was available regarding the association of liver fibrosis with HFpEF according to obesity status and metabolic goal achievement status in T2DM patients. In the present study, we verified the significant association of liver fibrosis with increased HFpEF risk in T2DM patients. Since optimal control of HbA1c, BP, and LDL-C has been shown to have effects on liver histology (33, 34) as well as diabetic microvascular and macrovascular complications in T2DM patients (34), we investigated whether the associations of NAFLD and its advanced fibrosis with HFpEF differed by HbA1c, BP, and LDL-C goal attainment status. We found that advanced hepatic fibrosis, but not simple steatosis, was significantly associated with HFpEF risk, irrespective of HbA1c and BP goal attainment status. Further, HbA1c and LDL-C goal attainment status modified this association.
Studies showed that poor or suboptimal glycemic control may cause microvascular remodeling and cardiac fibrosis (35) and thus is correlated with an increased risk of heart failure (36). In the present study, we can assess the impact of liver fibrosis on HFpEF in T2DM aside from glycemic control by adjusting the effect of HbA1c level in logistic regression. We also assessed this association in a subgroup with optimal HbA1c control. The significant and independent associations of liver fibrosis with HFpEF in the entire study patients as well as in the subgroup who achieved the HbA1c goal attainment in our study highlight that liver fibrosis, independent of hyperglycemia, predisposes T2DM patients to an increased HFpEF risk. Interestingly, we also found that liver fibrosis was associated with a greater OR for HFpEF in those with optimal HbA1c control than in those with poor HbA1c control, and that the OR was higher in patients with optimal HbA1c but with liver fibrosis than in patients with poor HbA1c control but without liver fibrosis. Although the mechanisms for this are not known, it may be explained partly by the glycemic fluctuations. A recent study showed that patients with low HbA1c but high glycemic variability had a worse left ventricular diastolic function than patients with high HbA1c but low glycemic variability (37). Emerging evidence showed that patients with T2DM and NAFLD usually require more intensive anti-diabetic therapies to achieve an optimal glycemic control (38).
Dyslipidemia frequently coexisted with T2DM (39), in which overproduction of very low-density lipoproteins commonly occurs (40). Dyslipidemia induced lipotoxicity may eventually cause increased lipid accumulation in cardiomyocytes (41), which may increase the epicardial fat thickness, and impairment in mitochondrial function (42, 43), which may alter the myocardial energy metabolism. Evidence showed that increased epicardial fat volume and impaired myocardial glucose uptake are well-established risk factors for myocardial remodeling and diastolic dysfunction (44, 45). In the present study, we found that simple steatosis as well as liver fibrosis increased the risk of HFpEF in patients with poor LDL-C control. Reports showed that lipid-lowering drug use can reduce epicardial fat thickness (46). Hence, lipid control is important in preventing HFpEF in T2DM. We also noted an additive effect of liver fibrosis and poor LDL-C control on HFpEF. Hence, liver fibrosis further increased the HFpEF risk in patients with poor LDL-C control.
The non-obese NAFLD phenotype has sparked interest because of its high prevalence (47). Reports convinced that non-obese NAFLD subjects had severe liver histology and cardiovascular risk profiles that were identical or even worse than obese NAFLD subjects (48). Reports showed that non-obese NAFLD was more prevalent in diabetic patients compared with the general population (49), suggesting that non-obese NAFLD contributes to a large share of the disease burden of diabetes. In the present study, we found that fibrosis was associated with an increased risk of HFpEF in both non-obese and obese patients. Further, simple steatosis in non-obese patients was also associated with an increased HFpEF risk. This suggested that steatosis and liver fibrosis in T2DM patients, even if they were not obese, might be identified as an indicator of the presence of HFpEF. One possible explanation for these results may be due to a decreased capacity for storing fat in adipose tissue in non-obese NAFLD patients (50). Alterations in fatty acid trafficking lead to ectopic fat deposition in heart (51).
The hypothetical pathogenesis of the interaction of fibrosis and metabolic risk factors on HFpEF was shown in Figure 2. Circulating inflammatory mediators are often increased in NAFLD patients (30, 52). For example, increased tumor necrosis factor (TNF)-α and interleukin (IL)-6 contribute to hepatocyte injuries. Then the damaged hepatocytes will release IL-33 that can promote heart profibrogenic (53, 54). Adipokines have a close relationship with metabolic risk factors and NAFLD. For example, leptin levels were significantly increased in NAFLD. The higher levels of circulating leptin were associated with increased severity of NAFLD (55). Further, the higher levels of circulating leptin can also incur cardiac hypertrophy and endothelial dysfunction (55).
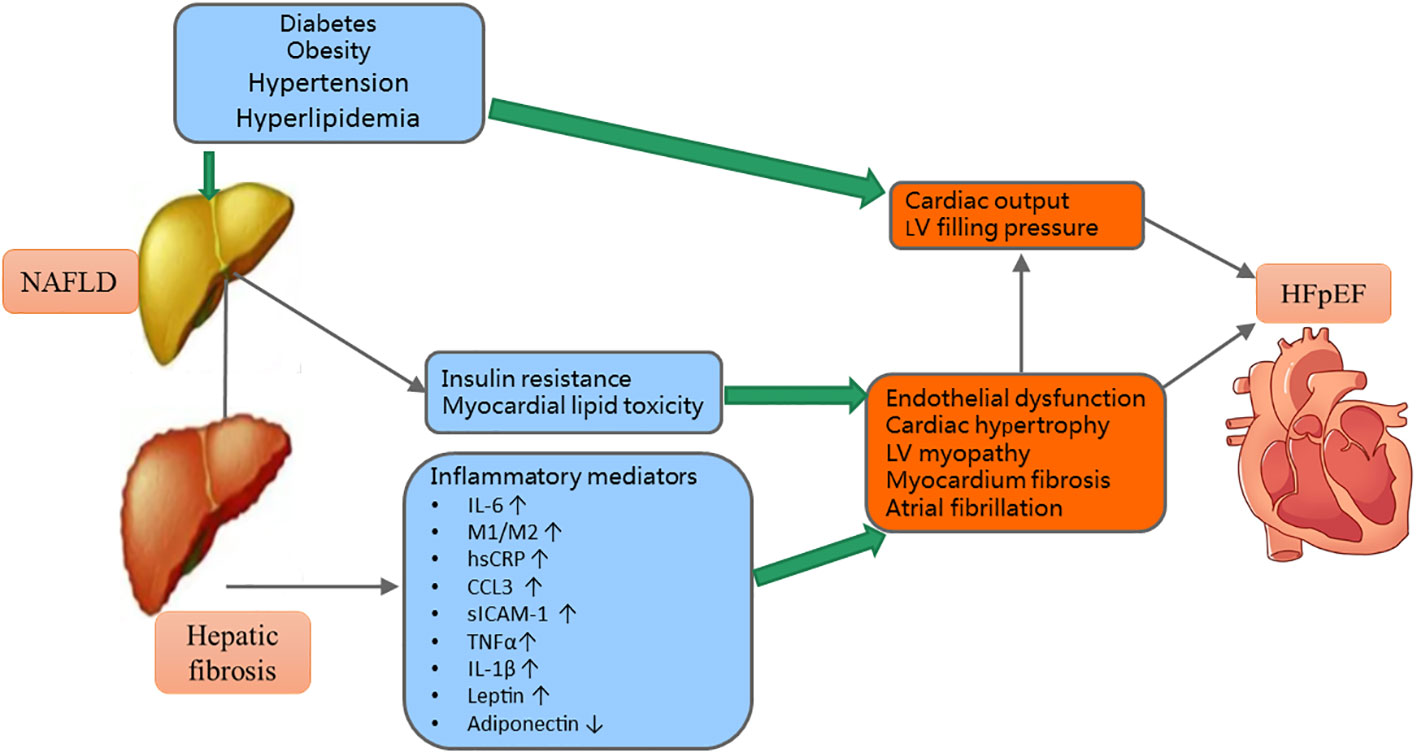
Figure 2 The mechanisms of the interactions of metabolic risk factors and hepatic fibrosis on HFpEF. NAFLD, nonalcoholic fatty liver disease; LV, left ventricular; HFpEF, heart failure with preserved ejection fraction; IL, interleukin; M1/M2, macrophage phenotype 1/2 ratio; hsCRP, high sensitivity C-reactive protein; CCL, chemokine ligand; sICAM, soluble intracellular adhesion molecule; TNF, tumor necrosis factor; ↑= increased; ↓= decrease.
The main strength of this study is the large number of T2DM patients included from an academic hospital. Further, we can get access to clinical, laboratory, and imaging data in medical records, which provided more in-depth clinical information that are not usually available in large epidemiological surveys.
There are several limitations. First, NAFLD was diagnosed by ultrasonography, which has a sub-optimal sensitivity in detecting mild-to-moderate steatosis, therefore missing patients with mild and/or moderate steatosis, rather than liver histopathology or other tools such as vibration-controlled transient elastography to quantify liver steatosis. Nevertheless, liver ultrasonography has been confirmed as an accurate and reliable tool for detecting fatty liver. Due to the relatively low cost and lack of radiation exposure, ultrasonography is widely used for identifying fatty liver in clinical settings and population studies. In the absence of liver biopsy to identify hepatic fibrosis, misclassification may have occurred. Second, although we adjusted for multiple potential confounding variables, residual and unmeasured confounding might not be fully addressed. Third, EF was measured by 2-dimensional M-mode echocardiography rather than the Simpsons method or the global longitudinal strain, which were more accurate in evaluating EF (56). Data on Tricuspid regurgitation velocity and left atrial volume index, which reflect left ventricular filling pressure, as well as data on septal, lateral, and atrial strain, which can reflect septal and atrial systolic and diastolic function (57, 58) were not available. Fourth, dynamic BMI, HbA1c, BP, and LDL-C changes during the process of diabetes management were not available, we cannot assess how these parameters change affect HFpEF risk. Finally, the cross-sectional study design makes it difficult to infer causality between the lipid parameters and NAFLD risk.
5 Conclusion
In conclusion, in patients with T2DM, advanced hepatic fibrosis was significantly associated with HFpEF risk, irrespective of BMI categories, HbA1c, BP, and LDL-C goal attainment status. Hepatic fibrosis increased the risk of HFpEF even in diabetic patients without obesity or achieving good HbA1c, BP, and LDL-C control.
Data availability statement
The raw data supporting the conclusions of this article will be made available by the authors, without undue reservation.
Ethics statement
The studies involving humans were approved by the ethics committee of Tongji Hospital. The studies were conducted in accordance with the local legislation and institutional requirements. Written informed consent for participation was not required from the participants or the participants’ legal guardians/next of kin in accordance with the national legislation and institutional requirements.
Author contributions
WJ: data collection, statistical analyses, interpreting data, and writing of the draft manuscript; ZL: study design, interpreting data, and critical revision of the manuscript. SL: data collection; TD: study design, interpreting data, and critical revision of the manuscript. All authors contributed to the article and approved the submitted version.
Funding
This study was supported by the Bethune Charitable Foundation 2020 (to TD) and Sen-Mei China Diabetes Research Fund Z-2017-26-1902 (to ZL).
Acknowledgments
The authors thank all study participants for their cooperation.
Conflict of interest
The authors declare that the research was conducted in the absence of any commercial or financial relationships that could be construed as a potential conflict of interest.
Publisher’s note
All claims expressed in this article are solely those of the authors and do not necessarily represent those of their affiliated organizations, or those of the publisher, the editors and the reviewers. Any product that may be evaluated in this article, or claim that may be made by its manufacturer, is not guaranteed or endorsed by the publisher.
Supplementary material
The Supplementary Material for this article can be found online at: https://www.frontiersin.org/articles/10.3389/fendo.2023.1183075/full#supplementary-material
References
1. Seferović PM, Petrie MC, Filippatos GS, Anker SD, Rosano G, Bauersachs J, et al. Type 2 diabetes mellitus and heart failure: a position statement from the Heart Failure Association of the European Society of Cardiology. Eur J Heart Fail (2018) 20(5):853–72. doi: 10.1002/ejhf.1170
2. Seferovic PM, Paulus WJ. Clinical diabetic cardiomyopathy: a two-faced disease with restrictive and dilated phenotypes. Eur Heart J (2015) 36(27):1718–27, 27a-27c. doi: 10.1093/eurheartj/ehv134
3. Tsao CW, Lyass A, Enserro D, Larson MG, Ho JE, Kizer JR, et al. Temporal trends in the incidence of and mortality associated with heart failure with preserved and reduced ejection fraction. JACC Heart Fail (2018) 6(8):678–85. doi: 10.1016/j.jchf.2018.03.006
4. Authors/Task Force M, McDonagh TA, Metra M, Adamo M, Gardner RS, Baumbach A, et al. ESC Guidelines for the diagnosis and treatment of acute and chronic heart failure: Developed by the Task Force for the diagnosis and treatment of acute and chronic heart failure of the European Society of Cardiology (ESC). With the special contribution of the Heart Failure Association (HFA) of the ESC. Eur J Heart Fail (2021) 24(1):4–131. doi: 10.1002/ejhf.2333
5. Majumdar A, Tsochatzis EA. Changing trends of liver transplantation and mortality from non-alcoholic fatty liver disease. Metabolism (2020) 111:154291. doi: 10.1016/j.metabol.2020.154291
6. Williamson RM, Price JF, Glancy S, Perry E, Nee LD, Hayes PC, et al. Prevalence of and risk factors for hepatic steatosis and nonalcoholic Fatty liver disease in people with type 2 diabetes: the Edinburgh Type 2 Diabetes Study. Diabetes Care (2011) 34(5):1139–44. doi: 10.2337/dc10-2229
7. Younossi ZM, Golabi P, de Avila L, Paik JM, Srishord M, Fukui N, et al. The global epidemiology of NAFLD and NASH in patients with type 2 diabetes: A systematic review and meta-analysis. J Hepatol (2019) 71(4):793–801. doi: 10.1016/j.jhep.2019.06.021
8. Jarvis H, Craig D, Barker R, Spiers G, Stow D, Anstee QM, et al. Metabolic risk factors and incident advanced liver disease in non-alcoholic fatty liver disease (NAFLD): A systematic review and meta-analysis of population-based observational studies. PloS Med (2020) 17(4):e1003100. doi: 10.1371/journal.pmed.1003100
9. Targher G, Lonardo A, Byrne CD. Nonalcoholic fatty liver disease and chronic vascular complications of diabetes mellitus. Nat Rev Endocrinol (2018) 14(2):99–114. doi: 10.1038/nrendo.2017.173
10. Lee H, Kim G, Choi YJ, Huh BW, Lee BW, Kang ES, et al. Association between non-alcoholic steatohepatitis and left ventricular diastolic dysfunction in type 2 diabetes mellitus. Diabetes Metab J (2020) 44(2):267–76. doi: 10.4093/dmj.2019.0001
11. Omote K, Verbrugge FH, Borlaug BA. Heart failure with preserved ejection fraction: mechanisms and treatment strategies. Annu Rev Med (2022) 73:321–37. doi: 10.1146/annurev-med-042220-022745
12. Hicklin HE, Gilbert ON, Ye F, Brooks JE, Upadhya B. Hypertension as a road to treatment of heart failure with preserved ejection fraction. Curr Hypertens Rep (2020) 22(10):82. doi: 10.1007/s11906-020-01093-7
13. American Diabetes Association Professional Practice C. 2Classification and diagnosis of diabetes: standards of medical care in diabetes-2022. Diabetes Care (2022) 45(Suppl 1):S17–38doi: 10.2337/dc22-S002
14. Angulo P. Nonalcoholic fatty liver disease. New Engl J Med (2002) 346(16):1221–31. doi: 10.1056/NEJMra011775
15. Heart Failure Society of A, Lindenfeld J, Albert NM, Boehmer JP, Collins SP, Ezekowitz JA, et al. HFSA 2010 comprehensive heart failure practice guideline. J Card Fail (2010) 16(6):e1–194. doi: 10.1016/j.cardfail.2010.04.004
16. WHO Expert Consultation. Appropriate body-mass index for Asian populations and its implications for policy and intervention strategies. Lancet (London England) (2004) 363(9403):157–63. doi: 10.1016/S0140-6736(03)15268-3
17. American Diabetes Association Professional Practice C, Draznin B, Aroda VR, Bakris G, Benson G, Brown FM, et al. 6. Glycemic targets: standards of medical care in diabetes-2022. Diabetes Care (2022) 45(Suppl 1):S83–96. doi: 10.2337/dc22-S006
18. American Diabetes Association Professional Practice C. 10. Cardiovascular disease and risk management: standards of medical care in diabetes-2022. Diabetes Care (2022) 45(Suppl 1):S144–S74. doi: 10.2337/dc22-S010
19. Nagueh SF, Smiseth OA, Appleton CP, Byrd BF 3rd, Dokainish H, Edvardsen T, et al. Recommendations for the evaluation of left ventricular diastolic function by echocardiography: an update from the american society of echocardiography and the european association of cardiovascular imaging. Eur Heart J Cardiovasc Imaging (2016) 17(12):1321–60. doi: 10.1093/ehjci/jew082
20. Angulo P, Hui JM, Marchesini G, Bugianesi E, George J, Farrell GC, et al. The NAFLD fibrosis score: a noninvasive system that identifies liver fibrosis in patients with NAFLD. Hepatology. (2007) 45(4):846–54. doi: 10.1002/hep.21496
21. Harrison SA, Oliver D, Arnold HL, Gogia S, Neuschwander-Tetri BA. Development and validation of a simple NAFLD clinical scoring system for identifying patients without advanced disease. Gut. (2008) 57(10):1441–7. doi: 10.1136/gut.2007.146019
22. Tavaglione F, Jamialahmadi O, De Vincentis A, Qadri S, Mowlaei ME, Mancina RM, et al. Development and validation of a score for fibrotic nonalcoholic steatohepatitis. Clin Gastroenterol Hepatol (2022) 21(6):1523–32.e1. doi: 10.1016/j.cgh.2022.03.044
23. Vilar-Gomez E, Chalasani N. Non-invasive assessment of non-alcoholic fatty liver disease: Clinical prediction rules and blood-based biomarkers. J Hepatol (2018) 68(2):305–15. doi: 10.1016/j.jhep.2017.11.013
24. Matthews DR, Hosker JP, Rudenski AS, Naylor BA, Treacher DF, Turner RC. Homeostasis model assessment: insulin resistance and beta-cell function from fasting plasma glucose and insulin concentrations in man. Diabetologia (1985) 28(7):412–9. doi: 10.1007/BF00280883
25. Argano C, Natoli G, Mularo S, Nobili A, Monaco ML, Mannucci PM, et al. Impact of diabetes mellitus and its comorbidities on elderly patients hospitalized in internal medicine wards: data from the rePoSi registry. Healthcare (Basel) (2022) 10(1):86. doi: 10.3390/healthcare10010086
26. Cosentino F, Grant PJ, Aboyans V, Bailey CJ, Ceriello A, Delgado V, et al. ESC Guidelines on diabetes, pre-diabetes, and cardiovascular diseases developed in collaboration with the EASD. Eur Heart J (2019) 41(2):255–323. doi: 10.1093/eurheartj/ehz486
27. Chung GE, Lee J-H, Lee H, Kim MK, Yim JY, Choi S-Y, et al. Nonalcoholic fatty liver disease and advanced fibrosis are associated with left ventricular diastolic dysfunction. Atherosclerosis (2018) 272:137–44. doi: 10.1016/j.atherosclerosis.2018.03.027
28. Bonapace S, Perseghin G, Molon G, Canali G, Bertolini L, Zoppini G, et al. Nonalcoholic fatty liver disease is associated with left ventricular diastolic dysfunction in patients with type 2 diabetes. Diabetes Care (2012) 35(2):389–95. doi: 10.2337/dc11-1820
29. Mantovani A, Pernigo M, Bergamini C, Bonapace S, Lipari P, Pichiri I, et al. Nonalcoholic fatty liver disease is independently associated with early left ventricular diastolic dysfunction in patients with type 2 diabetes. PloS One (2015) 10(8):e0135329. doi: 10.1371/journal.pone.0135329
30. Itier R, Guillaume M, Ricci JE, Roubille F, Delarche N, Picard F, et al. Non-alcoholic fatty liver disease and heart failure with preserved ejection fraction: from pathophysiology to practical issues. ESC Heart Fail (2021) 8(2):789–98. doi: 10.1002/ehf2.13222
31. Sheka AC, Adeyi O, Thompson J, Hameed B, Crawford PA, Ikramuddin S. Nonalcoholic steatohepatitis: A review. JAMA (2020) 323(12):1175–83. doi: 10.1001/jama.2020.2298
32. Lee YH, Kim KJ, Yoo ME, Kim G, Yoon HJ, Jo K, et al. Association of non-alcoholic steatohepatitis with subclinical myocardial dysfunction in non-cirrhotic patients. J Hepatol (2018) 68(4):764–72. doi: 10.1016/j.jhep.2017.11.023
33. Barb D, Portillo-Sanchez P, Cusi K. Pharmacological management of nonalcoholic fatty liver disease. Metabolism (2016) 65(8):1183–95. doi: 10.1016/j.metabol.2016.04.004
34. Ferguson D, Finck BN. Emerging therapeutic approaches for the treatment of NAFLD and type 2 diabetes mellitus. Nat Rev Endocrinol (2021) 17(8):484–95. doi: 10.1038/s41574-021-00507-z
35. Longo M, Scappaticcio L, Cirillo P, Maio A, Carotenuto R, Maiorino MI, et al. Glycemic control and the heart: the tale of diabetic cardiomyopathy continues. Biomolecules (2022) 12(2):272. doi: 10.3390/biom12020272
36. Iribarren C, Karter AJ, Go AS, Ferrara A, Liu JY, Sidney S, et al. Glycemic control and heart failure among adult patients with diabetes. Circulation (2001) 103(22):2668–73. doi: 10.1161/01.cir.103.22.2668
37. Yokota S, Tanaka H, Mochizuki Y, Soga F, Yamashita K, Tanaka Y, et al. Association of glycemic variability with left ventricular diastolic function in type 2 diabetes mellitus. Cardiovasc Diabetol (2019) 18(1):166. doi: 10.1186/s12933-019-0971-5
38. Cusi K. Treatment of patients with type 2 diabetes and non-alcoholic fatty liver disease: current approaches and future directions. Diabetologia (2016) 59(6):1112–20. doi: 10.1007/s00125-016-3952-1
39. Athyros VG, Doumas M, Imprialos KP, Stavropoulos K, Georgianou E, Katsimardou A, et al. Diabetes and lipid metabolism. Hormones (Athens) (2018) 17(1):61–7. doi: 10.1007/s42000-018-0014-8
40. Verges B. Pathophysiology of diabetic dyslipidaemia: where are we? Diabetologia (2015) 58(5):886–99. doi: 10.1007/s00125-015-3525-8
41. Shah AS, Sadayappan S, Urbina EM. Lipids: a potential molecular pathway towards diastolic dysfunction in youth-onset type 2 diabetes. Curr Atheroscl Rep (2022) 24(2):109–17. doi: 10.1007/s11883-022-00989-w
42. McGavock JM, Victor RG, Unger RH, Szczepaniak LS. Adiposity of the heart, revisited. Ann Intern Med (2006) 144(7):517–24. doi: 10.7326/0003-4819-144-7-200604040-00011
43. Lopaschuk GD, Ussher JR, Folmes CD, Jaswal JS, Stanley WC. Myocardial fatty acid metabolism in health and disease. Physiol Rev (2010) 90(1):207–58. doi: 10.1152/physrev.00015.2009
44. Iacobellis G, Leonetti F, Singh N, Sharma AM. Relationship of epicardial adipose tissue with atrial dimensions and diastolic function in morbidly obese subjects. Int J Cardiol (2007) 115(2):272–3. doi: 10.1016/j.ijcard.2006.04.016
45. Lee M, Kim KJ, Chung TH, Bae J, Lee YH, Lee BW, et al. Nonalcoholic fatty liver disease, diastolic dysfunction, and impaired myocardial glucose uptake in patients with type 2 diabetes. Diabetes Obes Metab (2021) 23(4):1041–51. doi: 10.1111/dom.14310
46. Beltowski J. Epicardial adipose tissue: The new target for statin therapy. Int J Cardiol (2019) 274:353–4. doi: 10.1016/j.ijcard.2018.08.098
47. Ye Q, Zou B, Yeo YH, Li J, Huang DQ, Wu Y, et al. Global prevalence, incidence, and outcomes of non-obese or lean non-alcoholic fatty liver disease: a systematic review and meta-analysis. Lancet Gastroenterol Hepatol (2020) 5(8):739–52. doi: 10.1016/s2468-1253(20)30077-7
48. Younes R, Govaere O, Petta S, Miele L, Tiniakos D, Burt A, et al. Caucasian lean subjects with non-alcoholic fatty liver disease share long-term prognosis of non-lean: time for reappraisal of BMI-driven approach? Gut (2022) 71(2):382–90. doi: 10.1136/gutjnl-2020-322564
49. Kim SS, Cho HJ, Kim HJ, Kang DR, Berry JR, Kim JH, et al. Nonalcoholic fatty liver disease as a sentinel marker for the development of diabetes mellitus in non-obese subjects. Dig Liver Dis (2018) 50(4):370–7. doi: 10.1016/j.dld.2017.12.018
50. Shida T, Oshida N, Suzuki H, Okada K, Watahiki T, Oh S, et al. Clinical and anthropometric characteristics of non-obese non-alcoholic fatty liver disease subjects in Japan. Hepatol Res (2020) 50(9):1032–46. doi: 10.1111/hepr.13543
51. McQuaid SE, Hodson L, Neville MJ, Dennis AL, Cheeseman J, Humphreys SM, et al. Downregulation of adipose tissue fatty acid trafficking in obesity: a driver for ectopic fat deposition? Diabetes (2011) 60(1):47–55. doi: 10.2337/db10-0867
52. Stahl EP, Dhindsa DS, Lee SK, Sandesara PB, Chalasani NP, Sperling LS. Nonalcoholic fatty liver disease and the heart: JACC state-of-the-art review. J Am Coll Cardiol (2019) 73(8):948–63. doi: 10.1016/j.jacc.2018.11.050
53. Pejnovic N, Jeftic I, Jovicic N, Arsenijevic N, Lukic ML. Galectin-3 and IL-33/ST2 axis roles and interplay in diet-induced steatohepatitis. World J Gastroenterol (2016) 22(44):9706–17. doi: 10.3748/wjg.v22.i44.9706
54. Wettersten N, Maisel AS. Biomarkers for heart failure: an update for practitioners of internal medicine. Am J Med (2016) 129(6):560–7. doi: 10.1016/j.amjmed.2016.01.013
55. Polyzos SA, Aronis KN, Kountouras J, Raptis DD, Vasiloglou MF, Mantzoros CS. Circulating leptin in non-alcoholic fatty liver disease: a systematic review and meta-analysis. Diabetologia (2016) 59(1):30–43. doi: 10.1007/s00125-015-3769-3
56. Nagueh SF, Smiseth OA, Appleton CP, Byrd BF 3rd, Dokainish H, Edvardsen T, et al. Recommendations for the evaluation of left ventricular diastolic function by echocardiography: an update from the american society of echocardiography and the european association of cardiovascular imaging. J Am Soc Echocardiogr (2016) 29(4):277–314. doi: 10.1016/j.echo.2016.01.011
57. Menet A, Bernard A, Tribouilloy C, Leclercq C, Gevaert C, Guyomar Y, et al. Clinical significance of septal deformation patterns in heart failure patients receiving cardiac resynchronization therapy. Eur Heart J Cardiovasc Imaging (2017) 18(12):1388–97. doi: 10.1093/ehjci/jew289
58. Jin X, Nauta JF, Hung CL, Ouwerkerk W, Teng TK, Voors AA, et al. Left atrial structure and function in heart failure with reduced (HFrEF) versus preserved ejection fraction (HFpEF): systematic review and meta-analysis. Heart Fail Rev (2022) 27(5):1933–55. doi: 10.1007/s10741-021-10204-8
Glossary
Keywords: non-alcoholic fatty liver disease, advanced liver fibrosis, heart failure with preserved ejection fraction, metabolic goal achievement, type 2 diabetes mellitus
Citation: Jiang W, Liu Z, Liu S and Du T (2023) Associations of advanced liver fibrosis with heart failure with preserved ejection fraction in type 2 diabetic patients according to obesity and metabolic goal achievement status. Front. Endocrinol. 14:1183075. doi: 10.3389/fendo.2023.1183075
Received: 09 March 2023; Accepted: 06 October 2023;
Published: 24 October 2023.
Edited by:
Christiano Argano, ARNAS Ospedali Civico Di Cristina Benfratelli, ItalyReviewed by:
Alfredo Caturano, University of Campania Luigi Vanvitelli, ItalyEmir Yonas, YARSI University, Indonesia
Copyright © 2023 Jiang, Liu, Liu and Du. This is an open-access article distributed under the terms of the Creative Commons Attribution License (CC BY). The use, distribution or reproduction in other forums is permitted, provided the original author(s) and the copyright owner(s) are credited and that the original publication in this journal is cited, in accordance with accepted academic practice. No use, distribution or reproduction is permitted which does not comply with these terms.
*Correspondence: Tingting Du, YWR1dHRzeHhAMTYzLmNvbQ==
†These authors have contributed equally to this work and share first authorship