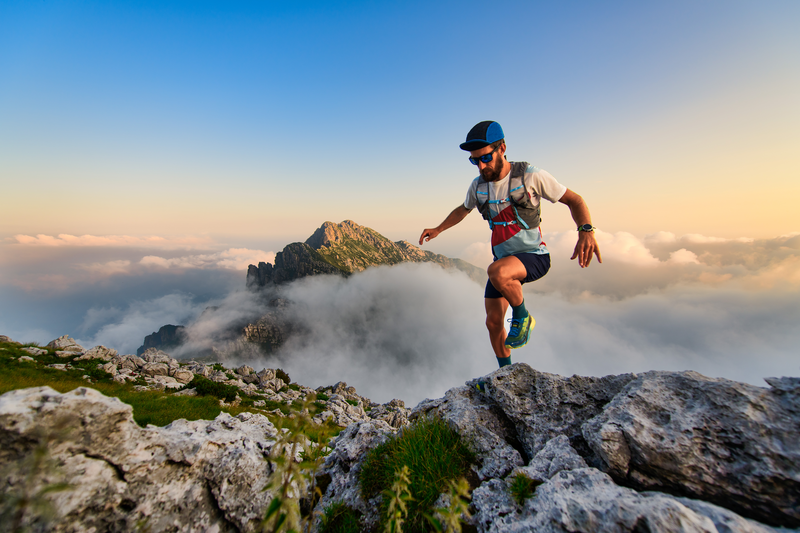
94% of researchers rate our articles as excellent or good
Learn more about the work of our research integrity team to safeguard the quality of each article we publish.
Find out more
ORIGINAL RESEARCH article
Front. Endocrinol. , 10 May 2023
Sec. Endocrinology of Aging
Volume 14 - 2023 | https://doi.org/10.3389/fendo.2023.1162936
This article is part of the Research Topic (Osteo)Sarcopenia & Sarcopenic Obesity, volume II View all 11 articles
Background: Emerging evidence suggested that coronavirus disease 2019 (COVID-19) patients were more prone to acute skeletal muscle loss and suffer sequelae, including weakness, arthromyalgia, depression and anxiety. Meanwhile, it was observed that sarcopenia (SP) was associated with susceptibility, hospitalization and severity of COVID-19. However, it is not known whether there is causal relationship between COVID‐19 and SP-related traits. Mendelian randomization (MR) was a valid method for inferring causality.
Methods: Data was extracted from the COVID‐19 Host Genetic Initiative and the UK Biobank without sample overlapping. The MR analysis was performed with inverse variance weighted, weighted median, MR-Egger, RAPS and CAUSE, MR-APSS. Sensitivity analysis was conducted with MR-Egger intercept test, Cochran’s Q test, MR-PRESSO to eliminate pleiotropy.
Results: There was insufficient result in the MR-APSS method to support a direct causal relationship after the Bonferroni correction. Most other MR results were also nominally consistent with the MR-APSS result.
Conclusions: Our study first explored the causal relationship between COVID-19 and SP-related traits, but the result indicated that they may indirectly interact with each other. We highlighted that older people had better absorb enough nutrition and strengthen exercise to directly cope with SP during the COVID-19 pandemic.
The coronavirus disease 2019 (COVID-19) has evolved into an ongoing global pandemic affecting more than 600 million people, resulting in nearly 7 million deaths (1). Age, concurrent frailty and comorbidities were associated with higher risk of being positive for COVID-19, hospitalized and mortality (2). Meanwhile, accumulating evidence suggests that COVID-19 survivors could experience various sequelae, mainly including weakness, arthromyalgia, depression, anxiety and memory loss (3). Due to the complicated pathogenesis of COVID-19, the extremely challenging pandemic has forced people to live with COVID-19, which means it is important to evaluate the relevance between COVID-19 and other comorbidities.
Sarcopenia (SP) is the loss of skeletal muscle mass associated with aging which causes an involution of muscle strength, and/or low physical performance (4). Skeletal muscle-related traits have been widely reported in both acute COVID-19 and post-acute sequelae of COVID-19 (5). Recent findings have shown that higher grip strength was associated with a lower risk of COVID-19 hospitalization and a better prognosis (6, 7). Another study reported that there was no significant difference in grip strength among COVID-19 patients with different severity after 12 weeks (8). A retrospective study showed that an acute skeletal muscle loss was evident in consecutive hospitalized patients with COVID-19 compared with those without COVID-19 and contributed to poor clinical outcomes (9). COVID-19 patients with SP had a higher number of persistent symptoms than patients without SP, but that was not statistically significant (10). These observational studies show that there appears to be a correlation between COVID-19 and SP, but it is inconsistent. These results also made it elusive to assess the causal relationship between COVID‐19 and SP-related traits.
Mendelian randomization (MR) is a valid approach to infer possible causality between exposure and outcome, reducing bias from confounding factors and reverse causality in epidemiological studies (11). In the present study, we performed a two-sample MR to assess the potential causal effect between COVID‐19 and SP-related traits using instrumental variables (IVs) from the summary genome‐wide association study (GWAS) datasets. Overall, Results obtained in this study may help for identify the role of SP in the pandemic to reduce infection and attenuate clinical symptoms. It can also provide new insight into dealing with the post-acute sequelae of COVID-19 to treat or prevent the persistence of these long-lasting symptoms.
The MR analysis was performed to explore the causality between COVID‐19 and SP-related traits. In the forward MR analysis, COVID‐19 was considered as the exposure and SP-related traits were considered as the outcome, whereas the reverse MR analysis investigated SP-related traits as the exposure and COVID‐19 as the outcome. The following 3 main assumptions were satisfied (1): the IV is tightly associated to exposure; (2) the IV is not related to any confounder of the exposure-outcome connection; (3) the IV can only affect the outcome via the exposure (11).
In this MR study, GWAS summary statistics for COVID-19 phenotypes were extracted from the COVID‐19 Host Genetic Initiative (HGI) (Round 5) (12). COVID-19 phenotypes included severity (4,792-1,054,664), hospitalization (8,316-1,549,095), susceptibility (3,2494-1,316,207). The COVID-19 cases were diagnosed by laboratory confirmation or by electrical health records (using physician notes or ICD), or self-reported COVID-19 infections from the patients. Severe COVID-19 cases were defined as patients who died or required respiratory support (including bilevel positive airway pressure, continuous positive airway pressure, intubation, or high-flow nasal cannula). The controls were defined as the individuals enrolled in the cohorts and not included as cases. The COVID-19 related data was retrieved from the European population except the UK Biobank participants. The data and more information can be found online.
All the GWAS summary statistics for SP-related traits were extracted from the UK Biobank. UK Biobank is a large-scale biomedical database and research resource, globally accessible to approved researchers undertaking vital research into the most common and life-threatening diseases, containing in-depth genetic and health information from 500 000 UK participants (13). Appendicular lean mass (ALM) has been proposed as a validated and reliable indicator of muscle mass in older adults (14). ALM was quantified by appendicular fat-free mass using the bioelectrical impedance analysis with 450,243 UK Biobank individuals and adjusted by appendicular fat mass and other covariates (15). Grip strength has been widely recognized as a significant indicator of SP (16). The hand grip strength was measured with a calibrated device in a simple and non-invasive way and adjusted for hand size (17). The UK Biobank grip strength data was adjusted for age, age2, sex, sex × age, and sex × age2 (13), including 461,089 individuals of European descent for right hand grip strength and 461,026 individuals for left hand grip strength (18). The summary‐level statistics of walking pace were also obtained from the UK Biobank, including 459,915 individuals of European ancestry (18).
The genetic IVs of COVID-19 and SP-related traits were retrieved from publicly available database without sample overlapping. Ethical permission was not applicable to this study. More details for phenotype and previously ethical approval can be found in the original publications or GWAS (12, 15, 18).
Independent single nucleotide polymorphisms (SNPs) at the genome‐wide significance level (p < 5 × 10-8) were selected as IVs for exposure (clumping r2 = 0.001 and kb = 10,000) (19). Related data of IVs were also extracted from the outcome datasets without proxies. After harmonizing each pair of the exposure and outcome datasets, the inverse variance weighted (IVW) model were conducted to assess the causality. The IVW method can provide the most accurate and stable estimation of causal effects when all IVs were valid without directional pleiotropy (20). MR‐Egger intercept test was employed to evaluate horizontal pleiotropy (21). MR pleiotropy residual sum and outlier (MR-PRESSO) was conducted to identify and obtain corrected results by removing pleiotropic IVs (22). After that, The IVW method was reperformed to assess the robustness. Several sensitivity analyses were also performed, including weighted median estimation, MR‐Egger regression, MR Robust Adjusted Profile Score (RAPS). The weighted median method could control the Type 1 error rates and provide consistent causal estimates when more than 50% IVs were valid and enrolled (23). MR-Egger regression could test IVs with considerable pleiotropy and heterogeneity, whereas this approach had poor statistical power and required larger sample size (21). RAPS could tackle the idiosyncratic pleiotropy even for up to hundreds of weak IVs (24). Heterogeneity across these selected IVs was assessed by Cochran’s Q statistic. F statistic was calculated to test the strength of genetic IVs and genetic IV with F statistics > 10 was statistically considered as a strong instrument to minimize bias. The proportion of variance explained was also measured.
Actually, a small subset of SNPs were included as IVs for causal inference due to the strict inclusion and exclusion criteria, especially for COVID-19. MR Causal Analysis Using Summary Effect Estimates (CAUSE) and MR Accounting for Pleiotropy and Sample Structure simultaneously (MR-APSS) were employed to improve statistical power in the analysis, mainly by relaxing the threshold to utilize more IVs instead of only IVs at the genome‐wide significance level (25, 26). Compared with other methods, the CAUSE method could avoid more false positives and calculate the shared (non-causal) effect, accounting for correlated pleiotropy induced by confounders or unmeasured shared factors. The q value was also calculated as an estimate of the proportion of pleiotropic variants (25). Default parameters were used in the CAUSE procedures (p < 5 × 10-3) (https://jean997.github.io/cause/ldl_cad.html). MR-APSS was a recently proposed method in 2022, accounting for sample structure as a major confounding factor including cryptic relatedness, population stratification, and sample overlap (26). We employed the same parameters as used in an originally example recommended by the authors (p < 5 × 10-5) (https://github.com/YangLabHKUST/MR-APSS/blob/master/MRAPSS_Rpackage_Tutorial.pdf).
Concerning multiple testing of COVID‐19 and SP-related traits, we conservatively adjusted the p-values after the Bonferroni correction (p = 0.05/12 = 4.17E-03). The MR analyses were conducted with the R packages “MungeSumstats”, “TwosampleMR”, “CAUSE”, “MRAPSS” in the R statistical software (Version 4.1.3).
In the forward MR analysis, we analyzed the causal effect of COVID-19 on SP-related traits. The IVW results suggested that susceptibility, hospitalization and severity of COVID-19 had no causal effect on ALM, right hand grip strength, left hand grip strength and walking pace after the Bonferroni correction (Table 1; Figure 1). Consistently, the weighted median, the RAPS, the MR‐Egger, the CAUSE, the MR-APSS methods further strengthened the hypothesis that COVID-19 was not a causal risk factor for SP-related traits (Table 2). Notably, several results indicated the nominal causality of COVID-19 on SP-related traits using the IVW and the RAPS methods, contradicting the results with the CAUSE, the MR-APSS methods. The median shared effect ranged from –0.04 to 0.03 in the CAUSE methods, suggesting rarely bias induced by horizontal pleiotropy. The low q indicated poor correlation between genetic effects of COVID-19 on SP-related traits.
Figure 1 Forest plot of MR IVW analyses between COVID-19 and SP-related traits. IVW, inverse-variance weighted; CI, confidence interval; ALM, appendicular lean mass; COVID-19, coronavirus disease 2019. MR, mendelian randomization; SP, sarcopenia.
Table 2 Mendelian randomization estimates of COVID-19 on sarcopenia-related traits using the CAUSE and MR-APSS methods.
In the reverse MR analysis, similar results were identified in the five MR tests, reflecting that SP-related traits had no causal effect on COVID-19 after the Bonferroni correction (Tables 3, 4). The median shared effect ranged from –3.02 to 0.36, meaning bias induced by pleiotropic variants.
Table 4 Mendelian randomization estimates of sarcopenia-related traits on COVID-19 using the CAUSE and MR-APSS methods.
To evaluate the robustness of the above results, extensive sensitivity analyses were performed, including Cochran’s Q test, MR-Egger intercept test, MR-PRESSO global test, and F statistics (Table 5). The Cochran’s Q test identified 2 pair of the exposure and outcome datasets so that a random-effects model was applied for them. After removing outliers detected by MR‐PRESSO (Supplementary Table 1), we only observed significantly horizontal pleiotropy of walking pace on hospitalization in MR-Egger intercept test. All the F statistics of selected IVs were above than 10, indicating that they were valid enough to minimize potential bias. The proportion of variance explained grew with the increasing IVs, especially in the MR-APSS, the CAUSE methods (Supplementary Table 2). Details of IVs were provided in Additional Tables.
Table 5 Sensitivity analysis of the primary causal association between COVID-19 and sarcopenia-related traits .
Based on the MR results in our analysis, we conservatively summarized that there was insufficient evidence to determine a causal link between COVID-19 and SP-related traits after the Bonferroni correction. Most MR results were also nominally consistent with the conclusion. We performed the first bi-directional two-sample MR analysis to evaluate causal relationship between COVID-19 and SP-related traits, using the MR-APSS method.
Actually, several studies with related themes were reported. Three MR studies indicated that genetic evidence did not support a significant causal effect between COVID‐19 and telomere length (27–29), although the cohort study in UK Biobank showed that shorter telomere length was associated with higher risk of adverse COVID-19 outcomes (29). A MR study including 261,000 older participants estimated that telomere length would not affect grip strength, sarcopenia, or falls (30). Telomere length did not occupy a unique position in the causal relationship between COVID-19 and SP-related traits. Another three MR studies suggested that physical activity had no causal effect on COVID-19 outcomes after the Bonferroni correction, but the results nominally contradicted each other (31–33). Meanwhile, the observational study also reported a protective effect of objectively measured physical activity on COVID-19 outcomes (33). A meta-analysis of 7 randomized controlled trials showed that exercise could improve muscle mass, muscle strength, and walking speed in 3 months (34). A recent study including 435,504 UK Biobank participants observed a paradoxical result that lean mass index was not associated with COVID-19 phenotypes in a prospective cohort study while lean mass had a significant positive causal effect on COVID-19 outcomes in related MR analysis, which might require more robust MR Methods and better lean mass related data sources (35). Combined, due to ethical and practical constraints, cross-sectional designs and most low-quality randomized controlled trials could only provide correlation rather than causality. Meanwhile, although MR study could evaluate causality, it was also affected by quality of data sources and MR methods. Our MR results so far did not conflict with most findings of related MR studies or RCTs.
Undoubtedly, there were many clinical studies with high quality observed that COVID-19 was associated with acute SP in hospital and SP in long COVID-19 syndrome (9, 10). It was also observed that the presence of SP in the general population was positively correlated with the infection rate of COVID-19 (36), which contributed to poor clinical outcomes. Obviously, they appeared to form a dangerous vicious cycle. However, our MR results did not provide sufficient evidence to support a direct interaction between COVID-19 and SP, so that other factors may participate in this cycle to assist its formation. Malnutrition, reduced activity, distress and anxiety were likely to play indispensable roles in the cycle. Compared to discharged patients, patients with COVID-19 who died had higher nutritional and SP risk, lower albumin and total protein (37, 38). The muscle would atrophy significantly within two days after fixation and progress over the next 5 days (39). Patients with COVID-19 usually stay in hospital for more than 10 days (40, 41). During the acute period of COVID-19, common symptoms included depression mood (32.6%), anxiety (35.7%), insomnia (41.9%) (42). During the lockdown period, malnutrition, reduced activity, distress and anxiety were also prevalent (43–45), which was also positively correlated with susceptibility, hospitalization and severity of COVID-19 (46). These factors might act as competitive confounders in an observational study or mediating factors in a MR study. Cognitive impairment, frailty and other aging-associated diseases had the potential to serve as candidate factors (47, 48). On the basis of these observations and our MR results, we highlighted that older people should pay more attention to prevention, diagnosis, and treatment of SP instead of specific interactions between COVID-19 and SP, which would make it easier to break the dangerous vicious cycle and improve quality of life. In addition, targeting other mediating factors might also play a role. Nutritional supplementation and muscle training could provide significant improvement in muscle function and strength for COVID-19 survivors (49, 50).
In the COVID-19 pandemic, it has been reported that patients with more severe COVID-19 infection had a higher elevated serum creatine kinase level and more prone to rhabdomyolysis (51, 52), indicating that COVID-19 could cause damage to skeletal muscle. The mechanisms of individual organ damage might involve a systemic inflammatory response (53). In autopsy analysis, severe acute respiratory syndrome coronavirus 2(SARS-CoV2) virus particles were detected in organs including the heart, liver and kidney (54–56), while SARS-CoV virus particle could not be detected in skeletal muscle in SARS patients (57). Combined our MR results, we prefer that skeletal muscle injury is attributed to systemic inflammation instead of direct virus invasion. Nowadays, most countries and regions stopped requiring SARS-CoV-2 testing in public places, and mandatory quarantine (58). After vaccination, older people can adopt healthy lifestyles by increasing physical activity, improving glycemic control and body weight to reverse the situation in preparation for the next COVID-wave. Obviously, future studies across RCT or basic research from a variety of ethnic backgrounds are required to completely disentangle pathogenic mechanisms and develop effective interventions for attenuate clinical symptoms.
This study is the first bi-directional MR study to evaluate causal relationship between COVID-19 and SP-related traits, using the MR-APSS method. All parameters were set according to recommendations and thresholds were not relaxed. The COVID-19 related data was retrieved from the European population except the UK Biobank participants and the sarcopenia related data was extracted from the UK Biobank, which avoided sample overlapping and reduced bias. Extremely strict parameters and F statistic were used to ensure the validity of IV. CAUSE and MR-APSS were performed to include more IVs to improve statistical power, and consistent results were obtained. Nevertheless, there were several potential limitations in our MR analysis. First, all the data came from the European population therefore our result might only apply to Europeans. Second, it was difficult to completely remove mediation and pleiotropy so that we cannot rule out the possibility that mediating factors mediating the causality between COVID-19 and SP-related traits. Third, though the GWAS data was constantly being updated, better data sources were still required, especially for COVID-19.
The mechanisms between COVID-19 and SP have not yet been fully elucidated. Our MR results did not support a direct causal relationship after the Bonferroni correction, indicating that they may indirectly interact with each other through systemic inflammatory response and other diseases. Our new insights might inform better practices to recognize, evaluate and both prevent and treat SP in the COVID-19 pandemic. We highlighted that older people should absorb adequate nutrition and strengthen exercise to cope with SP and break the dangerous vicious cycle.
The original contributions presented in the study are included in the article/Supplementary Material. Further inquiries can be directed to the corresponding authors.
CL had the idea and drafted the final manuscript, NL performed data analysis, YZ and BX created the tables. Other authors gave constructive suggestions during the process. TX and HL drafted the final manuscript. All authors contributed to the article and approved the submitted version.
This work was supported by Research Project of Human Health Commission (grant number 202204073071).
All data used in this study were obtained from openly available databases and consortiums. The authors thank all investigators for providing the data publicly.
The authors declare that the research was conducted in the absence of any commercial or financial relationships that could be construed as a potential conflict of interest.
All claims expressed in this article are solely those of the authors and do not necessarily represent those of their affiliated organizations, or those of the publisher, the editors and the reviewers. Any product that may be evaluated in this article, or claim that may be made by its manufacturer, is not guaranteed or endorsed by the publisher.
The Supplementary Material for this article can be found online at: https://www.frontiersin.org/articles/10.3389/fendo.2023.1162936/full#supplementary-material
1. WHO coronavirus COVID-19 dashboard 2022 . Available at: https://covid19.who.int/.
2. Mak JKL, Kuja-Halkola R, Wang YZ, Hagg S, Jylhava J. Frailty and comorbidity in predicting community COVID-19 mortality in the UK biobank: the effect of sampling. J Am Geriatr Soc (2021) 69(5):1128–39. doi: 10.1111/jgs.17089
3. Han Q, Zheng B, Daines L, Sheikh A. Long-term sequelae of COVID-19: a systematic review and meta-analysis of one-year follow-up studies on post-COVID symptoms. Pathogens (2022) 11(2):269. doi: 10.3390/pathogens11020269
4. Fernández-Lázaro D, Garrosa E, Seco-Calvo J, Garrosa M. Potential satellite cell-linked biomarkers in aging skeletal muscle tissue: proteomics and proteogenomics to monitor sarcopenia. Proteomes (2022) 10(3):29. doi: 10.3390/proteomes10030029
5. Soares MN, Eggelbusch M, Naddaf E, Gerrits KHL, van der Schaaf M, van den Borst B, et al. Skeletal muscle alterations in patients with acute covid-19 and post-acute sequelae of covid-19. J Cachexia Sarcopenia Muscle (2022) 13(1):11–22. doi: 10.1002/jcsm.12896
6. Pucci G, D’Abbondanza M, Curcio R, Alcidi R, Campanella T, Chiatti L, et al. Handgrip strength is associated with adverse outcomes in patients hospitalized for COVID-19-associated pneumonia. Intern Emerg Med (2022) 17(7):1997–2004. doi: 10.1007/s11739-022-03060-3
7. Cheval B, Sieber S, Maltagliati S, Millet GP, Formanek T, Chalabaev A, et al. Muscle strength is associated with COVID-19 hospitalization in adults 50 years of age or older. J Cachexia Sarcopenia Muscle (2021) 12(5):1136–43. doi: 10.1002/jcsm.12738
8. Karasu AU, Karataş L, Yildiz Y, Günendi Z. Natural course of muscular strength, physical performance, and musculoskeletal symptoms in hospitalized patients with COVID-19Arch phys med rehabil. (2022) 104(1):18–26. doi: 10.1016/j.apmr.2022.09.001
9. Attaway A, Welch N, Dasarathy D, Amaya-Hughley J, Bellar A, Biehl M, et al. Acute skeletal muscle loss in SARS-CoV-2 infection contributes to poor clinical outcomes in COVID-19 patients. J Cachexia Sarcopenia Muscle (2022) 13(5):2436–46. doi: 10.1002/jcsm.13052
10. Martone AM, Tosato M, Ciciarello F, Galluzzo V, Zazzara MB, Pais C, et al. Sarcopenia as potential biological substrate of long COVID-19 syndrome: prevalence, clinical features, and risk factors. J Cachexia Sarcopenia Muscle (2022) 13(4):1974–82. doi: 10.1002/jcsm.12931
11. Skrivankova VW, Richmond RC, Woolf BAR, Yarmolinsky J, Davies NM, Swanson SA, et al. Strengthening the reporting of observational studies in epidemiology using mendelian randomization: the STROBE-MR statement. JAMA (2021) 326(16):1614–21. doi: 10.1001/jama.2021.18236
12. Initiative C-HG. Mapping the human genetic architecture of COVID-19. Nature (2021) 600(7889):472–7. doi: 10.1038/s41586-021-03767-x
13. Sudlow C, Gallacher J, Allen N, Beral V, Burton P, Danesh J, et al. UK Biobank: an open access resource for identifying the causes of a wide range of complex diseases of middle and old age. PloS Med (2015) 12(3):e1001779. doi: 10.1371/journal.pmed.1001779
14. Cawthon PM, Peters KW, Shardell MD, McLean RR, Dam TT, Kenny AM, et al. Cutpoints for low appendicular lean mass that identify older adults with clinically significant weakness. J Gerontol A Biol Sci Med Sci (2014) 69(5):567–75. doi: 10.1093/gerona/glu023
15. Pei YF, Liu YZ, Yang XL, Zhang H, Feng GJ, Wei XT, et al. The genetic architecture of appendicular lean mass characterized by association analysis in the UK biobank study. Commun Biol (2020) 3(1):608. doi: 10.1038/s42003-020-01334-0
16. Cruz-Jentoft AJ, Bahat G, Bauer J, Boirie Y, Bruyere O, Cederholm T, et al. Sarcopenia: revised European consensus on definition and diagnosis. Age Ageing (2019) 48(1):16–31. doi: 10.1093/ageing/afy169
17. Biobank U. Grip strength measurement [updated 2011 . Available at: https://biobank.ndph.ox.ac.uk/showcase/ukb/docs/Gripstrength.pdf.
18. Mitchell RE EB, Mitchell R, Raistrick CA, Paternoster L, Hemani G, Gaunt TR. MRC IEU UK biobank GWAS pipeline version 2. University of Bristol (2019). doi: 10.5523/bris.pnoat8cxo0u52p6ynfaekeigi
19. Clarke L, Zheng-Bradley X, Smith R, Kulesha E, Xiao C, Toneva I, et al. The 1000 genomes project: data management and community access. Nat Methods (2012) 9(5):459–62. doi: 10.1038/nmeth.1974
20. Burgess S, Butterworth A, Thompson SG. Mendelian randomization analysis with multiple genetic variants using summarized data. Genet Epidemiol (2013) 37(7):658–65. doi: 10.1002/gepi.21758
21. Bowden J, Davey Smith G, Burgess S. Mendelian randomization with invalid instruments: effect estimation and bias detection through egger regression. Int J Epidemiol (2015) 44(2):512–25. doi: 10.1093/ije/dyv080
22. Verbanck M, Chen CY, Neale B, Do R. Detection of widespread horizontal pleiotropy in causal relationships inferred from mendelian randomization between complex traits and diseases. Nat Genet (2018) 50(5):693–8. doi: 10.1038/s41588-018-0099-7
23. Bowden J, Davey Smith G, Haycock PC, Burgess S. Consistent estimation in mendelian randomization with some invalid instruments using a weighted median estimator. Genet Epidemiol (2016) 40(4):304–14. doi: 10.1002/gepi.21965
24. Zhao Q, Wang J, Hemani G, Bowden J, Small DS. Statistical inference in two-sample summary-data mendelian randomization using robust adjusted profile score. Ann Statistics (2020) 48(3):1742–69. doi: 10.1214/19-AOS1866
25. Morrison J, Knoblauch N, Marcus JH, Stephens M, He X. Mendelian randomization accounting for correlated and uncorrelated pleiotropic effects using genome-wide summary statistics. Nat Genet (2020) 52(7):740–7. doi: 10.1038/s41588-020-0631-4
26. Hu X, Zhao J, Lin Z, Wang Y, Peng H, Zhao H, et al. Mendelian randomization for causal inference accounting for pleiotropy and sample structure using genome-wide summary statistics. Proc Natl Acad Sci U S A (2022) 119(28):e2106858119. doi: 10.1073/pnas.2106858119
27. Huang D, Lin S, He J, Wang Q, Zhan Y. Association between COVID-19 and telomere length: a bidirectional mendelian randomization study. J Med Virol (2022) 94(11):5345–53. doi: 10.1002/jmv.28008
28. Jiang L, Tang BS, Guo JF, Li JC. Telomere length and COVID-19 outcomes: a two-sample bidirectional mendelian randomization study. Front Genet (2022) 13:805903. doi: 10.3389/fgene.2022.805903
29. Wang Q, Codd V, Raisi-Estabragh Z, Musicha C, Bountziouka V, Kaptoge S, et al. Shorter leukocyte telomere length is associated with adverse COVID-19 outcomes: a cohort study in UK biobank. EBioMedicine (2021) 70:103485. doi: 10.1016/j.ebiom.2021.103485
30. Kuo CL, Pilling LC, Kuchel GA, Ferrucci L, Melzer D. Telomere length and aging-related outcomes in humans: a mendelian randomization study in 261,000 older participants. Aging Cell (2019) 18(6):e13017. doi: 10.1111/acel.13017
31. Li S, Hua X. Modifiable lifestyle factors and severe COVID-19 risk: a mendelian randomisation study. BMC Med Genomics (2021) 14(1):38. doi: 10.1186/s12920-021-00887-1
32. Chen X, Hong X, Gao W, Luo S, Cai J, Liu G, et al. Causal relationship between physical activity, leisure sedentary behaviors and COVID-19 risk: a mendelian randomization study. J Transl Med (2022) 20(1):216. doi: 10.1186/s12967-022-03407-6
33. Zhang X, Li X, Sun Z, He Y, Xu W, Campbell H, et al. Physical activity and COVID-19: an observational and mendelian randomisation study. J Glob Health (2020) 10(2):020514. doi: 10.7189/jogh.10.020514
34. Yoshimura Y, Wakabayashi H, Yamada M, Kim H, Harada A, Arai H. Interventions for treating sarcopenia: a systematic review and meta-analysis of randomized controlled studies. J Am Med Dir Assoc (2017) 18(6):553 e1– e16. doi: 10.1016/j.jamda.2017.03.019
35. Gao M, Wang Q, Piernas C, Astbury NM, Jebb SA, Holmes MV, et al. Associations between body composition, fat distribution and metabolic consequences of excess adiposity with severe COVID-19 outcomes: observational study and mendelian randomisation analysis. Int J Obes (Lond) (2022) 46(5):943–50. doi: 10.1038/s41366-021-01054-3
36. Xu Y, Xu JW, You P, Wang BL, Liu C, Chien CW, et al. Prevalence of sarcopenia in patients with COVID-19: a systematic review and meta-analysis. Front Nutr (2022) 9:925606. doi: 10.3389/fnut.2022.925606
37. da Silva CL, Sousa TMM, de Sousa Junior JB, Nakano EY. Nutritional factors associated with mortality in hospitalized patients with COVID-19. Clin Nutr Open Sci (2022) 45:17–26. doi: 10.1016/j.nutos.2022.08.001
38. Vong T, Yanek LR, Wang L, Yu H, Fan C, Zhou E, et al. Malnutrition increases hospital length of stay and mortality among adult inpatients with COVID-19. Nutrients (2022) 14(6):1310. doi: 10.3390/nu14061310
39. Kilroe SP, Fulford J, Jackman SR, LJC VANL, Wall BT. Temporal muscle-specific disuse atrophy during one week of leg immobilization. Med Sci Sports Exerc (2020) 52(4):944–54. doi: 10.1249/MSS.0000000000002200
40. Zhao W, Zha X, Wang N, Li D, Li A, Yu S. Clinical characteristics and durations of hospitalized patients with COVID-19 in Beijing: a retrospective cohort study. Cardiovasc Innov Applications (2021) 6(1):33–44. doi: 10.15212/CVIA.2021.0019
41. Thai PQ, Toan DTT, Son DT, Van HTH, Minh LN, Hung LX, et al. Factors associated with the duration of hospitalisation among COVID-19 patients in Vietnam: a survival analysis. Epidemiol Infect (2020) 148:e114. doi: 10.1017/S0950268820001259
42. Rogers JP, Chesney E, Oliver D, Pollak TA, McGuire P, Fusar-Poli P, et al. Psychiatric and neuropsychiatric presentations associated with severe coronavirus infections: a systematic review and meta-analysis with comparison to the COVID-19 pandemic. Lancet Psychiatry (2020) 7(7):611–27. doi: 10.1016/S2215-0366(20)30203-0
43. Verma S, Mishra A. Depression, anxiety, and stress and socio-demographic correlates among general Indian public during COVID-19. Int J Soc Psychiatry (2020) 66(8):756–62. doi: 10.1177/0020764020934508
44. Ghanem J, Colicchio B, Andrès E, Geny B, Dieterlen A. Lockdown effect on elderly nutritional health. J Clin Med (2021) 10(21):5052. doi: 10.3390/jcm10215052
45. Kite C, Lagojda L, Clark CCT, Uthman O, Denton F, McGregor G, et al. Changes in physical activity and sedentary behaviour due to enforced COVID-19-Related lockdown and movement restrictions: a protocol for a systematic review and meta-analysis. Int J Environ Res Public Health (2021) 18(10):5251. doi: 10.3390/ijerph18105251
46. Barrea L, Vetrani C, Caprio M, Cataldi M, Ghoch ME, Elce A, et al. From the ketogenic diet to the Mediterranean diet: the potential dietary therapy in patients with obesity after CoVID-19 infection (Post CoVID syndrome). Curr Obes Rep (2022) 11(3):144–65. doi: 10.1007/s13679-022-00475-z
47. Ciarambino T, Crispino P, Minervini G, Giordano M. COVID-19 and frailty. Vaccines (Basel) (2023) 11(3):606. doi: 10.3390/vaccines11030606
48. Kashtanova DA, Erema VV, Gusakova MS, Sutulova ER, Yakovchik AY, Ivanov MV, et al. Mortality and survival in nonagenarians during the COVID-19 pandemic: unstable equilibrium of aging. Front Med (Lausanne) (2023) 10:1132476. doi: 10.3389/fmed.2023.1132476
49. Galluzzo V, Zazzara MB, Ciciarello F, Savera G, Pais C, Calvani R, et al. Fatigue in covid-19 survivors: the potential impact of a nutritional supplement on muscle strength and function. Clin Nutr ESPEN (2022) 51:215–21. doi: 10.1016/j.clnesp.2022.08.029
50. Del Corral T, Garrido RF, Plaza-Manzano G, Fernández-de-Las-Peñas C, Navarro-Santana M, López-de-Uralde-Villanueva I. Home-based respiratory muscle training on quality of life and exercise tolerance in long-term post-COVID-19: randomized controlled trial. Ann Phys Rehabil Med (2023) 66(1):101709. doi: 10.1016/j.rehab.2022.101709
51. Mao L, Jin H, Wang M, Hu Y, Chen S, He Q, et al. Neurologic manifestations of hospitalized patients with coronavirus disease 2019 in wuhan, China. JAMA Neurol (2020) 77(6):683–90. doi: 10.1001/jamaneurol.2020.1127
52. Suwanwongse K, Shabarek N. Rhabdomyolysis as a presentation of 2019 novel coronavirus disease. Cureus (2020) 12(4):e7561. doi: 10.7759/cureus.7561
53. Liu J, Li S, Liu J, Liang B, Wang X, Wang H, et al. Longitudinal characteristics of lymphocyte responses and cytokine profiles in the peripheral blood of SARS-CoV-2 infected patients. EBioMedicine (2020) 55:102763. doi: 10.1016/j.ebiom.2020.102763
54. Puelles VG, Lütgehetmann M, Lindenmeyer MT, Sperhake JP, Wong MN, Allweiss L, et al. Multiorgan and renal tropism of SARS-CoV-2. New Engl J Med (2020) 383(6):590–2. doi: 10.1056/NEJMc2011400
55. Farkash EA, Wilson AM, Jentzen JM. Ultrastructural evidence for direct renal infection with SARS-CoV-2. J Am Soc Nephrol (2020) 31(8):1683–7. doi: 10.1681/ASN.2020040432
56. Wichmann D, Sperhake J-P, Lütgehetmann M, Steurer S, Edler C, Heinemann A, et al. Autopsy findings and venous thromboembolism in patients with COVID-19. Ann Internal Med (2020) 173(4):268–77. doi: 10.7326/M20-2003
57. Ding Y, He L, Zhang Q, Huang Z, Che X, Hou J, et al. Organ distribution of severe acute respiratory syndrome (SARS) associated coronavirus (SARS-CoV) in SARS patients: implications for pathogenesis and virus transmission pathways. J Pathol (2004) 203(2):622–30. doi: 10.1002/path.1560
Keywords: COVID-19, sarcopenia, mendelian randomization, long COVID-19, aging
Citation: Liu C, Liu N, Zeng Y, Xiao B, Wang P, Zhou C, Xia Y, Zhao Z, Xiao T and Li H (2023) COVID‐19 and sarcopenia-related traits: a bidirectional Mendelian randomization study. Front. Endocrinol. 14:1162936. doi: 10.3389/fendo.2023.1162936
Received: 10 February 2023; Accepted: 27 April 2023;
Published: 10 May 2023.
Edited by:
Yannis Dionyssiotis, General University Hospital of Patras, GreeceReviewed by:
Xinrui Wu, Jishou University, ChinaCopyright © 2023 Liu, Liu, Zeng, Xiao, Wang, Zhou, Xia, Zhao, Xiao and Li. This is an open-access article distributed under the terms of the Creative Commons Attribution License (CC BY). The use, distribution or reproduction in other forums is permitted, provided the original author(s) and the copyright owner(s) are credited and that the original publication in this journal is cited, in accordance with accepted academic practice. No use, distribution or reproduction is permitted which does not comply with these terms.
*Correspondence: Hui Li, bGlodWl4QGNzdS5lZHUuY24=; Tao Xiao, eGlhb3Rhb3h5bEBjc3UuZWR1LmNu
Disclaimer: All claims expressed in this article are solely those of the authors and do not necessarily represent those of their affiliated organizations, or those of the publisher, the editors and the reviewers. Any product that may be evaluated in this article or claim that may be made by its manufacturer is not guaranteed or endorsed by the publisher.
Research integrity at Frontiers
Learn more about the work of our research integrity team to safeguard the quality of each article we publish.