- 1Department of Nephrology, Charité - Universitätsmedizin Berlin, Berlin, Germany
- 2Fifth Department of Medicine (Nephrology/Endocrinology/Rheumatology, Pneumonology), University Medical Center Mannheim, University of Heidelberg, Mannheim, Germany
- 3Institute of Pharmacy, Freie Universität Berlin, Berlin, Germany
- 4Klinik für Innere Medizin, Bundeswehrkrankenhaus Berlin, Berlin, Germany
- 5Diaverum Renal Care Center, Diaverum MVZ Am Neuen Garten Standort Ludwigsfelde, Potsdam, Germany
- 6European Center for Angioscience ECAS, Medical Faculty Mannheim of the University of Heidelberg, Mannheim, Germany
- 7Reproductive, Genetic Hospital of CITIC-Xiangya, Changsha, China
- 8Institute of Medical Diagnostics, IMD, Berlin, Germany
Background: 25-hydroxyvitamin D (25(OH)D) and potentially also 1,25-dihydroxyvitamin D (1,25(OH)2D) inhibits the synthesis of parathyroid hormone (PTH) in the chief cells of the parathyroid gland. Clinical studies showing a negative correlation between (25(OH)D and PTH are in good agreement with these findings in basic science studies. However, PTH was measured in these studies with the currently clinically used 2nd or 3rd generation intact PTH (iPTH) assay systems. iPTH assays cannot distinguish between oxidized forms of PTH and non-oxidized PTH. Oxidized forms of PTH are the by far most abundant form of PTH in the circulation of patients with impaired kidney function. Oxidation of PTH causes a loss of function of PTH. Given that the clinical studies done so far were performed with an PTH assay systems that mainly detect oxidized forms of PTH, the real relationship between bioactive non-oxidized PTH and 25(OH)D as well as 1,25(OH)2D is still unknown.
Methods: To address this topic, we compared for the first time the relationship between 25(OH)D as well as 1,25(OH)2D and iPTH, oxPTH as well as fully bioactive n-oxPTH in 531 stable kidney transplant recipients in the central clinical laboratories of the Charité. Samples were assessed either directly (iPTH) or after oxPTH (n-oxPTH) was removed using a column that used anti-human oxPTH monoclonal antibodies, a monoclonal rat/mouse parathyroid hormone antibody (MAB) was immobilized onto a column with 500 liters of plasma samples. Spearman correlation analysis and Multivariate linear regression were used to evaluate the correlations between the variables.
Results: There was an inverse correlation between 25(OH)D and all forms of PTH, including oxPTH (iPTH: r=-0.197, p<0.0001; oxPTH: r=-0.203, p<0.0001; n-oxPTH: r=-0.146, p=0.001). No significant correlation was observed between 1,25(OH)2D and all forms of PTH. Multiple linear regression analysis considering age, PTH (iPTH, oxPTH and n-oxPTH), serum calcium, serum phosphor, serum creatinine, fibroblast growth factor 23 (FGF23), osteoprotegerin (OPG), albumin, and sclerostin as confounding factors confirmed these findings. Subgroup analysis showed that our results are not affected by sex and age.
Conclusion: In our study, all forms of PTH are inversely correlated with 25-hydroxyvitamin D (25(OH)D). This finding would be in line with an inhibition of the synthesis of all forms of PTH (bioactive n-oxPTH and oxidized forms of PTH with minor or no bioactivity) in the chief cells of the parathyroid glad.
1 Introduction
The parathyroid polypeptide hormone (PTH) is synthesized and cleaved into its active form in the parathyroid glands. PTH is vital for calcium homeostasis, and it has various end-organ targets by which it can regulate the calcium levels, such as the bones, kidneys, and intestines. Calcium levels in the blood act as a negative feedback loop, signaling the parathyroid glands to stop releasing PTH (1). In vitro and in vivo, FGF23 inhibits PTH mRNA production and secretion in an alpha klotho (KL)-dependent way (2). The PTH hormone increases calcium levels when serum calcium concentration is low (1, 3, 4). Then, intracellular calcium concentrations increase, inhibiting exocytosis of PTH via signal transduction across the CaSR (5).
PTH’s active form contains 84 amino acids as a single-chain polypeptide hormone (5). There are two methionine residues in PTH, located at positions 8 and 18. Oxidation of these residues inhibits or eliminates PTH’s biological function (6–8). After oxidation they are separated into four forms (1) n-oxPTH; (2) PTH oxidized at methionine residue 8 (ox (Met8)PTH); (3) ox (Met18)PTH; (4) PTH oxidized at both residues 8 and 18 (ox (Met8,18)PTH). The bioactivity degree from high to low was: n-oxPTH, ox (Met18)PTH, ox (Met8)PTH, and ox (Met8,18)PTH (6). In CKD stage 2-4 children, 90% of iPTH is oxidized (oxPTH); in adult CKD patients on dialysis, 89.5% of iPTH is oxidized (oxPTH), and in kidney transplant patients, 89% of iPTH is oxidized (oxPTH) (9).
Vitamin D is present in a variety of forms in the blood. The two forms in the liver and kidneys are 25-hydroxyvitamin D (25 (OH)D) and 1,25-dihydroxyvitamin D (1,25(OH)2D). Vitamin D status can be assessed by measuring the body’s distribution of 25(OH) D in the blood (10–13). Circulating blood levels of 1,25 (OH)2 D are much lower than those of 1,25 (OH)2 D (14). Vitamin D is mainly synthesized in the kidney (as well as in pregnant women’s placentas) and is circulated in the bloodstream. As a consequence, 1,25 (OH)2 vitamin D concentrations are dependent on both the supply of 25(OH) vitamin D and the kidney fraction (15–17). 25 (OH)D has the most prolonged half-life (2 to 3 weeks), while 1,25(OH)2D has a short half-life (1-3 days) (18). The serum concentrations of 1,25(OH)2D are around 0.1% of that of 25(OH)D concentrations. PTH, hypocalcemia, and hypophosphatemia result in the induction of CYP27B1 in the kidney, which produces 1-alpha-hydroxylation, resulting in higher concentrations of 1,25(OH)2D (5, 19). Through secondary hyperparathyroidism, an inadequate supply of substrate 25(OH)D will stimulate the renal CYP27B1-hydroxylase to maintain or increase the production of 1,25(OH)2D metabolites, whereas a deficiency of substrate for the extrarenal CYP27B1-hydroxylase results in a decrease in 1,25(OH)2D products (20). The vitamin D receptor (VDR) on the parathyroid gland stimulates 1,25(OH)2D to inhibit PTH directly (21).
Nowadays, most published articles focus on the PTH and 25(OH)D in different diseases or populations (22–24). The relationship between PTH and vitamin D in kidney transplant patients is poorly studied, specifically analyzing 25(OH)D and 1,25(OH)2D simultaneously. In this study, we analyzed the relationship between different statuses of vitamin D and the relationship of PTH forms (iPTH, oxPTH, and n-oxPTH) with 25(OH)D, 1,25(OH)2D, serum calcium, serum phosphor, creatinine, FGF23, Osteoprotegerin (OPG), Alkaline phosphatase (ALP), and sclerostin. Moreover, we studied the relationship between iPTH, oxPTH, and n-oxPTH with 25(OH)D, 1,25(OH)2D in two subgroups (female/male and age above 50 years/below 50 years).
2 Materials and methods
2.1 Study population
A total of 531 of the 600 kidney transplant patients undergoing routine check-up in the transplant outpatient clinic Charité-Mitte, Berlin, Germany, had 25VD levels and a functional graft were included in this study cohort (Figure 1). The exclusion criteria are patients with an active infection, malignancy, acute rejection, recent cardiovascular events, or those unwilling to participate. Local ethics committees approved this study, and we obtained informed consent from all study participants.
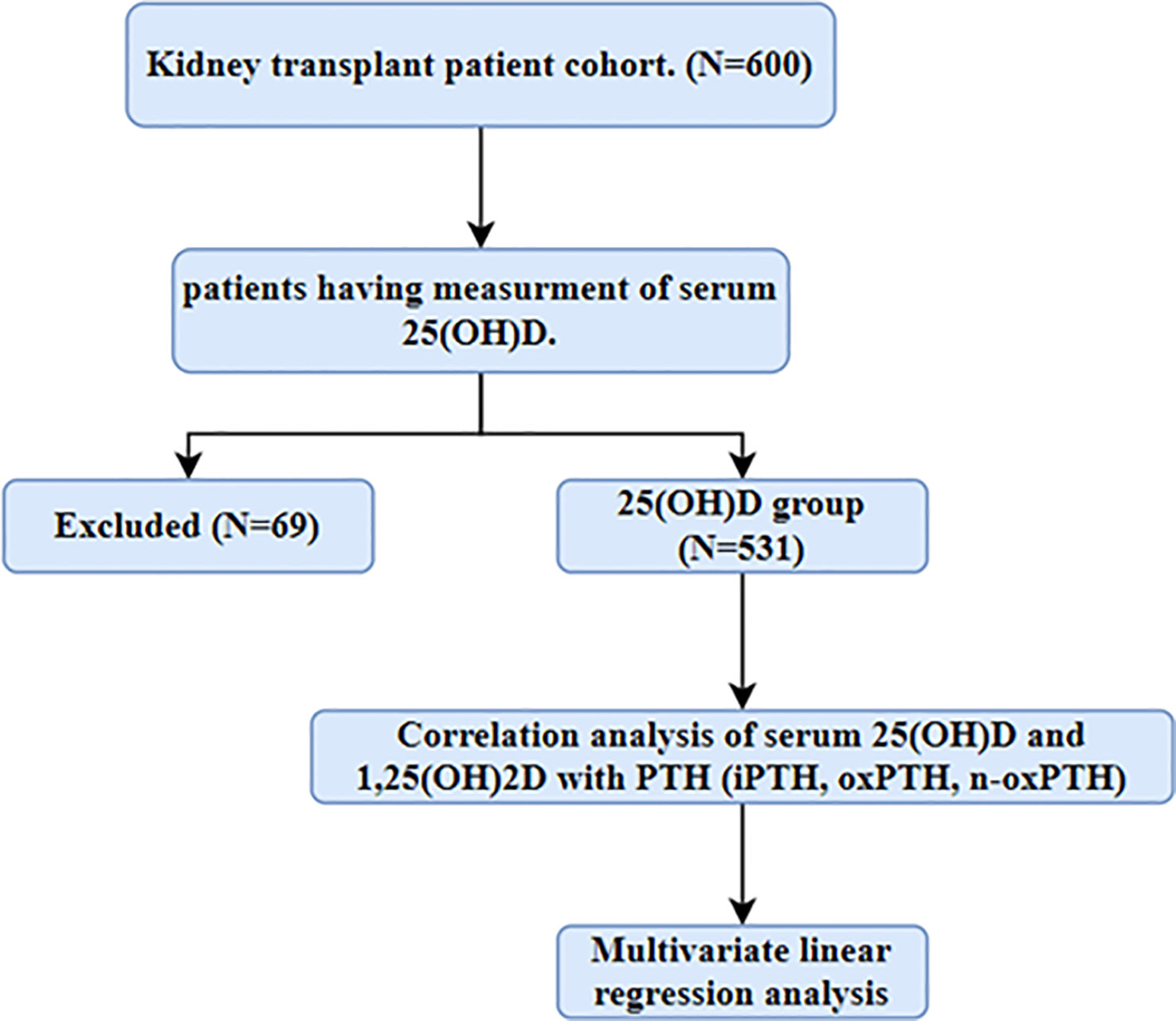
Figure 1 Flow-chart of study population. Patients who have measurement of serum 25(OH)D was included in this study.
Samples of patients’ blood and urine were collected routinely from April 2012 until December 2012. Those samples were kept frozen at −80°C. Baseline data, i.e., post-transplant duration, cold ischemia time, age, and gender of the recipient, were collected from patients’ records. In addition, clinical parameters such as 25-hydroxyvitamin D, 1,25-dihydroxy vitamin D, iPTH, oxPTH, n-oxPTH, total cholesterol, calcium, and phosphorus were all analyzed in the central clinical laboratories of the Charité.
2.2 Assays
Second and third-generation PTH assays (also known as intact PTH assays), which are now utilized in clinical practice, cannot distinguish between oxPTH and n-oxPTH. Both oxPTH and n-oxPTH are measured (iPTH = n-oxPTH + oxPTH). Samples were assessed either directly (iPTH) or after oxPTH (n-oxPTH) was removed using a column that used anti-human oxPTH monoclonal antibodies, a monoclonal rat/mouse parathyroid hormone antibody (MAB) was immobilized onto a column with 500 liters of plasma samples. At room temperature, the columns were mixed end-over-end for 2 h and then washed with 250 l of ammonium acetate buffer pH 7.0 and, after washing with 250 l of ammonium acetate buffer pH 7.0 containing 20% acetonitrile, 2 times with elution buffer (0.05 M formic acid, pH 3.5). Separate fractions of the flow-through, wash fraction, and eluate were lyophilized. Reconstituted samples were then analyzed by Roche Intact PTH assay with 500 l of PBS buffer, pH 7.4 (25). The commercially available ELISA used to measure cFGF23 was the FGF23 (C-terminal) multi-matrix ELISA with catalog number BI-20702, produced by Biomedica in Austria, according to the manufacturer’s instructions (https://www.bmgrp.com/wp-content/uploads/2019/03/bi-20702-fgf23-elisa-validation-data-150306.pdf). Sclerostin and OPG were measured using commercially available kits (Catalogue BI-20492, and BI-20403, Biomedica Medizinprodukte GmbH, Vienna, Austria) according to the instructions of the manufacturer as recently described (26–28). Apart from PTH isoforms, FGF23, OPG and sclerostin, all clinical laboratory parameters were examined in the university hospital Charité’s central clinical laboratories and subjected to routine quality checks according to German law.
2.3 Statistical analysis
SPSS version 23.0 (IBM corporation, New York, USA) was used for statistical evaluation. The mean was used to represent all continuous variable parameters. To assess the correlation between variables, we used Spearman correlation analysis. The Multivariate linear regression was performed using iPTH, oxPTH, and n-oxPTH as the dependent variables. Parameters recognized in the literature as affecting PTH concentration (age, serum calcium, serum phosphor, creatinine, FGF23, OPG, ALP, and sclerostin) were introduced into a multivariate linear regression model. Statistically significant differences were considered as p<0.05. All figures were made by GraphPad Prism 8 (GraphPad Software Corporation, California, USA) and presented as mean ± SEM.
3 Results
3.1 Characteristics of the study population
Table 1 shows the characteristics of the study population. In this study cohort, the mean age was 54.74 years, and there were 202 females and 329 of males. The frequency distributions of 25(OH)D, 1,25(OH)2D, iPTH, oxPTH, and n-oxPTH showed an approximately normal distribution (Supplementary Figures 1A, B).
3.2 Correlation of serum 25(OH)D with 1,25(OH)2D; correlation of iPTH with oxPTH, and n-oxPTH
In transplant patients, 25(OH)D was correlated with 1,25(OH)2D (r=0.120, p=0.009) (Supplementary Figure 2). OxPTH had a strong relationship with iPTH (r=0.998, p<0.0001), in comparison, n-oxPTH was not that correlated with iPTH (r=0.866, p<0.0001) (Figure 2).
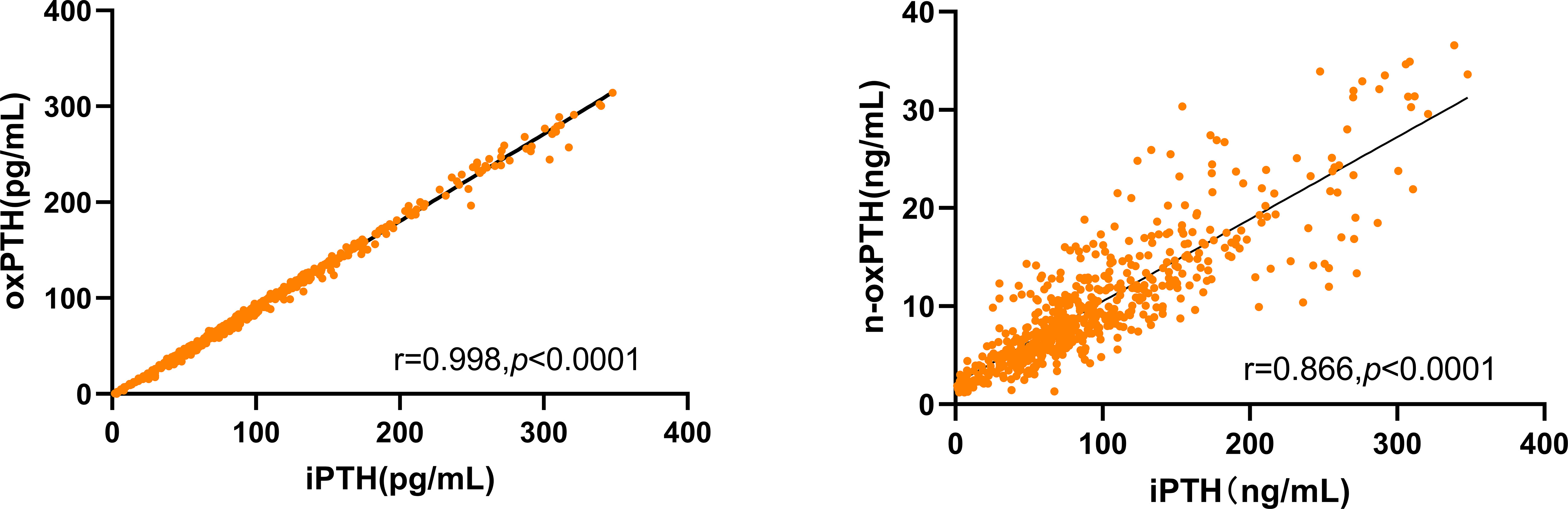
Figure 2 Correlation of oxPTH and n-oxPTH with iPTH in 531 kidney transplant patients. oxPTH and n-oxPTH were both significant related with iPTH in our study.
3.3 Correlation of serum 25(OH)D with clinical chemistry parameters
25(OH)D correlated with iPTH (r=-0.197, p<0.0001); oxPTH (r=-0.203, p<0.0001), n-oxPTH (r=-0.146, p=0.001) (Table 2, Figure 3A). According to the concentration of 25(OH)D, the study cohort was divided into 11 groups: (0-10ng/ml; 10-20ng/ml; 20-30ng/ml; 30-40ng/ml; 40-50ng/ml; 50-60ng/ml; 60-70ng/ml; 70-80ng/ml; 80-90ng/ml; 90-100ng/ml; ≥100ng/ml), and the mean ± SEM of PTH (iPTH, oxPTH and n-oxPTH) in each group was taken for graphing. There were negative correlations across the sexes (iPTH, female: r=-0.265, p<0.001; male: r=-0.147, p=0.009); (oxPTH, female: r=-0.258, p<0.001; male: r=-0.160, p=0.004) (n-oxPTH, female: r=-0.272, p<0.001; male: r=-0.056, p=0.316) (Supplementary Figure 3A); and different stage of age (iPTH, age <50 years: r=-0.162, p=0.026; age ≥50 years: r=-0.234, p<0.0001); (oxPTH, age <50 years: r=-0.172, p=0.018; age ≥50 years: r=-0.235, p<0.0001); (n-oxPTH, age <50 years: r=-0.086, p=0.241; age ≥50 years: r=-0.187, p=0.001) (Supplementary Figure 4A). Furthermore, the multivariate linear regression for 25(OH)D considering age, PTH (iPTH, oxPTH and n-oxPTH), serum calcium, serum phosphor, serum creatinine, FGF23, OPG, ALP and sclerostin showed that 25(OH)D was significantly correlated with iPTH (unstandardized coefficients B: -0.094, p=53×10-6) (Table 3A); oxPTH (unstandardized coefficients B: -0.108, p=2×10-5) (Table 3B); and n-oxPTH (unstandardized coefficients B: -0.561, p=0.015) (Table 3C)
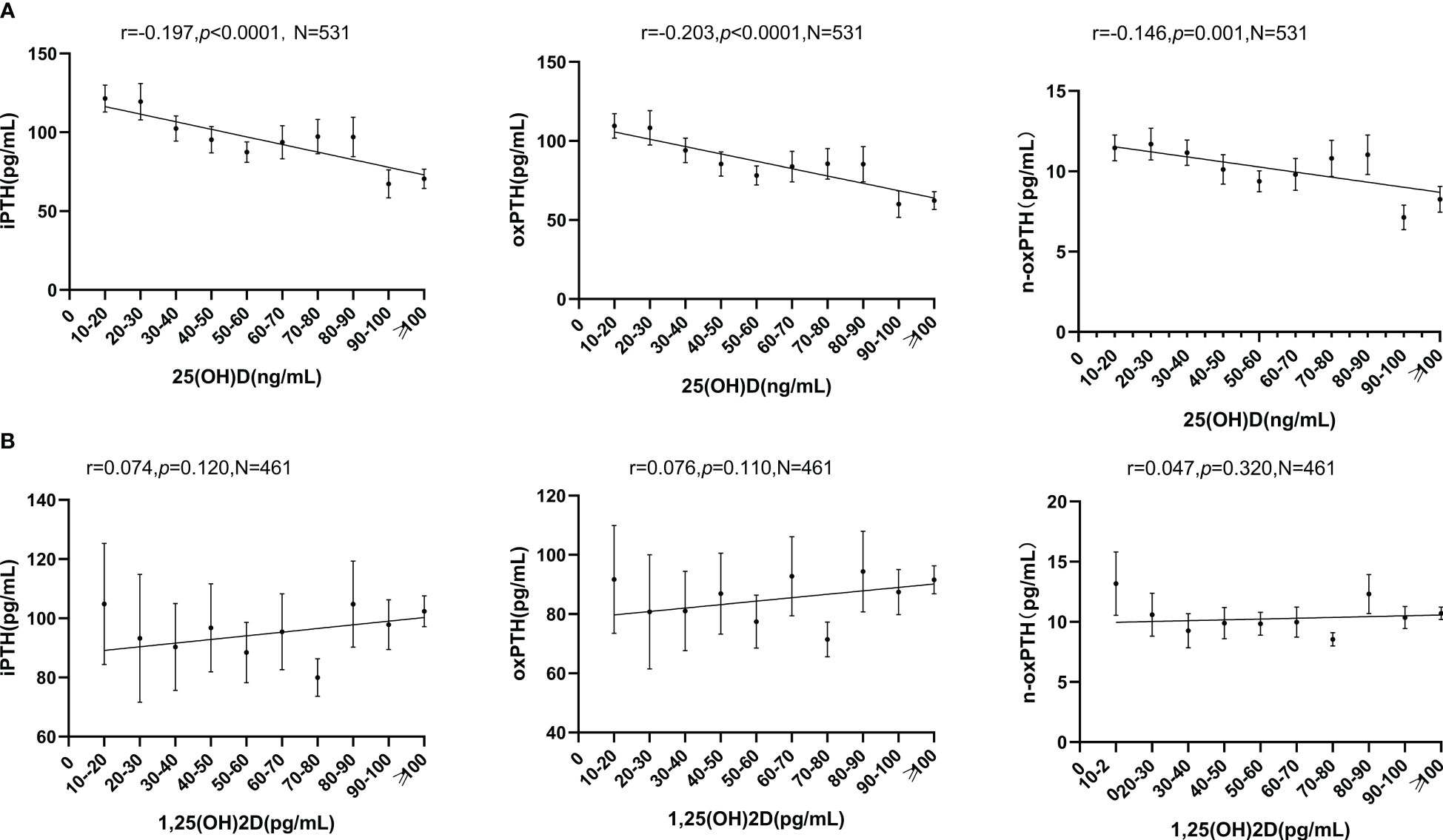
Figure 3 (A) Relationship between 25(OH)D and PTH (iPTH, oxPTH, n-oxPTH). All forms of PTH were significant related with 25(OH)D. Spearman correlation analysis was used. Graphs were presented as mean ± SEM. (B) Relationship between 1,25(OH)2D and PTH (iPTH, oxPTH, n-oxPTH). All forms of PTH were not related with 1,25(OH)2D. Spearman correlation analysis was used. Graphs were presented as mean ± SEM.
3.4 Correlation of serum 1,25(OH)2D with clinical chemistry parameters
There are some differences between 1,25(OH)2D and PTH (iPTH, oxPTH and n-oxPTH): no correlation with iPTH (r=0.074, p=0.120); oxPTH (r=0.076, p=0.110); n-oxPTH (r=0.047, p=0.320) (Table 2, Figure 3B), but 1,25(OH)2D correlated with PTH in females (iPTH: r=0.198, p=0.01; oxPTH: r=0.198, p=0.01; n-oxPTH: r=0.160, p=0.036) (Supplementary Figure 3B); as well as in age <50 years subgroup (iPTH: r=0.179, p=0.022; oxPTH: r=0.178, p=0.023; n-oxPTH: r=0.162, p=0.038) (Supplementary Figure 4B). 1,25(OH)2D was not correlated with iPTH, oxPTH and n-oxPTH in male (Supplementary Figure 3B) and age ≥50 years (Supplementary Figure 4B). When serum 25(OH)D≥30ng/mL, 1,25(OH)2D was significantly correlated with iPTH (r=0.119, p=0.030) and oxPTH (r=0.120, p=0.027) (Supplementary Figure 5). Multiple linear regression analysis performed in different concentrations of 25(OH)D (<30ng/ml and≥30ng/ml) confirmed these findings (Tables 4A–C).
4 Discussion
In the current study, we analyzed the potential correlation between two forms of vitamin D (25(OH)D and 1,25(OH)2D) on one hand and three forms of PTH (iPTH, oxPTH and n-oxPTH) on the other hand in 531 transplant patients. The present study revealed a negative comparable correlation between 25(OH)D and all forms of PTH, including oxPTH, the potentially not bioactive or less active form of PTH (Figure 3A). No significant correlation was observed between 1,25(OH)2D and all the forms of PTH (Figure 3B). Subgroup analysis showed that the above described key findings are not affected by sex and age. OxPTH showed an almost perfect positive correlation with iPTH (r=0.998, p<0.0001) (Figure 2), however, this positive correlation was less perfect between oxPTH and n-oxPTH (r=0.866, p<0.0001) (Figure 2).
iPTH is simply the sum of oxPTH and n-oxPTH (29). The perfect correlation between iPTH and oxPTH and the less perfect correlation between iPTH and n-oxPTH might indicate that iPTH is mainly reflecting oxPTH (means the sum of all oxidized forms of PTH) which might be an indicator for oxidative stress rather than biologically active PTH. In agreement with this notion, oxPTH has a substantially lower metabolic clearance rate than n-oxPTH (30, 31). Along with this line, almost 89.48% iPTH was oxidized in the current study. Comparable results regarding the correlations between iPTH, oxPTH and n-oxPTH were also reported in EVOLVE trail—a 2867 participants study (32), and other three observational studies (33–35). Two important remarks would be also relevant in this regard. First, it was reported that PTH oxidation takes place in vivo, thus we can exclude the possibility of ex vivo oxidation of PTH due to preanalytical conditions (36). Second, the cohort analyzed in the present study are kidney transplant patients. Their kidney function recovered only partially, they suffer from oxidative stress leading to increased percentage of oxPTH.
The effect of vitamin D supplementation on n-oxPTH concentrations in hypertensive vitamin D-deficient individuals with maintained renal function was studied in a clinical trial– the Styrian Vitamin D Hypertension Trial evaluated 108 vitamin D-deficient hypertensive patients, treated either with vitamin D (2880 IE daily) or placebo for 8 weeks (37). It was shown that both total PTH (tPTH) and n-oxPTH concentrations dropped after supplementation. tPTH decreased more than n-oxPTH. This indicates that vitamin D supplementation increases the non-oxidized fraction of PTH while decreasing the oxidized proportion (37). A challenge here is the lack for approved standard normal values for n-oxPTH by international guideline boards (36). Vitamin D has been shown to have antioxidant properties both in vitro and in vivo, in rats as well as in humans (38–41). PTH oxidation may thus be influenced by vitamin D supplementation, as current evidence suggests (37). In any case, the finding that both total PTH (tPTH) and n-oxPTH concentrations dropped after supplementation, see above, and the finding in the current study that oxPTH, iPTH and n-oxPTH correlates inversely with 25(OH)D in our study do support the hypothesis that 25(OH)D inhibits the synthesis of all forms of PTH probably already at the level of the parathyroid gland – in other words: 25(OH)D has an negative effect on the synthesis of n-oxPTH and oxPTH in the chief cells of the parathyroid gland.
Low vitamin D status, as well as low dietary intakes of vitamin D and calcium, are considered independent factors increasing PTH levels (42, 43). This present study reveals a strong negative correlation between 25(OH)D and iPTH, which is in line with other studies (43–65). However, our study is the first to show that both bioinactive oxPTH and bioactive n-oxPTH are inversely correlated with 25(OH)D in kidney transplant patients. In this context, it is important to note that oxPTH represents a mixture of oxidized forms of PTH (Met8-oxPTH, Met18-oxPTH and Met8, Met18-dioxPTH). These forms do have different biological properties. Met18 in the PTH molecule is more susceptible to oxidation than Met8 in the PTH molecule (66). Met8 is located in a hydrophobic pocket, which hampers oxidation of this residue as compared to Met(18) (67). Compared to n-oxPTH, PTH molecule with oxidized Met(8) has a less reverse turn structure and more nonordered structure (8). The resulting structural change is still more like n-oxPTH if only Met 18 is oxidized, and thus agrees with the degree of residual biological activity. As of now, the available methods for measuring n-oxPTH cannot differentiate and quantitate the different forms of oxPTH.
Inconsistent results regarding the correlations between 1,25(OH)2D and PTH were reported showing no significant correlation (68), significant positive correlation (58), or significant negative correlation (69, 70). Our current study, when analyzing the whole population, showed clearly that the serum 1,25(OH)2D was associated with iPTH and oxPTH only under the conditions of 25(OH)D≥30ng/ml, and the multivariate linear regression has also confirmed this result. Recently, a large cross-sectional study showed that 25(OH)D and iPTH were inversely correlated across sex and different stages of age but serum 1,25(OH)2D was only positively associated with iPTH in the 25(OH)D<40ng/ml subgroup and in women (71). And in a study of 909 men without known chronic kidney disease and when not taking antidiabetic medications, it was found that a high 1,25(OH)2D/25(OH)D ratio was significantly correlated with higher levels of PTH than the level of 1,25(OH)2D alone (58). There are studies showing that circulating 25(OH)D concentrations in healthy adults have no effect on circulating 1,25(OH)2D (43, 72). Thus circulating 25(OH)D may influence PTH secretion and parathyroid growth via 1,25(OH)2D generated within parathyroid chief cells.
Although our study is the first cross-sectional study to simultaneously study the association of 25(OH)D and 1,25(OH)2D with different forms of PTH in renal transplant patients, there are still some limitations. Our study did not compare vitamin D and PTH before and after transplantation due to the lack of such data. We were also uncertain whether the subjects were supplemented with vitamin D or not. The clinical assay used to measure oxPTH and n-oxPTH is only able to differentiate non-oxidized PTH from all forms oxidized PTH (Met8-oxPTH, Met18-oxPTH and Met8, Met18-di-oxPTH). Since Met18-oxPTH is partially bioactive (73), it would have been of interest to measure the oxidized forms of PTH separately. However, this is currently not possible with the methods available for clinical research so far.
5 Conclusions
In conclusion, iPTH, oxPTH as well as n-oxPTH are inversely and independent of confounding factors correlated with 25(OH)D but not with1,25(OH)2D. Previous basic science studies have shown that one oxPTH form (Met18-oxPTH) is also bioactive. It is well established that PTH synthesis is blocked in the parathyroid gland by vitamin D. Hence, our finding would be in line with an inhibition of the synthesis of all forms of PTH (bioactive n-oxPTH and oxidized forms of PTH with minor or no bioactivity) in the chief cells of the parathyroid glad.
Data availability statement
The original contributions presented in the study are included in the article/Supplementary Material. Further inquiries can be directed to the corresponding author.
Ethics statement
The studies involving human participants were reviewed and approved by Charité-Mitte, Berlin, Germany. The patients/participants provided their written informed consent to participate in this study. The study was conducted according to the guidelines of the Declaration of Helsinki, approved by the Ethical Committee of the University of Potsdam (reference number:3a-2013, approval date: February 2, 2014).
Author contributions
BH conceived the research idea and participated in the writing and revision of the manuscript. JZ contributed to the literature search and data extraction. JZ and AH analyzed the data and wrote the paper. C-FH revised the manuscript. BH, PK, BK, and BKK amended the manuscript. All authors made contributions to the conception and/or implementation of the study, were involved in reviewing and revising the manuscript. All authors contributed to the article and approved the submitted version.
Acknowledgments
China Scholarship Council supports JZ.
Conflict of interest
The authors declare that the research was conducted in the absence of any commercial or financial relationships that could be construed as a potential conflict of interest.
Publisher’s note
All claims expressed in this article are solely those of the authors and do not necessarily represent those of their affiliated organizations, or those of the publisher, the editors and the reviewers. Any product that may be evaluated in this article, or claim that may be made by its manufacturer, is not guaranteed or endorsed by the publisher.
Supplementary material
The Supplementary Material for this article can be found online at: https://www.frontiersin.org/articles/10.3389/fendo.2023.1178166/full#supplementary-material
Supplementary Figure 1 | (A) Distribution of 25(OH)D, 1,25(OH)2D. (B) Distribution of iPTH, oxPTH, n-oxPTH.
Supplementary Figure 2 | Correlation of 25(OH)D and 1,25(OH)2D in 531 kidney transplant patients.
Supplementary Figure 3 | (A) Relationship between serum 25(OH)D and PTH in females and males. (B) Relationship between serum 1,25(OH)2D and PTH in females and males.
Supplementary Figure 4 | (A) Relationship between serum 25(OH)D and serum PTH in age < 50 years group and age ≥50 years group. (B) Relationship between serum 1,25(OH)2D and serum PTH in age < 50 years group and age ≥50 years group.
Supplementary Figure 5 | Relationship between serum 1,25(OH)2D and serum PTH (iPTH, oxPTH and n-oxPTH) in 25(OH)D< 30 ng/mL and 25(OH)D ≥30 ng/mL group.
References
1. Silver J, Russell J, Sherwood LM. Regulation by vitamin d metabolites of messenger ribonucleic acid for preproparathyroid hormone in isolated bovine parathyroid cells. Proc Natl Acad Sci U.S.A. (1985) 82(12):4270–3. doi: 10.1073/pnas.82.12.4270
2. Ben-Dov IZ, Galitzer H, Lavi-Moshayoff V, Goetz R, Kuro-o M, Mohammadi M, et al. The parathyroid is a target organ for FGF23 in rats. J Clin Invest (2007) 117(12):4003–8. doi: 10.1172/JCI32409
3. Galesanu C, Mocanu V. VITAMIN d DEFICIENCY AND THE CLINICAL CONSEQUENCES. Rev Med Chir Soc Med Nat Iasi (2015) 119(2):310–8.
4. Bouillon R, Carmeliet G. Vitamin d insufficiency: definition, diagnosis and management. Best Pract Res Clin Endocrinol Metab (2018) 32(5):669–84. doi: 10.1016/j.beem.2018.09.014
5. Evenepoel P, Bover J, Ureña Torres P. Parathyroid hormone metabolism and signaling in health and chronic kidney disease. Kidney Int (2016) 90(6):1184–90. doi: 10.1016/j.kint.2016.06.041
6. Frelinger AL, Zull JE. Oxidized forms of parathyroid hormone with biological activity. separation and characterization of hormone forms oxidized at methionine 8 and methionine 18. J Biol Chem (1984) 259(9):5507–13.
7. Horiuchi N. Effects of oxidation of human parathyroid hormone on its biological activity in continuously infused, thyroparathyroidectomized rats. J Bone Miner Res (1988) 3(3):353–8. doi: 10.1002/jbmr.5650030316
8. Zull JE, Smith SK, Wiltshire R. Effect of methionine oxidation and deletion of amino-terminal residues on the conformation of parathyroid hormone. circular dichroism studies. J Biol Chem (1990) 265(10):5671–6.
9. Hocher B, Oberthür D, Slowinski T, Querfeld U, Schaefer F, Doyon A, et al. Modeling of oxidized PTH (oxPTH) and non-oxidized PTH (n-oxPTH) receptor binding and relationship of oxidized to non-oxidized PTH in children with chronic renal failure, adult patients on hemodialysis and kidney transplant recipients. Kidney Blood Press Res (2013) 37(4-5):240–51. doi: 10.1159/000350149
10. Holick MF, Binkley NC, Bischoff-Ferrari HA, Gordon CM, Hanley DA, Heaney RP, et al. Evaluation, treatment, and prevention of vitamin d deficiency: an endocrine society clinical practice guideline. J Clin Endocrinol Metab (2011) 96(7):1911–30. doi: 10.1210/jc.2011-0385
11. Klingberg E, Oleröd G, Konar J, Petzold M, Hammarsten O. Seasonal variations in serum 25-hydroxy vitamin d levels in a Swedish cohort. Endocrine (2015) 49(3):800–8. doi: 10.1007/s12020-015-0548-3
12. Wielders JP, Wijnberg FA. Preanalytical stability of 25(OH)-vitamin D3 in human blood or serum at room temperature: solid as a rock. Clin Chem (2009) 55(8):1584–5. doi: 10.1373/clinchem.2008.117366
13. Cashman KD, van den Heuvel EG, Schoemaker RJ, Prévéraud DP, Macdonald HM, Arcot J. 25-hydroxyvitamin d as a biomarker of vitamin d status and its modeling to inform strategies for prevention of vitamin d deficiency within the population. Adv Nutr (2017) 8(6):947–57. doi: 10.3945/an.117.015578
14. Jones G, Prosser DE, Kaufmann M. Cytochrome P450-mediated metabolism of vitamin d. J Lipid Res (2014) 55(1):13–31. doi: 10.1194/jlr.R031534
15. Fraser DR, Kodicek E. Unique biosynthesis by kidney of a biological active vitamin d metabolite. Nature (1970) 228(5273):764–6. doi: 10.1038/228764a0
16. Sutton AL, MacDonald PN. Vitamin d: more than a "bone-a-fide" hormone. Mol Endocrinol (2003) 17(5):777–91. doi: 10.1210/me.2002-0363
17. Wagner CL, Hollis BW, Kotsa K, Fakhoury H, Karras SN. Vitamin d administration during pregnancy as prevention for pregnancy, neonatal and postnatal complications. Rev Endocr Metab Disord (2017) 18(3):307–22. doi: 10.1007/s11154-017-9414-3
18. Zerwekh JE. Blood biomarkers of vitamin d status. Am J Clin Nutr (2008) 87(4):1087s–91s. doi: 10.1093/ajcn/87.4.1087S
19. . R. Vitamin D: Photosynthesis, metabolism, and action to clinical applications. Endocrinology. (2001).
20. Adams JS, Hewison M. Update in vitamin d. J Clin Endocrinol Metab (2010) 95(2):471–8. doi: 10.1210/jc.2009-1773
21. Ursem SR, Vervloet MG, de Jongh RT, Heijboer AC. Oxidation of parathyroid hormone. Clin Chim Acta (2020) 506:84–91. doi: 10.1016/j.cca.2020.03.020
22. Hellwege JN, Zhu X, Huang X, Shrubsole MJ, Fan L, Li B, et al. Blunted PTH response to vitamin d insufficiency/deficiency and colorectal neoplasia risk. Clin Nutr (2021) 40(5):3305–13. doi: 10.1016/j.clnu.2020.10.057
23. Charoenngam N, Sriussadaporn S. Vitamin d and parathyroid hormone status in community-dwelling elderly patients with mild-to-moderate kidney impairment. Int Urol Nephrol (2021) 53(5):973–83. doi: 10.1007/s11255-020-02695-5
24. Hu Y, Li S, Wang J, Zheng D, Zhang H, Yu W, et al. Threshold for relationship between vitamin d and parathyroid hormone in Chinese women of childbearing age. Int J Environ Res Public Health (2021) 18(24). doi: 10.3390/ijerph182413060
25. Zeng S, Querfeld U, Feger M, Haffner D, Hasan AA, Chu C, et al. Relationship between GFR, intact PTH, oxidized PTH, non-oxidized PTH as well as FGF23 in patients with CKD. FASEB J (2020) 34(11):15269–81. doi: 10.1096/fj.202000596R
26. Chu C, Elitok S, Zeng S, Xiong Y, Hocher CF, Hasan AA, et al. C-terminal and intact FGF23 in kidney transplant recipients and their associations with overall graft survival. BMC Nephrol (2021) 22(1):125. doi: 10.1186/s12882-021-02329-7
27. Zeng S, Slowinski T, Pommer W, Hasan AA, Gaballa MMS, Lu Y, et al. Sclerostin is an independent risk factor for all-cause mortality in kidney transplant recipients. Clin Exp Nephrol (2020) 24(12):1177–83. doi: 10.1007/s10157-020-01956-y
28. Zeng S, Hasan AA, Chu C, Xiong Y, Hocher JG, Elitok S, et al. Osteoprotegerin is an independent risk factor predicting death in stable renal transplant recipients. Clin Nephrol (2021) 96(3):129–37. doi: 10.5414/CN110470
29. Hocher B, Armbruster FP, Stoeva S, Reichetzeder C, Grön HJ, Lieker I, et al. Measuring parathyroid hormone (PTH) in patients with oxidative stress–do we need a fourth generation parathyroid hormone assay? PloS One (2012) 7(7):e40242. doi: 10.1371/journal.pone.0040242
30. Hruska KA, Korkor A, Martin K, Slatopolsky E. Peripheral metabolism of intact parathyroid hormone. role of liver and kidney and the effect of chronic renal failure. J Clin Invest (1981) 67(3):885–92. doi: 10.1172/JCI110106
31. Hocher B, Zeng S. Clear the fog around parathyroid hormone assays: what do iPTH assays really measure? Clin J Am Soc Nephrol (2018) 13(4):524–6. doi: 10.2215/CJN.01730218
32. Hocher B, Godes M, Reichetzeder C, Tsuprykov O, Chertow GM, Parfrey PS, et al. Non-oxidized PTH (n-oxPTH) is associated with cardiovascular events and all-cause mortality in patients with secondary hyperparathyroidism undergoing hemodialysis who participated in the EVOLVE trial. J Am Soc Nephrol (2014) 25:874A.
33. Hocher B, Pasch A. Hope for CKD-MBD patients: new diagnostic approaches for better treatment of CKD-MBD. Kidney Dis (Basel) (2017) 3(1):8–14. doi: 10.1159/000477244
34. Chertow GM, Block GA, Correa-Rotter R, Drüeke TB, Floege J, Goodman WG, et al. Effect of cinacalcet on cardiovascular disease in patients undergoing dialysis. N Engl J Med (2012) 367(26):2482–94. doi: 10.1056/NEJMoa1205624
35. Lu YP, Zeng S, Chu C, Hasan AA, Slowinski T, Yin LH, et al. Non-oxidized PTH (n-oxPTH) is associated with graft loss in kidney transplant recipients. Clin Chim Acta (2020) 508:92–7. doi: 10.1016/j.cca.2020.05.022
36. Ursem SR, Vervloet MG, Hillebrand JJG, de Jongh RT, Heijboer AC. Oxidation of PTH: in vivo feature or effect of preanalytical conditions? Clin Chem Lab Med (2018) 56(2):249–55. doi: 10.1515/cclm-2017-0313
37. Ursem S, Francic V, Keppel M, Schwetz V, Trummer C, Pandis M, et al. The effect of vitamin d supplementation on plasma non-oxidised PTH in a randomised clinical trial. Endocr Connect (2019) 8(5):518–27. doi: 10.1530/EC-19-0097
38. Sardar S, Chakraborty A, Chatterjee M. Comparative effectiveness of vitamin D3 and dietary vitamin e on peroxidation of lipids and enzymes of the hepatic antioxidant system in sprague–dawley rats. Int J Vitam Nutr Res (1996) 66(1):39–45.
39. Tarcin O, Yavuz DG, Ozben B, Telli A, Ogunc AV, Yuksel M, et al. Effect of vitamin d deficiency and replacement on endothelial function in asymptomatic subjects. J Clin Endocrinol Metab (2009) 94(10):4023–30. doi: 10.1210/jc.2008-1212
40. Uberti F, Lattuada D, Morsanuto V, Nava U, Bolis G, Vacca G, et al. Vitamin d protects human endothelial cells from oxidative stress through the autophagic and survival pathways. J Clin Endocrinol Metab (2014) 99(4):1367–74. doi: 10.1210/jc.2013-2103
41. Wiseman H. Vitamin d is a membrane antioxidant. ability to inhibit iron-dependent lipid peroxidation in liposomes compared to cholesterol, ergosterol and tamoxifen and relevance to anticancer action. FEBS Lett (1993) 326(1-3):285–8. doi: 10.1016/0014-5793(93)81809-e
42. Dawson-Hughes B, Harris SS, Krall EA, Dallal GE. Effect of calcium and vitamin d supplementation on bone density in men and women 65 years of age or older. N Engl J Med (1997) 337(10):670–6. doi: 10.1056/NEJM199709043371003
43. Vieth R, Ladak Y, Walfish PG. Age-related changes in the 25-hydroxyvitamin d versus parathyroid hormone relationship suggest a different reason why older adults require more vitamin d. J Clin Endocrinol Metab (2003) 88(1):185–91. doi: 10.1210/jc.2002-021064
44. Mendes MM, Hart KH, Lanham-New SA, Botelho PB. Association between 25-hydroxyvitamin d, parathyroid hormone, vitamin d and calcium intake, and bone density in healthy adult women: a cross-sectional analysis from the d-SOL study. Nutrients (2019) 11(6). doi: 10.3390/nu11061267
45. Mendes MM, Hart KH, Lanham-New SA, Botelho PB. Suppression of parathyroid hormone as a proxy for optimal vitamin d status: further analysis of two parallel studies in opposite latitudes. Nutrients (2020) 12(4). doi: 10.3390/nu12040942
46. Jemielita TO, Leonard MB, Baker J, Sayed S, Zemel BS, Shults J, et al. Association of 25-hydroxyvitamin d with areal and volumetric measures of bone mineral density and parathyroid hormone: impact of vitamin d-binding protein and its assays. Osteoporos Int (2016) 27(2):617–26. doi: 10.1007/s00198-015-3296-6
47. Aggarwal A, Yadav AK, Ramachandran R, Kumar V, Kumar V, Sachdeva N, et al. Bioavailable vitamin d levels are reduced and correlate with bone mineral density and markers of mineral metabolism in adults with nephrotic syndrome. Nephrol (Carlton) (2016) 21(6):483–9. doi: 10.1111/nep.12638
48. Heo YJ, Lee YJ, Lee K, Kim JH, Shin CH, Lee YA, et al. Total, bioavailable and free 25-hydroxyvitamin d levels as functional indicators for bone parameters in healthy children. PloS One (2021) 16(10):e0258585. doi: 10.1371/journal.pone.0258585
49. Saad RK, Akiki VC, Rahme M, Ajjour S, Assaad M, El-Hajj Fuleihan GA. Time trends and predictors of hypovitaminosis d across the life course: 2009-2016. Metabolism (2020) 105:154138. doi: 10.1016/j.metabol.2020.154138
50. Ciacci C, Bilancio G, Russo I, Iovino P, Cavallo P, Santonicola A, et al. 25-hydroxyvitamin d, 1,25-dihydroxyvitamin d, and peripheral bone densitometry in adults with celiac disease. Nutrients (2020) 12(4). doi: 10.3390/nu12040929
51. Shen M, Li Z, Lv D, Yang G, Wu R, Pan J, et al. Seasonal variation and correlation analysis of vitamin d and parathyroid hormone in hangzhou, southeast China. J Cell Mol Med (2020) 24(13):7370–7. doi: 10.1111/jcmm.15330
52. Cirillo M, Bilancio G, Guarino E, Cavallo P, Lombardi C, Costanzo S, et al. Vitamin d status and indices of mineral homeostasis in the population: differences between 25-hydroxyvitamin d and 1,25-dihydroxyvitamin d. Nutrients (2019) 11(8). doi: 10.3390/nu11081777
53. Wyskida M, Owczarek AJ, Chełmecka E, Szczerbowska I, Mossakowska M, Grodzicki T, et al. Parathyroid hormone response to different vitamin d levels in population-based old and very-old polish cohorts. Exp Gerontol (2019) 127:110735. doi: 10.1016/j.exger.2019.110735
54. McKee A, Lima Ribeiro SM, Malmstrom TK, Perry HM, Miller DK, Farr SS, et al. Screening for vitamin d deficiency in black americans: comparison of total, free, bioavailable 25 hydroxy vitamin d levels with parathyroid hormone levels and bone mineral density. J Nutr Health Aging (2018) 22(9):1045–50. doi: 10.1007/s12603-018-1080-y
55. DeBoer MD, Weber DR, Zemel BS, Denburg MR, Herskovitz R, Long J, et al. Bone mineral accrual is associated with parathyroid hormone and 1,25-dihydroxyvitamin d levels in children and adolescents. J Clin Endocrinol Metab (2015) 100(10):3814–21. doi: 10.1210/jc.2015-1637
56. Metzger M, Houillier P, Gauci C, Haymann JP, Flamant M, Thervet E, et al. Relation between circulating levels of 25(OH) vitamin d and parathyroid hormone in chronic kidney disease: quest for a threshold. J Clin Endocrinol Metab (2013) 98(7):2922–8. doi: 10.1210/jc.2013-1294
57. Valcour A, Blocki F, Hawkins DM, Rao SD. Effects of age and serum 25-OH-vitamin d on serum parathyroid hormone levels. J Clin Endocrinol Metab (2012) 97(11):3989–95. doi: 10.1210/jc.2012-2276
58. Karhapää P, Pihlajamäki J, Pörsti I, Kastarinen M, Mustonen J, Niemelä O, et al. Glomerular filtration rate and parathyroid hormone are associated with 1,25-dihydroxyvitamin d in men without chronic kidney disease. J Intern Med (2012) 271(6):573–80. doi: 10.1111/j.1365-2796.2011.02471.x
59. Houghton LA, Szymlek-Gay EA, Gray AR, Ferguson EL, Deng X, Heath AL. Predictors of vitamin d status and its association with parathyroid hormone in young new Zealand children. Am J Clin Nutr (2010) 92(1):69–76. doi: 10.3945/ajcn.2009.29055
60. Moosgaard B, Vestergaard P, Heickendorff L, Melsen F, Christiansen P, Mosekilde L. Plasma 25-hydroxyvitamin d and not 1,25-dihydroxyvitamin d is associated with parathyroid adenoma secretion in primary hyperparathyroidism: a cross-sectional study. Eur J Endocrinol (2006) 155(2):237–44. doi: 10.1530/eje.1.02197
61. Holick MF, Siris ES, Binkley N, Beard MK, Khan A, Katzer JT, et al. Prevalence of vitamin d inadequacy among postmenopausal north American women receiving osteoporosis therapy. J Clin Endocrinol Metab (2005) 90(6):3215–24. doi: 10.1210/jc.2004-2364
62. Abrams SA, Griffin IJ, Hawthorne KM, Gunn SK, Gundberg CM, Carpenter TO. Relationships among vitamin d levels, parathyroid hormone, and calcium absorption in young adolescents. J Clin Endocrinol Metab (2005) 90(10):5576–81. doi: 10.1210/jc.2005-1021
63. Bates CJ, Carter GD, Mishra GD, O'Shea D, Jones J, Prentice A. In a population study, can parathyroid hormone aid the definition of adequate vitamin d status? a study of people aged 65 years and over from the British national diet and nutrition survey. Osteoporos Int (2003) 14(2):152–9. doi: 10.1007/s00198-002-1338-3
64. Malabanan A, Veronikis IE, Holick MF. Redefining vitamin d insufficiency. Lancet (1998) 351(9105):805–6. doi: 10.1016/S0140-6736(05)78933-9
65. Thomas MK, Lloyd-Jones DM, Thadhani RI, Shaw AC, Deraska DJ, Kitch BT, et al. Hypovitaminosis d in medical inpatients. N Engl J Med (1998) 338(12):777–83. doi: 10.1056/NEJM199803193381201
66. Frelinger AL, Zull JE. The role of the methionine residues in the structure and function of parathyroid hormone. Arch Biochem Biophys (1986) 244(2):641–9. doi: 10.1016/0003-9861(86)90632-6
67. Moskovitz J. Methionine sulfoxide reductases: ubiquitous enzymes involved in antioxidant defense, protein regulation, and prevention of aging-associated diseases. Biochim Biophys Acta (2005) 1703(2):213–9. doi: 10.1016/j.bbapap.2004.09.003
68. Panda DK, Miao D, Bolivar I, Li J, Huo R, Hendy GN, et al. Inactivation of the 25-hydroxyvitamin d 1alpha-hydroxylase and vitamin d receptor demonstrates independent and interdependent effects of calcium and vitamin d on skeletal and mineral homeostasis. J Biol Chem (2004) 279(16):16754–66. doi: 10.1074/jbc.M310271200
69. Finkelstein JS, Schoenfeld DA. Effects of gonadal suppression on the regulation of parathyroid hormone and 1,25-dihydroxyvitamin d secretion in women. J Clin Endocrinol Metab (1999) 84(6):2151–6. doi: 10.1210/jc.84.6.2151
70. Delmez JA, Tindira C, Grooms P, Dusso A, Windus DW, Slatopolsky E. Parathyroid hormone suppression by intravenous 1,25-dihydroxyvitamin d. a role for increased sensitivity to calcium. J Clin Invest (1989) 83(4):1349–55. doi: 10.1172/JCI114022
71. Chen X, Chu C, Doebis C, Xiong Y, Cao Y, Krämer BK, et al. Vitamin d status and its association with parathyroid hormone in 23,134 outpatients. J Steroid Biochem Mol Biol (2022) p:106101. doi: 10.1016/j.jsbmb.2022.106101
72. Segersten U, Correa P, Hewison M, Hellman P, Dralle H, Carling T, et al. 25-hydroxyvitamin D(3)-1alpha-hydroxylase expression in normal and pathological parathyroid glands. J Clin Endocrinol Metab (2002) 87(6):2967–72. doi: 10.1210/jcem.87.6.8604
Keywords: 25-hydroxyvitamin D, 1,25-dihydroxyvitamin D, parathyroid hormone, intact parathyroid hormone, oxidized parathyroid hormone, non-oxidized parathyroid hormone
Citation: Zuo J, Hasan AA, Hocher C-F, Kalk P, Kleuser B, Krämer BK and Hocher B (2023) Inverse correlation of intact PTH, oxidized PTH as well as non-oxidized PTH with 25-hydroxyvitamin D3 in kidney transplant recipients. Front. Endocrinol. 14:1178166. doi: 10.3389/fendo.2023.1178166
Received: 02 March 2023; Accepted: 17 May 2023;
Published: 31 May 2023.
Edited by:
Mohammed S. Razzaque, Lake Erie College of Osteopathic Medicine, United StatesReviewed by:
Ignacio Portales, Massachusetts General Hospital and Harvard Medical School, United StatesAna Valea, Iuliu Hatieganu University of Medicine and Pharmacy Cluj-Napoca, Romania
Mihaela Stanciu, Lucian Blaga University of Sibiu, Romania
Copyright © 2023 Zuo, Hasan, Hocher, Kalk, Kleuser, Krämer and Hocher. This is an open-access article distributed under the terms of the Creative Commons Attribution License (CC BY). The use, distribution or reproduction in other forums is permitted, provided the original author(s) and the copyright owner(s) are credited and that the original publication in this journal is cited, in accordance with accepted academic practice. No use, distribution or reproduction is permitted which does not comply with these terms.
*Correspondence: Berthold Hocher, berthold.hocher@medma.uni-heidelberg.de
†These authors have contributed equally to this work