- 1Public Research Platform, First Hospital of Jilin University, Changchun, Jilin, China
- 2College of Basic Medical Sciences, Jilin University, Changchun, Jilin, China
- 3Nephrology Department, First Hospital of Jilin University, Changchun, Jilin, China
Diabetic nephropathy (DN) is the most common microvascular complication in diabetes and one of the leading causes of end-stage renal disease. The standard treatments for patients with classic DN focus on blood glucose and blood pressure control, but these treatments can only slow the progression of DN instead of stopping or reversing the disease. In recent years, new drugs targeting the pathological mechanisms of DN (e.g., blocking oxidative stress or inflammation) have emerged, and new therapeutic strategies targeting pathological mechanisms are gaining increasing attention. A growing number of epidemiological and clinical studies suggest that sex hormones play an important role in the onset and progression of DN. Testosterone is the main sex hormone in males and is thought to accelerate the occurrence and progression of DN. Estrogen is the main sex hormone in females and is thought to have renoprotective effects. However, the underlying molecular mechanism by which sex hormones regulate DN has not been fully elucidated and summarized. This review aims to summarize the correlation between sex hormones and DN and evaluate the value of hormonotherapy in DN.
Introduction
Diabetic nephropathy (DN) is one of the most common and serious complications of diabetes mellitus and a major cause of chronic kidney disease and end-stage renal disease (ESRD) (1–4). The occurrence and progression of DN are closely related to patient blood glucose levels, blood pressure, genetic background and age (5, 6). Unlike other renal diseases, once macroalbuminuria occurs, DN will remain throughout life, which makes DN a major cause of death in patients with diabetes. DN patients at the end stage of renal failure rely on dialysis and kidney transplantation. Therefore, preventing and treating DN has become a pressing problem worldwide. Many studies have shown that the occurrence and development of DN are closely correlated with sex (7). In addition to social roles, psychological cognition and behavioral habits, the most important difference between the sexes is sex hormones. Especially in women, sex hormones vary greatly throughout life, from infancy to adolescence, sexual maturity, pregnancy, perimenopause and postmenopause. However, the underlying molecular mechanism by which sex hormones regulate DN has not been fully elucidated. Moreover, based on the impact of sex hormone imbalances on the development of DN, hormone therapy in patients with diabetes may alleviate diabetic kidney injury to a certain extent and is a potentially valuable therapeutic strategy for DN patients.
In this article, we summarized the effects of sex hormone changes on DN development by searching and reviewing published articles. We hope our work will provide information on the correlation between sex hormones and DN and provide new clues for the treatment of DN.
Sex hormones
Sex hormones are steroidal hormones synthesized mainly by the gonads, the placenta, and the reticular cortex of the adrenal gland in animals. In female animals, the ovaries mainly secrete two types of sex hormones: estrogen and progesterone. In male animals, the testes secrete androgens, mainly testosterone.
The synthesis of sex hormones is based on cholesterol, which is converted to pregnenolone by cytochrome P-11A (CYP11A). Pregnenolone can be converted to progesterone by 3βHSDI and transported from the outer mitochondrial membrane to the inner mitochondrial membrane by transporters (8). There are two ways to synthesize androstenedione. First, pregnenolone is converted to dehydroepiandrosterone by CYP17 and then to androstenedione; second, progesterone is converted to 17α-hydroxyprogesterone and then to androstenedione (8). Androstenedione is converted to testosterone by the enzyme 17HSD3, which is converted to estradiol via aromatase (CYP19) (8, 9). Figure 1 shows the synthesis of sex hormones.
Most sex hormones are metabolically inactivated in a similar manner: by forming more water-soluble conjugates, such as glucuronides or sulfate esters, in metabolic organs, such as the liver and kidneys. These conjugates are then excreted in urine or secreted into the intestine with bile and excreted in feces (10, 11).
Testosterone
Testosterone is a steroid hormone. It is the main sex hormone and anabolic hormone in the male body and is mainly synthesized by the interstitial cells of the testicles. Other organs, such as the adrenal glands and ovaries, also produce small amounts of testosterone.
Androgen receptor (AR) is encoded by the AR gene on the X chromosome and is widely distributed in various tissues and organs, including the endothelium and kidney (12). AR plays an important role in the development and maintenance of the reproductive, musculoskeletal, cardiovascular, immune, neurological and hematopoietic systems (12, 13). When not bound to testosterone, AR is bound in the cytoplasm by heat shock protein (HSP) and chaperone proteins. When interacting with testosterone or dihydrotestosterone, AR is released from HSP and chaperone proteins and translocates to the nucleus to produce the corresponding biological effects (14). Sankar et al. reported that AR was a key determinant of the response to testosterone, and circulating levels of testosterone can influence spatial cognition in adult males (15).
Estrogen
Estrogens are produced by the placenta and ovaries of female animals and promote the development of secondary sexual characteristics and the maturation of sexual organs in females. There are three main types of estrogens in females, estrone (E1), estradiol (E2) and estriol (E3). These estrogens play important roles in regulating many physiological functions, such as cell proliferation and differentiation, development, body homeostasis and metabolism (16–20). Under physiological and pathophysiological conditions, the effects of estrogen are mediated by estrogen receptors α/β and G protein-coupled estrogen receptors (GPER). These receptors are involved in the development of many diseases, including DN, cancer, neurodegenerative diseases, and cardiovascular, metabolic and autoimmune diseases (21–24).
Alterations in sex hormones in diabetes
Alterations in sex hormones between the sexes
Under physiological conditions, sex hormone levels and their functions in men and women alter with increasing age. Both testosterone and estrogen have been found to decline with age in men and women (25–28). Gambineri et al. summarized the reasons for the difference in circulating sex hormone levels between the sexes, and they believed that it is due to the difference in the synthesis site of sex hormones, the conversion rate of sex hormones to each other, and the binding degree of sex hormones to sex hormone binding globulin (SHBG) in the two sexes (29).
Alterations in sex hormones in diabetes
Diabetes can cause an imbalance in sex hormones in patients (30). Studies have shown that compared with men without diabetes, men with diabetes have decreased levels of testosterone and increased levels of E2. However, testosterone levels are higher and E2 levels are lower in women with diabetes than in those without diabetes, suggesting that diabetes is associated with an imbalance in sex hormones (30–37). In females with diabetes (compared with females without diabetes), the decreased level of E2 may reduce creatinine clearance and increase urine albumin excretion and tubular fibrosis in the kidney, which may increase the risk of developing renal complications (38).
Insulin levels have a significant impact on the functional regulation of the hypothalamic-pituitary-gonadal axis (HPGA) (39). Normally, insulin is secreted by pancreatic β cells. Then, it binds to insulin receptors and activates intracellular protein tyrosine kinase (PTK). Activated PTK can phosphorylate and activate insulin receptor substrates (IRS) to activate phosphoinositide 3-kinase (PI3K). The activated PI3K signaling cascade enhances gonadotropin-releasing hormone (GnRH) secretion in the hypothalamus, which stimulates the pituitary secretion of luteotropic hormone (LH) and follicle-stimulating hormone (FSH) and eventually induces the release of sex hormones by the gonads (40). Approximately 5% of sex steroids are present in the blood and enter cells through specific receptors on the plasmalemma (41). During diabetes, altered levels of SHBG, increased levels of oxidative stress and increased levels of CYP19 activity are present in adipose tissue. This results in the conversion of testosterone and androstenedione to estradiol and estrone, respectively, which contribute to reducing serum testosterone concentrations in men with diabetes (42–47). In addition, the disruption of glucolipid metabolism, the reduced bioavailability of insulin during diabetes and the reduced activity of CYP19 in the ovaries of diabetic rats, as determined by Bozkurt et al., might be responsible for the reduced levels of estradiol in females with diabetes (48–50).
The level of insulin can be affected by leptin. Leptin is a type of adipokine that is secreted by adipose tissue. It can regulate energy metabolism and may regulate reproductive function by regulating the release of GnRH in the hypothalamus (39, 51, 52). The level of insulin can also affect the generation of leptin (53, 54). Under normal circumstances, leptin phosphorylates IRS-2 on hypothalamic leptin receptors, activating PI3K and stimulating the release of GnRH (55, 56). Under diabetic circumstances, the feedback between insulin and leptin is disordered, thus impairing the release of GnRH and ultimately reducing sex hormone secretion.
In contrast, altered levels of sex hormones may be a predisposing factor for diabetes. CYP19 is the limiting enzyme for estradiol synthesis. Jones et al. found that in aromatase-knockout (ArKO) female mice, glucose oxidation was decreased and obesity and insulin levels were increased (57). A study showed that decreased CYP19 activity combined with low concentrations of dihydrotestosterone (DHT) downregulates the expression of transforming growth factor-β (TGF-β) and type IV collagen and inhibits the level of glomerulosclerosis and tubular interstitial fibrosis, thus attenuating the progression of renal complications in male diabetic rats (35). Takeda et al. showed that a short-term E2 treatment could reverse the development of glucose intolerance and insulin resistance by enhancing lipid metabolism in male ArKO mice (58).
Role of sex hormones in the development of DN
Sex differences in the development of DN
The occurrence and development of DN are affected by sex to a great extent (59–61). Observations in humans and animals showed that the level of sex steroids in males and females are altered by DN. Plasma testosterone levels in men were decreased to levels similar to those in women, while plasma estradiol levels in women were decreased to levels similar to those in men. In many DN models, male animals tend to progress more quickly than female animals. In type 1 and type 2 diabetes, the prevalence of microproteinuria and macroproteinuria is higher in males than in females, and the risk of microproteinuria and progression to macroproteinuria is also higher (7, 62–65). This phenomenon is also seen in nondiabetic renal diseases. Neugarten et al. found that men with chronic renal disease show a more rapid decline in renal function than women with chronic renal disease (66).
However, other studies showed an opposite result, as they reported that women with diabetes have a higher risk of progressing to ESRD than men with diabetes (67). When the women with diabetes included in the statistics were older (postmenopausal), they had a higher rate of progression to ESRD (68). In the Irbesartan DN trial and the angiotensin II (AngII) receptor antagonist Losartan study, postmenopausal women with diabetes developed end-stage renal disease at a faster rate than men with diabetes (69, 70). In addition, age at diagnosis of type 1 diabetes also has an impact on the timing of the onset of ESRD in both sexes. Men diagnosed with type 1 diabetes before puberty had a delayed onset of ESRD, while women diagnosed at puberty face a higher risk of ESRD (71, 72).
Role of testosterone in DN
The risk of renal complications in men with diabetes is higher than that in premenopausal women with diabetes. Testosterone is considered to be more conducive to the genesis of DN in males. Kang et al. reported that men have a higher risk of renal complications (73). Sharon et al. reported that the decrease in testosterone may partly attenuate kidney injury in males (74). Jan et al. reported that men with type 1 diabetes have a higher risk of ESRD and mortality (75). In contrast, the effect of testosterone on the progression of DN in females with diabetes is rarely mentioned, and females are considered to be less influenced by testosterone (73).
Role of estrogen in DN
Changes in estrogen levels affect the occurrence of DN, and estrogen may have different effects in males and females with diabetes (76, 77). As mentioned above, the level of circulating testosterone in men with diabetes is decreased, while the level of E2 is increased (33, 36, 37). The increased level of E2 may increase the risk of renal complications in men (38, 60, 78). In male STZ-induced diabetic rats, inhibition of testosterone transformation to estradiol attenuates inflammation and the expression of type IV collagen and TGF-β; hence, the progression of DN is reduced (78).
As the most important sex hormone in women, estrogen has been shown to prevent podocyte apoptosis. Estrogen can also inhibit type I/IV collagen synthesis in mesangial cells and promote the degradation of the extracellular matrix, which are critical factors that induce tubular fibrosis (79–81). The effect of estrogen on the female kidney may vary at the postmenopause stage. William et al. reported that women at the postmenopause stage have a higher risk of renal complications (70). Lewis et al. found that kidney function was reduced in women with diabetes with an average age of 58 (69). Studies have shown that women who undergo ovariectomy (OVX) have a higher risk of diabetes and other complications (82–84). Mankhey et al. reported that in STZ-induced diabetic female rats, OVX could enhance DN, whereas 17-β-estradiol replacement therapy could attenuate DN (38). Therefore, estrogen is considered to have a renal protective function in women with diabetes.
Sex hormones affect the genesis of DN and its underlying mechanisms
Patients with diabetes who progress to nephropathy have significantly higher initial mean blood pressure, cholesterol, HbA1c, low-density lipoprotein (LDL) cholesterol and triglyceride levels (85). The development of DN includes renal hemodynamic changes, sugar/lipid metabolic disorders, and the effects of oxidative stress and inflammation. These changes cause glomerular basement membrane thickening, mesangial matrix accumulation, glomerular sclerosis and tubular epithelial cell injury, which eventually lead to renal tubular fibrosis, proteinuria and the leakage of large molecules (86–88).
●Oxidative stress and inflammation
In the diabetic state, NADPH oxidases (Nox proteins) are activated to produce excess reactive oxygen species (ROS) through the electron transport chain (89). When too many ROS accumulate, they attack organs, including the kidney, and this is accompanied by the depletion of antioxidants. Additionally, the oxidative/antioxidant system balance is disrupted, resulting in oxidative stress (89, 90). The kidney contains a high density of mitochondria. Excess ROS lead to oxidative damage to mitochondrial proteins and mitochondrial DNA (mtDNA). Then, the kidney fails to filter and reabsorb Na+, glucose and other metabolites from the urine, and vascular permeability is increased (91, 92). Testosterone may reduce the activation of STAT3 to increase the production of ROS (93). Mustafa and Mehmet found that estradiol had positive effects on the antioxidant defense system and tissue lipid peroxidation in OVX diabetic rats, possibly by enhancing the antioxidant activities in the kidney, thus protecting against diabetes (94). Hong et al. found that estrogen can inactivate Nox, inhibit the production of superoxide anions, and reduce oxidative stress in the kidney, thus reducing kidney injury (95, 96).
The high glucose environment of diabetes also leads to increased production of advanced glycation end products (AGEs), which interact with their receptor RAGE to activate NF-κB. Then, inflammatory responses occur, producing multiple proinflammatory and profibrotic molecules (97–100). T and B lymphocytes are subsequently activated (101). Activated T lymphocytes can produce proinflammatory cytokines (e.g., IL-17, IL-6, TNF-α and IFN-γ) or recruit and activate macrophages (102–108). Activated B lymphocytes can induce the formation of inflammatory immune complexes and produce proinflammatory cytokines (e.g., IL-6, IL-10 and TNF) (106, 109–111). After proinflammatory cytokines are released, the cascade amplifies the NF-κB signal, produces more proinflammatory cytokines and recruits adjacent macrophages to the inflammatory site in tubules, which leads to kidney infiltration, increases the expression of proinflammatory and profibrotic molecules (e.g. type I/IV collagen and TGF-β), and exacerbates renal tubular fibrosis (101, 111).
In the diabetic state, testosterone can phosphorylate and activate C-jun (a molecule that functions in renal inflammation) (112–114). Activated C-jun may upregulate monocyte chemoattractant protein-1 (MCP-1) expression. This promotes tubular epithelial cells to attract macrophages to the injury site of tubules, causing local inflammation and tubular cell apoptosis. The activation of C-jun can also upregulate the expression kidney injury molecule-1 and directly induce tubular fibrosis (114, 115). In SD male rats, once inflammation occurs in the kidney, testosterone can upregulate the expression of the proinflammatory cytokine TNF-α to exacerbate the inflammatory response and increase the expression of profibrotic substances to promote tubule epithelial-mesenchymal transition (EMT) and promote renal fibrosis (116).
Tubular fibrosis is the outcome of the inflammatory response in the kidney and is led by TGF-β (a key molecule that can stimulate the production of several extracellular matrix proteins that accumulate in the diabetic kidney, including type IV collagen, fibronectin and laminin). EMT of the renal tubular epithelium leads to tubular fibrosis (117, 118). In the state of diabetes, DHT upregulates the expression of TGF-β in diabetic male rats and accelerates the production of the early fibrosis marker connective tissue growth factor (CTGF). Additionally, epithelial cells acquire a fibroblast phenotype, leading to the genesis of tubular fibrosis (60).
Estrogen can interfere with the expression of TGF-β and its downstream signaling pathway via members of the small mother against decapentaplegic (Smad) protein family (Smad2/Smad3/Smad6/Smad7) (80, 119). Studies have shown that in STZ-induced diabetic female rats, E2 regulates the activity of TGF-β by downregulating profibrotic signaling molecules (Smad2, Smad3) and upregulating antifibrotic signaling molecules (Smad6, Smad7) (80). Thus, E2 can reduce proteinuria and ECM protein expression associated with diabetic glomerulosclerosis and renal tubular fibrosis and play a renoprotective role in females with diabetes (80). Regulation of casein kinase II (CK2) is another mechanism by which E2 may regulate TGF-β activity. CK2 is a serine/threonine protein kinase that, when activated, phosphorylates early growth reactivity 1 (EGR-1). EGR-1 typically binds to specific protein 1 (Sp1), preventing Sp1 from binding to target sequences. Ck2 induces EGR-1 phosphorylation in response to TGF-β to prevent the formation of the EGR-1/Sp1 complex, and the level of free Sp1 increases. Sp1, in turn, binds to target sequences in the promoters of type IV collagen and increases its synthesis. In murine mesangial cells, E2 treatment prevented the TGF-β-induced increase in CK2 expression and activity, thereby inhibiting TGF-β signaling and type IV collagen upregulation (120).
In addition to regulating TGF-β expression and activity in renal cells, E2 can also indirectly regulate TGF-β in the kidney by regulating macrophage infiltration. Macrophages are a key source of TGF-β in diabetic kidneys. In a spontaneously hypertensive rat model of kidney disease, the level of macrophage infiltration in the kidney was higher in males than in females, and OVX in females increased the number of macrophages. Similarly, OVX in diabetic female rats increased macrophage infiltration, and this effect could be normalized by E2 treatment (80, 121). These data suggest that E2 inhibits macrophage infiltration, thereby preventing the production of TGF-β by a major source and potentially protecting the kidney from injury.
●Hemodynamic changes
Increases in ROS are generated by persistent hyperglycemia and can lead to dilatation of the afferent glomerular arteriole, hyperfiltration, hypertransfusion and high internal pressure in the kidney in the early stages of diabetes (122). A prolonged high filtration load due to high glucose increases sodium-glucose cotransporter protein 2 levels in the proximal tubules, and the resorption of glucose and sodium chloride increases. This leads to dysfunctional tubuloglomerular feedback and results in the disruption of the afferent/efferent arteriole balance and increased glomerular unit plasma flow (123). This abnormal status ultimately increases the renal glomerular filtration rate (GFR) and causes glomerulosclerosis.
Before adolescence, sex does not play a significant role in the incidence of DN (124). With aging and the occurrence of chronic complications associated with diabetes mellitus, DN tends to begin earlier in men than in women because testosterone can activate the renin-angiotensin-aldosterone system (RAAS) (73). The RAAS is one of the primary control systems that regulates the balance of blood pressure and fluids, and the kidney is the organ that activates the RAAS. The major bioactive hormone in the RAAS is AngII, which is cleaved from angiotensinogen and can promote vasoconstriction, fibrosis, inflammation and apoptosis (125–128). AngII receptors can be divided into two types according to their length: ATR1 (40 kDa) and ATR2 (41 kDa). ATR1 is considered to be associated with increased blood pressure and vasoconstriction, while AT2R is considered to be associated with reduced blood pressure and inflammation inhibition (127, 128). DHT upregulates ATR1 expression in sexually mature SD male rats (73). The activity of AngII might be modulated by angiotensin-converting enzyme 2 (ACE2) or 3β-HSD4 in males. ACE2 is a zinc metalloproteinase that may degrade AngII to Ang-(1-7) (128–130). Oudit et al. found that the loss of ACE2 exacerbated the degree of glomerulosclerosis in male mice (131). 3β-HSD4 is a ketone reductase whose activity is regulated by angiotensin; it can reduce testosterone and progesterone to inactive metabolites. Under normal conditions, 3β-HSD4 protects the kidney from the potential negative effects of testosterone; in patients with diabetes with increased AngII levels, the loss of 3β-HSD4 activity may increase the susceptibility of the kidney to testosterone-induced damage (132).
Estrogen has a regulatory effect on the RAAS. It can attenuate AngII-induced hypertension and reduce renal insufficiency (73, 130, 133–135). Nitric oxide (NO) can dilate blood vessels, and endothelial cells produce NO through endothelial nitric oxide synthase (eNOS) to regulate vascular tone (136). NO can counteract the vasoconstrictive effects of AngII (137). Acute hyperglycemia induces a state of oxidative stress in the endothelium, which reduces NO production and leads to endothelial dysfunction (137). Estrogen can upregulate eNOS expression to accelerate NO release or increase NO bioavailability to relax blood vessels and lower blood pressure, thereby reducing glomerular sclerosis (138–141). Estrogen can also stimulate NO release and attenuate glomerular sclerosis and renal fibrosis by upregulating ATR2 expression in the renal medulla (142).
●Metabolic disorders
There are two aspects of abnormal glucose metabolism in patients with diabetes. AGEs bind to their receptors to activate the NF-κB pathway and stimulate the production of vascular endothelial growth factor (VEGF), TGF-β and MCP, leading to glomerular podocyte loss, expansion of the glomerular extracellular matrix and progressive glomerulosclerosis (143). Second, protein kinase C is activated by high glucose levels. This results in decreased production of eNOS and increased production of VEGF, which destabilize the endothelial microenvironment and activate the NF-κB pathway. The NF-κB-mediated inflammatory response leads to tubular fibrosis (99).
Persistent hyperglycemia in patients with diabetes can promote fatty acid synthesis and triglyceride accumulation. Excessive lipid accumulation in the glomerulus and renal tubules leads to podocyte dysfunction and damage to proximal tubular epithelial cells and tubular interstitial tissue (144). In addition, proteinuria in patients with diabetes may also serve as a carrier of fatty acids in urine. This leads to the accumulation of fatty acids in the kidney, thus exacerbating renal tubular injury in patients with diabetes (145). In OVX diabetic female rats, due to the lack of estrogen, lipid metabolism disorders occur, and fasting blood glucose levels and the insulin resistance value (HOMA-IR) were significantly increased compared with those in the control group (146).
Generally, glucose/lipid metabolic disorders may induce DN through oxidative stress, inflammation and hemodynamic changes. Therefore, the role of sex hormones in the modulation of these processes is the same as stated above.
The effects of sex hormones that may function in the occurrence of DN are illustrated in Figure 2, and the molecules affected by sex hormones in the progression of DN are listed in Table 1.
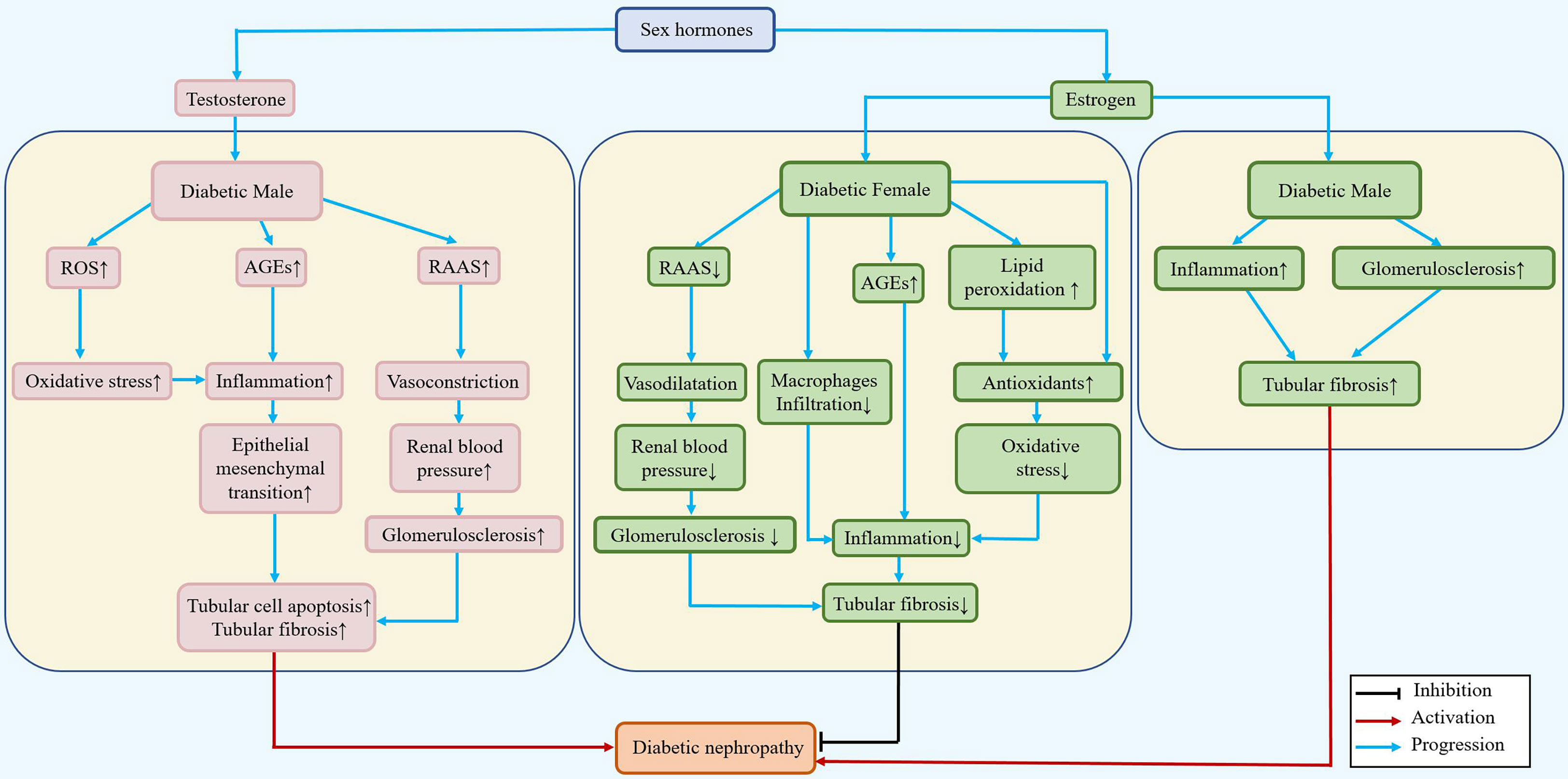
Figure 2 Differential roles of estrogen/testosterone in the pathogenesis of DN. During diabetes, testosterone can increase the renal blood pressure and inflammatory response, accelerate the epithelial mesenchymal transition, and lead to renal tubular fibrosis in males. In females, estradiol can reduce renal tubular fibrosis by reducing blood pressure, downregulating the inflammatory response and epithelial mesenchymal transition, and playing a renoprotective role.
Effects of sex hormone replacement therapies for DN
Effects of sex hormone replacement therapies in females with DN
Using E2 supplementation therapy for DN obtains good results in reducing kidney injury in women; for example, Szekacs et al. reported that in postmenopausal women with DN, estradiol supplementation reduces albuminuria (147). Raloxifene is a type of selective estrogen receptor modulator. It may attenuate glomerulosclerosis and albuminuria in women with DN and slow the progression of nephropathy (148–151). In addition, Bahaa et al. also found that progesterone treatment can attenuate DN in females (152). However, the risk or side effects of sex hormone therapies are nonnegligible. Eliassen et al. reported that E2 supplementation in premenopausal women increases their risk of breast cancer, but Dixon et al. found that raloxifene does not have side effects similar to those of E2 (149, 153). Moreover, the side effects of progesterone in the treatment of DN have been less frequently reported (152).
Effects of sex hormone replacement therapies in males with DN
Using sex hormone therapy for males with DN has been less commonly reported. Qin Xu et al. found that DHT has a dose-dependent effect in DN male rats. DHT at low concentrations (0.75 mg) can partly ease the progression of nephropathy, while DHT at high concentrations (2.0 mg) has the opposite effects in the kidney (154).
Icariin is a recently discovered GPER agonist. Qi et al. reported that icariin has antioxidative stress and antifibrotic effects in DN male rats, but whether it has side effects is unclear and not reported (155).
Table 2 summarizes the existing preclinical/clinical/animal experiments using sex hormone replacement therapies and their roles in the treatment of DN models.
Conclusions
In summary, many studies have shown that the occurrence and progression of DN are closely related to sex hormones. Testosterone can exacerbate DN by activating the RAAS or phosphorylating C-jun to induce tubular fibrosis, so DN usually progresses faster in male patients than in female patients. Estradiol can upregulate the expression of eNOS and increase the level of NO to alleviate the vasoconstriction effect of AngII to reduce tubular fibrosis. In addition, estradiol can alter the level of Smad family members and reduce macrophage infiltration and CK2 activation to alleviate tubular fibrosis. Thus, estradiol is thought to play a protective role in DN. Along with that for new targets for treatment, understanding the effect of sex hormones will provide a new combined therapeutic strategy for DN. Particular challenges are presented and placed within the context of future treatments against DN.
Author contributions
WW and DY conceived the manuscript. JL and DY drafted the manuscript. JL drew the figures. JL, WS and WW proofread the manuscript and made revisions. LL and XA collected the references. All authors contributed to the article and approved the submitted version.
Funding
This work was supported in part by National Natural Science Foundation of China (82000688 to WW), Jilin International Collaboration Grant (20220402066GH to DY), Natural Science Foundation of Jilin Province (20210101339JC to WW and 20200201428JC to WS), the Subject Arrangement Program from Science and Technology Department of Jilin Province (20200201123JC to DY), and Science and technology research project of Jilin Provincial Department of Education (JJKH20211185KJ to WW).
Conflict of interest
The authors declare that the research was conducted in the absence of any commercial or financial relationships that could be construed as a potential conflict of interest.
Publisher’s note
All claims expressed in this article are solely those of the authors and do not necessarily represent those of their affiliated organizations, or those of the publisher, the editors and the reviewers. Any product that may be evaluated in this article, or claim that may be made by its manufacturer, is not guaranteed or endorsed by the publisher.
References
1. Erfanpoor S, Etemad K, Kazempour S, Hadaegh F, Hasani J, Azizi F, et al. Diabetes, hypertension, and incidence of chronic kidney disease, is there any multiplicative or additive interaction? Int J Endocrinol Metab (2021) 19:e101061. doi: 10.5812/ijem.101061
2. Ahmed MA, Ferede YM, Takele WW. Incidence and predictors of chronic kidney disease in type-II diabetes mellitus patients attending at the amhara region referral hospitals, Ethiopia, a follow-up study. PLoS One (2022) 17:e0263138. doi: 10.1371/journal.pone.0263138
3. Caramori ML, Rossing P. Diabetic kidney disease. In: Feingold KR, Anawalt B, Blackman MR, Boyce A, Chrousos G, Corpas E KR, Anawalt B, Blackman MR, Boyce A, Chrousos G, Corpas E, et al. edtors. Endotext [Internet] South Dartmouth MA: MDText.com, Inc. (2022) 2000.
4. Mishriky BM, Cummings DM, Powell JR. Diabetes-related microvascular complications - a practical approach. Prim Care (2022) 49:239–54. doi: 10.1016/j.pop.2021.11.008
5. Weldegiorgis M, Woodward M. The impact of hypertension on chronic kidney disease and end-stage renal disease is greater in men than women, a systematic review and meta-analysis. BMC Nephrol (2020) 21:506. doi: 10.1186/s12882-020-02151-7
6. Banerjee D, Winocour P, Chowdhury TA, De P, Wahba M, Montero R, et al. Management of hypertension and renin-angiotensin-aldosterone system blockade in adults with diabetic kidney disease, association of British clinical diabetologists and the renal association UK guideline update 2021. BMC Nephrol (2022) 23:9. doi: 10.1186/s12882-021-02587-5
7. Sridhar VS, Yau K, Benham JL, Campbell DJT, Cherney DZI. Sex and gender related differences in diabetic kidney disease. Semin Nephrol (2022) 42:170–84. doi: 10.1016/j.semnephrol.2022.04.007
8. Payne AH, Hales DB. Overview of steroidogenic enzymes in the pathway from cholesterol to active steroid hormones. Endocr Rev (2004) 25:947–70. doi: 10.1210/er.2003-0030
9. Papadopoulos V, Baraldi M, Guilarte TR, Knudsen TB, Lacapere JJ, Lindemann P, et al. Translocator protein (18kDa), new nomenclature for the peripheral-type benzodiazepine receptor based on its structure and molecular function. Trends Pharmacol Sci (2006) 27:402–9. doi: 10.1016/j.tips.2006.06.005
10. Tsuchiya Y, Nakajima M, Yokoi T. Cytochrome P450-mediated metabolism of estrogens and its regulation in human. Cancer Lett (2005) 227:115–24. doi: 10.1016/j.canlet.2004.10.007
11. Schmidtova E. [Testosterone–effects, metabolism and genetic determination]. Cesk Fysiol (2008) 57:61–75.
12. Rana K, Davey RA, Zajac JD. Human androgen deficiency, insights gained from androgen receptor knockout mouse models. Asian J Androl (2014) 16:169–77. doi: 10.4103/1008-682X.122590
13. Kelly DM, Jones TH. Testosterone, a metabolic hormone in health and disease. J Endocrinol (2013) 217:R25–45. doi: 10.1530/JOE-12-0455
14. Davey RA, Grossmann M. Androgen receptor structure, function and biology, from bench to bedside. Clin Biochem Rev (2016) 37:3–15.
15. Sankar JS, Hampson E. Androgen receptor polymorphism, mental rotation, and spatial visualization in men. Psychoneuroendocrinology (2021) 129:105239. doi: 10.1016/j.psyneuen.2021.105239
16. Kitajima Y, Ono Y. Estrogens maintain skeletal muscle and satellite cell functions. J Endocrinol (2016) 229:267–75. doi: 10.1530/JOE-15-0476
17. Arnal JF, Lenfant F, Metivier R, Flouriot G, Henrion D, Adlanmerini M, et al. Membrane and nuclear estrogen receptor alpha actions, from tissue specificity to medical implications. Physiol Rev (2017) 97:1045–87. doi: 10.1152/physrev.00024.2016
18. Wilkinson HN, Hardman MJ. The role of estrogen in cutaneous ageing and repair. Maturitas (2017) 103:60–4. doi: 10.1016/j.maturitas.2017.06.026
19. Trenti A, Tedesco S, Boscaro C, Trevisi L, Bolego C, Cignarella A. Estrogen, angiogenesis, immunity and cell metabolism, solving the puzzle. Int J Mol Sci (2018) 15:19. doi: 10.3390/ijms19030859
20. El-Gendy AA, Elsaed WM, Abdallah HI. Potential role of estradiol in ovariectomy-induced derangement of renal endocrine functions. Ren Fail (2019) 41:507–20. doi: 10.1080/0886022X.2019.1625787
21. Vrtacnik P, Ostanek B, Mencej-Bedrac S, Marc J. The many faces of estrogen signaling. Biochem Med (Zagreb) (2014) 24:329–42. doi: 10.11613/BM.2014.035
22. Hara Y, Waters EM, McEwen BS, Morrison JH. Estrogen effects on cognitive and synaptic health over the lifecourse. Physiol Rev (2015) 95:785–807. doi: 10.1152/physrev.00036.2014
23. Kovats S. Estrogen receptors regulate innate immune cells and signaling pathways. Cell Immunol (2015) 294:63–9. doi: 10.1016/j.cellimm.2015.01.018
24. Arao Y, Korach KS. The physiological role of estrogen receptor functional domains. Essays Biochem (2021) 65:867–75. doi: 10.1042/EBC20200167
25. Landgren BM, Collins A, Csemiczky G, Burger HG, Baksheev L, Robertson DM. Menopause transition, annual changes in serum hormonal patterns over the menstrual cycle in women during a nine-year period prior to menopause. J Clin Endocrinol Metab (2004) 89:2763–9. doi: 10.1210/jc.2003-030824
26. Horstman AM, Dillon EL, Urban RJ, Sheffield-Moore M. The role of androgens and estrogens on healthy aging and longevity. J Gerontol A Biol Sci Med Sci (2012) 67:1140–52. doi: 10.1093/gerona/gls068
27. Yasui T, Matsui S, Tani A, Kunimi K, Yamamoto S, Irahara M. Androgen in postmenopausal women. J Med Invest (2012) 59:12–27. doi: 10.2152/jmi.59.12
28. Ketchem JM, Bowman EJ, Isales CM. Male Sex hormones, aging, and inflammation. Biogerontology (2023) 24:1–25. doi: 10.1007/s10522-022-10002-1
29. Gambineri A, Pelusi C. Sex hormones, obesity and type 2 diabetes, is there a link? Endocr Connect (2019) 8:R1–9. doi: 10.1530/EC-18-0450
30. Maric C. Sex, diabetes and the kidney. Am J Physiol Renal Physiol (2009) 296:F680–688. doi: 10.1152/ajprenal.90505.2008
31. Nilsson S, Makela S, Treuter E, Tujague M, Thomsen J, Andersson G, et al. Mechanisms of estrogen action. Physiol Rev (2001) 81:1535–65. doi: 10.1152/physrev.2001.81.4.1535
32. Salonia A, Lanzi R, Scavini M, Pontillo M, Gatti E, Petrella G, et al. Sexual function and endocrine profile in fertile women with type 1 diabetes. Diabetes Care (2006) 29:312–6. doi: 10.2337/diacare.29.02.06.dc05-1067
33. Grossmann M, Thomas MC, Panagiotopoulos S, Sharpe K, Macisaac RJ, Clarke S, et al. Low testosterone levels are common and associated with insulin resistance in men with diabetes. J Clin Endocrinol Metab (2008) 93:1834–40. doi: 10.1210/jc.2007-2177
34. Vikan T, Schirmer H, Njolstad I, Svartberg J. Low testosterone and sex hormone-binding globulin levels and high estradiol levels are independent predictors of type 2 diabetes in men. Eur J Endocrinol (2010) 162:747–54. doi: 10.1530/EJE-09-0943
35. Manigrasso MB, Sawyer RT, Hutchens ZM Jr., Flynn ER, Maric-Bilkan C. Combined inhibition of aromatase activity and dihydrotestosterone supplementation attenuates renal injury in male streptozotocin (STZ)-induced diabetic rats. Am J Physiol Renal Physiol (2012) 302:F1203–1209. doi: 10.1152/ajprenal.00569.2011
36. Dhindsa S, Reddy A, Karam JS, Bilkis S, Chaurasia A, Mehta A, et al. Prevalence of subnormal testosterone concentrations in men with type 2 diabetes and chronic kidney disease. Eur J Endocrinol (2015) 173:359–66. doi: 10.1530/EJE-15-0359
37. Hackett G, Heald AH, Sinclair A, Jones PW, Strange RC, Ramachandran S. Serum testosterone, testosterone replacement therapy and all-cause mortality in men with type 2 diabetes, retrospective consideration of the impact of PDE5 inhibitors and statins. Int J Clin Pract (2016) 70:244–53. doi: 10.1111/ijcp.12779
38. Mankhey RW, Bhatti F, Maric C. 17β-estradiol replacement improves renal function and pathology associated with diabetic nephropathy. Am J Physiology-Renal Physiol (2005) 288:F399–405. doi: 10.1152/ajprenal.00195.2004
39. Schoeller EL, Schon S, Moley KH. The effects of type 1 diabetes on the hypothalamic, pituitary and testes axis. Cell Tissue Res (2012) 349:839–47. doi: 10.1007/s00441-012-1387-7
40. Bruning JC, Gautam D, Burks DJ, Gillette J, Schubert M, Orban PC, et al. Role of brain insulin receptor in control of body weight and reproduction. Science (2000) 289:2122–5. doi: 10.1126/science.289.5487.2122
41. Saito K, Cui H. Emerging roles of estrogen-related receptors in the brain, potential interactions with estrogen signaling. Int J Mol Sci (2018) 19:1091. doi: 10.3390/ijms19041091
42. Amory JK, Bremner WJ. Regulation of testicular function in men, implications for male hormonal contraceptive development. J Steroid Biochem Mol Biol (2003) 85:357–61. doi: 10.1016/S0960-0760(03)00205-X
43. Maneesh M, Jayalakshmi H, Singh TA, Chakrabarti A. Impaired hypothalamic-pituitary-gonadal axis function in men with diabetes mellitus. Indian J Clin Biochem (2006) 21:165–8. doi: 10.1007/BF02913088
44. Rochira V, Zirilli L, Genazzani AD, Balestrieri A, Aranda C, Fabre B, et al. Hypothalamic-pituitary-gonadal axis in two men with aromatase deficiency, evidence that circulating estrogens are required at the hypothalamic level for the integrity of gonadotropin negative feedback. Eur J Endocrinol (2006) 155:513–22. doi: 10.1530/eje.1.02254
45. Costanzo PR, Suarez SM, Scaglia HE, Zylbersztein C, Litwak LE, Knoblovits P. Evaluation of the hypothalamic-pituitary-gonadal axis in eugonadal men with type 2 diabetes mellitus. Andrology (2014) 2:117–24. doi: 10.1111/j.2047-2927.2013.00163.x
46. Cheung KK, Luk AO, So WY, Ma RC, Kong AP, Chow FC, et al. Testosterone level in men with type 2 diabetes mellitus and related metabolic effects, a review of current evidence. J Diabetes Investig (2015) 6:112–23. doi: 10.1111/jdi.12288
47. Schianca GP, Fra GP, Brustia F, Bellan M, Pirovano A, Gualerzi A, et al. Testosterone plasma concentration is associated with insulin resistance in Male hypertensive patients. Exp Clin Endocrinol Diabetes (2017) 125:171–5. doi: 10.1055/s-0042-121492
48. Burul-Bozkurt N, Pekiner C, Kelicen P. Diabetes alters aromatase enzyme levels in gonadal tissues of rats. Naunyn Schmiedebergs Arch Pharmacol (2010) 382:33–41. doi: 10.1007/s00210-010-0518-5
49. Burul-Bozkurt N, Pekiner C, Kelicen P. Diabetes alters aromatase enzyme levels in sciatic nerve and hippocampus tissues of rats. Cell Mol Neurobiol (2010) 30:445–51. doi: 10.1007/s10571-009-9469-0
50. Heppner KM, Baquero AF, Bennett CM, Lindsley SR, Kirigiti MA, Bennett B, et al. GLP-1R signaling directly activates arcuate nucleus kisspeptin action in brain slices but does not rescue luteinizing hormone inhibition in ovariectomized mice during negative energy balance. eNeuro (2017) 4(1):ENEURO.0198-16.2016. doi: 10.1523/ENEURO.0198-16.2016
51. Quennell JH, Mulligan AC, Tups A, Liu X, Phipps SJ, Kemp CJ, et al. Leptin indirectly regulates gonadotropin-releasing hormone neuronal function. Endocrinology (2009) 150:2805–12. doi: 10.1210/en.2008-1693
52. German JP, Wisse BE, Thaler JP, Oh IS, Sarruf DA, Ogimoto K, et al. Leptin deficiency causes insulin resistance induced by uncontrolled diabetes. Diabetes (2010) 59:1626–34. doi: 10.2337/db09-1918
53. Wabitsch M, Jensen PB, Blum WF, Christoffersen CT, Englaro P, Heinze E, et al. Insulin and cortisol promote leptin production in cultured human fat cells. Diabetes (1996) 45:1435–8. doi: 10.2337/diab.45.10.1435
54. Barr VA, Malide D, Zarnowski MJ, Taylor SI, Cushman SW. Insulin stimulates both leptin secretion and production by rat white adipose tissue. Endocrinology (1997) 138:4463–72. doi: 10.1210/endo.138.10.5451
55. Carvalheira JB, Torsoni MA, Ueno M, Amaral ME, Araujo EP, Velloso LA, et al. Cross-talk between the insulin and leptin signaling systems in rat hypothalamus. Obes Res (2005) 13:48–57. doi: 10.1038/oby.2005.7
56. Childs GV, Odle AK, MacNicol MC, MacNicol AM. The importance of leptin to reproduction. Endocrinology (2021) 162:bqaa204. doi: 10.1210/endocr/bqaa204
57. Jones ME, Thorburn AW, Britt KL, Hewitt KN, Wreford NG, Proietto J, et al. Aromatase-deficient (ArKO) mice have a phenotype of increased adiposity. Proc Natl Acad Sci U.S.A. (2000) 97:12735–40. doi: 10.1073/pnas.97.23.12735
58. Takeda K, Toda K, Saibara T, Nakagawa M, Saika K, Onishi T, et al. Progressive development of insulin resistance phenotype in male mice with complete aromatase (CYP19) deficiency. J Endocrinol (2003) 176:237–46. doi: 10.1677/joe.0.1760237
59. Zhang XX, Kong J, Yun K. Prevalence of diabetic nephropathy among patients with type 2 diabetes mellitus in China, a meta-analysis of observational studies. J Diabetes Res (2020) 2020:2315607. doi: 10.1155/2020/2315607
60. Ziller N, Kotolloshi R, Esmaeili M, Liebisch M, Mrowka R, Baniahmad A, et al. Sex differences in diabetes- and TGF-beta1-Induced renal damage. Cells (2020) 9:2236. doi: 10.3390/cells9102236
61. Chesnaye NC, Dekker FW, Evans M, Caskey FJ, Torino C, Postorino M, et al. Renal function decline in older men and women with advanced chronic kidney disease-results from the EQUAL study. Nephrol Dial Transplant (2021) 36:1656–63. doi: 10.1093/ndt/gfaa095
62. Orchard TJ, Dorman JS, Maser RE, Becker DJ, Drash AL, Ellis D, et al. Prevalence of complications in IDDM by sex and duration. Pittsburgh epidemiology of diabetes complications study II. Diabetes (1990) 39:1116–24. doi: 10.2337/diab.39.9.1116
63. Raile K, Galler A, Hofer S, Herbst A, Dunstheimer D, Busch P, et al. Diabetic nephropathy in 27,805 children, adolescents, and adults with type 1 diabetes, effect of diabetes duration, A1C, hypertension, dyslipidemia, diabetes onset, and sex. Diabetes Care (2007) 30:2523–8. doi: 10.2337/dc07-0282
64. Maric C, Sullivan S. Estrogens and the diabetic kidney. Gend Med (2008) 5 Suppl A:S103–113. doi: 10.1016/j.genm.2008.03.010
65. Dong W, Zhao Y, Liu D, Liu Y, Li F, Li M. Sex-specific association between type 1 diabetes and the risk of end-stage renal disease, a systematic review and meta-analysis. Endocrine (2020) 69:30–8. doi: 10.1007/s12020-020-02255-y
66. Neugarten J, Acharya A, Silbiger SR. Effect of gender on the progression of nondiabetic renal disease, a meta-analysis. J Am Soc Nephrol (2000) 11:319–29. doi: 10.1681/ASN.V112319
67. Shen Y, Cai R, Sun J, Dong X, Huang R, Tian S, et al. Diabetes mellitus as a risk factor for incident chronic kidney disease and end-stage renal disease in women compared with men, a systematic review and meta-analysis. Endocrine (2017) 55:66–76. doi: 10.1007/s12020-016-1014-6
68. Yu MK, Lyles CR, Bent-Shaw LA, Young BA, Pathways A. Risk factor, age and sex differences in chronic kidney disease prevalence in a diabetic cohort, the pathways study. Am J Nephrol (2012) 36:245–51. doi: 10.1159/000342210
69. Lewis EJ, Hunsicker LG, Rodby RA, Collaborative Study G. A clinical trial in type 2 diabetic nephropathy. Am J Kidney Dis (2001) 38:S191–194. doi: 10.1053/ajkd.2001.27442
70. Keane WF, Brenner BM, de Zeeuw D, Grunfeld JP, McGill J, Mitch WE, et al. The risk of developing end-stage renal disease in patients with type 2 diabetes and nephropathy, the RENAAL study. Kidney Int (2003) 63:1499–507. doi: 10.1046/j.1523-1755.2003.00885.x
71. Mollsten A, Svensson M, Waernbaum I, Berhan Y, Schon S, Nystrom L, et al. Cumulative risk, age at onset, and sex-specific differences for developing end-stage renal disease in young patients with type 1 diabetes, a nationwide population-based cohort study. Diabetes (2010) 59:1803–8. doi: 10.2337/db09-1744
72. Harjutsalo V, Maric C, Forsblom C, Thorn L, Waden J, Groop PH, et al. Sex-related differences in the long-term risk of microvascular complications by age at onset of type 1 diabetes. Diabetologia (2011) 54:1992–9. doi: 10.1007/s00125-011-2144-2
73. Kang AK, Miller JA. Effects of gender on the renin-angiotensin system, blood pressure, and renal function. Curr Hypertens Rep (2002) 4:143–51. doi: 10.1007/s11906-002-0039-9
74. Anderson S, Chapman JG, Oyama TT, Komers R. Effect of orchiectomy on renal function in control and diabetic rats with chronic inhibition of nitric oxide. Clin Exp Pharmacol Physiol (2010) 37:19–23. doi: 10.1111/j.1440-1681.2009.05206.x
75. Skupien J, Smiles AM, Valo E, Ahluwalia TS, Gyorgy B, Sandholm N, et al. Variations in risk of end-stage renal disease and risk of mortality in an international study of patients with type 1 diabetes and advanced nephropathy. Diabetes Care (2019) 42:93–101. doi: 10.2337/dc18-1369
76. Wells CC, Riazi S, Mankhey RW, Bhatti F, Ecelbarger C, Maric C. Diabetic nephropathy is associated with decreased circulating estradiol levels and imbalance in the expression of renal estrogen receptors. Gend Med (2005) 2:227–37. doi: 10.1016/S1550-8579(05)80052-X
77. Ma HY, Chen S, Du Y. Estrogen and estrogen receptors in kidney diseases. Ren Fail (2021) 43:619–42. doi: 10.1080/0886022X.2021.1901739
78. Manigrasso MB, Sawyer RT, Marbury DC, Flynn ER, Maric C. Inhibition of estradiol synthesis attenuates renal injury in male streptozotocin-induced diabetic rats. Am J Physiol Renal Physiol (2011) 301:F634–640. doi: 10.1152/ajprenal.00718.2010
79. Kwan G, Neugarten J, Sherman M, Ding Q, Fotadar U, Lei J, et al. Effects of sex hormones on mesangial cell proliferation and collagen synthesis. Kidney Int (1996) 50:1173–9. doi: 10.1038/ki.1996.425
80. Dixon A, Maric C. 17beta-estradiol attenuates diabetic kidney disease by regulating extracellular matrix and transforming growth factor-beta protein expression and signaling. Am J Physiol Renal Physiol (2007) 293:F1678–1690. doi: 10.1152/ajprenal.00079.2007
81. Kummer S, Jeruschke S, Wegerich LV, Peters A, Lehmann P, Seibt A, et al. Estrogen receptor alpha expression in podocytes mediates protection against apoptosis in-vitro and in-vivo. PLoS One (2011) 6:e27457. doi: 10.1371/journal.pone.0027457
82. Appiah D, Winters SJ, Hornung CA. Bilateral oophorectomy and the risk of incident diabetes in postmenopausal women. Diabetes Care (2014) 37:725–33. doi: 10.2337/dc13-1986
83. Appiah D, Winters SJ, Allison MA, Baumgartner RN, Groves FD, Myers JA, et al. Cardiovascular disease among women with and without diabetes mellitus and bilateral oophorectomy. Diabetes Res Clin Pract (2015) 108:473–81. doi: 10.1016/j.diabres.2015.02.017
84. Honigberg MC, Zekavat SM, Aragam K, Finneran P, Klarin D, Bhatt DL, et al. Association of premature natural and surgical menopause with incident cardiovascular disease. JAMA (2019) 322:2411–21. doi: 10.1001/jama.2019.19191
85. Ravid M, Brosh D, Ravid-Safran D, Levy Z, Rachmani R. Main risk factors for nephropathy in type 2 diabetes mellitus are plasma cholesterol levels, mean blood pressure, and hyperglycemia. Arch Intern Med (1998) 158:998–1004. doi: 10.1001/archinte.158.9.998
86. Caramori ML, Kim Y, Huang C, Fish AJ, Rich SS, Miller ME, et al. Cellular basis of diabetic nephropathy, 1. study design and renal structural-functional relationships in patients with long-standing type 1 diabetes. Diabetes (2002) 51:506–13. doi: 10.2337/diabetes.51.2.506
87. Chen YM, Miner JH. Glomerular basement membrane and related glomerular disease. Transl Res (2012) 160:291–7. doi: 10.1016/j.trsl.2012.03.004
88. Herman-Edelstein M, Doi SQ. Pathophysiology of diabetic nephropathy. In: Proteinuria, basic mechanisms, pathophysiology and clinical relevance (Switzerland: Springer International Publishing Switzerland) (2016). p. 41–65.
89. Jha JC, Banal C, Chow BS, Cooper ME, Jandeleit-Dahm K. Diabetes and kidney disease, role of oxidative stress. Antioxid Redox Signal (2016) 25:657–84. doi: 10.1089/ars.2016.6664
90. Singh DK, Winocour P, Farrington K. Oxidative stress in early diabetic nephropathy, fueling the fire. Nat Rev Endocrinol (2011) 7:176–84. doi: 10.1038/nrendo.2010.212
91. Forbes JM, Thorburn DR. Mitochondrial dysfunction in diabetic kidney disease. Nat Rev Nephrol (2018) 14:291–312. doi: 10.1038/nrneph.2018.9
92. Wei PZ, Szeto CC. Mitochondrial dysfunction in diabetic kidney disease. Clin Chim Acta (2019) 496:108–16. doi: 10.1016/j.cca.2019.07.005
93. Reed DK, Arany I. Sex hormones differentially modulate STAT3-dependent antioxidant responses during oxidative stress in renal proximal tubule cells. In Vivo (2014) 28:1097–100.
94. Ulas M, Cay M. 17beta-estradiol and vitamin e modulates oxidative stress-induced kidney toxicity in diabetic ovariectomized rat. Biol Trace Elem Res (2011) 144:821–31. doi: 10.1007/s12011-011-9025-x
95. Ji H, Zheng W, Menini S, Pesce C, Kim J, Wu X, et al. Female protection in progressive renal disease is associated with estradiol attenuation of superoxide production. Gend Med (2007) 4:56–71. doi: 10.1016/S1550-8579(07)80009-X
96. Ronis MJ, Blackburn ML, Shankar K, Ferguson M, Cleves MA, Badger TM. Estradiol and NADPH oxidase crosstalk regulates responses to high fat feeding in female mice. Exp Biol Med (Maywood) (2019) 244:834–45. doi: 10.1177/1535370219853563
97. Li JH, Wang W, Huang XR, Oldfield M, Schmidt AM, Cooper ME, et al. Advanced glycation end products induce tubular epithelial-myofibroblast transition through the RAGE-ERK1/2 MAP kinase signaling pathway. Am J Pathol (2004) 164:1389–97. doi: 10.1016/S0002-9440(10)63225-7
98. Tan AL, Forbes JM, Cooper ME. AGE, RAGE, and ROS in diabetic nephropathy. Semin Nephrol (2007) 27:130–43. doi: 10.1016/j.semnephrol.2007.01.006
99. Navarro-Gonzalez JF, Mora-Fernandez C, Muros de Fuentes M, Garcia-Perez J. Inflammatory molecules and pathways in the pathogenesis of diabetic nephropathy. Nat Rev Nephrol (2011) 7:327–40. doi: 10.1038/nrneph.2011.51
100. Xu Y, Nie L, Yin YG, Tang JL, Zhou JY, Li DD, et al. Resveratrol protects against hyperglycemia-induced oxidative damage to mitochondria by activating SIRT1 in rat mesangial cells. Toxicol Appl Pharmacol (2012) 259:395–401. doi: 10.1016/j.taap.2011.09.028
101. Ruiz-Ortega M, Rayego-Mateos S, Lamas S, Ortiz A, Rodrigues-Diez RR. Targeting the progression of chronic kidney disease. Nat Rev Nephrol (2020) 16:269–88. doi: 10.1038/s41581-019-0248-y
102. Ivanov II, McKenzie BS, Zhou L, Tadokoro CE, Lepelley A, Lafaille JJ, et al. The orphan nuclear receptor RORgammat directs the differentiation program of proinflammatory IL-17+ T helper cells. Cell (2006) 126:1121–33. doi: 10.1016/j.cell.2006.07.035
103. Eller K, Kirsch A, Wolf AM, Sopper S, Tagwerker A, Stanzl U, et al. Potential role of regulatory T cells in reversing obesity-linked insulin resistance and diabetic nephropathy. Diabetes (2011) 60:2954–62. doi: 10.2337/db11-0358
104. Liu G, Ma H, Qiu L, Li L, Cao Y, Ma J, et al. Phenotypic and functional switch of macrophages induced by regulatory CD4+CD25+ T cells in mice. Immunol Cell Biol (2011) 89:130–42. doi: 10.1038/icb.2010.70
105. Moon JY, Jeong KH, Lee TW, Ihm CG, Lim SJ, Lee SH. Aberrant recruitment and activation of T cells in diabetic nephropathy. Am J Nephrol (2012) 35:164–74. doi: 10.1159/000334928
106. Duran-Salgado MB, Rubio-Guerra AF. Diabetic nephropathy and inflammation. World J Diabetes (2014) 5:393–8. doi: 10.4239/wjd.v5.i3.393
107. Lei L, Mao Y, Meng D, Zhang X, Cui L, Huo Y, et al. Percentage of circulating CD8+ T lymphocytes is associated with albuminuria in type 2 diabetes mellitus. Exp Clin Endocrinol Diabetes (2014) 122:27–30. doi: 10.1055/s-0033-1358666
108. Zhang C, Xiao C, Wang P, Xu W, Zhang A, Li Q, et al. The alteration of Th1/Th2/Th17/Treg paradigm in patients with type 2 diabetes mellitus, relationship with diabetic nephropathy. Hum Immunol (2014) 75:289–96. doi: 10.1016/j.humimm.2014.02.007
109. Navarro-Gonzalez JF, Mora-Fernandez C. The role of inflammatory cytokines in diabetic nephropathy. J Am Soc Nephrol (2008) 19:433–42. doi: 10.1681/ASN.2007091048
110. Smith MJ, Simmons KM, Cambier JC. B cells in type 1 diabetes mellitus and diabetic kidney disease. Nat Rev Nephrol (2017) 13:712–20. doi: 10.1038/nrneph.2017.138
111. Albvr VR, Tan SH, Candasamy M, Bhattamisra SK. Diabetic nephropathy, an update on pathogenesis and drug development. Diabetes Metab Syndr (2019) 13:754–62. doi: 10.1016/j.dsx.2018.11.054
112. Derijard B, Hibi M, Wu IH, Barrett T, Su B, Deng T, et al. JNK1, a protein kinase stimulated by UV light and ha-ras that binds and phosphorylates the c-jun activation domain. Cell (1994) 76:1025–37. doi: 10.1016/0092-8674(94)90380-8
113. Sabapathy K, Wagner EF. JNK2, a negative regulator of cellular proliferation. Cell Cycle (2004) 3:1520–3. doi: 10.4161/cc.3.12.1315
114. De Borst MH, Prakash J, Melenhorst WB, van den Heuvel MC, Kok RJ, Navis G, et al. Glomerular and tubular induction of the transcription factor c-jun in human renal disease. J Pathol (2007) 213:219–28. doi: 10.1002/path.2228
115. Verzola D, Villaggio B, Procopio V, Gandolfo MT, Gianiorio F, Fama A, et al. Androgen-mediated apoptosis of kidney tubule cells, role of c-jun amino terminal kinase. Biochem Biophys Res Commun (2009) 387:531–6. doi: 10.1016/j.bbrc.2009.07.056
116. Metcalfe PD, Leslie JA, Campbell MT, Meldrum DR, Hile KL, Meldrum KK. Testosterone exacerbates obstructive renal injury by stimulating TNF-alpha production and increasing proapoptotic and profibrotic signaling. Am J Physiol Endocrinol Metab (2008) 294:E435–443. doi: 10.1152/ajpendo.00704.2006
117. Carew RM, Wang B, Kantharidis P. The role of EMT in renal fibrosis. Cell Tissue Res (2012) 347:103–16. doi: 10.1007/s00441-011-1227-1
118. Sun YB, Qu X, Caruana G, Li J. The origin of renal fibroblasts/myofibroblasts and the signals that trigger fibrosis. Differentiation (2016) 92:102–7. doi: 10.1016/j.diff.2016.05.008
119. Doublier S, Lupia E, Catanuto P, Periera-Simon S, Xia X, Korach K, et al. Testosterone and 17beta-estradiol have opposite effects on podocyte apoptosis that precedes glomerulosclerosis in female estrogen receptor knockout mice. Kidney Int (2011) 79:404–13. doi: 10.1038/ki.2010.398
120. Zdunek M, Silbiger S, Lei J, Neugarten J. Protein kinase CK2 mediates TGF-β1-stimulated type IV collagen gene transcription and its reversal by estradiol1. Kidney Int (2001) 60:2097–108. doi: 10.1046/j.1523-1755.2001.00041.x
121. Sullivan JC, Semprun-Prieto L, Boesen EI, Pollock DM, Pollock JS. Sex and sex hormones influence the development of albuminuria and renal macrophage infiltration in spontaneously hypertensive rats. Am J Physiology-Regulatory Integr Comp Physiol (2007) 293:R1573–9. doi: 10.1152/ajpregu.00429.2007
122. Sugahara M, Pak WLW, Tanaka T, Tang SCW, Nangaku M. Update on diagnosis, pathophysiology, and management of diabetic kidney disease. Nephrol (Carlton) (2021) 26:491–500. doi: 10.1111/nep.13860
123. Tuttle KR. Back to the future, glomerular hyperfiltration and the diabetic kidney. Diabetes (2016) 66:14–6. doi: 10.2337/dbi16-0056
124. Harvey JN. The influence of sex and puberty on the progression of diabetic nephropathy and retinopathy. Diabetologia (2011) 54:1943–5. doi: 10.1007/s00125-011-2185-6
125. Miller JA, Anacta LA, Cattran DC. Impact of gender on the renal response to angiotensin II. Kidney Int (1999) 55:278–85. doi: 10.1046/j.1523-1755.1999.00260.x
126. Labandeira-Garcia JL, Rodriguez-Pallares J, Villar-Cheda B, Rodriguez-Perez AI, Garrido-Gil P, Guerra MJ. Aging, angiotensin system and dopaminergic degeneration in the substantia nigra. Aging Dis (2011) 2:257–74.
127. Sparks MA, Crowley SD, Gurley SB, Mirotsou M, Coffman TM. Classical renin-angiotensin system in kidney physiology. Compr Physiol (2014) 4:1201–28. doi: 10.1002/cphy.c130040
128. Rianto F, Hoang T, Revoori R, Sparks MA. Angiotensin receptors in the kidney and vasculature in hypertension and kidney disease. Mol Cell Endocrinol (2021) 529:111259. doi: 10.1016/j.mce.2021.111259
129. Clotet S, Soler MJ, Rebull M, Gimeno J, Gurley SB, Pascual J, et al. Gonadectomy prevents the increase in blood pressure and glomerular injury in angiotensin-converting enzyme 2 knockout diabetic male mice. Effects renin-angiotensin system J Hypertens (2016) 34:1752–65. doi: 10.1097/HJH.0000000000001015
130. Clotet-Freixas S, Soler MJ, Palau V, Anguiano L, Gimeno J, Konvalinka A, et al. Sex dimorphism in ANGII-mediated crosstalk between ACE2 and ACE in diabetic nephropathy. Lab Invest (2018) 98:1237–49. doi: 10.1038/s41374-018-0084-x
131. Oudit GY, Herzenberg AM, Kassiri Z, Wong D, Reich H, Khokha R, et al. Loss of angiotensin-converting enzyme-2 leads to the late development of angiotensin II-dependent glomerulosclerosis. Am J Pathol (2006) 168:1808–20. doi: 10.2353/ajpath.2006.051091
132. Cai Q, Keck M, McReynolds MR, Klein JD, Greer K, Sharma K, et al. Effects of water restriction on gene expression in mouse renal medulla, identification of 3βHSD4 as a collecting duct protein. Am J Physiology-Renal Physiol (2006) 291:F218–24. doi: 10.1152/ajprenal.00413.2005
133. Xue B, Pamidimukkala J, Lubahn DB, Hay M. Estrogen receptor-alpha mediates estrogen protection from angiotensin II-induced hypertension in conscious female mice. Am J Physiol Heart Circ Physiol (2007) 292:H1770–1776. doi: 10.1152/ajpheart.01011.2005
134. Pingili AK, Davidge KN, Thirunavukkarasu S, Khan NS, Katsurada A, Majid DSA, et al. 2-methoxyestradiol reduces angiotensin II-induced hypertension and renal dysfunction in ovariectomized female and intact Male mice. Hypertension (2017) 69:1104–12. doi: 10.1161/HYPERTENSIONAHA.117.09175
135. Ogola BO, Zimmerman MA, Sure VN, Gentry KM, Duong JL, Clark GL, et al. G Protein-coupled estrogen receptor protects from angiotensin II-induced increases in pulse pressure and oxidative stress. Front Endocrinol (Lausanne) (2019) 10:586. doi: 10.3389/fendo.2019.00586
136. Albrecht EW, Stegeman CA, Heeringa P, Henning RH, van Goor H. Protective role of endothelial nitric oxide synthase. J Pathol (2003) 199:8–17. doi: 10.1002/path.1250
137. Takahashi T, Harris RC. Role of endothelial nitric oxide synthase in diabetic nephropathy, lessons from diabetic eNOS knockout mice. J Diabetes Res (2014) 2014:590541. doi: 10.1155/2014/590541
138. Dantas AP, Tostes RC, Fortes ZB, Costa SG, Nigro D, Carvalho MH. In vivo evidence for antioxidant potential of estrogen in microvessels of female spontaneously hypertensive rats. Hypertension (2002) 39:405–11. doi: 10.1161/hy0202.102993
139. Tostes RC, Nigro D, Fortes ZB, Carvalho MH. Effects of estrogen on the vascular system. Braz J Med Biol Res (2003) 36:1143–58. doi: 10.1590/S0100-879X2003000900002
140. Chang Y, Han Z, Zhang Y, Zhou Y, Feng Z, Chen L, et al. G Protein-coupled estrogen receptor activation improves contractile and diastolic functions in rat renal interlobular artery to protect against renal ischemia reperfusion injury. BioMed Pharmacother (2019) 112:108666. doi: 10.1016/j.biopha.2019.108666
141. Giandalia A, Giuffrida AE, Gembillo G, Cucinotta D, Squadrito G, Santoro D, et al. Gender differences in diabetic kidney disease, focus on hormonal, genetic and clinical factors. Int J Mol Sci (2021) 22:5808. doi: 10.3390/ijms22115808
142. Neugarten J, Golestaneh L. Gender and the prevalence and progression of renal disease. Adv Chronic Kidney Dis (2013) 20:390–5. doi: 10.1053/j.ackd.2013.05.004
143. Rabbani N, Thornalley PJ. Advanced glycation end products in the pathogenesis of chronic kidney disease. Kidney Int (2018) 93:803–13. doi: 10.1016/j.kint.2017.11.034
144. Herman-Edelstein M, Scherzer P, Tobar A, Levi M, Gafter U. Altered renal lipid metabolism and renal lipid accumulation in human diabetic nephropathy. J Lipid Res (2014) 55:561–72. doi: 10.1194/jlr.P040501
145. Opazo-Rios L, Mas S, Marin-Royo G, Mezzano S, Gomez-Guerrero C, Moreno JA, et al. Lipotoxicity and diabetic nephropathy, novel mechanistic insights and therapeutic opportunities. Int J Mol Sci (2020) 21:2632. doi: 10.3390/ijms21072632
146. Tawfik SH, Mahmoud BF, Saad MI, Shehata M, Kamel MA, Helmy MH. Similar and additive effects of ovariectomy and diabetes on insulin resistance and lipid metabolism. Biochem Res Int (2015) 2015:567945. doi: 10.1155/2015/567945
147. Szekacs B, Vajo Z, Varbiro S, Kakucs R, Vaslaki L, Acs N, et al. Postmenopausal hormone replacement improves proteinuria and impaired creatinine clearance in type 2 diabetes mellitus and hypertension. BJOG (2000) 107:1017–21. doi: 10.1111/j.1471-0528.2000.tb10406.x
148. Andersson B, Johannsson G, Holm G, Bengtsson BA, Sashegyi A, Pavo I, et al. Raloxifene does not affect insulin sensitivity or glycemic control in postmenopausal women with type 2 diabetes mellitus, a randomized clinical trial. J Clin Endocrinol Metab (2002) 87:122–8. doi: 10.1210/jcem.87.1.8168
149. Dixon A, Wells CC, Singh S, Babayan R, Maric C. Renoprotective effects of a selective estrogen receptor modulator, raloxifene, in an animal model of diabetic nephropathy. Am J Nephrol (2007) 27:120–8. doi: 10.1159/000099837
150. Hadjadj S, Gourdy P, Zaoui P, Guerci B, Roudaut N, Gautier JF, et al. Effect of raloxifene – a selective oestrogen receptor modulator – on kidney function in post-menopausal women with type 2 diabetes, results from a randomized, placebo-controlled pilot trial. Diabetes Med (2007) 24:906–10. doi: 10.1111/j.1464-5491.2007.02165.x
151. Ishani A, Blackwell T, Jamal SA, Cummings SR, Ensrud KE, Investigators M. The effect of raloxifene treatment in postmenopausal women with CKD. J Am Soc Nephrol (2008) 19:1430–8. doi: 10.1681/ASN.2007050555
152. Al-Trad B, Ashankyty IM, Alaraj M. Progesterone ameliorates diabetic nephropathy in streptozotocin-induced diabetic rats. Diabetol Metab Syndr (2015) 7:97. doi: 10.1186/s13098-015-0097-1
153. Eliassen AH, Missmer SA, Tworoger SS, Spiegelman D, Barbieri RL, Dowsett M, et al. Endogenous steroid hormone concentrations and risk of breast cancer among premenopausal women. J Natl Cancer Inst (2006) 98:1406–15. doi: 10.1093/jnci/djj376
154. Xu Q, Prabhu A, Xu S, Manigrasso MB, Maric C. Dose-dependent effects of dihydrotestosterone in the streptozotocin-induced diabetic rat kidney. Am J Physiol Renal Physiol (2009) 297:F307–315. doi: 10.1152/ajprenal.00135.2009
155. Qi MY, Kai C, Liu HR, Su YH, Yu SQ. Protective effect of icariin on the early stage of experimental diabetic nephropathy induced by streptozotocin via modulating transforming growth factor beta1 and type IV collagen expression in rats. J Ethnopharmacol (2011) 138:731–6. doi: 10.1016/j.jep.2011.10.015
156. Chin M, Isono M, Isshiki K, Araki S, Sugimoto T, Guo B, et al. Estrogen and raloxifene, a selective estrogen receptor modulator, ameliorate renal damage in db/db mice. Am J Pathol (2005) 166:1629–36. doi: 10.1016/S0002-9440(10)62473-X
Keywords: sex hormones, estrogen, testosterone, diabetic nephropathy, metabolism
Citation: Liu J, Liu Z, Sun W, Luo L, An X, Yu D and Wang W (2023) Role of sex hormones in diabetic nephropathy. Front. Endocrinol. 14:1135530. doi: 10.3389/fendo.2023.1135530
Received: 01 January 2023; Accepted: 22 March 2023;
Published: 18 April 2023.
Edited by:
Saleem Aladaileh, University of Hafr Al Batin, Saudi ArabiaReviewed by:
Anqun Chen, Second Xiangya Hospital, Central South University, ChinaIvonne Löffler, University Hospital Jena, Germany
Joel Neugarten, Albert Einstein College of Medicine, United States
Copyright © 2023 Liu, Liu, Sun, Luo, An, Yu and Wang. This is an open-access article distributed under the terms of the Creative Commons Attribution License (CC BY). The use, distribution or reproduction in other forums is permitted, provided the original author(s) and the copyright owner(s) are credited and that the original publication in this journal is cited, in accordance with accepted academic practice. No use, distribution or reproduction is permitted which does not comply with these terms.
*Correspondence: Dehai Yu, eXVkZWhhaUBqbHUuZWR1LmNu; Wanning Wang, d3duQGpsdS5lZHUuY24=