- 1Parturition Research Group, Institute of Reproductive and Developmental Biology, Department of Metabolism, Digestion and Reproduction, Imperial College London, London, United Kingdom
- 2The March of Dimes European Prematurity Research Centre at Imperial College London, London, United Kingdom
- 3The Parasol Foundation Centre for Women’s Health and Cancer Research, St Mary’s Hospital, Imperial College Healthcare National Health Service (NHS) Trust, London, United Kingdom
- 4Department of Obstetrics & Gynaecology, Chelsea and Westminster Hospital National Health Service (NHS) Trust, London, United Kingdom
Introduction: Preterm birth is one of the major causes of neonatal morbidity and mortality across the world. Both term and preterm labour are preceded by inflammatory activation in uterine tissues. This includes increased leukocyte infiltration, and subsequent increase in chemokine and cytokine levels, activation of pro-inflammatory transcription factors as NF-κB and increased prostaglandin synthesis. Prostaglandin F2α (PGF2α) is one of the myometrial activators and stimulators.
Methods: Here we investigated the role of PGF2α in pro-inflammatory signalling pathways in human myometrial cells isolated from term non-labouring uterine tissue. Primary myometrial cells were treated with G protein inhibitors, calcium chelators and/or PGF2α. Nuclear extracts were analysed by TranSignal cAMP/Calcium Protein/DNA Array. Whole cell protein lysates were analysed by Western blotting. mRNA levels of target genes were analysed by RT-PCR.
Results: The results show that PGF2α increases inflammation in myometrial cells through increased activation of NF-κB and MAP kinases and increased expression of COX-2. PGF2α was found to activate several calcium/cAMP-dependent transcription factors, such as CREB and C/EBP-β. mRNA levels of NF-κB-regulated cytokines and chemokines were also elevated with PGF2α stimulation. We have shown that the increase in PGF2α-mediated COX-2 expression in myometrial cells requires coupling of the FP receptor to both Gαq and Gαi proteins. Additionally, PGF2α-induced calcium response was also mediated through Gαq and Gαi coupling.
Discussion: In summary, our findings suggest that PGF2α-induced inflammation in myometrial cells involves activation of several transcription factors – NF-κB, MAP kinases, CREB and C/EBP-β. Our results indicate that the FP receptor signals via Gαq and Gαi coupling in myometrium. This work provides insight into PGF2α pro-inflammatory signalling in term myometrium prior to the onset of labour and suggests that PGF2α signalling pathways could be a potential target for management of preterm labour.
Introduction
Preterm birth (PTB) is defined by the World Health Organisation as birth completed before the 37th week of gestation (1). Every year, approximately 15 million babies are born preterm, with rates of preterm birth increasing worldwide (2). Preterm birth and the associated complications are the major cause of neonatal death and illness around the world. Preterm labour shares many common pathways with term labour, however, preterm labour results from early activation of these pathways (3). Towards the end of pregnancy and in labour, the uterus goes through a set of gradual biochemical, anatomical, endocrine and immune changes that transform it from a quiescent state maintaining pregnancy, to the activated contractile state, ready for labour (4–6). Key transformations within reproductive tissues include cervical remodelling and dilatation, fetal and decidual membrane activation and an increase in myometrial contractility (5, 7–9). An increase in myometrial contractility results from changes in expression of proteins that promote intercellular connectivity and excitability of myocytes (6, 10–12). Through the increasing activity of metalloproteinases in the cervix, the structure of the cervix changes and begins to soften and dilate (13–16). Activation of decidua and fetal membranes involves the degradation of extracellular matrix proteins and consequential weakening of the membranes (9, 17). This leads to their separation from decidua in the lower uterine segment and eventually their rupture (17, 18).
Although the full mechanism that controls and triggers the onset of labour is yet to be understood, several studies have shown that the onset of both term and preterm labour is associated with the activation of inflammatory pathways (19–21). Parturition is characterised by an increased leukocyte infiltration into the uterus with the levels of cytokines and chemokines such as IL-6, IL-8, CCL2, CCL5, TNF-α and IL-1β being elevated in term and preterm labour (22–25). Several cytokines (including IL-1β and TNF-α) contribute to an increase in prostaglandin production by activating phospholipid metabolism and stimulating cyclooxygenase 2 (COX-2) expression (26). IL-6 and IL-1β increase oxytocin secretion with IL-6 also increasing the expression of the oxytocin (OT) receptor (27, 28). Cooperative effects of IL-1β and prostaglandin F2α (PGF2α) on IL-6 and COX-2 upregulation in myometrial cells were recently described (29). IL-1β, IL-6 and TNF-α stimulate translocation of nuclear factor-κB (NF-κB) to the nucleus and thus triggering the transcription of several pro-inflammatory mediators (30). In preterm labour, the stretch and infiltrating pro-inflammatory cytokines can further increase the expression of chemokines in myometrium through activation of NF-κB (24). Additionally, the upregulation of a cassette of genes related to uterine activation and myometrial contractility takes place in the myometrium prior to labour (31). These so-called, contraction-associated proteins (CAPs), include ion channel proteins, connexin-43 and the receptors for oxytocin and prostaglandins.
The prostaglandin biosynthetic pathway is activated prior to the onset of labour with cytosolic phospholipase A2 (PLA2) and COX-2 enzymes participating in key steps of the prostaglandin biosynthesis in reproductive tissues (32, 33). In the uterus, prostaglandin E2 (PGE2) and PGF2α stimulate myometrial contractility (4), and contribute to parturition-associated fetal membrane activation and rupture through stimulation of matrix metalloproteinase activity, leading to extracellular matrix degradation (32, 34). Additionally, prostaglandin-mediated matrix metalloproteinase (MMP) activity contributes to cervical ripening (35–37). PGF2α activates signalling pathways that lead to the activation of NF-κB, mitogen-activated protein kinases (MAPK), phosphatidylinositol-4,5-bisphospate 3-kinase (PI3K) and calcineurin/nuclear factor of activated T-cells (NFAT) in myometrial cells (38). Additionally, it has been reported that PGF2α also increases the expression of several uterine activation proteins (such as connexin-43, oxytocin receptor (OTR), COX-2) (39) and pro-inflammatory cytokines and chemokines in myometrium through the activation of phospholipase C (PLC) and protein kinase C (PKC) pathways (40). In turn, these cytokines drive further production of prostaglandins resulting in a feed-forward loop.
The effects of PGF2α are mediated through its FP receptor, belonging to a family of G protein-coupled receptors (GPCRs). The FP receptor signals through interactions with multiple G proteins, including Gαq/11, Gα12/13 and Gβγ (presumed to originate from Gαi) (41–44). In human myometrium, the coupling of the FP receptor to Gαq/11 has been shown to activate PLCβ (42) leading to the release of intracellular calcium essential for myometrial contraction, however, the role of G proteins in PGF2α-mediated inflammation is yet to be elucidated. This study aims to investigate the signalling pathways involved in pro-inflammatory signalling mediated by PGF2α in human myometrium with the focus on G protein-mediated signalling, transcription factor activation and the role of calcium.
As PGF2α drives myometrial contractility via FP receptor coupling to a Gαq/11-calcium pathway, we hypothesised that PGF2α-mediated inflammation in human myometrium is via coupling to Gαi. This could highlight a role for specific FP receptor/G protein signalling pathways to be exploited as potential therapeutic target for PTB prevention. By profiling the effects of PGF2α on inflammatory pathway transcription factors, MAP kinases, cytokines and COX-2, we investigated the involvement of Gαi and Gαq coupling and calcium in PGF2α-mediated signalling in primary myometrial cells.
Methods
Myometrial tissue collection
Myometrial tissue was collected from patients undergoing planned caesarean sections at term prior to labour onset. Myometrial tissue was taken from the upper margin of the lower uterine segment incision and kept in phosphate-buffered saline (PBS) at 4°C until further processing. Myometrial tissue samples were obtained with informed written consent. This research study was approved by the local ethics committees (Riverside Ethics Committee (Ref 3358), and London Harrow Research Ethics Committee (Ref 19/LO/1657).
Primary myometrial smooth muscle cell culture
For isolation of myometrial smooth muscle cells, myometrial tissue was washed in phosphate-buffered saline and dissected into small pieces within 24 hours of collection. Dissected tissue was then digested in the filter-sterilised (0.2μm) collagenase solution (collagenase 1A 1mg/ml, collagenase 1mg/ml, bovine serum albumin 2mg/ml dissolved in a 50:50 mixture of serum-free high glucose formulation of Dulbecco’s Modified Eagle’s Medium (DMEM) and DMEM/Nutrient F-12 HAM) for 45 minutes at 37°C. The enzymatic activity was stopped by addition of complete DMEM containing 10% fetal bovine serum (FBS), 2mM L-glutamine and 100 U/mL penicillin-streptomycin. The mixture was filtered through a cell strainer (40μm) and cells were isolated by centrifugation at 3000 rpm (1841xg) for 2 minutes. Cells were resuspended in complete DMEM and seeded into a culture flask (T25) to be grown at 37°C, 5% CO2. Cells were subcultured when they reached 90-95% confluence. Cells were washed with PBS and incubated in Trypsin-EDTA solution (0.5 g/L porcine trypsin and 0.2 g/L EDTA x 4Na in Hanks′ Balanced Salt Solution with phenol red) for 5 minutes. The enzyme activity was stopped by addition of DMEM containing 10% FBS. The cell suspension was centrifuged at 1000 rpm (205xg) for 5 minutes. The cells were resuspended in complete DMEM and seeded into flasks or plates. Primary myometrial smooth muscle cells were used for all the experiments presented in this study. Cells were used between passage numbers 2 and 6.
To investigate PGF2α-mediated inflammatory signalling in myometrium, human myometrial cells were treated with PGF2α (1µM). The selected concentration was previously reported to stimulate myometrial contractility and to increase the uterine activation proteins expression (39, 40, 45).
Whole cell protein extraction
For whole cell protein extraction, modified radioimmunoprecipitation assay (RIPA) buffer (1% (v/v) Triton X-100, 1% (w/v) Sodium deoxycholate, 0.1% (v/v) SDS, 150mM NaCl, 10mM Tris, pH 7.4, 1mM EDTA, 1mM PMSF, 0.5% v/v protease inhibitor cocktail, 1% v/v phosphatase inhibitor cocktail) was used. Cell monolayers were scraped off with a cell scraper and collected in 1.5ml microfuge tubes. Cell lysates were centrifuged at 13300xg for 30 minutes at 4°C. Supernatants were transferred to clean microfuge tubes and stored at -20°C until further analysis.
Nuclear/cytosolic protein extraction
Nuclear and cytosolic proteins were extracted from fresh cells. Following completion of treatment, the culture media were removed from the wells and 60 µl of cytosolic extraction buffer A was added to each well. Cells were scraped with plate scraper and the lysate was left to incubate on ice for 10 minutes. 20% Nonidet P-40 was added to each tube (to a final concentration of 1%) and the tubes were vortexed for 10 seconds. The lysates were then centrifuged at 13300xg for 30 seconds at 4°C. The supernatants containing the cytosolic fraction were transferred to clean microfuge tubes, snap-frozen on dry ice and stored at -80°C. The pellet was resuspended in 40µl of nuclear buffer B and the lysates were incubated on ice while shaking for 15 minutes. The lysates were centrifuged at 13300xg for 5 minutes at 4°C. The supernatants containing the nuclear protein fraction were transferred to clean microfuge tubes, snap-frozen on dry ice and then stored at -80°C.
Protein quantification
Protein concentration of whole cell extracts were determined by using detergent compatible (DC) protein assay reagents (Bio-Rad) following the manufacturer’s protocol. Briefly, 20µl of reagent S was added to each ml of reagent A. 25µl of the working mixture was added to 5 µl of standards and samples in 96-well microtiter plates. Then, 200 µl of reagent B was added to the wells. Samples and standards were prepared in duplicates. After 15 minutes of incubation, absorbance was read at 650nm using Optimax microplate reader. Bovine serum albumin standards of known concentrations which were prepared in protein extraction buffer were used as a reference to determine the protein concentration of the lysates.
Western blotting
Extracted protein samples were denatured with protein loading buffer at 80°C for 10 minutes. Protein samples (10-20μg) were loaded onto precast Tris-Glycine polyacrylamide gels (4-20%, Bio-Rad) and resolved by electrophoresis (100V for first 10 minutes, followed by 140V for 60-90 minutes). Following electrophoresis, proteins were transferred to a 0.2µm polyvinylidene fluoride membrane by a semi-dry transfer using Bio-Rad TransBlot Turbo Blotting System (2.5A (constant), 7 minutes). Membranes were blocked in 5% skimmed milk solution in Tris-buffered saline with Tween (TBS-T) (w/v) for 1 hour and then were hybridised with primary antibody at 4°C overnight. Following the incubation with primary antibodies, membranes were washed in TBS-T and then incubated with secondary antibodies conjugated to horseradish peroxidase (HRP) for 1h at room temperature. Membranes were washed in TBS-T and the target proteins were detected using Clarity Western ECL Substrate (Bio-Rad) or Pierce ECL 2 Western Blotting Substrate (Thermo Scientific). ImageQuant LAS4000 Luminescent Image Analyser (GE Healthcare) and Amersham Hyperfilm ECL (GE Healthcare) were used to image the bands. Densitometric analysis was performed using ImageQuant TL v8.1 (GE Healthcare).
RNA extraction
Total RNA was extracted by guanidium thiocyanate-phenol-chloroform extraction using RNA STAT-60 (Amsbio) following the manufacturer’s recommendation. The cells in each well were lysed with 600μl of RNA STAT-60. Cell monolayer was scraped with a clean filter tip, the lysate was passed through a pipette tip several times and transferred to a clean microfuge tube. The homogenate was kept at room temperature for 5 minutes. Chloroform (Sigma-Aldrich) (0.2ml per 1ml of RNA STAT-60) was added to each microtube, the tubes were vortexed for 15 seconds and then centrifuged at 12000xg for 15 minutes at 4°C. The aqueous phase was transferred to microtubes containing 0.5ml of isopropanol. The microtubes were left at room temperature for 15 minutes and then centrifuged at 13300xg for 20 minutes at 4°C. The supernatant was removed, and the pellets were washed with 0.5ml of 75% ethanol and then centrifuged at 12000xg for 10 minutes. The supernatant was discarded, and the pellets were allowed to air dry. RNA was resuspended in 20μl of DEPC-treated water. The concentration and the purity of the extracted RNA were determined using NanoDrop ND-1000 spectrometer. The RNA extracts were stored at -80°C.
DNase treatment and first-strand cDNA synthesis
DNase I was used to remove any potential contamination with DNA in the RNA extracts. 2μg of RNA was mixed with DEPC-treated water, 1μl of DNase I (Sigma-Aldrich) and 1μl of Reaction Buffer (Sigma Aldrich) in the total reaction volume of 20μl. The reaction mix was incubated at room temperature for 15 minutes. The reaction was inactivated by the addition of 1μl of Stop Solution and subsequent heating of the mixture for 10 minutes at 70°C. The reaction mixture was chilled on ice.
DNase I-treated reaction mix was incubated with 1μl 10mM Deoxynucleotide Mix (Sigma-Aldrich) and 1μl Oligo-dT (Sigma-Aldrich) for 10 minutes at 70°C and then chilled on ice. 10μl M-MLV Reverse Transcriptase Buffer, 1μl M-MLV Reverse Transcriptase, 0.5μl Ribonuclease Inhibitor and 6.5μl DEPC-treated water were added to the mixture and the mixture was incubated at room temperature for 15 minutes, then at 37°C for 50 minutes, and then at 85°C for 10 minutes. cDNA was stored at -20°C until further analysis.
Real-time PCR
Quantification of target cDNA was performed by real time polymerase chain reaction with SYBR® Green JumpStart™ Taq ReadyMix™ (Sigma-Aldrich) and the primers specific for the target cDNA (Table 1). Real time PCR was carried out in 96-well plates using StepOnePlus Real Time PCR system (Applied Biosystems). Each reaction mix (total volume: 20μl) contained 10μl SYBR® Green JumpStart™ Taq ReadyMix™, 0.2μl Reference Dye for Quantitative PCR, 0.3μl forward primer (20nM), 0.3μl reverse primer (20nM), 5.2μl DEPC-treated water, and 4μl of diluted cDNA. Reactions were run in duplicates. Following cycling conditions were used: 2 min at 95°C, 40 to 45 cycles of 15 sec at 95°C, 30 sec at 60°C, 100 sec at 72°C; melt curve: 15 sec at 85°C, 1 min at 60°C and 15 sec at 95°C. Gene expression levels (cycle threshold values) for all target genes were normalised to the expression levels of the constitutively expressed gene GAPDH. The comparative cycle threshold method (2-ΔΔCt) was used to determine relative differences in gene expression.
Intracellular calcium response
Myometrial cells were seeded in individual 35mm dishes with 14mm glass microwells (MaTek Corporation) and were grown to near confluence in DMEM – high glucose medium containing 10% FBS, 2mM L-glutamine and 100 U/ml penicillin-streptomycin. Prior to treatment, the cells were kept in serum-depleted growth medium (DMEM - low glucose with/containing 1% FBS, 2mM L-glutamine and 100 U/ml penicillin-streptomycin) overnight. An equal volume of 2X Fluo-4 Direct™ calcium reagent loading solution was added to culture dishes containing cells with growth medium. The cells were incubated with the calcium reagent for 30 minutes at 37°C and then for 30 minutes in the dark at room temperature. Fluorescence intensity was measured using Leica SP5 confocal microscope (Objective: HC PL FLUOTAR 20.0x0.50 DRY) with the settings for excitation at 494 nm and emission at 516 nm. Basal level of fluorescence was measured for 3 minutes and the fluorescence intensity levels in response to treatments were measured for 15 minutes. Fluorescence intensity values in response to treatments were normalised to basal level of fluorescence recorded for non-stimulated cells. Fluorescent intensity plots and the maximum fluorescence intensity values were recorded using Leica Application Suite Advanced Fluorescence Lite Software and analysed using MS Excel and GraphPad Prism. Fluorescence intensity values are expressed in arbitrary units (AU).
Transcription factor array
TranSignal cAMP/Calcium Protein/DNA Array (Panomics) was used to identify transcription factors that become activated with PGF2α receptor stimulation (Table 2). Myometrial cells were treated with PGF2α (1µM, 30 minutes) and nuclear extracts were used for the protein/DNA array protocol as per manufacturer’s protocol.
Nuclear extracts (3-5μg/μl) from PGF2α-treated myometrial cells were incubated with TranSignal Probe Mix (biotin-labelled DNA binding oligonucleotides) to allow the formation of DNA/protein complexes. The protein/DNA complexes (TF-bound probes) were isolated from free probes by spin column separation. The labelled probes from the DNA/protein complexes were then extracted and hybridised to TranSignal array membrane.
For detection, the membranes were blocked in a blocking buffer (Panomics), incubated with streptavidin-HRP conjugate (Panomics) and then washed in wash buffer (Panomics). Membranes were then incubated with detection buffer (Panomics) and enhanced chemiluminescence reagents (Panomics). Membranes were exposed using chemiluminescence imaging system - ImageQuant LAS4000 luminescent image analyser (GE Healthcare) and Amersham Hyperfilm ECL (GE Healthcare). The density of the spots was measured using Fiji ImageJ and ImageQuant TL v8.1 (GE Healthcare). The local area surrounding each spot was used for background subtraction. The density values of each spot were normalised to the average density of the positive control spots on each membrane. Ratio between treated and non-stimulated controls was calculated. Two-fold increase in spot density was considered significant (as per manufacturer’s recommendation).
Statistical analysis
Statistical analysis was performed on all presented data. Data was tested for normality using Kolmogorov- Smirnov test. Normally distributed data was analysed by analysis of variance (ANOVA) followed by Tukey’s or Dunnett’s multiple comparisons tests. Data that was not normally distributed was analysed using Kruskal-Wallis test with Dunn’s multiple comparisons test. All analyses were carried out using GraphPad Prism 9. All results are presented as means of biological replicates with standard errors of the mean (SEM), and probability value of p<0.05 was considered statistically significant.
Results
PGF2α increases inflammation in human myometrium
PGF2α is a uterotonic agent that also participates in uterine activation prior to labour (39). To study the effects of PGF2α on inflammatory signalling pathways involving NF-κB, MAP kinases and COX-2 in myometrium, cultured human myometrial cells were treated with PGF2α (1µM) and the levels of target proteins were assessed by Western blotting.
Treatment of term myometrial cells with PGF2α resulted in the activation of p65 subunit of NF-κB (p<0.01 at 15 min), MAP kinases p38 (p<0.05 at 15 min) and Extracellular signal-regulated kinase (ERK) (p<0.01 at 15 min) (Figures 1A–C). Additionally, PGF2α increased the expression of COX-2 (p<0.001 at 6h), a rate-limiting enzyme in prostaglandin synthesis (Figure 1D). It has been well established that NF-κB participates in the regulation of pro-inflammatory gene expression associated with the onset of labour (46). Therefore, RT-qPCR was used to examine the effects of PGF2α stimulation on downstream NF-κB-regulated gene expression. As expected, the levels of COX-2 mRNA increased significantly within 1h of PGF2α treatment resulting in a 6-fold increase in expression (p<0.01) (Figure 1E). Moreover, PGF2α upregulated multiple pro-labour cytokines and chemokines including IL-8, IL-6 and CCL5 by 12.7-fold (p<0.0001 at 4h), 4.7-fold (p<0.05 at 6h), and 4.4-fold (p<0.01 at 6h), respectively (Figures 1F–H). The expression of CCL2 (2.9-fold) and IL-1β (2.2-fold) mRNA showed increasing trend with PGF2α stimulation but did not reach statistical significance (Figures 1I, J). The effects of PGF2α on downstream NF-κB regulated genes were transient with the mRNA levels returning to basal levels by 24h.
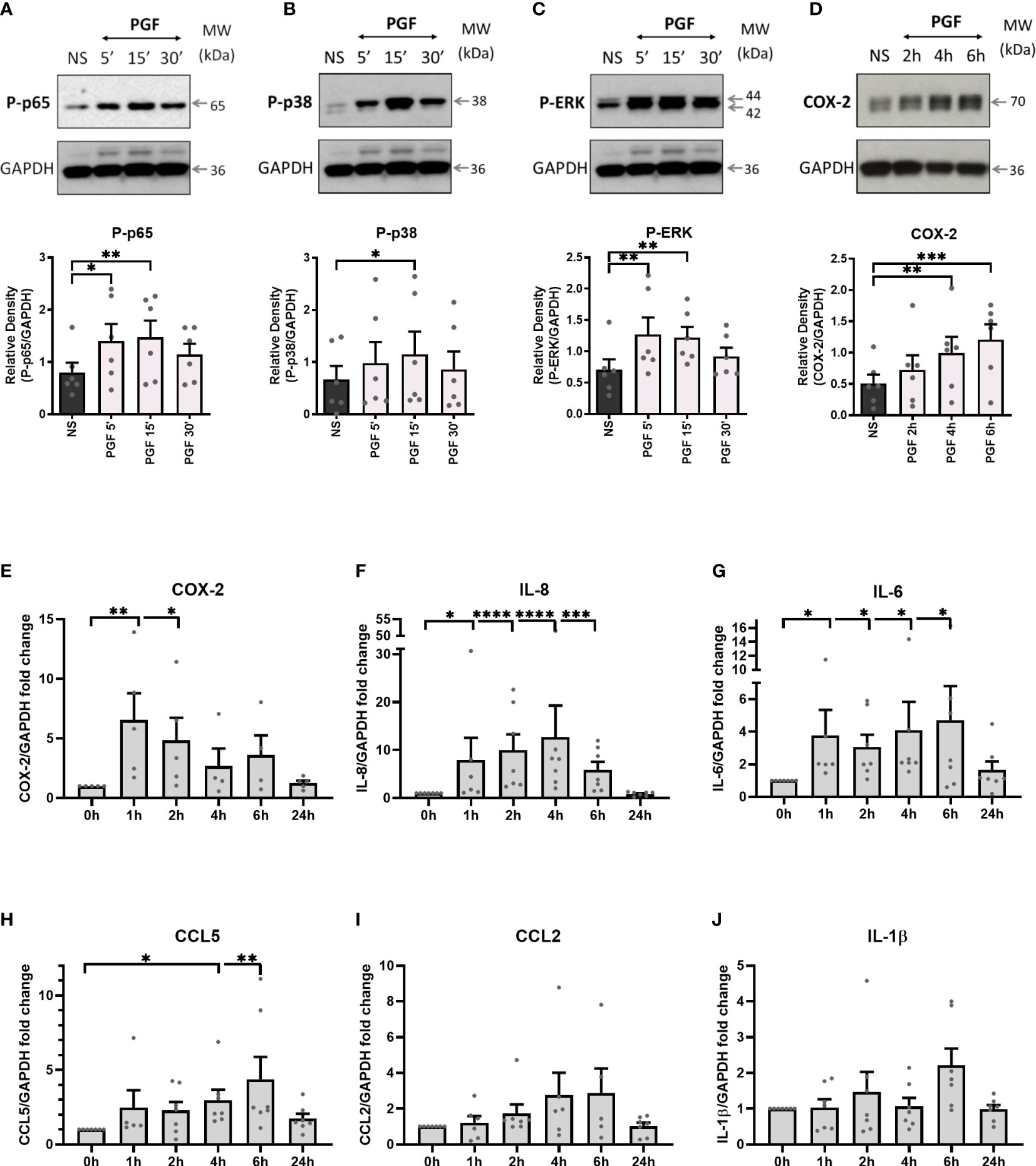
Figure 1 Prostaglandin F2α increases inflammation in myometrium. Primary myometrial smooth muscle cells isolated from term non-labouring myometrium were stimulated with prostaglandin F2α (1μM) for 5 min, 15 min, 30 min, 1h, 2h, 4h, 6h, and 24h. Proteins levels of phospho-p65 (A), phospho-p38 (B), phospho-ERK (C) and COX-2 (D) following treatment with PGF2α were analysed by Western blotting. Graphs are showing relative densities of proteins normalised to GAPDH. Individual repeats are presented as dots. Representative blots (including the loading control) are shown above their corresponding densitometry graphs (n=6) (NS – non-stimulated, PGF - prostaglandin F2α). Total RNA was extracted from myometrial cells that were treated with PGF2α. mRNA levels of COX-2 (E), IL-8 (F), IL-6 (G), CCL5 (H), CCL2 (I) and IL-1β (J) were measured using RT-qPCR. Results in the graphs are expressed as fold change relative to non-stimulated control. (n=5-7); * p < 0.05, ** p<0.01, *** p<0.001, **** p<0.0001 vs NS, ANOVA with Dunnett’s post-test).
The effects of PGF2α on COX-2 require both Gαq and Gαi coupling
As the FP receptor can couple to multiple G proteins, we aimed to determine the role of Gαq and Gαi proteins in PGF2α-stimulated pro-inflammatory signalling. Myometrial cells were pre-treated either with Gαi protein inhibitor (pertussis toxin, PTX, 200 ng/ml) (Figures 2A-D) or with Gαq inhibitor (UBO-QIC (1μM)) (Figures 3A-D) and then stimulated with PGF2a (1μM). The concentrations of G protein inhibitors were selected based on previously published studies (47–50). Treatment of myometrial cells with PTX decreased the PGF2α-stimulated activation of ERK MAP kinase (38% decrease at 15 min) and significantly reduced the upregulation of COX-2 (p<0.05 at 6h) (Figures 2C, D) but did not have a clear effect on NF-κB p65 or p38 MAP kinase activation (Figures 2A, B). Notably, treatment with the Gαq inhibitor, UBO-QIC, also resulted in reduced PGF2α-induced expression of COX-2 (p<0.05 at 6h), potentially via NF-κB activation which was decreased by 43% at 15 min (Figures 3A, D). These results show that PGF2α-driven COX-2 upregulation requires both Gαq and Gαi coupling. We suggest that FP receptor coupling to Gαi could potentially regulate COX-2 expression via activation of ERK, whereas the mechanism through which Gαq regulates COX-2 may involve NF-κB but this requires further investigation.
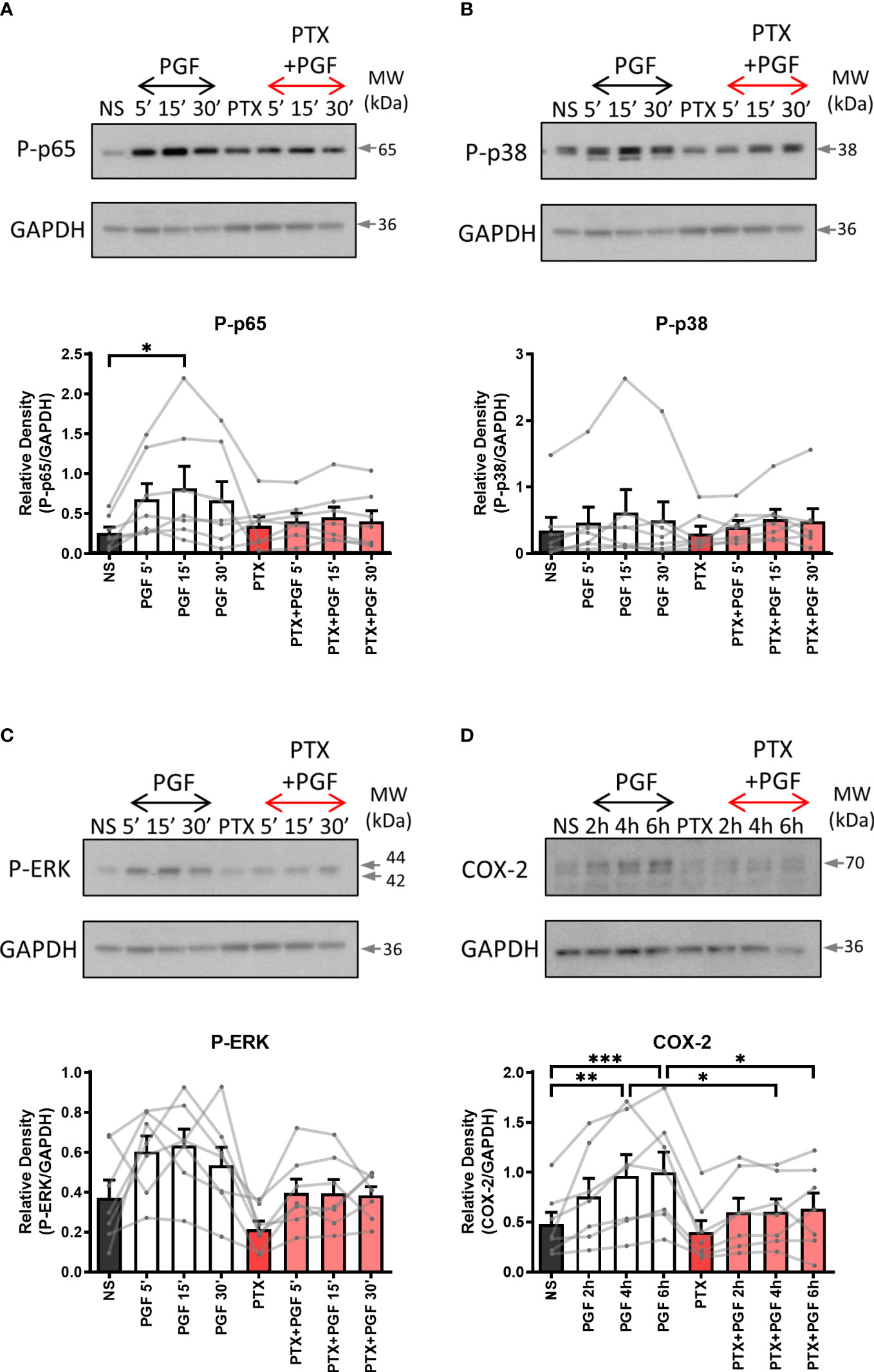
Figure 2 The inhibition of Gαi coupling reduces prostaglandin F2α-mediated COX-2 expression. Primary myometrial cells isolated from term non-labouring myometrium were pre-treated with pertussis toxin (PTX, 200ng/ml) prior to stimulation with PGF2α (1μM). Changes in the protein levels of phospho-p65 (A), phospho-p38 (B), phospho-ERK (C) and COX-2 (D) were measured using Western blotting. Individual repeats are presented as dots connected with lines. Representative Western blots are shown above their corresponding densitometry graphs. Values are presented as mean ± SEM (n=7, ANOVA with Tukey post-test, * p < 0.05, ** p<0.01, *** p<0.001 vs NS or PGF2α-treated).
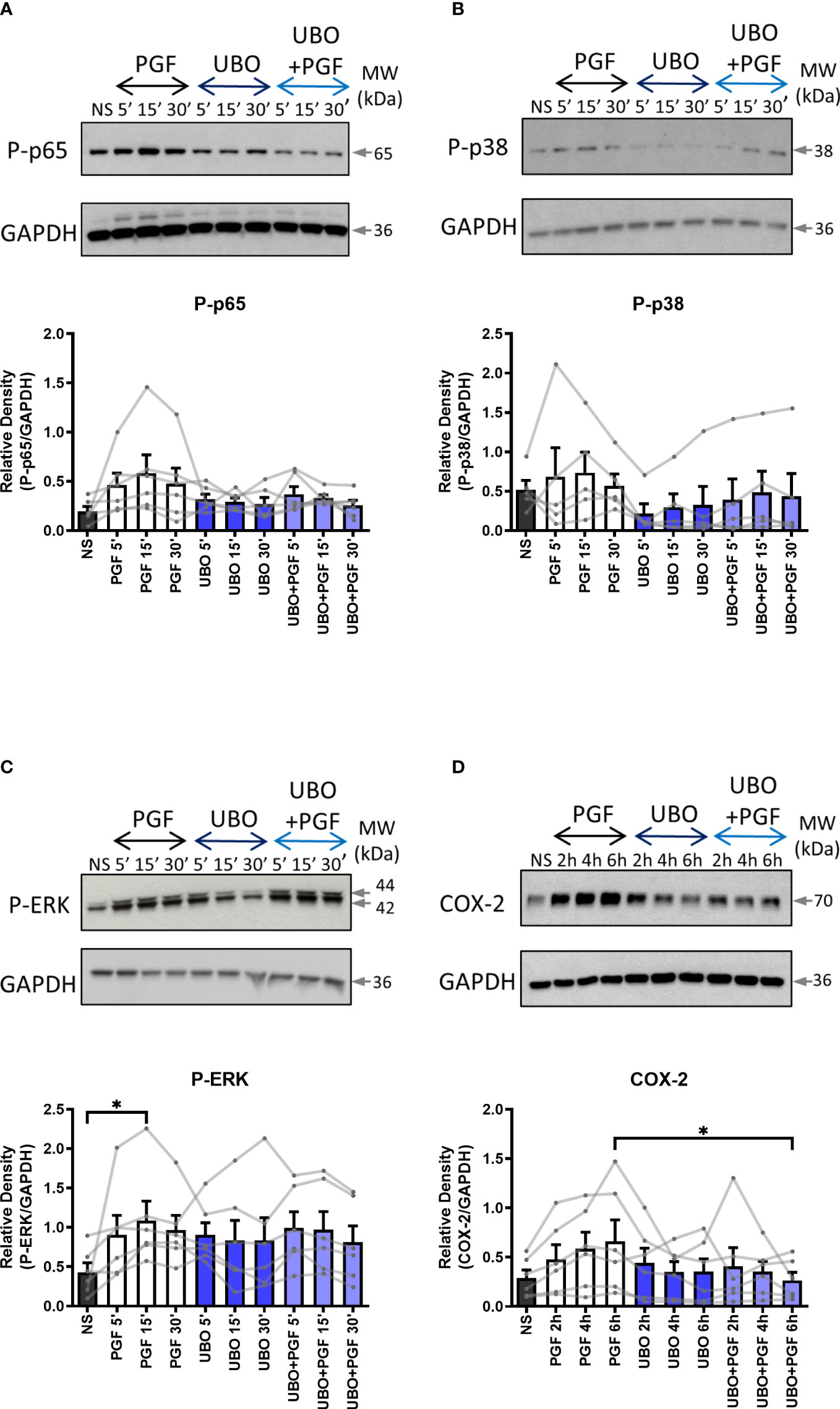
Figure 3 The inhibition of Gαq coupling reduces prostaglandin F2α-mediated COX-2 expression. Primary myometrial cells isolated from term non-labouring myometrium were pre-treated with UBO-QIC (1μM) prior to stimulation with PGF2α (1μM). Changes in the protein levels of phospho-p65 (A), phospho-p38 (B), phospho-ERK (C) and COX-2 (D) were measured using Western blotting. Individual repeats are presented as dots connected with lines. Representative Western blots are shown above their corresponding densitometry graphs. Values are presented as mean ± SEM (n=5-6, ANOVA with Tukey post-test, * p<0.05 vs NS or PGF2α-treated).
PGF2α-mediated calcium response is driven through both Gαq and Gαi protein coupling
FP receptor activation and G protein coupling increases intracellular calcium concentration which can result from an influx of calcium ions across plasma membrane or through calcium release from internal stores (51). We have shown that the FP receptor couples to both Gαq and Gαi in response to PGF2α binding. Through pre-treatment with G protein inhibitors, we confirmed that both G proteins are involved in the calcium response following PGF2α stimulation as calcium response was significantly reduced when Gαq (p<0.05) and Gαi (p<0.05) proteins were inhibited in myometrial cells (Figures 4A, B).
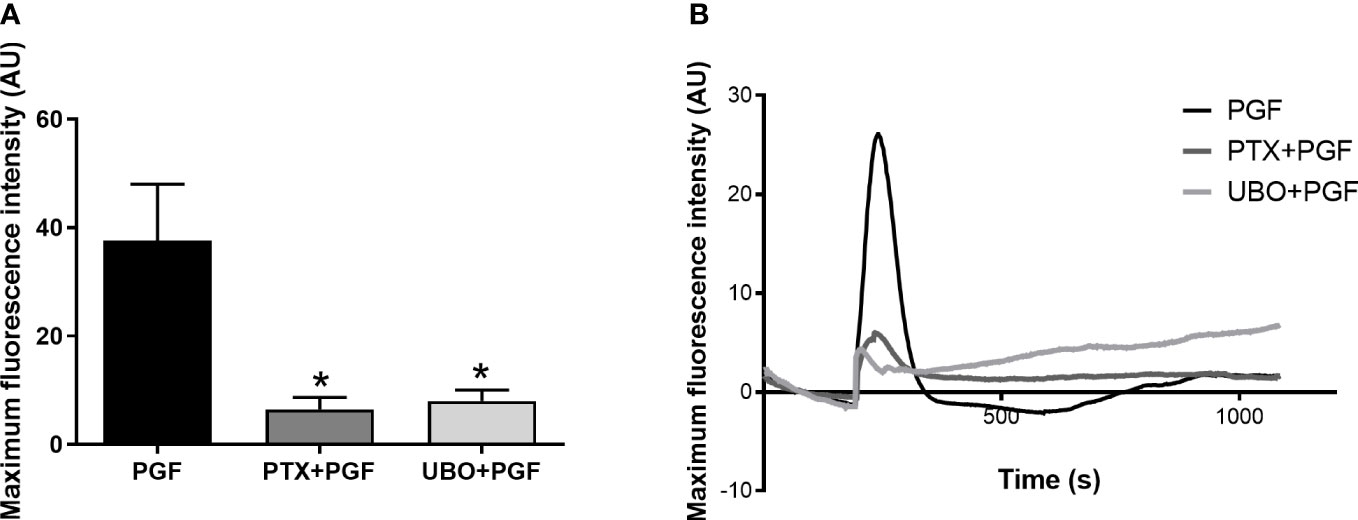
Figure 4 Gαi and Gαq coupling are involved in calcium response to prostaglandin F2α stimulation in myometrial cells. Primary myometrial cells isolated from term non-labouring myometrium were loaded with calcium sensitive dye, Fluo-4-Direct, and stimulated with PGF2α in the presence or absence of G protein inhibitors: PTX or UBO-QIC. Maximum fluorescence intensities (A) are expressed as mean ± SEM in arbitrary units (AU) (n=4, Kruskal-Wallis test with Dunn’s post-test). * p<0.05 vs NS or PGF2α-treated. Average plots (B) for mean fluorescent intensity for each treatment expressed in AU.
PGF2α-mediated calcium response plays a role in NF-κB activation and upregulation of COX-2
To examine the role of extracellular and intracellular calcium in pro-inflammatory signalling in myometrium, primary myometrial cells were pre-treated with extracellular calcium chelator EGTA (1mM) for 2 hours or intracellular chelator BAPTA-AM (10µM) for 30 minutes prior to stimulation with PGF2α (1µM). The concentration of calcium chelators was selected based on concentrations used in previously published studies (47, 52, 53). Depleting extra- or intra- cellular calcium with calcium chelators had distinctively different effects on PGF2α-mediated activation of NF-κB, MAP kinases and COX-2 expression in myometrial cells. There was a decreasing trend in p38 MAP kinase activation (70% decrease at 5 min) and a decrease in COX-2 expression (39% decrease at 6h) with the chelation of extracellular calcium (Figures 5B, D) but little effect on p65 and ERK activation (Figures 5A, C). The depletion of intracellular calcium with BAPTA-AM treatment significantly reduced the activation of p65 subunit of NF-κB (p<0.01 at 15 min) (Figure 6A), however, BAPTA-AM by itself appeared to induce MAP kinase activation (phospho-p38: p<0.05 at 30 min; phospho-ERK: p<0.001 at 30 min) and COX-2 expression (1.5-fold), therefore, it was not possible to determine the effects of intracellular calcium depletion on these proteins (Figures 6B–D). These results indicate that calcium originating from both outside the cell and intracellular stores may play a role in PGF2α-induced pro-inflammatory signalling.
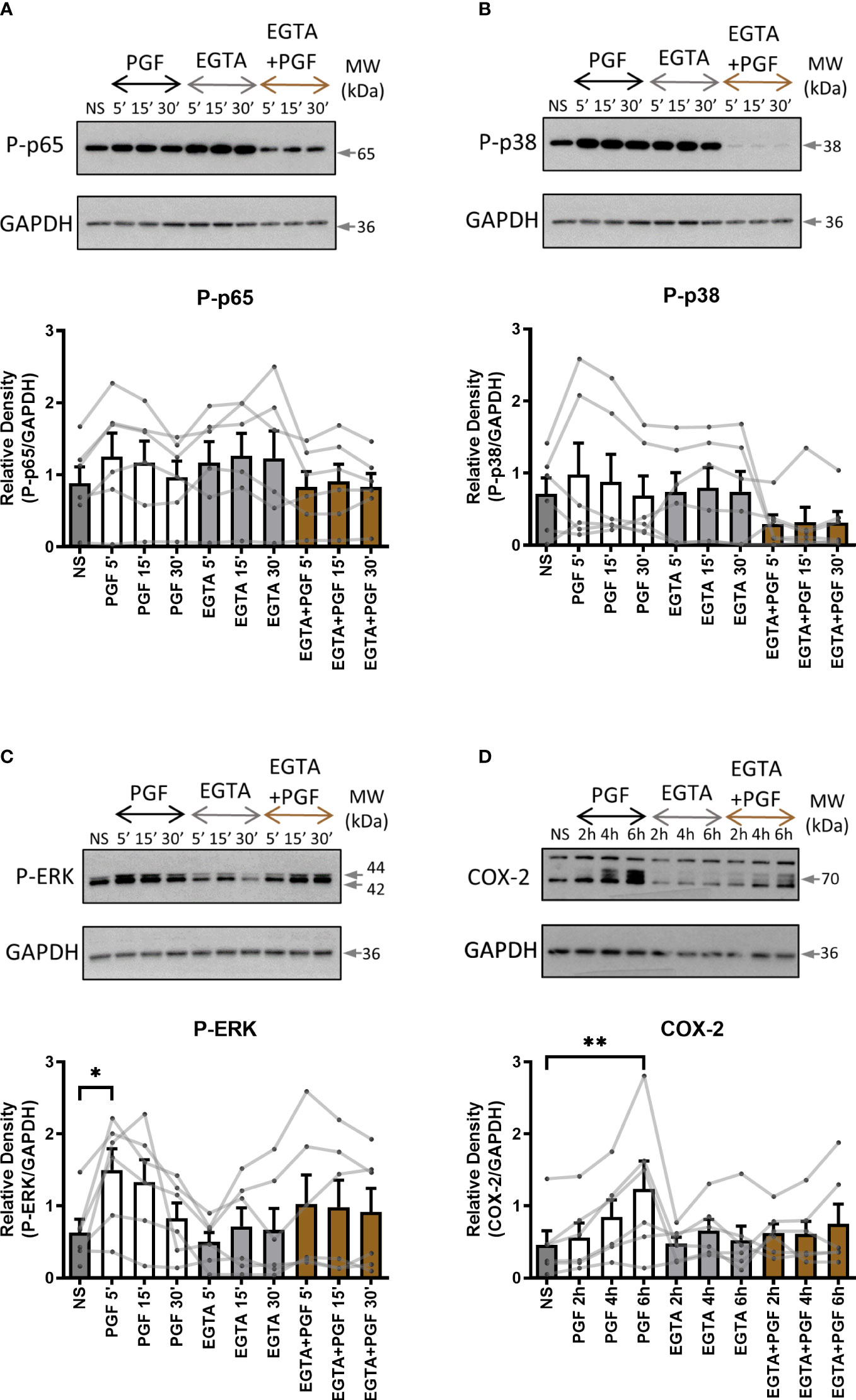
Figure 5 The role of extracellular calcium in prostaglandin F2α-mediated pro-inflammatory signalling. Primary myometrial smooth muscle cells isolated from term non-labouring myometrium were pre-treated with EGTA (1 mM) prior to stimulation with PGF2α. Changes in the protein levels of phospho-p65 (A), phospho-p38 (B), phospho-ERK (C) and COX-2 (D) were measured using Western blotting. Individual repeats are presented as dots connected with lines. Representative Western blots are shown above their corresponding graphs. Values are presented as mean ± SEM (n=6, * p < 0.05, ** p<0.01 vs NS, ANOVA with Tukey post-test).
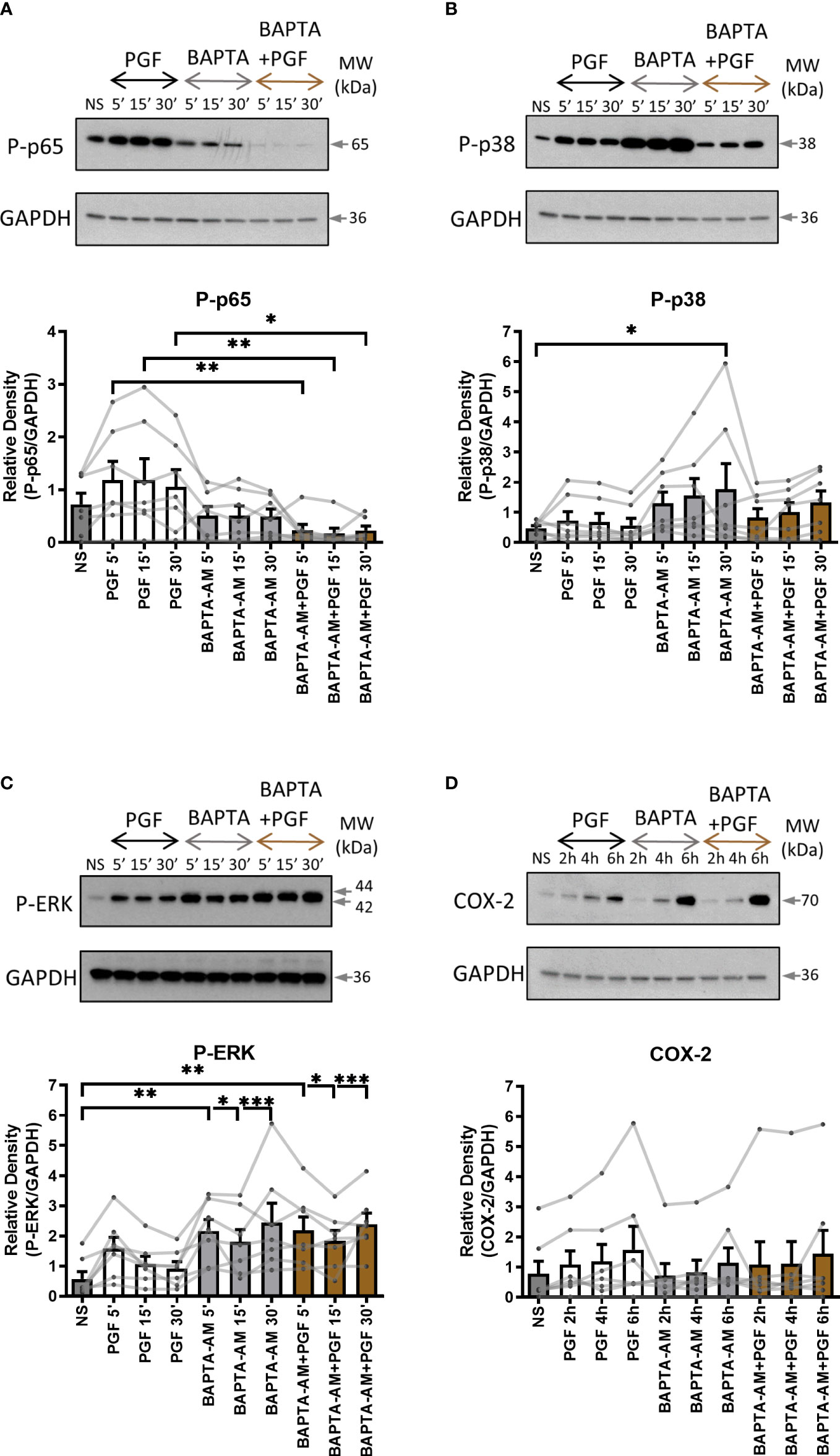
Figure 6 The chelation of extracellular and intracellular calcium decreases prostaglandin F2α-mediated activation of NF-κB. Primary myometrial smooth muscle cells isolated from term non-labouring myometrium were pre-treated with BAPTA-AM (10μM) prior to stimulation with PGF2α. Changes in the protein levels of phospho-p65 (A), phospho-p38 (B), phospho-ERK (C) and COX-2 (D) were measured using Western blotting. Individual repeats are presented as dots connected with lines. Representative Western blots are shown above their corresponding graphs. Values are presented as mean ± SEM (n=7, ANOVA with Tukey post-test, * p < 0.05, ** p<0.01, *** p<0.001 vs NS or PGF2α-treated).
PGF2α activates a range of transcription factors in myometrial cells
We have shown that PGF2α couples to Gαq and Gαi to regulate COX-2 expression. We have demonstrated that PGF2α activates NF-κB and MAP kinases. However, a wide range of transcription factors can be activated by G-protein and calcium signalling and these may be regulating COX-2 expression. Therefore, to identify the detailed mechanism involved in PGF2α-induced inflammation, protein/DNA array (TranSignal™ cAMP/Calcium Protein/DNA Array) was used to examine the effects of PGF2α on additional transcription factors associated with calcium response (n=2). Treatment of myometrial cells with PGF2α increased the activation of several calcium/cAMP-regulated transcription factors. Twenty transcription factors were tested and 14 were found to be upregulated by more than 2-fold (Figure 7). The stimulation of myometrial cells with PGF2α markedly increased the activation of AP1 (9.8-fold), C/EBP (19.6-fold), CREB (17.1-fold), GATA-3 (14.2-fold), GATA-4 (4.9-fold), NF-E1 (7.1-fold), and OCT-1 (5-fold). The following transcription factors were also upregulated by more than 2-fold with PGF2α treatment but to a smaller extent than the transcription factors above: ATF (2.9-fold), EGR (2.1-fold), HSE (3.1-fold), NF-κB (2.5-fold), Rel (2.3-fold) and Sp-1 (2.7-fold).
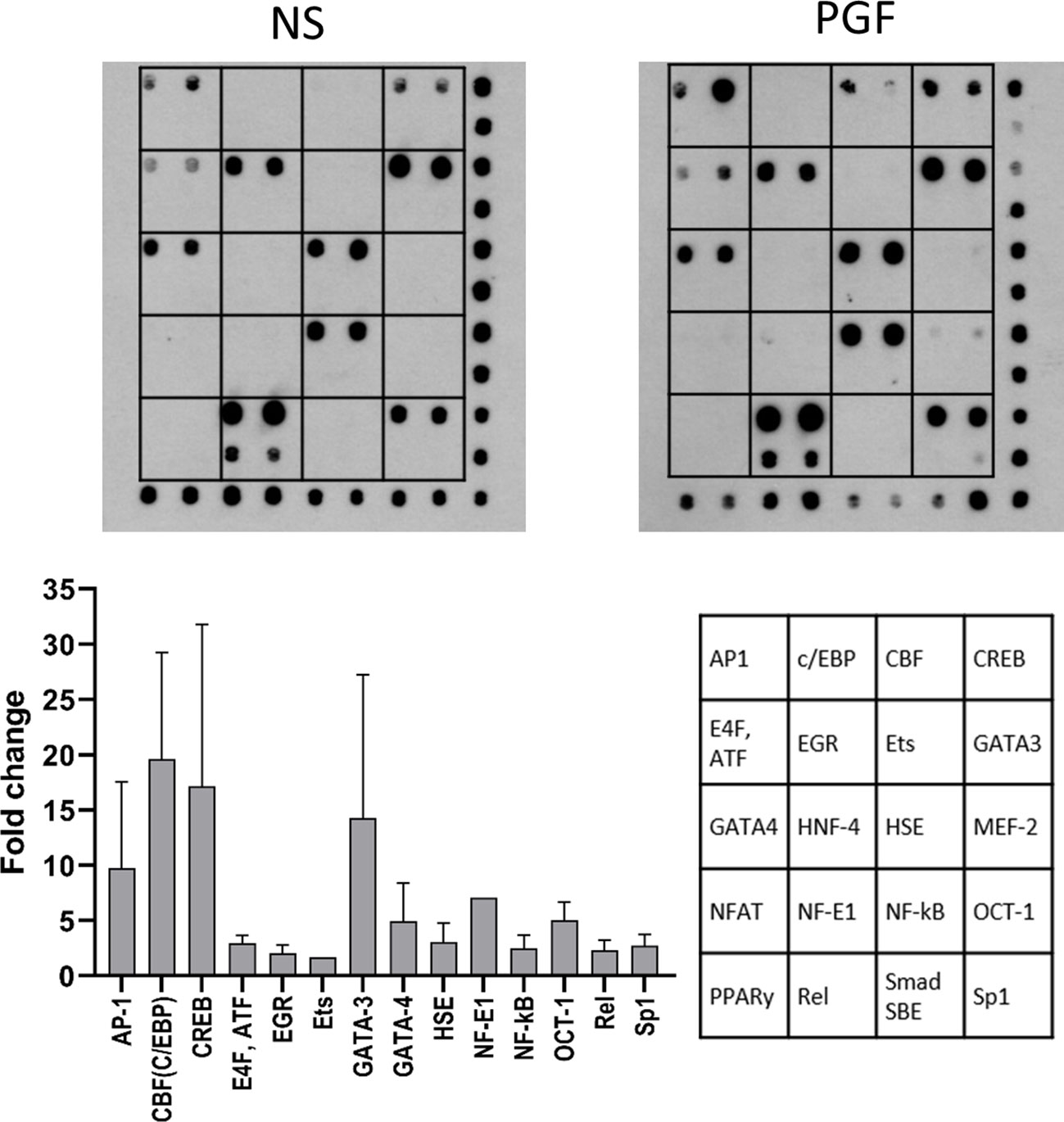
Figure 7 Prostaglandin F2α increases the activation of cAMP/calcium-regulated transcription factors. Primary myometrial cells isolated from term non-labouring myometrium were treated with PGF2α (1µM, 30 minutes). The activity of cAMP/calcium regulated transcription factors was determined using TranSignal cAMP/Calcium Protein/DNA Array. The graph shows the fold change increase for the transcription factors whose activity increased more than 1.5-fold. Values are presented as mean ± Range (n=2). Representative array dot blots for non-stimulated and PGF2α-treated samples are shown above. (AP-1 - Activator protein 1, C/EBP - CCAAT-enhancer-binding proteins, CBF - CCAAT-binding factor (CCAT- enhancer binding protein zeta), CREB - cAMP response element-binding protein, E4F, ATF - E4F transcription factor, Activating transcription factor family, EGR - Early growth response protein, Ets - Erythroblast transformation specific transcription factor family, GATA-3 - GATA binding protein 3 (Trans-acting T-cell-specific transcription factor GATA-3), GATA-4 - GATA binding protein 4, HNF-4 - Hepatocyte nuclear factor 4, HSE - Heat shock transcription factor, MEF-2 - Myocyte-specific enhancer factor 2A, NFAT - Nuclear factor of activated T-cells, NF-E1 - Yin-Yang-1 transcription factor, NF-κB - nuclear factor kappa-light-chain-enhancer of activated B cells, OCT-1 - Octamer-binding transcription factor 1 (POU domain, class 2, transcription factor 1), PPARγ - Peroxisome proliferator-activated receptor gamma, Rel - Proto-oncogene c-Rel, Smad SBE - Mothers against decapentaplegic homolog, SMAD binding elements, Sp1 - Specificity protein 1).
PGF2α activates calcium-regulated transcription factors via G protein coupling
From the protein-DNA array, we have seen a high level of upregulation in CREB and C/EBP following PGF2α stimulation. As the COX-2 promoter region contains binding sites for CREB and C/EBP-β transcription factors, we investigated the effects of PGF2α on cAMP/calcium-regulated transcription factors CREB and C/EBP-β. The results showed increased levels of phospho-CREB as early as 5 minutes after PGF2α treatment and remained elevated for the duration of the treatment (p<0.05 at 30 min) (Figure 8A). The levels of the activating C/EBP-β LAP subunit increased following 5 minutes (p<0.05) of treatment and increased further during longer treatment time periods (2.5-fold increase, p<0.0001 at 6h), whereas the levels of the inhibitory C/EBP-β LIP subunit were increased to a lesser extent than C/EBP-β LAP (1.6-fold increase, p<0.01 at 6h) (Figure 8B) resulting in an increase in C/EBP-β LAP to LIP ratio over time.
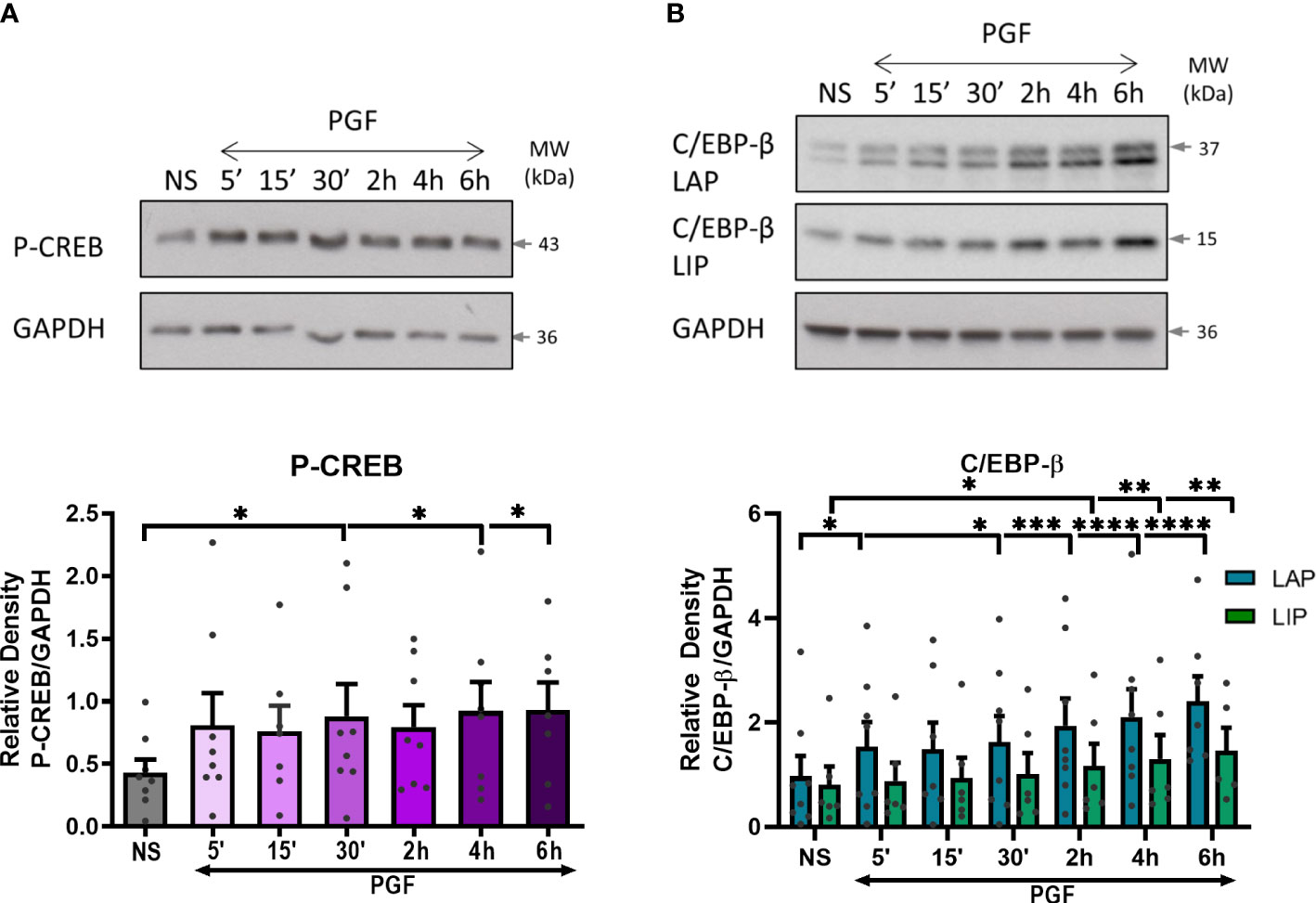
Figure 8 Prostaglandin F2α increases CREB and C/EBP-β activation in myometrium. Primary myometrial cells isolated from term non-labouring myometrium were treated with PGF2α (1μM) for 5, 15, 30 minutes and 2, 4, 6 hours. Changes in the protein levels of phospho-CREB (A) and C/EBP-β (B) were measured using Western blotting. Individual repeats are presented as dots. Representative Western blots (including the loading control, GAPDH) are shown above their corresponding densitometry graphs. Values are presented as mean ± SEM (n=5, ANOVA with Dunnett’s post-test; * p<0.05, ** p<0.01, *** p<0.001, **** p<0.0001 vs NS).
To determine the role of Gαq and Gαi proteins in PGF2α-mediated activation of CREB and C/EBP-β, myometrial cells were pre-treated with either Gαi or Gαq inhibitor. There was no decrease in CREB or C/EBP-β activation with Gαi inhibition (Figures 9A, B). However, there was a decreasing trend in the activation of C/EBP-β LAP with Gαq inhibition (43% decrease at 6h) but no effect on CREB activation (Figures 9C, D), suggesting that PGF2α-mediated activation of C/EBP-β could possibly occur via Gαq signalling pathways.
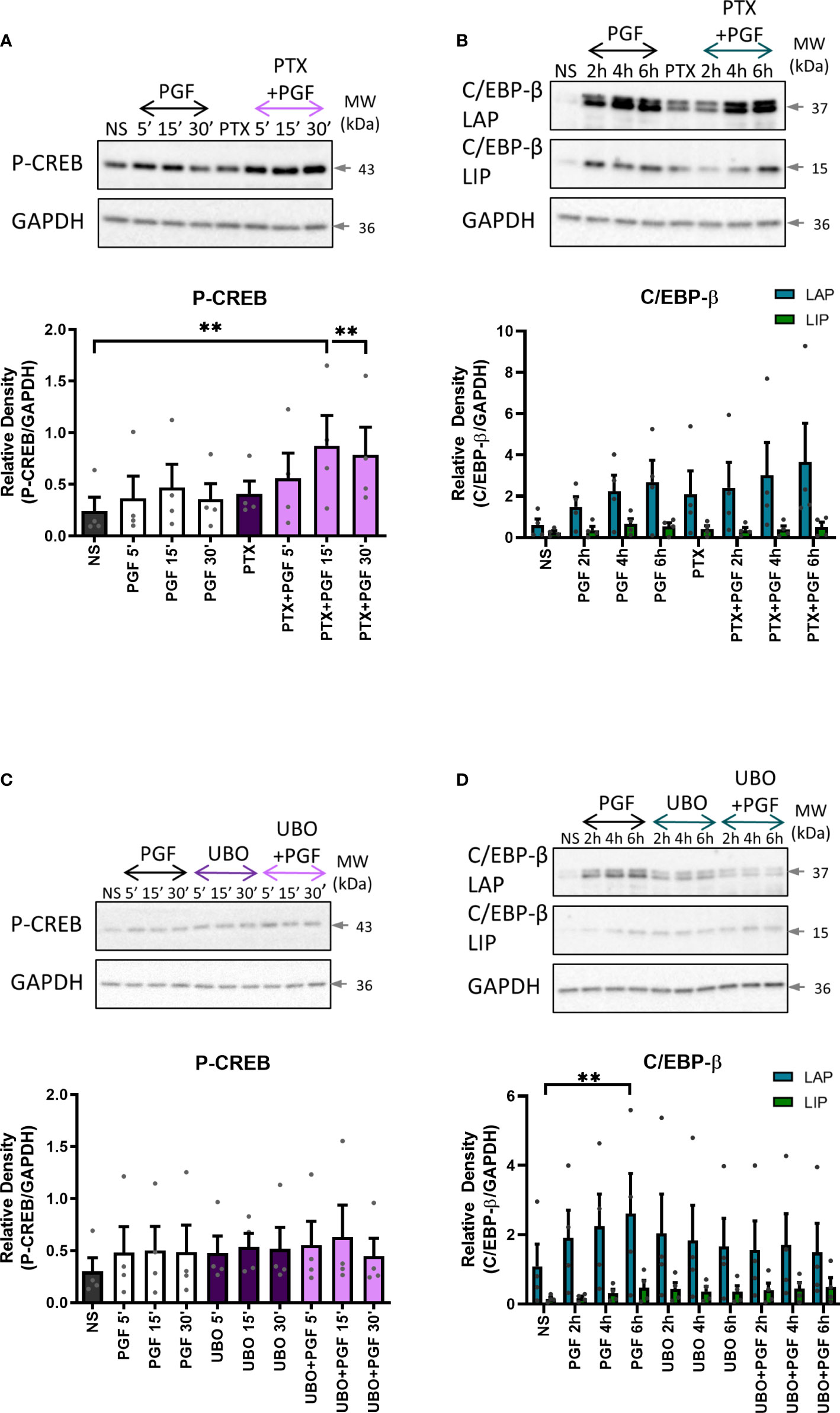
Figure 9 The role of Gαi and Gαq protein coupling in prostaglandin F2α-mediated activation of cAMP/calcium-dependent transcription factors. Primary myometrial cells isolated from term non-labouring myometrium were pre-treated with pertussis toxin (200ng/ml) (A, B) or UBO-QIC (1μM) (C, D) prior to stimulation with PGF2α (1μM). Changes in the protein levels of phospho-CREB (A, C) and C/EBP-β (B, D) were measured using Western blotting. Individual repeats are presented as dots. Representative Western blots are shown above their corresponding densitometry graphs. Values are presented as mean ± SEM (n=4, ANOVA with Tukey post-test, ** p<0.01 vs NS).
Discussion
It is widely accepted that term and preterm labour are preceded by inflammatory activation within the uterine tissues. Building on our current understanding of PGF2α as an inflammatory mediator, we have demonstrated that PGF2α activates the calcium response and pro-inflammatory signalling pathways in human myometrium through Gαq and also Gαi coupling of the FP receptor (Figure 10). PGF2α increases the activation of NF-κB, MAP kinases, CREB and C/EBP-β, and in turn upregulates the expression of cytokines and chemokines as well as COX-2. PGF2α-mediated COX-2 upregulation has been shown to require both Gαi and Gαq protein coupling of the FP receptor. Additionally, we have shown that the calcium response to PGF2α stimulation in myometrial cells occurs via Gαq and Gαi-mediated pathways.
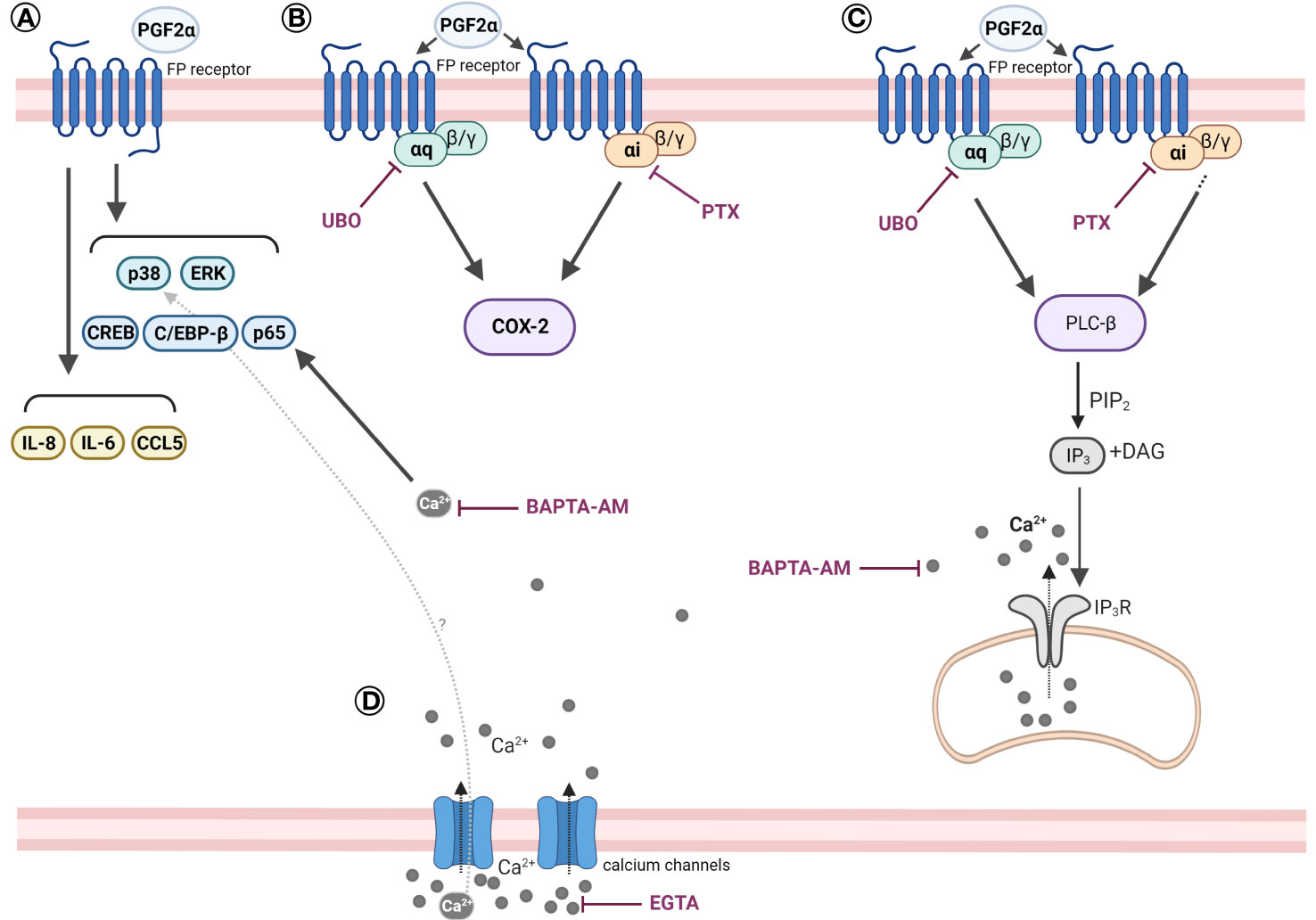
Figure 10 Scheme summarising the effects of PGF2α in human myometrial smooth muscle cells. (A) PGF2α induces pro-inflammatory signalling in myometrial cells. PGF2α increases the activation of NF-κB, MAP kinases, CREB, and C/EBP-β. PGF2α upregulates the expression of IL-8, IL-6, CCL5. (B) PGF2α-mediated COX-2 upregulation requires both Gαi and Gαq protein coupling of the FP receptor. (C) PGF2α stimulation triggers a calcium response in myometrial cells via Gαq and Gαi-mediated pathways. (D) PGF2α-mediated calcium response plays a role in NF-κB activation. Extracellular calcium influx might play a role in PGF2α-mediated p38 MAP kinase activation. Created with BioRender.com. BAPTA-AM, 1,2-bis(o-Aminophenoxy)ethane-N,N,N’,N’-tetraacetic acid tetrakis(acetoxymethyl ester) (calcium chelator); CCL5, Chemokine (C-C motif) ligand 5; C/EBP-β, CCAAT/enhancer-binding protein beta; COX-2, Cyclooxygenase 2; CREB, cAMP response element-binding protein; DAG, Diacylglycerol; EGTA, Ethylene glycol-bis(β-aminoethyl ether)-N,N,N’,N’-tetraacetic acid (calcium chelator); ERK, Extracellular signal-regulated kinase; IL-6, Interleukin 6; IL-8, Interleukin 8; IP3, Inositol trisphosphate; IP3R, Inositol trisphosphate receptor; PGF2α, Prostaglandin F2α; PIP2, Phosphatidylinositol 4,5-bisphosphate; PLC-β, Phospholipase C beta; PTX, Pertussis toxin; p38, p38 mitogen-activated protein kinase; UBO, UBO-QIC (FR900359).
PGF2α is a potent uterotonin, however, the role of PGF2α goes beyond the stimulation of myometrial contractions. PGF2α plays an important signalling role during uterine transformation prior to labour onset. We found that in myometrial cells, PGF2α increased the activation of p65 subunit of NF-κB, which is a prototypical pro-inflammatory transcription factor. It regulates the expression of several pro-inflammatory and pro-labour genes and thus plays an important role in the onset of term and preterm labour (54–56). Additionally, PGF2α increases transcription and protein levels of the inducible COX-2 enzyme. This indicates that there is a positive feed-forward loop between PGF2α and COX-2 in myometrium which is complementary to the findings of Xu et al. (39). Growing evidence suggests that parturition is characterised by infiltration of leukocytes into the uterus leading to increased expression and release of pro-inflammatory cytokines and chemokines (23, 57, 58). PGF2α can contribute to the establishment of a pro-labour environment in the uterus through promoting an increase in expression of pro-inflammatory and chemotactic cytokines such IL-8, IL-6, CCL5, IL-1β and CCL2. The increased activation of NF-κB and MAP kinases in response to PGF2α stimulation might be one of the driving forces for the increased cytokine output by myometrial cells. Several genes encoding pro-inflammatory cytokines and chemokines contain the NF-κB binding site in their promoters (55, 59). NF-κB activation upregulates genes for CCL2, CCL5, IL-8 and IL-6 (56). Moreover, it has been reported that IL-1β, IL-6 and CCL2 production by myocytes is ERK-dependent, with the production of IL-1β and CCL2 also being NF-κB-dependent (40). Increased levels of chemotactic cytokines in the uterus promote the recruitment of more leukocytes to further increase additional cytokine production (60–62), resulting in a positive feed-forward loop (40, 63). Pro-inflammatory cytokines IL-1β and IL-6, which are released by invading leukocytes, have the ability to activate NF-κB and C/EBP, resulting in subsequent expression of inflammatory mediators and uterine activation proteins such as OT receptor and COX-2 (30, 64, 65).
Our study indicates that the activity of the PGF2α receptor in human myometrium is mediated by coupling to several G proteins. Sales et al. have previously reported that in endometrial adenocarcinoma cell line, PGF2α-mediated upregulation of COX-2 occurs via Gαq signalling pathway (66). Here we report that inhibition of Gαq and Gαi coupling reduces PGF2α-induced COX-2 expression in human myocytes, therefore indicating that both G proteins are required to mediate the effects of PGF2α on COX-2 expression. Similar to our findings, Ohmichi et al. reported that in addition to traditional Gαq coupling, PGF2α has the ability to activate MAPK through Gβγ originating from Gαi coupling in rat puerperal myometrial cells (41). Studies in rat hepatocytes and rabbit kidney cells have suggested that the FP receptor can couple to Gαi protein (67, 68).
Our previous studies are indicative of potential crosstalk between PGF2α and oxytocin (OT) receptors. We reported that oxytocin receptor antagonists atosiban and nolasiban were able to inhibit both OT-induced and PGF2α-induced myometrial contractions and a novel tocolytic, OBE002, an FP receptor antagonist, reduced PGF2α-induced and OT-induced contractions (45, 69). The FP receptor has been previously reported to form heterodimers with angiotensin II type I receptor (AT1R) in HEK293 cells and vascular smooth muscle cells with the heteromer showing marked differences in signalling in comparison to monomers (70, 71). Direct physical interaction between the FP receptor and the OT receptor has not been studied or reported before, however functional crosstalk can also occur in the absence of physical interaction (72). This receptor crosstalk could affect many aspects of PGF2α-regulated signalling pathways as the OT receptor couples to several G proteins (48, 73). OT-mediated Gαq coupling leads to increased myometrial contractility, however, it has been shown that oxytocin mediates inflammatory effects in human gestational tissues via Gαi activation (48). It is therefore possible that Gαi-mediated effects on COX-2 that we have observed in response to PGF2α stimulation could be a result of FP receptor and OT receptor interaction. Further studies are needed to examine the presence of FP/OT receptor heteromers and to determine the functional downstream implications of such interaction.
Uterine contractions are initiated through an increase in intracellular calcium concentrations in myometrial cells (74). It has been well established that FP receptor coupling to Gαq leads to the mobilisation of intracellular calcium (42, 75). Our findings suggest that coupling of the FP receptor to both Gαq and Gαi proteins can trigger an increase in intracellular calcium concentration in myocytes. Typically, a receptor coupling to Gαq protein family leads to PLCβ activation which converts phosphatidylinositol 4,5-bisphosphate (PIP2) into diacylglycerol (DAG) and inositol trisphosphate (IP3), leading to a stimulation of calcium release from intracellular stores. However, in addition to this traditional pathway of PLCβ activation, it has been previously reported that PLCβ isoforms can be activated by Gβγ subunit which originates from Gαi complex (76–79). As we have shown that Gαi coupling plays a role in PGF2α-induced COX-2 expression, it is possible that this alternative pathway can at least partially mediate the effects of PGF2α on PLCβ activation and subsequent calcium response. Our results indicate that the FP receptor in myometrium couples to Gαq and Gαi proteins. Further investigation of G protein coupling to a wider range of G proteins using primary myometrial cells with knocked down individual G proteins would help us complete the picture of PGF2α-mediated pro-inflammatory signalling in myometrium, as the understanding the signalling pathways involved in PGF2α-mediated effects is essential to our understanding of parturition.
PGF2α, acting through its receptor elicits a strong calcium response in myometrial cells, mainly through release of calcium from stores. Several reports suggest that PGF2α stimulation of myometrial cells leads to an influx of calcium ions from extracellular space either through voltage-gated calcium channels or capacitative/store-controlled calcium entry (51, 75). PGF2α also triggers calcium release from intracellular stores, which is mediated through PLCβ activation (42, 51, 80–83). Our results indicate that calcium participates as a second messenger in pro-inflammatory signalling pathways. PGF2α-stimulated activation of NF-κB is affected by availability of intracellular calcium whereas extracellular calcium might play a role in PGF2α-induced p38 MAPK activation and COX-2 expression. Calcium-dependent agonist-induced activation of transcription factors has been previously reported in literature in several cell types (84–89). Through a transcription factor array, we have identified that PGF2α activates 14 out of 20 calcium-regulated transcription factors (90). Of these, C/EBP-β activation by PGF2α has been shown to be via Gαq coupling which could drive COX-2 expression. Transcriptional regulation of COX-2 by C/EBP-β has been previously demonstrated in various cell types such as fibroblasts, vascular and cardiac endothelial cells (91). Overall, our results suggest that PGF2α-mediated signalling may require presence of calcium ions to regulate various aspects of the pro-inflammatory response.
This study has potential limitations. The aim of the study was to investigate PGF2α-induced pro-inflammatory signalling in myometrium in order to evaluate FP receptor-mediated signalling pathways as potential targets for preterm labour treatment and prevention. Our study investigated responses to PGF2α stimulation in cells isolated from lower uterine segment. Although, uterine regional differences in pro-labour gene expression have been reported (92), primary myometrial smooth muscle cells from upper and lower segment have the ability to respond to stimulation with IL-1β and PGF2α resulting in increases in COX-2 expression and cytokine production (39, 40, 92). We have also previously demonstrated that activation of NF-κB occurs in both upper and lower segment of the uterus (56). However, it would be beneficial to study and compare the G protein activation and calcium responses in the cells from the upper uterine segment in the future. Another limitation of our study is a wide inter-sample variability which may be confounding some of the results. The myometrial smooth muscle cells used in this study were isolated from term non-labouring uterine tissue, however some patients may have been closer to labour onset than others. Towards the end of gestation, the uterus undergoes an array of phenotypic changes that culminate in labour (93). Transcriptional and gene expression analysis revealed differences in gene expression between labouring and non-labouring myometrial tissue (19, 94). Basal level of uterine activation therefore differs between patient samples and can alter their ability to respond to stimuli (55).
PGF2α plays a major role in pathophysiology of preterm labour through its role in inflammatory activation of myometrium and via stimulation of myometrial contractility. The FP receptor therefore appears to be a good target for pharmacological management of preterm labour. However, the signalling pleiotropy associated with GPCRs presents a challenge in designing effective therapeutics. A widely used OTR antagonist, atosiban, was shown to activate pro-inflammatory pathways in amnion epithelial cells through biased agonist effect on the OT receptor (48). Moreover, a study by Fillion et al. proposed asymmetric recruitment of β-arrestins in AT1R/FP dimers (70). These studies collectively highlight the need for the development of compounds that would modify specific signalling pathways, particularly when targeting G protein-coupled receptors.
In summary, this study has demonstrated the induction of pro-inflammatory response by PGF2α in primary human myometrial cells. The results of our study suggest that upregulation of COX-2 by PGF2α may be linked to upstream activation of MAP kinase, NF-κB and C/EBP-β, and involves calcium-dependent signal transduction by Gαi and Gαq proteins. These findings contribute to our understanding of myometrial preparation for the onset of labour and highlight the need to further investigate myometrium-specific mechanisms in order to develop tocolytic drugs with better uterine selectivity.
Data availability statement
The raw data supporting the conclusions of this article will be made available by the authors, without undue reservation.
Ethics statement
The studies involving human participants were reviewed and approved by Riverside Ethics Committee (Ref 3358), London Harrow Research Ethics Committee (Ref 19/LO/1657). The patients/participants provided their written informed consent to participate in this study.
Author contributions
LR, SK, AH, LS, DM, PB and VT contributed to conception and design of the study. LR performed the experiments. LR, SK and VT analysed and interpreted the data. LR and SK wrote the first draft of the manuscript and prepared the figures. All authors contributed to critical revision of the manuscript, read and approved the submitted version.
Funding
This work was supported by Genesis Research Trust (Grant ref. P08006), ObsEva (Grant ref. P61689), the National Institute for Health Research Biomedical Research Centre (NIHR BRC) based at Imperial Healthcare NHS Trust (Grant ref. P45272) and Imperial College London.
Acknowledgments
The authors would like to acknowledge that a part of this work was previously published in a PhD thesis.
Conflict of interest
The PhD project of LR was partially funded by ObsEva. PB is a consultant to ObsEva and holds shares in the company.
The remaining authors declare that the research was conducted in the absence of any commercial or financial relationships that could be construed as a potential conflict of interest.
Publisher’s note
All claims expressed in this article are solely those of the authors and do not necessarily represent those of their affiliated organizations, or those of the publisher, the editors and the reviewers. Any product that may be evaluated in this article, or claim that may be made by its manufacturer, is not guaranteed or endorsed by the publisher.
References
1. Howson CP, Kinney MV, McDougall L, Lawn JE. Born too soon preterm birth action g. born too soon: preterm birth matters. Reprod Health (2013) 10 Suppl 1:S1. doi: 10.1186/1742-4755-10-S1-S1
2. Blencowe H, Cousens S, Chou D, Oestergaard M, Say L, Moller AB, et al. Born too soon: the global epidemiology of 15 million preterm births. Reprod Health (2013) 10 Suppl 1:S2. doi: 10.1186/1742-4755-10-S1-S2
3. Challis JRG, Matthews SG, Gibb W, Lye SJ. Endocrine and paracrine regulation of birth at term and preterm. Endocr Rev (2000) 21(5):514–50. doi: 10.1210/edrv.21.5.0407
4. Vannuccini S, Bocchi C, Severi FM, Challis JR, Petraglia F. Endocrinology of human parturition. Ann Endocrinol (Paris) (2016) 77(2):105–13. doi: 10.1016/j.ando.2016.04.025
5. Pique-Regi R, Romero R, Garcia-Flores V, Peyvandipour A, Tarca AL, Pusod E, et al. A single-cell atlas of the myometrium in human parturition. JCI Insight (2022) 7(5):e153921. doi: 10.1172/jci.insight.153921
6. Renthal NE, Williams KC, Montalbano AP, Chen CC, Gao L, Mendelson CR. Molecular regulation of parturition: a myometrial perspective. Cold Spring Harb Perspect Med (2015) 5(11):a023069. doi: 10.1101/cshperspect.a023069
7. Ilicic M, Zakar T, Paul JW. The regulation of uterine function during parturition: an update and recent advances. Reprod Sci (2020) 27(1):3–28. doi: 10.1007/s43032-019-00001-y
8. Ulrich CC, Arinze V, Wandscheer CB, Copley Salem C, Nabati C, Etezadi-Amoli N, et al. Matrix metalloproteinases 2 and 9 are elevated in human preterm laboring uterine myometrium and exacerbate uterine contractility. Biol Reprod (2019) 100(6):1597–604. doi: 10.1093/biolre/ioz054
9. Maymon E, Romero R, Pacora P, Gomez R, Mazor M, Edwin S, et al. A role for the 72 kda gelatinase (Mmp-2) and its inhibitor (Timp-2) in human parturition, premature rupture of membranes and intraamniotic infection. J Perinat Med (2001) 29(4):308–16. doi: 10.1515/JPM.2001.044
10. Sheldon RE, Mashayamombe C, Shi SQ, Garfield RE, Shmygol A, Blanks AM, et al. Alterations in gap junction Connexin43/Connexin45 ratio mediate a transition from quiescence to excitation in a mathematical model of the myometrium. J R Soc Interface (2014) 11(101):20140726. doi: 10.1098/rsif.2014.0726
11. McCloskey C, Rada C, Bailey E, McCavera S, van den Berg HA, Atia J, et al. The inwardly rectifying k+ channel Kir7.1 controls uterine excitability throughout pregnancy. EMBO Mol Med (2014) 6(9):1161–74. doi: 10.15252/emmm.201403944
12. Shchuka VM, Abatti LE, Hou H, Khader N, Dorogin A, Wilson MD, et al. The pregnant myometrium is epigenetically activated at contractility-driving gene loci prior to the onset of labor in mice. PloS Biol (2020) 18(7):e3000710. doi: 10.1371/journal.pbio.3000710
13. Masson LE, O'Brien CM, Gautam R, Thomas G, Slaughter JC, Goldberg M, et al. In vivo raman spectroscopy monitors cervical change during labor. Am J Obstet Gynecol (2022) 227(2):275 e1– e14. doi: 10.1016/j.ajog.2022.02.019
14. Choi SJ, Jung KL, Oh SY, Kim JH, Roh CR. Cervicovaginal matrix metalloproteinase-9 and cervical ripening in human term parturition. Eur J Obstet Gynecol Reprod Biol (2009) 142(1):43–7. doi: 10.1016/j.ejogrb.2008.08.009
15. Yoshida K, Jayyosi C, Lee N, Mahendroo M, Myers KM. Mechanics of cervical remodelling: insights from rodent models of pregnancy. Interface Focus (2019) 9(5):20190026. doi: 10.1098/rsfs.2019.0026
16. Yellon SM. Contributions to the dynamics of cervix remodeling prior to term and preterm birth. Biol Reprod (2017) 96(1):13–23. doi: 10.1095/biolreprod.116.142844
17. Flores-Espinosa P, Olmos-Ortiz A, Granados-Cepeda M, Quesada-Reyna B, Vega-Sanchez R, Velazquez P, et al. Prolactin protects the structural integrity of human fetal membranes by downregulating inflammation-induced secretion of matrix metalloproteinases. Immunol Invest (2022) 51(5):1313–29. doi: 10.1080/08820139.2021.1936012
18. Choudhury RH, Dunk CE, Lye SJ, Harris LK, Aplin JD, Jones RL. Decidual leucocytes infiltrating human spiral arterioles are rich source of matrix metalloproteinases and degrade extracellular matrix in vitro and in situ. Am J Reprod Immunol (2019) 81(1):e13054. doi: 10.1111/aji.13054
19. Sharp GC, Hutchinson JL, Hibbert N, Freeman TC, Saunders PT, Norman JE. Transcription analysis of the myometrium of labouring and non-labouring women. PloS One (2016) 11(5):e0155413. doi: 10.1371/journal.pone.0155413
20. Sivarajasingam SP, Imami N, Johnson MR. Myometrial cytokines and their role in the onset of labour. J Endocrinol (2016) 231(3):R101–R19. doi: 10.1530/JOE-16-0157
21. Romero R, Espinoza J, Goncalves LF, Kusanovic JP, Friel L, Hassan S. The role of inflammation and infection in preterm birth. Semin Reprod Med (2007) 25(1):21–39. doi: 10.1055/s-2006-956773
22. Young A, Thomson AJ, Ledingham M, Jordan F, Greer IA, Norman JE. Immunolocalization of proinflammatory cytokines in myometrium, cervix, and fetal membranes during human parturition at term. Biol Reprod (2002) 66(2):445–9. doi: 10.1095/biolreprod66.2.445
23. Osman I, Young A, Ledingham MA, Thomson AJ, Jordan F, Greer IA, et al. Leukocyte density and pro-inflammatory cytokine expression in human fetal membranes, decidua, cervix and myometrium before and during labour at term. Mol Hum Reprod (2003) 9(1):41–5. doi: 10.1093/molehr/gag001
24. Hua R, Pease JE, Sooranna SR, Viney JM, Nelson SM, Myatt L, et al. Stretch and inflammatory cytokines drive myometrial chemokine expression Via nf-kappab activation. Endocrinology (2012) 153(1):481–91. doi: 10.1210/en.2011-1506
25. Gomez-Lopez N, Tanaka S, Zaeem Z, Metz GA, Olson DM. Maternal circulating leukocytes display early chemotactic responsiveness during late gestation. BMC Pregnancy Childbirth (2013) 13 Suppl 1(Suppl 1):S8. doi: 10.1186/1471-2393-13-S1-S8
26. Peltier MR. Immunology of term and preterm labor. Reprod Biol Endocrinol (2003) 1:122. doi: 10.1186/1477-7827-1-122
27. Friebe-Hoffmann U, Chiao JP, Rauk PN. Effect of il-1beta and il-6 on oxytocin secretion in human uterine smooth muscle cells. Am J Reprod Immunol (2001) 46(3):226–31. doi: 10.1034/j.1600-0897.2001.d01-6.x
28. Rauk PN, Friebe-Hoffmann U, Winebrenner LD, Chiao JP. Interleukin-6 up-regulates the oxytocin receptor in cultured uterine smooth muscle cells. Am J Reprod Immunol (2001) 45(3):148–53. doi: 10.1111/j.8755-8920.2001.450305.x
29. Leimert KB, Verstraeten BSE, Messer A, Nemati R, Blackadar K, Fang X, et al. Cooperative effects of sequential Pgf(2 alpha) and il-1 beta on il-6 and cox-2 expression in human myometrial cells. Biol Reprod (2019) 100(5):1370–85. doi: 10.1093/biolre/ioz029
30. Christiaens I, Zaragoza DB, Guilbert L, Robertson SA, Mitchell BF, Olson DM. Inflammatory processes in preterm and term parturition. J Reprod Immunol (2008) 79(1):50–7. doi: 10.1016/j.jri.2008.04.002
31. Challis JRG. Mechanism of parturition and preterm labor. Obstet Gynecol Surv (2000) 55(10):650–60. doi: 10.1097/00006254-200010000-00025
32. Olson DM, Zaragoza DB, Shallow MC, Cook JL, Mitchell BF, Grigsby P, et al. Myometrial activation and preterm labour: evidence supporting a role for the prostaglandin f receptor–a review. Placenta (2003) 24 Suppl A:S47–54. doi: 10.1053/plac.2002.0938
33. Zakar T, Hertelendy F. Regulation of prostaglandin synthesis in the human uterus. J Matern Fetal Med (2001) 10(4):223–35. doi: 10.1080/714904332
34. McLaren J, Taylor DJ, Bell SC. Prostaglandin E(2)-dependent production of latent matrix metalloproteinase-9 in cultures of human fetal membranes. Mol Hum Reprod (2000) 6(11):1033–40. doi: 10.1093/molehr/6.11.1033
35. Fletcher HM, Mitchell S, Simeon D, Frederick J, Brown D. Intravaginal misoprostol as a cervical ripening agent. Br J Obstet Gynaecol (1993) 100(7):641–4. doi: 10.1111/j.1471-0528.1993.tb14230.x
36. Yoshida M, Sagawa N, Itoh H, Yura S, Takemura M, Wada Y, et al. Prostaglandin F(2alpha), cytokines and cyclic mechanical stretch augment matrix metalloproteinase-1 secretion from cultured human uterine cervical fibroblast cells. Mol Hum Reprod (2002) 8(7):681–7. doi: 10.1093/molehr/8.7.681
37. Yellon SM. Immunobiology of cervix ripening. Front Immunol (2019) 10:3156. doi: 10.3389/fimmu.2019.03156
38. Xu C, You X, Liu W, Sun Q, Ding X, Huang Y, et al. Prostaglandin F2alpha regulates the expression of uterine activation proteins Via multiple signalling pathways. Reproduction (2015) 149(1):139–46. doi: 10.1530/REP-14-0479
39. Xu C, Long A, Fang X, Wood SL, Slater DM, Ni X, et al. Effects of Pgf2alpha on the expression of uterine activation proteins in pregnant human myometrial cells from upper and lower segment. J Clin Endocrinol Metab (2013) 98(7):2975–83. doi: 10.1210/jc.2012-2829
40. Xu C, Liu W, You X, Leimert K, Popowycz K, Fang X, et al. Pgf2alpha modulates the output of chemokines and pro-inflammatory cytokines in myometrial cells from term pregnant women through divergent signaling pathways. Mol Hum Reprod (2015) 21(7):603–14. doi: 10.1093/molehr/gav018
41. Ohmichi M, Koike K, Kimura A, Masuhara K, Ikegami H, Ikebuchi Y, et al. Role of mitogen-activated protein kinase pathway in prostaglandin F2alpha-induced rat puerperal uterine contraction. Endocrinology (1997) 138(8):3103–11. doi: 10.1210/endo.138.8.5305
42. Carrasco MP, Phaneuf S, Asboth G, Lopez Bernal A. Fluprostenol activates phospholipase c and Ca2+ mobilization in human myometrial cells. J Clin Endocrinol Metab (1996) 81(6):2104–10. doi: 10.1210/jcem.81.6.8964835
43. Fujino H, Pierce KL, Srinivasan D, Protzman CE, Krauss AH, Woodward DF, et al. Delayed reversal of shape change in cells expressing Fp(B) prostanoid receptors. possible role of receptor resensitization. J Biol Chem (2000) 275(38):29907–14. doi: 10.1074/jbc.M003467200
44. Sanborn BM, Yue C, Wang W, Dodge KL. G Protein signalling pathways in myometrium: affecting the balance between contraction and relaxation. Rev Reprod (1998) 3(3):196–205. doi: 10.1530/ror.0.0030196
45. Kim SH, Riaposova L, Ahmed H, Pohl O, Chollet A, Gotteland JP, et al. Oxytocin receptor antagonists, atosiban and nolasiban, inhibit prostaglandin F2alpha-induced contractions and inflammatory responses in human myometrium. Sci Rep (2019) 9(1):5792. doi: 10.1038/s41598-019-42181-2
46. Liu T, Zhang L, Joo D, Sun S-C. Nf-Kb signaling in inflammation. Signal Transduction Targeted Ther (2017) 2(1):17023. doi: 10.1038/sigtrans.2017.23
47. Kandola MK, Sykes L, Lee YS, Johnson MR, Hanyaloglu AC, Bennett PR. Ep2 receptor activates dual G protein signaling pathways that mediate contrasting proinflammatory and relaxatory responses in term pregnant human myometrium. Endocrinology (2014) 155(2):605–17. doi: 10.1210/en.2013-1761
48. Kim SH, MacIntyre DA, Hanyaloglu AC, Blanks AM, Thornton S, Bennett PR, et al. The oxytocin receptor antagonist, atosiban, activates pro-inflammatory pathways in human amnion Via G(Alphai) signalling. Mol Cell Endocrinol (2016) 420:11–23. doi: 10.1016/j.mce.2015.11.012
49. Lieb S, Littmann T, Plank N, Felixberger J, Tanaka M, Schafer T, et al. Label-free versus conventional cellular assays: functional investigations on the human histamine H(1) receptor. Pharmacol Res (2016) 114:13–26. doi: 10.1016/j.phrs.2016.10.010
50. Jonas KC, Chen S, Virta M, Mora J, Franks S, Huhtaniemi I, et al. Temporal reprogramming of calcium signalling Via crosstalk of gonadotrophin receptors that associate as functionally asymmetric heteromers. Sci Rep (2018) 8(1):1–11. doi: 10.1038/s41598-018-20722-5
51. Ruttner Z, Ivanics T, Slaaf DW, Reneman RS, Toth A, Ligeti L. In vivo monitoring of intracellular free calcium changes during uterine activation by prostaglandin F(2alpha) and oxytocin. J Soc Gynecol Investig (2002) 9(5):294–8. doi: 10.1016/s1071-5576(02)00169-7
52. Chin-Smith EC, Willey FR, Slater DM, Taggart MJ, Tribe RM. Nuclear factor of activated T-cell isoform expression and regulation in human myometrium. Reprod Biol Endocrinol (2015) 13:83. doi: 10.1186/s12958-015-0086-0
53. Molnar M, Hertelendy F. Regulation of intracellular free calcium in human myometrial cells by prostaglandin F2 alpha: comparison with oxytocin. J Clin Endocrinol Metab (1990) 71(5):1243–50. doi: 10.1210/jcem-71-5-1243
54. Lawrence T. The nuclear factor nf-Kb pathway in inflammation. Cold Spring Harb Perspect Biol (2009) 1(6):a001651. doi: 10.1101/cshperspect.a001651
55. Lindstrom TM, Bennett PR. The role of nuclear factor kappa b in human labour. Reproduction (2005) 130(5):569–81. doi: 10.1530/rep.1.00197
56. Khanjani S, Kandola MK, Lindstrom TM, Sooranna SR, Melchionda M, Lee YS, et al. Nf-Kb regulates a cassette of Immune/Inflammatory genes in human pregnant myometrium at term. J Cell Mol Med (2011) 15(4):809–24. doi: 10.1111/j.1582-4934.2010.01069.x
57. Goldenberg RL, Culhane JF, Iams JD, Romero R. Epidemiology and causes of preterm birth. Lancet (2008) 371(9606):75–84. doi: 10.1016/S0140-6736(08)60074-4
58. Shynlova O, Nedd-Roderique T, Li Y, Dorogin A, Lye SJ. Myometrial immune cells contribute to term parturition, preterm labour and post-partum involution in mice. J Cell Mol Med (2013) 17(1):90–102. doi: 10.1111/j.1582-4934.2012.01650.x
60. Shynlova O, Tsui P, Dorogin A, Lye SJ. Monocyte chemoattractant protein-1 (Ccl-2) integrates mechanical and endocrine signals that mediate term and preterm labor. J Immunol (2008) 181(2):1470–9. doi: 10.4049/jimmunol.181.2.1470
61. Srikhajon K, Shynlova O, Preechapornprasert A, Chanrachakul B, Lye S. A new role for monocytes in modulating myometrial inflammation during human labor. Biol Reprod (2014) 91(10):1–12. doi: 10.1095/biolreprod.113.114975
62. Lim R, Lappas M. Inhibition of Gpr91 reduces inflammatory mediators involved in active labor in myometrium. Mediators Inflammation (2020) 2020:6454282. doi: 10.1155/2020/6454282
63. Golightly E, Jabbour HN, Norman JE. Endocrine immune interactions in human parturition. Mol Cell Endocrinol (2011) 335(1):52–9. doi: 10.1016/j.mce.2010.08.005
64. Terzidou V, Lee Y, Lindstrom T, Johnson M, Thornton S, Bennett PR. Regulation of the human oxytocin receptor by nuclear factor-kappab and Ccaat/Enhancer-binding protein-beta. J Clin Endocrinol Metab (2006) 91(6):2317–26. doi: 10.1210/jc.2005-2649
65. Malinin NL, Boldin MP, Kovalenko AV, Wallach D. Map3k-related kinase involved in nf-kappab induction by tnf, Cd95 and il-1. Nature (1997) 385(6616):540–4. doi: 10.1038/385540a0
66. Sales KJ, Grant V, Jabbour HN. Prostaglandin E2 and F2alpha activate the fp receptor and up-regulate cyclooxygenase-2 expression via the cyclic amp response element. Mol Cell Endocrinol (2008) 285(1-2):51–61. doi: 10.1016/j.mce.2008.01.016
67. Melien O, Thoresen GH, Sandnes D, Ostby E, Christoffersen T. Activation of P42/P44 mitogen-activated protein kinase by angiotensin ii, vasopressin, norepinephrine, and prostaglandin F2alpha in hepatocytes is sustained, and like the effect of epidermal growth factor, mediated through pertussis toxin-sensitive mechanisms. J Cell Physiol (1998) 175(3):348–58. doi: 10.1002/(SICI)1097-4652(199806)175:3<348::AID-JCP13>3.0.CO;2-1
68. Hebert RL, Carmosino M, Saito O, Yang G, Jackson CA, Qi Z, et al. Characterization of a rabbit kidney prostaglandin F(2alpha) receptor exhibiting G(I)-restricted signaling that inhibits water absorption in the collecting duct. J Biol Chem (2005) 280(41):35028–37. doi: 10.1074/jbc.M505852200
69. Pohl O, Chollet A, Kim SH, Riaposova L, Spezia F, Gervais F, et al. Obe022, an oral and selective prostaglandin F2alpha receptor antagonist as an effective and safe modality for the treatment of preterm labor. J Pharmacol Exp Ther (2018) 366(2):349–64. doi: 10.1124/jpet.118.247668
70. Fillion D, Devost D, Sleno R, Inoue A, Hebert TE. Asymmetric recruitment of beta-Arrestin1/2 by the angiotensin ii type I and prostaglandin F2alpha receptor dimer. Front Endocrinol (Lausanne) (2019) 10:162. doi: 10.3389/fendo.2019.00162
71. Sleno R, Devost D, Petrin D, Zhang A, Bourque K, Shinjo Y, et al. Conformational biosensors reveal allosteric interactions between heterodimeric At1 angiotensin and prostaglandin F2alpha receptors. J Biol Chem (2017) 292(29):12139–52. doi: 10.1074/jbc.M117.793877
72. Kim SH, Bennett PR, Terzidou V. Advances in the role of oxytocin receptors in human parturition. Mol Cell Endocrinol (2017) 449:56–63. doi: 10.1016/j.mce.2017.01.034
73. Arrowsmith S, Wray S. Oxytocin: its mechanism of action and receptor signalling in the myometrium. J Neuroendocrinol (2014) 26(6):356–69. doi: 10.1111/jne.12154
74. Wray S, Prendergast C. The myometrium: from excitation to contractions and labour. Adv Exp Med Biol (2019) 1124:233–63. doi: 10.1007/978-981-13-5895-1_10
75. Fu X, Favini R, Kindahl K, Ulmsten U. Prostaglandin F2alpha-induced ca++ oscillations in human myometrial cells and the role of Ru 486. Am J Obstet Gynecol (2000) 182(3):582–8. doi: 10.1067/mob.2000.102707
76. Katz A, Wu D, Simon MI. Subunits beta gamma of heterotrimeric G protein activate beta 2 isoform of phospholipase c. Nature (1992) 360(6405):686–9. doi: 10.1038/360686a0
77. Wu D, Katz A, Simon MI. Activation of phospholipase c beta 2 by the alpha and beta gamma subunits of trimeric gtp-binding protein. Proc Natl Acad Sci U.S.A. (1993) 90(11):5297–301. doi: 10.1073/pnas.90.11.5297
78. Jiang H, Kuang Y, Wu Y, Smrcka A, Simon MI, Wu D. Pertussis toxin-sensitive activation of phospholipase c by the C5a and fmet-Leu-Phe receptors. J Biol Chem (1996) 271(23):13430–4. doi: 10.1074/jbc.271.23.13430
79. Murthy KS, Coy DH, Makhlouf GM. Somatostatin receptor-mediated signaling in smooth muscle. activation of phospholipase c-Beta3 by gbetagamma and inhibition of adenylyl cyclase by Galphai1 and galphao. J Biol Chem (1996) 271(38):23458–63. doi: 10.1074/jbc.271.38.23458
80. Molnar M, Hertelendy F. Signal transduction in rat myometrial cells: comparison of the actions of endothelin-1, oxytocin and prostaglandin F2 alpha. Eur J Endocrinol (1995) 133(4):467–74. doi: 10.1530/eje.0.1330467
81. Molnar M, Rigo J Jr., Hertelendy F. Signal transduction in human myometrial cells. Acta Physiol Hung (1996) 84(2):89–97.
82. Luckas MJ, Taggart MJ, Wray S. Intracellular calcium stores and agonist-induced contractions in isolated human myometrium. Am J Obstet Gynecol (1999) 181(2):468–76. doi: 10.1016/s0002-9378(99)70580-6
83. Goupil E, Tassy D, Bourguet C, Quiniou C, Wisehart V, Pétrin D, et al. A novel biased allosteric compound inhibitor of parturition selectively impedes the prostaglandin F2α-mediated Rho/Rock signaling pathway*. J Biol Chem (2010) 285(33):25624–36. doi: 10.1074/jbc.M110.115196
84. Crossthwaite AJ, Hasan S, Williams RJ. Hydrogen peroxide-mediated phosphorylation of Erk1/2, Akt/Pkb and jnk in cortical neurones: dependence on Ca(2+) and Pi3-kinase. J Neurochem (2002) 80(1):24–35. doi: 10.1046/j.0022-3042.2001.00637.x
85. Gewirtz AT, Rao AS, Simon PO, Merlin D, Carnes D, Madara JL, et al. Salmonella typhimurium induces epithelial il-8 expression Via Ca 2+-mediated activation of the nf-Kb pathway. J Clin Invest (2000) 105(1):79–92. doi: 10.1172/JCI8066
86. Melien O, Nilssen LS, Dajani OF, Sand KL, Iversen JG, Sandnes DL, et al. Ca2+-mediated activation of erk in hepatocytes by norepinephrine and prostaglandin F2 alpha: role of calmodulin and src kinases. BMC Cell Biol (2002) 3:5. doi: 10.1186/1471-2121-3-5
87. Kennedy A, Martinez K, Chung S, LaPoint K, Hopkins R, Schmidt SF, et al. Inflammation and insulin resistance induced by trans-10, cis-12 conjugated linoleic acid depend on intracellular calcium levels in primary cultures of human adipocytes. J Lipid Res (2010) 51(7):1906–17. doi: 10.1194/jlr.M005447
88. Jeong HJ, Hong SH, Park RK, An NH, Kim HM. Ethanol induces the production of cytokines Via the Ca2+, map kinase, hif-1alpha, and nf-kappab pathway. Life Sci (2005) 77(17):2179–92. doi: 10.1016/j.lfs.2005.04.014
89. Sanders D, Pelloux J, Brownlee C, Harper JF. Calcium at the crossroads of signaling. Plant Cell (2002) 14 Suppl:S401–17. doi: 10.1105/tpc.002899
90. Riaposova L. The effect of combination tocolysis on the inhibition of human myometrial contractions and inflammation in preterm labour [PhD thesis]. London: Imperial College London (2021).
91. Wu KK, Liou JY, Cieslik K. Transcriptional control of cox-2 Via C/Ebpbeta. Arteriosclerosis Thrombosis Vasc Biol (2005) 25(4):679–85. doi: 10.1161/01.ATV.0000157899.35660.61
92. Mosher AA, Rainey KJ, Bolstad SS, Lye SJ, Mitchell BF, Olson DM, et al. Development and validation of primary human myometrial cell culture models to study pregnancy and labour. BMC Pregnancy Childbirth (2013) 13 Suppl 1:S7. doi: 10.1186/1471-2393-13-S1-S7
93. Shynlova O, Lee YH, Srikhajon K, Lye SJ. Physiologic uterine inflammation and labor onset: integration of endocrine and mechanical signals. Reprod Sci (2013) 20(2):154–67. doi: 10.1177/1933719112446084
Keywords: prostaglandin F2 alpha (PGF2α), myometrium, cyclooxygenase-2 (COX-2), inflammation, preterm birth, labour, G protein-coupled receptor (GPCR)
Citation: Riaposova L, Kim SH, Hanyaloglu AC, Sykes L, MacIntyre DA, Bennett PR and Terzidou V (2023) Prostaglandin F2α requires activation of calcium-dependent signalling to trigger inflammation in human myometrium. Front. Endocrinol. 14:1150125. doi: 10.3389/fendo.2023.1150125
Received: 23 January 2023; Accepted: 06 June 2023;
Published: 19 July 2023.
Edited by:
Jan Tesarik, MARGen Clinic, SpainReviewed by:
Kelycia B. Leimert, University of Alberta, CanadaTomislav Vladic, Independent Researcher, Stockholm, Sweden
Ananth Kumar Kammala, University of Texas Medical Branch at Galveston, United States
Jose Galaz, Wayne State University, United States
Copyright © 2023 Riaposova, Kim, Hanyaloglu, Sykes, MacIntyre, Bennett and Terzidou. This is an open-access article distributed under the terms of the Creative Commons Attribution License (CC BY). The use, distribution or reproduction in other forums is permitted, provided the original author(s) and the copyright owner(s) are credited and that the original publication in this journal is cited, in accordance with accepted academic practice. No use, distribution or reproduction is permitted which does not comply with these terms.
*Correspondence: Vasso Terzidou, v.terzidou@imperial.ac.uk