- 1Shanghai Key Laboratory for Endocrine Tumors, Shanghai Clinical Center for Endocrine and Metabolic Diseases, Key Laboratory for Endocrine and Metabolic Diseases of the Chinese Health Ministry, Ruijin Hospital, Shanghai Jiao Tong University School of Medicine, Shanghai, China
- 2Laboratory for Endocrine and Metabolic Diseases, Institute of Health Sciences, Shanghai Jiao Tong University School of Medicine, and Shanghai Institutes for Biological Sciences, Chinese Academy of Sciences, Shanghai, China
Background: Idiopathic hyperaldosteronism (IHA) is one of the most common types of primary aldosteronism (PA), an important cause of hypertension. Although high dietary sodium is a major risk factor for hypertension, there is no consensus on the recommended dietary sodium intake for IHA.
Objective: This study investigated the effect of a low-sodium diet on hemodynamic variables and relevant disease biomarkers in IHA patients, with the aim of providing a useful reference for clinical treatment.
Methods: Fifty IHA patients were evenly randomized into two groups and provided, after a 7-day run-in period (100 mmol/d sodium), either a low-sodium diet (50 mmol/d sodium) or a normal sodium diet (100 mmol/d sodium) for an additional 7 days. After the 14-day intervention (conducted without potassium supplementation), changes in blood pressure (BP) and serum potassium were evaluated in both groups.
Results: After the dietary intervention, the low sodium group exhibited, compared to the normal sodium group, decreased BP (SBP: 121.8 ± 12.8 vs. 129.9 ± 12.1 mmHg, p < 0.05; DBP: 82.6 ± 7.6 vs. 86.4 ± 8.2 mmHg, p < 0.05; MAP: 95.7 ± 8.8 vs. 100.9 ± 8.4 mmHg, p < 0.05) and increased serum potassium levels (3.38 ± 0.33 vs. 3.07 ± 0.27 mmol/L, p < 0.001). The low sodium group showed also better control of both BP and serum potassium: BP <140/90 mmHg in 70.0% of total patients (76.0% vs. 64.0%, in the low and normal sodium groups, respectively; p > 0.05), BP <130/85 mmHg in 38.0% of total patients (56.0% vs. 20.0%, p < 0.05), BP <120/80 mmHg in 28.0% of total patients (44.0% vs. 12.0%, p < 0.05); serum potassium ≥3.5 mmol/L in 22.0% of total patients (32.0% vs. 12.0% in the low and normal sodium groups, respectively; p = 0.088). There were differences between the controlled BP group (<120/80 mmHg) and the non-controlled BP group (≥120/80 mmHg) in gender, BP at baseline, and type of diet (low vs. normal sodium). Female gender and low-sodium diet were protective factors for BP control.
Conclusions: A low-sodium diet is effective in lowering BP and elevating serum potassium in IHA patients. Female patients on a low-sodium diet are more likely to achieve BP control (<120/80 mmHg). We advocate a dietary sodium intake of 50 mmol/d for IHA patients.
Clinical trial registration: https://clinicaltrials.gov, Identifier NCT05649631.
Introduction
Primary aldosteronism (PA) is considered the most common form of endocrine hypertension, accounting for 4%–10% of hypertension cases (1, 2). PA is characterized by hypertension, low serum potassium, and high plasma aldosterone concentration (PAC). PA patients are at greater risk of cardiovascular, metabolic, and renal disease than those with essential hypertension (3–8). Subtypes of PA include aldosterone-producing adenoma (APA), unilateral adrenal hyperplasia (UAH), bilateral adrenal hyperplasia (BAH; also called idiopathic hyperaldosteronism, IHA), adrenocortical carcinoma, and familial hyperaldosteronism. Among these PA subtypes, APA and IHA are the two most common forms (~90% of PA cases) (9). Unilateral PA (APA and UAH) is treated with surgery, while IHA can usually be treated by medication. Spironolactone, a competitive antagonist of the mineralocorticoid receptor and a potassium-preserving diuretic, is commonly used to treat PA. However, it has dose-dependent side effects, including gynecomastia and impotence in males and menstrual irregularities in females. Results from early studies showed that the incidence of gynecomastia was 10% at a spironolactone dose of 25 mg/day (10), and 52% or more at a dose of 150 mg/day (11). Thus, alternative or adjuvant therapies to reduce the dose of spironolactone are in urgent need.
Sodium in diet is mainly derived from NaCl (salt) and is essential for cellular homeostasis and body function. One gram of sodium is contained in ~2.5 g of salt, and almost 90% of sodium in the diet comes from table salt. Both the World Health Organization (WHO) (12) and the European Society of Hypertension/European Society of Cardiology (ESH/ESC) (13) recommend a daily salt intake of no more than 5 g (equivalent to 87 mmol/L of sodium) in the general population and in hypertensive patients. However, research has showed that in most countries the average salt intake is ~10 g/d (14, 15). Sodium intake is particularly high among Chinese people. In a large research study that included population samples from East Asian and Western countries, the Beijing population had the highest average sodium intake, reaching 300 mmol/d for males and 250 mmol/d for females (i.e. 17.6 and 14.8 g of salt, respectively) (16).
Aldosterone excess has a marked effect on taste perception. A prospective study found that NaCl taste perception was significantly impaired in patients with PA, which evidenced a NaCl taste recognition threshold about twice as high as that observed in essential hypertension (17). In a retrospective study, it was estimated that PA patients consumed at least 10 g of salt per day in their diet, with significantly higher mean intake reported for unilateral PA compared to bilateral PA (11.9 g/d vs. 10.4g/d, respectively) (18). There is a direct linear relationship between salt intake and risk of cardiovascular disease and/or death (19–21), and several studies have shown that sodium is an important causative factor in essential hypertension (22–25). Thus, target organ deterioration is greatly increased by both hyperaldosteronism and high sodium intake (26).
Reducing sodium intake in essential hypertensive patients has been shown to lower blood pressure (BP) (27) and reduce cardiovascular events (28). Similarly, reducing sodium intake in patients with PA can significantly improve left ventricular mass index (29). Regulation of sodium and potassium homeostasis occurs primarily in the renal tubules, and high sodium intake determines increased renal excretion of potassium (30, 31). Therefore, we conducted the present trial based on the hypothesis that in patients with IHA serum potassium would be increased by reducing sodium intake.
A reduction in sodium intake is usually followed by a drop in blood volume, which activates the renin-angiotensin-aldosterone system (RAAS) (32, 33). A study has shown a mild increase in plasma renin activity (PRA) after moderate reduction in sodium intake (34), but it is not clear whether a similar change may occur in PA. A retrospective study showed that elevated PRA contributed to a reduction in cardiovascular events (35), and similar findings were found in an observational study (36). Hence, in IHA patients an elevation in PRA after reducing sodium intake would represent a positive effect.
The current guidelines for PA do not specify a recommended intake of sodium, and the effect of reduced sodium intake on PA is unclear. Based on this, and the above considerations, the goal of this study was to investigate the effect of a low-sodium diet on IHA and to provide a useful reference for clinical practice.
Methods
Patients
We screened 168 inpatients (all of them are Chinese Han population) with suspected PA who were admitted to Ruijin Hospital Affiliated to Shanghai Jiao Tong University School of Medicine from July 2021 to September 2022. A total of 150 patients diagnosed with PA by saline infusion test (SIT) were initially evaluated for study inclusion. According to adrenal computed tomography (CT) and adrenal venous sampling (AVS), we identified 81 UPA, 56 IHA, and 13 unclassifiable PA cases. None of the patients had restricted their sodium intake before definitive subtype diagnosis. We conducted a dietary intervention on 52/56 IHA cases, as four patients refused to participate in the trial. Two patients withdrew from the trial during its run-in period (one with serum potassium <2.8 mmol/L and another who could not tolerate the dietary arrangement). A total of 50 IHA patients were randomly assigned to either a low sodium group or a normal sodium diet group (25 per group) and completed the dietary intervention trial (Figure 1). This study was approved by the Ethics Committee of Ruijin Hospital, Shanghai Jiao Tong University of Medicine, and all patients provided written informed consent to participate.
Enrollment criteria
Inclusion criteria were: 1) 18–70 years of age; 2) diagnosed as PA by SIT; 3) no lateralization of aldosterone secretion according to AVS; and 4) serum potassium ≥ 2.8 mmol/L after the run-in period. Exclusion criteria included: 1) impaired renal function [creatinine clearance rate (Ccr) <60 ml/min]; 2) impaired liver function [alanine transaminase (ALT) and aspartate transaminase (AST) >2.5 times upper limit of normal]; 3) patients with heart failure [New York Heart Association (NYHA) ≥class 3 or ejection fraction (EF) <50%]; 4) patients with stroke or acute infarction in the last 6 months; 5) patients who are pregnant or breastfeeding; 6) patients who cannot tolerate the dietary arrangements; and 7) patients with history of malignant tumors in the last 6 months.
Diagnosis criteria
PA was diagnosed according to the 2016 Endocrine Society Clinical Practice Guidelines (9). Patients with aldosterone-to-renin ratio (ARR) >30 (ng/dl)/(mg/dl/h) underwent a confirmatory test (SIT). Diagnosis of PA was established if PAC >10 ng/dl after SIT. Adrenal CT and AVS were used to differentiate between unilateral PA and bilateral PA. Cannulation was considered successful if the cortisol adrenal vein/cortisol peripheral vein ratio was greater than 3 without adrenal corticotropic hormone (ACTH) stimulation. The cortisol-corrected aldosterone (A/C) ratio served to correct adrenal venous aldosterone levels for differing degrees of dilution of adrenal vs. peripheral venous blood. (A/C) adrenal vein/(A/C) contralateral adrenal vein >2 was considered as evidence of dominant aldosterone secretion. IHA was diagnosed by: 1) a biochemical diagnosis of PA; and 2) no lateralization of aldosterone secretion according to AVS.
Research design
This study was a single-center randomized controlled trial which lasted 14 days and consisted of two stages (run-in period and intervention period), each one comprising 7 days without potassium supplementation. If participants met the enrollment criteria at the end of the run-in period, they were randomly assigned (random number method) 1:1 to the low sodium group (50 mmol/d sodium) or the normal sodium group (100 mmol/d sodium) until the end of the intervention period. We took into account both the sodium contained in the food and the sodium in the salt. The primary outcome was the change in BP and serum potassium, and their corresponding control rates. The secondary outcome was the change in RAAS activity.
Management of blood pressure and serum potassium
The patients were prepared from the pharmacological standpoint by stopping spironolactone and other diuretics at least 4 weeks and ACEI and ARB medications at least 2 weeks before SIT; all patients were put on a long-acting Ca2+ channel blocker at the time when these measurements were made. From the first day of the trial, patients were given nifedipine controlled-release tablets (30 mg/d) to lower BP. During the run-in period, the drug was discontinued if the subject’s BP was less than 90/60mmHg, and drug dosage increased to 60 mg/d if the subject had an increase in BP (>180/110 mmHg). During the entire dietary intervention, the BP-lowering protocol was the same as in the run-in period. There was no potassium supplementation during the trial, and patients were withdrawn from the study if they could not tolerate the diet or if their serum potassium fell below 2.8 mmol/L.
Diet management
Meal recipes were designed by professional nutritionists in our hospital. Based on dietary guidelines of China (37) and studies of low-sodium diets in essential hypertension (27), we specified 100 mmol and 50 mmol sodium per day for the normal sodium diet and the low-sodium diet, respectively, and controlled the dietary sodium intake of the participants by adding salt packets (NaCl) to salt-free meals. A daily salt-free meal with 1800 kcal or 1600 kcal was provided for males and females, respectively. The ratio of carbohydrates/protein/fat was 4:3:3. The normal sodium group received 100 mmol sodium per day in both the run-in and the intervention periods, and the low sodium group received 100 mmol sodium per day in the run-in period and 50 mmol sodium per day during the intervention period. The participants consumed all food and salt provided, and were not offered additional food or drink throughout the study.
Clinical and biochemical tests
Systolic blood pressure (SBP), diastolic blood pressure (DBP), and mean arterial pressure (MAP) = DBP + 1/3 (SBP-DBP) were determined twice a day (7 a.m. and 16 p.m.) using an Omron electronic blood pressure monitor. Patients sat quietly in a quiet environment for 5–10 min before starting BP measurements, and were not allowed to drink strong tea, coffee, or alcohol for the preceding 30 min. The subject’s arm was placed at the same level as the right atrium and abducted to 45°, and the cuff was emptied of gas using a uniform electronic sphygmomanometer with the lower edge of the cuff 2–3 cm from the elbow fossa. BP was measured again at 1–2 min intervals and values averaged. We considered the average of BP at 7 a.m. and 16 p.m.
Peripheral venous blood was collected on the mornings of Day 1 (before the run-in period), Day 8 (end of the run-in period), and Day 15 (end of the intervention period) for routine hematology, liver and kidney function, PRA, PAC, c-reactive protein (CRP), brain natriuretic peptide (BNP), and troponin I (TNI) measurements. We tested serum electrolytes and 24-h urine electrolytes daily, and recorded daily water intake and fasting weight in the morning. Blood samples were kept at -4°C immediately after collection and sent for testing within 2 h.
Biochemical measurements
Blood samples for clinical chemistry analysis were collected after an overnight fast of at least 10 h. All tests were performed in a College of American Pathologists-accredited laboratory (No. 7217913). Serum aldosterone and plasma renin activities were measured with a radioimmunoassay kit (Beckman Coulter Corp.) following the manufacturer’s instructions. Serum cortisol and serum ACTH were measured via immunoluminescence and radioimmunoassay, respectively (Beckman Coulter Corp.), following the manufacturer’s instructions.
Statistical analysis
SPSS 26.0 software was used for statistical analyses. Normally distributed data were presented as means ± standard deviation (SD) and non-normally distributed data were expressed as median and interquartile intervals (IQ 25-75%). Categorical variables were presented as frequencies or percentages. The t-test and chi-square test were used for comparisons between two groups for continuous and categorical variables, respectively. Multivariable regression analysis was performed to investigate the factors influencing BP after dietary interventions. Scatter plots and bar charts were plotted using GraphPad (8.0) software; p < 0.05 on two-side tests was defined as significant. Sample size was calculated based on previous studies, assuming that SBP drops by 4.6 (3.2–5.9) mmHg for patients in the low sodium group and by 2.1 (0.8–3.4) mmHg for patients in the normal sodium group (27). A sample size of 21 patients per group was estimated to provide the trial with 90% power to detect differences at a two-sided alpha level of 0.05.
Results
Baseline characteristics of participants
We recruited 50 IHA patients for the dietary intervention trial and assigned them to the low sodium group or the normal sodium group (25 patients per group) in a randomized manner. The trial’s timeline is shown in Figure 2. Baseline characteristics of the two groups are presented in Table 1. Before the trial, there was no difference between the normal and low sodium groups in gender (male, 60.0% vs. 48.0%, p > 0.05), age (52.2 ± 10.3 vs. 53.2 ± 10.7 years, p > 0.05), BMI (26.0 ± 5.1 vs. 25.7 ± 4.6 kg/m2, p > 0.05), SBP (141.7 ± 12.6 vs. 144.0 ± 12.0 mmHg, p > 0.05), DBP (90.2 ± 9.9 vs. 91.2 ± 8.7 mmHg, p > 0.05), PRA [0.41 (0.24, 0.83) vs. 0.49 (0.34, 0.70) ng/ml/h, p > 0.05], PAC [28.6 (17.9, 45.8) vs. 33.0 (21.2, 39.1) ng/dl, p > 0.05], serum sodium (143.4 ± 2.1 vs. 142.6 ± 2.0 mmol/L, p > 0.05), and serum potassium (3.23 ± 0.36 vs. 3.28 ± 0.29 mmol/L, p > 0.05). Before the trial, the defined daily dose (DDD) (38), i.e. the assumed average maintenance daily dose for a drug used in adults for its main indication, was only mildly different (1.7 ± 1.1 vs. 2.0 ± 1.3, p > 0.05) between the two groups.
Changes in clinical and biochemical parameters after the dietary intervention
During the run-in period two participants (one in the low sodium group and the other in the normal sodium group) had BP <90/60 mmHg and discontinued their antihypertensive medication. Another participant in the normal sodium diet group had BP consistently greater than 180/110 mmHg and his antihypertensive medication was increased to 60 mg/d. Parameters after the dietary intervention for both groups are presented in Table 2. After the 14-day dietary intervention, there was a slight, non-significant decrease in body weight in both groups. After the run-in period, BP decreased comparably in the normal and low sodium groups: SBP, 134.0 ± 11.3 vs. 130.7 ± 13.4 mmHg, p > 0.05; DBP, 86.2 ± 8.0 vs. 85.8 ± 8.1 mmHg, p > 0.05; MAP, 102.1 ± 7.8 vs.100.8 ± 8.8 mmHg, p > 0.05, respectively. At the end of the intervention period, no significant change in BP was detected in patients in the normal sodium group, whereas a further decrease in BP was recorded in those in the low sodium group. Respective BP values were: SBP, 129.9 ± 12.1 vs. 121.8 ± 12.8 mmHg, p < 0.05 (Figure 3A); DBP, 86.4 ± 8.2 vs. 82.6 ± 7.6 mmHg, p < 0.05 (Figure 3B); and MAP, 100.9 ± 8.4 vs. 95.7 ± 8.8 mmHg, p < 0.05.
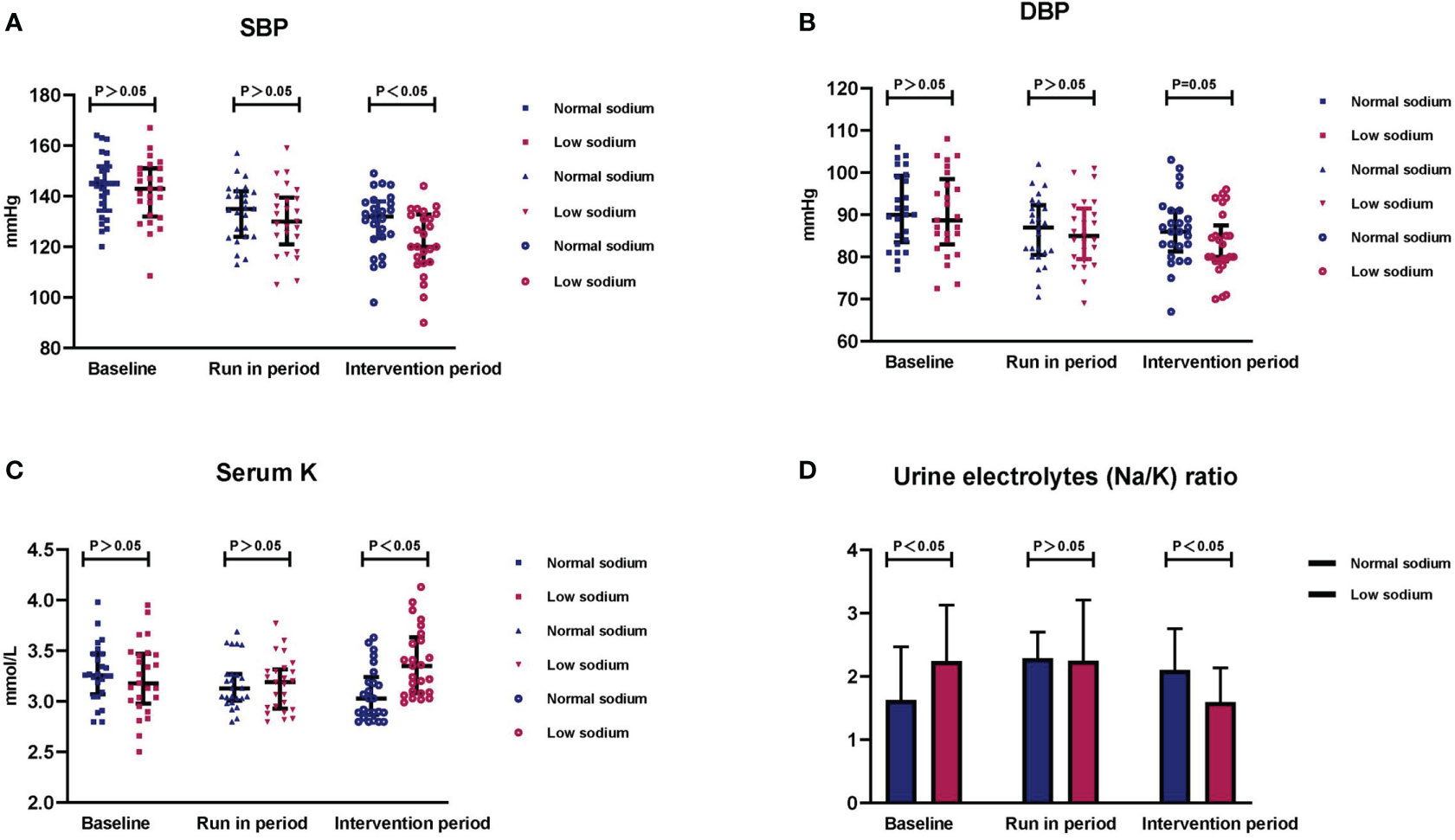
Figure 3 Changes in blood pressure, serum potassium, and urinary electrolytes after the dietary intervention. Summary data of (A) SBP, (B) DBP, (C) serum potassium, and (D) 24-h urine sodium to potassium ratio (Na+/K+) in the two study groups after the intervention period. Data are shown as median with interquartile ranges.
After discontinuation of potassium supplementation, serum potassium decreased similarly in both groups after the run-in period (3.18 ± 0.25 vs. 3.17 ± 0.26 mmol/L for the normal and low sodium groups, respectively; p > 0.05). After the intervention period, serum potassium did not change significantly in the normal sodium group, and was significantly increased in the low sodium group (3.07 ± 0.27 vs. 3.38 ± 0.33 mmol/L, respectively; p < 0.001) (Figure 3C). Serum sodium remained relatively stable during the trial in both groups.
Supplementary Table 1 shows the changes of parameters (SBP, DBP, MAP, and serum potassium) within the low and normal sodium groups. Patients who received 100 mmol/d sodium (normal sodium diet) during the run-in period had significant reductions in BP (SBP mean decrease of 10.1 ± 11.0 mmHg, DBP mean decrease of 5.0 ± 7.0 mmHg, and MAP mean decrease of 6.7 ± 7.4 mmHg), and the above indicators did not change significantly after the intervention period. Similarly, patients in the low sodium group had a significant decrease in BP after the run-in period (SBP mean decrease 11.1 ± 11.0 mmHg, DBP mean decrease 4.4 ± 8.2 mmHg, MAP, mean decrease 6.6 ± 8.5 mmHg), and a further decrease after the intervention period (SBP mean decrease 8.8 ± 6.1 mmHg, DBP mean decrease of 3.2 ± 6.3 mmHg, MAP mean decrease of 5.1 ± 5.2 mmHg). After the intervention period, the mean increase in potassium was 0.22 ± 0.20 mmol/L in the low sodium group, and it was not increased in the normal sodium group.
After the run-in period, no significant differences between the low and normal sodium groups were detected for 24-h urinary sodium (93.9 ± 16.6 vs. 97.8 ± 16.7 mmol/L/24 h, p > 0.05), 24-h urinary potassium (42.1 ± 15.5 vs. 48.0 ± 15.0 mmol/L/24 h, p > 0.05), and urinary electrolyte ratio (sodium/potassium) [2.3 (1.7, 3.2 vs. 2.3 (1.6, 2.8), p > 0.05]. After the intervention period, these parameters were all significantly lower in the low sodium group compared to the normal sodium group [24-h urinary sodium, 54.3 ± 24.5 vs. 96.1 ± 27.0 mmol/L/24 h, p < 0.05; 24-h urinary potassium, 33.0 ± 10.8 vs. 45.4 ± 13.9 mmol/L/24 h, p < 0.05; urinary electrolyte ratio, 1.6 (1.2, 2.1) vs. 2.1 (1.6, 2.8), p < 0.05 (Figure 3D)].
After the run-in period, the low and normal sodium groups showed no differences in PRA [0.60 (0.41, 1.28) vs. 0.43 (0.29, 0.96) ng/ml/h, p > 0.05] or PAC [28.2 (18.9, 40.7) vs. 21.7 (19.1, 34.5) ng/dl, p > 0.05]. After the intervention phase, both PRA and PAC were mildly increased in the low and normal sodium groups, with no significant intergroup differences: PRA [0.78 (0.49, 1.45) vs. 0.63 (0.33, 1.03) ng/ml/h, p > 0.05], PAC [29.9 (20.5, 35.5) vs. 26.6 (20.7, 54.1) ng/dl, p > 0.05]. After the intervention period, CRP was lower in the low sodium group than in the normal sodium group [0.8 (0.5, 1.1) vs. 1.2 (1.0, 2.7) mg/L, respectively; p < 0.05]. In turn, BNP was significantly decreased in both groups, attaining post-trial values that were not statistically different from each other [32.3 (20.0, 74.9) vs. 61.1 (33.4, 83.3) pg/ml, p > 0.05]. After the dietary intervention, uric acid decreased in patients in the normal sodium group (from 331.7 ± 89.5 to 315.2 ± 96.0 µmol/L) and increased in patients in the low sodium group (from 303.8 ± 92.1 to 318.0 ± 103.0 µmol/L), with a statistical difference noted between post-intervention values for the two groups (315.2 ± 96.0 vs. 318.0 ± 103.0 µmol/L, p < 0.05). There was no statistical difference in urea nitrogen and Ccr between the two groups.
Serum potassium and BP control rates
We analyzed the control rates of BP and serum potassium at different levels after the dietary intervention (Table 3). Control rates for SBP <120 mmHg (48.0% vs. 20.0%, p < 0.05) and DBP <80 mmHg (52.0% vs. 20.0%, p < 0.05) were higher in the low sodium group, whereas the normal sodium group exhibited higher control of 80≤ DBP <90 mmHg (52.0% vs. 24.0%, p < 0.05). There was no significant difference in the control of other BP levels between the two groups (p > 0.05). In addition, the SBP/DBP control rate in the low sodium group was overall higher than in the normal sodium group: <140/90 mmHg, 76.0% vs. 64.0%, respectively; p > 0.05; mean, 70.0%), <130/85 mmHg, 56.0% vs. 20.0%; p < 0.05; mean, 38.0%), <120/80 mmHg, 44.0% vs. 12.0%; p < 0.05; mean, 28.0%).
After the intervention period, patients in the low sodium group had, compared to the normal sodium group, a higher but not significantly different control rate of serum potassium ≥3.5 mmol/L (32.0% vs. 12.0%, p > 0.05), 3.2≤ serum potassium <3.5 mmol/L (32.0% vs. 12.0%, p > 0.05), and 3.0≤ serum potassium <3.2 mmol/L (32.0% vs. 28.0%, p > 0.05). The proportion of patients with serum potassium <3.0 mmol/L was higher in the normal sodium group (48.0% vs. 4.0%, p < 0.05).
Factors affecting BP control
Based on the 2018 ESC/ESH guidelines (39) and the specificity of PA, we defined BP (SBP/DBP) <120/80 mmHg as BP control and compared the differences between the controlled BP and the uncontrolled BP groups (Table 4). After the trial, 14 of the 50 patients (28.0%) had controlled BP. Patients in the controlled BP group had the following characteristics: more females (71.4% vs. 36.1%, p < 0.05), lower SBP (135.0 ± 12.6 vs. 145.9 ± 10.8 mmHg, p < 0.05), and lower MAP (103.4 ± 10.2 vs. 109.9 ± 8.3 mmHg, p < 0.05) at baseline, and were mostly allocated to the low-sodium diet (78.6% vs. 38.9%, p < 0.05). There were no differences in baseline DBP, DDD, PRA, PAC, ARR, serum sodium, and serum potassium between groups (all p > 0.05). The univariate logistic regression analysis showed significant associations for female gender [OR (CI 95%): 0.226 (0.059, 0.867), p < 0.05], SBP at baseline [0.918 (0.861, 0.979), p < 0.05], MAP at baseline [0.919 (0.851, 0.919), p < 0.05], and low-sodium diet [0.174 (0.041, 0.734), p < 0.05] and controlled BP (<120/80 mmHg). Further multiple regression analysis revealed that female gender [0.157 (0.026, 0.959), p < 0.05] and low-sodium diet [0.070 (0.009, 0.519), p < 0.05] were independent protective factors for controlled BP (Table 5).
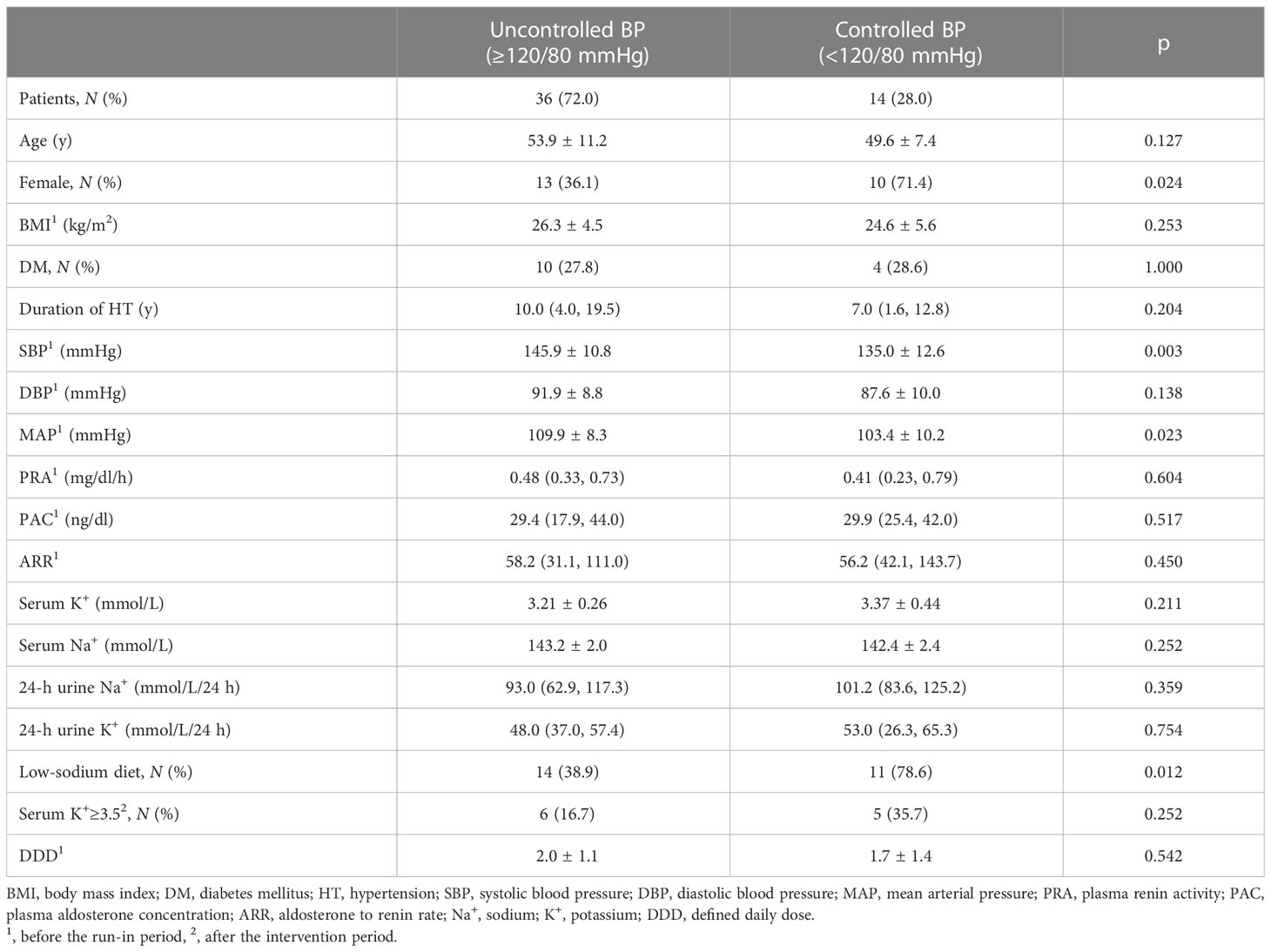
Table 4 Demographic and clinical characteristics of patients with controlled and uncontrolled BP after the dietary intervention.
Discussion
Recent studies reported high prevalence of PA (29.1%) in patients with resistant hypertension (40) and association of this condition with severe target organ damage and cardiovascular events (41). IHA accounts for 60% of PA cases (9), is defined by excess aldosterone originating from bilateral adrenal glands, and is usually treated medically with mineralocorticoid receptor antagonists (MRAs) (42). These regimens have the disadvantage of being poorly tolerated when used at high doses. Therefore, we conducted this study with the aim of evaluating a non-pharmacological alternative to MRA or measures aimed at helping reduce its dosage. Our study has several important findings. First, it suggests a specific recommended sodium intake, which could be an important addition to current guidelines (43), and highlights that sodium levels in food ingredients should not be ignored. After the run-in period (100 mmol/d sodium), BP decreased more in both groups (SBP ~10 mmHg, MAP ~6.6 mmHg) and there was a further decrease in BP (SBP ~8.8 mmHg, MAP ~5.1 mmHg) after the intervention period in patients in the low sodium group (50 mmol/d sodium). Similar results were reported in the Dietary Approaches to Stop Hypertension (DASH) study (27), although the latter was conducted in patients with mild hypertension. There are a number of factors that can influence the effect of dietary sodium on BP. For instance, a study showed that the antihypertensive effect of a low-sodium diet varies with ethnicity and BP levels (22), but our study does not have these caveats.
Second, in our study the total control rate of SBP <120 mmHg was 34.0% (17/50 patients), with greater representation of patients in the low, compared to the normal, sodium group (48.0% vs. 20.0%, respectively; p < 0.05). Meanwhile, the total control rate of DBP <80 mmHg was 36.0% (18/50 patients) with, again, a higher control rate recorded in the low sodium group (52.0% vs. 20.0% in the normal sodium group; p < 0.05). This implies that reducing dietary sodium intake can lead to better control of BP in IHA. We also found that a low-sodium diet provides better control of BP (SBP/DBP <120/80 mmHg) in female patients. It is well known that sex hormones play an important role in BP regulation. Premenopausal women typically have a lower BP compared to age-matched men, and both endogenous and exogenous estrogens have hypotensive effects (44–46). Therefore, in line with mounting evidence that a low-sodium diet complements other non-pharmacological and pharmacological interventions aimed at lowering BP, we recommend a low-sodium diet as a first consideration for managing BP in patients, and specially women, with IHA. Third, our data showed that reducing sodium intake in IHA can effectively raise serum potassium levels. Normal kidney function is essential for regulating the balance of sodium and potassium in the body, as renal potassium excretion is influenced by sodium intake (47). When sodium intake decreases, urinary potassium elimination is reduced, and serum potassium increases. Even after discontinuing of potassium supplementation, before initiating the trial, serum potassium decreased only mildly (mean reduction of 0.08 ± 0.33 mmol/L) after supplying 100 mmol sodium intake per day during the run-in period. Moreover, after the trial, serum potassium levels were augmented (mean increase of 0.22 ± 0.20 mmol/L) in the low sodium group. Although serum potassium exceeded 3 mmol/L in both groups after reducing sodium intake, the average level was significantly higher in the low, compared to the normal, sodium diet group (3.38 ± 0.33 vs. 3.07 ± 0.27 mmol/L, respectively; p < 0.001), which may favor reducing or eliminating the need for potassium supplements or MRAs. Worthy of note, we found that by the end of the trial (conducted without potassium supplementation), in the low sodium group 96.0% of patients had serum potassium ≥3.0 mmol/L, 64.0% had serum potassium ≥3.2 mmol/L, and 32.0% had serum potassium ≥3.5 mmol/L. Therefore, serum potassium levels can be effectively raised by reducing sodium intake.
Changes in PRA following reduced sodium intake may be beneficial for IHA. Hundemer et al. (35) showed that PRA may be a predictor of cardiovascular outcomes and may serve as an important indicator of treatment response. In our study, PRA rose in both groups after the dietary intervention, but there was no difference between the two groups. This may be due to the short duration of the trial and/or inadequate sample size. Excessive sodium intake inhibits the RAAS, which in turn reduces sodium reabsorption and thus promotes its excretion (48). Furthermore, IHA patients have an elevated NaCl taste threshold, influenced by high aldosterone levels (17), which determines a daily sodium intake significantly higher than that specified by dietary guideline recommendations (18). Therefore, a low-sodium diet is fundamentally apt to control BP and serum potassium and to improve long-term prognosis in IHA patients.
In conclusion, reducing dietary sodium was effective in lowering BP and raising serum potassium in patients with IHA who were maintained on low-dose antihypertensive medications without potassium supplementation. These effects were more pronounced in participants consuming 50 mmol, rather than 100 mmol, sodium per day over the trial’s duration. Specifically, in the low-sodium group (50 mmol/d sodium) 44.0% of patients had normal BP (<120/80 mmHg) and 32.0% of patients had normal serum potassium (3.5–5.5 mmol/L) at the end of the trial. In addition, the low-sodium diet was associated with better BP control in female IHA patients.
There are some limitations in our study. First, as it lasted only 2 weeks, potential long-term effects of the low-sodium diet on IHA need to be further explored. Second, excessive aldosterone concentrations persist after adopting a low-sodium diet, an issue that may be adequately addressed by low-dose MRA treatment.
Data availability statement
The data analyzed in this study is subject to the following licenses/restrictions: Data available on request from the authors. Requests to access these datasets should be directed to Lihua Zhou, emxoMTg4MDEyM0AxMjYuY29t.
Ethics statement
The studies involving human participants were reviewed and approved by Ruijin Hospital Ethics Committee Shanghal JiaoTong University School of Medicine. The patients/participants provided their written informed consent to participate in this study.
Author contributions
LZ, YJ and WW contributed to conception and design of the study. LZ organized the database and performed the statistical analysis. LZ and YJ wrote the first draft of the manuscript. CZ, TS, LJ, WZ, XZ, and LW wrote sections of the manuscript. Both LZ and YJ are responsible for research design and implementation, data analysis, and writing the manuscript. All authors contributed to manuscript revision, read, and approved the submitted version.
Funding
This study was supported by the National Key Research and Development Program of China (2021YFC2501600, 2021YFC2501603), the Shanghai Shenkang Hospital Development Center (SHDC2020CR2002A, SHDC2020CR6015), Natural Science Foundation of Shanghai (22ZR1439100), and National Natural Science Foundation of China (82170797).
Acknowledgments
We are grateful to Prof. Guang Ning, MD, for his critical revision and input on the study design.
Conflict of interest
The authors declare that the research was conducted in the absence of any commercial or financial relationships that could be construed as a potential conflict of interest.
Publisher’s note
All claims expressed in this article are solely those of the authors and do not necessarily represent those of their affiliated organizations, or those of the publisher, the editors and the reviewers. Any product that may be evaluated in this article, or claim that may be made by its manufacturer, is not guaranteed or endorsed by the publisher.
Supplementary material
The Supplementary Material for this article can be found online at: https://www.frontiersin.org/articles/10.3389/fendo.2023.1124479/full#supplementary-material
Supplementary Table 1 | Intra-group comparison of blood pressure and serum potassium. Normal-G, normal sodium diet group; Low-G, low-sodium diet group; SBP, systolic blood pressure; DBP, diastolic blood pressure; MAP, mean blood pressure; K+, potassium; BP-related variables expressed in mmHg; Serum K+ levels expressed in mmol/L. p0, comparison of baseline and run-in periods; p1, comparison of run-in and intervention periods; p2, comparison of baseline and intervention periods; 1 #, change between baseline and run-in period; 2 #, change between run-in and intervention period.
References
1. Hannemann A, Wallaschofski H. Prevalence of primary aldosteronism in patient's cohorts and in population-based studies–a review of the current literature. Horm Metab Res (2012) 44(3):157–62. doi: 10.1055/s-0031-1295438
2. Xu Z, Yang J, Hu J, Song Y, He W, Luo T, et al. Primary aldosteronism in patients in China with recently detected hypertension. J Am Coll Cardiol (2020) 75(16):1913–22. doi: 10.1016/j.jacc.2020.02.052
3. Monticone S, D'Ascenzo F, Moretti C, Williams TA, Veglio F, Gaita F, et al. Cardiovascular events and target organ damage in primary aldosteronism compared with essential hypertension: a systematic review and meta-analysis. Lancet Diabetes Endocrinol (2018) 6(1):41–50. doi: 10.1016/S2213-8587(17)30319-4
4. Rossi GP. Primary aldosteronism: JACC state-of-the-Art review. J Am Coll Cardiol (2019) 74(22):2799–811. doi: 10.1016/j.jacc.2019.09.057
5. Quinkler M, Born-Frontsberg E, Fourkiotis VG. Comorbidities in primary aldosteronism. Horm Metab Res (2010) 42(6):429–34. doi: 10.1055/s-0029-1243257
6. Reincke M, Meisinger C, Holle R, Quinkler M, Hahner S, Beuschlein F, et al. Is primary aldosteronism associated with diabetes mellitus? results of the German conn's registry. Horm Metab Res (2010) 42(6):435–9. doi: 10.1055/s-0029-1246189
7. Reincke M, Fischer E, Gerum S, Merkle K, Schulz S, Pallauf A, et al. Observational study mortality in treated primary aldosteronism: the German conn's registry. Hypertension (2012) 60(3):618–24. doi: 10.1161/HYPERTENSIONAHA.112.197111
8. Mulatero P, Monticone S, Bertello C, Viola A, Tizzani D, Iannaccone A, et al. Long-term cardio- and cerebrovascular events in patients with primary aldosteronism. J Clin Endocrinol Metab (2013) 98(12):4826–33. doi: 10.1210/jc.2013-2805
9. Funder JW, Carey RM, Mantero F, Murad MH, Reincke M, Shibata H, et al. The management of primary aldosteronism: case detection, diagnosis, and treatment: an endocrine society clinical practice guideline. J Clin Endocrinol Metab (2016) 101(5):1889–916. doi: 10.1210/jc.2015-4061
10. Pitt B, Zannad F, Remme WJ, Cody R, Castaigne A, Perez A, et al. The effect of spironolactone on morbidity and mortality in patients with severe heart failure. randomized aldactone evaluation study investigators. N Engl J Med (1999) 341(10):709–17. doi: 10.1056/NEJM199909023411001
11. Jeunemaitre X, Chatellier G, Kreft-Jais C, Charru A, DeVries C, Plouin PF, et al. Efficacy and tolerance of spironolactone in essential hypertension. Am J Cardiol (1987) 60(10):820–5. doi: 10.1016/0002-9149(87)91030-7
12. World Health Organization. Guideline: Sodium intake for adults and children. Geneva: world health organization copyright © 2012. World Health Organization (2012). Available at: https://www.ncbi.nlm.nih.gov/books/NBK132015/?report=reader
13. Williams B, Mancia G, Spiering W, Agabiti Rosei E, Azizi M, Burnier M, et al. 2018 Practice guidelines for the management of arterial hypertension of the European society of hypertension and the European society of cardiology: ESH/ESC task force for the management of arterial hypertension. J Hypertens (2018) 36(12):2284–309. doi: 10.1097/HJH.0000000000001961
14. Powles J, Fahimi S, Micha R, Khatibzadeh S, Shi P, Ezzati M, et al. Global, regional and national sodium intakes in 1990 and 2010: a systematic analysis of 24 h urinary sodium excretion and dietary surveys worldwide. BMJ Open (2013) 3(12):e003733. doi: 10.1136/bmjopen-2013-003733
15. Thout SR, Santos JA, McKenzie B, Trieu K, Johnson C, McLean R, et al. The science of salt: updating the evidence on global estimates of salt intake. J Clin Hypertens (Greenwich) (2019) 21(6):710–21. doi: 10.1111/jch.13546
16. Zhou BF, Stamler J, Dennis B, Moag-Stahlberg A, Okuda N, Robertson C, et al. Nutrient intakes of middle-aged men and women in China, Japan, united kingdom, and united states in the late 1990s: the INTERMAP study. J Hum Hypertens (2003) 17(9):623–30. doi: 10.1038/sj.jhh.1001605
17. Adolf C, Gorge V, Heinrich DA, Hoster E, Schneider H, Handgriff L, et al. Altered taste perception for sodium chloride in patients with primary aldosteronism: a prospective cohort study. Hypertension (2021) 77(4):1332–40. doi: 10.1161/HYPERTENSIONAHA.120.16440
18. Adolf C, Heinrich DA, Holler F, Lechner B, Nirschl N, Sturm L, et al. Patients with primary aldosteronism respond to unilateral adrenalectomy with long-term reduction in salt intake. J Clin Endocrinol Metab (2020) 105(3):e484–93. doi: 10.1210/clinem/dgz051
19. Aburto NJ, Ziolkovska A, Hooper L, Elliott P, Cappuccio FP, Meerpohl JJ. Effect of lower sodium intake on health: systematic review and meta-analyses. Bmj (2013) 346(apr03 3):f1326–f. doi: 10.1136/bmj.f1326
20. Strazzullo P, D'Elia L, Kandala NB, Cappuccio FP. Salt intake, stroke, and cardiovascular disease: meta-analysis of prospective studies. BMJ (2009) 339:b4567. doi: 10.1136/bmj.b4567
21. Poggio R, Gutierrez L, Matta MG, Elorriaga N, Irazola V, Rubinstein A. Daily sodium consumption and CVD mortality in the general population: systematic review and meta-analysis of prospective studies. Public Health Nutr (2015) 18(4):695–704. doi: 10.1017/S1368980014000949
22. Graudal NA, Hubeck-Graudal T, Jurgens G, Graudal NA. Effects of low sodium diet versus high sodium diet on blood pressure, renin, aldosterone, catecholamines, cholesterol, and triglyceride. Cochrane Database of Systematic Reviews (2011). doi: 10.1002/14651858.CD004022.pub3
23. Parfrey PS, Markandu ND, Roulston JE, Jones BE, Jones JC, MacGregor GA. Relation between arterial pressure, dietary sodium intake, and renin system in essential hypertension. Br Med J (Clin Res Ed) (1981) 283(6284):94–7. doi: 10.1136/bmj.283.6284.94
24. Mente A, O'Donnell MJ, Rangarajan S, McQueen MJ, Poirier P, Wielgosz A, et al. Association of urinary sodium and potassium excretion with blood pressure. N Engl J Med (2014) 371(7):601–11. doi: 10.1056/NEJMoa1311989
25. Welsh CE, Welsh P, Jhund P, Delles C, Celis-Morales C, Lewsey JD, et al. Urinary sodium excretion, blood pressure, and risk of future cardiovascular disease and mortality in subjects without prior cardiovascular disease. Hypertension (2019) 73(6):1202–9. doi: 10.1161/HYPERTENSIONAHA.119.12726
26. Pimenta E, Gordon RD, Stowasser M. Salt, aldosterone and hypertension. J Hum Hypertens (2013) 27(1):1–6. doi: 10.1038/jhh.2012.27
27. Sacks FM, Svetkey LP, Vollmer WM, Appel LJ, Bray GA, Harsha D, et al. Effects on blood pressure of reduced dietary sodium and the dietary approaches to stop hypertension (DASH) diet. DASH-sodium collaborative research group. N Engl J Med (2001) 344(1):3–10. doi: 10.1056/NEJM200101043440101
28. He FJ, Tan M, Ma Y, MacGregor GA. Salt reduction to prevent hypertension and cardiovascular disease: JACC state-of-the-Art review. J Am Coll Cardiol (2020) 75(6):632–47. doi: 10.1016/j.jacc.2019.11.055
29. Catena C, Colussi G, Novello M, Verheyen ND, Bertin N, Pilz S, et al. Dietary salt intake is a determinant of cardiac changes after treatment of primary aldosteronism: a prospective study. Hypertension (2016) 68(1):204–12. doi: 10.1161/HYPERTENSIONAHA.116.07615
30. Luft FC, Rankin LI, Bloch R, Weyman AE, Willis LR, Murray RH, et al. Cardiovascular and humoral responses to extremes of sodium intake in normal black and white men. Circulation (1979) 60(3):697–706. doi: 10.1161/01.CIR.60.3.697
31. O'Donnell M, Mente A, Rangarajan S, McQueen MJ, Wang X, Liu L, et al. Urinary sodium and potassium excretion, mortality, and cardiovascular events. N Engl J Med (2014) 371(7):612–23. doi: 10.1056/NEJMoa1311889
32. He FJ, MacGregor GA. Salt, blood pressure and the renin-angiotensin system. J Renin Angiotensin Aldosterone Syst (2003) 4(1):11–6. doi: 10.3317/jraas.2003.001
33. Alderman MH, Cohen HW. Dietary sodium intake and cardiovascular mortality: controversy resolved? Curr Hypertens Rep (2012) 14(3):193–201. doi: 10.1007/s11906-012-0275-6
34. He FJ, Li J, Macgregor GA. Effect of longer term modest salt reduction on blood pressure: cochrane systematic review and meta-analysis of randomised trials. BMJ (2013) 346:f1325. doi: 10.1136/bmj.f1325
35. Hundemer GL, Curhan GC, Yozamp N, Wang M, Vaidya A. Cardiometabolic outcomes and mortality in medically treated primary aldosteronism: a retrospective cohort study. Lancet Diabetes Endocrinol (2018) 6(1):51–9. doi: 10.1016/S2213-8587(17)30367-4
36. Hundemer GL, Curhan GC, Yozamp N, Wang M, Vaidya A. Incidence of atrial fibrillation and mineralocorticoid receptor activity in patients with medically and surgically treated primary aldosteronism. JAMA Cardiol (2018) 3(8):768–74. doi: 10.1001/jamacardio.2018.2003
37. Committee of Experts. Chinese Dieary reference intakes. Beijing: People's Health Publishing House (2022). Available at: https://www.cnsoc.org/bookpublica/0522202019.html.
38. WHO Collaborating Centre for Drug Statistics Methodology. Guidelines for ATC classification and DDD assignment. (2022). Available at: https://www.whocc.no/atc_ddd_index_and_guidelines/guidelines/.
39. Williams B, Mancia G, Spiering W, Agabiti Rosei E, Azizi M, Burnier M, et al. 2018 ESC/ESH guidelines for the management of arterial hypertension. Eur Heart J (2018) 39(33):3021–104. doi: 10.1093/eurheartj/ehy339
40. Parasiliti-Caprino M, Lopez C, Prencipe N, Lucatello B, Settanni F, Giraudo G, et al. Prevalence of primary aldosteronism and association with cardiovascular complications in patients with resistant and refractory hypertension. J Hypertens (2020) 38(9):1841–8. doi: 10.1097/HJH.0000000000002441
41. Monticone S, Burrello J, Tizzani D, Bertello C, Viola A, Buffolo F, et al. Prevalence and clinical manifestations of primary aldosteronism encountered in primary care practice. J Am Coll Cardiol (2017) 69(14):1811–20. doi: 10.1016/j.jacc.2017.01.052
42. Reincke M, Bancos I, Mulatero P, Scholl UI, Stowasser M, Williams TA. Diagnosis and treatment of primary aldosteronism. Lancet Diabetes Endocrinol (2021) 9(12):876–92. doi: 10.1016/S2213-8587(21)00210-2
43. Naruse M, Katabami T, Shibata H, Sone M, Takahashi K, Tanabe A, et al. Japan Endocrine society clinical practice guideline for the diagnosis and management of primary aldosteronism 2021. Endocr J (2022) 69(4):327–59. doi: 10.1507/endocrj.EJ21-0508
44. Hernández I, Delgado JL, Díaz J, Quesada T, Teruel MJ, Llanos MC, et al. 17beta-estradiol prevents oxidative stress and decreases blood pressure in ovariectomized rats. Am J Physiol Regul Integr Comp Physiol (2000) 279(5):R1599–605. doi: 10.1152/ajpregu.2000.279.5.R1599
45. Dubey RK, Oparil S, Imthurn B, Jackson EK. Sex hormones and hypertension. Cardiovasc Res (2002) 53(3):688–708. doi: 10.1016/S0008-6363(01)00527-2
46. Reckelhoff JF. Gender differences in the regulation of blood pressure. Hypertension (2001) 37(5):1199–208. doi: 10.1161/01.HYP.37.5.1199
47. Pechere-Bertschi A, Olivier V, Burnier M, Udwan K, de Seigneux S, Ponte B, et al. Dietary sodium intake does not alter renal potassium handling and blood pressure in healthy young males. Nephrol Dial Transplant (2022) 37(3):548–57. doi: 10.1093/ndt/gfaa381
Keywords: idiopathic hyperaldosteronism, low sodium diet, sodium, blood pressure, potassium, randomized controlled trial
Citation: Zhou L, Jiang Y, Zhang C, Su T, Jiang L, Zhou W, Zhong X, Wu L and Wang W (2023) Effects of a low-sodium diet in patients with idiopathic hyperaldosteronism: a randomized controlled trial. Front. Endocrinol. 14:1124479. doi: 10.3389/fendo.2023.1124479
Received: 15 December 2022; Accepted: 03 April 2023;
Published: 19 April 2023.
Edited by:
Valentina Morelli, Istituto Auxologico Italiano, ItalyReviewed by:
Carmen Aresta, Italian Auxological Institute (IRCCS), ItalyJinbo Hu, First Affiliated Hospital of Chongqing Medical University, China
Copyright © 2023 Zhou, Jiang, Zhang, Su, Jiang, Zhou, Zhong, Wu and Wang. This is an open-access article distributed under the terms of the Creative Commons Attribution License (CC BY). The use, distribution or reproduction in other forums is permitted, provided the original author(s) and the copyright owner(s) are credited and that the original publication in this journal is cited, in accordance with accepted academic practice. No use, distribution or reproduction is permitted which does not comply with these terms.
*Correspondence: Weiqing Wang, d3Fpbmd3QHNoc211LmVkdS5jbg==
†These authors have contributed equally to this work and share first authorship