- Laboratory of Experimental Endocrinology, Department of Pharmacology, Escola Paulista de Medicina (EPM), Universidade Federal de São Paulo (UNIFESP), São Paulo, SP, Brazil
Prostate cancer remains the most prevalent cancer among men worldwide. This cancer is hormone-dependent; therefore, androgen, estrogen, and their receptors play an important role in development and progression of this disease, and in emergence of the castration-resistant prostate cancer (CRPC). Galectins are a family of β-galactoside-binding proteins which are frequently altered (upregulated or downregulated) in a wide range of tumors, participating in different stages of tumor development and progression, but the molecular mechanisms which regulate its expression are still poorly understood. This review provides an overview of the current and emerging knowledge on Galectin-3 in cancer biology with focus on prostate cancer and the interplay with estrogen receptor (ER) signaling pathways, present in androgen-independent prostate cancer cells. We suggest a molecular mechanism where ER, Galectin-3 and β-catenin can modulate nuclear transcriptional events, such as, proliferation, migration, invasion, and anchorage-independent growth of androgen-independent prostate cancer cells. Despite a number of achievements in targeted therapy for prostate cancer, CRPC may eventually develop, therefore new effective drug targets need urgently to be found. Further understanding of the role of Galectin-3 and ER in prostate cancer will enhance our understanding of the molecular mechanisms of prostate cancer development and the future treatment of this disease.
1. Introduction
According to World Health Organization statistics, about 1.41 million new cases of prostate cancer were detected in 2020 (1). The androgen receptor (AR) is the main factor in the pathogenesis of this disease (reviewed by 2), and most patients benefit from androgen deprivation therapy, but disease recurrence and the emergence of castration-resistant prostate cancer (CRPC) are frequent after this treatment (reviewed by 3). Treatments for CRPC, that prolong the life of the patient, have emerged, including AR pathway inhibitors, radioisotope therapy, systemic taxane chemotherapy, and cell immunotherapy. However, such therapeutic strategies are limited (reviewed by 4, 5). Thus, the high prevalence of these tumors, lack of effective biomarkers and limited effective treatment highlight the importance of basic research in this disease for further treatment. This review provides an overview of the current and emerging knowledge on Galectin-3 in cancer biology with focus on prostate cancer and the interplay with estrogen receptor (ER) signaling pathways, present in androgen-independent prostate cancer cells. It is important to mention that the promoter region of the human LGALS3 gene contains regulatory elements for several transcription factors (6; reviewed by 7) and ERs may interact with these transcription factors (reviewed by 8). ERs have both classical transcriptional nuclear properties and membrane-initiated rapid action that may function either separately as distinct pathways, or together as a fully integrated network (reviewed by 9). These molecular mechanisms induced by activation of ERs may be involved in the expression of the GAL-3 in androgen-independent prostate cancer cells.
2. Structural characteristics, nucleus and cytoplasmic shuttling and functions of the Galectin-3 in cancer
Galectin proteins are characterized by specific binding of β-galactosides through evolutionarily conserved sequence elements of the carbohydrate-recognition domain (CRD). Galectin family consists of 15 members, divided into three main groups: (i) prototype group (Galectin-1, -2, -5, -7, -10, -11, -13, -14, and -15); (ii) tandem repeat group (Galectin-4, -6, -8, -9, and -12); (iii) and chimera type (Galectin-3) (reviewed by 7).
The human 29- to 35-kDa protein Galectin-3 is encoded by the LGALS3 gene (10) and this protein has three distinct structural motifs: (i) a short N-terminal domain containing a serine phosphorylation site; (ii) a repetitive proline-rich collagen-α-like sequence cleavable by matrix metalloproteases; and (iii) a globular C-terminal domain containing a carbohydrate-binding motif and an NWGR anti-death motif (reviewed by 7, 11).
The expression of the Galectin-3 is observed mainly in the cytoplasm, and also in the nucleus. This protein can be secreted by non-classical secretory pathways, by a mechanism that remains unclear, but a study has shown the involvement of exosomes (12). In addition, both the nucleus and cytoplasmic shuttling of the Galectin-3 has been reported (13, 14). The N-terminal domain contains a serine 6 phosphorylation site, which plays an important role in nuclear transport (reviewed by 7, 15, 16). The CRD region is important for localization of the Galectin-3 in cells, since both nuclear import sequences (NLS) and nuclear export sequences (NES) are present in this region (reviewed by 7, 14, 17). Galectin-3 is translocated to the nucleus through the importin-α/β, and the amino acid residue Arg (224) is essential for its active nuclear translocation and its molecular stability (15, 16). Galectin-3 carries a functional NES, recognized by the CRM1 exportin, being inhibited by Leptomycin B (18). The secreted Galectin-3 mediates cell migration, through the binding with galactose-containing glycoproteins in the cell surface. Cytoplasmic Galectin-3 exhibits anti-apoptotic activity and regulates several signal transduction pathways, whereas nuclear Galectin-3 has been associated with pre-mRNA splicing and gene expression (reviewed by 17, 19).
Galectin-3 is involved in many significant biological processes linked to cancer development and progression (20; reviewed by 21, 22; 19, 23). In addition, Galectin-3 exerts a role as a pro-tumor factor by acting within the tumor microenvironment to suppress immune surveillance (24).
The increase in the expression of the Galectin-3 is observed by RT-PCR or immunohistochemistry in different types of cancers, including breast, colon, gastric, hepatocellular, anaplastic large-cell lymphoma, head and neck squamous cell, tongue, non-small cell lung, and well differentiated thyroid (reviewed by 23, 25). Other studies showed a decrease in the expression of the Galectin-3 in breast, ovary, prostate cancers, advanced uterine adenocarcinoma, basal cell of the skin, epithelial skin, and malignant salivary gland neoplasms, compared to the corresponding normal tissue (reviewed by 23, 26).
3. Molecular regulatory mechanisms responsible for the expression of the Galectin-3 in cancer
Changes in the expression of the Galectin-3 are commonly seen in cancer and pre-cancerous conditions. However, the molecular regulatory mechanisms responsible for the level of expression of the Galectin-3 in tumor cells are not yet clear. Understanding these molecular mechanisms could contribute to the development of new approaches for cancer treatment.
DNA methylation in the gene promoter region is not the only factor regulating the expression of the Galectin-3 (27), since several features are involved in the protein regulation, in both transcriptional and translational levels (reviewed by 7). The levels of the Galectin-3 are not directly increased by a specific factor, but it would be dependent on the differentiation status of the cells or the type of the tissues.
Several features, for exemple runt-related protein (RUNX) family, homeodomain-interacting protein kinase 2 (HIPK2), nuclear factor kB (NF-kB), inflammation cytokines, and some intracellular signal pathways are involved in the regulation of the expression of the Galectin-3 and on cell growth, differentiation, proliferation, apoptosis, migration, angiogenesis, invasion, metastasis, and radiation resistance (reviewed by 7, 23).
Regarding these factors, two binding sites for RUNX1 and one binding site for RUNX2 were identified in the LGALS3 promoter region in human pituitary cell line HP75. Knockdown of either RUNX1 or RUNX2 resulted in downregulation of the Galectin-3, decreasing cell proliferation (28). HIPK2 is important for repression of the Galectin-3 upon induction of p53-dependent apoptosis (29).
NF-κB and Jun induced the expression of the Galectin-3 by UV-light in glioblastoma cells (30). Another study showed that Nucling, which is a stress-inducible protein associated with apoptosomes, inhibits the expression of the Galectin-3 by interfering with activation of the NF-kB (31).
Ras/mitogen-activated protein kinase (MAPK) kinase 1 (MEK1 or MKK1)- dependent/protein-1 activator (AP-1) signal transduction pathway plays an important role in the expression of the Galectin-3 in macrophages stimulated by Phorbol 12-myristate 13-acetate (PMA) (32).
Taken together, it is evident that the regulation of expression of the Galectin-3 is a complex, fine-tuned mechanism that involves numerous transcription factors and signaling pathways, and which depends on cell type, external stimuli and environmental conditions.
4. Expression of the Galectin-3 and prostate cancer
The studies in prostate cancer have mainly focused on Galectin-1 and Galectin-3, but the importance of Galectin-4, Galectin-7, Galectin-8, and Galectin-9 has also been highlighted (reviewed by 11, 33, 34). In relation to Galectin-3, different studies have indicated decrease of the expression over disease progression. In primary prostate cancer and metastatic disease, the expression of the Galectin-3 decreased compared to normal and premalignant tissue (35, 36). Normal prostate tissue showed heterogenous expression of the Galectin-3. In stage II tumors, however, a dramatic decrease in the expression of the Galectin-3 in both PIN and tumor sections was detected, with only 10.5% of these samples expressing this protein (37). In prostate cancer and adjacent non-tumoral tissue, the expression of the Galectin-3 was also examined by van den Brûle et al. (38). They found that most of the non-tumoral tissue exhibited moderate immunostaining for Galectin-3 localized in both nucleus and cytoplasm and prostate cancer cells showed decrease of the expression of the Galectin-3 or not expression compared to the normal tissue. In the hormone-sensitive prostate cancer tissue when compared to the respective benign tissue either localized far distant from the malignant lesion or directly neighboring the primary tumor, the expression of the Galectin-3 was significantly decreased in the prostate cancer tissue (39). The cellular localization of the Galectin-3 was shown in benign, adjacent-benign and tumor tissues. Median Galectin-3 staining scores significantly decreased from benign to adjacent-benign and to tumor tissues (40).
In addition, the expression of the Galectin-3 was also investigated in normal, BPH, and various stages of the prostate cancer which showed decreasing immunopositivity during stage evolution. Galectin-3 was found strongly expressed both in nucleus and cytoplasm in normal, BPH, and HGPIN, a precursor lesion to development of invasive prostatic adenocarcinoma tissues. Moreover, localization of the Galectin-3 seems to vary during tumor stage evolution. In particular, stage I tumors showed a strong immunopositivity in both the nucleus and the cytoplasm, whereas in more advanced stages, immunostaining was less intense and localized mainly in cytoplasm, with rare, occasional nucleus positivity (41).
On the other hand, recent study using biopsy samples, representing different stages of the primary prostate cancer, showed that prostate specific membrane antigen (PSMA), Galectin-1 and Galectin-3 are the most abundantly expressed glycoproteins. Galectin-3 correlated with the expression of PSMA, independently of PSA and Gleason score at diagnosis (42). The level of the Galectin-3 in the serum increased in metastatic prostate cancer (43) or decreased in prostatic adenocarcinomas (44) compared to healthy individuals. A prospective clinical study analyzed Galectin-3 and prostate specific antigen (PSA) (and their respective autoantibodies) levels in the serum and described positive associations between Galectin-3 and PSA levels in 76 men with different stages of prostate cancer and in 19 healthy control individuals (45, 46).
Galectin-3, in addition to different oligomeric forms, can to be cleaved by matrix metalloproteinases (MMP)-2/-9. In mouse models of breast and prostate cancers, this cleavage is associated with angiogenesis, tumor growth, and resistance to apoptosis (47).
Emerging studies are associating the expression of the Galectin-3 with the immune response against prostate cancer, as well as to the success of effective immunotherapy. Tiraboschi et al. (48) showed that when Galectin-3 is expressed by prostate tumor cells, it can control the tumor growth and decrease the number of tumor infiltrated T cells, suggesting that this protein is the principal immunological checkpoint responsible of the failure of immunotherapy in advanced prostate cancer. In addition, low doses of docetaxel inhibited the expression of the Galectin-3 in prostate cancer cells as well as in clinical samples of patients with metastatic cancer and CRPC, controlling tumor recurrence by increasing proliferation and infiltration of CD8+ cytotoxic T (49).
Further investigation is important to elucidate the relationship between the expression of the Galectin-3 and its regulation, cleavage and function in different stages of prostate cancer and CRPC.
Our laboratory and other groups have shown the expression of the Galectin-3 in androgen-independent prostate cancer cells PC-3 (derived from bone metastasis) and DU-145 (derived from brain metastasis), used in vitro and in xenograft implants, as a CRPC models (50–54). The expression of the Galectin-3 is higher in DU-145 cells and human post pubertal prostate epithelial cells (PNT1A) than in PC-3 cells (54). On the other hand, the androgen-dependent prostate cancer cell LNCaP do not express Galectin-3 (51, 52). Furthermore, neither overexpression of the Galectin-3 in LNCaP cells (LNCaP-GAL-3 cells) (51, 52) nor knockdown of the Galectin-3 in PC-3 cells change the expression of AR (51); similarly, overexpression of the AR in PC-3 cells does not have regulatory effect on the expression of the Galectin-3 (51). Taken together, these studies indicated that AR and Galectin-3 are not involved in the regulation of each other’s protein expression. Other hormones and their receptors, such as ER, and growth factors present in tumor microenvironment may be involved in the regulation of the expression of the Galectin-3.
LNCaP-GAL-3 cells promote both cell migration and invasion in an androgen-independent manner compared to control LNCaP cells and the transcriptional activity of the AR with treatment with dihydrotestosterone is enhanced in these cells (52). Furthermore, several AR-target genes, such as kallikrein related peptidase 3 (KLK3), and transmembrane protease, serine 2 (TMPRSS2) are increased (52). These AR-target genes in LNCaP-GAL-3 are not fully inhibited by anti-androgen, such as bicalutamide or MDV3100, whereas their expression in LNCaP cells is completely inhibited by anti-androgen, suggesting that Galectin-3 may be involved in resistance to anti-androgen (52). Galectin-3 also enhances anchorage-independent growth and xenograft tumor growth even after castration (52).
Knockdown of the Galectin-3 by siRNA reduced cell migration, invasion, cell proliferation, anchorage-independent colony formation of the PC-3 cells (50), and impaired tumor growth (50, 52). Inhibition of the Galectin-3 with pharmacological strategies impaired angiogenesis and metastasis (55; reviewed by 11). Furthermore, Galectin-3 can inhibit apoptosis in prostate cancer cells (51, 56).
Taken together, these results demonstrated that the levels and the cellular location of the Galectin-3 vary during prostate cancer progession, since the malignant transformation of prostate cells is associated with cellular redistribution of the Galectin-3 and a decrease in tissue levels of this protein (57). Functional studies using prostate cancer cell lines suggest that the expression of the Galectin-3 is not regulated by AR, but other hormones and their receptors, such as ER, could be involved in the regulation of the expression of the Galectin-3.
5. Molecular regulatory mechanisms responsible for the expression of the Galectin-3 induced by estrogen signaling in androgen-independent prostate cancer cells
17β-estradiol (E2) impacts normal and malignant tissue development via estrogen receptors ERα (ESR1) and ERβ (ESR2), either through ligand-activated transcriptional regulation (genomic pathway) or by triggering cytoplasmic-signaling cascades (rapid action or nongenomic pathway). The possible convergence of genomic and rapid pathways on target genes is an attractive mechanism by which ER can finely regulate the gene expression in different cells (reviewed by 9, 58).
ER, in addition to AR, plays an important biological function as a transcription factor and regulatory protein in prostate cancer (reviewed by 59–62). The traditional paradigm regarding the roles of the two ERs in the prostate is that ERα is oncogenic and promotes cell proliferation and survival, whereas ERβ is predominantly protective, being anti-carcinogenic and pro-apoptotic (reviewed by 59, 62). However, increasing evidences have shown that ERβ may be potentially oncogenic in prostate cancer (reviewed by 59). A variety of factors contribute to the uncertainties surrounding molecular action and tissue expression of ERs. Some antibodies used for ERβ are not specific and/or inadequately validated; the immunohistochemical analyses of estrogen receptors rely on cell permeabilization and reagent specificity, as well as changes in results have been shown depending on tissue fixation and processing, including antigen retrieval methods (reviewed by 63–65). The presence of ERβ splice variants in human tissues and cell lines, that have modified C-terminal and will not be recognized by antibodies raised against the C-terminal peptide of ERβ. Therefore, antibodies raised against the N-terminal of ERβ are important because they evaluate ERβ1 and all of its splice variants, but it is important that the epitopes should not include threonine, serine or tyrosine residues which are phosphorylated (reviewed by 65). A further problem is the passage of the acquired cell line, its time in culture and the presence of Mycoplasma. All cell lines need to be authenticated by DNA profiling, and contamination by Mycoplasma and other microorganisms excluded (66).
Our group has shown the presence of ERα and ERβ by Western blot and immunofluorescence analyses in androgen-independent prostate cancer cells PC-3 and DU-145 and in human post pubertal prostate epithelial cells PNT1A, using different antibodies for ERα and ERβ (67, 68), previously validated (64) and also positive control cells for these receptors (67, 69). On the other hand, ERβ was not observed in PC-3 and DU-145 cells (70) and also in human prostate (71). One of the problems in these studies may be the use of N-terminal antibodies, as previously reported (reviewed by 65) and/or different sensitivity of antibodies used depending on the expression level of ERβ, as shown in male reproductive organs (reviewed by 72). Furthermore, immortalized cell lines may have variable expression of certain factors across passage numbers and laboratories (66). We suggest that the data must be interpreted carefully in relation to the expression of ERβ and immunohistochemical and Western blot studies need to be supplemented with other methods.
In androgen-independent prostate cancer cells PC-3 and DU-145, ERα and ERβ are mostly located outside the cell nucleus (67, 68), while in human post pubertal prostate epithelial cells PNT1A (68) and in positive control cells (primary Sertoli cells and human testicular embryonal carcinoma NT2/D1 cells; 67, 69), these receptors are predominantly located in the nucleus, using these same antibody. The activation of ERα and ERβ increases the phosphorylation of extracellular signal-regulated kinase1 and 2 (ERK1/2) in PC-3 and DU-145 cells (67, 68) (illustration in Figures 1A, B) and non-receptor tyrosine kinase (SRC) in PC-3 cells (76) (illustration in Figure 1A). Furthermore, activation of ERβ in PC-3 cells increases the phosphorylation of serine/threonine kinases (AKT) (73). The activation of the complex ERα/SRC or ERβ/SRC increases the expression of the active non-phosphorylated β-catenin and vascular endothelial growth factor (VEGF) (75) (illustration in Figure 1A). Furthermore, the activation of ERβ increases the migration, invasion, and anchorage-independent growth of PC-3 cells (75). The activation of ERα also increases the invasion and anchorage-independent growth of these cells (75, 77) (illustration in Figure 1A). These effects are blocked by pretreatment with PKF 118–310, a compound that disrupts the complex β-catenin/TCF/LEF, suggesting that ERs/β-catenin are involved in all cellular characteristics of tumor development in vitro (75). All together, these results support an oncogenic role for ERα and ERβ in PC-3 cells.
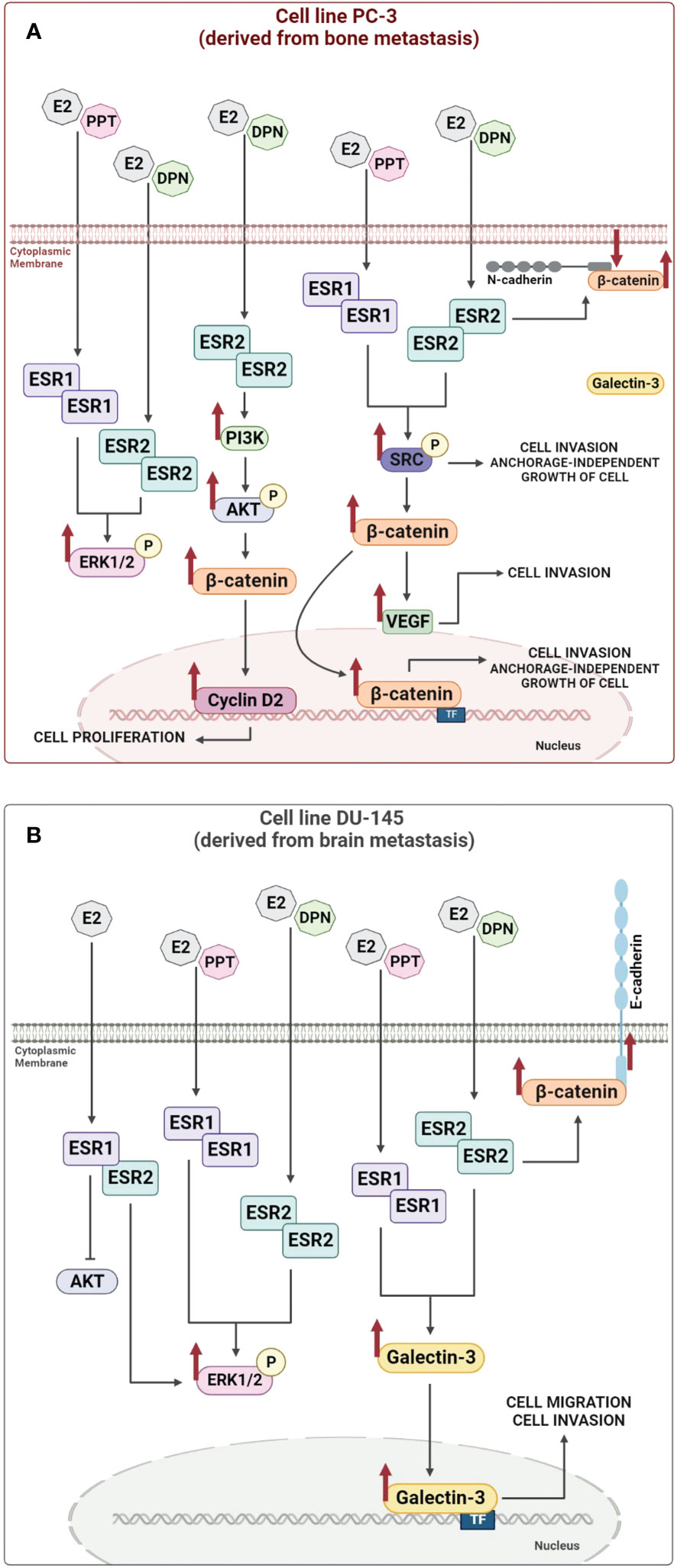
Figure 1 Illustration of the molecular regulatory mechanisms responsible for the expression of the non-phosphorylated β-catenin and Galectin-3 induced by estrogen receptor activation. Activation of estrogen receptors ESR1 and ESR2 by E2, PPT or DPN increases (arrows) the phosphorylation of SRC, ERK1/2 or AKT and the expression of non-phosphorylated β-catenin, Cyclin D2, VEGF and Galectin-3, which in turn can modulate nuclear transcriptional events, such as, proliferation, migration, invasion, and anchorage-independent growth of androgen-independent prostate cancer cells PC-3 (A) and DU-145 (B) (see 54, 67, 68, 73–76 for more information). TF (transcription factor).
In DU-145 cells, in addition to the presence of ERα and ERβ, formation of ERα/β heterodimers is observed and the activation of ERK1/2, but not AKT, by these receptors (68) (illustration in Figure 1B). The treatment with E2, ERα-selective agonist PPT or ERβ-selective agonist DPN for 24h increases the expression of the Galectin-3 compared to untreated DU-145 cells (control) (54) (illustration in Figure 1B). The activation of ERβ also increases the expression of β-catenin in the cellular membrane of DU-145 cells (74) (illustration in Figure 1B).
It is important to mention that the promoter region of the human LGALS3 gene contains binding sites for specificity protein 1 (Sp1), cAMP response element binding protein (CREB), AP-1, NF-κB and sis-inducible element (SIE) (6; reviewed by 7) and several of these transcription factors interact with ERs (reviewed by 8) and may play a role on the expression of the Galectin-3. ERs also activate two major pathways regulating cell proliferation and survival, SRC/MAPK and PI3K/AKT pathways (rapid or non-genomic signaling) (reviewed by 9, 78). Indeed, in DU-145 cells, the activation of ERα and ERβ increases the phosphorylation of ERK1/2, but not of AKT (68). Thus, direct activation of signaling cascades (non-genomic activity) by ERs combined with the transcriptional regulation (genomic activity) may be involved in the expression of the Galectin-3 in DU-145 cells. The direct transcriptional regulation remains to be explored in these cells. Furthermore, the activation of ERα and ERβ increases the migration and invasion of the DU-145 cells. These processes are inhibited by VA03 (specific inhibitor of Galectin-3), indicating the involvement of the complex ERα- ERβ/Galectin-3 (54).
In summary, ERs can mediate the rapid E2 actions in PC-3 (illustration in Figure 1A) and DU-145 cells (illustration in Figure 1B) and, respectively, increase the expression of non-phosphorylated β-catenin and Galectin-3, which in turn can modulate nuclear transcriptional events, such as, proliferation, migration, invasion, and anchorage-independent growth of these cells (illustration in Figure 1), indicating the oncogenic role of ERα and ERβ in both cells.
The activation of ERβ by selective-agonist DPN also promoted survival and migration of the CPEC cell line (cells expressing prostate-specific antigens), established from prostate cancer patients (79). In LNCaP cells (androgen-dependent prostate cancer cell), ERβ was able to drive the cells into S-phase and promote cell proliferation and epidermal growth factor secretion (80). In CRPC, ERβ plays a role in AR-dependent gene transcription (81), mediating the transition from hormone-sensitive to CRPC (82). The expression of ERβ is augmented in bone and lymph node metastases (83) and high expression of ER correlates with poor clinical prognosis (82, 84).
6. Conclusions and future perspectives
This review highlights the importance of Galectin-3 in the pathogenesis, diagnosis and treatment of prostate cancer. The activation of signaling cascades by membrane/cytoplasm-localized ERs is involved in the expression of Galectin-3 and non-phosphorylated β-catenin in androgen-independent prostate cancer cells. Nuclear ERs collaboration in this process still remains to be explored. ER, Galectin-3 and non-phosphorylated β-catenin can modulate nuclear transcriptional events, such as, proliferation, migration, invasion, and anchorage-independent growth of these cells.
It is important to mention that previous studies have shown that Galectin-3 binds to β-catenin/TCF complex, colocalizes in the nucleus, and induces the transcriptional activity of TCF-4. The β-catenin-Galectin-3-binding sequences were identified in the N-and C-termini of the proteins encompassing amino acid residues 1 to 131 and 143 to 250, respectively (85). In human colon cancer cells, Galectin-3 mediates AKT phosphorylation, thereby increasing phosphorylation of Glycogen synthase kinase-3β (GSK-3β) and decreases its activity and reduction in β-catenin degradation (86). β-Catenin can then translocate to the nucleus, bind to TCF4, and activate the transcription of its specific target genes (86). Thus, the presence of ER, Galectin-3 and non-phosphorylated β-catenin in androgen-independent prostate cancer cells shows a complex picture, which remains to be explored.
Several therapeutic avenues are emerging from the characterization of these signaling pathways discussed in this review. Investigators have made great progress in understanding how prostate cancer can disseminate early in the disease course and relapse months or decades later. Although clinical researchers have had modest gains thus far, much more work is needed to refine the basic science and translate this knowledge to the clinic.
Ethics statement
All experimental procedures were approved by the Research Ethical Committee at EPM-UNIFESP (#3527220917).
Author contributions
DSS: Conceived and designed the analysis; Collected the data; Performed the analysis; Wrote the manuscript. CM: Contributed with analysis tools. CMV: Contributed data or analysis tools. GJSP: Conceived and designed the analysis, performed the revision of the manuscript. CSP: Conceived and designed the analysis, performed the revision of the manuscript. All authors contributed to the article and approved the submitted version.
Funding
These studies were supported by Fundação de Amparo à Pesquisa do Estado de São Paulo (FAPESP, grant number 2020/01285-2 to C.S.P.)
Acknowledgments
We thank Elizabeth Kanashiro and Caroline Zito Romera for technical assistance. Confocal microscope Leica Microsystems TCSSP8, facility from the Instituto Nacional de Farmacologia e Biologia Molecular (INFAR), EPM-UNIFESP, was supported by Financiadora de Estudos e Projetos (FINEP) and Fundação de Amparo à Pesquisa do Estado de São Paulo (FAPESP). Research (C.S.P.) and Doctoral (C.M.) fellowships were supported by Conselho Nacional de Desenvolvimento Científico e Tecnológico (CNPq). Doctoral fellowship (D.S.S.) was supported by Coordenação de Aperfeiçoamento de Pessoal de Nível Superior (CAPES).
Conflict of interest
The authors declare that the research was conducted in the absence of any commercial or financial relationships that could be construed as a potential conflict of interest.
Publisher’s note
All claims expressed in this article are solely those of the authors and do not necessarily represent those of their affiliated organizations, or those of the publisher, the editors and the reviewers. Any product that may be evaluated in this article, or claim that may be made by its manufacturer, is not guaranteed or endorsed by the publisher.
References
1. World Health Organization. Estimated number of new cases in 2020. In: World, both sexes, all ages (2020). Available at: https://gco.iarc.fr/today/home.
2. Howard N, Clementino M, Kim D, Wang L, Verma A, Shi X, et al. New developments in mechanisms of prostate cancer progression. Semin Cancer Biol (2019) 57:111–6. doi: 10.1016/j.semcancer.2018.09.003
3. Baciarello G, Gizzi M, Fizazi K. Advancing therapies in metastatic castration-resistant prostate cancer. Expert Opin Pharmacother (2018) 19:1797–804. doi: 10.1080/14656566.2018.1527312
4. Teo MY, Rathkopf DE, Kantoff P. Treatment of advanced prostate cancer. Annu Rev Med (2019) 70:479–99. doi: 10.1146/annurev-med-051517-011947
5. Huang J, Lin B, Li B. Anti-androgen receptor therapies in prostate cancer: A brief update and perspective. Front Oncol (2022) 12:865350. doi: 10.3389/fonc.2022.865350. eCollection 2022.
6. Kadrofske MM, Openo KP, Wang JL. The human LGALS3 (galectin 3) gene: determination of the gene structure and functional characterization of the promoter. Arch Biochem Biophysics (1998) 349:7–20. doi: 10.1006/abbi.1997.0447
7. Dumic J, Dabelic S, Flögel M. Galectin-3: an open-ended story. Biochim Biophys Acta (2006) 1760:616–35. doi: 10.1016/j.bbagen.2005.12.020
8. Heldring N, Pike A, Andersson S, Matthews J, Cheng G, Hartman J, et al. Estrogen receptors: how do they signal and what are their targets. Physiol Rev (2007) 87:905–31. doi: 10.1152/physrev.00026.2006
9. Mauvais-Jarvis F, Lange CA, Levin ER. Membrane-initiated estrogen, androgen, and progesterone receptor signaling in health and disease. Endocrine Rev (2022) 43:720–42. doi: 10.1210/endrev/bnab041
10. Raimond J, Zimonjic DB, Mignon C, Mattei M, Popescu NC, Monsigny M, et al. Mapping of the galectin-3 gene (LGALS3) to human chromosome 14 at region 14q21-22. Mamm Genome: Off J Int Mamm Genome Soc (1997) 8(9):706–7. doi: 10.1007/s003359900548
11. Farhad M, Rolig AS, Redmond WL. The role of galectin-3 in modulating tumor growth and immunosuppression within the tumor microenvironment. Oncoimmunology (2018) 7:e1434467. doi: 10.1080/2162402X.2018.1434467
12. Inohara H, Raz A. Identification of human melanoma cellular and secreted ligands for galectin-3. Biochem Biophys Res Commun (1994) 201:1366–75. doi: 10.1006/bbrc.1994.1854
13. Davidson PJ, Davis MJ, Patterson RJ, Ripoche MA, Poirier F, Wang JL. Shuttling of galectin-3 between the nucleus and cytoplasm. Glycobiology (2002) 12:329–37. doi: 10.1093/glycob/12.5.329
14. Haudek KC, Spronk KJ, Voss PG, Patterson RJ, Wang JL, Arnoys EJ. Dynamics of galectin-3 in the nucleus and cytoplasm. Biochim Biophys Acta (2010) 1800:181–9. doi: 10.1016/j.bbagen.2009.07.005
15. Nakahara S, Hogan V, Inohara H, Raz A. Importin-mediated nuclear translocation of galectin-3. J Biol Chem (2006) 281:39649–59. doi: 10.1074/jbc.M608069200
16. Nakahara S, Oka N, Wang Y, Hogan V, Inohara H, Raz A. Characterization of the nuclear import pathways of galectin-3. Cancer Res (2006) 66:9995–10006. doi: 10.1158/0008-5472.CAN-06-1772
17. Kim SJ, Chun KH. Non-classical role of galectin-3 in cancer progression: translocation to nucleus by carbohydrate-recognition independent manner. BMB Rep (2020) 53:173–80. doi: 10.5483/BMBRep.2020.53.4.020
18. Li SY, Davidson PJ, Lin NY, Patterson RJ, Wang JL, Arnoys EJ. Transport of galectin-3 between the nucleus and cytoplasm. II Identification Signal Nucl export. Glycobiol (2006) 16:612–22. doi: 10.1093/glycob/cwj089
19. Funasaka T, Raz A, Nangia-Makker P. Nuclear transport of galectin-3 and its therapeutic implications. Semin Cancer Biol (2014) 27:30–8. doi: 10.1016/j.semcancer.2014.03.004
20. Dagher SF, Wang JL, Patterson RJ. Identification of galectin-3 as a factor in pre-mRNA splicing. Proc Natl Acad Sci United States America (1995) 92:1213–7. doi: 10.1073/pnas.92.4.1213
21. Califice S, Castronovo V, van Den Brûle F. Galectin-3 and cancer (Review). Int J Oncol (2004) 25:983–92. doi: 10.3892/ijo.25.4.983
22. Liu FT, Rabinovich GA. Galectins as modulators of tumour progression. Nat Rev Cancer (2005) 5:29–41. doi: 10.1038/nrc1527
23. Wang L, Guo XL. Molecular regulation of galectin-3 expression and therapeutic implication in cancer progression. Biomed Pharmacother (2016) 78:165–71. doi: 10.1016/j.biopha.2016.01.014
24. Ruvolo PP. Galectin 3 as a guardian of the tumor microenvironment. Biochim Biophys Acta (2016) 1863:427–37. doi: 10.1016/j.bbamcr.2015.08.008
25. Ebrahim AH, Alalawi Z, Mirandola L, Rakhshanda R, Dahlbeck S, Nguyen D, et al. Galectins in cancer: carcinogenesis, diagnosis and therapy. Ann Trans Med (2014) 2:88. doi: 10.3978/j.issn.2305-5839.2014.09.12
26. Sciacchitano S, Lavra L, Morgante A, Ulivieri A, Magi F, De Francesco GP, et al. Galectin-3: One molecule for an alphabet of diseases, from a to z. Int J Mol Sci (2018) 19:379. doi: 10.3390/ijms19020379
27. Ruebel KH, Jin L, Qian X, Scheithauer BW, Kovacs K, Nakamura N, et al. Effects of DNA methylation on galectin-3 expression in pituitary tumors. Cancer Res (2005) 65:1136–40. doi: 10.1158/0008-5472.CAN-04-3578
28. Zhang HY, Jin L, Stilling GA, Ruebel KH, Coonse K, Tanizaki Y, et al. RUNX1 and RUNX2 upregulate galectin-3 expression in human pituitary tumors. Endocrine (2009) 35:101–11. doi: 10.1007/s12020-008-9129-z
29. Cecchinelli B, Lavra L, Rinaldo C, Iacovelli S, Gurtner A, Gasbarri A, et al. Repression of the antiapoptotic molecule galectin-3 by homeodomain-interacting protein kinase 2-activated p53 is required for p53-induced apoptosis. Mol Cell Biol (2006) 26:4746–57. doi: 10.1128/MCB.00959-05
30. Dumic J, Lauc G, Flögel M. Expression of galectin-3 in cells exposed to stress-roles of jun and NF-kappaB. Cell Physiol Biochem: Int J Exp Cell Physiology Biochem Pharmacol (2000) 10:149–58. doi: 10.1159/000016345
31. Liu L, Sakai T, Sano N, Fukui K. Nucling mediates apoptosis by inhibiting expression of galectin-3 through interference with nuclear factor kappaB signalling. Biochem J (2004) 380(Pt 1):31–41. doi: 10.1042/BJ20031300
32. Kim K, Mayer EP, Nachtigal M. Galectin-3 expression in macrophages is signaled by Ras/MAP kinase pathway and up-regulated by modified lipoproteins. Biochim Biophys Acta (2003) 1641:13–23. doi: 10.1016/s0167-4889(03)00045-4
33. Compagno D, Gentilini LD, Jaworski FM, Pérez IG, Contrufo G, Laderach DJ. Glycans and galectins in prostate cancer biology, angiogenesis and metastasis. Glycobiology (2014) 24:899–906. doi: 10.1093/glycob/cwu055
34. Martínez-Bosch N, Rodriguez-Vida A, Juanpere N, Lloreta J, Rovira A, Albanell J, et al. Galectins in prostate and bladder cancer: tumorigenic roles and clinical opportunities. Nat Rev Urol (2019) 16:433–45. doi: 10.1038/s41585-019-0183-5
35. Ellerhorst J, Troncoso P, Xu XC, Lee J, Lotan R. Galectin-1 and galectin-3 expression in human prostate tissue and prostate cancer. Urological Res (1999) 27:362–7. doi: 10.1007/s002400050164
36. Li QK, Lih T-SM, Wang Y, Hu Y, Höti N, Chan DW, et al. Improving the detection of aggressive prostate cancer using immunohistochemical staining of protein marker panels. Am J Cancer Res (2022) 12:1323–36.
37. Pacis RA, Pilat MJ, Pienta KJ, Wojno K, Raz A, Hogan V, et al. Decreased galectin-3 expression in prostate cancer. Prostate (2000) 44:118–23. doi: 10.1002/1097-0045(20000701)44:2<118::aid-pros4>3.0.co;2-u
38. van den Brûle FA, Waltregny D, Liu FT, Castronovo V. Alteration of the cytoplasmic/nuclear expression pattern of galectin-3 correlates with prostate carcinoma progression. Int J Cancer (2000) 89:361–7. doi: 10.1002/1097-0215(20000720)89:4<361::aid-ijc8>3.0.co;2-u
39. Merseburger AS, Kramer MW, Hennenlotter J, Simon P, Knapp J, Hartmann JT, et al. Involvement of decreased galectin-3 expression in the pathogenesis and progression of prostate cancer. Prostate (2008) 68:72–7. doi: 10.1002/pros.20688
40. Knapp JS, Lokeshwar SD, Vogel U, Hennenlotter J, Schwentner C, Kramer MW, et al. Galectin-3 expression in prostate cancer and benign prostate tissues: correlation with biochemical recurrence. World J Urol (2013) 31:351–8. doi: 10.1007/s00345-012-0925-y
41. Ahmed H, Cappello F, Rodolico V, Vasta GR. Evidence of heavy methylation in the galectin 3 promoter in early stages of prostate adenocarcinoma: development and validation of a methylated marker for early diagnosis of prostate cancer. Trans Oncol (2009) 2:146–56. doi: 10.1593/tlo.09118
42. Sharma S, Cwiklinski K, Sykes DE, Mahajan SD, Chevli K, Schwartz SA, et al. Use of glycoproteins-Prostate-Specific membrane antigen and galectin-3 as primary tumor markers and therapeutic targets in the management of metastatic prostate cancer. Cancers (2022) 14:2704. doi: 10.3390/cancers14112704
43. Balan V, Wang Y, Nangia-Makker P, Kho D, Bajaj M, Smith D, et al. Galectin-3: a possible complementary marker to the PSA blood test. Oncotarget (2013) 4:542–9. doi: 10.18632/oncotarget.923
44. Geisler C, Gaisa NT, Pfister D, Fuessel S, Kristiansen G, Braunschweig T, et al. Identification and validation of potential new biomarkers for prostate cancer diagnosis and prognosis using 2D-DIGE and MS. BioMed Res Int (2015) 2015:454256. doi: 10.1155/2015/454256
45. Nakajima K, Heilbrun LK, Hogan V, Smith D, Heath E, Raz A. Positive associations between galectin-3 and PSA levels in prostate cancer patients: a prospective clinical study-I. Oncotarget (2016) 7:82266–72. doi: 10.18632/oncotarget.12619
46. Nakajima K, Heilbrun LK, Smith D, Hogan V, Raz A, Heath E. The influence of PSA autoantibodies in prostate cancer patients: a prospective clinical study-II. Oncotarget (2017) 8:17643–50. doi: 10.18632/oncotarget.12620
47. Nangia-Makker P, Wang Y, Raz T, Tait L, Balan V, Hogan V, et al. Cleavage of galectin-3 by matrix metalloproteases induces angiogenesis in breast cancer. Int J Cancer (2010) 127:2530–41. doi: 10.1002/ijc.25254
48. Tiraboschi C, Gentilini L, Jaworski FM, Corapi E, Velazquez C, Chauchereau A, et al. Galectin-3 as a new negative checkpoint of the immune response is the key target for effective immunotherapy against prostate cancer. BioRxiv preprint (2019) 8(2):e001535. doi: 10.1101/763409
49. Tiraboschi C, Gentilini L, Velazquez C, Corapi E, Jaworski FM, Garcia Garcia JD, et al. Combining inhibition of galectin-3 with and before a therapeutic vaccination is critical for the prostate-tumor-free outcome. J Immunother Cancer (2020) 8(2):e001535. doi: 10.1136/jitc-2020-001535
50. Wang Y, Nangia-Makker P, Tait L, Balan V, Hogan V, Pienta KJ, et al. Regulation of prostate cancer progression by galectin-3. Am J Pathol (2009) 174:1515–23. doi: 10.2353/ajpath.2009.080816
51. Wang Y, Balan V, Gao X, Reddy PG, Kho D, Tait L, et al. The significance of galectin-3 as a new basal cell marker in prostate cancer. Cell Death Dis (2013) 4:e753. doi: 10.1038/cddis.2013.277
52. Dondoo TO, Fukumori T, Daizumoto K, Fukawa T, Kohzuki M, Kowada M, et al. Galectin-3 is implicated in tumor progression and resistance to anti-androgen drug through regulation of androgen receptor signaling in prostate cancer. Anticancer Res (2017) 37:125–34. doi: 10.21873/anticanres.11297
53. Yuan X, Liu L, Wang W, Gao Y, Zhang D, Jia T-T, et al. Development of (G3-C12)-mediated camptothecin polymeric prodrug targeting to galectin-3 receptor against androgen-independent prostate cancer. Int J Pharmaceutics (2020) 580:119123. doi: 10.1016/j.ijpharm.2020.119123
54. Souza DS. Estrogen receptors regulate the expression and function of β-catenin and galectin-3 in androgen-independent prostate cancer cells DU-145. In: [doctoral’s thesis]. São Paulo (SP: Universidade Federal São Paulo (2022).
55. Guha P, Kaptan E, Bandyopadhyaya G, Kaczanowska S, Davila E, Thompson K, et al. Cod glycopeptide with picomolar affinity to galectin-3 suppresses T-cell apoptosis and prostate cancer metastasis. Proc Natl Acad Sci United States America (2013) 110:5052–7. doi: 10.1073/pnas.1202653110
56. Fukumori T, Oka N, Takenaka Y, Nangia-Makker P, Elsamman E, Kasai T, et al. Galectin-3 regulates mitochondrial stability and antiapoptotic function in response to anticancer drug in prostate cancer. Cancer Res (2006) 66:3114–9. doi: 10.1158/0008-5472.CAN-05-3750
57. Lima T, Perpétuo L, Henrique R. Galectin-3 in prostate cancer and heart diseases: a biomarker for these two frightening pathologies? Mol Biol Rep (2022). doi: 10.1007/s11033-022-08207-1
58. Acconcia F, Marino M. The effects of 17β-estradiol in cancer are mediated by estrogen receptor signaling at the plasma membrane. Front Physiol (2011) 2:30. doi: 10.3389/fphys.2011.00030
59. Nelson AW, Tilley WD, Neal DE, Carroll JS. Estrogen receptor beta in prostate cancer: friend or foe? Endocrine-Related Cancer (2014) 21:T219–34. doi: 10.1530/ERC-13-0508
60. Bonkhoff H. Estrogen receptor signaling in prostate cancer: Implications for carcinogenesis and tumor progression. Prostate (2018) 78:2–10. doi: 10.1002/pros.23446
61. Di Zazzo E, Galasso G, Giovannelli P, Di Donato M, Castoria G. Estrogens and their receptors in prostate cancer: Therapeutic implications. Front Oncol (2018) 8:2. doi: 10.3389/fonc.2018.00002
62. Li J, Liu Q, Jiang C. Signal crosstalk and the role of estrogen receptor beta (ERβ) in prostate cancer. Med Sci Monitor: Int Med J Exp Clin Res (2022) 28:e935599. doi: 10.12659/MSM.935599
63. Cooke PS, Nanjappa MK, Ko C, Prins GS, Hess RA. Estrogens in Male physiology. Physiol Rev (2017) 97:995–1043. doi: 10.1152/physrev.00018.2016
64. Nelson AW, Groen AJ, Miller JL, Warren AY, Holmes KA, Tarulli GA, et al. Comprehensive assessment of estrogen receptor beta antibodies in cancer cell line models and tissue reveals critical limitations in reagent specificity. Mol Cell Endocrinol (2017) 440:138–50. doi: 10.1016/j.mce.2016.11.016
65. Gustafsson JA, Strom A, Warner M. Update on ERbeta. J Steroid Biochem Mol Biol (2019) 191:105312. doi: 10.1016/j.jsbmb.2019.02.007
66. Masters JR. Human cancer cell lines: fact and fantasy. Nat Rev Mol Cell Biol (2000) 1:233e236. doi: 10.1038/35043102
67. Pisolato R, Lombardi AP, Vicente CM, Lucas TF, Lazari MF, Porto CS. Expression and regulation of the estrogen receptors in PC-3 human prostate cancer cells. Steroids (2016) 107:74–86. doi: 10.1016/j.steroids.2015.12.021
68. Souza DS, Lombardi APG, Vicente CM, Lucas TFG, Erustes AG, Pereira GJS, et al. Estrogen receptors localization and signaling pathways in DU-145 human prostate cancer cells. Mol Cell Endocrinol (2019) 483:11–23. doi: 10.1016/j.mce.2018.12.015
69. Macheroni C, Gameiro Lucas TF, Souza DS, Vicente CM, Pereira GJDS, Junior IDSV, et al. Activation of estrogen receptor ESR1 and ESR2 induces proliferation of the human testicular embryonal carcinoma NT2/D1 cells. Mol Cell Endocrinol (2022) 554:111708. doi: 10.1016/j.mce.2022.111708
70. Lafront C, Germain L, Weidmann C, Audet-Walsh É. A systematic study of the impact of estrogens and selective estrogen receptor modulators on prostate cancer cell proliferation. Sci Rep (2020) 10(1):4024. doi: 10.1038/s41598-020-60844-3
71. Andersson S, Sundberg M, Pristovsek N, Ibrahim A, Jonsson P, Katona B, et al. Insufficient antibody validation challenges oestrogen receptor beta research. Nat Commun (2017) 8:15840. doi: 10.1038/ncomms15840
72. Hess RA, Cooke PS. Estrogen in the male: a historical perspective. Biol Reprod (2018) 99:27–44. doi: 10.1093/biolre/ioy043
73. Lombardi AP, Pisolato R, Vicente CM, Lazari MF, Lucas TF, Porto CS. Estrogen receptor beta (ERβ) mediates expression of β-catenin and proliferation in prostate cancer cell line PC-3. Mol Cell Endocrinol (2016) 430:12–24. doi: 10.1016/j.mce.2016.04.012
74. Silva RS, Lombardi APG, de Souza DS, Vicente CM, Porto CS. Activation of estrogen receptor beta (ERβ) regulates the expression of n-cadherin, e-cadherin and β-catenin in androgen-independent prostate cancer cells. Int J Biochem Cell Biol (2018) 96:40–50. doi: 10.1016/j.biocel.2018.01.008
75. Lombardi APG, Vicente CM, Porto CS. Estrogen receptors promote migration, invasion and colony formation of the androgen-independent prostate cancer cells PC-3 through β-catenin pathway. Front Endocrinol (2020) 11:184. doi: 10.3389/fendo.2020.00184
76. Lombardi APG, Cavalheiro RP, Porto CS, Vicente CM. Estrogen receptor signaling pathways involved in invasion and colony formation of androgen-independent prostate cancer cells PC-3. Int J Mol Sci (2021) 22:1153. doi: 10.3390/ijms22031153
77. Semenas J, Wang T, Sajid Syed Khaja A, Firoj Mahmud A, Simoulis A, Grundström T, et al. Targeted inhibition of ERα signaling and PIP5K1α/Akt pathways in castration-resistant prostate cancer. Mol Oncol (2021) 15:968–86. doi: 10.1002/1878-0261.12873
78. Thiebaut C, Vlaeminck-Guillem V, Trédan O, Poulard C, Le Romancer M. Non-genomic signaling of steroid receptors in cancer. Mol Cell Endocrinol (2021) 538:111453. doi: 10.1016/j.mce.2021.111453
79. Rossi V, Di Zazzo E, Galasso G, De Rosa C, Abbondanza C, Sinisi AA, et al. Estrogens modulate somatostatin receptors expression and synergize with the somatostatin analog pasireotide in prostate cells. Front Pharmacol (2019) 10:28. doi: 10.3389/fphar.2019.00028
80. Migliaccio A, Castoria G, Di Domenico M, de Falco A, Bilancio A, Lombardi M, et al. Steroid-induced androgen receptor-oestradiol receptor beta-src complex triggers prostate cancer cell proliferation. EMBO J (2000) 19:5406–17. doi: 10.1093/emboj/19.20.5406
81. Yang M, Wang J, Wang L, Shen C, Su B, Qi M, et al. Estrogen induces androgen repressed SOX4 expression to promote progression of prostate cancer cells. Prostate (2015) 75:1363–75. doi: 10.1002/pros.23017
82. Zellweger T, Stürm S, Rey S, Zlobec I, Gsponer JR, Rentsch CA, et al. Estrogen receptor β expression and androgen receptor phosphorylation correlate with a poor clinical outcome in hormone-naive prostate cancer and are elevated in castration-resistant disease. Endocrine-Related Cancer (2013) 20:403–13. doi: 10.1530/ERC-12-0402
83. Zhu X, Leav I, Leung YK, Wu M, Liu Q, Gao Y, et al. Dynamic regulation of estrogen receptor-beta expression by DNA methylation during prostate cancer development and metastasis. Am J Pathol (2004) 164:2003–12. doi: 10.1016/s0002-9440(10)63760-1
84. Horvath LG, Henshall SM, Lee CS, Head DR, Quinn DI, Makela S, et al. Frequent loss of estrogen receptor-beta expression in prostate cancer. Cancer Res (2001) 61:5331–5.
85. Shimura T, Takenaka Y, Tsutsumi S, Hogan V, Kikuchi A, Raz A. Galectin-3, a novel binding partner of beta-catenin. Cancer Res (2004) 64:6363–7. doi: 10.1158/0008-5472.CAN-04-1816
Keywords: Galectin-3, β-catenin, ERα, ERβ, prostate cancer cells
Citation: Souza DS, Macheroni C, Pereira GJS, Vicente CM and Porto CS (2023) Molecular regulation of prostate cancer by Galectin-3 and estrogen receptor. Front. Endocrinol. 14:1124111. doi: 10.3389/fendo.2023.1124111
Received: 14 December 2022; Accepted: 13 February 2023;
Published: 03 March 2023.
Edited by:
Roger Chammas, University of São Paulo, BrazilReviewed by:
Mauricio Rodriguez-Dorantes, National Institute of Genomic Medicine (INMEGEN), MexicoAmado A. Quintar, National University of Cordoba, Argentina
Etienne Audet-Walsh, Laval University, Canada
Copyright © 2023 Souza, Macheroni, Pereira, Vicente and Porto. This is an open-access article distributed under the terms of the Creative Commons Attribution License (CC BY). The use, distribution or reproduction in other forums is permitted, provided the original author(s) and the copyright owner(s) are credited and that the original publication in this journal is cited, in accordance with accepted academic practice. No use, distribution or reproduction is permitted which does not comply with these terms.
*Correspondence: Catarina Segreti Porto, Y3Nwb3J0b0B1bmlmZXNwLmJy; Y3NlZ3JldGlwb3J0b0BnbWFpbC5jb20=