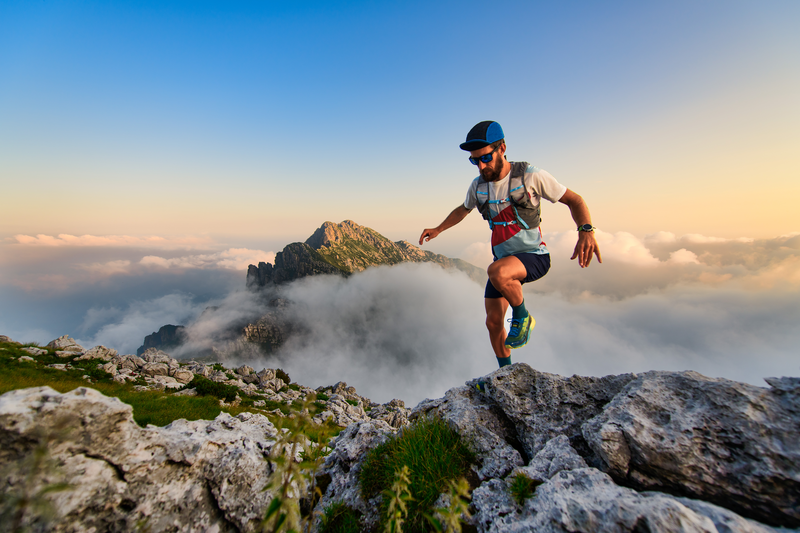
94% of researchers rate our articles as excellent or good
Learn more about the work of our research integrity team to safeguard the quality of each article we publish.
Find out more
REVIEW article
Front. Endocrinol. , 14 April 2023
Sec. Reproduction
Volume 14 - 2023 | https://doi.org/10.3389/fendo.2023.1110572
This article is part of the Research Topic Spermatogenesis: from Stem Cells to Spermatozoa View all 5 articles
Spermatogenesis is a multi-step process of male germ cell (Gc) division and differentiation which occurs in the seminiferous tubules of the testes under the regulation of gonadotropins – Follicle Stimulating Hormone (FSH) and Luteinising hormone (LH). It is a highly coordinated event regulated by the surrounding somatic testicular cells such as the Sertoli cells (Sc), Leydig cells (Lc), and Peritubular myoid cells (PTc). FSH targets Sc and supports the expansion and differentiation of pre-meiotic Gc, whereas, LH operates via Lc to produce Testosterone (T), the testicular androgen. T acts on all somatic cells e.g.- Lc, PTc and Sc, and promotes the blood-testis barrier (BTB) formation, completion of Gc meiosis, and spermiation. Studies with hypophysectomised or chemically ablated animal models and hypogonadal (hpg) mice supplemented with gonadotropins to genetically manipulated mouse models have revealed the selective and synergistic role(s) of hormones in regulating male fertility. We here have briefly summarized the present concept of hormonal control of spermatogenesis in rodents and primates. We also have highlighted some of the key critical questions yet to be answered in the field of male reproductive health which might have potential implications for infertility and contraceptive research in the future.
An alarming decline in the sperm count of men has become a global concern (1). Spermatogenesis occurs within testicular seminiferous tubules under the regulation of gonadotropins – Follicle Stimulating Hormone (FSH) and Luteinising hormone (LH) and involves regulated division and differentiation of male germ cells (Gc) to sperm (2). In mammals, it is a multi-step event that includes i) establishment of spermatogonial stem cells (SSC) ii) self-renewal and differentiation of SSC to form spermatogonial progenitor cells (SPC) iii) spermatogonial expansion and differentiation, iv) meiotic initiation of differentiated spermatogonia v) meiotic progression of spermatocytes to spermatids vi) maturation of spermatids to spermatozoa and vii) spermiation (3). This entire process is extremely rapid (around 35 days in mice, 52 days in rats, 46 days in rhesus macaque and 64 days in humans) with incredible intrinsic speed (1000 sperm/sec) (3).
The hypothalamo-hypophysial-testicular axis (HHT axis) is a three-tier neuro-endocrine circuit with hierarchical regulatory cascades (both stimulatory and inhibitory feedback loops) (4). Under the influence of hypothalamic KNDy (K= Kisspeptin, N= Neurokinin B and Dy = Dynorphin) neurons, specific nuclei located at mediobasal/preoptic/arcuate/infundibular area synthesize and release decapeptide GnRH in a pulsatile manner (5). The GnRH further stimulates pituitary-gonadotrophs to secrete gonadotropins (LH and FSH). The differential pulse frequency and amplitude of GnRH, selectively augments either LH or FSH (high and low frequencies favor LH and FSH respectively) release (5). LH acts on the interstitial Leydig cells (Lc) to produce the testicular androgen—testosterone (T) (6). Sertoli cells (Sc) are the major component of the seminiferous tubules that express the receptors for both FSH (FSH receptor, FSH-R) as well as T (androgen receptor, AR) and provide critical micro-environment for Gc nourishment and differentiation (6). Sc-produced inhibin and Lc-generated T selectively suppress the release of FSH from the pituitary and GnRH from the hypothalamus respectively (4–6).
Within twenty years of their identification (7), clinical cases of familial hypogonadism due to isolated gonadotropic deficiency started to get reported frequently (8, 9). In 1971, GnRH (previously known as LHRH) was purified and subsequently got recognized for the Nobel Prize in 1977 (10–12). The same year, a naturally occurring mutation in GnRH [termed as hypogonadal (hpg)] was reported in mice confirming the absolute necessity of gonadotropins in gonadal functions and gametogenesis (13). During the 1980s to mid-1990s classical endocrinological studies employed hypophysectomised or GnRH-depleted (either immunologically or pharmacologically) animal models supplemented with purified or recombinant gonadotropins (either alone or in combination) indicating the probable functions of FSH and LH (via T) in spermatogenesis (14–17). From the late 1990s, the success of genetically manipulated mouse models (both gain-in-function or knockout strategies) has further revealed the selective and synergistic role(s) of FSH and LH in regulating male fertility (18–21). This article briefly discusses the critical gonadotropic control of spermatogenesis. We further highlight currently unanswered areas in gonadotropin biology having potential implications on male infertility and contraceptive research.
We have prepared a PRISMA flow diagram (Figure 1) to systematically document the advancement of knowledge in the role of gonadotrophic hormones in the regulation of spermatogenesis in mammals. The flow chart is self-explanatory; in brief, we looked into the PubMed® database for papers dealing with the topic in hand in the last decade. We only included original research papers, whose full text is deposited in the said database and concerns studies performed only on mammalian species. Thus, we narrowed down the total number of cited articles to 64 from 752 with the help of imposed inclusion and exclusion criteria. However, to address the regulation of mammalian spermatogenesis by gonadotropins from a broader developmental perspective and for the benefit of general readers, we have cited a substantial number of additional scientific articles in this review paper. Figure 2 is the schematic representation of the HHT axis showing the site of sperm production. Figure 3 represents the developmental (from the fetal stage to adulthood) changes in plasma hormonal profiles of mice and men. Figure 4 displays a comparative picture of the initial critical steps in male germ cell differentiation in rodents, non-human primates, and humans.
Figure 1 PRISMA flow diagram of selection of articles published in last decade related to gonadotropic regulation of spermatogenesis in mammals.
Figure 2 Hormonal control of spermatogenesis by the hypothalamo-hypophysial-testicular axis through a three-tier neuro-endocrine circuit. Curved blue arrows indicate a renewal of the cells; solid and dotted colored arrows denote the primary action and feedback action of the hormones. A-R, androgen receptor; BTB, blood-testis barrier; FSH, follicle stimulating hormone; FSH-R, FSH receptor; LH, luteinizing hormone; LH-R, LH receptor; T, testosterone. Only one seminiferous tubule has been shown to contain the germ cells; for others, it has been intentionally not shown, only to keep the figure less complicated for viewing of the readers.
Figure 3 Changes in the endocrinal profiles in the course of the development of male gonads from the fetal stages to adulthood. (A, B): Comparison of gonadal cell numbers in rodents and humans. (C, D): Comparison of hormonal levels in rodents and humans. ALc, adult Leydig cell; AMH, anti-Mullerian hormone; FLc, fetal Leydig cell; FSH, follicle stimulating hormone; GnRH, gonadotropin-releasing hormone; LH, luteinizing hormone; NLc, neonatal Leydig cell; Sc, Sertoli cell; T, testosterone.
Figure 4 Comparison of stages of testicular development of the male germ cells among rodents, non-human primates, and humans. Note that the stem cell property differs between rodents and primates; the number of detectable stages of differentiation of the male germ cells varies significantly among all these three groups of animals. Colored curved arrows denote cell renewal; red question marks indicate unknown pathway.
FSH is a glycoprotein hormone having disulfide-rich heterodimers, a common α subunit (sharing with TSH and LH), and a unique β subunit. Evolving pieces of evidence suggest that pituitary-derived activins are the primary stimulators of FSH generation by gonadotrope cells. Activins control transcription of the FSH component gene (Fshβ) in vitro via SMAD3, SMAD4, and FOXL2 (22–25). FSH acts on Sc via FSH-R (Figure 2), a G protein-coupled receptor (GPCR), which transmits its signal by recruiting the intracellular GTP binding proteins (G-proteins, either stimulatory Gαs or inhibitory Gαi) associated with it (26). Dual coupling of Gαs or Gαi to FSH-R differentially modulates the activity of adenylyl cyclase (AC) to regulate FSH-induced cAMP production within Sc (26). The concentration of cAMP subsequently directs the multiple downstream signaling cascades such as canonical Protein Kinase A (PKA) or other (PKC, PI3K, Akt/PKB, and ERK1/ERK2) pathways highlighting the pleiotropic effects of FSH in Sc (26). The robust cAMP response in Sc results in the activation of PKA which in turn phosphorylates cAMP Response Element Binding protein (CREB) to induce the transcription of genes such as Stem cell factor (SCF), Glial cell line-derived neurotrophic factor (Gdnf), Androgen binding protein (Abp), Kruppel-like factor 4 (Klf4), Transferrin etc, that play a critical role in Gc differentiation (6, 26–30).
In rats, FSH-R is first detected at E14.5 [embryonic age in days (E)], whereas the fetal plasma FSH concentration rises from E 19.5- 21, peaks at P5 [post-natal age in days (P)], then substantially drops during P15-20, finally recovered to a steady state by P40-50 (31, 32); similar events occur in mice (Figures 3A, C). On the other hand, FSH is uniformly detectable in human fetal circulation from 12-18 week of gestation (WG), peaks during 20-22 WG and then gradually declines in term pregnancy (Figures 3B, D) (33, 34), whereas specific binding of FSH is observed in human and rhesus monkey (Macaca mulata) testes during 8–16 and 19–22 WG, respectively (35, 36). In post-natal life, FSH concentration first raises upto the adult range within a week of parturition and stays stable till 4-6 months, then declines and gets undetectable during the juvenile period prior to its re-elevation at puberty (4, 5). Although circulatory FSH levels remain relatively constant in adult men and rats (4, 5), the expression pattern of FSH-R cyclically changes in a stage-specific manner, maximal during stages XIII–II and minimal at VII–VIII (37). FSH has been shown to suppress FSH-R transcription at 6-8 hr (38) in cultured Sc and subsequently gets recovered by FSH at 24-48 hr (39).
In utero life, FSH has been shown to induce Sc proliferation and augments AMH (Anti Müllerian Hormone) production in both rodents (40) and primates (41) and this fetal expansion of the Sc population critically regulates the maximal spermatogenic output in adult testes (42–45). Such FSH-driven Sc proliferation gets continued in neonatal (upto P15) rats and infant primates (upto 3-6 months) and ceases with functional maturation of Sc during pubertal development (27–30). It is interesting to note here that unlike puberty, FSH induced cAMP production is limited during infancy in both rats (27, 28) and rhesus monkeys (29, 30) and therefore Sc fails to support robust Gc differentiation at younger ages despite being exposed to sufficiently high levels of FSH and FSH-R (27–29). Unlike pubertal cells, diminished plasma membrane localization of FSH-R protein in rats (27) and limited expression of Gαs protein in monkeys are considered to be the underlie causes of such poor cAMP response by FSH in infant Sc (29).
In hypophysectomised or GnRH depleted (via pharmacological or immunological inhibition) rats, administrations of FSH alone show partial spermatogenic restoration (46, 47). For example, FSH replacement in GnRH antagonist-treated rats significantly rescues spermatogonia B and early spermatocytes (48). Immuno-neutralization of FSH in post-natal rats indicates FSH promotes Sc proliferation and Gc survival in neonatal age, whereas pre-meiotic Gc differentiation in pubertal age (49). Exogenous administration of FSH alone in pre-pubertal hpg mice fails to induce sperm production (50). Similarly, pituitary independent transgenic expression of human (h) FSH (51) or mutated [at Asp567Gly and constitutively active (capable of FSH independent cAMP production)] h-FSH-R (h-FSH-R*) (52) in male hpg mouse leads to incomplete meiotic progression. Furthermore, although h-FSH-R* over-expression augments proliferation/development of Sc/pre or early meiotic Gc in wild-type testes (53) this hyper-active receptor fails to maintain normal spermatogenesis during experimental deprivation of gonadotropins (54). However, over-expression of h-FSH-R* shows LH-independent steroidogenic activity (55). Notably, over-expression of FSH-Rs [either h-FSH-R* (along with normal h-FSH-R) or another hyper-mutated (at Asp-580-His, constitutively active (capable of FSH independent cAMP productive) mouse (m) FSH-R (m-FSH-R*)] do not affect normal spermatogenic maintenance (55). Finally, both FSH or FSH-R Knock-out (KO) mice demonstrate reduced testis size with reduced numbers of Sc and Gc (spermatogonia, spermatocytes and round spermatids) leading to sub-fertility (56–58) concluding dispensable role of FSH in rodents. However, this dogma has recently been challenged as the expression of hyper-active m-FSH-R* shown to rescue male fertility in LH-Receptor (LH-R) KO mice with a complete absence of testicular androgens (due to exogenous flutamide treatment) (59).
FSH has been shown to be mitogenic for Sc and induce early differentiation in spermatogonia A in rhesus and cynomolgus monkeys (long-tailed macaque; Macaca fascicularis) (15–17). However, five finish men with an inactivating mutation in FSH-R have been reported to have variable degrees of spermatogenic failure without complete loss of fertility (60). In multiple hypogonadotropic hypogonadal clinical studies (61–64) and/or experimentally induced and/or gonadotropin deficient non-human primates (65–68), supplementations of FSH alone (independent of LH/T) results to limited spermatogenic recovery without appearance of either elongated spermatid or spermatozoa. FSH has been shown to regulate the number of pachytene spermatocytes in adult men (69). These reports suggest that like rodents, FSH plays only a supportive role in regulating male fertility in men. However, there are substantial contradictory reports available in men indicating an absolute requirement of FSH for sperm production. For example, hCG-mediated suppression of circulatory FSH in adult men results into poor sperm counts, with one individual developing complete azoospermia, which later gets recovered by FSH supplementation alone (70). Similarly, a hypophysectomized man with complete gonadotropin deficiency fathered three children having h-FSH-R* (71). Finally, complete infertility has been observed in men lacking normal circulating FSH due to mutated FSH-β (72–74). Furthermore, two cases of isolated FSH deficiency with normal FSH-β gene and usual LH/T levels [first, two young men having moderate testicular hypotrophy (75, 76), second, a 19 years old boy being homozygous for a novel silent polymorphism (G/T substitution) in FSH-β promoter (77),] show severe sperm abnormalities to complete azoospermia respectively. Intriguingly, immuno-neutralization of circulatory FSH shows acute spermatogenic abnormalities in both bonnet monkeys (Macaca radiata) (78) and men (79) suggesting FSH vaccination as a promising male contraceptive strategy (80). Taken together, the critical contribution of FSH in regulating primate spermatogenesis is still currently disputed (15, 17, 81, 82).
LH binds to LH-R expressed by interstitial Lc and indirectly exerts its actions on spermatogenesis through T–AR interaction via regulating Sc functions (Figure 2) (6, 82). In rats, fetal plasma LH concentration gets elevated from E 18- 21, then rises at P5-7, further gets reduced during P 20-25, rises again by P35 to peak at P60 and remains constant thereafter throughout adulthood prior to aging (P 400-500) (31, 32). In humans, pituitary LH is measurable from 12-18 WG (which is around 10-fold lower than placental hCG), peaks during 20-22 WG and then gradually decline in term pregnancy (Figures 3B, D) (33, 34). However, such a pattern remains inconsistent with the corresponding T profile which peaks during 12-14 WG and then drops during the second trimester corroborating with placental hCG (83). In post-natal life, LH concentration first raises upto the adult range within a week of parturition and then stays stable till 4-6 months, subsequently gets undetectable during the juvenile period, and finally shows the pubertal elevation by reaching its maximal range (4, 5).
Classical histological studies have identified two developmentally diverse populations of Lc e.g.- fetal (FLc) and adult (ALc) (83). FLc originate from coelomic epithelium and notch active Nestin-positive perivascular cells located at the gonad–mesonephros borders, and get specified as Nr5a1 or Ad4BP/SF-1 expressing cells by E 12.5 in fetal mouse testes (84). These cells produce androstenedione (precursor of T, due to lack of HSD17β3 enzyme) and play a critical role in initial virilization and patterning of the male external genitalia (84). However, in neonatal (P 5-15) testis, FLc undergo massive dedifferentiation and during puberty (P 15-21) gradually get replaced by T producing ALc (85, 86). FLc also secretes INSL3, a member of the insulin-relaxin family of peptides that acts on the body through the G-protein-coupled receptor relaxin/insulin-like family peptide receptor 2 (RXFP2). Missense mutations or ablation of Insl3 or Rxfp2 causes cryptorchidism leading to azoospermia (87, 88). However, unlike rodents, primate Lc shows a triphasic developmental pattern (83–86). In human, FLc peak during 12-14 WG (83) and subsequently get dedifferentiated by the end of the second trimester and is replaced by a unique population of neonatal-Lc (NLc) just during/after birth which persist for first 4-6 months of infantile age, when the HHT axis remains active (89). During the onset of juvenile period (inactivation of the HHT axis) massive involution occurs in the NLc population and finally ALc population originates from the dedifferentiating NLc population during puberty (83).
Like FSH-R, LH-R/LHCG-R is also a GPCR that recruits cAMP-dependent PKA pathway to induce the expression and activation of steroidogenic acute regulatory protein (STAR) at the outer mitochondrial membrane of ALc leading to cholesterol trafficking for initiation of steroidogenesis and eventually biosynthesize T (90). However, despite being responsive towards LH signal, FLc of both rodents and primates are independent of fetal LH action (83). FLc number or external genitalia remain unaffected in hpg (13), LH-RKO (91), LH-βKO (92) and ARKO (93, 94) adult male mice suggesting murine FLc are functionally independent of LH or T. In contrast, although patients having LH-β mutations show normal masculinized development (95–99), LHCG-R mutations lead to pseudo-hermaphroditism (100) indicating definite role of hCG on FLc functioning in men. However, in both the species LH is absolutely required for ALc function (83) as evident from various mouse models [hpg (13), LH-RKO (91), LH-βKO (92) and ARKO (93, 94)], etc and mutations in human LH-β/LHCGR genes resulting masculinized fetus but compromised pubertal development and complete azoospermia due to total absence of functional pituitary LH and testicular T (100). It is interesting to note here that fertility can be restored in men with isolated LH deficiency due to mutations in the LHβ gene by long-term hCG supplementations within the critical “window of testicular susceptibility” during pubertal development (101).
Stimulation of LH (resulting T) in rhesus and cynomolgus monkeys leads to spermatogonial differentiation and initiation of Gc meiosis without insignificant rise in Sc number (15, 17, 102–105). LH/hCG (or T) mediated absolute recovery of spermatogenesis has been demonstrated in gonadotropin withdrawal models (either by hypophysectomy or treatment of GnRH receptor antagonist or active immunization against GnRH) in adult rodents (106–111), men (64, 112, 113) and non-human primates (114–118). Exogenous supplementations of T or LH/hCG alone have been shown to induce complete spermatogenesis in immature hpg mice (119, 120) or natural or induced hypogonadal men (121, 122). Genetic ablations of LH-β or LH-R in mice further show cryptorchid testes with spermatogenic arrest and male infertility (91, 92). Human patients having inactivated LHCG-R or LH-β frequently show pseudohermaphroditism and cryptorchidism with Lc hypoplasia and spermatogenic arrest (123–132). Interestingly, a unique homozygous deletion on exon 10 in LHCG-R has been reported in an azoospermic man having normal phenotype with diminished LH signaling (but not towards hCG) indicating higher potency of hCG on ALc (123). In contrast, activating mutations in LH-β or LHCG-R were shown to be associated with precocious puberty and Lc hyperplasia (133–148). Such precocious puberty with Lc hyperplasia followed by infertility has been observed in mice over-expressing hyper-active (Asp582Gly) LH-R (149). However, spermatogenesis has been reported in a man with a splice-mutation (homozygous point mutation G to A at -1 position of intron-10 to exon-11 junction) in LHCG-R with severe loss of T production (150). A more surprising study has been reported in a 43 years old man with a homozygous deletion of nine bases in LHβ gene generating a deletion of amino acids from 10 to 12 (His, Pro, Ile) in the amino-terminal critical for conformational changes leading to undetectable LH (high FSH) with very low T (151). Paradoxically, this isolated LH deficiency case eventually shows sub-optimal but spontaneous spermatogenesis (151). It is important here to note that, despite high (20-100 fold) intra-testicular T (IIT) concentration has been considered to be critical for spermatogenic initiation (152, 153), low levels of T are sufficient to drive spermatogenic maintenance as evident by spontaneous spermatogenesis in LH-RKO mice at 12 months of age (154).
LH operates spermatogenic regulations through testicular androgen T and AR (155). T is essential for suppression of AMH (156, 157), pubertal maturation of testicular somatic cells (e.g.- PTc, Sc, Lc in developmental order) (2), the establishment of Blood-testis barrier (BTB) (158), meiotic progression of Gc and spermiation (159). The free titer of T depends upon the extent of the presence of sex hormone-binding globulin (SHBG) which binds to T with strong affinity; thus, SBHG regulates the process of spermatogenesis by controlling the serum concentration of biologically active T (160, 161). The absolute requirement of T on male fertility has been confirmed from ARKO (ubiquitously lacking AR) mice (93, 94). Despite most of the somatic testicular cells (Sc, PTc, Lc etc) express AR, Gc do not have functional AR (2, 3). Cell-specific selective ablation of AR [Sc specific i.e. SCARKO (162–164), Lc specific i.e. LcARKO (165, 166), PTc specific i.e. PTARKO (167, 168) or Gc specific i.e. GcARKO (169, 170)] demonstrated that AR expressed by Sc plays a pivotal role in the progression of Gc meiosis (20, 21, 155). Furthermore, the crossing of hpg mice with ARKO or SCARKO mice followed by T/5α- dihydrotestosterone (DHT) supplementation confirmed the critical significance of Sc-mediated AR signaling in spermatogenesis (171). The transition of round to elongated spermatid is fully dependent on T action transmitted via Sc (159).
In Sc, AR signals via both classical and non-classical manner (155). In the classical pathway, T (or 5α-DHT) activated AR binds to specific DNA sequences having Androgen Response Elements (ARE) and initiates the androgen-dependent transcriptional events e.g. Rhox5 expression (155). However, in a non-classical pathway, T gets coupled with membrane-bound AR and triggers the binding of the proline-rich region of AR with the SH3 domain of membrane bound SRC kinase leading to stimulation of EGF receptor and subsequently activates MAP (RAF, MEK, ERK) kinase or CREB cascade inducing several genes which lack typical AREs on their promoters e.g. Ldha, Claudin11, etc (155). In vitro studies show that T regulates spermiation via a non-classical pathway (155), however, in vivo studies suggest that classical pathway is most crucial for meiotic completion of Gc and fertility (159).
A productive synergy between FSH and LH (via T) has been observed in regulating maximal spermatogenic output (6, 14, 16, 17). For example, combined FSH and LH/hCG/T stimulations show better spermatogenic restoration than independent hormonal treatment in induced GnRH-depleted adult rats (16, 111) or primates (172–174). Patients suffering from hypogonadotropic hypogonadism show appreciable testicular maturation with sufficient Gc differentiation with combined FSH and hCG administrations (175–177). Pulsatile stimulations of LH and FSH together for only 11 days demonstrate enhanced Gc differentiation (upto spermatogonia B and primary spermatocytes) as compared to independent treatment of either LH or FSH in juvenile male monkeys (104). Moreover, T augments genes involved in FSH signalling pathway (e.g.- FSH-R, Gαs and Ric8b etc) resulting in elevated cAMP response in pubertal monkey Sc (178). These reports suggest that a coordinated network of FSH and T signalling in Sc facilitate the timely onset of the first spermatogenic wave in pubertal primates (14, 16, 17). Finally, spermatogenesis in Sc specific isolated or double (both FSH-R and AR) knockout mice gets affected more severely than single genetic ablation (either FSH-R or ARKO/SCARKO) confirming a dynamic synchronization between FSH and T action regulating the spermatogenic output thus male fertility (179–181)
For the past 50 years, various laboratories across the globe have significantly contributed in revealing the gonadotropic regulation of spermatogenesis (16, 17) with potential clinical implications (182, 183). Table 1 describes the critical role(s) of FSH and LH (T) in spermatogenesis, whereas Table 2 highlights the significant discoveries/advancements accomplished during past five decades in a chronological order.
Table 2 Chronological representation of the pioneering progress in gonadotropin biology during past decades.
In summary, hypothalamic KNDy neurons induce GnRH discharge which further stimulates the secretion of gonadotropins (FSH and LH) from pituitary. High and low pulse frequencies of GnRH selectively favor either LH or FSH release. Multiple experimental/natural models (e.g.- hypophysectomised or pharmacological/immunological deprivation of GnRH, hpg mice or hypogonadal men), inactivating or hyper-activating mutations in FSH-R/LHCG-R in men, murine genetic KOs collectively show the crucial role of FSH and LH (via T) in spermatogenic development and maintenance. In rodents, FSH essentially supports Sc proliferation and survival, division, and differentiation of pre-meiotic Gc, but fails independently to direct the completion of spermatogenesis. However, the sole role of FSH still remains controversial in men. On the other hand, LH (via T) founds to be indispensable for regulating male fertility in both species and Sc-mediated AR signaling found to be is most critical for the transition of round to elongated spermatids and the induction of spermiation. A productive synergy between FSH and T has been established to optimize the spermatogenic capacity both qualitatively and quantitatively. A recent report indicated the presence of a mesenchymal transcription factor (Tcf) 21 positive interstitial progenitor population acting as a potential reservoir during injury-induced ALc regeneration (187).
However, despite such extensive information generated during past decades translational progress in terms of clinical success has not been achieved yet in the field of gonadotropin biology toward treating infertility in men or developing reversal male contraceptives (1). This is largely due to limited numbers of hormone [FSH and LH (T)]-responsive genes identified so far with defining impact on spermatogenesis identified till date from multiple in vitro (184) and in vivo (185) studies. Future studies utilizing a cutting-edge single-cell transcriptomics approach are required to identify and investigate such putative gonadotropic inducible genes crucial for regulating male fertility with the following probable objectives/outcomes: significant advancement in classifying and curing idiopathic male infertility, bioengineering of fertilizable spermatozoa ex vivo, and sustainable development of potential male contraceptive targets (186, 188).
IB conceived the idea and designed and prepared the initial draft. SD prepared the figures, revised the manuscript and generated the final form with inputs from AB. All authors contributed to the article and approved the submitted version.
IB acknowledges the financial support from the University Grants Commission (F.30104/2015BSR) and Department of Science and Technology (ECR/2018/000868) New Delhi and Core fund to Dept. of Zoology, Central University of Kerala, Kasaragod, Kerala, India. SD appreciates the support obtained from Prof. Raviraja NS, Co-ordinator, Manipal Centre for Biotherapeutics Research, MAHE, Manipal. SD thanks DBT (BT/RLF/Re-entry/08/2019), New Delhi, India, for financial assistance. AB appreciates financial support received from DBT (BT/PR32910/MED/97/473/2020). However, the funder was not involved in the study design, collection, analysis, interpretation of data, or writing of this review.
SD appreciates the support obtained from Prof. Raviraja NS, Co-ordinator, Manipal Centre for Biotherapeutics Research, MAHE, Manipal.
The authors declare that the research was conducted in the absence of any commercial or financial relationships that could be construed as a potential conflict of interest.
All claims expressed in this article are solely those of the authors and do not necessarily represent those of their affiliated organizations, or those of the publisher, the editors and the reviewers. Any product that may be evaluated in this article, or claim that may be made by its manufacturer, is not guaranteed or endorsed by the publisher.
1. Agarwal A, Baskaran S, Parekh N, Cho CL, Henkel R, Vij S, et al. Male Infertility. Lancet (2021) 397(10271):319–33.
2. Sharpe RM. Regulation of spermatogenesis. In: Knobil E, Neil JD, editors. The physiology of reproduction. New York: NY:Raven Press (1994). p. 1363–434.
4. Plant TM. 60 YEARS OF NEUROENDOCRINOLOGY: The hypothalamo-pituitary-gonadal axis. J Endocrinol (2015) 226(2):T41–54.
5. Herbison AE. Control of puberty onset and fertility by gonadotropin-releasing hormone neurons. Nat Rev Endocrinol (2016) 12(8):452–66.
6. Walker WH, Cheng J. FSH and testosterone signaling in sertoli cells. Reproduction (2005) 130(1):15–28.
7. Lunenfeld B. Gonadotropin stimulation: past, present and future. Reprod Med Biol (2011) 11(1):11–25.
8. Kallman FJ, Schoenfeld WA, Barrera SE. The genetic aspects of primary hypogonadism. Am J Ment Defic (1944) 48:203–36.
9. Nowakowski H, Lenz W. Genetic aspects in male hypogonadism. Recent Prog Horm Res (1961) 17):53–95.
10. Amoss M, Burgus R, Blackwell R, Vale W, Fellows R, Guillemin R. Purification, amino acid composition and n-terminus of the hypothalamic luteinizing hormone releasing factor (LRF) of ovine origin. Biochem Biophys Res Commun (1971) 44(1):205–10. doi: 10.1016/S0006-291X(71)80179-1
11. Matsuo H, Baba Y, Nair RMG, Arimura A, Schally AV. Structure of the porcine LH- and FSH-releasing hormone. i. the proposed amino acid sequence. Biochem Biophys Res Commun (1971) 43(6):1334–9. doi: 10.1016/S0006-291X(71)80019-0
12. Wade N. Guillemin and schally: a race spurred by rivalry. Science (1978) 200(4341):510–3. doi: 10.1126/science.201.4355.510.a
13. Cattanach BM, Iddon CA, Charlton HM, Chiappa SA, Fink G. Gonadotrophin-releasing hormone deficiency in a mutant mouse with hypogonadism. Nature (1977) 269(5626):338–40. doi: 10.1038/269338a0
14. McLachlan RI, O’Donnell L, Meachem SJ, Stanton PG, de Kretser DM, Pratis K, et al. Identification of specific sites of hormonal regulation in spermatogenesis in rats, monkeys, and man. Recent Prog Horm Res (2002) 57:149–79. doi: 10.1210/rp.57.1.149
15. Plant TM, Marshall GR. The functional significance of FSH in spermatogenesis and the control of its secretion in Male primates. Endocr Rev (2001) 22(6):764–86. doi: 10.1210/edrv.22.6.0446
16. Ruwanpura SM, McLachlan RI, Meachem SJ. Hormonal regulation of male germ cell development. J Endocrinol (2010) 205(2):117–31. doi: 10.1677/JOE-10-0025
17. Ramaswamy S, Weinbauer GF. Endocrine control of spermatogenesis: Role of FSH and LH/ testosterone. Spermatogenesis (2015) 4(2):e996025.
18. Kumar TR. Mouse models for the study of synthesis, secretion, and action of pituitary gonadotropins. Prog Mol Biol Transl Sci (2016) 143:49–84. doi: 10.1016/bs.pmbts.2016.08.006
19. Jonas KC, Oduwole OO, Peltoketo H, Rulli SB, Huhtaniemi IT. Mouse models of altered gonadotrophin action: insight into male reproductive disorders. Reproduction (2014) 148(4):R63–70. doi: 10.1530/REP-14-0302
20. de Gendt K, Verhoeven G. Tissue- and cell-specific functions of the androgen receptor revealed through conditional knockout models in mice. Mol Cell Endocrinol (2012) 352(1–2):13–25. doi: 10.1016/j.mce.2011.08.008
21. Wang RS, Yeh S, Tzeng CR, Chang C. Androgen receptor roles in spermatogenesis and fertility: lessons from testicular cell-specific androgen receptor knockout mice. Endocr Rev (2009) 30(2):119–32. doi: 10.1210/er.2008-0025
22. Li Y, Schang G, Wang Y, Zhou X, Levasseur A, Boyer A, et al. Conditional deletion of FOXL2 and SMAD4 in gonadotropes of adult mice causes isolated FSH deficiency. Endocrinology (2018) 159(7):2641–55. doi: 10.1210/en.2018-00100
23. Fortin J, Boehm U, Weinstein MB, Graff JM, Bernard DJ. Follicle-stimulating hormone synthesis and fertility are intact in mice lacking SMAD3 DNA binding activity and SMAD2 in gonadotrope cells. FASEB J (2014) 28(3):1474–85. doi: 10.1096/fj.13-237818
24. Tran S, Zhou X, Lafleur C, Calderon MJ, Ellsworth BS, Kimmins S, et al. Impaired fertility and FSH synthesis in gonadotrope-specific Foxl2 knockout mice. Mol Endocrinol (2013) 27(3):407–21. doi: 10.1210/me.2012-1286
25. Li Y, Schang G, Boehm U, Deng CX, Graff J, Bernard DJ. SMAD3 regulates follicle-stimulating hormone synthesis by pituitary gonadotrope cells in vivo. J Biol Chem (2017) 292(6):2301–14. doi: 10.1074/jbc.M116.759167
26. Ulloa-Aguirre A, Reiter E, Crepieux P. FSH receptor signaling: Complexity of interactions and signal diversity. Endocrinology (2018) 159(8):3020–35. doi: 10.1210/en.2018-00452
27. Bhattacharya I, Pradhan BS, Sarda K, Gautam M, Basu S, Majumdar SS. A switch in sertoli cell responsiveness to FSH may be responsible for robust onset of germ cell differentiation during prepubartal testicular maturation in rats. Am J Physiol Endocrinol Metab (2012) 303(7):E886–98. doi: 10.1152/ajpendo.00293.2012
28. Bhattacharya I, Sharma SS, Sarkar H, Gupta A, Pradhan BS, Majumdar SS. FSH mediated cAMP signalling upregulates the expression of gα subunits in pubertal rat sertoli cells. Biochem Biophys Res Commun (2021) 569:100–5. doi: 10.1016/j.bbrc.2021.06.094
29. Majumdar SS, Sarda K, Bhattacharya I, Plant TM. Insufficient androgen and FSH signaling may be responsible for the azoospermia of the infantile primate testes despite exposure to an adult-like hormonal milieu. Hum Reprod (2012) 27(8):2515–25. doi: 10.1093/humrep/des184
30. Bhattacharya I, Basu S, Sarda K, Gautam M, Nagarajan P, Pradhan BS, et al. Low levels of gαs and Ric8b in testicular sertoli cells may underlie restricted FSH action during infancy in primates. Endocrinology (2015) 156(3):1143–55. doi: 10.1210/en.2014-1746
31. Chowdhury M, Steinberger E. Pituitary and plasma levels of gonadotrophins in foetal and newborn male and female rats. J Endocrinol (1976) 69(3):381–4. doi: 10.1677/joe.0.0690381
32. Ketelslegers JM, Hetzel WD, Sherins RJ, Catt KJ. Developmental changes in testicular gonadotropin receptors: plasma gonadotropins and plasma testosterone in the rat. Endocrinology (1978) 103(1):212–22. doi: 10.1210/endo-103-1-212
33. Clements JA, Reyes FI, Winter JSD, Faiman C. Studies on human sexual development. III. fetal pituitary and serum, and amniotic fluid concentrations of LH, CG, and FSH. J Clin Endocrinol Metab (1976) 42(1):9–19.
34. Dunkel L, Alfthan H, Stenman UH, Selstam G, Rosberg S, Albertsson-Wikland K. Developmental changes in 24-hour profiles of luteinizing hormone and follicle-stimulating hormone from prepuberty to midstages of puberty in boys. J Clin Endocrinol Metab (1992) 74(4):890–7. doi: 10.1210/jcem.74.4.1548356
35. Huhtaniemi IT, Yamamoto M, Ranta T, Jalkanen J, Jaffe RB. Follicle-stimulating hormone receptors appear earlier in the primate fetal testis than in the ovary. J Clin Endocrinol Metab (1987) 65(6):1210–4. doi: 10.1210/jcem-65-6-1210
36. Lee BC, Pineda JL, Spiliotis BE, Brown TJ, Bercu BB. Male Sexual development in the nonhuman primate. III. sertoli cell culture and age-related differences. Biol Reprod (1983) 28(5):1207–15.
37. Heckert LL, Griswold MD. The expression of the follicle-stimulating hormone receptor in spermatogenesis. Recent Prog Horm Res (2002) 57:129–48. doi: 10.1210/rp.57.1.129
38. Maguire SM, Tribley WA, Griswold MD. Follicle-stimulating hormone (FSH) regulates the expression of FSH receptor messenger ribonucleic acid in cultured sertoli cells and in hypophysectomized rat testis. Biol Reprod (1997) 56(5):1106–11. doi: 10.1095/biolreprod56.5.1106
39. Viswanathan P, Wood MA, Walker WH. Follicle-stimulating hormone (FSH) transiently blocks FSH receptor transcription by increasing inhibitor of deoxyribonucleic acid binding/differentiation-2 and decreasing upstream stimulatory factor expression in rat sertoli cells. Endocrinology (2009) 150(8):3783–91. doi: 10.1210/en.2008-1261
40. Al-Attar L, Noël K, Dutertre M, Belville C, Forest MG, Burgoyne PS, et al. Hormonal and cellular regulation of sertoli cell anti-müllerian hormone production in the postnatal mouse. J Clin Invest (1997) 100(6):1335–43. doi: 10.1172/JCI119653
41. Grinspon RP, Urrutia M, Rey RA. Male Central hypogonadism in paediatrics - the relevance of follicle-stimulating hormone and sertoli cell markers. Eur Endocrinol (2018) 14(2):67–71. doi: 10.17925/EE.2018.14.2.67
42. Orth JM. The role of follicle-stimulating hormone in controlling sertoli cell proliferation in testes of fetal rats. Endocrinology (1984) 115(4):1248–55. doi: 10.1210/endo-115-4-1248
43. Orth JM, Gunsalus GL, Lamperti AA. Evidence from sertoli cell-depleted rats indicates that spermatid number in adults depends on numbers of sertoli cells produced during perinatal development. Endocrinology (1988) 122(3):787–94. doi: 10.1210/endo-122-3-787
44. Johnston H, Baker PJ, Abel M, Charlton HM, Jackson G, Fleming L, et al. Regulation of sertoli cell number and activity by follicle-stimulating hormone and androgen during postnatal development in the mouse. Endocrinology (2004) 145(1):318–29. doi: 10.1210/en.2003-1055
45. Allan CM, Garcia A, Spaliviero J, Zhang FP, Jimenez M, Huhtaniemi I, et al. Complete sertoli cell proliferation induced by follicle-stimulating hormone (FSH) independently of luteinizing hormone activity: evidence from genetic models of isolated FSH action. Endocrinology (2004) 145(4):1587–93. doi: 10.1210/en.2003-1164
46. Mc lachlan RI, Wreford NG, Kretser DMD, Robertson DM. The effects of recombinant follicle-stimulating hormone on the restoration of spermatogenesis in the gonadotropin-releasing hormone-immunized adult rat. Endocrinology (1995) 136(9):4035–43. doi: 10.1210/endo.136.9.7649112
47. Russell LD, Kershaw M, Borg KE, Shennawy A, Rulli SS, Gates RJ, et al. Hormonal regulation of spermatogenesis in the hypophysectomized rat: FSH maintenance of cellular viability during pubertal spermatogenesis. J Androl (1998) 19(3):308–19.
48. Hikim APS, Swerdloff RS. Temporal and stage-specific effects of recombinant human follicle-stimulating hormone on the maintenance of spermatogenesis in gonadotropin-releasing hormone antagonist-treated rat. Endocrinology (1995) 136(1):253–61. doi: 10.1210/endo.136.1.7828538
49. Meachem SJ, Ruwanpura SM, Ziolkowski J, Ague JM, Skinner MK, Loveland KL. Developmentally distinct in vivo effects of FSH on proliferation and apoptosis during testis maturation. J Endocrinol (2005) 186(3):429–46. doi: 10.1677/joe.1.06121
50. Singh J, Handelsman DJ. Neonatal administration of FSH increases sertoli cell numbers and spermatogenesis in gonadotropin-deficient (hpg) mice. J Endocrinol (1996) 151(1):37–48. doi: 10.1677/joe.0.1510037
51. Allan CM, Haywood M, Swaraj S, Spaliviero J, Koch A, Jimenez M, et al. A novel transgenic model to characterize the specific effects of follicle-stimulating hormone on gonadal physiology in the absence of luteinizing hormone actions. Endocrinology (2001) 142(6):2213–20. doi: 10.1210/endo.142.6.8092
52. Haywood M, Tymchenko N, Spaliviero J, Koch A, Jimenez M, Gromoll J, et al. An activated human follicle-stimulating hormone (FSH) receptor stimulates FSH-like activity in gonadotropin-deficient transgenic mice. Mol Endocrinol (2002) 16(11):2582–91. doi: 10.1210/me.2002-0032
53. Allan CM, Lim P, Robson M, Spaliviero J, Handelsman DJ. Transgenic mutant D567G but not wild-type human FSH receptor overexpression provides FSH-independent and promiscuous glycoprotein hormone sertoli cell signaling. Am J Physiol Endocrinol Metab (2009) 296(5):E1022-28. doi: 10.1152/ajpendo.90941.2008
54. Allan CM, Garcia A, Spaliviero J, Jimenez M. Maintenance of spermatogenesis by the activated human (Asp567Gly) FSH receptor during testicular regression due to hormonal withdrawal. Biol Reprod (2006) 74(5):938–44. doi: 10.1095/biolreprod.105.048413
55. McDonald R, Sadler C, Kumar TR. Gain-of-Function genetic models to study FSH action. Front Endocrinol (Lausanne) (2019) 10(FEB). doi: 10.3389/fendo.2019.00028
56. Abel MH, Wootton AN, Wilkins V, Huhtaniemi I, Knight PG, Charlton HM. The effect of a null mutation in the follicle-stimulating hormone receptor gene on mouse reproduction. Endocrinology (2000) 141(5):1795–803. doi: 10.1210/endo.141.5.7456
57. Dierich A, Sairam MR, Monaco L, Fimia GM, Gansmuller A, Lemeur M, et al. Impairing follicle-stimulating hormone (FSH) signaling in vivo: targeted disruption of the FSH receptor leads to aberrant gametogenesis and hormonal imbalance. Proc Natl Acad Sci U.S.A. (1998) 95(23):13612–7. doi: 10.1073/pnas.95.23.13612
58. Kumar TR, Wang Y, Lu N, Matzuk MM. Follicle stimulating hormone is required for ovarian follicle maturation but not male fertility. Nat Genet (1997) 15(2):201–4. doi: 10.1038/ng0297-201
59. Oduwole OO, Peltoketo H, Poliandri A, Vengadabady L, Chrusciel M, Doroszko M, et al. Constitutively active follicle-stimulating hormone receptor enables androgen-independent spermatogenesis. J Clin Invest (2018) 128(5):1787–92. doi: 10.1172/JCI96794
60. Tapanainen JS, Aittomäki K, Min J, Vaskivuo T, Huhtaniemi IT. Men homozygous for an inactivating mutation of the follicle-stimulating hormone (FSH) receptor gene present variable suppression of spermatogenesis and fertility. Nat Genet (1997) 15(2):205–6. doi: 10.1038/ng0297-205
61. Bremner WJ, Matsumoto AM, Sussman AM, Paulsen C. Follicle-stimulating hormone and human spermatogenesis. J Clin Invest (1981) 68(4):1044–52. doi: 10.1172/JCI110327
62. Foresta C, Bettella A, Ferlin A, Garolla A, Rossato M. Evidence for a stimulatory role of follicle-stimulating hormone on the spermatogonial population in adult males. Fertil Steril (1998) 69(4):636–42. doi: 10.1016/S0015-0282(98)00008-9
63. Matsumoto AM, Karpas AE, Paulsen CA, Bremner WJ. Reinitiation of sperm production in gonadotropin-suppressed normal men by administration of follicle-stimulating hormone. J Clin Invest (1983) 72(3):1005–15. doi: 10.1172/JCI111024
64. Matsumoto AM, Paulsen CA, Bremner WJ. Stimulation of sperm production by human luteinizing hormone in gonadotropin-suppressed normal men. J Clin Endocrinol Metab (1984) 59(5):882–7. doi: 10.1210/jcem-59-5-882
65. van Alphen MMA, van de Kant HJG, de Rooij DG. Follicle-stimulating hormone stimulates spermatogenesis in the adult monkey. Endocrinology (1988) 123(3):1449–55. doi: 10.1210/endo-123-3-1449
66. Marshall GR, Zorub DS, Plant TM. Follicle-stimulating hormone amplifies the population of differentiated spermatogonia in the hypophysectomized testosterone-replaced adult rhesus monkey (Macaca mulatta). Endocrinology (1995) 136(8):3504–11. doi: 10.1210/endo.136.8.7628387
67. Weinbauer GF, Behre HM, Fingscheidt U, Nieschlag E. Human follicle-stimulating hormone exerts a stimulatory effect on spermatogenesis, testicular size, and serum inhibin levels in the gonadotropin-releasing hormone antagonist-treated nonhuman primate (Macaca fascicularis). Endocrinology (1991) 129(4):1831–9. doi: 10.1210/endo-129-4-1831
68. Simorangkir DR, Ramaswamy S, Marshall GR, Pohl CR, Plant TM. A selective monotropic elevation of FSH, but not that of LH, amplifies the proliferation and differentiation of spermatogonia in the adult rhesus monkey (Macaca mulatta). Hum Reprod (2009) 24(7):1584–95. doi: 10.1093/humrep/dep052
69. Matthiesson KL, McLachlan RI, O’Donnell L, Frydenberg M, Robertson DM, Stanton PG, et al. The relative roles of follicle-stimulating hormone and luteinizing hormone in maintaining spermatogonial maturation and spermiation in normal men. J Clin Endocrinol Metab (2006) 91(10):3962–9. doi: 10.1210/jc.2006-1145
70. Matsumoto AM, Karpas AE, Bremner WJ. Chronic human chorionic gonadotropin administration in normal men: evidence that follicle-stimulating hormone is necessary for the maintenance of quantitatively normal spermatogenesis in man. J Clin Endocrinol Metab (1986) 62(6):1184–92. doi: 10.1210/jcem-62-6-1184
71. Gromoll J, Simoni M, Nieschlag E. An activating mutation of the follicle-stimulating hormone receptor autonomously sustains spermatogenesis in a hypophysectomized man. J Clin Endocrinol Metab (1996) 81(4):1367–70.
72. Phillip M, Arbelle JE, Segev Y, Parvari R. Male Hypogonadism due to a mutation in the gene for the beta-subunit of follicle-stimulating hormone. N Engl J Med (1998) 338(24):1729–32. doi: 10.1056/NEJM199806113382404
73. Simoni M, Casarini L. Mechanisms in endocrinology: Genetics of FSH action: a 2014-and-beyond view. Eur J Endocrinol (2014) 170(3):R91–107. doi: 10.1530/EJE-13-0624
74. Zheng J, Mao J, Cui M, Liu Z, Wang X, Xiong S, et al. Novel FSHβ mutation in a male patient with isolated FSH deficiency and infertility. Eur J Med Genet (2017) 60(6):335–9. doi: 10.1016/j.ejmg.2017.04.004
75. Layman LC, Porto ALA, Xie J, da Motta LACR, da Motta LDC, Weiser W, et al. FSH beta gene mutations in a female with partial breast development and a male sibling with normal puberty and azoospermia. J Clin Endocrinol Metab (2002) 87(8):3702–7.
76. Rougier C, Hieronimus S, Panaïa-Ferrari P, Lahlou N, Paris F, Fenichel P. Isolated follicle-stimulating hormone (FSH) deficiency in two infertile men without FSH β gene mutation: Case report and literature review. Ann Endocrinol (Paris) (2019) 80(4):234–9. doi: 10.1016/j.ando.2019.06.002
77. Mantovani G, Borgato S, Beck-Peccoz P, Romoli R, Borretta G, Persani L. Isolated follicle-stimulating hormone (FSH) deficiency in a young man with normal virilization who did not have mutations in the FSHβ gene. Fertil Steril (2003) 79(2):434–6.
78. Moudgal NR, Sairam MR, Krishnamurthy HN, Sridhar S, Krishnamurthy H, Khan H. Immunization of male bonnet monkeys (M. radiata) with a recombinant FSH receptor preparation affects testicular function and fertility. Endocrinology (1997) 138(7):3065–8.
79. Moudgal NR, Murthy GS, Prasanna Kumar KM, Martin F, Suresh R, Medhamurthy R, et al. Responsiveness of human male volunteers to immunization with ovine follicle stimulating hormone vaccine: results of a pilot study. Hum Reprod (1997) 12(3):457–63.
80. Moudgal NR, Dighe RR. Is FSH based contraceptive vaccine a feasible proposition for the human Male? Reprod Immunol (1999), 346–57.
81. Moudgal NR, Sairam MR. Is there a true requirement for follicle stimulating hormone in promoting spermatogenesis and fertility in primates? Hum Reprod (1998) 13(4):916–9.
82. Nieschlag E, Simoni M, Gromoll J, Weinbauer GF. Role of FSH in the regulation of spermatogenesis: clinical aspects. Clin Endocrinol (Oxf) (1999) 51(2):139–46. doi: 10.1046/j.1365-2265.1999.00846.x
83. Teerds KJ, Huhtaniemi IT. Morphological and functional maturation of leydig cells: from rodent models to primates. Hum Reprod Update (2015) 21(3):310–28. doi: 10.1093/humupd/dmv008
84. Kumar DL, DeFalco T. A perivascular niche for multipotent progenitors in the fetal testis. Nat Commun (2018) 9(1). doi: 10.1038/s41467-018-06996-3
85. Shima Y, Morohashi K-I. Leydig progenitor cells in fetal testis. Mol Cell Endocrinol (2017) 445:55–64. doi: 10.1016/j.mce.2016.12.006
86. Inoue M, Baba T, Morohashi K-I. Recent progress in understanding the mechanisms of leydig cell differentiation. Mol Cell Endocrinol (2018) 468:39–46. doi: 10.1016/j.mce.2017.12.013
87. Huang X, Jia J, Sun M, Li M, Liu N. Mutational screening of the INSL 3 gene in azoospermic males with a history of cryptorchidism. Andrologia (2016) 48(7):835–9. doi: 10.1111/and.12522
88. Nowacka-Woszuk J, Krzeminska P, Nowak T, Gogulski M, Switonski M, Stachowiak M. Analysis of transcript and methylation levels of INSL3 and RXFP2 in undescended and descended dog testes suggested promising biomarkers associated with cryptorchidism. Theriogenology (2020) 157:483–89. doi: 10.1016/j.theriogenology.2020.08.029
89. Bhattacharya I, Sen Sharma S, Majumdar SS. Pubertal orchestration of hormones and testis in primates. Mol Reprod Dev (2019) 86(11):1505–30. doi: 10.1002/mrd.23246
90. Zirkin BR, Papadopoulos V. Leydig cells: formation, function, and regulation. Biol Reprod (2018) 99(1):101–11. doi: 10.1093/biolre/ioy059
91. Zhang FP, Poutanen M, Wilbertz J, Huhtaniemi I. Normal prenatal but arrested postnatal sexual development of luteinizing hormone receptor knockout (LuRKO) mice. Mol Endocrinol (2001) 15(1):172–83. doi: 10.1210/mend.15.1.0582
92. Ma X, Dong Y, Matzuk MM, Kumar TR. Targeted disruption of luteinizing hormone beta-subunit leads to hypogonadism, defects in gonadal steroidogenesis, and infertility. Proc Natl Acad Sci U.S.A. (2004) 101(49):17294–9. doi: 10.1073/pnas.0404743101
93. Yeh S, Tsai MY, Xu Q, Mu XM, Lardy H, Huang KE, et al. Generation and characterization of androgen receptor knockout (ARKO) mice: an in vivo model for the study of androgen functions in selective tissues. Proc Natl Acad Sci U.S.A. (2002) 99(21):13498–503. doi: 10.1073/pnas.212474399
94. O’Shaughnessy PJ, Johnston H, Willerton L, Baker PJ. Failure of normal adult leydig cell development in androgen-receptor-deficient mice. J Cell Sci (2002) 115(Pt 17):3491–6. doi: 10.1242/jcs.115.17.3491
95. Axelrod L, Neer RM, Kliman B. Hypogonadism in a male with immunologically active, biologically inactive luteinizing hormone: an exception to a venerable rule. J Clin Endocrinol Metab (1979) 48(2):279–87. doi: 10.1210/jcem-48-2-279
96. Weiss J, Axelrod L, Whitcomb RW, Harris PE, Crowley WF, Jameson JL. Hypogonadism caused by a single amino acid substitution in the beta subunit of luteinizing hormone. N Engl J Med (1992) 326(3):179–83. doi: 10.1056/NEJM199201163260306
97. Valdes-Socin H, Salvi R, Daly AF, Gaillard RC, Quatresooz P, Tebeu PM, et al. Hypogonadism in a patient with a mutation in the luteinizing hormone beta-subunit gene. N Engl J Med (2004) 351(25):2619–25. doi: 10.1056/NEJMoa040326
98. Lofrano-Porto A, Barra GB, Giacomini LA, Nascimento PP, Latronico AC, Casulari LA, et al. Luteinizing hormone beta mutation and hypogonadism in men and women. N Engl J Med (2007) 357(9):897–904. doi: 10.1056/NEJMoa071999
99. Basciani S, Watanabe M, Mariani S, Passeri M, Persichetti A, Fiore D, et al. Hypogonadism in a patient with two novel mutations of the luteinizing hormone β-subunit gene expressed in a compound heterozygous form. J Clin Endocrinol Metab (2012) 97(9):3031–8. doi: 10.1210/jc.2012-1986
100. Themmen APN, Huhtaniemi IT. Mutations of gonadotropins and gonadotropin receptors: elucidating the physiology and pathophysiology of pituitary-gonadal function. Endocr Rev (2000) 21(5):551–83. doi: 10.1210/edrv.21.5.0409
101. Grumbach MM. Commentary: A window of opportunity: The diagnosis of gonadotropin deficiency in the male infant. J Clin Endocrinol Metab (2005) 90:3122–7. doi: 10.1210/jc.2004-2465
102. Marshall GR, Plant TM. Puberty occurring either spontaneously or induced precociously in rhesus monkey (Macaca mulatta) is associated with a marked proliferation of sertoli cells. Biol Reprod (1996) 54(6):1192–9. doi: 10.1095/biolreprod54.6.1192
103. Majumdar SS, Winters SJ, Plant TM. A study of the relative roles of follicle-stimulating hormone and luteinizing hormone in the regulation of testicular inhibin secretion in the rhesus monkey (Macaca mulatta)*. Endocrinology (1997) 138:1363–73. doi: 10.1210/endo.138.4.5058
104. Ramaswamy S, Plant TM, Marshall GR. Pulsatile stimulation with recombinant single chain human luteinizing hormone elicits precocious sertoli cell proliferation in the juvenile male rhesus monkey (Macaca mulatta). Biol Reprod (2000) 63(1):82–8. doi: 10.1095/biolreprod63.1.82
105. Ramaswamy S, Walker WH, Aliberti P, Sethi R, Marshall GR, Smith A, et al. The testicular transcriptome associated with spermatogonia differentiation initiated by gonadotrophin stimulation in the juvenile rhesus monkey (Macaca mulatta). Hum Reprod (2017) 32(10):2088–100. doi: 10.1093/humrep/dex270
106. Santulli R, Sprando RL, Awoniyi CA, Ewing LL, Zirkin BR, Zirkin BR. To what extent can spermatogenesis be maintained in the hypophysectomized adult rat testis with exogenously administered testosterone? Endocrinology (1990) 126(1):95–102. doi: 10.1210/endo-126-1-95
107. Awoniyi CA, Sprando RL, Santulli R, Chandrashekar V, Ewing LL, Zirkin BR, et al. Restoration of spermatogenesis by exogenously administered testosterone in rats made azoospermic by hypophysectomy or withdrawal of luteinizing hormone alone. Endocrinology (1990) 127(1):177–84. doi: 10.1210/endo-127-1-177
108. Awoniyi CA, Santulli R, Chandrashekar V, Schanbacher BD, Zirkin BR. Quantitative restoration of advanced spermatogenic cells in adult male rats made azoospermic by active immunization against luteinizing hormone or gonadotropin-releasing hormone. Endocrinology (1989) 125(3):1303–9. doi: 10.1210/endo-125-3-1303
109. Sun YT, Irby DC, Robertson DM, de Kretser DM. The effects of exogenously administered testosterone on spermatogenesis in intact and hypophysectomized rats. Endocrinology (1989) 125(2):1000–10. doi: 10.1210/endo-125-2-1000
110. Rea MA, Marshall GR, Weinbauer GF, Nieschlag E. Testosterone maintains pituitary and serum FSH and spermatogenesis in gonadotrophin-releasing hormone antagonist-suppressed rats. J Endocrinol (1986) 108(1):101–7. doi: 10.1677/joe.0.1080101
111. Bartlett JMS, Weinbauer GF, Nieschlag E. Differential effects of FSH and testosterone on the maintenance of spermatogenesis in the adult hypophysectomized rat. J Endocrinol (1989) 121(1):49–58. doi: 10.1677/joe.0.1210049
112. Whitcomb RW, Crowley WF. Clinical review 4: Diagnosis and treatment of isolated gonadotropin-releasing hormone deficiency in men. J Clin Endocrinol Metab (1990) 70(1):3–7. doi: 10.1210/jcem-70-1-3
113. Swerdloff RS, Bagatell CJ, Wang C, Anawalt BD, Berman N, Steiner B, et al. Suppression of spermatogenesis in man induced by nal-glu gonadotropin releasing hormone antagonist and testosterone enanthate (TE) is maintained by TE alone. J Clin Endocrinol Metab (1998) 83(10):3527–33.
114. Weinbauer GF, Limberger A, Behre HM, Nieschlag E. Can testosterone alone maintain the gonadotrophin-releasing hormone antagonist-induced suppression of spermatogenesis in the non-human primate? J Endocrinol (1994) 142(3):485–95. doi: 10.1677/joe.0.1420485
115. Marshall GR, Wickings EJ, Lüdecke DK, Nieschlag E. Stimulation of spermatogenesis in stalk-sectioned rhesus monkeys by testosterone alone. J Clin Endocrinol Metab (1983) 57(1):152–9. doi: 10.1210/jcem-57-1-152
116. Marshall GR, Wickings EJ, Nieschlag E. Testosterone can initiate spermatogenesis in an immature nonhuman primate, macaca fascicularis. Endocrinology (1984) 114(6):2228–33. doi: 10.1210/endo-114-6-2228
117. Marshall GR, Jockenhovel F, Ludecke D, Nieschlag E. Maintenance of complete but quantitatively reduced spermatogenesis in hypophysectomized monkeys by testosterone alone. Acta Endocrinol (Copenh) (1986) 113(3):424–31. doi: 10.1530/acta.0.1130424
118. Weinbauer GF, Göckeler E, Nieschlag E. Testosterone prevents complete suppression of spermatogenesis in the gonadotropin-releasing hormone antagonist-treated nonhuman primate (Macaca fascicularis). J Clin Endocrinol Metab (1988) 67(2):284–90. doi: 10.1210/jcem-67-2-284
119. Singh J, O’neill C, Handelsman DJ. Induction of spermatogenesis by androgens in gonadotropin-deficient (hpg) mice. Endocrinology (1995) 136(12):5311–21. doi: 10.1210/endo.136.12.7588276
120. Singh J, Handelsman DJ. The effects of recombinant FSH on testosterone-induced spermatogenesis in gonadotrophin-deficient (hpg) mice. J Androl (1996) 17(4):382–93.
121. Burris AS, Rodbard HW, Winters SJ, Sherins RJ. Gonadotropin therapy in men with isolated hypogonadotropic hypogonadism: the response to human chorionic gonadotropin is predicted by initial testicular size. J Clin Endocrinol Metab (1988) 66(6):1144–51. doi: 10.1210/jcem-66-6-1144
122. Fraietta R, Zylberstejn DS, Esteves SC. Hypogonadotropic hypogonadism revisited. Clinics (Sao Paulo) (2013) 68 Suppl 1(Suppl 1):81–8. doi: 10.6061/clinics/2013(Sup01)09
123. Gromoll J, Eiholzer U, Nieschlag E, Simoni M. Male Hypogonadism caused by homozygous deletion of exon 10 of the luteinizing hormone (LH) receptor: differential action of human chorionic gonadotropin and LH. J Clin Endocrinol Metab (2000) 85(6):2281–6. doi: 10.1210/jcem.85.6.6636
124. Martens JWM, Lumbroso S, Verhoef-Post M, Georget V, Richter-Unruh A, Szarras-Czapnik M, et al. Mutant luteinizing hormone receptors in a compound heterozygous patient with complete leydig cell hypoplasia: abnormal processing causes signaling deficiency. J Clin Endocrinol Metab (2002) 87(6):2506–13. doi: 10.1210/jcem.87.6.8523
125. Richter-Unruh A, Martens JWM, Verhoef-Post M, Wessels HT, Kors WA, Sinnecker GHG, et al. Leydig cell hypoplasia: cases with new mutations, new polymorphisms and cases without mutations in the luteinizing hormone receptor gene. Clin Endocrinol (Oxf) (2002) 56(1):103–12. doi: 10.1046/j.0300-0664.2001.01437.x
126. Richter-Unruh A, Korsch E, Hiort O, Holterhus PM, Themmen AP, Wudy SA. Novel insertion frameshift mutation of the LH receptor gene: problematic clinical distinction of leydig cell hypoplasia from enzyme defects primarily affecting testosterone biosynthesis. Eur J Endocrinol (2005) 152(2):255–9. doi: 10.1530/eje.1.01852
127. Qiao J, Han B, Liu BL, Chen X, Ru Y, Cheng KX, et al. A splice site mutation combined with a novel missense mutation of LHCGR cause male pseudohermaphroditism. Hum Mutat (2009) 30(9):E855-65. doi: 10.1002/humu.21072
128. Simoni M, Tüttelmann F, Michel C, Böckenfeld Y, Nieschlag E, Gromoll J. Polymorphisms of the luteinizing hormone/chorionic gonadotropin receptor gene: association with maldescended testes and male infertility. Pharmacogenet Genomics (2008) 18(3):193–200. doi: 10.1097/FPC.0b013e3282f4e98c
129. Richard N, Leprince C, Gruchy N, Pigny P, Andrieux J, Mittre H, et al. Identification by array-comparative genomic hybridization (array-CGH) of a large deletion of luteinizing hormone receptor gene combined with a missense mutation in a patient diagnosed with a 46,XY disorder of sex development and application to prenatal diagnosis. Endocr J (2011) 58(9):769–76. doi: 10.1507/endocrj.K11E-119
130. Kossack N, Troppmann B, Richter-Unruh A, Kleinau G, Gromoll J. Aberrant transcription of the LHCGR gene caused by a mutation in exon 6A leads to leydig cell hypoplasia type II. Mol Cell Endocrinol (2013) 366(1):59–67. doi: 10.1016/j.mce.2012.11.018
131. Themmen APN. An update of the pathophysiology of human gonadotrophin subunit and receptor gene mutations and polymorphisms. Reproduction (2005) 130(3):263–74. doi: 10.1530/rep.1.00663
132. Latronico A, Arnhold IP. Inactivating mutations of the human luteinizing hormone receptor in both sexes. Semin Reprod Med (2012) 30(5):382–6. doi: 10.1055/s-0032-1324721
133. Steinberger E, Root A, Ficher M, Smith KD. The role of androgens in the initiation of spermatogenesis in man. J Clin Endocrinol Metab (1973) 37(5):746–51. doi: 10.1210/jcem-37-5-746
134. Shenker A, Laue L, Kosugi S, Merendino JJ, Minegishi T, Cutler GB. A constitutively activating mutation of the luteinizing hormone receptor in familial male precocious puberty. Nature (1993) 365(6447):652–4. doi: 10.1038/365652a0
135. Latronico AC, Shinozaki H, Guerra G Jr., Pereira MAA, Lemos Marini SH, Baptista MTM, et al. Gonadotropin-independent precocious puberty due to luteinizing hormone receptor mutations in Brazilian boys: a novel constitutively activating mutation in the first transmembrane helix. J Clin Endocrinol Metab (2000) 85(12):4799–805.
136. Soriano-Guillén L, Lahlou N, Chauvet G, Roger M, Chaussain JL, Carel JC. Adult height after ketoconazole treatment in patients with familial male-limited precocious puberty. J Clin Endocrinol Metab (2005) 90(1):147–51. doi: 10.1210/jc.2004-1438
137. Soriano-Guillen L, Mitchell V, Carel JC, Barbet P, Roger M, Lahlou N. Activating mutations in the luteinizing hormone receptor gene: a human model of non-follicle-stimulating hormone-dependent inhibin production and germ cell maturation. J Clin Endocrinol Metab (2006) 91(8):3041–7. doi: 10.1210/jc.2005-2564
138. Nagasaki K, Katsumata N, Ogawa Y, Kikuchi T, Uchiyama M. Novel C617Y mutation in the 7th transmembrane segment of luteinizing hormone/choriogonadotropin receptor in a Japanese boy with peripheral precocious puberty. Endocr J (2010) 57(12):1055–60. doi: 10.1507/endocrj.K10E-227
139. Liu G, Duranteau L, Carel JC, Monroe J, Doyle DA, Shenker A. Leydig-cell tumors caused by an activating mutation of the gene encoding the luteinizing hormone receptor. N Engl J Med (1999) 341(23):1731–6. doi: 10.1056/NEJM199912023412304
140. Zarrilli S, Lombardi G, Pacsano L, di Somma C, Colao A, Mirone V, et al. Hormonal and seminal evaluation of leydig cell tumour patients before and after orchiectomy. Andrologia (2000) 32(3):147–54. doi: 10.1046/j.1439-0272.2000.00356.x
141. Richter-Unruh A, Wessels HT, Menken U, Bergmann M, Schmittmann-Ohters K, Schaper J, et al. Male LH-independent sexual precocity in a 3.5-year-old boy caused by a somatic activating mutation of the LH receptor in a leydig cell tumor. J Clin Endocrinol Metab (2002) 87(3):1052–6.
142. Canto P, Söderlund D, Ramón G, Nishimura E, Méndez JP. Mutational analysis of the luteinizing hormone receptor gene in two individuals with leydig cell tumors. Am J Med Genet (2002) 108(2):148–52. doi: 10.1002/ajmg.10218
143. Sangkhathat S, Kanngurn S, Jaruratanasirikul S, Tubtawee T, Chaiyapan W, Patrapinyokul S, et al. Peripheral precocious puberty in a Male caused by leydig cell adenoma harboring a somatic mutation of the LHR gene: Report of a case. J Med Assoc Thai (2010) 93(9):1093.
144. Boot AM, Lumbroso S, Verhoef-Post M, Richter-Unruh A, Looijenga LHJ, Funaro A, et al. Mutation analysis of the LH receptor gene in leydig cell adenoma and hyperplasia and functional and biochemical studies of activating mutations of the LH receptor gene. J Clin Endocrinol Metab (2011) 96(7): E1197-205. doi: 10.1210/jc.2010-3031
145. Shenker A. Activating mutations of the lutropin choriogonadotropin receptor in precocious puberty. Recept Channels (2002) 8(1):3–18. doi: 10.3109/10606820212138
146. Polepalle SK, Shabaik A, Alagiri M. Leydig cell tumor in a child with spermatocyte maturation and no pseudoprecocious puberty. Urology (2003) 62(3):551. doi: 10.1016/S0090-4295(03)00469-2
147. Cajaiba MM, Reyes-Múgica M, Rios JCS, Nistal M. Non-tumoural parenchyma in leydig cell tumours: pathogenetic considerations. Int J Androl (2008) 31(3):331–6. doi: 10.1111/j.1365-2605.2007.00774.x
148. O’Grady MJ, McGrath N, Quinn FM, Capra ML, McDermott MB, Murphy NP. Spermatogenesis in a prepubertal boy. J Pediatr (2012) 161(2):369.
149. McGee SR, Narayan P. Precocious puberty and leydig cell hyperplasia in male mice with a gain of function mutation in the LH receptor gene. Endocrinology (2013) 154(10):3900–13. doi: 10.1210/en.2012-2179
150. Bruysters M, Christin-Maitre S, Verhoef-Post M, Sultan C, Auger J, Faugeron I, et al. A new LH receptor splice mutation responsible for male hypogonadism with subnormal sperm production in the propositus, and infertility with regular cycles in an affected sister. Hum Reprod (2008) 23(8):1917–23. doi: 10.1093/humrep/den180
151. Achard C, Courtillot C, Lahuna O, Méduri G, Soufir JC, Lière P, et al. Normal spermatogenesis in a man with mutant luteinizing hormone. N Engl J Med (2009) 361(19):1856–63. doi: 10.1056/NEJMoa0805792
152. Morse HC, Horike N, Rowley MJ, Heller CG. Testosterone concentrations in testes of normal men: effects of testosterone propionate administration. J Clin Endocrinol Metab (1973) 37(6):882–6. doi: 10.1210/jcem-37-6-882
153. Zirkin BR, Santulli R, Awoniyi CA, Ewing LL. Maintenance of advanced spermatogenic cells in the adult rat testis: quantitative relationship to testosterone concentration within the testis. Endocrinology (1989) 124(6):3043–9. doi: 10.1210/endo-124-6-3043
154. Zhang FP, Pakarainen T, Poutanen M, Toppari J, Huhtaniemi I. The low gonadotropin-independent constitutive production of testicular testosterone is sufficient to maintain spermatogenesis. Proc Natl Acad Sci U.S.A. (2003) 100(23):13692–7. doi: 10.1073/pnas.2232815100
155. Walker WH. Androgen actions in the testis and the regulation of spermatogenesis. Adv Exp Med Biol (2021) 1288:175–203. doi: 10.1007/978-3-030-77779-1_9
156. Christin-Maitre S, Young J. Androgens and spermatogenesis. Ann Endocrinol (Paris) (2022) 83(3):155–8. doi: 10.1016/j.ando.2022.04.010
157. Rey R, Lukas-Croisier C, Lasala C, Bedecarrás P. AMH/MIS: What we know already about the gene, the protein and its regulation. Mol Cell Endocrinol (2003) 211(1–2):21–31.
158. McCabe MJ, Allan CM, Foo CFH, Nicholls PK, McTavish KJ, Stanton PG. Androgen initiates sertoli cell tight junction formation in the hypogonadal (hpg) mouse. Biol Reprod (2012) 87(2).
159. Cooke PS, Walker WH. Male Fertility in mice requires classical and nonclassical androgen signaling. Cell Rep (2021) 36(7).
160. Dalmazzo A, Losano JDA, Angrimani DSR, Pereira IVA, Goissis MD, Francischini MCP, et al. Immunolocalisation and expression of oxytocin receptors and sex hormone-binding globulin in the testis and epididymis of dogs: Correlation with sperm function. Reprod Fertil Dev (2019) 31(9):1434–43.
161. Vos MJ, Mijnhout GS, Rondeel JMM, Baron W, Groeneveld PHP. Sex hormone binding globulin deficiency due to a homozygous missense mutation. J Clin Endocrinol Metab (2014) 99(9):E1798-802.
162. Chang C, Chen YT, der YS, Xu Q, RS W, Guillou F, et al. Infertility with defective spermatogenesis and hypotestosteronemia in male mice lacking the androgen receptor in sertoli cells. Proc Natl Acad Sci U.S.A. (2004) 101(18):6876–81. doi: 10.1073/pnas.0307306101
163. de Gendt K, Swinnen JV, Saunders PTK, Schoonjans L, Dewerchin M, Devos A, et al. A sertoli cell-selective knockout of the androgen receptor causes spermatogenic arrest in meiosis. Proc Natl Acad Sci U.S.A. (2004) 101(5):1327–32. doi: 10.1073/pnas.0308114100
164. Holdcraft RW, Braun RE. Androgen receptor function is required in sertoli cells for the terminal differentiation of haploid spermatids. Development (2004) 131(2):459–67. doi: 10.1242/dev.00957
165. O’Hara L, McInnes K, Simitsidellis I, Morgan S, Atanassova N, Slowikowska-Hilczer J, et al. Autocrine androgen action is essential for leydig cell maturation and function, and protects against late-onset leydig cell apoptosis in both mice and men. FASEB J (2015) 29(3):894–910. doi: 10.1096/fj.14-255729
166. Xu Q, Lin HY, Yeh Sd, Yu IC, Wang RS, Chen YT, et al. Infertility with defective spermatogenesis and steroidogenesis in male mice lacking androgen receptor in leydig cells. Endocrine (2007) 32(1):96–106. doi: 10.1007/s12020-007-9015-0
167. Zhang C, Yeh S, Chen YT, Wu CC, Chuang KH, Lin HY, et al. Oligozoospermia with normal fertility in male mice lacking the androgen receptor in testis peritubular myoid cells. Proc Natl Acad Sci U.S.A. (2006) 103(47):17718–23. doi: 10.1073/pnas.0608556103
168. Welsh M, Saunders PTK, Atanassova N, Sharpe RM, Smith LB. Androgen action via testicular peritubular myoid cells is essential for male fertility. FASEB J (2009) 23(12):4218–30. doi: 10.1096/fj.09-138347
169. Tsai MY, Yeh Sd, Wang RS, Yeh S, Zhang C, Lin HY, et al. Differential effects of spermatogenesis and fertility in mice lacking androgen receptor in individual testis cells. Proc Natl Acad Sci U.S.A. (2006) 103(50):18975–80. doi: 10.1073/pnas.0608565103
170. Lyon MF, Glenister PH, Lynn Lamoreux M. Normal spermatozoa from androgen-resistant germ cells of chimaeric mice and the role of androgen in spermatogenesis. Nature (1975) 258(5536):620–2. doi: 10.1038/258620a0
171. O’Shaughnessy PJ, Verhoeven G, de Gendt K, Monteiro A, Abel MH. Direct action through the sertoli cells is essential for androgen stimulation of spermatogenesis. Endocrinology (2010) 151(5):2343–8. doi: 10.1210/en.2009-1333
172. Arslan M, Weinbauer GF, Schlatt S, Shahab M, Nieschlag E. FSH and testosterone, alone or in combination, initiate testicular growth and increase the number of spermatogonia and sertoli cells in a juvenile non-human primate (Macaca mulatta). J Endocrinol (1993) 136(2):235–43. doi: 10.1677/joe.0.1360235
173. Schlatt S, Arslan M, Weinbauer GF, Behre HM, Nieschlag E. Endocrine control of testicular somatic and premeiotic germ cell development in the immature testis of the primate macaca mulatta. Eur J Endocrinol (1995) 133(2):235–47. doi: 10.1530/eje.0.1330235
174. Acosta AA, Khalifa E, Oehninger S. Pure human follicle stimulating hormone has a role in the treatment of severe male infertility by assisted reproduction: Norfolk’s total experience. Hum Reprod (1992) 7(8):1067–72. doi: 10.1093/oxfordjournals.humrep.a137794
175. Burgués S, Calderón MD. Subcutaneous self-administration of highly purified follicle stimulating hormone and human chorionic gonadotrophin for the treatment of male hypogonadotrophic hypogonadism. Spanish collaborative group on Male hypogonadotropic hypogonadism. Hum Reprod (1997) 12(5):980–6.
176. Kliesch S, Behre HM, Nieschlag E. Recombinant human follicle-stimulating hormone and human chorionic gonadotropin for induction of spermatogenesis in a hypogonadotropic male. Fertil Steril (1995) 63(6):1326–8. doi: 10.1016/S0015-0282(16)57619-5
177. Kung AWC, Zhong YY, Lam KSL, Wang C. Induction of spermatogenesis with gonadotrophins in Chinese men with hypogonadotrophic hypogonadism. Int J Androl (1994) 17(5):241–7. doi: 10.1111/j.1365-2605.1994.tb01249.x
178. Bhattacharya I, Basu S, Pradhan BS, Sarkar H, Nagarajan P, Majumdar SS. Testosterone augments FSH signaling by upregulating the expression and activity of FSH-receptor in pubertal primate sertoli cells. Mol Cell Endocrinol (2019) 482:70–80. doi: 10.1016/j.mce.2018.12.012
179. Haywood M, Spaliviero J, Jimemez M, King NJC, Handelsman DJ, Allan CM. Sertoli and germ cell development in hypogonadal (hpg) mice expressing transgenic follicle-stimulating hormone alone or in combination with testosterone. Endocrinology (2003) 144(2):509–17. doi: 10.1210/en.2002-220710
180. Abel MH, Baker PJ, Charlton HM, Monteiro A, Verhoeven G, de Gendt K, et al. Spermatogenesis and sertoli cell activity in mice lacking sertoli cell receptors for follicle-stimulating hormone and androgen. Endocrinology (2008) 149(7):3279–85. doi: 10.1210/en.2008-0086
181. O’Shaughnessy PJ, Monteiro A, Abel M. Testicular development in mice lacking receptors for follicle stimulating hormone and androgen. PloS One (2012) 7(4). doi: 10.1371/journal.pone.0035136
182. Grinspon RP, Bergadá I, Rey RA. Male Hypogonadism and disorders of sex development. Front Endocrinol (Lausanne) (2020) 11.
183. Young J, Xu C, Papadakis GE, Acierno JS, Maione L, Hietamäki J, et al. Clinical management of congenital hypogonadotropic hypogonadism. Endocr Rev (2019) 40(2):669–710.
184. Soffientini U, Rebourcet D, Abel MH, Lee S, Hamilton G, Fowler PA, et al. Identification of sertoli cell-specific transcripts in the mouse testis and the role of FSH and androgen in the control of sertoli cell activity. BMC Genomics (2017) 18(1).
185. Majumdar SS, Bhattacharya I. Genomic and post-genomic leads toward regulation of spermatogenesis. Prog Biophys Mol Biol (2013) 113(3):409–22.
186. Suzuki S, Diaz VD, Hermann BP. What has single-cell RNA-seq taught us about mammalian spermatogenesis? Biol Reprod (2019) 101(3):617–34.
187. Shen Y-C, Shami AN, Moritz L, Larose H, Manske GL, Ma Q, et al. TCF21+ mesenchymal cells contribute to testis somatic cell development, homeostasis, and regeneration in mice. Nat Commun (2021) 12(1).
Keywords: gonadotropins, blood-testis barrier, male fertility, spermatogenesis, infertility
Citation: Bhattacharya I, Dey S and Banerjee A (2023) Revisiting the gonadotropic regulation of mammalian spermatogenesis: evolving lessons during the past decade. Front. Endocrinol. 14:1110572. doi: 10.3389/fendo.2023.1110572
Received: 29 November 2022; Accepted: 23 March 2023;
Published: 14 April 2023.
Edited by:
Ludovic Dumont, Université de Rouen, FranceReviewed by:
Suresh Yenugu, University of Hyderabad, IndiaCopyright © 2023 Bhattacharya, Dey and Banerjee. This is an open-access article distributed under the terms of the Creative Commons Attribution License (CC BY). The use, distribution or reproduction in other forums is permitted, provided the original author(s) and the copyright owner(s) are credited and that the original publication in this journal is cited, in accordance with accepted academic practice. No use, distribution or reproduction is permitted which does not comply with these terms.
*Correspondence: Arnab Banerjee, YXJuYWJiQGdvYS5iaXRzLXBpbGFuaS5hYy5pbg==; Indrashis Bhattacharya, aW5kcmFzaGlzLmJoYXR0YWNoYXJ5YUBnbWFpbC5jb20=
†These authors have contributed equally to this work
Disclaimer: All claims expressed in this article are solely those of the authors and do not necessarily represent those of their affiliated organizations, or those of the publisher, the editors and the reviewers. Any product that may be evaluated in this article or claim that may be made by its manufacturer is not guaranteed or endorsed by the publisher.
Research integrity at Frontiers
Learn more about the work of our research integrity team to safeguard the quality of each article we publish.