- 1Laboratory for Experimental Feto-Maternal Medicine, Department of Obstetrics and Prenatal Medicine, University Medical Center of Hamburg-Eppendorf, Hamburg, Germany
- 2Institute of Immunology, University Medical Center of Hamburg-Eppendorf, Hamburg, Germany
Pregnant women are highly vulnerable to adverse environments. Accumulating evidence highlights that increasing temperatures associated with the ongoing climate change pose a threat to successful reproduction. Heat stress caused by an increased ambient temperature can result in adverse pregnancy outcomes, e.g., preterm birth, stillbirth and low fetal weight. The pathomechanisms through which heat stress interferes with pregnancy maintenance still remain vague, but emerging evidence underscores that the endocrine system is severely affected. It is well known that the endocrine system pivotally contributes to the physiological progression of pregnancy. We review – sometimes speculate - how heat stress can offset hormonal dysregulations and subsequently derail other systems which interact with hormones, such as the immune response. This may account for the heat-stress related threat to successful pregnancy progression, fetal development and long-term children’s health.
Introduction
Climate change manifests in various environmental threats, including rising temperatures and heat waves. These threats have been associated with severe health consequences, and heat-related illness, e.g., heat cramps, collapse or stroke (1). Pregnant women are highly vulnerable to environmental challenges (2). This vulnerability is attributable to the physiological changes of the maternal cardiovascular and respiratory system, as well as the adaptations of the endocrine and the immune system (Figure 1). An increasing number of epidemiological studies provide evidence that environmental heat stress triggers adverse pregnancy outcomes (3, 4). Additionally, heat stress may also interfere with the pre-conceptional phase, resulting in menstrual cycle aberrations and diminished fertility rates (5, 6). Heat stress poses a comprehensive threat to reproductive health with a wide range of personal, societal and socioeconomic consequences.
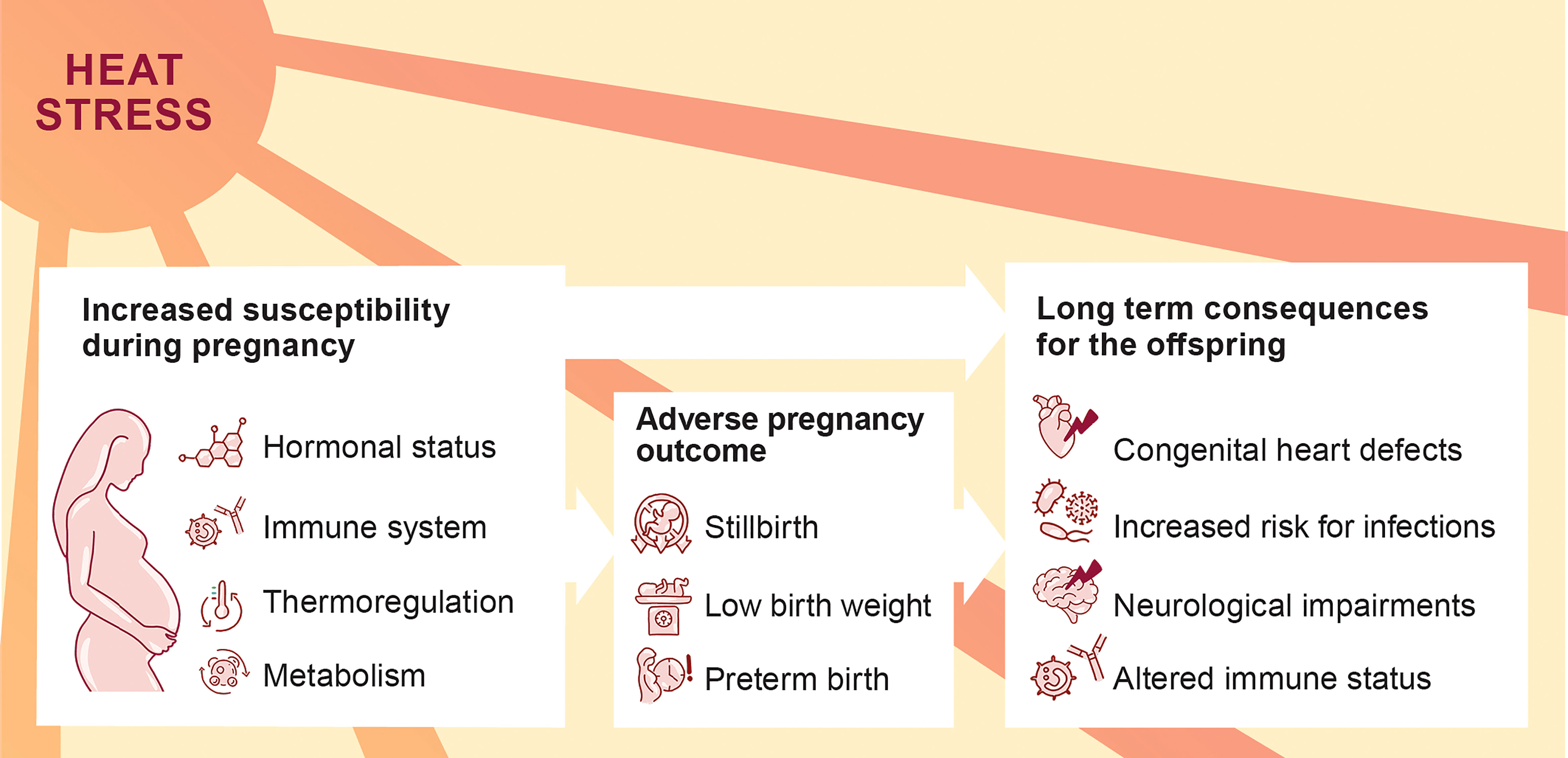
Figure 1 Increased susceptibility of pregnant women to heat stress with possible consequences for the progression of pregnancy and offspring’s health.
Successful mammalian reproductive outcome depends on a delicate balance of molecular and cellular markers. A key player is the endocrine system, which not only adapts to the demands of pregnancy by maintaining uterine quiescence, but also adjusts to predictive environmental challenges, e.g., circadian rhythm, as well as unpredicted environmental challenges such as heat stress (7, 8). Dependent on the type or intensity of the challenge, the endocrine system responds with aberrant hormonal levels, which may result in severe consequences for female health (9). The endocrine response is tightly linked to the immune response during pregnancy, through which the immunological tolerance towards the fetus is maintained (10). Therefore, environmental stressors pose a significant risk to disturb the immunological balance essential for feto-maternal tolerance via hormone-mediated pathways.
Heat can be defined as temperatures above the thermoneutral zone. The thermoneutral zone allows healthy adults to maintain a physiological body temperature via a constant metabolic rate (11). Thus, temperatures above a specific threshold are known to induce heat-related stress responses (12). However, this definitions falls short to take the individual perception of heat - based on the geographical region, acclimatization and personal discomfort - into account. This limitation is also reflected by the heterogeneity of heat stress definitions, exposure windows and exposure duration used in preclinically studies conducted in mice or livestock as summarized for this review in Table 1.
The ongoing climate change and the societal responsibility to guarantee maternal health emphasizes the need for in-depth research in order to understand the pathophysiology of heat in the context of female reproduction and hormonal dysbalance throughout women’s life (Figure 2). We here summarize recent findings addressing the impact of heat stress on the endocrine and immune system during the preconceptual phase and pregnancy in a number of mammalian species and refine the conceptual understanding on the endogenous stress response to heat stress.
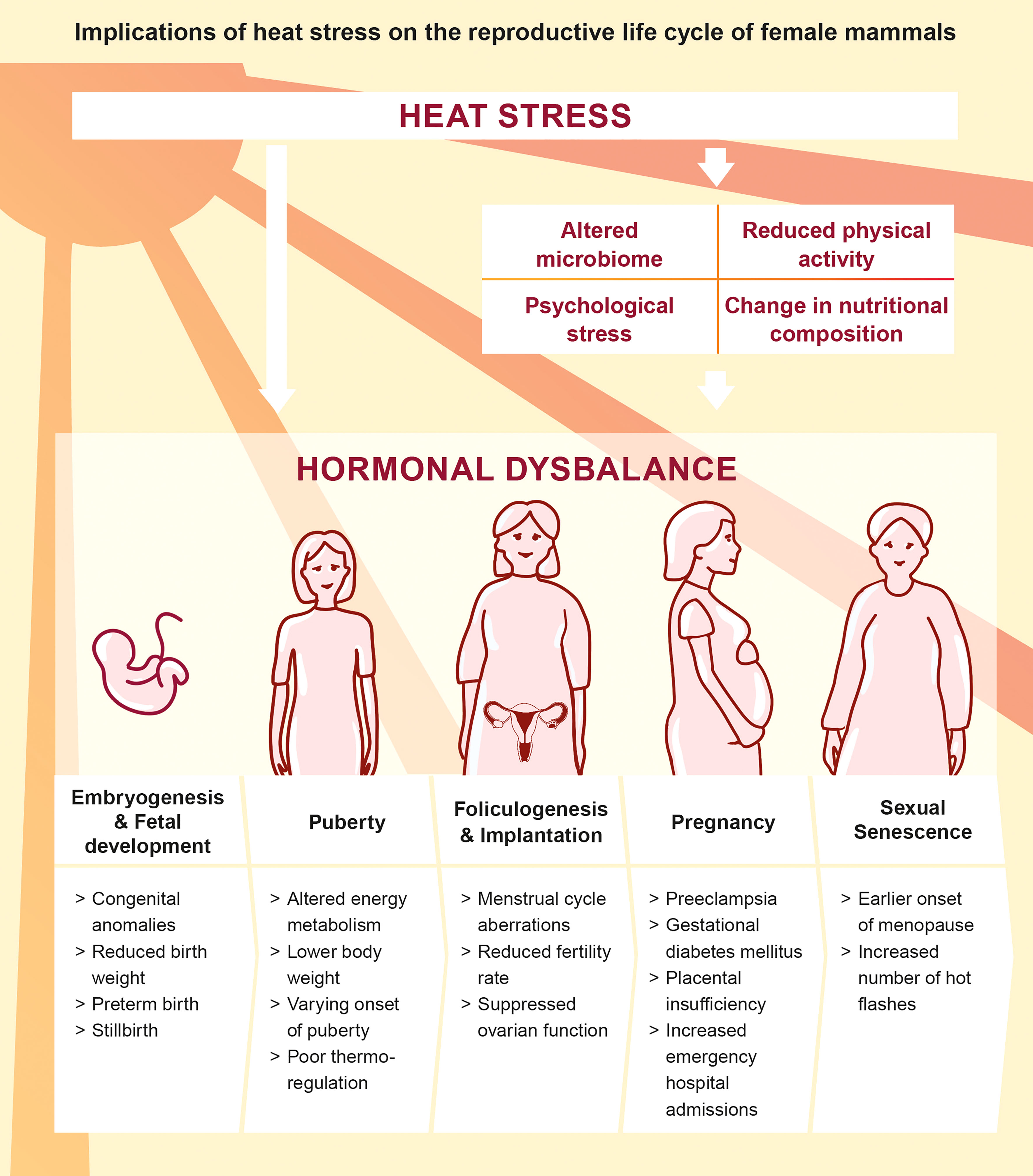
Figure 2 Direct and indirect implications of heat stress on the reproductive life cycle of female mammals (5, 26, 27, 37–45).
Heat stress: A threat to reproductive success
Infertility, which is defined as the failure to successfully conceive after more than 12 months of unprotected sexual intercourse, increasingly affects millions of couples worldwide (46). Despite its multifactorial origin, there are several studies indicating that environmental heat can indeed negatively impact fertility in mammals. A study analyzing the effects of high temperatures in the United States between 1931 and 2010 found a decrease in birth rates of 0.4% nine months after exposure to one additional heat day with a mean temperature above 26.7°C compared to an additional day with relatively colder temperatures between 15.6°C and 21.1°C (5). Likewise, a multi-site study revealed that a 1°C increase of the maximum temperature decreases the total fertility rate by 1.3 as shown for Italy, whereas fertility rates of individuals in countries with moderate climate (15-20°C) are unaffected (47). Although heat stress affects both sexes, reduction of reproductive success is partially explained by the observation that heat exposure alters porcine endometrial tissue during the pre-implantation period resulting in diminished fertility rates in females which might be caused by hormonal dysregulation (13). Work done in cattle or rats showed similar results by indicating an association of high ambient temperatures and alterations of the estrous cycle (14, 48). Thus, it is tempting to speculate that the observed reduction in fertility rates upon heat-exposure in women is also caused by alterations of the menstrual cycle. A study investigating the effect of seasonal changes on menstrual cycle length using smartphone application data of 310.000 women failed to support this notion (6). However, this lack in comparability may be explained by the fact that high ambient temperatures and seasonal changes are two different entities.
In addition to the effects of heat stress on women during preconception, exposure to heat has also been associated with the risk for adverse pregnancy outcomes (37). Beside stillbirth, these include preterm birth, low birth weight and congenital cardiac defects, which pose serious threats to the child and can cause long-lasting health impairments (49, 50). Among the possible pregnancy adversities, the risk for preterm birth has likely been best investigated and the majority of studies identified a risk perpetuation caused by heat, as highlighted by a current summary on the topic (51). A meta-analysis of six independent studies reported 1.16-fold higher odds of preterm birth after exposure to extreme heat, along with complications such as lower birthweight, congenital anomalies and stillbirth (3).
Understanding the pathogenesis of heat stress-induced pregnancy complications: From animal models to humans
Despite the fact that heat-associated pregnancy complications are increasingly in the focus of research endeavors, insights into the underlying pathogenesis are still sparse. This can be partly explained by a general neglect to include pregnant women in research studies. In contrast, the effect of heat stress on livestock has been extensively studied due to its economic importance. Also, cattle are generally kept in controlled environments, which facilitates investigating the effect of environmental stressors. Among mammals, certain milestones of reproduction are conserved, which allows to translate findings on the impact of heat stress in cattle to humans to a certain degree (52). Both species cycle continuously while not pregnant, are monovular - ovulation of one oocyte per cycle -, have a gestational period of 9 months and have ovaries with a similar size and morphology (53). Additionally, reproductive techniques such as artificial insemination, synchronization protocols and superovulation techniques, to name a few, are widely applied in cattle (54).
In addition to livestock, rodent models can also provide insights on the impact of heat stress on reproductive success, since rodents can be kept in controlled microenvironments. Due to the availability and possibility to generate genetically modified animals, rodents also serve as a powerful source of information to understand the pathogenesis of diseases (55, 56). In the context of pregnancy, mouse models have a high translational value due to a similar placental expression of paternal antigens as well as a comparable immune response (57).
However, large ruminant and small mono-gastric animals deviate with regard to their thermoregulation, which limits the translational value of some of the insights in livestock and rodents. Rodents regulate their body temperature by adapting food intake, ruminants rely on regulation of evaporative heat loss (58, 59). Additionally, the ratio of body surface area and body mass can also affect heat dissipation and thermal regulation (59).
Effect of heat stress on the hypothalamus-pituitary axis
In humans, heat stress is well known to induce acute neurological deficits and cognitive impairments (60). This may be partly attributed to alterations of the hypothalamic tissue. In rats exposed to severe heat challenges, hypothalamic neurons showed morphological aberrations and an increased frequency of pyknosis (15). Additionally, a moderate to severe heat challenge in rats caused higher levels of oxidative stress. These neuroinflammatory processes were accompanied by an increase in systemic corticotropin-releasing hormone (CRH) and adrenocorticotropin hormone (ACTH) levels, which could be an indicator for increased stress levels and subsequently altered hypothalamic-pituitary hormone secretion. Further, neuroinflammation might also contribute to an impaired temperature regulation and a decrease in heat tolerance (29).
Similar morphological impairments are observed in pregnant mice. Here, acute heat stress led to significantly elevated neuronal damage in the hypothalamus (29). Further signs of hypothalamic cell damage such as pyknosis, cell body shrinkage and apoptotic changes were detected. Similar to the study performed in rats, the authors of the present study also measured increased ACTH levels in heat-challenged pregnant mice. Additionally, morphological alterations were accompanied by significantly higher levels of oxidative stress and increased hypothalamic levels of the pro-inflammatory cytokines TNF-α and IL-1β. These observations not only provide strong evidence for the severe neuronal inflammation upon heat exposure, but also indicates that pregnancy perpetuates these effects. It is known, that the onset of pregnancy alters plasticity and neurophysiological activity of the brain and particularly the hypothalamus (61). These physiological changes might leave the hypothalamic regions more susceptible to heat stress, resulting in further impairment of the adjacent signaling axis. However, increased neuroinflammation during pregnancy is not limited to heat stress, since it was also observed in response to other stressors, such as sound stress or exposure to bacterial antigens (62). In mice, sound stress and bacterial antigens enhanced the permeability of mucosal membranes and subsequent infiltration of bacteria, which led to disseminated inflammation (62). Therefore, the reported consequences of heat stress might be as well attributed to a general stress response during pregnancy.
An impairment of the hypothalamic function in response to heat challenge can subsequently affect the secretion of pituitary hormones. In fact, a study performed in rats focusing on gonadotropins identified an increase in follicle-stimulating hormone (FSH) and a decrease in luteinizing hormone (LH) levels in response to heat challenge, whereas gonadotropin-releasing hormone (GnRH) concentrations were unaffected (16). Elevated FSH level upon heat stress have also been observed in dairy cows (17), whereas findings in prepubertal female mice are more conflicting (18). Interestingly, heat stress also caused neuroendocrine perturbations in male mice, mirrored by elevated levels of FSH and reduced levels of inhibin, along with altered reproductive function (19). Taken together, these observations underscore that heat stress directly affects the hypothalamic-pituitary axis, which is summarized in Figure 3.
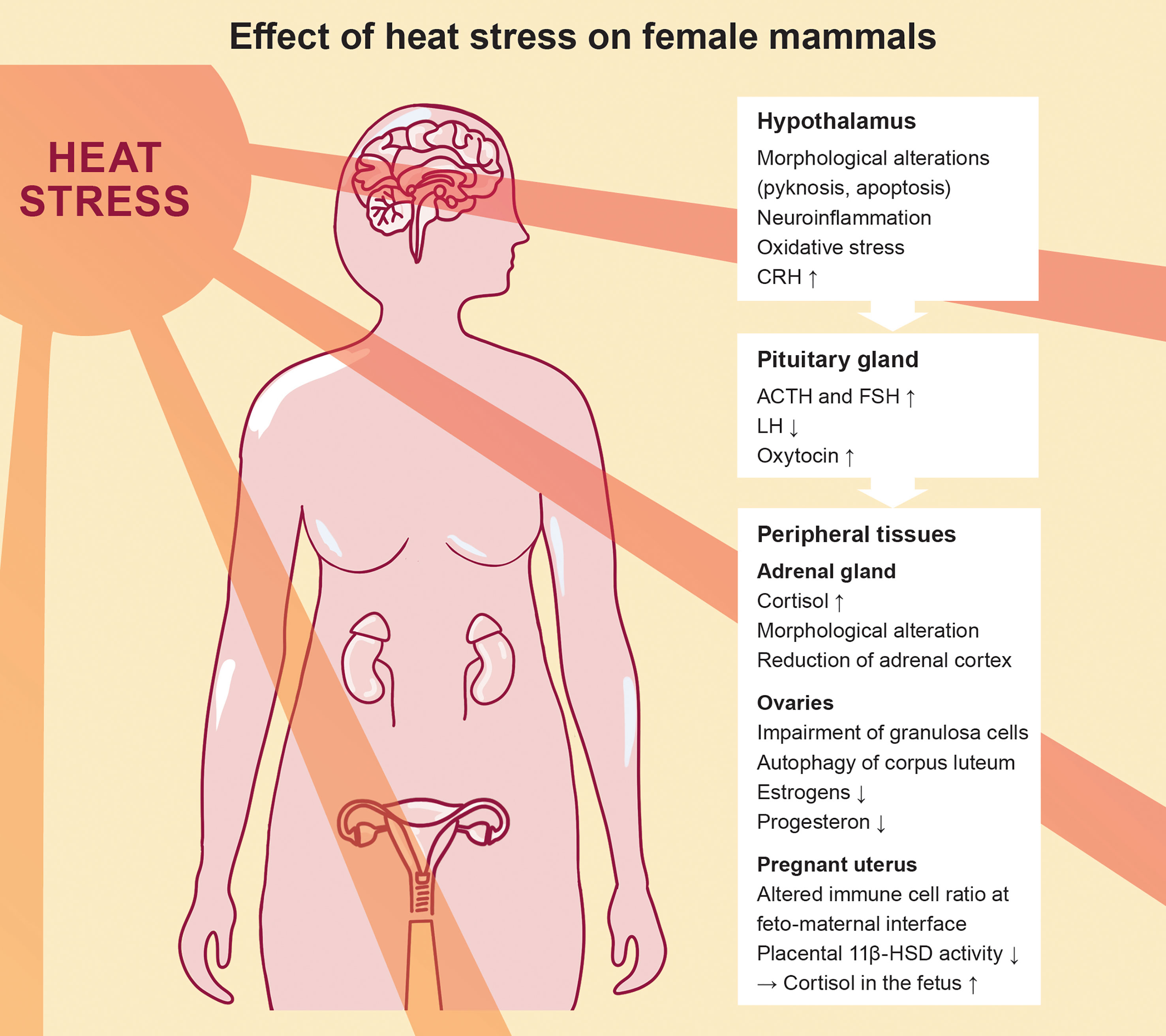
Figure 3 Effect of heat stress on the female mammalian endocrine system, highlighting the progression from the hypothalamus-pituitary axis to the peripheral tissues.
Peripheral tissues: Adrenal glands, uterus and placenta under heat stress
Physiologically, cortisol or respectively corticosterone levels depend on the secretion by the pituitary and adrenal gland. Both, the pituitary and the adrenal gland are affected by heat stress as indicated by increased organ weights in rats (20). Contradicting results are reported in another study, postulating a decrease in adrenal gland mass and volume attributable to a reduction of the adrenal cortex. More precisely, the alterations can be traced back to a smaller cell size in the zona fasciculata, which is the second of three layers of the adrenal cortex and the main location of cortisol production (21). Both studies differ greatly in the heat exposure protocol used in the experiments. Whereas the first study exposed rats to eight hours of heat stress (32°C) for seven consecutive days, the second study applied 60 minutes of heat stress (38°C) before sacrifice of animals. This opposed experimental design regarding acute or chronic heat exposure combined with the differences in temperatures (Table 1) limit comparability of studies and might explain the conflictive findings since moderate and severe heat stress have differential effects on thermoregulation (15).
Based on a comparative study investigating the activation of the HPA axis in rats by various stressors such as fasting, crowding and extreme ambient temperatures, exposure to heat resulted in the highest plasma corticosterone level – the cortisol analog in rodents (22). Both, short periods as well as prolonged exposure to heat led to an increase in blood ACTH and subsequently corticosterone levels (20, 23). Interestingly, evaluation of tissue corticosterone levels provided evidence for differences between peripheral organs after heat exposure, in which the lung-tissue has been proposed as the most accurate indicator for acute heat stress (23). Controversially, a study investigating the effects of heat stress in pre-pubertal rats reported lower corticosterone levels after heat exposure (24), which contradicts the finding that a pre-pubertal state is accompanied by a higher HPA axis reactivity (63, 64). However, the study in pre-pubertal rats assessed corticosterone levels two days after acute exposure to heat, which might already reflect a subsidence of the acute stress response.
Heat-triggered cortisol alterations have not only immediate effects on the maternal health, but might also be associated with longer lasting implications for the offspring. During pregnancy the placenta physiologically produces high amounts of CRH. Via a positive feedback on the maternal HPA axis, this results in a 40 fold higher CRH production from first trimester to term and a 3-5 fold rise in cortisol over gestation (65). Due to its properties as a steroid hormone, cortisol possesses the ability to cross the feto-maternal barrier. Thus, maternal cortisol levels affect the developing fetus. To protect the fetus from these high levels of maternal cortisol, the placenta functions as an important metabolizer (66). Placental metabolism of cortisol is mainly regulated by the enzyme 11-beta-hydroxysteroid dehydrogenase 2 (11ß-HSD2), which converts cortisol to a biologically inactive form (67). During heat stress in pregnant mice, expression of 11ß-HSD2 was reduced, along with an expected increase of cortisol in the fetus (68). This suggests that alterations of placental function – a key endocrine organ during pregnancy – may expose the fetus to cortisol surges upon heat stress. Changes in placental function might be attributed to heat-specific reductions of placental growth or to an altered HPA axis, since variations in 11β-HSD expression have also been reported upon other stress challenges in mice (30, 69). These cortisol surges can cause long-lasting health consequences for the offspring, resulting in growth retardation of the adrenal cortex and a decreased population of somatotropes – growth hormone producing cells – in the adenohypophysis (31) or a larger volume of the right amygdala associated with affective problems in girls (70).
In addition to cortisol, placental trophoblast cells produce human chorionic gonadotropin (hCG) at the beginning of pregnancy. hCG has a luteinizing effect on ovarian cells, which lengthens the lifespan of the corpus luteum and thus leads to sustained production of progesterone. At the moment there are no studies addressing the effect of heat exposure on hCG secretion.
The derogation of successful reproduction caused by heat stress does not only manifest through placental alterations, but might also be linked to heat-triggered effects on the uterus. In fact heat stress has been shown to affect the morphology of the preimplantational endometrium, especially affecting the luminal epithelial cells, and triggering aberrant local cell proliferation as well as the dilation of the uterine glands (16). During pregnancy the maintenance of uterine quiescence could be interrupted by increased FSH concentrations acting on the myometrium potentially favoring preterm births (71). Another hormone highly relevant for the induction of labor is oxytocin. Produced in the hypothalamus, oxytocin mediates, beside its anti-inflammatory and anti-apoptotic functions, uterine contraction during birth. Pretreatment of rats with oxytocin before the onset of heat stress protected them from heat stroke related symptoms such as lung edema and increased over-all well-being (25). In line with these protective capacities of oxytocin before exposure to heat, oxytocin concentrations are increased during heat stress (32). This suggests an acute protection mechanism, mediated by its effects on vascular resistance, cardiac output or the overall induction of a hypotensive response (72).
Heat stress modulates sex hormone levels
Several studies reported some form of dysfunction in the female gonads upon heat exposure. Irregular phenotypes of granulosa cells accompanied by detachment of oocytes from the granular cell layer could be found in acute and chronic heat stressed mice (26, 27). Consistent with the morphological changes of the granulosa cells, a decrease in estradiol concentrations upon chronic heat stress has been observed (16, 27, 73). This might be explained by decreased expression patterns of the enzyme aromatase in response to heat stress, which is the key hormone of the estrogen synthesis (27). Despite granulosa cell damage, gonadotropin receptors on the cell surface show diminished FSH receptor expression upon acute heat stress in rats, along with increased FSH receptor expression in chronic heat stressed rats (16, 74). However, the exact pathways by which heat stress influences morphological integrity and receptor expression of granulosa cells are unknown. Further, it needs to be investigated why granulosa cells seem to be more susceptible to heat-related damages compared to other cell types. The relevance of heat as a stressor was demonstrated by a study comparing estradiol levels after heat or psychological stress. Although exposure to both stressors lowered estradiol levels, this effect was more pronounced after heat stress. Interestingly, combination of both resulted in even lower levels of estradiol indicating cumulative effects on gonadal tissue damage (14).
Additionally, considering the fact that regulation of the hypothalamic-pituitary-gonadal (HPG) axis functions via negative and positive feedback mechanisms, low hormone concentrations due to impaired production by the gonadal tissues have been proposed to lead to a decreased feedback response in the hypothalamus and the pituitary gland and stimulate the secretion of gonadotropins (75).
Although estrogens are pivotal during pregnancy, few studies to date focused on the dynamics of estrogen levels throughout mammalian pregnancy in response to heat stress. One study dating back to 1982 failed to detect any heat-related alterations of free estrogens in chronic heat stress-exposed pregnant cows (33). However, the authors describe a decrease of estrone-sulfate upon heat stress, which indicates placental insufficiency. Since the placenta is the major source of estrogens during human pregnancy, future studies evaluating estrogen levels should therefore also consider placental functionality. Also, changes in estradiol levels might be particularly relevant in the context of thermoregulation, since estradiol promotes vasodilatation and thus facilitates heat dissipation lowering the body temperature (76, 77). Thus, a comprehensive study addressing possible changes of estrogens in combination with placental assessment might provide novel insights on the consequences of heat stress.
Besides its pivotal role in establishing fertility and pregnancy maintenance, progesterone is also a thermal influencer. In contrast to estrogen, progesterone promotes heat conservation and higher body temperature (76). An increase in progesterone, as evident during pregnancy, might thus explain the high susceptibility to heat stress due to an inability to dissipate heat, subsequently favoring pathological hyperthermic conditions. Although, the exact contribution of progesterone to thermoregulation is not fully understood, studies in rodents provide evidence that chronic heat stress resulted in reduced concentrations of progesterone (14). This observation was also confirmed in heat-stressed cattle (34). However, this effect was no longer evident in rats exposed to heat if challenged for a prolonged period of time (16). Reduction in progesterone levels might be linked to a decreased synthesis of pregnolone, the precursor of progesterone, which was diminished in bovine granulosa cells due to a decreased transcription of steroidogenic genes (78). However, to date there are no data available on how heat exposure alters the expression of steroidogenic genes in murine or even human cell culture experiments.
Comparable to the findings in non-pregnant mice, heat stress exposure of early pregnant mice led to a significant decrease in progesterone concentrations (35). Concurrently to these hormonal changes, increased markers of autophagy were detected in the steroid producing cells of the corpus luteum. Autophagy is relevant for regulating the function of the corpus luteum and might be relevant for luteal regression. Since progesterone is produced by the corpus luteum at the beginning of pregnancy, before the placenta takes over, regression by autophagy could contribute to decreased progesterone concentrations. This hypothesis is supported by observations in chronic heat stress-exposed cattle three weeks before gestation, where lower progesterone levels, reduced conception rates and lower transferable embryo were described (28).
Another explanation relates to the common progenitor of progesterone and cortisol: cholesterol-derived pregnenolone. Increased demand of cortisol in response to heat stress could lead to a competition of both syntheses and reduce progesterone availability (66).
Heat stress and the immune system during pregnancy
During the course of pregnancy, the maternal immune system undergoes gradual adaptations, which are essential to guarantee maternal tolerance towards the genetically foreign fetus. Dysregulation of these immunological adaptations is associated with pregnancy complications, such as recurrent spontaneous abortions, preterm birth or intrauterine growth restriction (79). Hormones significantly orchestrate the maternal immune adaptations during pregnancy (57). Thus, heat stress-induced alterations of the endocrine response can also affect the immune response in pregnant individuals. Progesterone primarily dampens the production of pro-inflammatory cytokines by local immune cells and induces more tolerogenic phenotypes in dendritic cells, which then enables them to promote regulatory T cells, essential for pregnancy maintenance (80, 81). In addition, progesterone also promotes immune tolerance by inducing immunomodulatory molecules at the feto-maternal interface (82). As discussed above, heat exposure seems to reduce progesterone concentrations. A decrease in progesterone might subsequently lead to a shift towards an inflammatory immune response at the beginning of pregnancy, with decreased tolerance towards the fetus, which then favors fetal loss (62). Towards the end of human pregnancy, progesterone contributes to maintain an anti-inflammatory environment until induction of labor. Thus, heat stress associated progesterone reduction might trigger inflammation processes prematurely and contribute to the pathogenesis of heat-induced preterm birth.
Glucocorticoids such as cortisol are well known for their anti-inflammatory capacity. Additionally, they are involved in cell recruitment of macrophages to the feto-maternal interface as demonstrated in experiments with glucocorticoid receptor knock-out mice (83). Although the exact function of glucocorticoids in the context of the initiation of labor has yet to be elucidated, it is well known that in addition to the increase of glucocorticoid levels during the course of pregnancy a significant surge takes place when approaching labor (84). Thus, increase of glucocorticoid levels – as observed upon heat exposure – might not only contribute to an impaired immune response early during pregnancy, but also towards term. Studies evaluating the immune cell function at the feto-maternal interface upon heat stress triggered hormone alterations are missing and should urgently be conducted starting with preclinical models. Due to the immunological dynamics of pregnancy, different exposure windows should be tested and clearly defined to assure reproducibility.
Studies testing the direct effect of heat stress on immune cells during pregnancy are sparse. One study evaluating placental immune response to heat stress in late gestational mice described an increase in the expression of inflammatory genes (36). Here elevated molecular expression of the macrophage marker CD68 were accompanied by an upregulation of genes associated with the complement system, such as C1qa, C3 and CD55. The authors hypothesize that heat stress led to a recruitment of macrophages to the placenta, which then activate the complement system. Additionally, a decrease of Csf1was observed. Csf1 can induce an anti-inflammatory phenotype in macrophages, suggesting that its decrease in response to heat stress skews macrophage function towards pro-inflammation. Macrophages have pleiotropic functions during pregnancy, therefore a possible change in function in response to heat stress must be confirmed in future studies.
Additionally, the immune system may not only be acutely affected by heat stress, since long-term consequences have also been postulated. Triggered by the acute stress response, heat stress may lead to an acclimatization that enhances cytoprotective anti-oxidative and anti-apoptotic pathways against future stress exposure (85). This mechanism, referred to as heat acclimation-mediated cross-tolerance, is mediated by epigenetic modification of histones, namely phosphorylation of histone H3 and acetylation of histone H4, which promote gene expression of cytoprotective mediators (86, 87). Since these observations were made in male rats, they urgently require further validation in pregnancy models.
Psychological consequences of heat stress: From sleep deprivation to climate anxiety
In addition to the discussed direct effects of heat exposure on hormone levels or immune homeostasis, heat may also indirectly modulate the endocrine pathways of HPG axis. Tropical nights (night-time minimum temperature equal or higher than 20°C) (88) are associated with sleep deprivation and reduced quality of sleep. Both have been linked to alterations of sex hormone concentrations, which negatively impact reproductive fitness in rats (89, 90). Here, the crosstalk between reproductive hormones and melatonin is of great interest, since sleep deprivation is known to impact the endogenous melatonin secretion. Melatonin modulates sex hormone synthesis especially in the hypothalamus and the pituitary gland (7). For the years 2050 and 2099, the predicted numbers of additional nights of insufficient sleep with 2010 as baseline average are estimated to be 6 respectively 14 per 100 individuals (90). Thus, the relevance of sleep deprived hormonal imbalances in the near future should not be underestimated.
Climate change poses a direct threat to mental health of pregnant women by favoring adverse pregnancy outcomes. Acute environmental events such as hurricanes, droughts or wildfires, and also chronic exposure to increased air pollution and high temperatures leads directly to mental health disorders such as posttraumatic stress syndrome or depression and indirectly by favoring water and food insecurities as well as migration (91). Recently, climate anxiety has been introduced as additional mechanism on how climate change effects mental well-being. Climate anxiety describes distress about the consequences of climate change, the existential threat and uncertainty that climate change poses, which cannot be anticipated neither in time nor place (92). Interestingly, this anxiety is strongly linked to the individual perception of climatic changes and does not necessarily correspond to personal experiences, since it includes individuals, who have not experienced climate-related adverse events. Climate anxiety itself is not pathophysiological, but poses a permanent psychological stressor and bares the potential to become chronic and thus, clinically evident. Especially adults at the beginning of their reproductive years reported to be extremely worried about climate change (93), which even results in a hesitancy to have children. Given the high prevalence of these negative emotions and beliefs concerning the future of families and children, climate anxiety constitutes a significant psychological stress factor that must be urgently evaluated in female and male adults during their reproductive years.
In contrast to high temperatures, which directly affect thermoregulation, the indirect effect of heat in inducing an endogenous stress response – mirrored by heat-induced sleep deprivation and climate anxiety – must be taken into account, since this enhanced stress perception perpetuates the endocrine stress response in pregnant as well as non-pregnant individuals.
Outlook
This review highlights the multifaceted consequences of heat stress on the endocrine balance and immune homeostasis, especially in the context of pregnancy. Clearly, many open questions remain before we fully understand how heat stress affects reproductive outcome. E.g., it remains to be tested if heat exposure directly affects the thermoregulation in pregnant women via inducing cell stress, hereby inducing endocrine and immunological aberrations. Or whether heat stress simply functions as an external stressor, which activates the endogenous stress response, hereby activating the hormonal stress response cascade. In fact, both pathways can alter fetal and childhood development trajectories and affect the endocrine hemostasis of this next generation. Also, the impact of acute vs. chronic heat stress effects remains to be elucidated, and multifactorial risk assessments should be considered in order to control for possible confounder of heat stress, such as ozone and air pollution. The limited number of studies addressing these observations in animal pregnancy models or human pregnancy cohorts leave a blind spot to the vulnerable societal group of pregnant women. Given the rapid progression of climate change and cumulating weather extremes, it is of particular importance to intensify efforts of local research groups and global initiatives. However, to address these knowledge gaps, universal definitions of chronic and acute heat stress need to be established, to allow for comparison between studies (Figure 4). Given the increasing number of studies reporting on pregnancy adversities due to environmental heat stress on a global scale, these observations demand an interdisciplinary expertise from clinicians, lab scientists, statisticans and epidemiologists.
Author contributions
All authors contributed to the article and approved the submitted version.
Funding
Writing of this review article was made possible by grants provided by the German Research Foundation (KFO296: AR232/25-2 and DI2103/2-2 to PA and AD, FOR5068: AR232/29-1 to PA) and the Authority for Science, Research and Equality, Hanseatic City of Hamburg, Germany (LFF-FV73) to PA and AD. Isabel Graf was supported by the Else Kröner-Fresenius-Stiftung iPRIME Scholarship (2021_EKPK.10), UKE, Hamburg. We further acknowledge financial support from the Open Access Publication Fund of UKE - Universitätsklinikum Hamburg-Eppendorf - and DFG - German Research Foundation.
Conflict of interest
The authors declare that the research was conducted in the absence of any commercial or financial relationships that could be construed as a potential conflict of interest.
Publisher’s note
All claims expressed in this article are solely those of the authors and do not necessarily represent those of their affiliated organizations, or those of the publisher, the editors and the reviewers. Any product that may be evaluated in this article, or claim that may be made by its manufacturer, is not guaranteed or endorsed by the publisher.
References
2. Weck RH, Paulose T, Flaws JA. Impact of environmental factors and poverty on pregnancy outcomes. Clin Obstet Gynecol (2008) 51(2):349–59. doi: 10.1097/GRF.0b013e31816f276e
3. Chersich MF, Pham MD, Areal A, Haghighi MM, Manyuchi A, Swift CP, et al. Associations between high temperatures in pregnancy and risk of preterm birth, low birth weight, and stillbirths: systematic review and meta-analysis. BMJ (2020) 371:m3811. doi: 10.1136/bmj.m3811
4. Carolan-Olah M, Frankowska D. High environmental temperature and preterm birth: A review of the evidence. Midwifery (2014) 30(1):50–9. doi: 10.1016/j.midw.2013.01.011
5. Barreca A, Deschenes O, Guldi M. Maybe next month? temperature shocks and dynamic adjustments in birth rates. Demography (2018) 55(4):1269–93. doi: 10.1007/s13524-018-0690-7
6. Tatsumi T, Sampei M, Saito K, Honda Y, Okazaki Y, Arata N, et al. Age-dependent and seasonal changes in menstrual cycle length and body temperature based on big data. Obstet Gynecol (2020) 136(4):666–74. doi: 10.1097/AOG.0000000000003910
7. Cipolla-Neto J, Amaral FG, Soares JM Jr., Gallo CC, Furtado A, Cavaco JE, et al. The crosstalk between melatonin and sex steroid hormones. Neuroendocrinology (2022) 112(2):115–29. doi: 10.1159/000516148
8. Sheng JA, Bales NJ, Myers SA, Bautista AI, Roueinfar M, Hale TM, et al. The hypothalamic-Pituitary-Adrenal axis: Development, programming actions of hormones, and maternal-fetal interactions. Front Behav Neurosci (2020) 14:601939. doi: 10.3389/fnbeh.2020.601939
9. Valsamakis G, Chrousos G, Mastorakos G. Stress, female reproduction and pregnancy. Psychoneuroendocrinology (2019) 100:48–57. doi: 10.1016/j.psyneuen.2018.09.031
10. Schumacher A, Costa SD, Zenclussen AC. Endocrine factors modulating immune responses in pregnancy. Front Immunol (2014) 5:196. doi: 10.3389/fimmu.2014.00196
11. Kingma BR, Frijns AJ, Schellen L, van Marken Lichtenbelt WD. Beyond the classic thermoneutral zone: Including thermal comfort. Temperature (Austin) (2014) 1(2):142–9. doi: 10.4161/temp.29702
12. Schwingshackl C, Sillmann J, Vicedo-Cabrera AM, Sandstad M, Aunan K. Heat stress indicators in CMIP6: Estimating future trends and exceedances of impact-relevant thresholds. Earth's Future. (2021) 9:e2020EF001885. doi: 10.1029/2020EF001885
13. Adur MK, Seibert JT, Romoser MR, Bidne KL, Baumgard LH, Keating AF, et al. Porcine endometrial heat shock proteins are differentially influenced by pregnancy status, heat stress, and altrenogest supplementation during the peri-implantation period. J Anim Sci (2022) 100(7):skac129. doi: 10.1093/jas/skac129
14. Han J, Yang D, Liu Z, Tian L, Yan J, Li K, et al. The damage effect of heat stress and psychological stress combined exposure on uterus in female rats. Life Scie (2021) 286:120053. doi: 10.1016/j.lfs.2021.120053
15. Chauhan NR, Kapoor M, Prabha Singh L, Gupta RK, Chand Meena R, Tulsawani R, et al. Heat stress-induced neuroinflammation and aberration in monoamine levels in hypothalamus are associated with temperature dysregulation. Neuroscience (2017) 358:79–92. doi: 10.1016/j.neuroscience.2017.06.023
16. An G, Chen X, Li C, Zhang L, Wei M, Chen J, et al. Pathophysiological changes in female rats with estrous cycle disorder induced by long-term heat stress. BioMed Res Int (2020) 2020:4701563. doi: 10.1155/2020/4701563
17. Roth Z, Meidan R, Braw-Tal R, Wolfenson D. Immediate and delayed effects of heat stress on follicular development and its association with plasma FSH and inhibin concentration in cows. J Reprod Fertil (2000) 120(1):83–90. doi: 10.1530/reprod/120.1.83
18. Zheng M, Nagaoka K, Watanabe G. Pre-pubertal exposure to high temperature impairs ovarian and adrenal gland function in female rats. J Vet Med Sci (2019) 81(2):279–86. doi: 10.1292/jvms.18-0644
19. Aldahhan RA, Stanton PG, Ludlow H, de Kretser DM, Hedger MP. Acute heat-treatment disrupts inhibin-related protein production and gene expression in the adult rat testis. Mol Cell Endocrinol (2019) 498:110546. doi: 10.1016/j.mce.2019.110546
20. Wang LI, Liu F, Luo Y, Zhu L, Li G. Effect of acute heat stress on adrenocorticotropic hormone, cortisol, interleukin-2, interleukin-12 and apoptosis gene expression in rats. BioMed Rep (2015) 3(3):425–9. doi: 10.3892/br.2015.445
21. Koko V, Djordjeviae J, Cvijiae G, Davidoviae V. Effect of acute heat stress on rat adrenal glands: a morphological and stereological study. J Exp Biol (2004) 207(Pt 24):4225–30. doi: 10.1242/jeb.01280
22. Djordjević J, Cvijić G, Davidović V. Different activation of ACTH and corticosterone release in response to various stressors in rats. Physiol Res (2003) 52:67–72.
23. Dou J, Montanholi YR, Wang Z, Li Z, Yu Y, Martell JE, et al. Corticosterone tissue-specific response in sprague dawley rats under acute heat stress. J Therm Biol (2019) 81:12–9. doi: 10.1016/j.jtherbio.2019.02.004
24. Mete F, Kilic E, Somay A, Yilmaz B. Effects of heat stress on endocrine functions & behaviour in the pre-pubertal rat. Indian J Med Res (2012) 135:233–9.
25. Lin CH, Tsai CC, Chen TH, Chang CP, Yang HH. Oxytocin maintains lung histological and functional integrity to confer protection in heat stroke. Sci Rep (2019) 9(1):18390. doi: 10.1038/s41598-019-54739-1
26. Bei M, Wang Q, Yu W, Han L, Yu J. Effects of heat stress on ovarian development and the expression of HSP genes in mice. J Therm Biol (2020) 89:102532. doi: 10.1016/j.jtherbio.2020.102532
27. Li J, Gao H, Tian Z, Wu Y, Wang Y, Fang Y, et al. Effects of chronic heat stress on granulosa cell apoptosis and follicular atresia in mouse ovary. J Anim Sci Biotechnol (2016) 7:57. doi: 10.1186/s40104-016-0116-6
28. Kasimanickam R, Kasimanickam V. Impact of heat stress on embryonic development during first 16 days of gestation in dairy cows. Sci Rep (2021) 11(1):14839. doi: 10.1038/s41598-021-94278-2
29. Lin CH, Chen SH, Chang CP, Lin KC. Hypothalamic impairment underlying heat intolerance in pregnant mice. Mol Cell Endocrinol (2019) 492:110439. doi: 10.1016/j.mce.2019.04.019
30. Mayvaneh F, Entezari A, Sadeghifar F, Baaghideh M, Guo Y, Atabati A, et al. Exposure to suboptimal ambient temperature during specific gestational periods and adverse outcomes in mice. Environ Sci pollut Res (2020) 27(36):45487–98. doi: 10.1007/s11356-020-10416-9
31. Watanabe YG. Immunohistochemical study on the fetal rat pituitary in hyperthermia-induced exencephaly. Zoolog Sci (2002) 19(6):689–94. doi: 10.2108/zsj.19.689
32. Dreiling CE, Carman FS 3rd, Brown DE. Maternal endocrine and fetal metabolic responses to heat stress. J Dairy Sci (1991) 74(1):312–27. doi: 10.3168/jds.S0022-0302(91)78175-7
33. Collier RJ, Doelger SG, Head HH, Thatcher WW, Wilcox CJ. Effects of heat stress during pregnancy on maternal hormone concentrations, calf birth weight and postpartum milk yield of Holstein cows. J Anim Sci (1982) 54(2):309–19. doi: 10.2527/jas1982.542309x
34. Nanas I, Barbagianni M, Dadouli K, Dovolou E, Amiridis GS. Ultrasonographic findings of the corpus luteum and the gravid uterus during heat stress in dairy cattle. Reprod Domest Anim. (2021) 56(10):1329–41. doi: 10.1111/rda.13996
35. Ullah S, Zhang M, Yu H, Mustafa S, Shafiq M, Wei Q, et al. Heat exposure affected the reproductive performance of pregnant mice: Enhancement of autophagy and alteration of subcellular structure in the corpus luteum. Reprod Biol (2019) 19(3):261–9. doi: 10.1016/j.repbio.2019.06.006
36. Guo H, Liu R, He J, Yao W, Zheng W. Heat stress modulates a placental immune response associated with alterations in the development of the fetal intestine and its innate immune system in late pregnant mouse. Front Physiol (2022) 13:841149. doi: 10.3389/fphys.2022.841149
37. Wells JC. Thermal environment and human birth weight. J Theor Biol (2002) 214(3):413–25. doi: 10.1006/jtbi.2001.2465
38. Samuels L, Nakstad B, Roos N, Bonell A, Chersich M, Havenith G, et al. Physiological mechanisms of the impact of heat during pregnancy and the clinical implications: review of the evidence from an expert group meeting. Int J Biometeorol (2022) 8:1505–13. doi: 10.1007/s00484-022-02301-6
39. Dahl GE, Skibiel AL, Laporta J. In utero heat stress programs reduced performance and health in calves. Vet Clin North Am Food Anim Pract (2019) 35(2):343–53. doi: 10.1016/j.cvfa.2019.02.005
40. Johnson JS, Boddicker RL, Sanz-Fernandez MV, Ross JW, Selsby JT, Lucy MC, et al. Effects of mammalian in utero heat stress on adolescent body temperature. Int J Hyperthermia (2013) 29(7):696–702. doi: 10.3109/02656736.2013.843723
41. Kim HD, Kim YJ, Jang M, Bae SG, Yun SH, Lee MR, et al. Heat stress during summer attenuates expression of the hypothalamic kisspeptin, an upstream regulator of the hypothalamic-Pituitary-Gonadal axis, in domestic sows. Anim (Basel) (2022) 12(21):2967. doi: 10.3390/ani12212967
42. Kronenberg F, Barnard RM. Modulation of menopausal hot flashes by ambient temperature. J Therm Biol (1992) 17(1):43–9. doi: 10.1016/0306-4565(92)90018-B
43. Ma L, Qi T, Zhang Y, Huang Y, Li S, Ying Q, et al. Occupational hazards and the onset of natural menopause. Maturitas (2023) 167:46–52. doi: 10.1016/j.maturitas.2022.08.012
44. Pace NP, Vassallo J, Calleja-Agius J. Gestational diabetes, environmental temperature and climate factors - from epidemiological evidence to physiological mechanisms. Early Hum Dev (2021) 155:105219. doi: 10.1016/j.earlhumdev.2020.105219
45. Thomas F, Renaud F, Benefice E, de Meeus T, Guegan JF. International variability of ages at menarche and menopause: patterns and main determinants. Hum Biol (2001) 73(2):271–90. doi: 10.1353/hub.2001.0029
46. https://www.who.int/health-topics/infertility#tab=tab_1; accessed March 28, 2023
47. Jensen PM, Sørensen M, Weiner J. Human total fertility rate affected by ambient temperatures in both the present and previous generations. Int J Biometeorol (2021) 65(11):1837–48. doi: 10.1007/s00484-021-02140-x
48. Mishra SR. Behavioural, physiological, neuro-endocrine and molecular responses of cattle against heat stress: an updated review. Trop Anim Health Prod (2021) 53(3):400. doi: 10.1007/s11250-021-02790-4
49. Hassan S, Jahanfar S, Inungu J, Craig JM. Low birth weight as a predictor of adverse health outcomes during adulthood in twins: a systematic review and meta-analysis. Syst Rev (2021) 10(1):186. doi: 10.1186/s13643-021-01730-5
50. Deindl P, Diemert A. From structural modalities in perinatal medicine to the frequency of preterm birth. Semin Immunopathol (2020) 42(4):377–83. doi: 10.1007/s00281-020-00805-0
51. Syed S, O'Sullivan TL, Phillips KP. Extreme heat and pregnancy outcomes: A scoping review of the epidemiological evidence. Int J Environ Res Public Health (2022) 19(4):2412. doi: 10.3390/ijerph19042412
52. Hansen PJ. Effects of heat stress on mammalian reproduction. Philos Trans R Soc Lond B Biol Sci (2009) 364(1534):3341–50. doi: 10.1098/rstb.2009.0131
53. Carter AM. Animal models of human pregnancy and placentation: alternatives to the mouse. Reproduction (2020) 160:129–43. doi: 10.1530/REP-20-0354
54. Sirard M-A. The ovarian follicle of cows as a model for human. In: Constantinescu G, Schatten H, editors. Animal models and human reproduction. New Jersey&Canada: Wiley-Blackwell (2017).
55. Aslanian-Kalkhoran L, Esparvarinha M, Nickho H, Aghebati-Maleki L, Heris JA, Danaii S, et al. Understanding main pregnancy complications through animal models. J Reprod Immunol (2022) 153:103676. doi: 10.1016/j.jri.2022.103676
56. Bonney EA. Demystifying animal models of adverse pregnancy outcomes: touching bench and bedside. Am J Reprod Immunol (2013) 69(6):567–84. doi: 10.1111/aji.12102
57. Moffett A, Shreeve N. Local immune recognition of trophoblast in early human pregnancy: controversies and questions. Nat Rev Immunol (2022), 1–14. doi: 10.1038/s41577-022-00777-2
58. Webster AJF. Metabolic responses of farm animals to high temperature. animal husbandry in warm climates: Proceedings of the international symposium on animal husbandry in warm climates. Viterbo, Italy: Pudoc (1991).
59. Abreu-Vieira G, Xiao C, Gavrilova O, Reitman ML. Integration of body temperature into the analysis of energy expenditure in the mouse. Mol Metab (2015) 4(6):461–70. doi: 10.1016/j.molmet.2015.03.001
60. Walter EJ, Carraretto M. The neurological and cognitive consequences of hyperthermia. Crit Care (2016) 20(1):199. doi: 10.1186/s13054-016-1376-4
61. Grattan DR, Ladyman SR. Neurophysiological and cognitive changes in pregnancy. Handb Clin Neurol (2020) 171:25–55. doi: 10.1016/B978-0-444-64239-4.00002-3
62. Friebe A, Douglas AJ, Solano E, Blois SM, Hagen E, Klapp BF, et al. Neutralization of LPS or blockage of TLR4 signaling prevents stress-triggered fetal loss in murine pregnancy. J Mol Med (2011) 89(7):689–99. doi: 10.1007/s00109-011-0743-5
63. Goldman L, Winget C, Hollingshead GW, Levine S. Postweaning development of negative feedback in the pituitary-adrenal system of the rat. Neuroendocrinology (1973) 12:199–211. doi: 10.1159/000122169
64. Romeo RD, Lee SJ, Chhua N, McPherson CR, McEwen BS. Testosterone cannot activate an adult-like stress response in prepubertal male rats. Neuroendocrinology (2004) 79(3):125–32. doi: 10.1159/000077270
65. Irwin JL, Meyering AL, Peterson G, Glynn LM, Sandman CA, Hicks LM, et al. Maternal prenatal cortisol programs the infant hypothalamic-pituitary-adrenal axis. Psychoneuroendocrinology (2021) 125:105106. doi: 10.1016/j.psyneuen.2020.105106
66. Solano ME, Arck PC. Steroids, pregnancy and fetal development. Front Immunol (2019) 10:3017. doi: 10.3389/fimmu.2019.03017
67. Blasco MJ, Lopez Bernal A, Turnbull AC. 11b-hydroxysteroid dehydrogenase activity of the human placenta during pregnancy. Horm metabol Res (1986) 18(9):638–41. doi: 10.1055/s-2007-1012393
68. Guo Y, Chen H, Wang Q-J, Qi X, Li Q, Fu W, et al. Prolonged melatonin treatment promote testicular recovery by enhancing RAC1-mediated apoptotic cell clearance and cell junction-dependent spermatogensis after heat stress. Theriogenology (2021) 162:22–31. doi: 10.1016/j.theriogenology.2020.12.015
69. Wieczorek A, Perani CV, Nixon M, Constancia M, Sandovici I, Zazara DE, et al. Sex-specific regulation of stress-induced fetal glucocorticoid surge by the mouse placenta. Am J Physiol Endocrinol Metab (2019) 317(1):E109–E20. doi: 10.1152/ajpendo.00551.2018
70. Buss C, Davis EP, Shahbaba B, Pruessner JC, Head K, Sandman CA. Maternal cortisol over the course of pregnancy and subsequent child amygdala and hippocampus volumes and affective problems. Proc Natl Acad Sci U S A. (2012) 109(20):E1312–9. doi: 10.1073/pnas.1201295109
71. Green ES, Arck PC. Pathogenesis of preterm birth: bidirectional inflammation in mother and fetus. Semin Immunopathol (2020) 42(4):413–29. doi: 10.1007/s00281-020-00807-y
72. Archer TL, Knape K, Liles D, Wheeler AS, Carter B. The hemodynamics of oxytocin and other vasoactive agents during neuraxial anesthesia for cesarean delivery: findings in six cases. Int J Obstet Anesth (2008) 17(3):247–54. doi: 10.1016/j.ijoa.2008.03.003
73. Luo M, Li L, Xiao C, Sun Y, Wang GL. Heat stress impairs mice granulosa cell function by diminishing steroids production and inducing apoptosis. Mol Cell Biochem (2016) 412(1-2):81–90. doi: 10.1007/s11010-015-2610-0
74. Shimizu T, Ohshima I, Ozawa M, Takahashi S, Tajima A, Shiota M, et al. Heat stress diminishes gonadotropin receptor expression and enhances susceptibility to apoptosis of rat granulosa cells. Reproduction (2005) 129(4):463–72. doi: 10.1530/rep.1.00502
75. Nielsen SE, Herrera AY. 1.14 - sex steroids, learning and memory. In: Pfaff DW, Joëls M, editors. Hormones, brain and behavior, 3rd ed. Oxford: Academic Press (2017) 399–422.
76. Charkoudian N, Hart ECJ, Barnes JN, Joyner MJ. Autonomic control of body temperature and blood pressure: influences of female sex hormones. Clin Auton Res (2017) 27(3):149–55. doi: 10.1007/s10286-017-0420-z
77. Silva NL, Boulant JA. Effects of testosterone, estradiol, and temperature on neurons in preoptic tissue slices. Am J Physiol (1986) 250(4):R625–32. doi: 10.1152/ajpregu.1986.250.4.R625
78. Khan A, Dou J, Wang Y, Jiang X, Khan MZ, Luo H, et al. Evaluation of heat stress effects on cellular and transcriptional adaptation of bovine granulosa cells. J Anim Sci Biotechnol (2020) 11:25. doi: 10.1186/s40104-019-0408-8
79. Yang F, Zheng Q, Jin L. Dynamic function and composition changes of immune cells during normal and pathological pregnancy at the maternal-fetal interface. Front Immunol (2019) 10:2317. doi: 10.3389/fimmu.2019.02317
80. Thiele K, Hierweger AM, Riquelme JIA, Solano ME, Lydon JP, Arck PC. Impaired progesterone-responsiveness of CD11c(+) dendritic cells affects the generation of CD4(+) regulatory T cells and is associated with intrauterine growth restriction in mice. Front Endocrinol (2019) 10:96. doi: 10.3389/fendo.2019.00096
81. Shah NM, Lai PF, Imami N, Johnson MR. Progesterone-related immune modulation of pregnancy and labor. Front Endocrinol (2019) 10:198. doi: 10.3389/fendo.2019.00198
82. Mulac-Jeričević B, Šućurović S, Gulic T, Szekeres-Bartho J. The involvement of the progesterone receptor in PIBF and gal-1 expression in the mouse endometrium. Am J Reprod Immunol (2019) 81(5):e13104. doi: 10.1111/aji.13104
83. Whirledge SD, Oakley RH, Myers PH, Lydon JP, DeMayo F, Cidlowski JA. Uterine glucocorticoid receptors are critical for fertility in mice through control of embryo implantation and decidualization. Proc Natl Acad Sci U S A. (2015) 112(49):15166–71. doi: 10.1073/pnas.1508056112
84. Carr BR, Parker CR Jr., Madden JD, MacDonald PC, Porter JC. Maternal plasma adrenocorticotropin and cortisol relationships throughout human pregnancy. Am J Obstet Gynecol (1981) 139(4):416–22. doi: 10.1016/0002-9378(81)90318-5
85. Horowitz M. Heat acclimation, epigenetics, and cytoprotection memory. Compr Physiol (2014) 4(1):199–230. doi: 10.1002/cphy.c130025
86. Tetievsky A, Horowitz M. Posttranslational modifications in histones underlie heat acclimation-mediated cytoprotective memory. J Appl Physiol (1985). (2010) 109(5):1552–61. doi: 10.1152/japplphysiol.00469.2010
87. Horowitz M. Heat acclimation-mediated cross-tolerance: Origins in within-life epigenetics? Front Physiol (2017) 8:548. doi: 10.3389/fphys.2017.00548
88. Alexander LV, Zhang X, Peterson TC, Caesar J, Gleason B, Klein Tank AMG, et al. Global observed changes in daily climate extremes of temperature and precipitation. J Geophys Res (2006) 111:D05109. doi: 10.1029/2005JD006290
89. Lateef OM, Akintubosun MO. Sleep and reproductive health. J Circadian Rhythms. (2020) 18:1. doi: 10.5334/jcr.190
90. Obradovich N, Migliorini R, Mednick SC, Fowler JH. Nighttime temperature and human sleep loss in a changing climate. Sci Adv (2017) 3(5):e1601555. doi: 10.1126/sciadv.1601555
91. Clayton S. Climate change and mental health. Curr Environ Health Rep (2021) 8(1):1–6. doi: 10.1007/s40572-020-00303-3
92. Clayton S. Climate anxiety: Psychological responses to climate change. J Anxiety Disord (2020) 74:102263. doi: 10.1016/j.janxdis.2020.102263
Keywords: climate change, pregnancy, hormones, endocrine system, heat stress, immune system, preterm birth, fetal development
Citation: Yüzen D, Graf I, Diemert A and Arck PC (2023) Climate change and pregnancy complications: From hormones to the immune response. Front. Endocrinol. 14:1149284. doi: 10.3389/fendo.2023.1149284
Received: 21 January 2023; Accepted: 21 March 2023;
Published: 05 April 2023.
Edited by:
Alexander N. Comninos, Imperial College Healthcare NHS Trust, United KingdomReviewed by:
Per M. Jensen, University of Copenhagen, DenmarkAmy Wise, University of the Witwatersrand, South Africa
Copyright © 2023 Yüzen, Graf, Diemert and Arck. This is an open-access article distributed under the terms of the Creative Commons Attribution License (CC BY). The use, distribution or reproduction in other forums is permitted, provided the original author(s) and the copyright owner(s) are credited and that the original publication in this journal is cited, in accordance with accepted academic practice. No use, distribution or reproduction is permitted which does not comply with these terms.
*Correspondence: Petra Clara Arck, p.arck@edu.de
†These authors have contributed equally to this work and share first authorship