- 1Guangdong Provincial Key Laboratory of Stomatology Hospital of Stomatology, Guanghua School of Stomatology, Sun Yat-sen University, South China Center of Craniofacial Stem Cell Research, Guangzhou, China
- 2Department of Temporomandibular Joint, Affiliated Stomatology Hospital of Guangzhou Medical University, Guangzhou Medical University, Guangzhou, China
- 3Guangzhou Key Laboratory of Basic and Applied Research of Oral Regenerative Medicine, Guangdong Engineering Research Center of Oral Restoration and Reconstruction, Guangzhou, China
Osteoarthritis (OA) is a disabling disease with significant morbidity worldwide. OA attacks the large synovial joint, including the peripheral joints and temporomandibular joint (TMJ). As a representative of peripheral joint OA, knee OA shares similar symptoms with TMJ OA. However, these two joints also display differences based on their distinct development, anatomy, and physiology. Extracellular vesicles (EVs) are phospholipid bilayer nanoparticles, including exosomes, microvesicles, and apoptotic bodies. EVs contain proteins, lipids, DNA, micro-RNA, and mRNA that regulate tissue homeostasis and cell-to-cell communication, which play an essential role in the progression and treatment of OA. They are likely to partake in mechanical response, extracellular matrix degradation, and inflammatory regulation during OA. More evidence has shown that synovial fluid and synovium-derived EVs may serve as OA biomarkers. More importantly, mesenchymal stem cell-derived EV shows a therapeutic effect on OA. However, the different function of EVs in these two joints is largely unknown based on their distinct biological characteristic. Here, we reviewed the effects of EVs in OA progression and compared the difference between the knee joint and TMJ, and summarized their potential therapeutic role in the treatment of OA.
1 Introduction
Extracellular vesicles (EVs) are nanoscale sphere-like phospholipid bilayer particles secreted by cells in a physiological or pathological state (1, 2). EVs can inherit bioactive substances from their host cells, including proteins, lipids, DNA, micro-RNA (miRNA), and mRNA (3). Nevertheless, EVs are not only carriers of bioactive substances. They also deliver their contents to target cells by specific ligand-receptor binding patterns or endocytosis (4). From this, EVs can mediate the communication between cells and affect the biological behavior of target cells (5). The diameter of EVs is generally 30-2000 nm. Based on particle size and generation pattern, EVs can be divided into three main types: exosomes, microvesicles, and apoptotic vesicles (apoVs) (6, 7). Exosomes exist in daily cellular activities and are usually considered to be intermediate between 30-150 nm. With the stage of endocytosis, sorting endosomes and multivesicular bodies, exosomes are finally assembled and released to specific tissue (8, 9). The diameter of microvesicles is larger than that of exosomes, which is about 200-1000 nm. Compared to exosomes formed by endocytosis, microvesicles shed directly from the cytoplasmic membrane (10). ApoVs are produced during the process of cell apoptosis, which include apoptotic bodies, apoptotic microvesicles, and apoptotic exosomes (11, 12). Different from other vesicles, the diameter of apoVs is variable and previous studies mainly focused on apoptotic bodies in 1-5 µm diameter (13–15). Recent studies show that apoptosis also encompass apoptotic microvesicles (100-1000 nm in diameter) and apoptotic exosomes (<150 nm in diameter) (16, 17). Emerging evidence shows EVs widely participate in cell activity and pathological processes.
As the population ages globally, joint trauma rate and the incidence of osteoarthritis (OA) are increasing yearly, affecting more than 240 million people and imposing a significant medical burden on society (18, 19). OA is a degenerative joint disease characterized by synovial inflammation, progressive cartilage degradation, and subchondral bone remodeling, leading to joint pain, deformity, and dysfunction (20). OA can affect both peripheral joints and temporomandibular joints (TMJ), which are synovial joints (21). The most affected peripheral joints are the joints of the fingers, knee, and hip joints. Their symptoms can interfere with work and normal daily activities (22). TMJ are the two synovial joints connecting the jawbone to the skull. Similar symptoms with peripheral and TMJ OA cause joint pain and movement limitation. Different from peripheral joint OA, TMJ OA is the terminal stage of TMJ disorder and often presents with abnormal jaw movement and restricted mouth opening, partly accompanied by tinnitus, headache, and other symptoms (23).
Traditional treatment emphasizes symptomatic treatment. However, it showed a limited effect in reversing the destruction of cartilage or subchondral bone (24). Although emerging stem cell therapies can promote cartilage tissue regeneration, shortcomings such as immune exclusion and tumorigenicity alarmed us (13, 25). The therapeutic effects of stem cells are mainly attributed to their paracrine effects, and EVs are one of the important components of paracrine secretion (26). Compared with stem cell therapy, EVs have many superiorities, including explicit pathways of effect, low immunogenicity, low tumorigenicity, easy preservation, and no need to consider cell survival and abnormal differentiation (27). So EVs possess a safer and greater tissue regeneration characteristic. Mesenchymal stem cell (MSC)-derived EVs have been shown to have notable effectiveness in OA on pain relief, inhibition of inflammation, immunomodulation, and cartilage tissue regeneration (28–31).
Furthermore, EVs carrying specific substances can be used as potential biomarkers for the diagnosis of OA and for monitoring the progression of OA (32). EVs, as a future cell-free therapy, show promising applications in joint diseases. It is expected to become a better alternative to MSC therapy in tissue regeneration. Although the significance of EVs in the pathogenesis and treatment of OA has been reviewed elsewhere (33–36), there is still lacking a review that summarizes the similarities and differences about EV function between peripheral joint and TMJ OA. This manuscript mainly compares the difference between the peripheral joints and TMJ and reviews the role of EVs in the pathogenesis and treatment of peripheral joints OA and TMJ OA. We also highlight the challenges facing EVs used as a conventional biologic agent for the treatment of OA and the significance of exploring next-generation EV-drug in the future.
2 The difference between peripheral joint and TMJ
2.1 The anatomy and development in TMJ and peripheral joint
The difference between the peripheral joint and TMJ is mainly generalized for anatomy and development. Peripheral joints include knee, hip, shoulder, elbow, wrist, and ankle joints, most of which are subordinate to synovial joints. The typical structure of synovial is comprised of two opposing skeletal elements and intermediate discs capsuled by synovial tissue, permitting a wide and low-friction movement (37). The knee is the largest synovial joint and is studied the most. Here, we used the knee joint as a representative to compare with TMJ, which both belong to synovial joints. As a typical single synovial joint, the knee comprises the patella, femur, tibia, fibula, meniscus, and surrounding ligaments and joint capsule (38). The TMJ also comprises the synovial joint capsule and ligaments, temporal fossa, articular tuberosity, mandibular condyle, and articular disc (39). Besides the typical synovial joint structures, TMJ is the only bilaterally linked joint characterized by sophisticated structure, precise regulation, and complicated functions (40). The other anatomical difference is the nerve distribution, which may be the basis for the distinct symptoms of TMJ OA. No sensory structures are included within the 3 cm diameter sphere centered on the meniscus of the knee. In contrast, several important anatomical structures and sensory nerves are within the 3 cm diameter sphere area centered on the TMJ disc, such as the cochlea, brain, trigeminal ganglion, mandibular nerve, and auriculotemporal nerve (41). The nerve-rich structure may be the reason that TMJ OA patients can be more susceptible to neurological symptoms (42).
The different growth pattern is the other reason for TMJ specificity. TMJ is derived from the cranial neural crest in the way of intramembranous ossification, whereas peripheral joints are mainly derived from cell migration of lateral plate mesoderm by endochondral ossification, which may contribute to the histological differences between the two joints (43). The articular cartilage type on the surface of the condyle and the knee joints is distinguishable. It is known that the articular surface of the knee is covered by hyaline cartilage with varying proportions of type I and type II collagen, whereas the surface of TMJ is covered by fibrocartilage and is composed mainly of type I collagen (32). In addition, the growth pattern of condylar is different from the long bones. Condylar have ever been regarded as a semi-epiphyseal plate of long bone, but now it has been proven to be wrong (44). The condylar cartilage derived from the cranial neural crest is secondary cartilage, undergoes endochondral ossification, and exhibits characteristic developmental processes, whereas the cartilage of long bones directly originates from embryonic cartilage primordia (45, 46) (Figure 1).
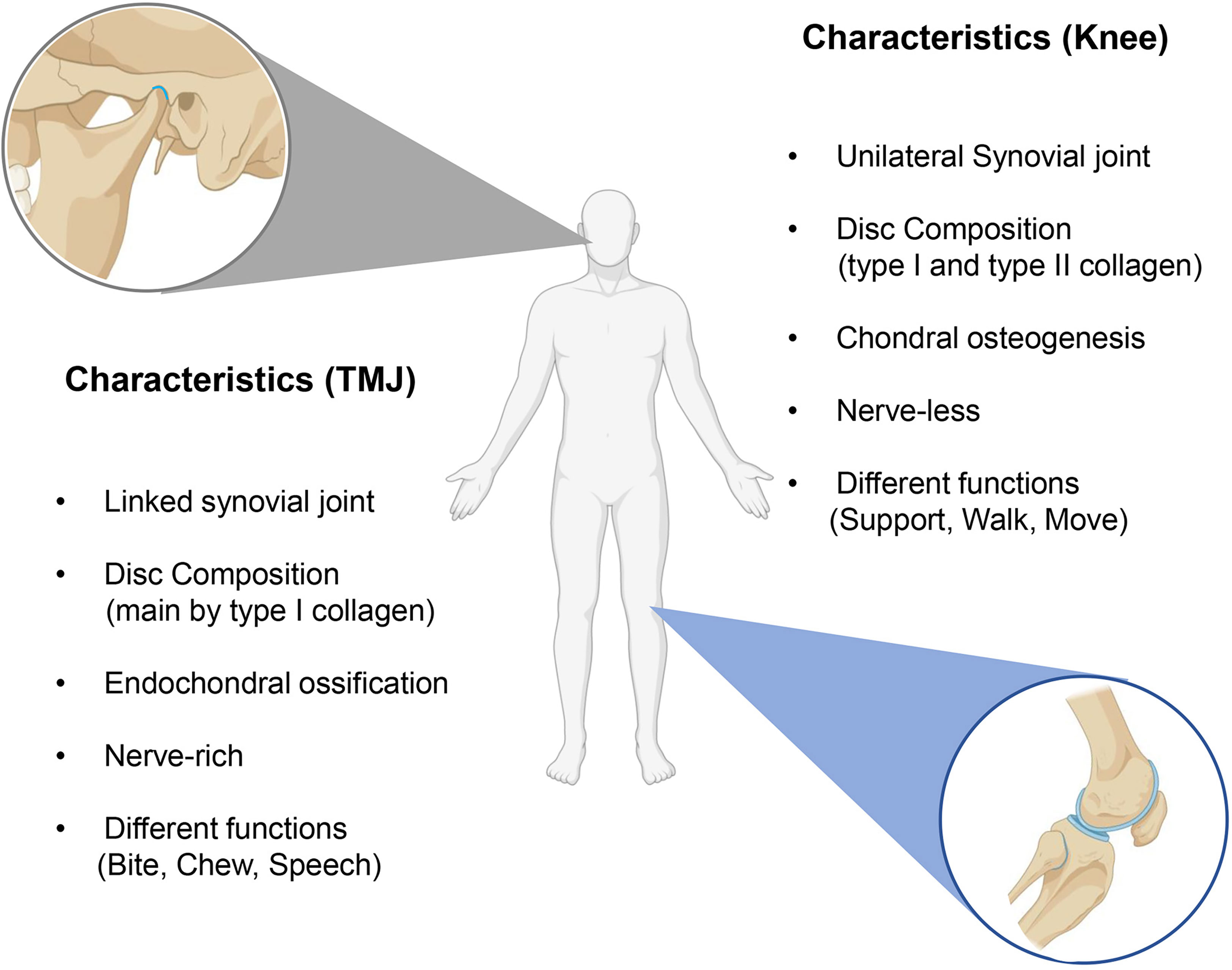
Figure 1 The differences between knee OA and TMJ OA. The main differences are the histological structure and functions.
2.2 Clinic manifestation in TMJ OA and peripheral joint-OA
OA is a common chronic degenerative joint disease worldwide, which is believed to be the fourth top cause of disability worldwide (22). OA is usually associated with genetics, trauma, old age, obesity, mechanical stress, mental elements, and other factors. However, the pathogenesis of OA is still unclear (47, 48). Typical clinical symptoms of knee OA are often joint pain and limitation of movement. Patients suffering from knee OA usually feel pain and transient morning stiffness at the early stage. With the progress of OA, a more severe lesion can be found, including subchondral bone cysts, bone marrow lesions, and osteophytes. These changes can be diagnosed by Cone Beam Computed Tomography (CBCT) and Magnetic Resonance Image (MRI) with osteophytes, bone marrow lesions, and meniscal tears (49). Despite similar joint symptoms, mental and biopsychosocial symptoms are also crucial in TMJ OA. TMJ OA presents hyperalgesia and neurological symptoms, including tinnitus, headache, and psychological disabilities (50). In addition, CBCT is relatively mild and often shows blurred and incomplete condylar cortex, localized bone resorption defects, bone redundancy formation, subchondral sclerosis, or cystic bone changes (51). The current goals for treating knee and TMJ OA are consistent, include: reducing pain in the joint area, ameliorating joint function, and slowing the progression of OA. Most patients are treated clinically by a combination of conservative and surgical treatment, which requires a personalized treatment plan that considers the patient’s situation, such as age and severity of the disease (52). In addition to traditional treatments, such as physical therapy, oral medications, intra-articular injections, minimally invasive arthroscopic surgery, and surgical operation, many emerging biological therapeutic approaches are currently under study, such as stem cell therapy, platelet-rich plasma, stromal vascular fraction, and EVs (53–56).
2.3 Animal models of OA and TMJ OA
OA is a multifactorial disease, mainly including aging, obesity, anatomical factors, mechanical loading, and genetic factors (Figure 2A). Despite all the above elements contributing to TMJ OA. Occlusion elements, mental and biopsychosocial factors also play an important role in the pathogenesis of TMJ OA (22, 50) (Figure 2B). Based on these risk factors, OA animal models are usually divided into induced, naturally occurring, and genetically modified models, while the knee is the most used joint for the animal OA model. Similar invasive approaches are suitable for both knee and TMJ, including surgical induction and chemical injection (monosodium iodoacetate, papain, collagenase). In comparison, the surgical methods to induce knee and TMJ OA are distinguished based on different anatomical structures (57). The differences between knee and TMJ OA models mainly display in non-invasive methods.
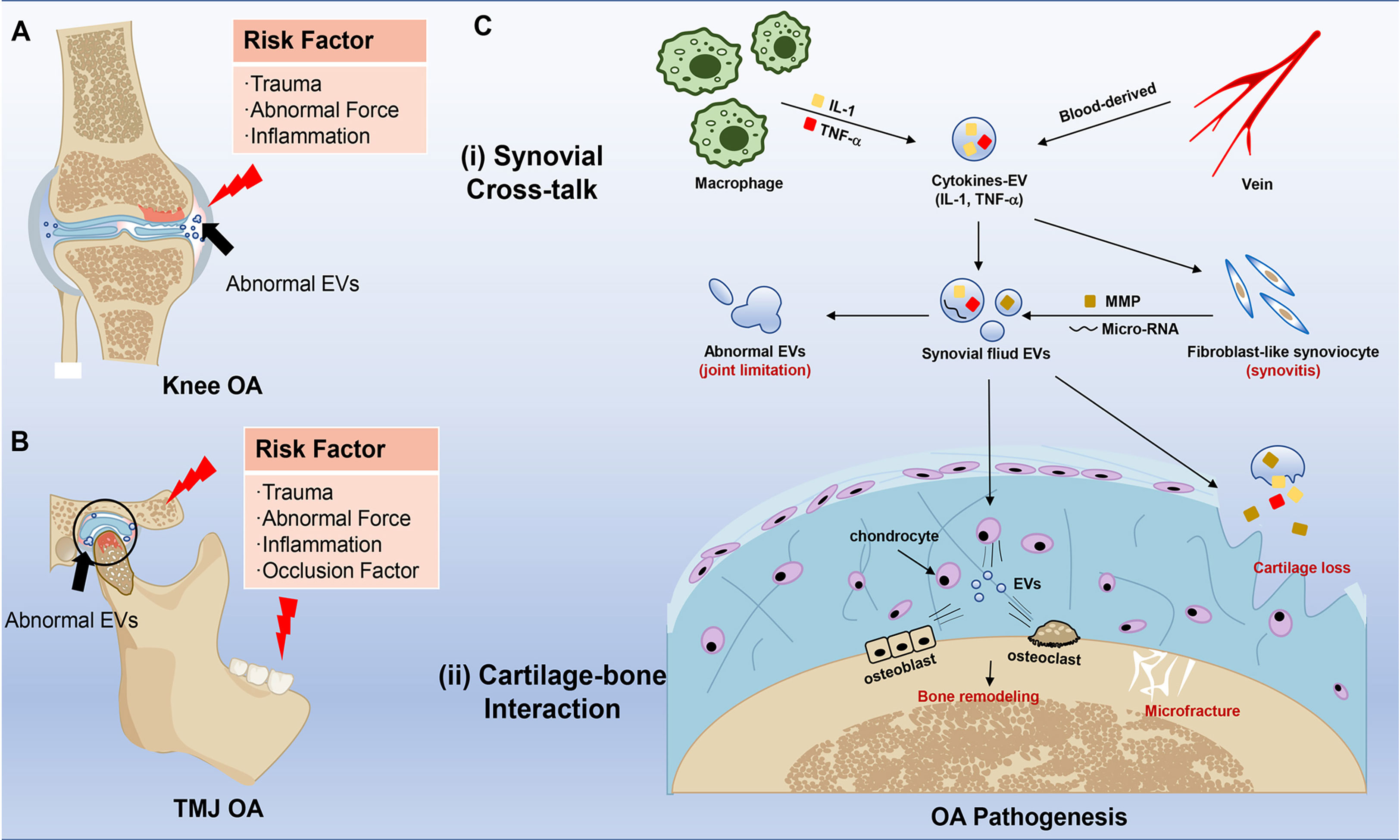
Figure 2 Risk factors and effects of EVs in knee OA and TMJ OA pathogenesis. (A) Risk factors in knee OA. The red pattern means inflammation and bone destruction, and the blue pattern means healthy joint with uniform EVs. The black arrow points to abnormal EVs in the joint. (B) Risk factors in TMJ OA. The red pattern means inflammation, and the black arrow points to abnormal EVs. (C) Schematic representation of EVs in the pathogenesis of OA. The red typeface stands for clinical change in OA. The major effects of EVs are mainly divided into two sites, including (i) Synovial Cross-talk and (ii) Cartilage-bone Interaction. The two sites also communicate with EVs.
Although the high-fat diet model and mechanical loading can apply to both knee and TMJ, more specific methods are required for TMJ OA (58, 59), including but not limited to disordered bite, excessive mouth opening, and soft food induction (58, 60). Intriguingly, the sleep deprivation model can be used to reduplicate the model of TMJ OA, which further explains the relationship between mental factors and TMJ OA (61). In addition, naturally occurring and genetically modified models are also used to study the progression of OA to simulate aging and genetic factors, which have been reviewed in detail (57). OA is a multi-factor process, and most animal models are single-factor models. It reminds us that choosing the appropriate animal model needs strict consideration according to the purpose of the study.
3 The role of EV in the pathogenesis of OA and TMJ OA
The pathogenesis of OA is complicated and diversified. It is not only a single disease, but a common final stage of joint failure contacted with body status, environmental impact, and triggered by other risk factors (62–64). In current views, OA is a degenerative disease characterized by the destruction of cartilage, synovial inflammation, and bone remodeling increase (64–66). These changes can be observed in the early stage of OA (49). OA usually needs several years to develop, meaning this disease needs long-term pathological stimulus and progresses to a worsened state.
EVs distribute in humor and tissues, deliver bioactive substances, and participate in cellular activity, which plays a vital role in the whole progression of OA. On the one hand, the serum-derived EVs carried bioactive substances like IL-1β which activated fibroblast-like synoviocytes (FLS) (67), and then these EV cargos can further raise monocytes and macrophages, which usually barely exist in normal synovial tissue. Then a low-grade inflammation can be continuous in synovial fluid and tissue in the whole stage of OA (68). On the other hand, synovitis is closely related to cartilage destruction (69, 70). Activated FLS can secret more EVs carrying inflammatory factors, micro-RNA, and matrix metalloproteinases (MMPs) to induce the apoptosis of chondrocytes and destroy the extracellular matrix (ECM) (71–73) (Figure 2C). So, it is essential to understand EVs’ function in OA seriously.
3.1 Inflammation and cytokines
OA has been described as an inflammatory disease for a long time. Many individuals with OA have joint inflammation symptoms like stiffness and pain. In addition, low-grade inflammation can be observed in patients during the whole progression of OA (74). The previous study showed that OA patients have a high level of inflammatory plasma proteins such as albumin and acid glycoproteins in their blood and synovial fluid (75). Recent research showed that other inflammatory factors also maintain a high concentration in OA synovial fluid, including complement components and cytokines (IL-1β, TNF, IL-6, IL-8) (76–79). Furthermore, these inflammatory mediators can be produced or overproduced by chondrocytes and synovial cells in OA (78). These evidences showed a close relationship between inflammation and OA.
Undoubtedly, inflammation factors are crucial in OA progression. These cytokines possess little organizational penetration and stability, so EVs are needed for bioactive cytokines carry to penetrate into deep cells or tissues (34, 80, 81). As a cell secretory mediator, EV has been demonstrated to contain many cytokines, miRNA, and other bioactive substances. EVs can be endocytosed by chondrocytes, FLS, and macrophages which are the main cells in the joint cavity microenvironment (82–84). These biological characteristics are the basis of EV to be effective. In knee OA, a mass of EVs protein cargoes has been identified, including inflammatory cytokines (for example, IL-1, IL-6, and TNF-α), immunoglobulin, complement component, fibrinogen, apolipoprotein and transforming growth factor β (35). EVs derived from synovial fluid of severe knee OA contained higher levels of numerous cytokines (85). Among all the cargoes of EVs in OA, two major players, IL-1β and TNF-α, can induce cartilage destruction and inflammation (71). It was well known that IL-1β and TNF-α increased in synovial fluid, synovial tissue, and cartilage of OA patients (86). On the condition of OA, the plasma EVs can carry a mass of TNF-α to participate in OA progression (87). Similarly, the EVs in OA patients’ synovial fluid also carry many inflammatory cytokines, including IL-1β and TNF-α (88). Subsequently, FLS strongly expressed IL-6, IL-8, and MMP, which can directly destroy the extracellular matrix stimulated by activated or apoptotic T cells and macrophages EV (89). This process demonstrated that the EVs from arthritis patients’ synovial fluid could further induce the FLS, secreting inflammatory cytokines and chemokines (90). FLS and immunocyte-derived EVs formed a high-concentration EV microenvironment in synovial fluid. These EVs can further be endocytosed by chondrocytes, which trigger chondrocytes to produce more inflammatory cytokines and chemokines (33). The other experiments demonstrated that the healthy chondrocytes treated with OA-derived EVs displayed elevated expression of inflammatory genes (88). OA is the result of the cascade reaction of inflammation. Immunocytes, FLS, and chondrocytes can be activated by EVs with different components and finally induce the production of MMP and destruction of ECM. Overall, the progression of OA in different joints is similar. The changes in knee joint OA can also be detected in TMJ OA (91–94). More and more evidence prove the relation between OA histological changes and EVs. However, the mechanism between the biological changes of EVs and synovial inflammation and cartilage loss is still unknown.
3.2 MicroRNA
People have realized the effect of miRNA on OA progression in recent years. miRNAs are a family of approximately 21-nucleotide-long RNAs that can regulate genetic expression by combining the 3’-UTR (95, 96). MicroRNA gets involved in all the known cellular activity and widely participates in disease progression, including OA (95). It has been demonstrated that miRNA differential expression is the characteristic of OA and EV-microRNA is highly related to OA progression (35, 49, 88). Compared with healthy people, the EVs from synovial fluid of the OA knee joint showed a differential microRNA pattern (88, 97). Recent research showed that 142 microRNAs were differentially expressed between damaged or non-damaged articular cartilage in peripheral joint OA patients (hip and knee) (98). Moreover, researchers collected the human synovial fibroblasts from healthy knees to verify in vitro. Likewise, approximately 340 miRNAs upregulated in FLS treated by IL-1β; meanwhile, only 11 miRNA increased in FLS-derived EVs (99).
The synovial fluid-derived EV-miRNA can be endocytosed by chondrocytes and stimulate inflammation in cartilage (88). Since EV-miRNA penetrated into cells, they can directly bind to specific mRNA or proteins to regulate cellular behaviors. Present research mainly focused on knee OA. For example, miR-181a-5p is a critical mediator involved in the cartilage destruction by promoting inflammatory, catabolic, and cell death activity (100, 101). Notably, not all the OA patients have the same EV-miRNA changes; EV-miRNA in OA displays a gender-specific pattern (88). Only one miRNA (miR-504-3p) were upregulated in both genders, which may promote cell apoptosis (88, 102). The other downregulated miRNAs were associated with cell adhesion molecules, whereas the upregulated miRNAs were related to biotin metabolism signaling. The female-specific EV-miRNAs most participate in estrogen pathway (35), which may explain the high incidence rate of OA in female (22).
TMJ OA also has similar mechanisms that microRNA can anchor target pathways to regulate OA progression, including Wnt, Smad/TGF-β, and PTEN (103–106). Like knee OA, TMJ OA also displays a high incidence rate in females, which may attribute to EV-miRNAs gender-specific expression. However, TMJ OA is different from knee joint OA, such as gender incidence rate and symptoms, and there is little research to detect the difference in TMJ OA.
3.3 Mechanical stimulation
It is undisputed that the mechanical factor is the key to OA progression. The joint is the force-bearing structure of our bodies. Owing to the existence of synovial fluid and disc, bone can move with very low friction. Even so, the response of different joints to force-loading is usually discrepant. For example, the knee usually bears a relatively large force, four times more than body weight in jogging, while TMJ can undergo a body weight when people bite (107, 108). Different function determines different mechanical adaptation, and overuse may cause joint structural changes. So, articular inflammation mainly concentrates on the functional joints like the knee, hip, hand, and TMJ (49). With further validation, we gradually realize that the mechanical factors are not just the physical “erosion” from the previous studies (109). It is a complicated system composed of mechanically sensitive pathways and functional proteins. In the early stage of OA, the microfracture began to emerge, and the cartilage was the first to appear in the microarchitectural changes with chondrocyte mitochondrial dysfunction (110, 111). The mechanical loading may further impact the chondrocyte, which secreted EVs containing mechanically sensitive substances like miR-221-3p to regulate cell communication in bone remodeling (112). However, the impact of mechanical is usually self-limiting. If we intervene timely, the cartilage dysfunction will recover and rebalance.
In the process of force loading, overuse or abnormal loading can cause cartilage loss and subchondral bone marrow lesions with meniscus degeneration or severe tears. Unlike the knee joint, TMJ are two linkage joints having a more precise mechanical system. Recent studies showed that increased loading force led to a thicker calcified cartilaginous layer of TMJ and caused osteochondral interface stiffness with mild disc displacement or perforation (110, 113). Although there is a difference in symptoms between the two joints, the initial mechanical response systems are both chondrocytes and ECM. EVs are one of the most important components in ECM and mediate cell-to-cell interaction in this system (114). In fact, it can detect a mass of apoVs in the ECM collected from knee OA (115, 116). ApoVs were also observed in the apoptotic chondrocytes of TMJ OA (117). Except for apoVs, the other EVs are mainly from articular cartilage that contains over 1700 bioactive substances, including type II transglutaminase, COL II, aggrecan, βig-H3 (TGFβ-induced protein), and GAPDH (80, 118). These EVs form the ECM and regulate the communication between cartilage and bones (119). In the process of knee OA, as the force loading, ECM-derived EVs respond to mechanical effects and are released by chondrocytes, and then interact with the surrounding cells by activating bone morphogenetic proteins (BMPs) and transforming growth factor-β (TGFβ). These changes will further activate downstream pathways, including Wnt and MAPK signaling pathways (109). Finally, these ECM-derived EVs can take away the ECM components, which contains lots of cytokines and cathepsin, such as MMP-2, causing cartilage loss and bone lesions (120). In addition, not only the cargos but EVs’ mechanical properties also impact the progression of OA. Studies showed that EVs in OA become inhomogeneous and soft (121), which indicated that mechanical loading may change the mechanical properties of EVs derived from cartilage and bone.
The TMJ OA showed a similar pathological process with knee OA by abnormal mechanical stress stimulation. These EVs and changes can also be found in TMJ OA, including TGFβ, BMPs, Wnt, and FGF pathways (60, 122). However, there are still some differences between TMJ OA and knee OA. Considering they have different origins and growth patterns, the expressions of the same genes also cause different endings. For example, small leucine-rich proteoglycans (biglycan/fibromodulin) are one of the ECM components, participating in both knee OA and TMJ OA (123). Intriguingly, TMJ OA was observed four months later than knee OA in biglycan/fibromodulin double-deficient mice (124, 125). Although we have reviewed current research, the specific pathways of knee and TMJ OA are still unclear. Overall, more direct evidence should be presented in mechanical-induced OA.
3.4 Genetic factor
OA is regarded as an idiopathic disease; however, genetic factor is also an important reason for OA development. OA shows the characteristic of familial aggregation, and the heritability rate of OA is around 35%-65% depending on different joints (126, 127). OA is a polygenic disease that cannot attribute to any single gene. Gene detection was done to analyze the susceptible gene including growth differentiation factor 5 (GDF5) and human leukocyte antigen (HLA) class II/III locus (126, 128). A European genome-wide association study (GWAS) confirmed two genes were related to knee pain in 171561 people, which were close to GDF5 and COL27A1 (129).
Compared with the early industrial and prehistoric eras, the OA prevalence rate of modern people has doubled (130). These genes have so low odds ratios that they are less responsible for OA. However, the paradoxical evidences seem unreasonable to explain the familial aggregation and mother-daughter inheritance patterns in OA. Recent research showed that mothers suffering from OA were more likely to pass it on to their offspring compared with fathers (131). Mother-to-child transmission patterns contain mother-to-embryo, which mainly depends on EVs except for gene recombination. Since mothers are inflammation sufferers, EVs can carry these inflammatory cytokines (IL-1β, TNF-α) and environmental factors, pass through the placental barrier into the fetus (132). These studies added EV regulatory factors to explain why OA presents as a matrilinear inheritance with a low-degree effect of susceptible genes. However, further studies are needed to elaborate.
4 The role of EVs in the treatment of peripheral OA and TMJ OA
The treatment of OA is always challenging. Currently, conservative and surgical treatments are used to treat OA, but there is no golden therapy for OA. The mild OA lesions can be treated with drugs or splints, while the severe ones may need surgical therapies. In 1957, the first hemiarthroplasty knee device was designed by McKeever, while Christensen designed a fossa-eminence prosthesis for TMJ hemiarthroplasty six years later (133, 134). Till now, knee arthroplasty and hip arthroplasty have been well developed, but TMJ arthroplasty rarely achieves satisfactory results (135, 136). With the progression of OA research, people have gradually realized the importance of biological factors in OA treatments. In 1985, IL-1 receptor antagonist (IL-1Ra) was first reported to find in human macrophages and synovial macrophages in OA patients (137). Autologous conditioned serum (ACS) therapy, mainly working through IL-1Ra, was studied in the mid-1990s (138). The preparation of autoserum is a mature technological process, and the autologous platelets can produce IL-1Ra and various kinds of cytokines in cell degranulation (139). People realize that IL-1Ra was not the only one in OA, and platelet-rich plasma (PRP) was studied subsequently (140).
Because of their preparation process, ACS and PRP retain lots of blood-derived EVs, which showed therapeutic effects in OA (141, 142). It was reported that blood-derived EVs partook the substance exchange and cell communication (141). Blood-derived EVs can inhibit inflammation and elicit chondroprotective gene expression (142). In addition, a recent study showed that blood-derived CD34+ EVs were the potential therapeutic targets for OA. Blood-derived CD34+ EVs were the only subpopulation that significantly correlated between plasma and synovial fluids containing lots of functional mitochondria and little pathogenic cytokines such as TNF-α and IFN-γ (143, 144). EVs from autologous blood-derived products may be the core components of treating OA. Although ACS and PRP showed significant effects in animal models, the complexity of autologous blood-derived products caused controversial clinical efficacy (145, 146). More biotherapies have been studied, including MSC therapy and EV therapy (34, 147). Compared with traditional stem cell therapy, EV administration does not have the risks of allogeneic and xenogeneic immunological rejection and malignant transformation, so it has opened a new era of stem cell therapy.
4.1 Stem cell therapy for OA
With the development of regenerative medicine, stem cell therapy was gradually studied. OA is a degenerative disease, so researchers mainly focused on tissue regeneration and immunoregulation, and stem cell meets requirements. MSCs have the characteristics of self-renew, multiple differentiation, and immune regulation, which contribute to tissue repair of OA (148–151). The clinical trial also showed that stem cell therapy could effectively treat knee OA (152). A recent multicenter randomized controlled clinical trial (phase I/II) demonstrated that bone marrow MSC (BMMSC) therapy could safely alleviate the symptoms of knee OA (153). In contrast, the successive clinical trial showed no significant difference between PRP therapy (154). In the treatment of TMJ OA, stem cells from different sources showed therapeutic effects and promoted condylar cartilage regeneration (24, 155–158). In addition, up to 2,021 randomized clinical trials of stem cell therapy showed a statistically significant superiority over hyaluronan in pain relief and mandible mobility (159). However, stem cell therapy has disadvantages, including immunogenicity and unstable phenotype, so it reminds us whether there is an alternative approach in biotherapy which can be more effective for OA (27).
4.2 EV treatment for peripheral joint OA
Compared to cells, EVs show lower immunogenicity. Therefore, it is safer to apply EVs in OA treatment than cells (160). EVs from a variety of sources, including synovial MSCs (SMSCs) (161–163), adipose-derived MSCs (ADMSCs) (164, 165), BMMSC (166), and human umbilical cord MSCs (hUMSCs) (167, 168), has been proved to be beneficial for peripheral joint OA treatment.
Perturbed balance of the local immune system is the key factor that causes clinical symptoms and organic damages in OA. During knee OA development, immunological cells exert proinflammatory factors, such as IL-1, IL-6, IL-8, and MMP-3, thereby inducing synovial inflammation and cartilage degradation (169). MSC-EVs have immunomodulatory effects in peripheral joint OA treatment. MSCs-EVs generate large amounts of anti-inflammatory cytokines, such as IL-10 and TGF-β1, and simultaneously suppress the production of the proinflammatory factors IL-1, IL-6, TNF-α, and IL-12. Moreover, MSC-EVs inhibit macrophage activation and induce the proinflammatory M1 phenotype to convert to the anti-inflammatory M2 phenotype. Together, MSC-EVs decrease local inflammatory reactions in knee OA (170).
Inflammatory response causes cartilage degeneration in peripheral joint OA, including cell death, matrix degradation, and finally, a loss of structure and function (160, 171). MSC-EVs prevent chondrocyte apoptosis (170) and facilitate cell migration and proliferation in peripheral joint OA (172). By mediating the expression of fibroblast growth factor (FGF)-2, survivin, and Bcl2/Bax, EVs directly promote chondrocyte proliferation or eliminate the inhibitory effect of pro-inflammatory factors like TNF-α and IL-1β (167, 170, 173, 174). Besides, MSCs-EVs can also control chondrocyte proliferation and migration by releasing miRNAs (164, 175, 176).
MSC-EVs induce the expression of matrix protein, while inhibit the expression of matrix-degrading enzymes, thereby promote ECM synthesis. MSC-EVs can reduce the expression of MMP-1, MMP-3, MMP-13, and ADAMTS-5 and increase COL II production (165). Previous research has elucidated that MSC-EVs regulate the expression of cartilage formation-related genes such as aggrecan, SRY box gene-9 (SOX9), COL9A1, COL2A1, and cartilage oligomeric matrix protein (COMP) while decreasing the expression of COL10A1, Runt-related transcription factor 2 (Runx2), and MMP-13, with their cargos, including miRNAs and proteins (177). Besides the natural EVs, there are emerging types of engineered EVs. Engineered EVs are designed for targeted therapy or controlled release and have better therapeutic properties, which have drawn increasing attention of researchers (34, 178, 179) (Figure 3).
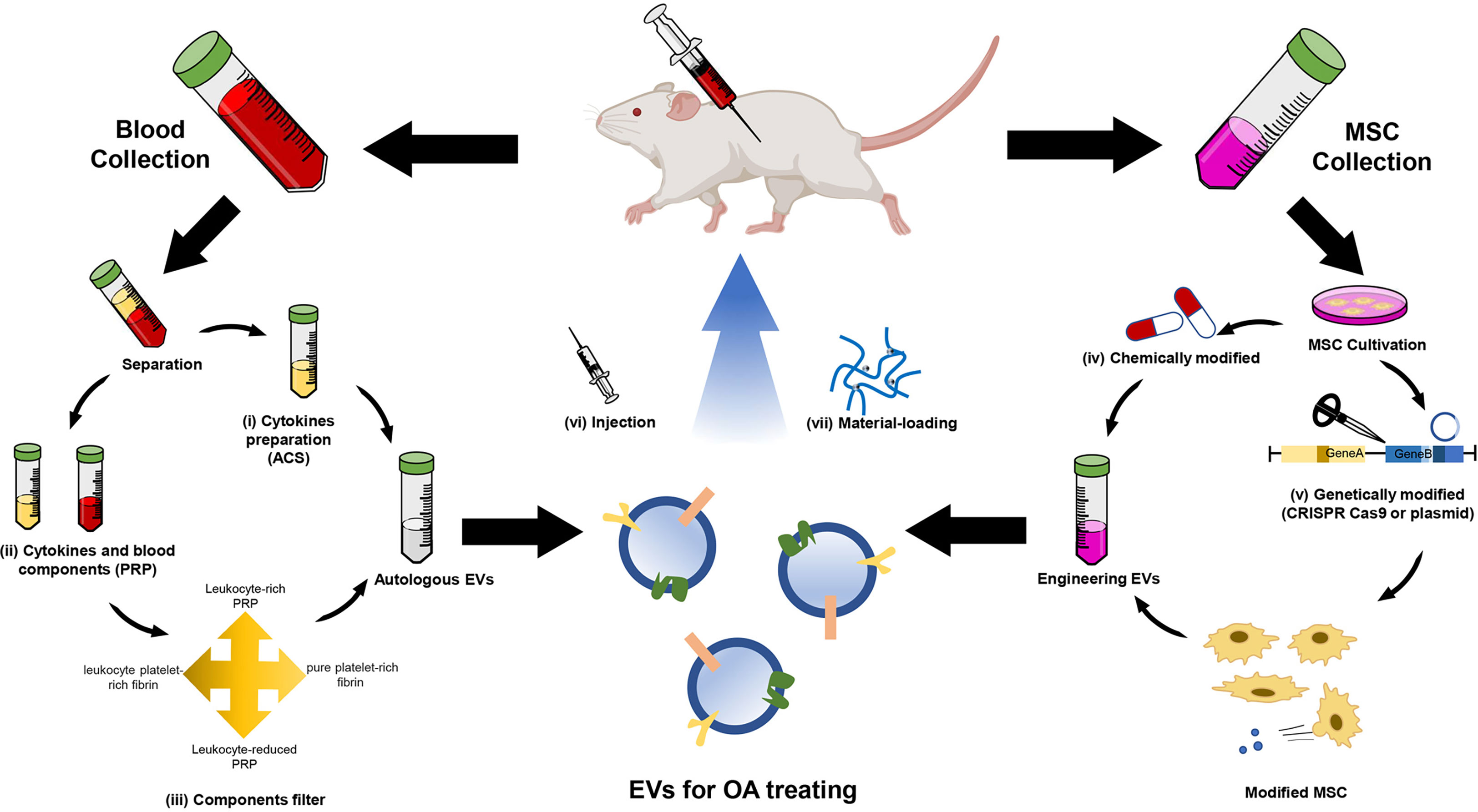
Figure 3 Schematic illustration of EV therapies for OA. EV therapy usually collects EVs from autologous EVs and engineering EVs. The autologous EVs are mainly derived from (i) Autologous conditioned serum (ACS) and (ii) platelet-rich plasma (PRP). The latter needs (iii) components filter and then produces four types of EV suspension. The engineering EVs are mainly derived from MSCs. Two strategies are used to modify the MSC-derived EVs, including (iv) chemical and (v) genetic modification. The modified MSC-secreted specific EVs contain miRNA and proteins in OA treatment. Autologous or engineering EVs are usually delivered by (vi) injection and (vii) material-loading.
4.3 EV treatment for TMJ OA
Studies on TMJ OA are relatively stagnant compared with knee OA (41). To date, few studies have demonstrated the effect of EVs on TMJ OA treatment. Based on current understanding, the mechanisms of EV therapeutic effect on TMJ OA are rather similar to OA. BMMSC-derived EVs could induce cartilage reconstruction in TMJ OA via the autotaxin–YAP signaling axis in chondrocytes (180). MSC-EVs activate AKT and ERK signaling in chondrocytes and then enhance the proliferation and migration of chondrocytes. The EVs can also activate AMP-activated protein kinase (AMPK) signaling, which contributes to restoring and maintaining chondrocyte matrix homeostasis (181). Moreover, MSC-EVs promote s-GAG synthesis and COL II protein expression, and suppress NO and MMP-13 production, to restore stromal homeostasis in a TMJ-OA, enhancing cartilage regeneration in chondrocytes (182).
4.4 Limitations of EV therapy in OA
EV therapy in OA still has limitations, including but not limited to the unclear mechanism, targeting and stability, and EV production. Although the engineered EVs exhibit targetable and stable properties, it is still unclear whether they may cause other problems (183). Moreover, there are difficulties in the preparation and preservation of EVs. The contamination from the EV separation process reduces the purity of EVs, and these confounding factors hamper EV usage in clinical practice (184, 185). Solving these problems is challenging, and more efforts should be devoted to EV research.
5 Conclusion and perspective
In summary, researchers have gradually realized the crucial function of EVs in OA progression. The effect of EV in OA is multiplex. EV-carried bioactive substances mediate cell-to-cell communication, and so does inflammation. Inflammation is passed and aggravated by EVs. Differed from Rheumatoid arthritis, which has a heavier inflammatory burden stimulated by immunocomplex than OA. EVs in OA cannot cause much stronger inflammatory storms, which may be attributed to their relatively low immunogenicity. The changes in EVs’ mechanical properties may limit joint movement. Meanwhile, the EV-carried bioactive substance may determine the fate of FLS and chondrocytes. This evidence suggests that OA is not only an inflammatory disease but also an EV-related disease.
There is no specific therapy for OA so reducing the risk factor is crucial to OA treatments. Although the joint replacement therapy succeeded in knee and hip, it was mainly for later period OA patients, and the treatment in TMJ failed. EV therapies may be a promising treatment for OA in peripheral joints and TMJ because of their low immunogenicity, low cytotoxicity, and long-term stability characteristics. Recently, the EVs from modified cells or loaded with drugs showed therapeutic effects in OA (182, 186, 187), which are attractive for this field. There are three forms of EVs, and the recent research of EV therapy mainly focused on exosomes, which showed therapeutic effects in OA treatment (188). Compared with exosomes, apoVs can be easier to obtain from tissues. It also contains more bioactive substances suitable for immunomodulation and regeneration (189, 190). Although there is currently no study in the treatment of OA with apoVs, considering its biological characteristics, we believe apoVs may be a potential candidate for OA therapy. However, the side effects of EVs are still unclear, which impels us to conduct a long-term safety assessment before the clinical transformation.
Author contributions
BY and XL wrote the initial draft. CF, WC, BM and YQ contributed to the manuscript revision and figure preparation. XK and QZ provided their conception, design and comments on the manuscript and approved the final version of the manuscript. All authors contributed to the article and approved the submitted version.
Funding
This work was supported by grants from the National Natural Science Foundation of China (82170924), the National Key R&D Program of China (2021YFA1100600), the Pearl River Talent Recruitment Program (2019ZT08Y485).
Conflict of interest
The authors declare that the research was conducted in the absence of any commercial or financial relationships that could be construed as a potential conflict of interest.
Publisher’s note
All claims expressed in this article are solely those of the authors and do not necessarily represent those of their affiliated organizations, or those of the publisher, the editors and the reviewers. Any product that may be evaluated in this article, or claim that may be made by its manufacturer, is not guaranteed or endorsed by the publisher.
References
1. Fais S, O’Driscoll L, Borras FE, Buzas E, Camussi G, Cappello F, et al. Evidence-based clinical use of nanoscale extracellular vesicles in nanomedicine. ACS Nano (2016) 10(4):3886–99. doi: 10.1021/acsnano.5b08015
2. Zaborowski MP, Balaj L, Breakefield XO, Lai CP. Extracellular vesicles: Composition, biological relevance, and methods of study. Bioscience. (2015) 65(8):783–97. doi: 10.1093/biosci/biv084
3. Colombo M, Raposo G, Thery C. Biogenesis, secretion, and intercellular interactions of exosomes and other extracellular vesicles. Annu Rev Cell Dev Biol (2014) 30:255–89. doi: 10.1146/annurev-cellbio-101512-122326
4. Simons M, Raposo G. Exosomes–vesicular carriers for intercellular communication. Curr Opin Cell Biol (2009) 21(4):575–81. doi: 10.1016/j.ceb.2009.03.007
5. Ludwig AK, Giebel B. Exosomes: small vesicles participating in intercellular communication. Int J Biochem Cell Biol (2012) 44(1):11–5. doi: 10.1016/j.biocel.2011.10.005
6. Shao H, Im H, Castro CM, Breakefield X, Weissleder R, Lee H. New technologies for analysis of extracellular vesicles. Chem Rev (2018) 118(4):1917–50. doi: 10.1021/acs.chemrev.7b00534
7. De Jong OG, Van Balkom BW, Schiffelers RM, Bouten CV, Verhaar MC. Extracellular vesicles: potential roles in regenerative medicine. Front Immunol (2014) 5:608. doi: 10.3389/fimmu.2014.00608
8. Piper RC, Katzmann DJ. Biogenesis and function of multivesicular bodies. Annu Rev Cell Dev Biol (2007) 23:519–47. doi: 10.1146/annurev.cellbio.23.090506.123319
9. Kowal J, Tkach M, Thery C. Biogenesis and secretion of exosomes. Curr Opin Cell Biol (2014) 29:116–25. doi: 10.1016/j.ceb.2014.05.004
10. Hugel B, Martinez MC, Kunzelmann C, Freyssinet JM. Membrane microparticles: two sides of the coin. Physiology (2005) 20:22–7. doi: 10.1152/physiol.00029.2004
11. Zheng C, Sui B, Zhang X, Hu J, Chen J, Liu J, et al. Apoptotic vesicles restore liver macrophage homeostasis to counteract type 2 diabetes. J Extracell Vesicles (2021) 10(7):e12109. doi: 10.1002/jev2.12109
12. Poon IKH, Parkes MAF, Jiang L, Atkin-Smith GK, Tixeira R, Gregory CD, et al. Moving beyond size and phosphatidylserine exposure: evidence for a diversity of apoptotic cell-derived extracellular vesicles in vitro. J Extracell Vesicles (2019) 8(1):1608786. doi: 10.1080/20013078.2019.1608786
13. Tang H, Luo H, Zhang Z, Yang D. Mesenchymal stem cell-derived apoptotic bodies: Biological functions and therapeutic potential. Cells. (2022) 11(23):3879. doi: 10.3390/cells11233879
14. Kalra H, Simpson RJ, Ji H, Aikawa E, Altevogt P, Askenase P, et al. Vesiclepedia: a compendium for extracellular vesicles with continuous community annotation. PloS Biol (2012) 10(12):e1001450. doi: 10.1371/journal.pbio.1001450
15. Elmore S. Apoptosis: A review of programmed cell death. Toxicol Pathol (2007) 35(4):495–516. doi: 10.1080/01926230701320337
16. Pavlyukov MS, Yu H, Bastola S, Minata M, Shender VO, Lee Y, et al. Apoptotic cell-derived extracellular vesicles promote malignancy of glioblastoma Via intercellular transfer of splicing factors. Cancer Cell (2018) 34(1):119–35.e10. doi: 10.1016/j.ccell.2018.05.012
17. Ainola M, Porola P, Takakubo Y, Przybyla B, Kouri VP, Tolvanen TA, et al. Activation of plasmacytoid dendritic cells by apoptotic particles - mechanism for the loss of immunological tolerance in sjogren’s syndrome. Clin Exp Immunol (2018) 191(3):301–10. doi: 10.1111/cei.13077
18. Abramson SB, Attur M. Developments in the scientific understanding of osteoarthritis. Arthritis Res Ther (2009) 11(3):227. doi: 10.1186/ar2655
19. Katz JN, Arant KR, Loeser RF. Diagnosis and treatment of hip and knee osteoarthritis: A review. JAMA. (2021) 325(6):568–78. doi: 10.1001/jama.2020.22171
20. Gharbi M, Deberg M, Henrotin Y. Application for proteomic techniques in studying osteoarthritis: a review. Front Physiol (2011) 2:90. doi: 10.3389/fphys.2011.00090
21. de Souza RF, Lovato da Silva CH, Nasser M, Fedorowicz Z, Al-Muharraqi MA. Interventions for the management of temporomandibular joint osteoarthritis. Cochrane Database Syst Rev (2012) 2012(4):CD007261. doi: 10.1002/14651858.CD007261.pub2
22. Hunter DJ, Bierma-Zeinstra S. Osteoarthritis. Lancet. (2019) 393(10182):1745–59. doi: 10.1016/S0140-6736(19)30417-9
23. Yi Y, Zhou X, Xiong X, Wang J. Neuroimmune interactions in painful TMD: Mechanisms and treatment implications. J Leukoc Biol (2021) 110(3):553–63. doi: 10.1002/JLB.3MR0621-731RR
24. Zhao Y, Xie L. An update on mesenchymal stem cell-centered therapies in temporomandibular joint osteoarthritis. Stem Cells Int (2021) 2021:6619527. doi: 10.1155/2021/6619527
25. Damjanov I, Andrews PW. Teratomas produced from human pluripotent stem cells xenografted into immunodeficient mice - a histopathology atlas. Int J Dev Biol (2016) 60(10-12):337–419. doi: 10.1387/ijdb.160274id
26. Vizoso FJ, Eiro N, Cid S, Schneider J, Perez-Fernandez R. Mesenchymal stem cell secretome: Toward cell-free therapeutic strategies in regenerative medicine. Int J Mol Sci (2017) 18(9):1852. doi: 10.3390/ijms18091852
27. Meldolesi J. News about therapies of alzheimer’s disease: Extracellular vesicles from stem cells exhibit advantages compared to other treatments. Biomedicines (2022) 10(1):105. doi: 10.3390/biomedicines10010105
28. Tofino-Vian M, Guillen MI, Alcaraz MJ. Extracellular vesicles: A new therapeutic strategy for joint conditions. Biochem Pharmacol (2018) 153:134–46. doi: 10.1016/j.bcp.2018.02.004
29. Rani S, Ryan AE, Griffin MD, Ritter T. Mesenchymal stem cell-derived extracellular vesicles: Toward cell-free therapeutic applications. Mol Ther (2015) 23(5):812–23. doi: 10.1038/mt.2015.44
30. Liu Z, Zhuang Y, Fang L, Yuan C, Wang X, Lin K. Breakthrough of extracellular vesicles in pathogenesis, diagnosis and treatment of osteoarthritis. Bioact Mater (2023) 22:423–52. doi: 10.1016/j.bioactmat.2022.10.012
31. Toh WS, Lai RC, Hui JHP, Lim SK. MSC exosome as a cell-free MSC therapy for cartilage regeneration: Implications for osteoarthritis treatment. Semin Cell Dev Biol (2017) 67:56–64. doi: 10.1016/j.semcdb.2016.11.008
32. Maehara M, Toyoda E, Takahashi T, Watanabe M, Sato M. Potential of exosomes for diagnosis and treatment of joint disease: Towards a point-of-Care therapy for osteoarthritis of the knee. Int J Mol Sci (2021) 22(5):2666. doi: 10.3390/ijms22052666
33. Withrow J, Murphy C, Liu Y, Hunter M, Fulzele S, Hamrick MW. Extracellular vesicles in the pathogenesis of rheumatoid arthritis and osteoarthritis. Arthritis Res Ther (2016) 18(1):286. doi: 10.1186/s13075-016-1178-8
34. Yin B, Ni J, Witherel CE, Yang M, Burdick JA, Wen C, et al. Harnessing tissue-derived extracellular vesicles for osteoarthritis theranostics. Theranostics. (2022) 12(1):207–31. doi: 10.7150/thno.62708
35. Mustonen AM, Nieminen P. Extracellular vesicles and their potential significance in the pathogenesis and treatment of osteoarthritis. Pharmaceuticals-Base. (2021) 14(4):315. doi: 10.3390/ph14040315
36. Lin J, Wang L, Lin J, Liu Q. The role of extracellular vesicles in the pathogenesis, diagnosis, and treatment of osteoarthritis. Molecules. (2021) 26(16):4987. doi: 10.3390/molecules26164987
37. Khan IM, Redman SN, Williams R, Dowthwaite GP, Oldfield SF, Archer CW. The development of synovial joints. Curr Top Dev Biol (2007) 79:1–36. doi: 10.1016/S0070-2153(06)79001-9
38. Makris EA, Hadidi P, Athanasiou KA. The knee meniscus: structure-function, pathophysiology, current repair techniques, and prospects for regeneration. Biomaterials. (2011) 32(30):7411–31. doi: 10.1016/j.biomaterials.2011.06.037
39. Alomar X, Medrano J, Cabratosa J, Clavero JA, Lorente M, Serra I, et al. Anatomy of the temporomandibular joint. Semin Ultrasound CT MR (2007) 28(3):170–83. doi: 10.1053/j.sult.2007.02.002
40. Wilkie G, Al-Ani Z. Temporomandibular joint anatomy, function and clinical relevance. Br Dent J (2022) 233(7):539–46. doi: 10.1038/s41415-022-5082-0
41. Bielajew BJ, Donahue RP, Espinosa MG, Arzi B, Wang DA, Hatcher DC, et al. Knee orthopedics as a template for the temporomandibular joint. Cell Rep Med (2021) 2(5):100241. doi: 10.1016/j.xcrm.2021.100241
42. Edvall NK, Gunan E, Genitsaridi E, Lazar A, Mehraei G, Billing M, et al. Impact of temporomandibular joint complaints on tinnitus-related distress. Front Neurosci (2019) 13:879. doi: 10.3389/fnins.2019.00879
43. Shah HN, Jones RE, Borrelli MR, Robertson K, Salhotra A, Wan DC, et al. Craniofacial and long bone development in the context of distraction osteogenesis. Plast Reconstr Surg (2021) 147(1):54e–65e. doi: 10.1097/PRS.0000000000007451
45. Bechtold TE, Kurio N, Nah HD, Saunders C, Billings PC, Koyama E. The roles of Indian hedgehog signaling in TMJ formation. Int J Mol Sci (2019) 20(24):6300. doi: 10.3390/ijms20246300
46. Mori-Akiyama Y, Akiyama H, Rowitch DH, de Crombrugghe B. Sox9 is required for determination of the chondrogenic cell lineage in the cranial neural crest. P Natl Acad Sci USA (2003) 100(16):9360–5. doi: 10.1073/pnas.1631288100
47. Michael JW, Schluter-Brust KU, Eysel P. The epidemiology, etiology, diagnosis, and treatment of osteoarthritis of the knee. Dtsch Arztebl Int (2010) 107(9):152–62. doi: 10.3238/arztebl.2010.0152
48. Dulay GS, Cooper C, Dennison EM. Knee pain, knee injury, knee osteoarthritis & work. Best Pract Res Clin Rheumatol (2015) 29(3):454–61. doi: 10.1016/j.berh.2015.05.005
49. Martel-Pelletier J, Barr AJ, Cicuttini FM, Conaghan PG, Cooper C, Goldring MB, et al. Osteoarthritis. Nat Rev Dis Primers (2016) 2:16072. doi: 10.1038/nrdp.2016.72
50. Ohrbach R, Dworkin SF. The evolution of TMD diagnosis: Past, present, future. J Dent Res (2016) 95(10):1093–101. doi: 10.1177/0022034516653922
51. Lewis EL, Dolwick MF, Abramowicz S, Reeder SL. Contemporary imaging of the temporomandibular joint. Dent Clin North Am (2008) 52(4):875–90. doi: 10.1016/j.cden.2008.06.001
52. Tanaka E, Detamore MS, Mercuri LG. Degenerative disorders of the temporomandibular joint: Etiology, diagnosis, and treatment. J Dent Res (2008) 87(4):296–307. doi: 10.1177/154405910808700406
53. Yang WT, Ke CY, Yeh KT, Huang SG, Lin ZY, Wu WT, et al. Stromal-vascular fraction and adipose-derived stem cell therapies improve cartilage regeneration in osteoarthritis-induced rats. Sci Rep (2022) 12(1):2828. doi: 10.1038/s41598-022-06892-3
54. Shahid M, Kundra R. Platelet-rich plasma (PRP) for knee disorders. EFORT Open Rev (2017) 2(1):28–34. doi: 10.1302/2058-5241.2.160004
55. Pelaez P, Damia E, Torres-Torrillas M, Chicharro D, Cuervo B, Miguel L, et al. Cell and cell free therapies in osteoarthritis. Biomedicines (2021) 9(11):1726. doi: 10.3390/biomedicines9111726
56. Li JJ, Hosseini-Beheshti E, Grau GE, Zreiqat H, Little CB. Stem cell-derived extracellular vesicles for treating joint injury and osteoarthritis. Nanomaterials (2019) 9(2):261. doi: 10.3390/nano9020261
57. McCoy AM. Animal models of osteoarthritis: Comparisons and key considerations. Vet Pathol (2015) 52(5):803–18. doi: 10.1177/0300985815588611
58. Bhatti FUR, Karydis A, Lee BS, Deguchi T, Kim D, Cho HS. Understanding early-stage posttraumatic osteoarthritis for future prospects of diagnosis: from knee to temporomandibular joint. Curr Osteoporos Rep (2021) 19(2):166–74. doi: 10.1007/s11914-021-00661-3
59. Johnson VL, Hunter DJ. The epidemiology of osteoarthritis. Best Pract Res Clin Rheumatol (2014) 28(1):5–15. doi: 10.1016/j.berh.2014.01.004
60. Liu Q, Yang H, Zhang M, Zhang J, Lu L, Yu S, et al. Initiation and progression of dental-stimulated temporomandibular joints osteoarthritis. Osteoarthritis Cartilage (2021) 29(5):633–42. doi: 10.1016/j.joca.2020.12.016
61. Chen G, Zhao H, Ma S, Chen L, Wu G, Zhu Y, et al. Circadian rhythm protein Bmal1 modulates cartilage gene expression in temporomandibular joint osteoarthritis via the MAPK/ERK pathway. Front Pharmacol (2020) 11:527744. doi: 10.3389/fphar.2020.527744
62. Corti MC, Rigon C. Epidemiology of osteoarthritis: prevalence, risk factors and functional impact. Aging Clin Exp Res (2003) 15(5):359–63. doi: 10.1007/BF03327356
63. Felson DT. Clinical practice. osteoarthritis of the knee. N Engl J Med (2006) 354(8):841–8. doi: 10.1056/NEJMcp051726
64. Rai MF, Sandell LJ. Inflammatory mediators: Tracing links between obesity and osteoarthritis. Crit Rev Eukar Gene (2011) 21(2):131–42. doi: 10.1615/CritRevEukarGeneExpr.v21.i2.30
65. Goldring SR. Alterations in periarticular bone and cross talk between subchondral bone and articular cartilage in osteoarthritis. Ther Adv Musculoskelet Dis (2012) 4(4):249–58. doi: 10.1177/1759720X12437353
66. Oehler S, Neureiter D, Meyer-Scholten C, Aigner T. Subtyping of osteoarthritic synoviopathy. Clin Exp Rheumatol (2002) 20(5):633–40.
67. Zeng G, Deng G, Xiao S, Li F. Fibroblast-like synoviocytes-derived exosomal PCGEM1 accelerates IL-1beta-induced apoptosis and cartilage matrix degradation by miR-142-5p/RUNX2 in chondrocytes. Immunol Invest (2022) 51(5):1284–301. doi: 10.1080/08820139.2021.1936010
68. Haseeb A, Haqqi TM. Immunopathogenesis of osteoarthritis. Clin Immunol (2013) 146(3):185–96. doi: 10.1016/j.clim.2012.12.011
69. Ayral X, Pickering EH, Woodworth TG, Mackillop N, Dougados M. Synovitis: a potential predictive factor of structural progression of medial tibiofemoral knee osteoarthritis – results of a 1 year longitudinal arthroscopic study in 422 patients. Osteoarthritis Cartilage (2005) 13(5):361–7. doi: 10.1016/j.joca.2005.01.005
70. Roemer FW, Guermazi A, Felson DT, Niu J, Nevitt MC, Crema MD, et al. Presence of MRI-detected joint effusion and synovitis increases the risk of cartilage loss in knees without osteoarthritis at 30-month follow-up: the MOST study. Ann Rheum Dis (2011) 70(10):1804–9. doi: 10.1136/ard.2011.150243
71. Kapoor M, Martel-Pelletier J, Lajeunesse D, Pelletier JP, Fahmi H. Role of proinflammatory cytokines in the pathophysiology of osteoarthritis. Nat Rev Rheumatol (2011) 7(1):33–42. doi: 10.1038/nrrheum.2010.196
72. Mehana EE, Khafaga AF, El-Blehi SS. The role of matrix metalloproteinases in osteoarthritis pathogenesis: An updated review. Life Sci (2019) 234:116786. doi: 10.1016/j.lfs.2019.116786
73. Hwang HS, Kim HA. Chondrocyte apoptosis in the pathogenesis of osteoarthritis. Int J Mol Sci (2015) 16(11):26035–54. doi: 10.3390/ijms161125943
74. Sellam J, Berenbaum F. The role of synovitis in pathophysiology and clinical symptoms of osteoarthritis. Nat Rev Rheumatol (2010) 6(11):625–35. doi: 10.1038/nrrheum.2010.159
75. Nettelbladt E, Sundblad L. Protein patterns in synovial fluid and serum in rheumatoid arthritis and osteoarthritis. Arthritis Rheumatol (1959) 2(2):144–51. doi: 10.1002/1529-0131(195904)2:2<144::AID-ART1780020206>3.0.CO;2-G
76. Gobezie R, Kho A, Krastins B, Sarracino DA, Thornhill TS, Chase M, et al. High abundance synovial fluid proteome: distinct profiles in health and osteoarthritis. Arthritis Res Ther (2007) 9(2):R36. doi: 10.1186/ar2172
77. Sohn DH, Sokolove J, Sharpe O, Erhart JC, Chandra PE, Lahey LJ, et al. Plasma proteins present in osteoarthritic synovial fluid can stimulate cytokine production via toll-like receptor 4. Arthritis Res Ther (2012) 14(1):R7. doi: 10.1186/ar3555
78. Pelletier JP, Martel-Pelletier J, Abramson SB. Osteoarthritis, an inflammatory disease: potential implication for the selection of new therapeutic targets. Arthritis Rheumatol (2001) 44(6):1237–47. doi: 10.1002/1529-0131(200106)44:6<1237::AID-ART214>3.0.CO;2-F
79. Kaneko S, Satoh T, Chiba J, Ju C, Inoue K, Kagawa J. Interleukin-6 and interleukin-8 levels in serum and synovial fluid of patients with osteoarthritis. Cytokines Cell Mol Ther (2000) 6(2):71–9. doi: 10.1080/13684730050515796
80. Rosenthal AK, Gohr CM, Ninomiya J, Wakim BT. Proteomic analysis of articular cartilage vesicles from normal and osteoarthritic cartilage. Arthritis Rheumatol (2011) 63(2):401–11. doi: 10.1002/art.30120
81. Meng W, He C, Hao Y, Wang L, Li L, Zhu G. Prospects and challenges of extracellular vesicle-based drug delivery system: considering cell source. Drug Deliv (2020) 27(1):585–98. doi: 10.1080/10717544.2020.1748758
82. Ni Z, Kuang L, Chen H, Xie Y, Zhang B, Ouyang J, et al. The exosome-like vesicles from osteoarthritic chondrocyte enhanced mature IL-1beta production of macrophages and aggravated synovitis in osteoarthritis. Cell Death Dis (2019) 10(7):522. doi: 10.1038/s41419-019-1739-2
83. Headland SE, Jones HR, Norling LV, Kim A, Souza PR, Corsiero E, et al. Neutrophil-derived microvesicles enter cartilage and protect the joint in inflammatory arthritis. Sci Transl Med (2015) 7(315):315ra190. doi: 10.1126/scitranslmed.aac5608
84. Platzer H, Nees TA, Reiner T, Tripel E, Gantz S, Hagmann S, et al. Impact of mononuclear cell infiltration on chondrodestructive MMP/ADAMTS production in osteoarthritic knee joints-an ex vivo study. J Clin Med (2020) 9(5):1279. doi: 10.3390/jcm9051279
85. Gao K, Zhu WX, Li H, Ma DJ, Liu WD, Yu WJ, et al. Association between cytokines and exosomes in synovial fluid of individuals with knee osteoarthritis. Mod Rheumatol (2020) 30(4):758–64. doi: 10.1080/14397595.2019.1651445
86. Attur MG, Patel IR, Patel RN, Abramson SB, Amin AR. Autocrine production of IL-1 beta by human osteoarthritis-affected cartilage and differential regulation of endogenous nitric oxide, IL-6, prostaglandin E2, and IL-8. Proc Assoc Am Physicians (1998) 110(1):65–72.
87. Zhang X, Hsueh MF, Huebner JL, Kraus VB. TNF-alpha carried by plasma extracellular vesicles predicts knee osteoarthritis progression. Front Immunol (2021) 12:758386. doi: 10.3389/fimmu.2021.758386
88. Kolhe R, Hunter M, Liu S, Jadeja RN, Pundkar C, Mondal AK, et al. Gender-specific differential expression of exosomal miRNA in synovial fluid of patients with osteoarthritis. Sci Rep (2017) 7(1):2029. doi: 10.1038/s41598-017-01905-y
89. Distler JH, Jungel A, Huber LC, Seemayer CA, Reich CF 3rd, Gay RE, et al. The induction of matrix metalloproteinase and cytokine expression in synovial fibroblasts stimulated with immune cell microparticles. Proc Natl Acad Sci U S A (2005) 102(8):2892–7. doi: 10.1073/pnas.0409781102
90. Berckmans RJ, Nieuwland R, Kraan MC, Schaap MC, Pots D, Smeets TJ, et al. Synovial microparticles from arthritic patients modulate chemokine and cytokine release by synoviocytes. Arthritis Res Ther (2005) 7(3):R536–44. doi: 10.1186/ar1706
91. Monasterio G, Castillo F, Rojas L, Cafferata EA, Alvarez C, Carvajal P, et al. Th1/Th17/Th22 immune response and their association with joint pain, imagenological bone loss, RANKL expression and osteoclast activity in temporomandibular joint osteoarthritis: A preliminary report. J Oral Rehabil (2018) 45(8):589–97. doi: 10.1111/joor.12649
92. Liu Q, Wang R, Hou S, He F, Ma Y, Ye T, et al. Chondrocyte-derived exosomes promote cartilage calcification in temporomandibular joint osteoarthritis. Arthritis Res Ther (2022) 24(1):44. doi: 10.1186/s13075-022-02738-5
93. Liu XW, Li XP, Hua BQ, Yang XQ, Zheng JF, Liu SG. WNT16 is upregulated early in mouse TMJ osteoarthritis and protects fibrochondrocytes against IL-1 beta induced inflammatory response by regulation of RUNX2/MMP13 cascade. Bone (2021) 143:115793. doi: 10.1016/j.bone.2020.115793
94. Xu M, Zhang X, He Y. An updated view on temporomandibular joint degeneration: Insights from the cell subsets of mandibular condylar cartilage. Stem Cells Dev (2022) 31(15-16):445–59. doi: 10.1089/scd.2021.0324
95. Krol J, Loedige I, Filipowicz W. The widespread regulation of microRNA biogenesis, function and decay. Nat Rev Genet (2010) 11(9):597–610. doi: 10.1038/nrg2843
96. Pritchard CC, Cheng HH, Tewari M. MicroRNA profiling: approaches and considerations. Nat Rev Genet (2012) 13(5):358–69. doi: 10.1038/nrg3198
97. Zhou Y, Ming J, Li Y, Li B, Deng M, Ma Y, et al. Exosomes derived from miR-126-3p-overexpressing synovial fibroblasts suppress chondrocyte inflammation and cartilage degradation in a rat model of osteoarthritis. Cell Death Discovery (2021) 7(1):37. doi: 10.1038/s41420-021-00418-y
98. Coutinho de Almeida R, Ramos YFM, Mahfouz A, den Hollander W, Lakenberg N, Houtman E, et al. RNA Sequencing data integration reveals an miRNA interactome of osteoarthritis cartilage. Ann Rheum Dis (2019) 78(2):270–7. doi: 10.1136/annrheumdis-2018-213882
99. Kato T, Miyaki S, Ishitobi H, Nakamura Y, Nakasa T, Lotz MK, et al. Exosomes from IL-1beta stimulated synovial fibroblasts induce osteoarthritic changes in articular chondrocytes. Arthritis Res Ther (2014) 16(4):R163. doi: 10.1186/ar4679
100. Nakamura A, Rampersaud YR, Nakamura S, Sharma A, Zeng F, Rossomacha E, et al. microRNA-181a-5p antisense oligonucleotides attenuate osteoarthritis in facet and knee joints. Ann Rheum Dis (2019) 78(1):111–21. doi: 10.1136/annrheumdis-2018-213629
101. Nakamura A, Rampersaud YR, Sharma A, Lewis SJ, Wu B, Datta P, et al. Identification of microRNA-181a-5p and microRNA-4454 as mediators of facet cartilage degeneration. JCI Insight (2016) 1(12):e86820. doi: 10.1172/jci.insight.86820
102. Li SM, Zhao YQ, Hao YL, Liang YY. Upregulation of miR-504-3p is associated with favorable prognosis of acute myeloid leukemia and may serve as a tumor suppressor by targeting MTHFD2. Eur Rev Med Pharmacol Sci (2019) 23(3):1203–13. doi: 10.26355/eurrev_201902_17013
103. Li W, Zhao S, Yang H, Zhang C, Kang Q, Deng J, et al. Potential novel prediction of TMJ-OA: MiR-140-5p regulates inflammation through Smad/TGF-beta signaling. Front Pharmacol (2019) 10:15. doi: 10.3389/fphar.2019.00015
104. Zhou L, Chen D, Liu P, Chen L, Su Y. miR-132-3p participates in the pathological mechanism of temporomandibular joint osteoarthritis by targeting PTEN. Arch Oral Biol (2022) 142:105511. doi: 10.1016/j.archoralbio.2022.105511
105. Yang JL, Xu YF, Xue X, Zhang M, Wang S, Qi K. MicroRNA-26b regulates BMSC osteogenic differentiation of TMJ subchondral bone through ll-catenin in osteoarthritis. Bone. (2022) 162:116448. doi: 10.1016/j.bone.2022.116448
106. Jiao K, Zhang M, Niu L, Yu S, Zhen G, Xian L, et al. Overexpressed TGF-beta in subchondral bone leads to mandibular condyle degradation. J Dent Res (2014) 93(2):140–7. doi: 10.1177/0022034513513034
107. Pizolato RA, Gaviao MB, Berretin-Felix G, Sampaio AC, Trindade Junior AS. Maximal bite force in young adults with temporomandibular disorders and bruxism. Braz Oral Res (2007) 21(3):278–83. doi: 10.1590/S1806-83242007000300015
108. D’Lima DD, Fregly BJ, Patil S, Steklov N, Colwell CW Jr. Knee joint forces: prediction, measurement, and significance Proc Inst Mech Eng H (2012) 226(2):95–102 doi: 10.1177/0954411911433372
109. Hodgkinson T, Kelly DC, Curtin CM, O’Brien FJ. Mechanosignalling in cartilage: an emerging target for the treatment of osteoarthritis. Nat Rev Rheumatol (2022) 18(2):67–84. doi: 10.1038/s41584-021-00724-w
110. Chen J, Gupta T, Barasz JA, Kalajzic Z, Yeh WC, Drissi H, et al. Analysis of microarchitectural changes in a mouse temporomandibular joint osteoarthritis model. Arch Oral Biol (2009) 54(12):1091–8. doi: 10.1016/j.archoralbio.2009.10.001
111. Thomas MA, Fahey MJ, Pugliese BR, Irwin RM, Antonyak MA, Delco ML. Human mesenchymal stromal cells release functional mitochondria in extracellular vesicles. Front Bioeng Biotechnol (2022) 10:870193. doi: 10.3389/fbioe.2022.870193
112. Shang XB, Boker KO, Taheri S, Lehmann W, Schilling AF. Extracellular vesicles allow epigenetic mechanotransduction between chondrocytes and osteoblasts. Int J Mol Sci (2021) 22(24):13282. doi: 10.3390/ijms222413282
113. Zhang J, Liao L, Zhu J, Wan X, Xie M, Zhang H, et al. Osteochondral interface stiffening in mandibular condylar osteoarthritis. J Dent Res (2018) 97(5):563–70. doi: 10.1177/0022034517748562
114. Rilla K, Mustonen AM, Arasu UT, Harkonen K, Matilainen J, Nieminen P. Extracellular vesicles are integral and functional components of the extracellular matrix. Matrix Biol (2019) 75-76:201–19. doi: 10.1016/j.matbio.2017.10.003
115. Heraud F, Heraud A, Harmand MF. Apoptosis in normal and osteoarthritic human articular cartilage. Ann Rheum Dis (2000) 59(12):959–65. doi: 10.1136/ard.59.12.959
116. Sharif M, Whitehouse A, Sharman P, Perry M, Adams M. Increased apoptosis in human osteoarthritic cartilage corresponds to reduced cell density and expression of caspase-3. Arthritis Rheumatol (2004) 50(2):507–15. doi: 10.1002/art.20020
117. Wang XD, Kou XX, He DQ, Zeng MM, Meng Z, Bi RY, et al. Progression of cartilage degradation, bone resorption and pain in rat temporomandibular joint osteoarthritis induced by injection of iodoacetate. PloS One (2012) 7(9):e45036. doi: 10.1371/journal.pone.0045036
118. Mitton E, Gohr CM, McNally MT, Rosenthal AK. Articular cartilage vesicles contain RNA. Biochem Biophys Res Commun (2009) 388(3):533–8. doi: 10.1016/j.bbrc.2009.08.038
119. Boere J, Malda J, van de Lest CHA, van Weeren PR, Wauben MHM. Extracellular vesicles in joint disease and therapy. Front Immunol (2018) 9:2575. doi: 10.3389/fimmu.2018.02575
120. Hayami T, Pickarski M, Wesolowski GA, McLane J, Bone A, Destefano J, et al. The role of subchondral bone remodeling in osteoarthritis: Reduction of cartilage degeneration and prevention of osteophyte formation by alendronate in the rat anterior cruciate ligament transection model. Arthritis Rheumatol (2004) 50(4):1193–206. doi: 10.1002/art.20124
121. Ben-Trad L, Matei CI, Sava MM, Filali S, Duclos ME, Berthier Y, et al. Synovial extracellular vesicles: Structure and role in synovial fluid tribological performances. Int J Mol Sci (2022) 23(19):11998. doi: 10.3390/ijms231911998
122. Lu K, Ma F, Yi D, Yu H, Tong LP, Chen D. Molecular signaling in temporomandibular joint osteoarthritis. J Orthop Transl (2022) 32:21–7. doi: 10.1016/j.jot.2021.07.001
123. Ameye L, Aria D, Jepsen K, Oldberg A, Xu T, Young MF. Abnormal collagen fibrils in tendons of biglycan/fibromodulin-deficient mice lead to gait impairment, ectopic ossification, and osteoarthritis. FASEB J (2002) 16(7):673–80. doi: 10.1096/fj.01-0848com
124. Ameye LG, Deberg M, Oliveira M, Labasse A, Aeschlimann JM, Henrotin Y. The chemical biomarkers C2C, Coll2-1, and Coll2-1NO2 provide complementary information on type II collagen catabolism in healthy and osteoarthritic mice. Arthritis Rheumatol (2007) 56(10):3336–46. doi: 10.1002/art.22875
125. Wadhwa S, Embree MC, Kilts T, Young MF, Ameye LG. Accelerated osteoarthritis in the temporomandibular joint of biglycan/fibromodulin double-deficient mice. Osteoarthritis Cartilage (2005) 13(9):817–27. doi: 10.1016/j.joca.2005.04.016
126. Fathollahi A, Aslani S, Jamshidi A, Mahmoudi M. Epigenetics in osteoarthritis: Novel spotlight. J Cell Physiol (2019) 234(8):12309–24. doi: 10.1002/jcp.28020
127. Zhai G, Ding C, Stankovich J, Cicuttini F, Jones G. The genetic contribution to longitudinal changes in knee structure and muscle strength: a sibpair study. Arthritis Rheumatol (2005) 52(9):2830–4. doi: 10.1002/art.21267
128. Chapman K, Valdes AM. Genetic factors in OA pathogenesis. Bone. (2012) 51(2):258–64. doi: 10.1016/j.bone.2011.11.026
129. Meng W, Adams MJ, Palmer CNA, Shi J, Auton A, 23andMe Research T, et al. Genome-wide association study of knee pain identifies associations with GDF5 and COL27A1 in UK biobank. Commun Biol (2019) 2:321. doi: 10.1038/s42003-019-0568-2
130. Wallace IJ, Worthington S, Felson DT, Jurmain RD, Wren KT, Maijanen H, et al. Knee osteoarthritis has doubled in prevalence since the mid-20th century. P Natl Acad Sci USA (2017) 114(35):9332–6. doi: 10.1073/pnas.1703856114
131. Weldingh E, Johnsen MB, Hagen KB, Osteras N, Risberg MA, Natvig B, et al. The maternal and paternal effects on clinically and surgically defined osteoarthritis. Arthritis Rheumatol (2019) 71(11):1844–8. doi: 10.1002/art.41023
132. Menon R, Shahin H. Extracellular vesicles in spontaneous preterm birth. Am J Reprod Immunol (2021) 85(2). doi: 10.1111/aji.13353
133. Christensen RW. The correction of mandibular ankylosis by arthroplasty and the insertion of a cast vitallium glenoid fossa: a new technique. a preliminary report of three cases. Am J Orthop (1963) 5:16–24.
134. The classic. Tibial plateau prosthesis. By Duncan C. McKeever. (1960) Clin Orthop Relat Res (1985) 192:3–12.
135. Chuong R, Piper MA. Cerebrospinal fluid leak associated with proplast implant removal from the temporomandibular joint. Oral Surg Oral Med Oral Pathol (1992) 74(4):422–5. doi: 10.1016/0030-4220(92)90286-Y
136. Hamelynck KJ. The history of mobile-bearing total knee replacement systems. Orthopedics. (2006) 29(9 Suppl):S7–12.
137. Towheed TE, Maxwell L, Anastassiades TP, Shea B, Houpt J, Robinson V, et al. Glucosamine therapy for treating osteoarthritis. Cochrane Database Syst Rev (2005) 2005(2):CD002946. doi: 10.1002/14651858.CD002946.pub2
138. Alvarez-Camino JC, Vazquez-Delgado E, Gay-Escoda C. Use of autologous conditioned serum (Orthokine) for the treatment of the degenerative osteoarthritis of the temporomandibular joint. review of the literature. Med Oral Patol Oral Cir Bucal (2013) 18(3):e433–8. doi: 10.4317/medoral.18373
139. Meijer H, Reinecke J, Becker C, Tholen G, Wehling P. The production of anti-inflammatory cytokines in whole blood by physico-chemical induction. Inflammation Res (2003) 52(10):404–7. doi: 10.1007/s00011-003-1197-1
140. Bennell KL, Paterson KL, Metcalf BR, Duong V, Eyles J, Kasza J, et al. Effect of intra-articular platelet-rich plasma vs placebo injection on pain and medial tibial cartilage volume in patients with knee osteoarthritis: The RESTORE randomized clinical trial. JAMA. (2021) 326(20):2021–30. doi: 10.1001/jama.2021.19415
141. Zhang X, Huebner JL, Kraus VB. Extracellular vesicles as biological indicators and potential sources of autologous therapeutics in osteoarthritis. Int J Mol Sci (2021) 22(15):8351. doi: 10.3390/ijms22158351
142. Otahal A, Kramer K, Kuten-Pella O, Weiss R, Stotter C, Lacza Z, et al. Characterization and chondroprotective effects of extracellular vesicles from plasma- and serum-based autologous blood-derived products for osteoarthritis therapy. Front Bioeng Biotechnol (2020) 8:584050. doi: 10.3389/fbioe.2020.584050
143. Zhang X, Hubal MJ, Kraus VB. Immune cell extracellular vesicles and their mitochondrial content decline with ageing. Immun Ageing (2020) 17:1. doi: 10.1186/s12979-019-0172-9
144. Petersen KK. Predicting pain after standard pain therapy for knee osteoarthritis - the first steps towards personalized mechanistic-based pain medicine in osteoarthritis. Scand J Pain (2023) 23(1):40–8. doi: 10.1515/sjpain-2022-0082
145. Rutgers M, Saris DB, Dhert WJ, Creemers LB. Cytokine profile of autologous conditioned serum for treatment of osteoarthritis, in vitro effects on cartilage metabolism and intra-articular levels after injection. Arthritis Res Ther (2010) 12(3):R114. doi: 10.1186/ar3050
146. Yin WJ, Xu HT, Sheng JG, An ZQ, Guo SC, Xie XT, et al. Advantages of pure platelet-rich plasma compared with leukocyte- and platelet-rich plasma in treating rabbit knee osteoarthritis. Med Sci Monit (2016) 22:1280–90. doi: 10.12659/MSM.898218
147. Wakitani S, Mitsuoka T, Nakamura N, Toritsuka Y, Nakamura Y, Horibe S. Autologous bone marrow stromal cell transplantation for repair of full-thickness articular cartilage defects in human patellae: two case reports. Cell Transpl (2004) 13(5):595–600. doi: 10.3727/000000004783983747
148. Song Y, Jorgensen C. Mesenchymal stromal cells in osteoarthritis: Evidence for structural benefit and cartilage repair. Biomedicines. (2022) 10(6):1278. doi: 10.3390/biomedicines10061278
149. Mamidi MK, Das AK, Zakaria Z, Bhonde R. Mesenchymal stromal cells for cartilage repair in osteoarthritis. Osteoarthritis Cartilage (2016) 24(8):1307–16. doi: 10.1016/j.joca.2016.03.003
150. Grassel S, Lorenz J. Tissue-engineering strategies to repair chondral and osteochondral tissue in osteoarthritis: use of mesenchymal stem cells. Curr Rheum Rep (2014) 16(10):452. doi: 10.1007/s11926-014-0452-5
151. Barry F, Murphy M. Mesenchymal stem cells in joint disease and repair. Nat Rev Rheumatol (2013) 9(10):584–94. doi: 10.1038/nrrheum.2013.109
152. Kim YS, Choi YJ, Koh YG. Mesenchymal stem cell implantation in knee osteoarthritis: an assessment of the factors influencing clinical outcomes. Am J Sports Med (2015) 43(9):2293–301. doi: 10.1177/0363546515588317
153. Lamo-Espinosa JM, Mora G, Blanco JF, Granero-Molto F, Nunez-Cordoba JM, Lopez-Elio S, et al. Intra-articular injection of two different doses of autologous bone marrow mesenchymal stem cells versus hyaluronic acid in the treatment of knee osteoarthritis: long-term follow up of a multicenter randomized controlled clinical trial (phase I/II). J Transl Med (2018) 16(1):213. doi: 10.1186/s12967-018-1591-7
154. Lamo-Espinosa JM, Blanco JF, Sanchez M, Moreno V, Granero-Molto F, Sanchez-Guijo F, et al. Phase II multicenter randomized controlled clinical trial on the efficacy of intra-articular injection of autologous bone marrow mesenchymal stem cells with platelet rich plasma for the treatment of knee osteoarthritis. J Transl Med (2020) 18(1):356. doi: 10.1186/s12967-020-02530-6
155. Kim H, Yang G, Park J, Choi J, Kang E, Lee BK. Therapeutic effect of mesenchymal stem cells derived from human umbilical cord in rabbit temporomandibular joint model of osteoarthritis. Sci Rep (2019) 9(1):13854. doi: 10.1038/s41598-019-50435-2
156. Cui D, Li H, Xu X, Ye L, Zhou X, Zheng L, et al. Mesenchymal stem cells for cartilage regeneration of TMJ osteoarthritis. Stem Cells Int (2017) 2017:5979741. doi: 10.1155/2017/5979741
157. Checinski M, Checinska K, Turosz N, Kaminska M, Nowak Z, Sikora M, et al. Autologous stem cells transplants in the treatment of temporomandibular joints disorders: A systematic review and meta-analysis of clinical trials. Cells (2022) 11(17):2709. doi: 10.3390/cells11172709
158. Shengjie C, Ting Z, Yu F, Yan L, Yehua G, Yanheng Z, et al. DPSCs attenuate experimental progressive TMJ arthritis by inhibiting the STAT1 pathway. J Dent Res (2020) 99(4):446–55.
159. Sembronio S, Tel A, Tremolada C, Lazzarotto A, Isola M, Robiony M. Temporomandibular joint arthrocentesis and microfragmented adipose tissue injection for the treatment of internal derangement and osteoarthritis: A randomized clinical trial. J Oral Maxillofac Surg (2021) 79(7):1447–56. doi: 10.1016/j.joms.2021.01.038
160. Yin H, Li M, Tian G, Ma Y, Ning C, Yan Z, et al. The role of extracellular vesicles in osteoarthritis treatment via microenvironment regulation. Biomater Res (2022) 26(1):52. doi: 10.1186/s40824-022-00300-7
161. Tao SC, Yuan T, Zhang YL, Yin WJ, Guo SC, Zhang CQ. Exosomes derived from miR-140-5p-overexpressing human synovial mesenchymal stem cells enhance cartilage tissue regeneration and prevent osteoarthritis of the knee in a rat model. Theranostics. (2017) 7(1):180–95. doi: 10.7150/thno.17133
162. Zhu Y, Wang Y, Zhao B, Niu X, Hu B, Li Q, et al. Comparison of exosomes secreted by induced pluripotent stem cell-derived mesenchymal stem cells and synovial membrane-derived mesenchymal stem cells for the treatment of osteoarthritis. Stem Cell Res Ther (2017) 8(1):64. doi: 10.1186/s13287-017-0510-9
163. Qiu M, Liu D, Fu Q. MiR-129-5p shuttled by human synovial mesenchymal stem cell-derived exosomes relieves IL-1β induced osteoarthritis via targeting HMGB1. Life Sci (2021) 269:118987. doi: 10.1016/j.lfs.2020.118987
164. Wu J, Kuang L, Chen C, Yang J, Zeng WN, Li T, et al. miR-100-5p-abundant exosomes derived from infrapatellar fat pad MSCs protect articular cartilage and ameliorate gait abnormalities via inhibition of mTOR in osteoarthritis. Biomaterials. (2019) 206:87–100. doi: 10.1016/j.biomaterials.2019.03.022
165. Woo CH, Kim HK, Jung GY, Jung YJ, Lee KS, Yun YE, et al. Small extracellular vesicles from human adipose-derived stem cells attenuate cartilage degeneration. J Extracell Vesicles (2020) 9(1):1735249. doi: 10.1080/20013078.2020.1735249
166. Cosenza S, Ruiz M, Toupet K, Jorgensen C, Noël D. Mesenchymal stem cells derived exosomes and microparticles protect cartilage and bone from degradation in osteoarthritis. Sci Rep (2017) 7(1):16214. doi: 10.1038/s41598-017-15376-8
167. Yan L, Wu X. Exosomes produced from 3D cultures of umbilical cord mesenchymal stem cells in a hollow-fiber bioreactor show improved osteochondral regeneration activity. Cell Biol Toxicol (2020) 36(2):165–78. doi: 10.1007/s10565-019-09504-5
168. Hu H, Dong L, Bu Z, Shen Y, Luo J, Zhang H, et al. miR-23a-3p-abundant small extracellular vesicles released from gelma/nanoclay hydrogel for cartilage regeneration. J Extracell Vesicles (2020) 9(1):1778883. doi: 10.1080/20013078.2020.1778883
169. Heard BJ, Barton KI, Chung M, Achari Y, Shrive NG, Frank CB, et al. Single intra-articular dexamethasone injection immediately post-surgery in a rabbit model mitigates early inflammatory responses and post-traumatic osteoarthritis-like alterations. J Orthop Res (2015) 33(12):1826–34. doi: 10.1002/jor.22972
170. Zhang S, Chuah SJ, Lai RC, Hui JHP, Lim SK, Toh WS. MSC exosomes mediate cartilage repair by enhancing proliferation, attenuating apoptosis and modulating immune reactivity. Biomaterials. (2018) 156:16–27. doi: 10.1016/j.biomaterials.2017.11.028
171. Haslauer CM, Elsaid KA, Fleming BC, Proffen BL, Johnson VM, Murray MM. Loss of extracellular matrix from articular cartilage is mediated by the synovium and ligament after anterior cruciate ligament injury. Osteoarthritis Cartilage (2013) 21(12):1950–7. doi: 10.1016/j.joca.2013.09.003
172. Riazifar M, Pone EJ, Lötvall J, Zhao W. Stem cell extracellular vesicles: Extended messages of regeneration. Annu Rev Pharmacol Toxicol (2017) 57:125–54. doi: 10.1146/annurev-pharmtox-061616-030146
173. Liu C, Li Y, Yang Z, Zhou Z, Lou Z, Zhang Q. Kartogenin enhances the therapeutic effect of bone marrow mesenchymal stem cells derived exosomes in cartilage repair. Nanomedicine (2020) 15(3):273–88. doi: 10.2217/nnm-2019-0208
174. Vonk LA, van Dooremalen SFJ, Liv N, Klumperman J, Coffer PJ, Saris DBF, et al. Mesenchymal stromal/stem cell-derived extracellular vesicles promote human cartilage regeneration in vitro. Theranostics (2018) 8(4):906–20. doi: 10.7150/thno.20746
175. Yan L, Liu G, Wu X. The umbilical cord mesenchymal stem cell-derived exosomal lncRNA H19 improves osteochondral activity through miR-29b-3p/FoxO3 axis. Clin Transl Med (2021) 11(1):e255. doi: 10.1002/ctm2.255
176. Chen X, Shi Y, Xue P, Ma X, Li J, Zhang J. Mesenchymal stem cell-derived exosomal microRNA-136-5p inhibits chondrocyte degeneration in traumatic osteoarthritis by targeting ELF3. Arthritis Res Ther (2020) 22(1):256. doi: 10.1186/s13075-020-02325-6
177. Mao G, Zhang Z, Hu S, Zhang Z, Chang Z, Huang Z, et al. Exosomes derived from miR-92a-3p-overexpressing human mesenchymal stem cells enhance chondrogenesis and suppress cartilage degradation via targeting WNT5A. Stem Cell Res Ther (2018) 9(1):247. doi: 10.1186/s13287-018-1004-0
178. Liu S, Xu X, Liang S, Chen Z, Zhang Y, Qian A, et al. The application of MSCs-derived extracellular vesicles in bone disorders: Novel cell-free therapeutic strategy. Front Cell Dev Biol (2020) 8:619. doi: 10.3389/fcell.2020.00619
179. Liang Y, Xu X, Li X, Xiong J, Li B, Duan L, et al. Chondrocyte-targeted MicroRNA delivery by engineered exosomes toward a cell-free osteoarthritis therapy. ACS Appl Mater Interfaces (2020) 12(33):36938–47. doi: 10.1021/acsami.0c10458
180. Wang Y, Zhao M, Li W, Yang Y, Zhang Z, Ma R, et al. BMSC-derived small extracellular vesicles induce cartilage reconstruction of temporomandibular joint osteoarthritis via autotaxin-YAP signaling axis. Front Cell Dev Biol (2021) 9:656153. doi: 10.3389/fcell.2021.656153
181. Zhang S, Teo KYW, Chuah SJ, Lai RC, Lim SK, Toh WS. MSC exosomes alleviate temporomandibular joint osteoarthritis by attenuating inflammation and restoring matrix homeostasis. Biomaterials. (2019) 200:35–47. doi: 10.1016/j.biomaterials.2019.02.006
182. Li Q, Yu H, Sun M, Yang P, Hu X, Ao Y, et al. The tissue origin effect of extracellular vesicles on cartilage and bone regeneration. Acta Biomater (2021) 125:253–66. doi: 10.1016/j.actbio.2021.02.039
183. Imai T, Takahashi Y, Nishikawa M, Kato K, Morishita M, Yamashita T, et al. Macrophage-dependent clearance of systemically administered B16BL6-derived exosomes from the blood circulation in mice. J Extracell Vesicles (2015) 4:26238. doi: 10.3402/jev.v4.26238
184. Fadadu PP, Mazzola AJ, Hunter CW, Davis TT. Review of concentration yields in commercially available platelet-rich plasma (PRP) systems: A call for PRP standardization. Reg Anesth Pain Med (2019) 44(6):rapm-2018-100356. doi: 10.1136/rapm-2018-100356
185. Brennan K, Martin K, FitzGerald SP, O’Sullivan J, Wu Y, Blanco A, et al. A comparison of methods for the isolation and separation of extracellular vesicles from protein and lipid particles in human serum. Sci Rep (2020) 10(1):1039. doi: 10.1038/s41598-020-57497-7
186. Ai M, Hotham WE, Pattison LA, Ma Q, Henson FMD, Smith ESJ. Role of human mesenchymal stem cells and derived extracellular vesicles in reducing sensory neuron hyperexcitability and pain behaviors in murine osteoarthritis. Arthritis Rheum (2022). doi: 10.1002/art.42353
187. de Liyis BG, Nolan J, Maharjana MA. Fibroblast growth factor receptor 1-bound extracellular vesicle as novel therapy for osteoarthritis. Biomedicine-Taiwan. (2022) 12(2):1–9. doi: 10.37796/2211-8039.1308
188. Xu X, Liang Y, Li X, Ouyang K, Wang M, Cao T, et al. Exosome-mediated delivery of kartogenin for chondrogenesis of synovial fluid-derived mesenchymal stem cells and cartilage regeneration. Biomaterials. (2021) 269:120539. doi: 10.1016/j.biomaterials.2020.120539
189. Lai H, Li J, Kou X, Mao X, Zhao W, Ma L. Extracellular vesicles for dental pulp and periodontal regeneration. Pharmaceutics (2023) 15(1):282. doi: 10.3390/pharmaceutics15010282
Keywords: osteoarthritis, TMJ, peripheral joint, extracellular vesicles, EV therapy
Citation: Yang B, Li X, Fu C, Cai W, Meng B, Qu Y, Kou X and Zhang Q (2023) Extracellular vesicles in osteoarthritis of peripheral joint and temporomandibular joint. Front. Endocrinol. 14:1158744. doi: 10.3389/fendo.2023.1158744
Received: 04 February 2023; Accepted: 21 February 2023;
Published: 06 March 2023.
Edited by:
Bingdong Sui, Air Force Medical University, ChinaCopyright © 2023 Yang, Li, Fu, Cai, Meng, Qu, Kou and Zhang. This is an open-access article distributed under the terms of the Creative Commons Attribution License (CC BY). The use, distribution or reproduction in other forums is permitted, provided the original author(s) and the copyright owner(s) are credited and that the original publication in this journal is cited, in accordance with accepted academic practice. No use, distribution or reproduction is permitted which does not comply with these terms.
*Correspondence: Qingbin Zhang, cWluZ2JpbnpoYW5nQGd6aG11LmVkdS5jbg==; Xiaoxing Kou, a291eGlhb3hpbmdAbWFpbC5zeXN1LmVkdS5jbg==
†These authors contributed equally to this work and share first authorship